Abstract
1. Mitochondrial and supernatant aspartate transaminases (EC 2.6.1.1) and supernatant alanine transaminase (EC 2.6.1.2) were purified 89-, 204- and 240-fold respectively, from dolphin muscle. Starch-gel electrophoresis of crude and purified preparations revealed that all three enzymes exist as single forms. 2. Km values of α-oxoglutarate, alanine, pyruvate and glutamate for the alanine transaminase were 0.45, 8.2, 0.87 and 15mm respectively. For the aspartate transaminases, the Km values of α-oxoglutarate, aspartate, oxalacetate and glutamate were 0.76, 0.50, 0.10 and 9.4mm respectively, for the mitochondrial form and 0.13, 2.4, 0.06 and 3.2mm respectively, for the supernatant form. 3. In all cases, as the assay pH value was decreased from pH7.3, the Km values of the α-oxo acids decreased whereas those of the amino acids increased. 4. The apparent equilibrium constants for the aspartate transaminases were independent of pH. These values were 9.2 and 6.8 for the mitochondrial and supernatant forms respectively, where [Formula: see text] 5. Studies of the inhibition of the aspartate transaminases by dicarboxylic acids indicated that these enzymes may be controlled by pools of metabolic intermediates. 6. Three key roles are suggested for the transaminases in the energy metabolism of the diving animal. First, it is believed that a combined action of the transaminases could enhance energy production during hypoxia by providing (a) fumarate from aspartate for the ATP-producing reversal of succinate dehydrogenase, and (b) α-oxoglutarate from glutamate for the GTP-producing succinyl thiokinase reaction. Secondly, diving mammals probably accumulate more NADH than other mammals during hypoxia. The aspartate transaminases seem particularly well suited for restoring and maintaining redox balance via the malate–aspartate cycle after aerobic metabolism is resumed. Finally, since the preferred fuel for aerobic work is fat, the combined reactions of the transaminases could be instrumental in providing increased supplies of oxaloacetate for sparking the tricarboxylic acid cycle.
