Abstract
A simple mathematical model for glycolysis in erythrocytes is presented which takes into account ATP synthesis and consumption. The system is described by four ordinary differential equations. Conditions in vivo are described by a stable steady state. The model predicts correctly the metabolite concentrations found in vivo. The parameters involved are in agreement with data on the separate steps. The metabolite changes found in pyruvate kinase-deficient erythrocytes and the species variations among erythrocytes from different animals are described satisfactorily. The roles of the enzymes in the control of metabolites and glycolytic flux are expressed in the form of a control matrix and control strengths [R. Heinrich & T.A. Rapoport (1974) Eur. J. Biochem. 42, 89-95] respectively. Erythrocytes from various species are shown to be adapted to a maximal ATP-consumption rate. The calculated eigenvalues reveal the pronounced time-hierarchy of the glycolytic reactions. Owing to the slowness of the 2,3-bisphospho-glycerate phosphatase reaction, quasi-steady states occur during the time-interval of about 0.5-2h incubation, which are defined by perturbed 2,3-bisphosphoglycerate concentrations. The theoretical predictions agree with experimental data. In the quasi-steady state the flux control is exerted almost entirely by the hexokinase-phosphofructokinase system. The model describes satisfactorily the time-dependent changes after addition of glucose to starved erythrocytes. The theoretical consequences are discussed of the conditions in vitro with lactate accumulation and the existence of a time-independent conservation quantity for the oxidized metabolites. Even in this closed system quasi-steady states