Full text
PDF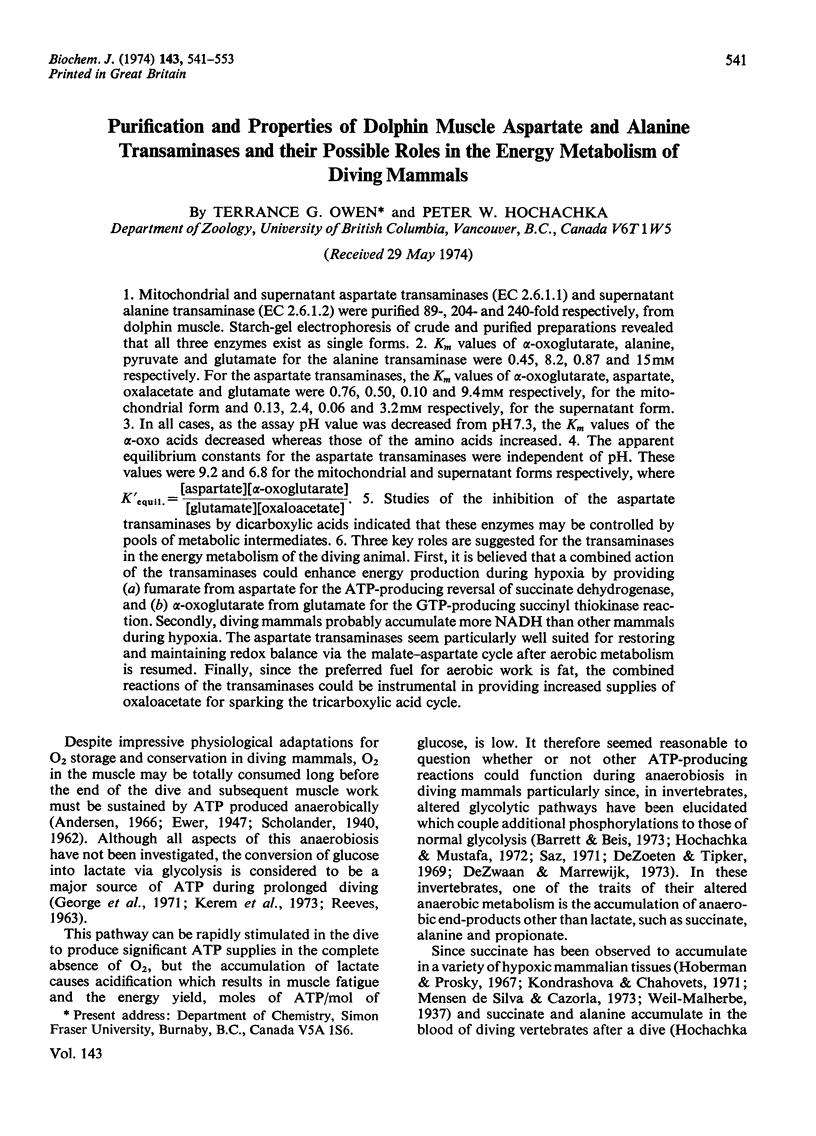
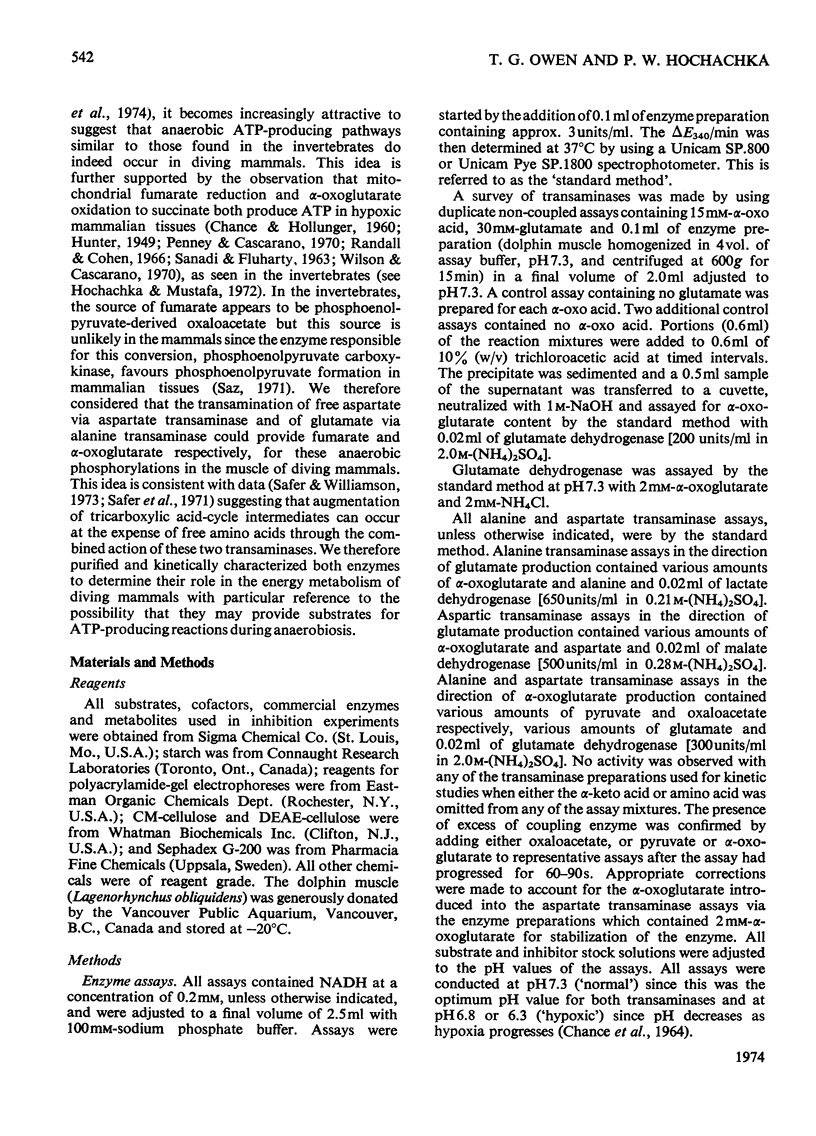
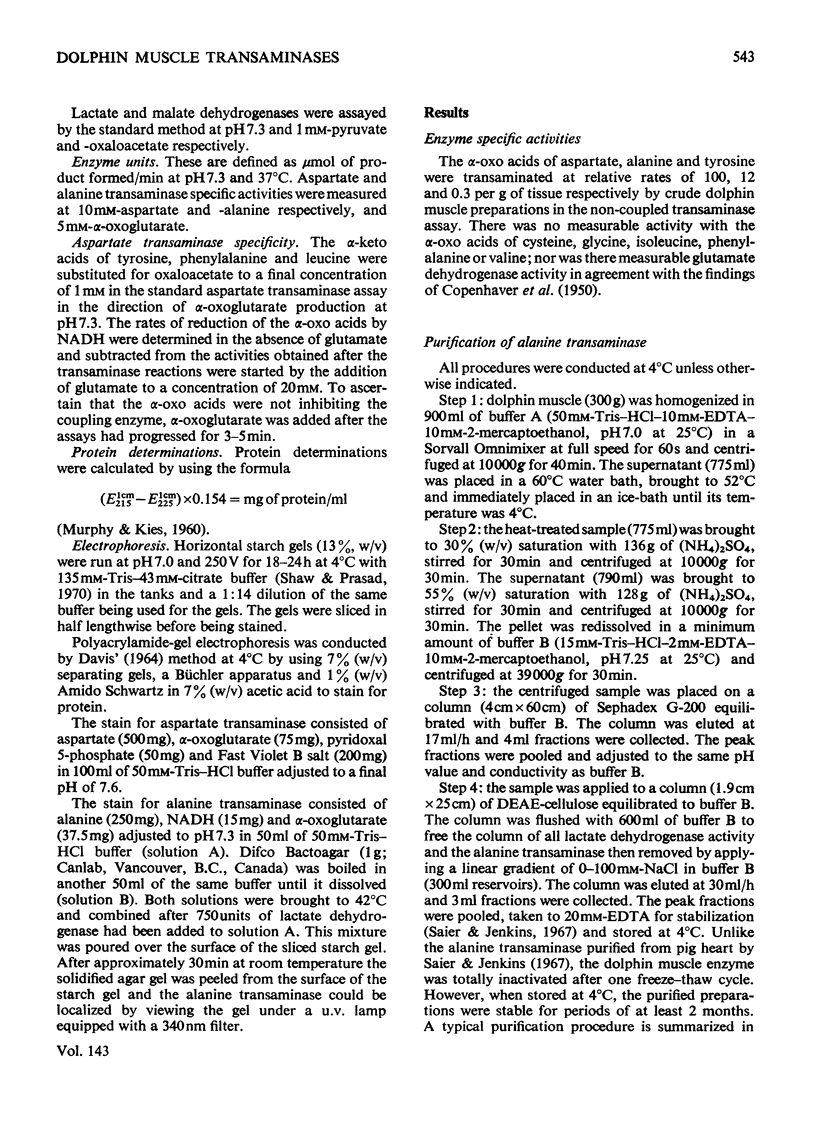
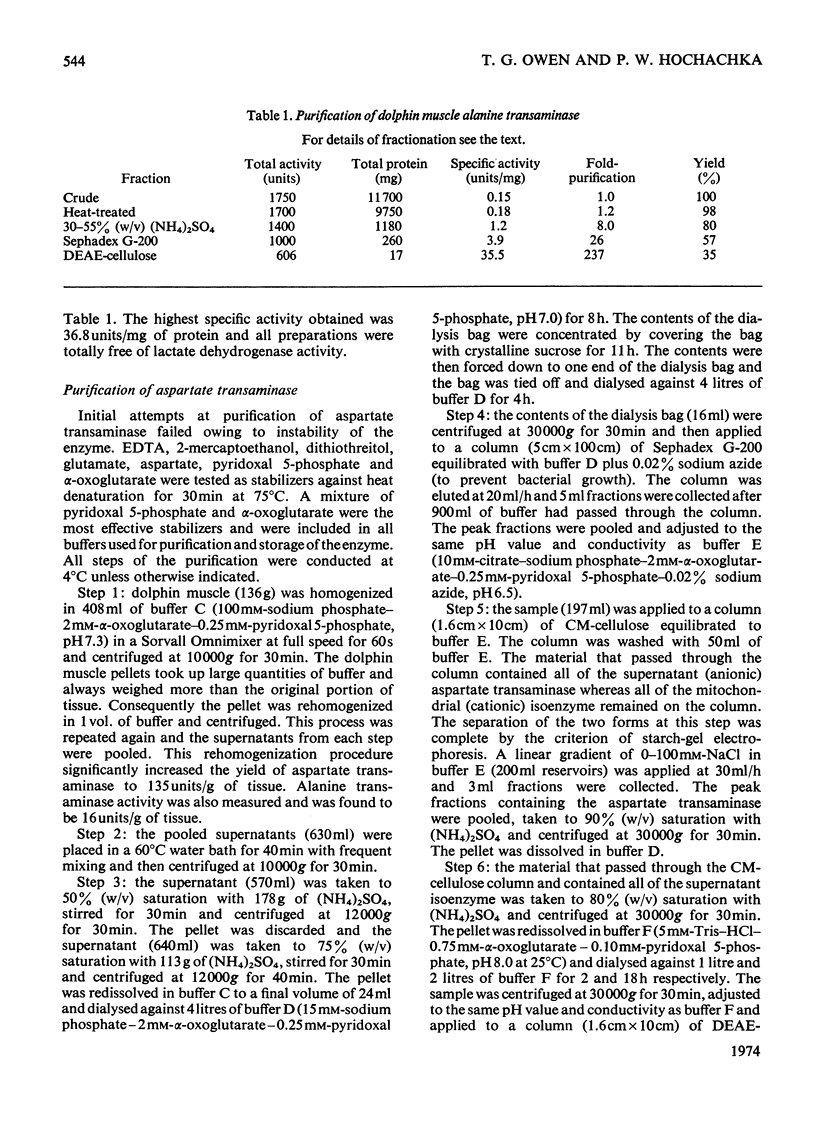
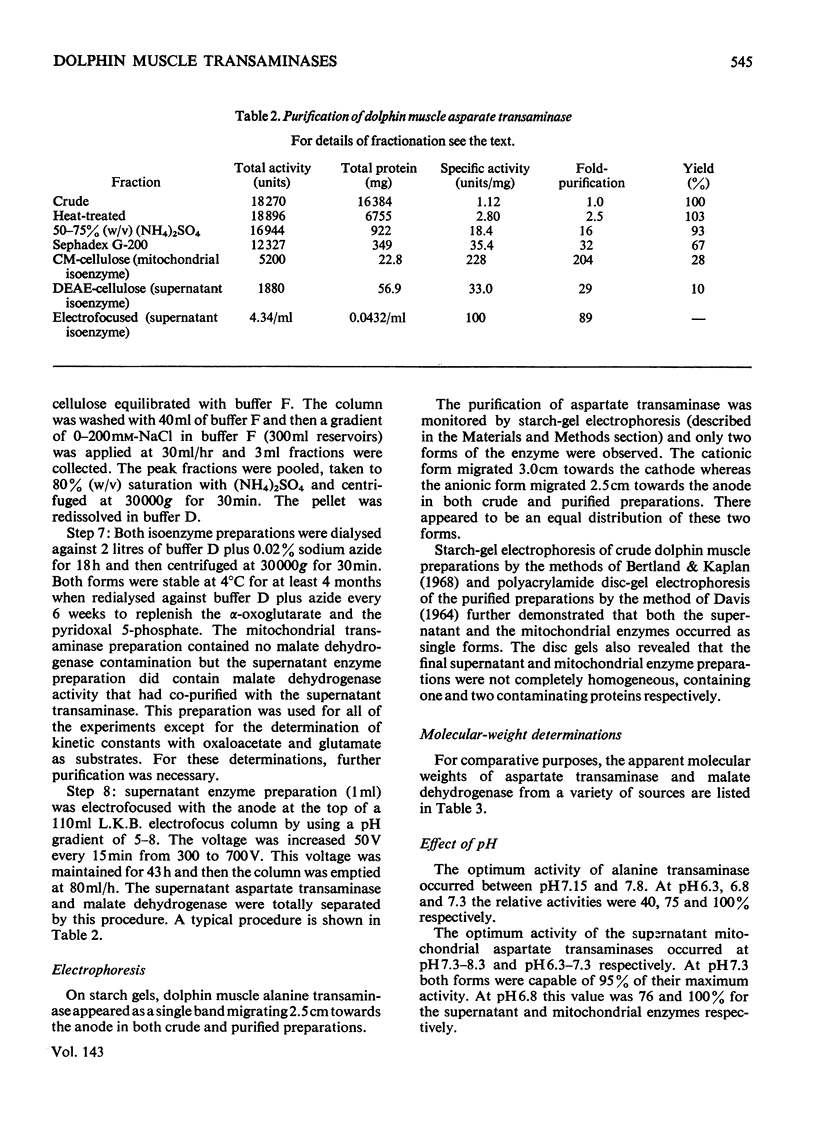
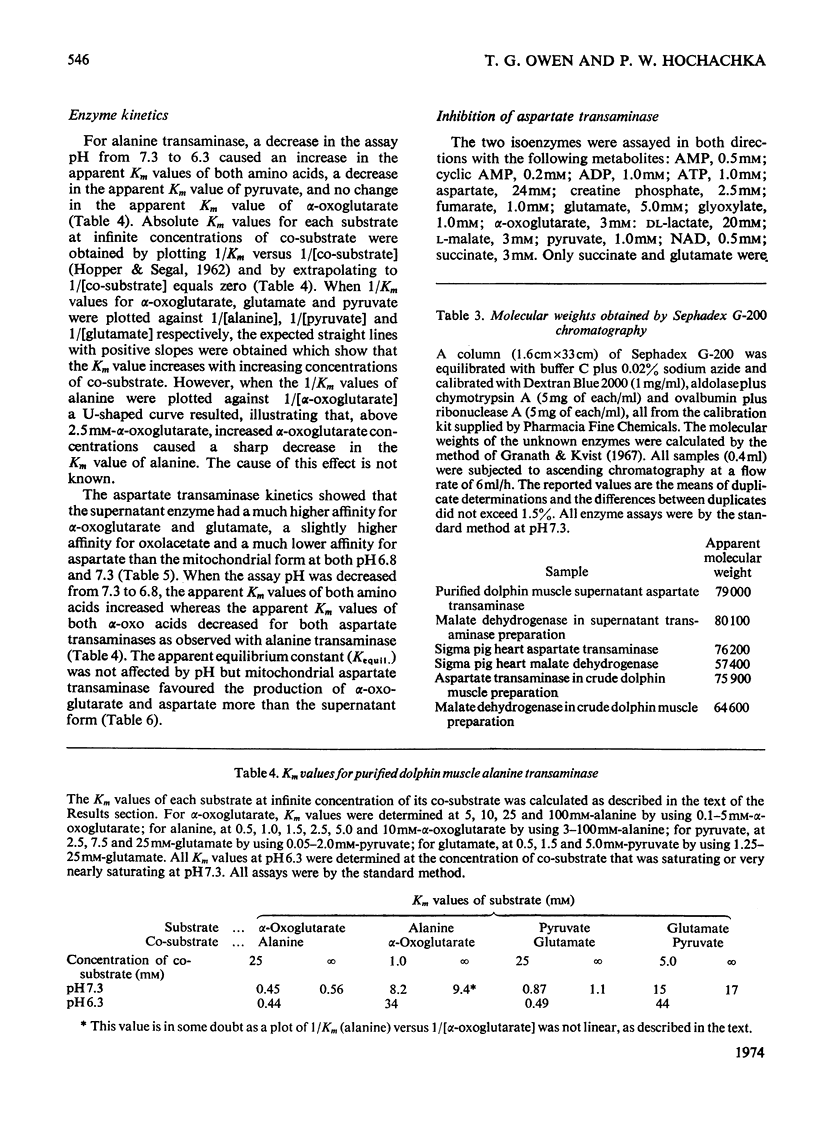
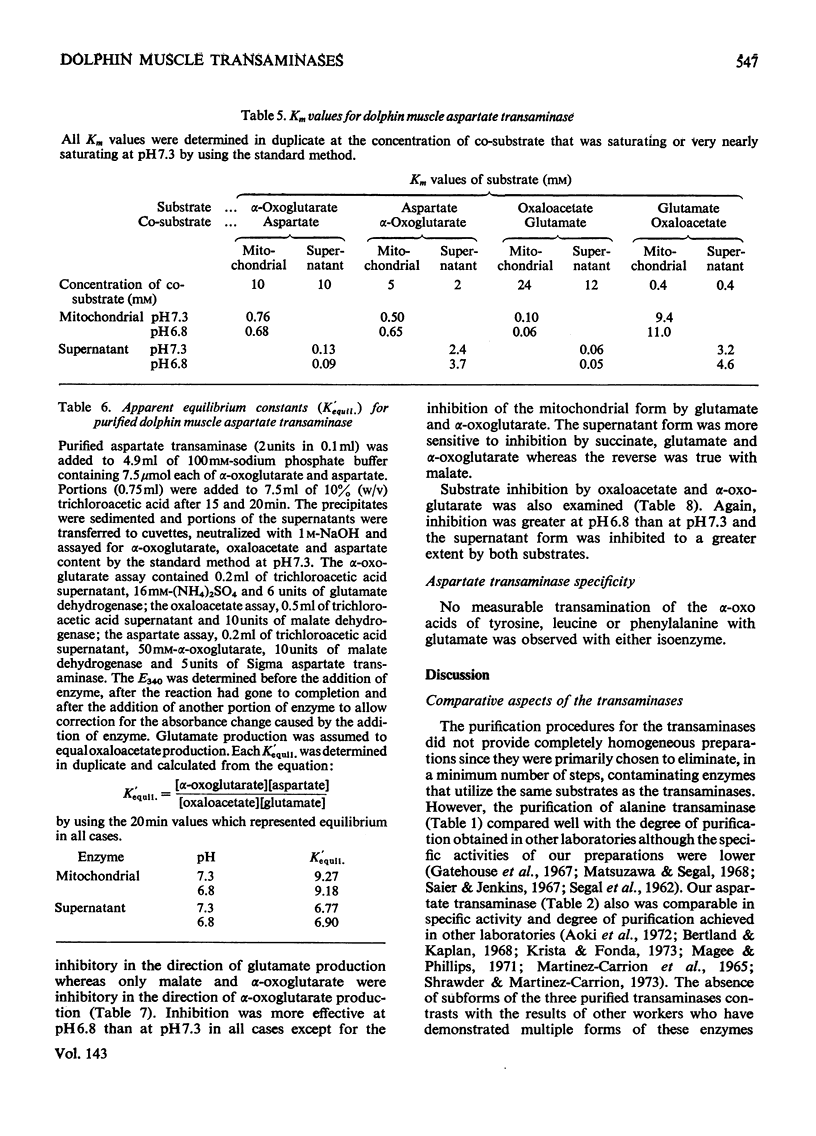
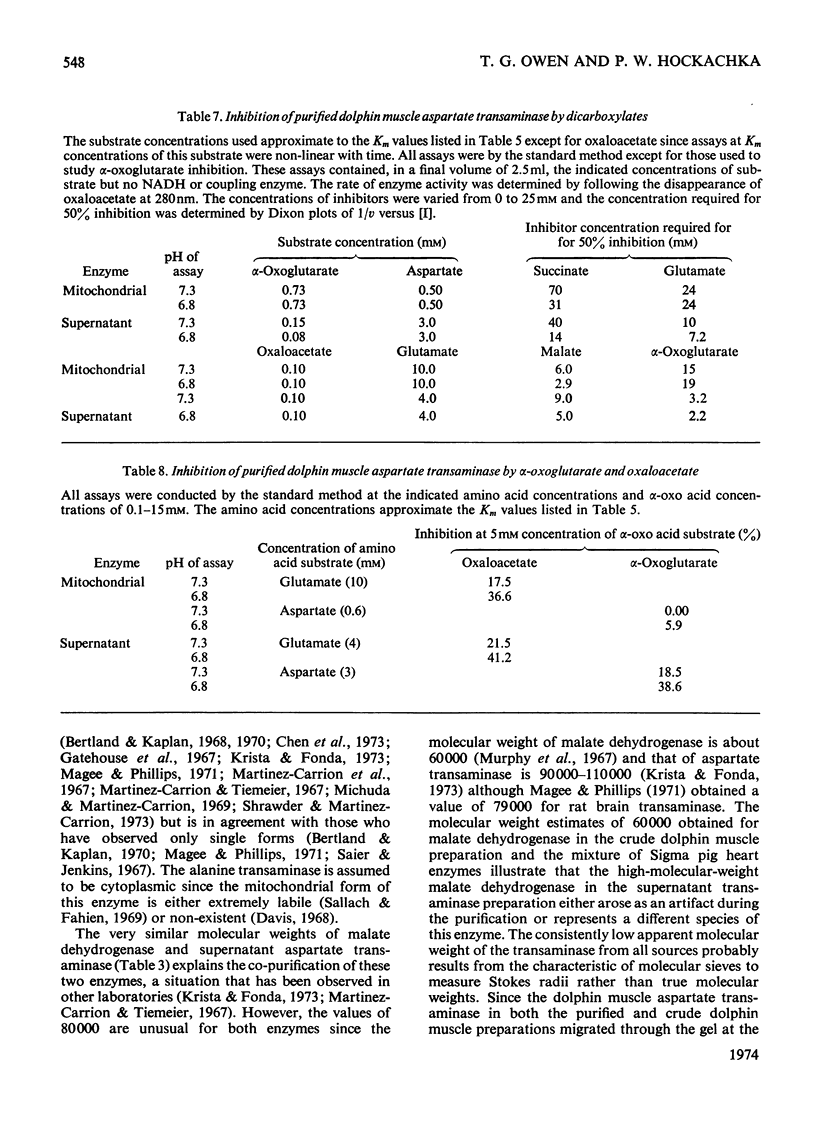
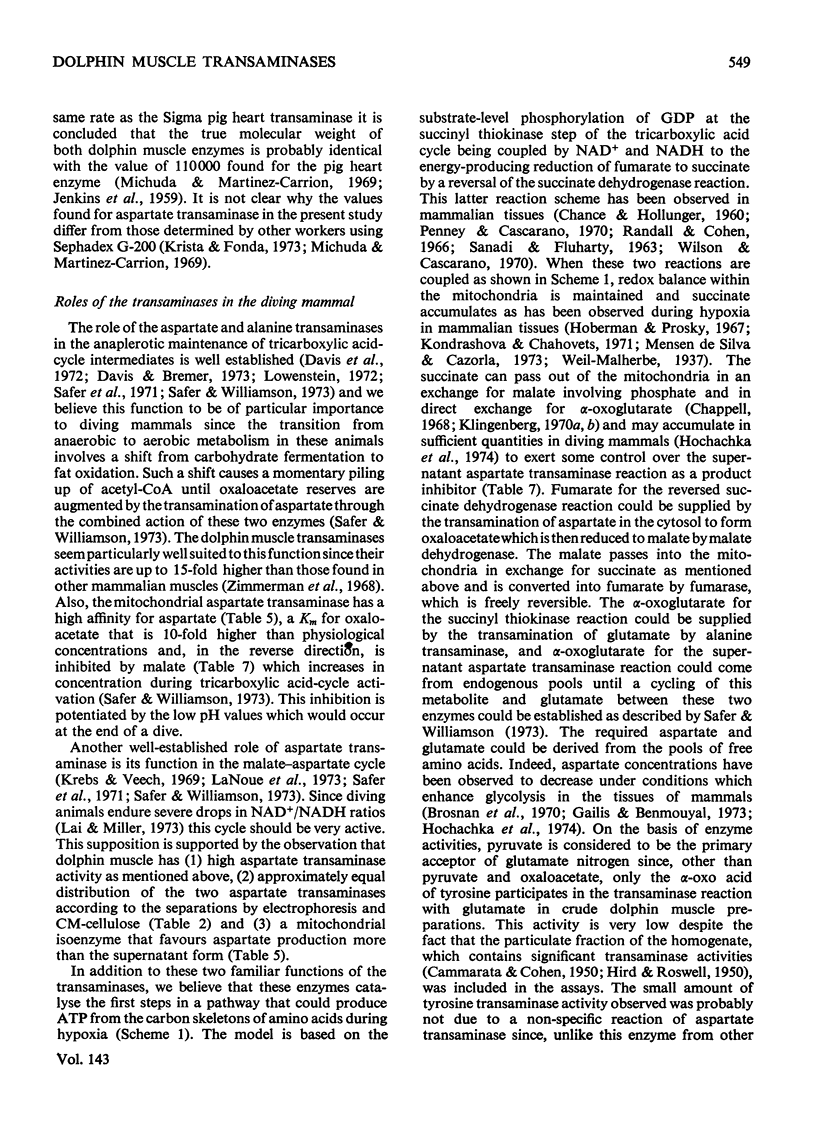
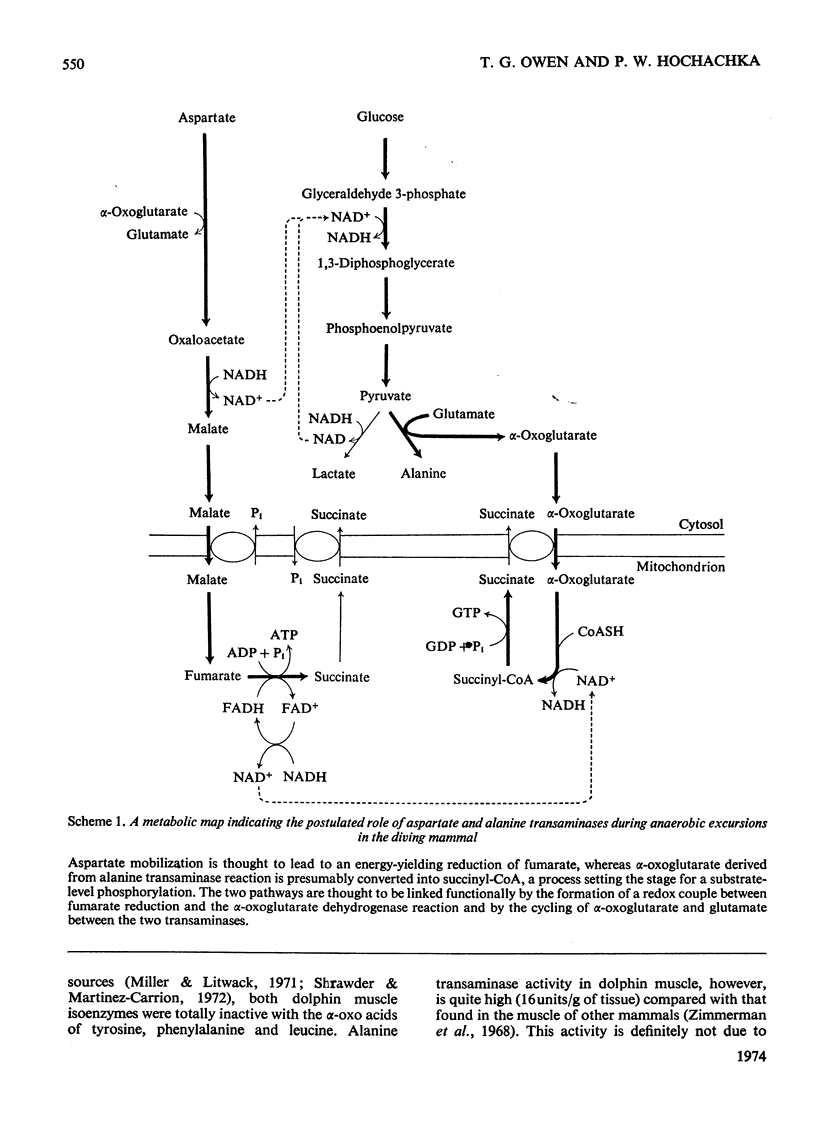
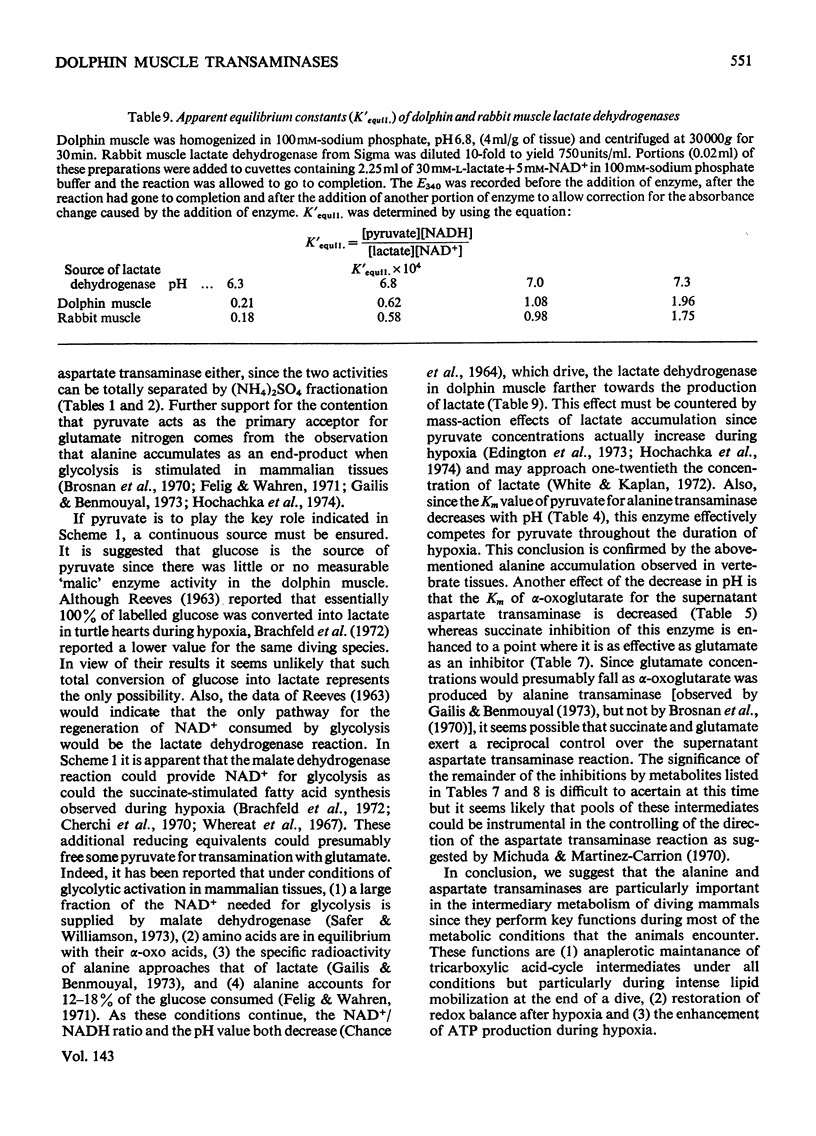
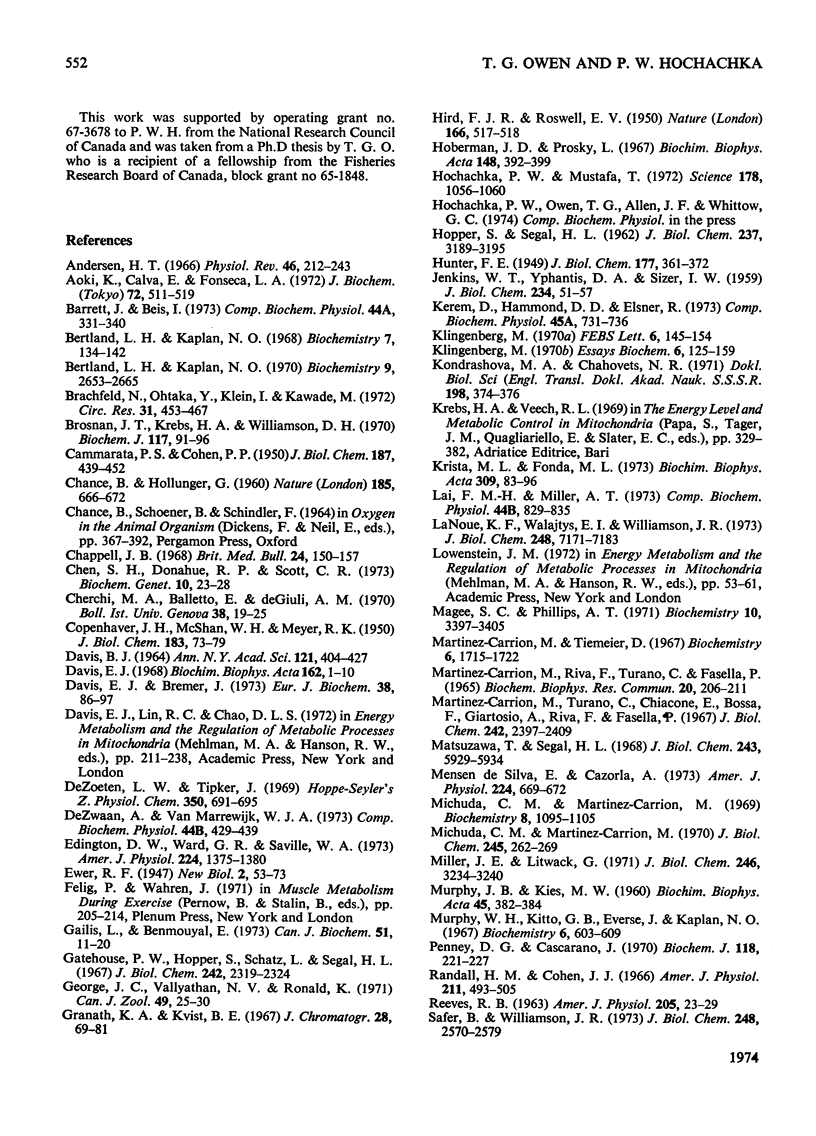

Selected References
These references are in PubMed. This may not be the complete list of references from this article.
- Andersen H. T. Physiological adaptations in diving vertebrates. Physiol Rev. 1966 Apr;46(2):212–243. doi: 10.1152/physrev.1966.46.2.212. [DOI] [PubMed] [Google Scholar]
- Aoki K., Calva E., Del Alba Fonseca L. Crystallization and immunological identification of dog heart aspartate aminotransferase isoenzymes. J Biochem. 1972 Sep;72(3):511–519. doi: 10.1093/oxfordjournals.jbchem.a129930. [DOI] [PubMed] [Google Scholar]
- Barrett J., Beis I. The redox state of the free nicotinamide-adenine dinucleotide couple in the cytoplasm and mitochondria of muscle tissue from Ascaris lumbricoides (Nematoda). Comp Biochem Physiol A Comp Physiol. 1973 Feb 1;44(2):331–340. doi: 10.1016/0300-9629(73)90486-6. [DOI] [PubMed] [Google Scholar]
- Bertland L. H., Kaplan N. O. Chicken heart soluble aspartate aminotransferase. Purification and properties. Biochemistry. 1968 Jan;7(1):134–142. doi: 10.1021/bi00841a018. [DOI] [PubMed] [Google Scholar]