occur which are characterized by approximately constant concentrations of all glycolytic metabolites except for the accumulation of lactate, fructose 1,6-bisphosphate and triose phosphate.
Full text
PDF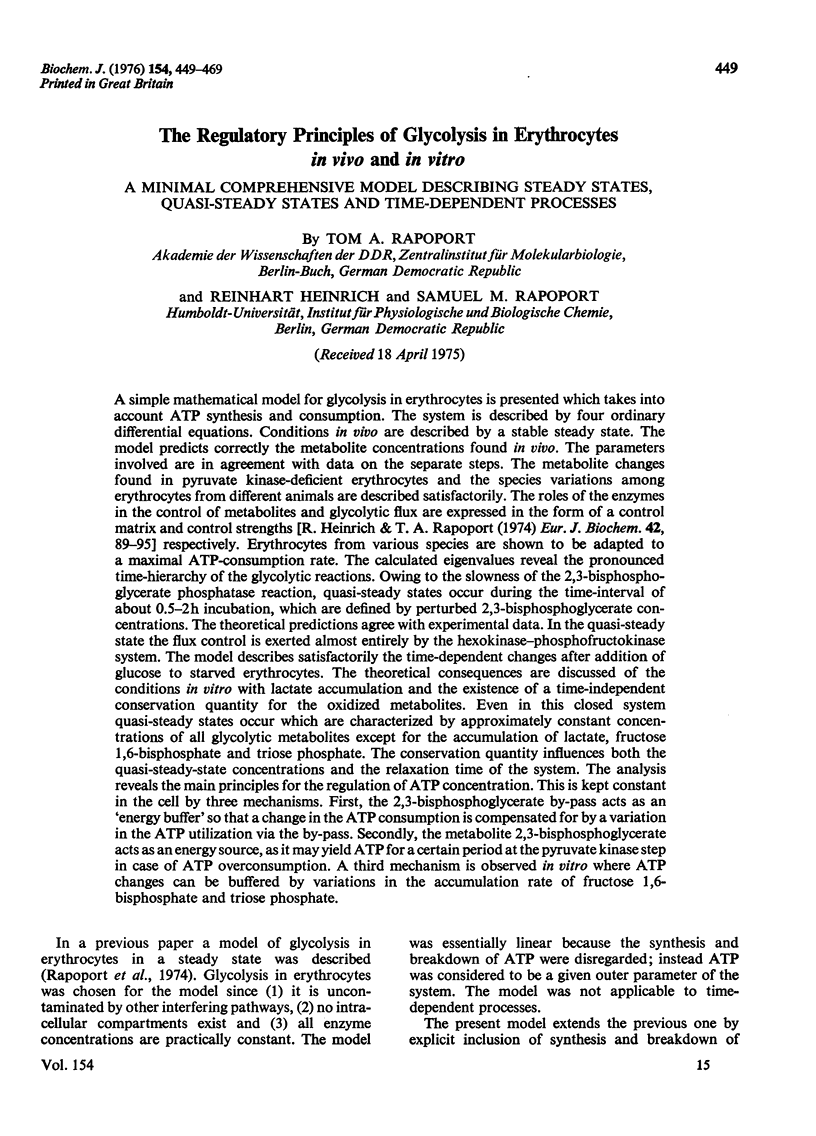
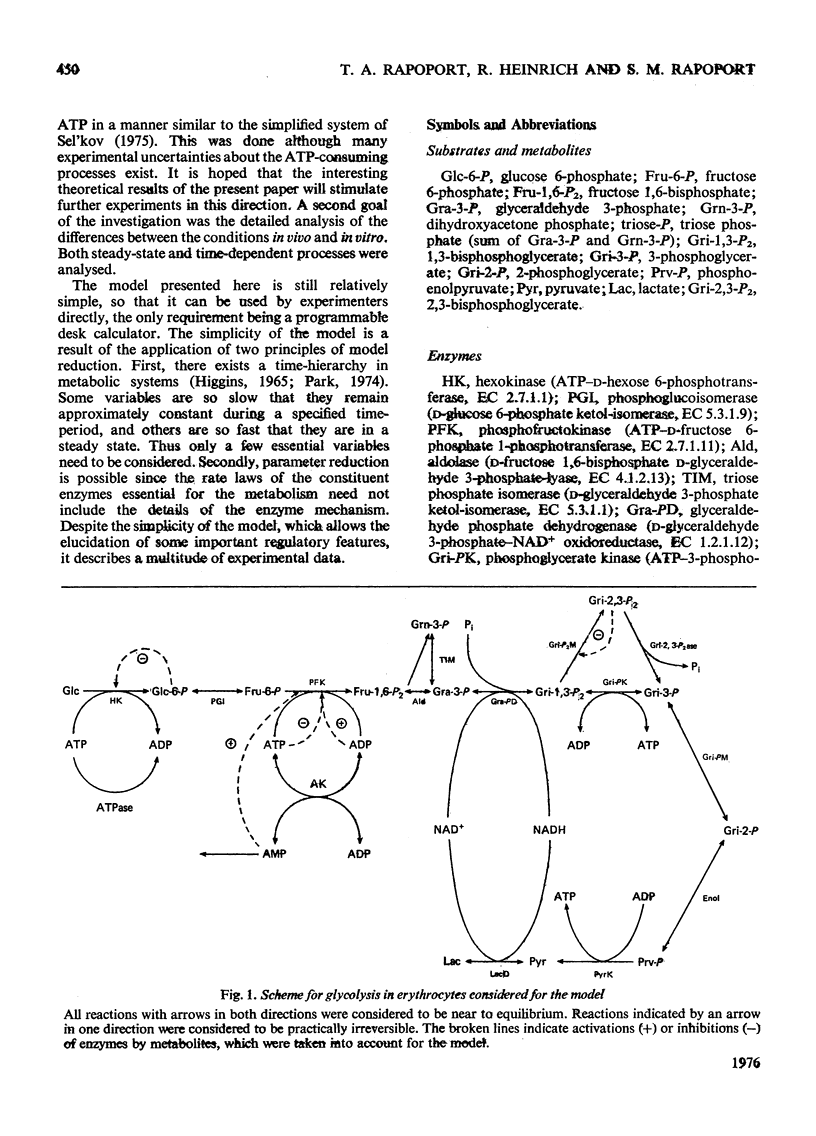
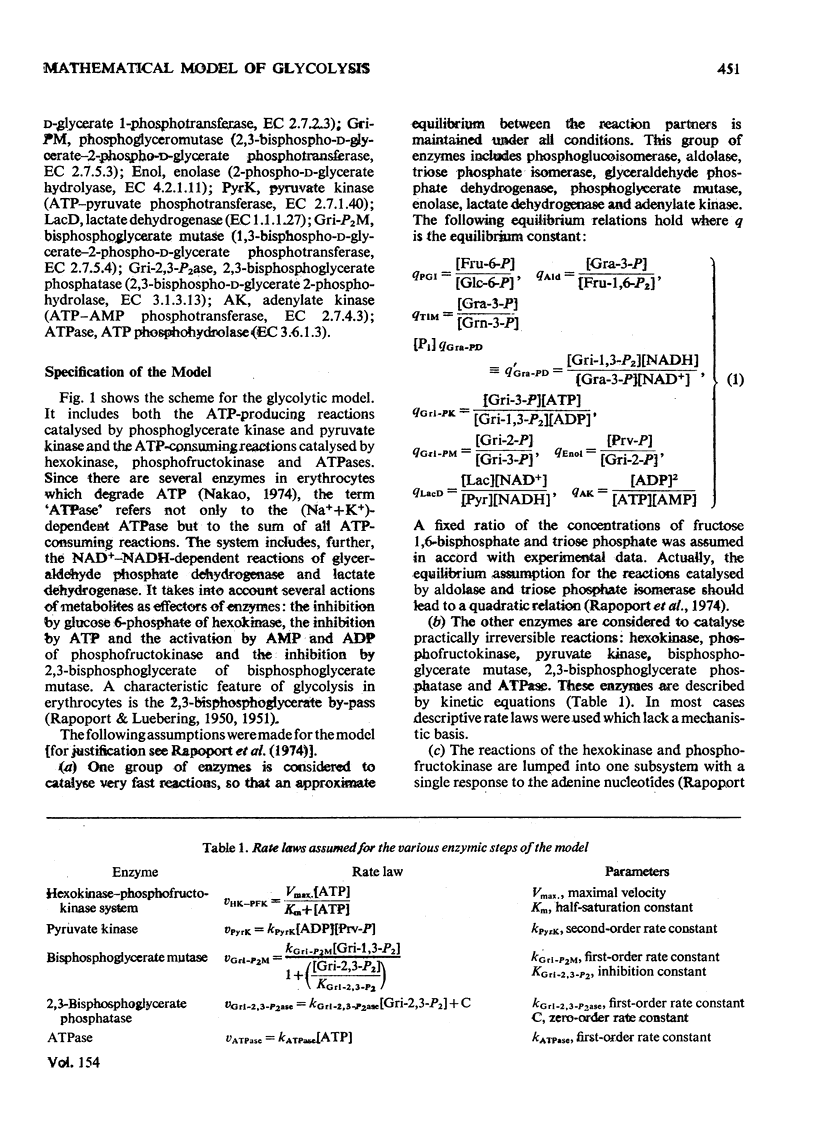
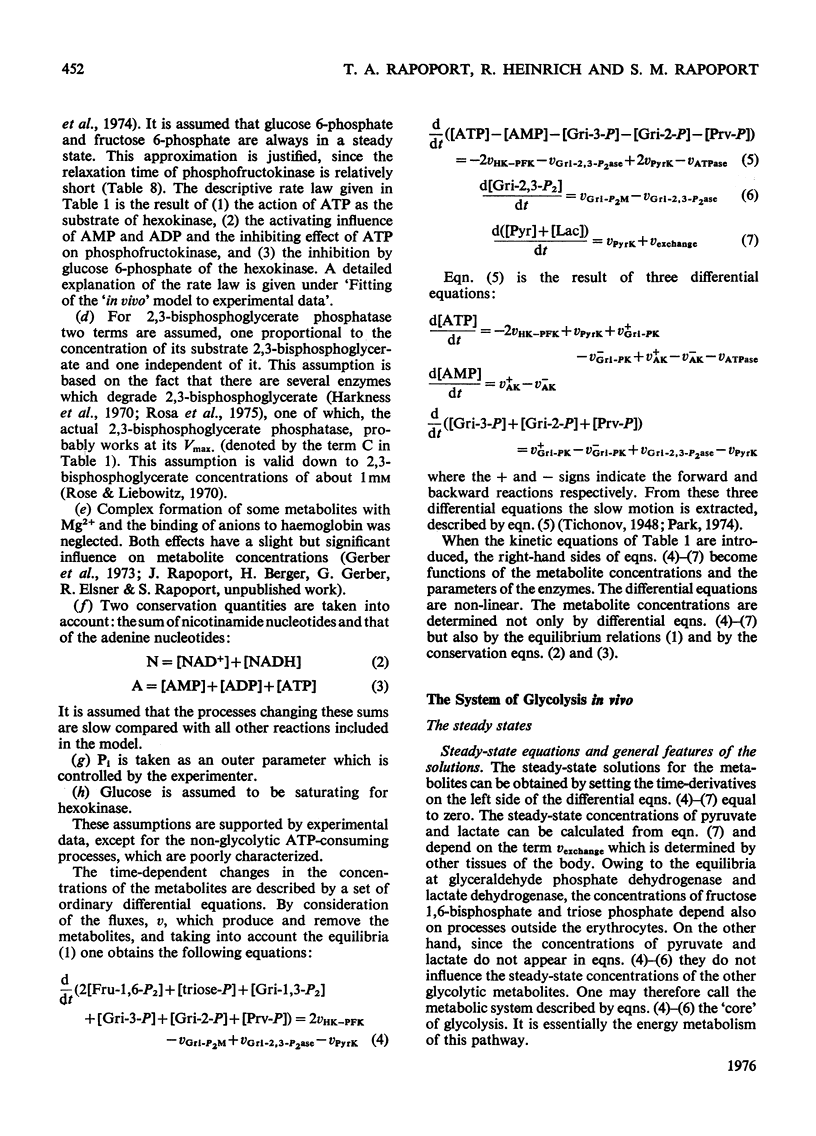
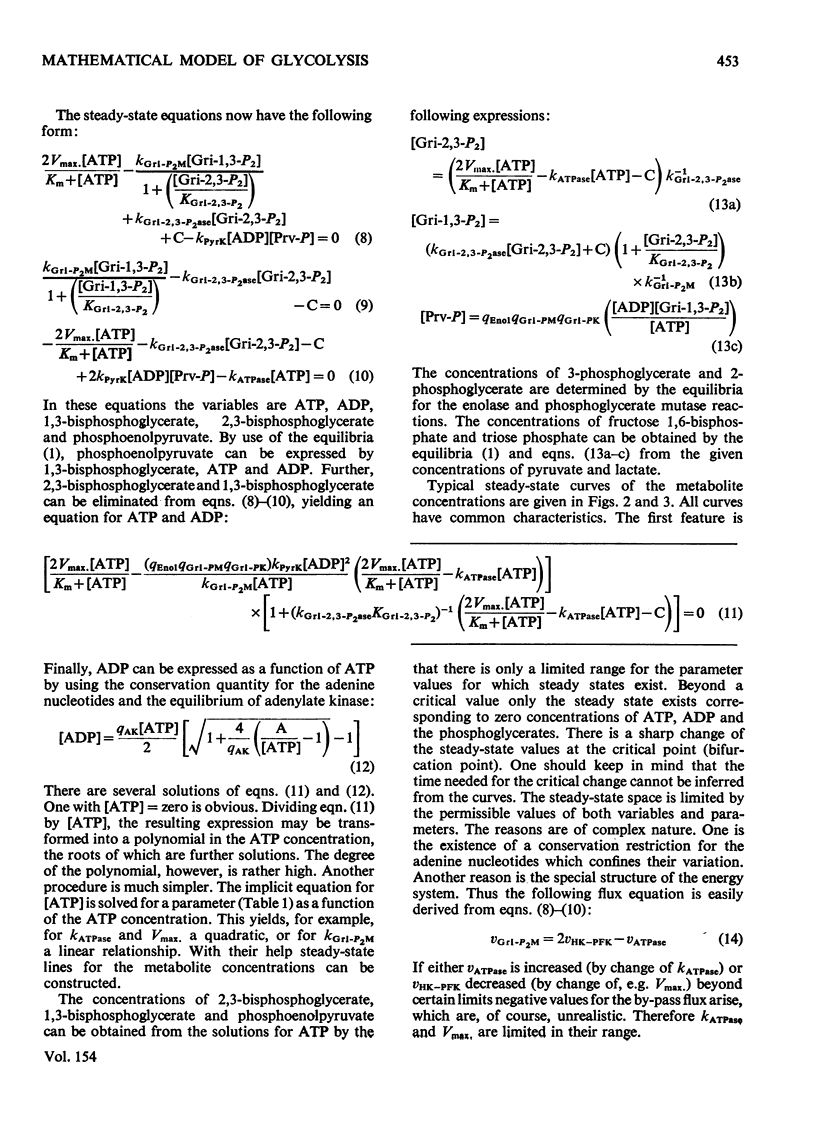
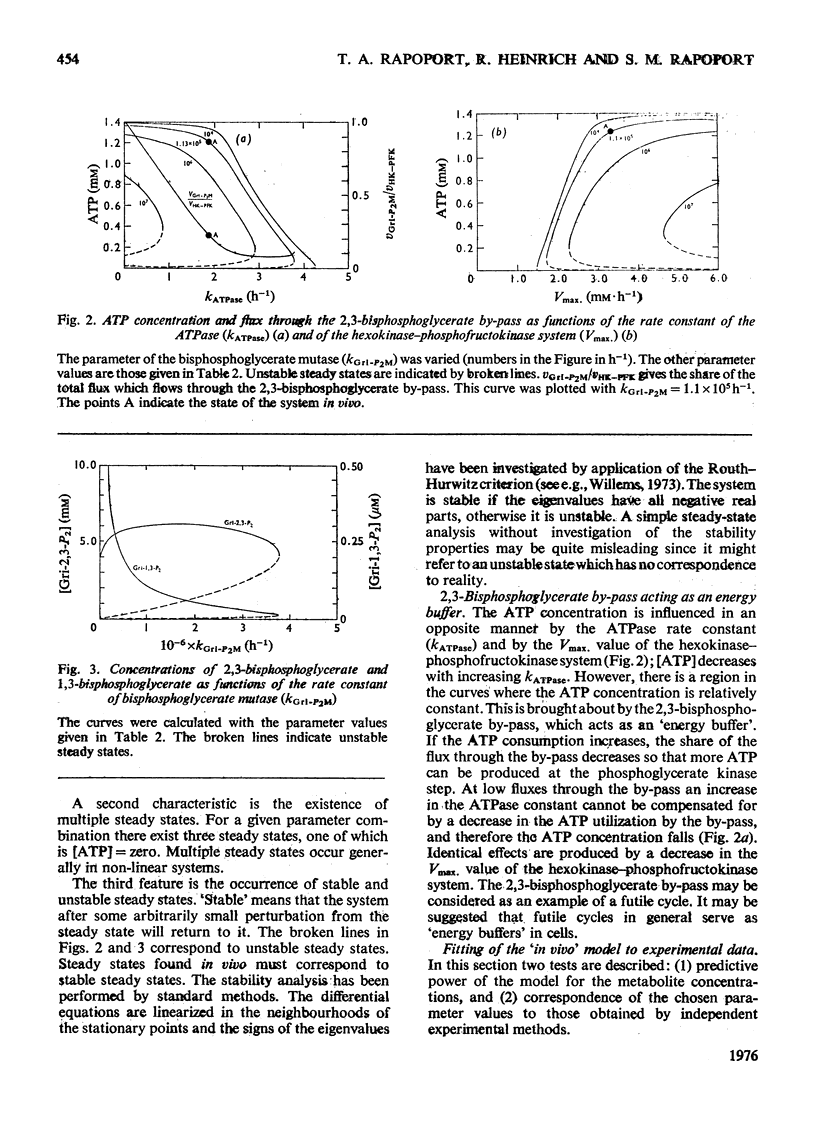
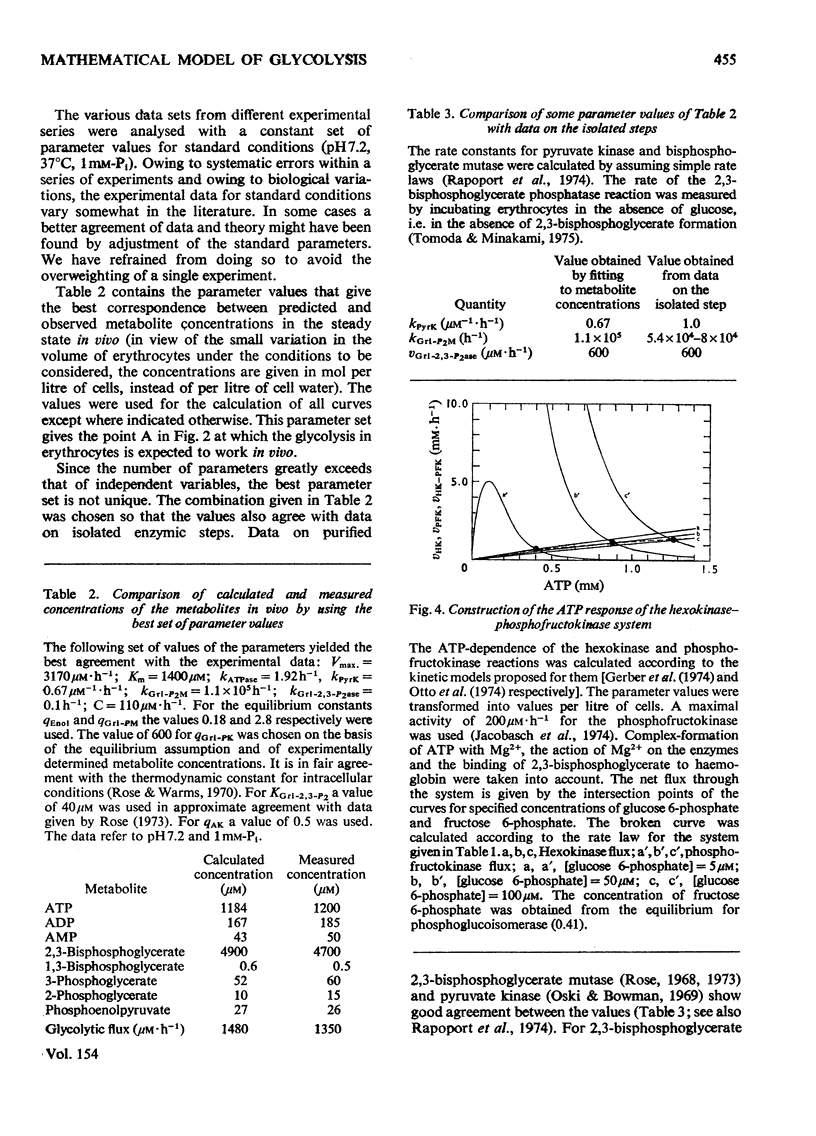
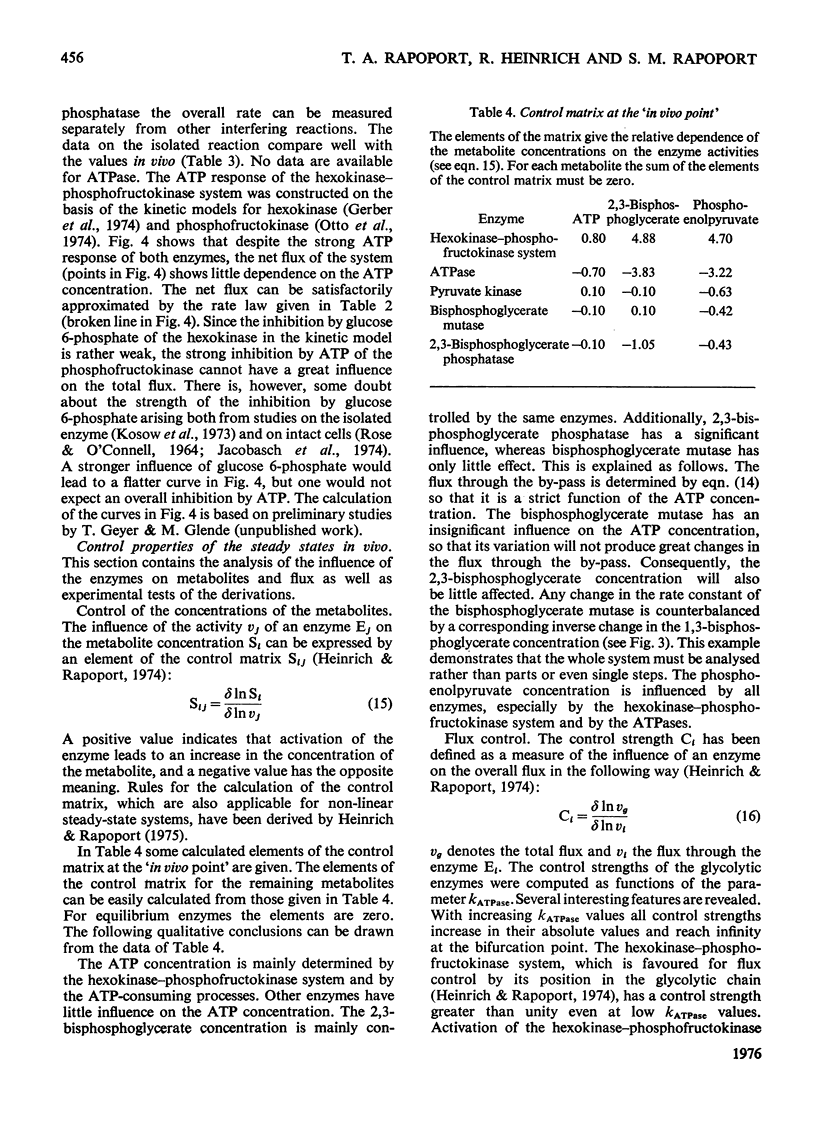
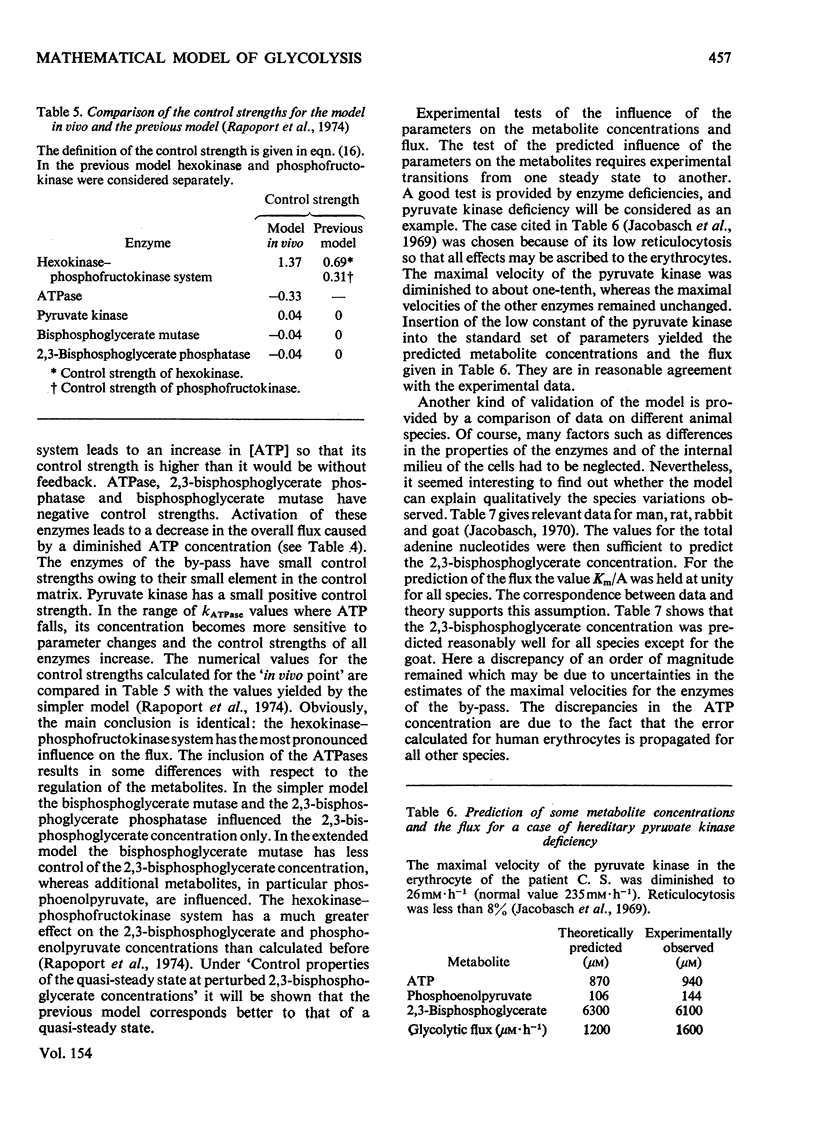