- Bertland L. H., Kaplan N. O. Studies on the conformations of the multiple forms of chicken heart aspartate aminotransferase. Biochemistry. 1970 Jun 23;9(13):2653–2665. doi: 10.1021/bi00815a014. [DOI] [PubMed] [Google Scholar]
- Brachfeld N., Otaka Y., Klein I., Kawade M. Substrate preference and metabolic activity of the aerobic and the hypoxic turtle heart. Circ Res. 1972 Sep;31(3):453–467. doi: 10.1161/01.res.31.3.453. [DOI] [PubMed] [Google Scholar]
- Brosnan J. T., Krebs H. A., Williamson D. H. Effects of ischaemia on metabolite concentrations in rat liver. Biochem J. 1970 Mar;117(1):91–96. doi: 10.1042/bj1170091. [DOI] [PMC free article] [PubMed] [Google Scholar]
- CAMMARATA P. S., COHEN P. P. The scope of the transamination reaction in animal tissues. J Biol Chem. 1950 Nov;187(1):439–452. [PubMed] [Google Scholar]
- CHANCE B., HOLLUNGER G. Energy-linked reduction of mitochondrial pyridine nucleotide. Nature. 1960 Mar 5;185:666–672. doi: 10.1038/185666a0. [DOI] [PubMed] [Google Scholar]
- Chappell J. B. Systems used for the transport of substrates into mitochondria. Br Med Bull. 1968 May;24(2):150–157. doi: 10.1093/oxfordjournals.bmb.a070618. [DOI] [PubMed] [Google Scholar]
- Chen S. H., Donahue R. P., Scott C. R. The genetics of glutamic-pyruvic transaminase in mice: inheritance, electrophoretic phenotypes, and postnatal changes. Biochem Genet. 1973 Sep;10(1):23–28. doi: 10.1007/BF00485745. [DOI] [PubMed] [Google Scholar]
- DAVIS B. J. DISC ELECTROPHORESIS. II. METHOD AND APPLICATION TO HUMAN SERUM PROTEINS. Ann N Y Acad Sci. 1964 Dec 28;121:404–427. doi: 10.1111/j.1749-6632.1964.tb14213.x. [DOI] [PubMed] [Google Scholar]
- Davis E. J., Bremer J. Studies with isolated surviving rat hearts. Interdependence of free amino acids and citric-acid-cycle intermediates. Eur J Biochem. 1973 Sep 21;38(1):86–97. doi: 10.1111/j.1432-1033.1973.tb03037.x. [DOI] [PubMed] [Google Scholar]
- Davis E. J. On the nature of malonate-insensitive oxidation of pyruvate and glutamate by heart sarcosomes. Biochim Biophys Acta. 1968 Jul 16;162(1):1–10. doi: 10.1016/0005-2728(68)90208-9. [DOI] [PubMed] [Google Scholar]
- De Zoeten L. W., Tipker J. Intermediary metabolism of the liver fluke Fasciola hepatica, II. Hydrogen transport and phosphorylation. Hoppe Seylers Z Physiol Chem. 1969 Jun;350(6):691–695. doi: 10.1515/bchm2.1969.350.1.691. [DOI] [PubMed] [Google Scholar]
- Edington D. W., Ward G. R., Saville W. A. Energy metabolism of working muscle: concentration profiles of selected metabolites. Am J Physiol. 1973 Jun;224(6):1375–1380. doi: 10.1152/ajplegacy.1973.224.6.1375. [DOI] [PubMed] [Google Scholar]
- Gailis L., Benmouyal E. Endogenous alanine, glutamate, aspartate, and glutamine in the perfused guinea-pig heart: effect of substrates and cardioactive agents. Can J Biochem. 1973 Jan;51(1):11–20. doi: 10.1139/o73-003. [DOI] [PubMed] [Google Scholar]
- Gatehouse P. W., Hopper S., Schatz L., Segal H. L. Further characterization of alanine aminotransferase of rat liver. J Biol Chem. 1967 May 25;242(10):2319–2324. [PubMed] [Google Scholar]
- George J. C., Vallyathan N. V., Ronald K. The harp seal, Pagophilus groenlandicus (Erxleben, 1777). VII. A histophysiological study of certain skeletal muscles. Can J Zool. 1971 Jan;49(1):25–30. doi: 10.1139/z71-006. [DOI] [PubMed] [Google Scholar]
- Granath K. A., Kvist B. E. Molecular weight distribution analysis by gel chromatography on Sephadex. J Chromatogr. 1967 May;28(1):69–81. doi: 10.1016/s0021-9673(01)85930-6. [DOI] [PubMed] [Google Scholar]
- HIRD F. J. R., ROWSELL E. V. Additional transaminations by insoluble particle preparations of rat liver. Nature. 1950 Sep 23;166(4221):517–518. doi: 10.1038/166517a0. [DOI] [PubMed] [Google Scholar]
- HOPPER S., SEGAL H. L. Kinetic studies of rat liver glutamicalanine transaminase. J Biol Chem. 1962 Oct;237:3189–3195. [PubMed] [Google Scholar]