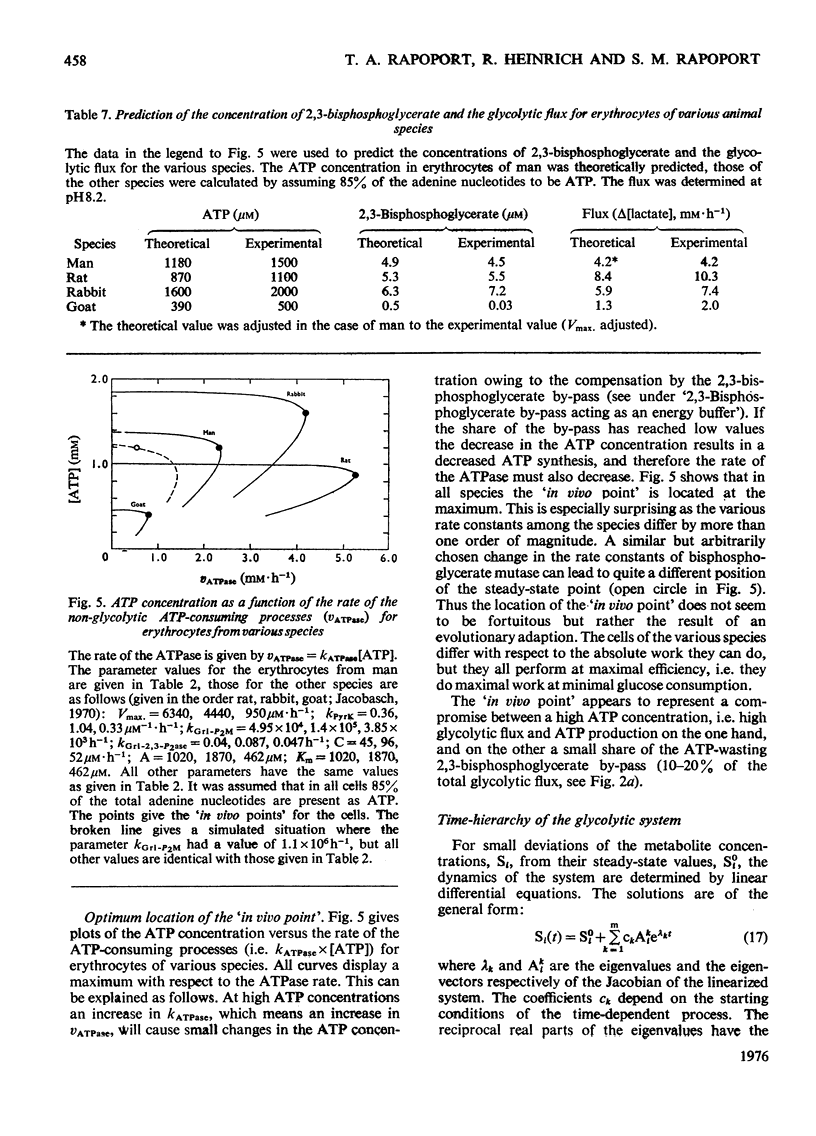
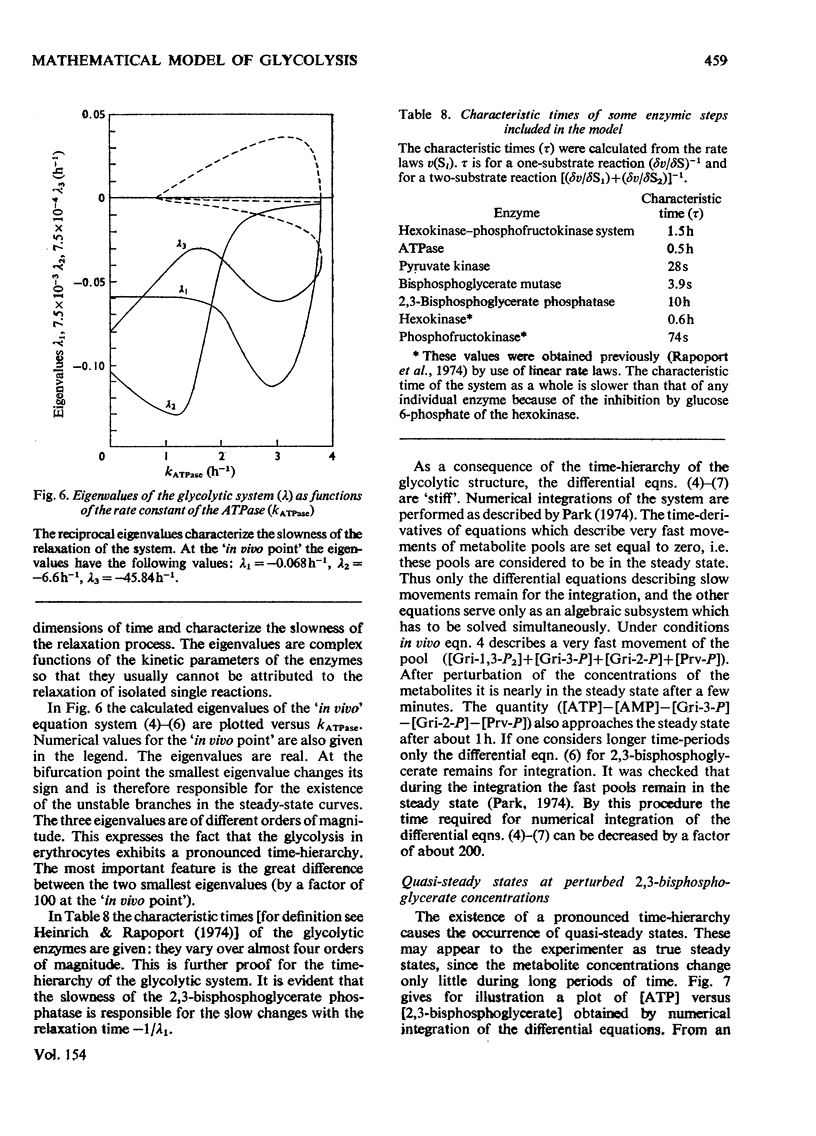
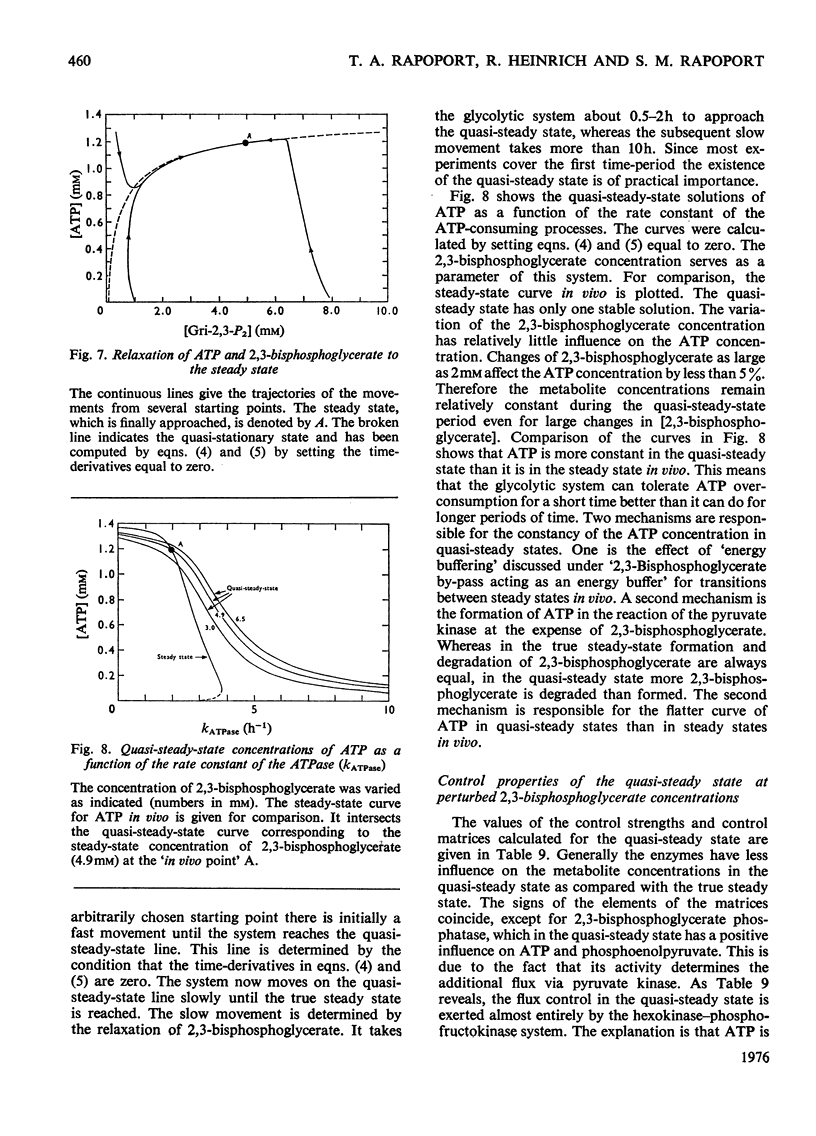
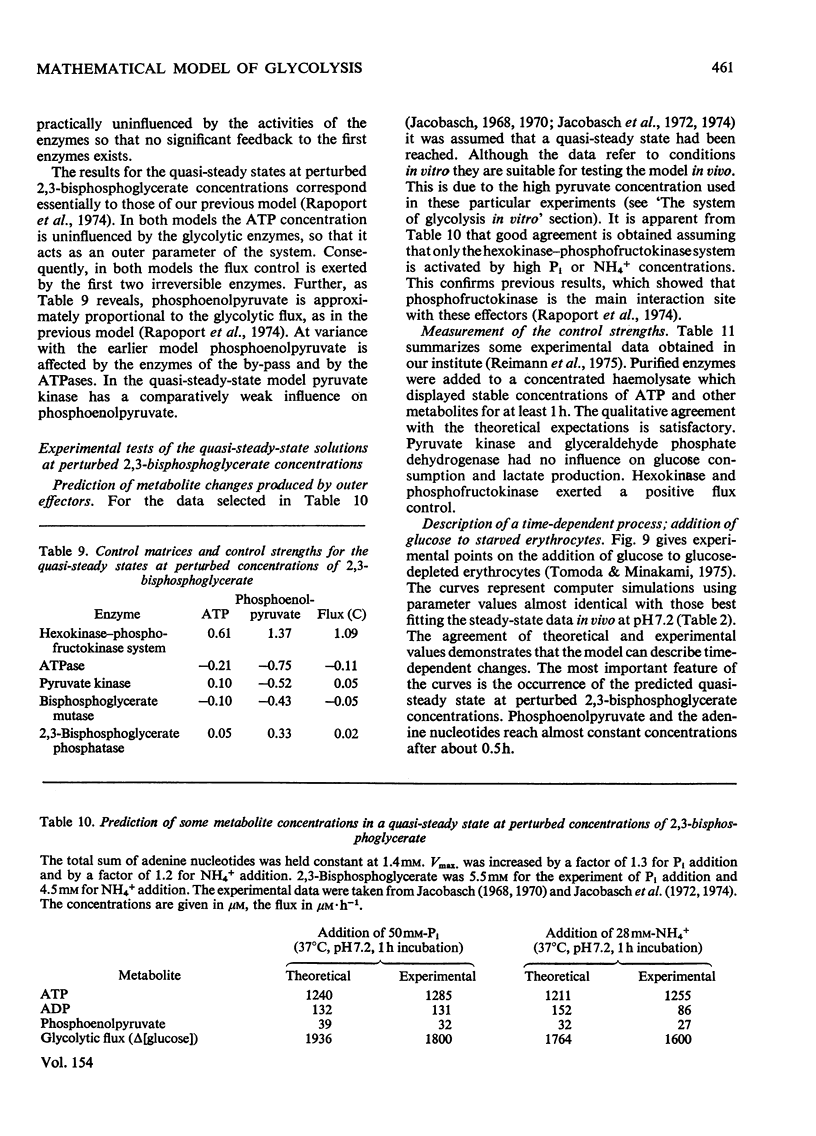
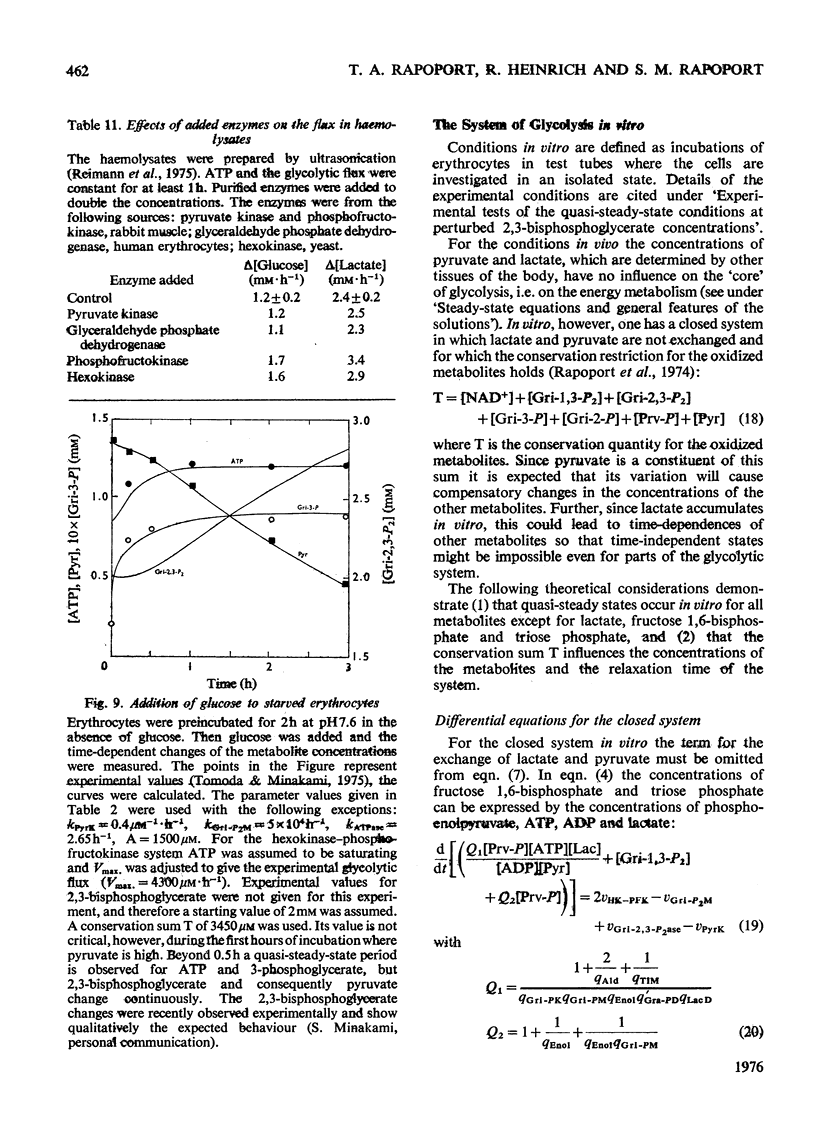
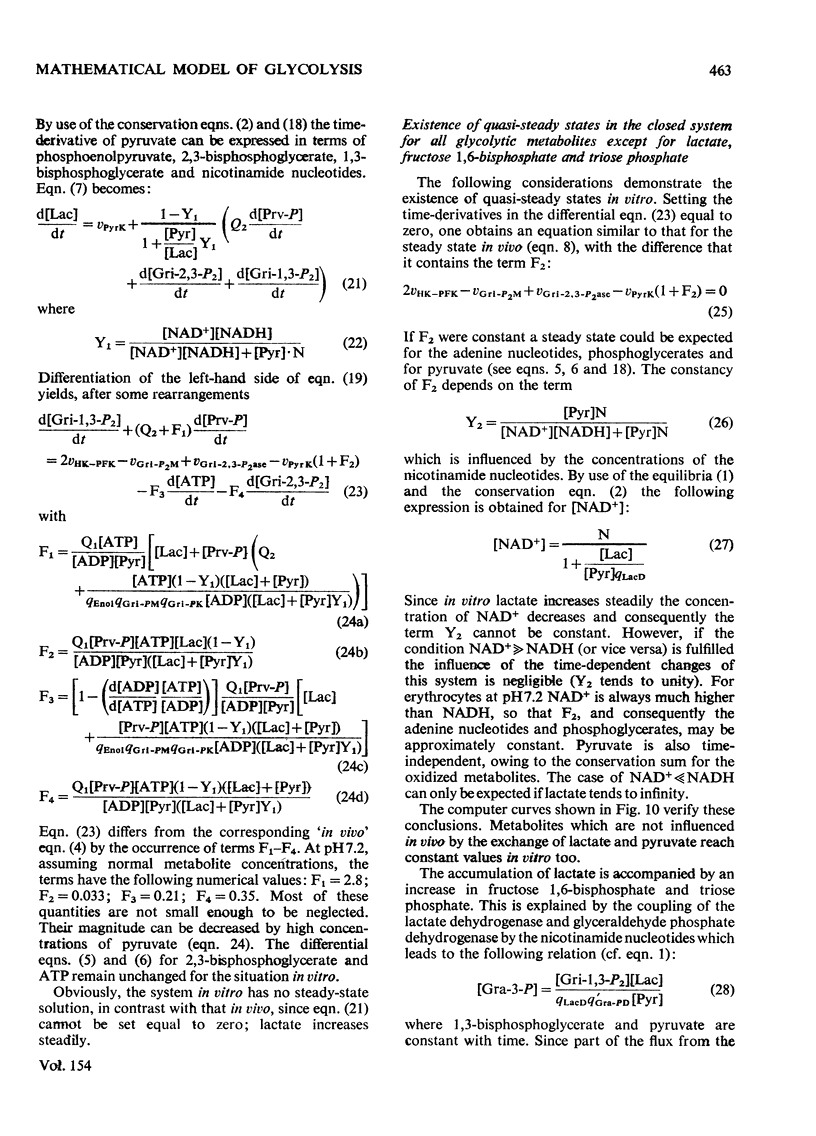
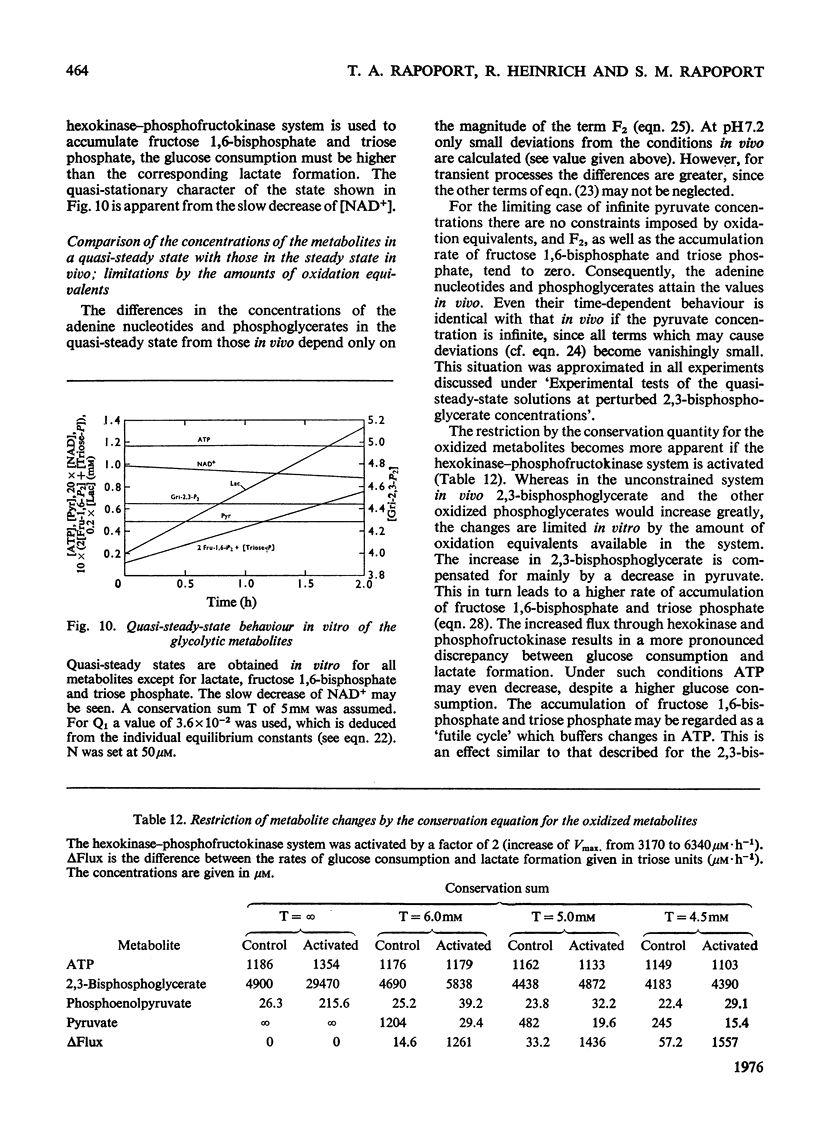
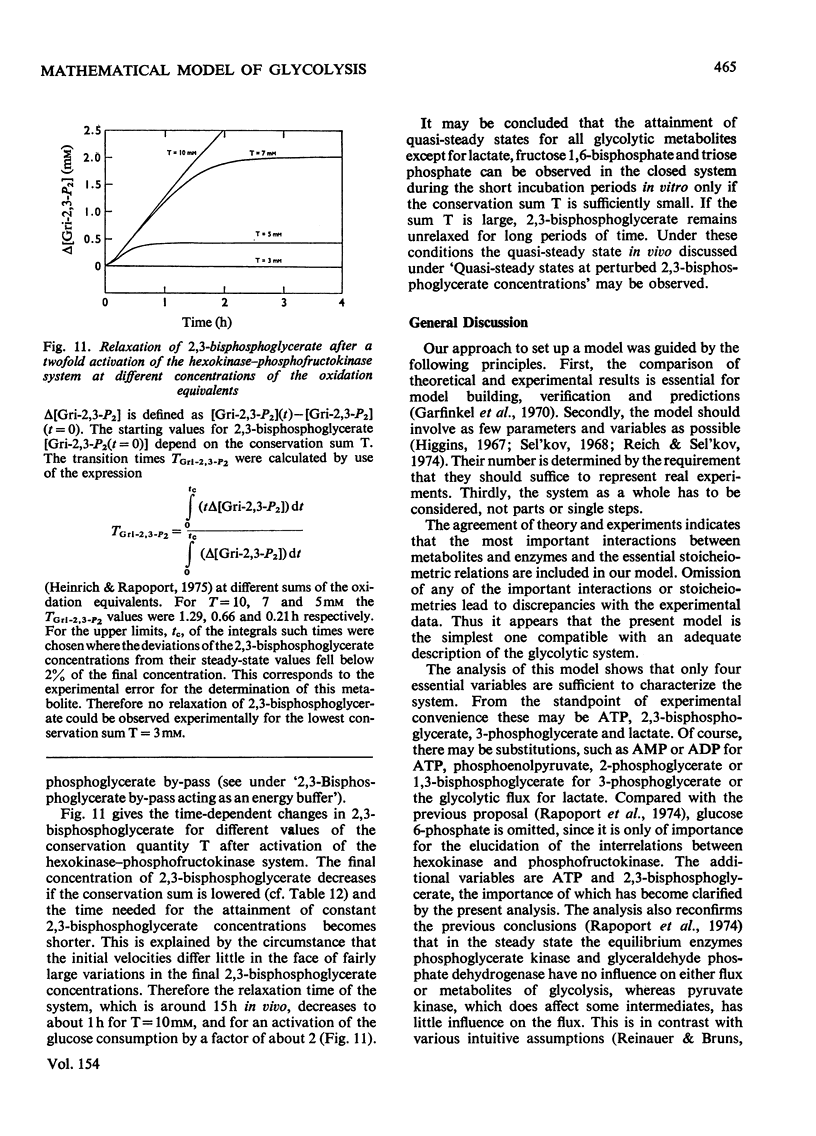
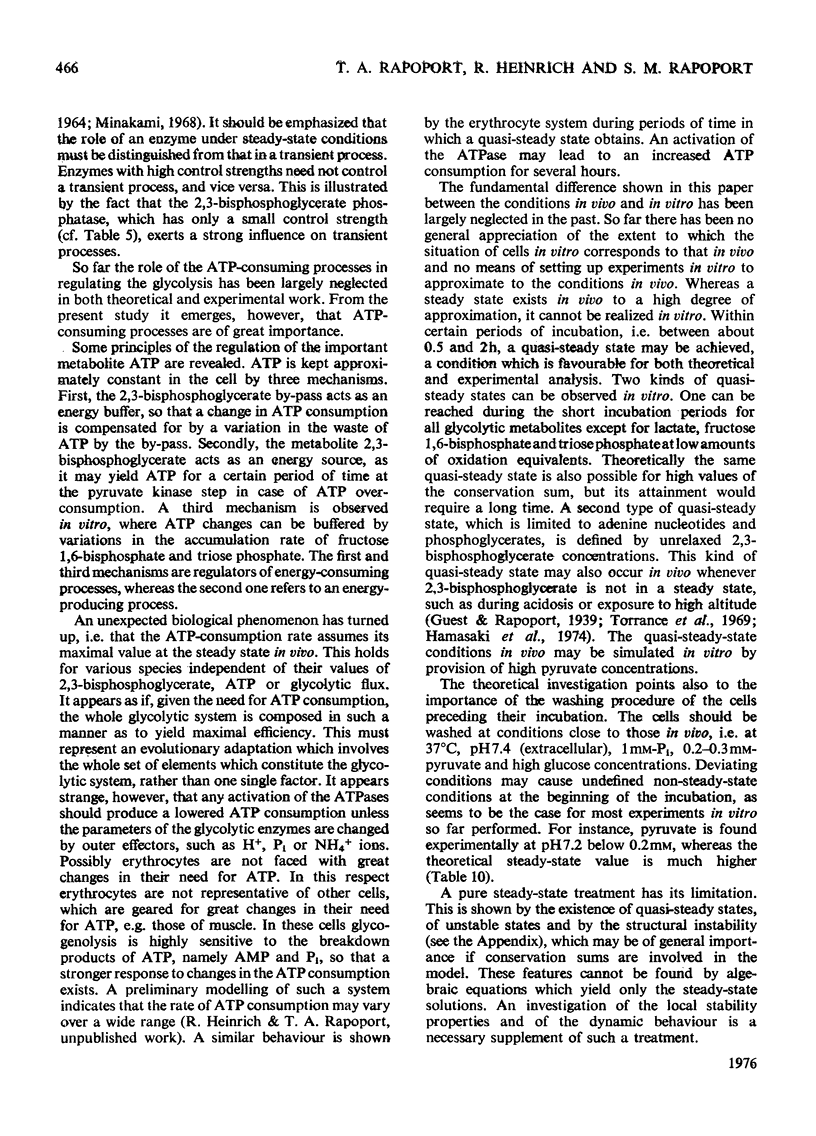
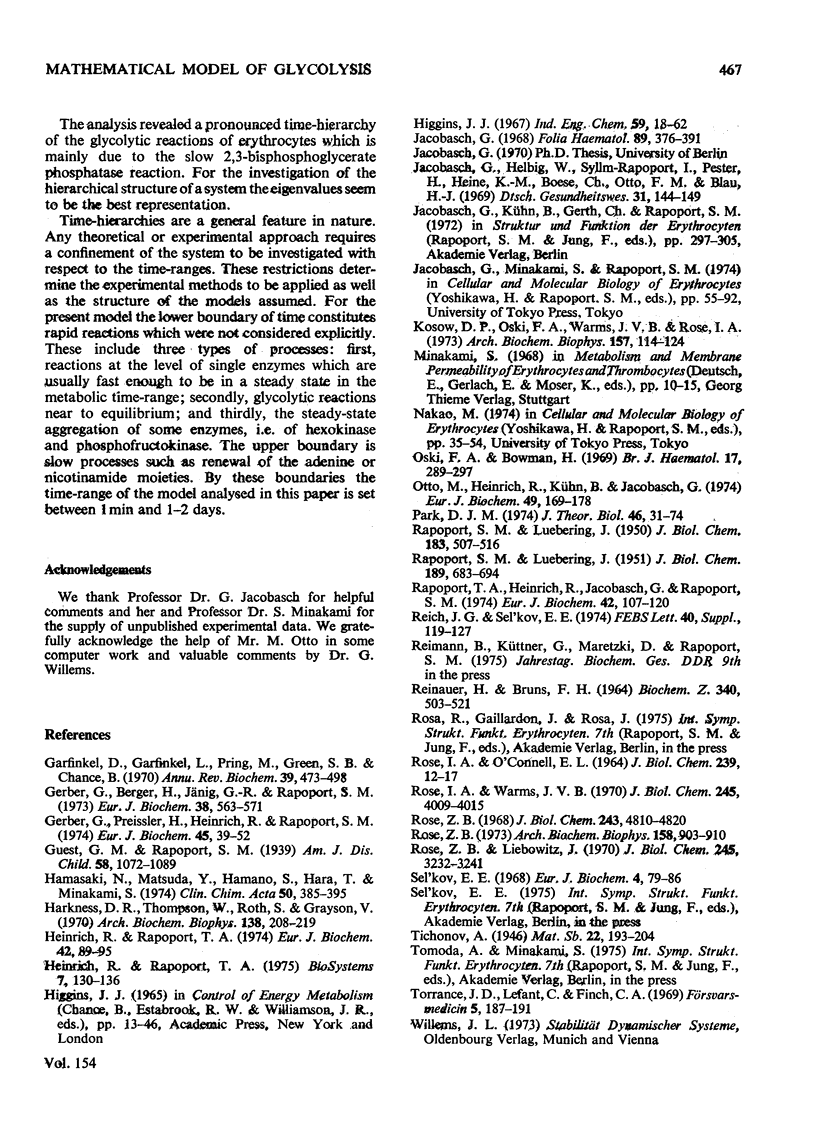
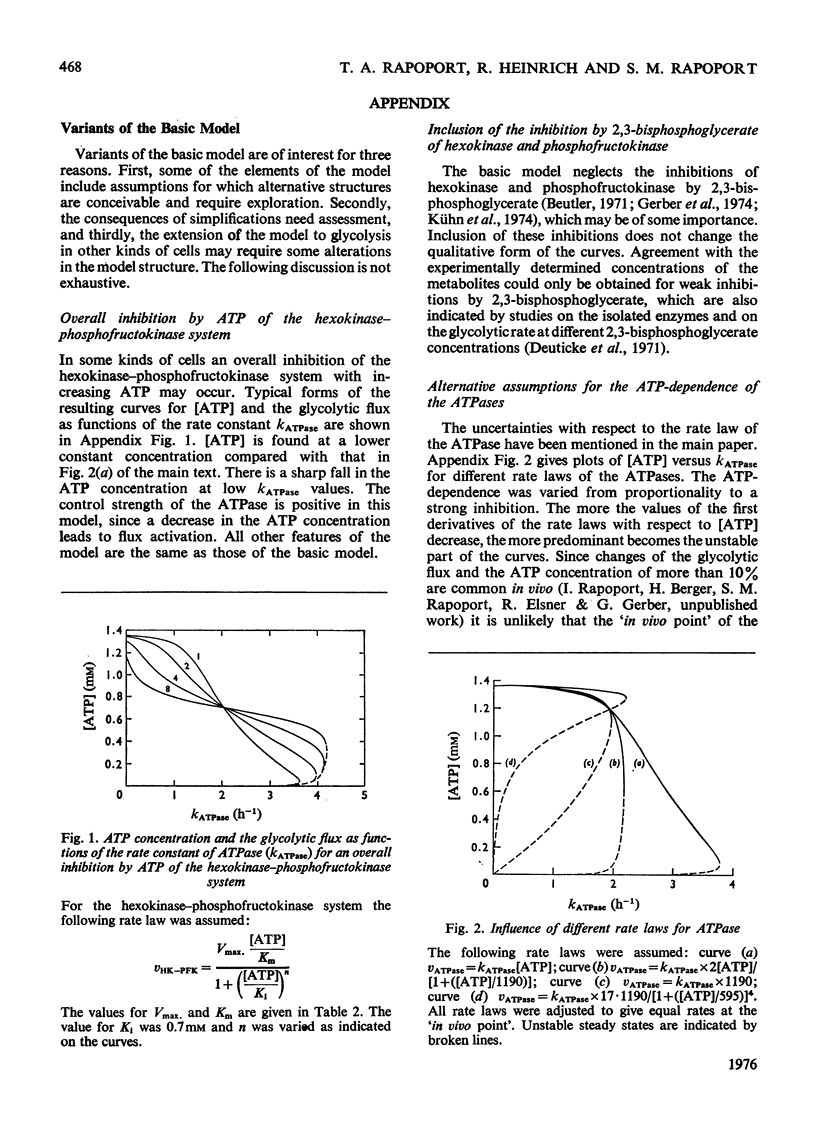
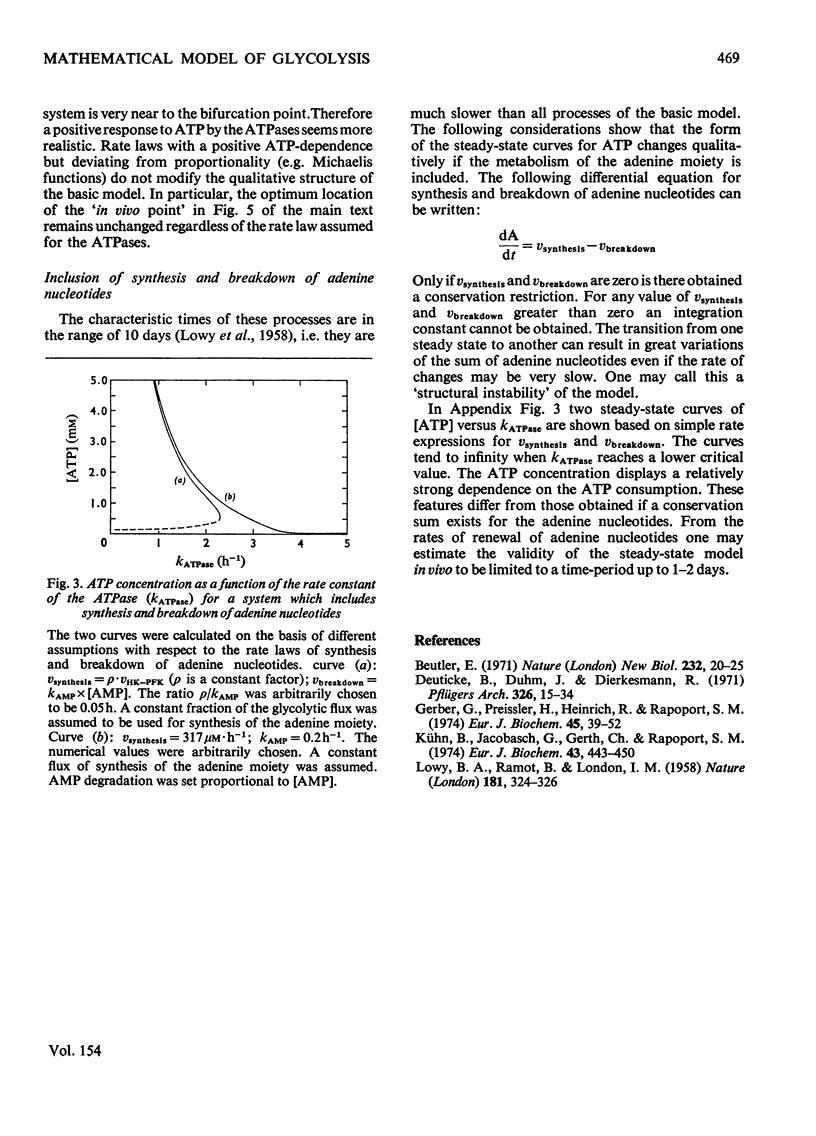
Selected References
These references are in PubMed. This may not be the complete list of references from this article.