- Hoberman H. D., Prosky L. Evidence of reduction of fumarate to succinate in perfused rat liver under conditions of reduced O2 tension. Biochim Biophys Acta. 1967 Nov 28;148(2):392–399. doi: 10.1016/0304-4165(67)90135-3. [DOI] [PubMed] [Google Scholar]
- Hochachka P. W., Mustafa T. Invertebrate facultative anaerobiosis. Science. 1972 Dec 8;178(4065):1056–1060. doi: 10.1126/science.178.4065.1056. [DOI] [PubMed] [Google Scholar]
- JENKINS W. T., YPHANTIS D. A., SIZER I. W. Glutamic aspartic transaminase. I. Assay, purification, and general properties. J Biol Chem. 1959 Jan;234(1):51–57. [PubMed] [Google Scholar]
- Kerem D., Hammond D. D., Elsner R. Tissue glycogen levels in the Weddell seal, Leptonychotes weddelli: a possible adaptation to asphyxial hypoxia. Comp Biochem Physiol A Comp Physiol. 1973 Jul 1;45(3):731–736. doi: 10.1016/0300-9629(73)90076-5. [DOI] [PubMed] [Google Scholar]
- Klingenberg M. Metabolite transport in mitochondria: an example for intracellular membrane function. Essays Biochem. 1970;6:119–159. [PubMed] [Google Scholar]
- Klingenberg M. Mitochondria metabolite transport. FEBS Lett. 1970 Feb 16;6(3):145–154. doi: 10.1016/0014-5793(70)80044-8. [DOI] [PubMed] [Google Scholar]
- Krista M. L., Fonda M. L. Beef brain cytoplasmic aspartate aminotransferase. Purification, kinetics, and physical properties. Biochim Biophys Acta. 1973 May 5;309(1):83–96. doi: 10.1016/0005-2744(73)90320-3. [DOI] [PubMed] [Google Scholar]
- LaNoue K. F., Walajtys E. I., Williamson J. R. Regulation of glutamate metabolism and interactions with the citric acid cycle in rat heart mitochondria. J Biol Chem. 1973 Oct 25;248(20):7171–7183. [PubMed] [Google Scholar]
- Lai F. M., Miller A. T., Jr Cytoplasmic and mitochondrial NAD + -NADH 2 ratios in rat brain and liver: effect of hypoxia. Comp Biochem Physiol B. 1973 Mar 15;44(3):829–835. doi: 10.1016/0305-0491(73)90232-0. [DOI] [PubMed] [Google Scholar]
- Magee S. C., Phillips A. T. Molecular properties of the multiple aspartate aminotransferases purified from rat brain. Biochemistry. 1971 Aug 31;10(18):3397–3405. doi: 10.1021/bi00794a013. [DOI] [PubMed] [Google Scholar]
- Martinez-Carrion M., Riva F., Turano C., Fasella P. Multiple forms of supernatant glutamate-aspartate transaminase from pig heart. Biochem Biophys Res Commun. 1965 Jul 12;20(2):206–211. doi: 10.1016/0006-291x(65)90347-5. [DOI] [PubMed] [Google Scholar]
- Martinez-Carrion M., Tiemeier D. Mitochondrial glutamate-aspartate transaminase. I. Structural comparison with the supernatant isozyme. Biochemistry. 1967 Jun;6(6):1715–1722. doi: 10.1021/bi00858a021. [DOI] [PubMed] [Google Scholar]
- Martinez-Carrion M., Turano C., Chiancone E., Bossa F., Giartosio A., Riva F., Fasella P. Isolation and characterization of multiple forms of glutamate-asparate aminotransferase from pig heart. J Biol Chem. 1967 May 25;242(10):2397–2409. [PubMed] [Google Scholar]
- Matsuzawa T., Segal H. L. Rat liver alanine aminotrasferase. Crystallization, composition, and role of sulfhydryl groups. J Biol Chem. 1968 Nov 25;243(22):5929–5934. [PubMed] [Google Scholar]
- Mensen de Silva E., Cazorla A. Lactate, -GP, and Krebs cycle in sea-level and high-altitude native guinea pigs. Am J Physiol. 1973 Mar;224(3):669–672. doi: 10.1152/ajplegacy.1973.224.3.669. [DOI] [PubMed] [Google Scholar]
- Michuda C. M., Martinez-Carrion M. Mitochondrial aspartate transaminase. II. Isolation and characterization of the multiple forms. Biochemistry. 1969 Mar;8(3):1095–1105. doi: 10.1021/bi00831a041. [DOI] [PubMed] [Google Scholar]
- Michuda C. M., Martinez-Carrion M. The isozymes of glutamate-aspartate transaminase. Mechanism of inhibition of dicarboxylic acids. J Biol Chem. 1970 Jan 25;245(2):262–269. [PubMed] [Google Scholar]
- Miller J. E., Litwack G. Purification, properties, and identity of liver mitochondrial tyrosine aminotransferase. J Biol Chem. 1971 May 25;246(10):3234–3240. [PubMed] [Google Scholar]
- Murphey W. H., Kitto G. B., Everse J., Kaplan N. Malate dehydrogenases. I. A survey of molecular size measured by gel filtration. Biochemistry. 1967 Feb;6(2):603–610. doi: 10.1021/bi00854a031. [DOI] [PubMed] [Google Scholar]
- Penney D. G., Cascarano J. Anaerobic rat heart. Effects of glucose and tricarboxylic acid-cycle metabolites on metabolism and physiological performance. Biochem J. 1970 Jun;118(2):221–227. doi: 10.1042/bj1180221. [DOI] [PMC free article] [PubMed] [Google Scholar]
- REEVES R. B. Control of glycogen utilization and glucose uptake in the anaerobic turtle heart. Am J Physiol. 1963 Jul;205:23–29. doi: 10.1152/ajplegacy.1963.205.1.23. [DOI] [PubMed] [Google Scholar]
- Randall H. M., Jr, Cohen J. J. Anaerobic CO2 production by dog kidney in vitro. Am J Physiol. 1966 Aug;211(2):493–505. doi: 10.1152/ajplegacy.1966.211.2.493. [DOI] [PubMed] [Google Scholar]
- SANADI D. R., FLUHARTY A. L. ON THE MECHANISM OF OXIDATIVE PHOSPHORYLATION. VII. THE ENERGY-REQUIRING REDUCTION OF PYRIDINE NUCLEOTIDE BY SUCCINATE AND THE ENERGY-YIELDING OXIDATION OF REDUCED PYRIDINE NUCLEOTIDE BY FUMARATE. Biochemistry. 1963 May-Jun;2:523–528. doi: 10.1021/bi00903a023. [DOI] [PubMed] [Google Scholar]
- Safer B., Smith C. M., Williamson J. R. Control of the transport of reducing equivalents across the mitochondrial membrane in perfused rat heart. J Mol Cell Cardiol. 1971 Jun;2(2):111–124. doi: 10.1016/0022-2828(71)90065-4. [DOI] [PubMed] [Google Scholar]
- Safer B., Williamson J. R. Mitochondrial-cytosolic interactions in perfused rat heart. Role of coupled transamination in repletion of citric acid cycle intermediates. J Biol Chem. 1973 Apr 10;248(7):2570–2579. [PubMed] [Google Scholar]
- Saier M. H., Jr, Jenkins W. T. Alanine aminotransferase. I. Purification and properties. J Biol Chem. 1967 Jan 10;242(1):91–100. [PubMed] [Google Scholar]
- Shaw C. R., Prasad R. Starch gel electrophoresis of enzymes--a compilation of recipes. Biochem Genet. 1970 Apr;4(2):297–320. doi: 10.1007/BF00485780. [DOI] [PubMed] [Google Scholar]
- Shrawder E. J., Martinez-Carrion M. Simultaneous isolation and characterization of chicken supernatant and mitochondrial isoenzymes of aspartate transaminase. J Biol Chem. 1973 Mar 25;248(6):2140–2146. [PubMed] [Google Scholar]
- Shrawder E., Martinez-Carrion M. Evidence of phenylalanine transaminase activity in the isoenzymes of aspartate transaminase. J Biol Chem. 1972 Apr 25;247(8):2486–2492. [PubMed] [Google Scholar]
- Weil-Malherbe H. Studies on brain metabolism: Formation of succinic acid. Biochem J. 1937 Feb;31(2):299–312. doi: 10.1042/bj0310299. [DOI] [PMC free article] [PubMed] [Google Scholar]
- Whereat A. F., Hull F. E., Orishimo M. W. The role of succinate in the regulation of fatty acid synthesis by heart mitochondria. J Biol Chem. 1967 Sep 25;242(18):4013–4022. [PubMed] [Google Scholar]
- White H. B., 3rd, Kaplan N. O. Separate physiological roles for two isozymes of pyridine nucleotide-linked glycerol-3-phosphate dehydrogenase in chickens. J Mol Evol. 1971;1(1):158–172. [PubMed] [Google Scholar]
- Wilson M. A., Cascarano J. The energy-yielding oxidation of NADH by fumarate in submitochondrial particles of rat tissues. Biochim Biophys Acta. 1970 Aug 4;216(1):54–62. doi: 10.1016/0005-2728(70)90158-1. [DOI] [PubMed] [Google Scholar]
- Zimmerman H. J., Dujovne C. A., Levy R. The correlation of serum levels of two transaminases with tissue levels in six vertebrate species. Comp Biochem Physiol. 1968 Jun;25(3):1081–1089. doi: 10.1016/0010-406x(68)90593-8. [DOI] [PubMed] [Google Scholar]