- Beutler E. 2,3-diphosphoglycerate affects enzymes of glucose metabolism in red blood cells. Nat New Biol. 1971 Jul 7;232(27):20–21. doi: 10.1038/newbio232020a0. [DOI] [PubMed] [Google Scholar]
- Deuticke B., Duhm J., Dierkesmann R. Maximal elevation of 2,3-diphosphoglycerate concentrations in human erythrocytes: influence on glycolytic metabolism and intracellular pH. Pflugers Arch. 1971;326(1):15–34. doi: 10.1007/BF00586792. [DOI] [PubMed] [Google Scholar]
- Garfinkel D., Garfinkel L., Pring M., Green S. B., Chance B. Computer applications to biochemical kinetics. Annu Rev Biochem. 1970;39:473–498. doi: 10.1146/annurev.bi.39.070170.002353. [DOI] [PubMed] [Google Scholar]
- Gerber G., Berger H., Jänig G. R., Rapoport S. M. Interaction of haemoglobin with ions. Quantitative description of the state of magnesium, adenosine 5'-triphosphate, 2,3-bisphosphoglycerate, and human haemoglobin under simulated intracellular conditions. Eur J Biochem. 1973 Oct 18;38(3):563–571. doi: 10.1111/j.1432-1033.1973.tb03091.x. [DOI] [PubMed] [Google Scholar]
- Gerber G., Preissler H., Heinrich R., Rapoport S. M. Hexokinase of human erythrocytes. Purification, kinetic model and its application to the conditions in the cell. Eur J Biochem. 1974 Jun 1;45(1):39–52. doi: 10.1111/j.1432-1033.1974.tb03527.x. [DOI] [PubMed] [Google Scholar]
- Gerber G., Preissler H., Heinrich R., Rapoport S. M. Hexokinase of human erythrocytes. Purification, kinetic model and its application to the conditions in the cell. Eur J Biochem. 1974 Jun 1;45(1):39–52. doi: 10.1111/j.1432-1033.1974.tb03527.x. [DOI] [PubMed] [Google Scholar]
- Harkness D. R., Thompson W., Roth S., Grayson V. The 2,3-diphosphoglyceric acid phosphatase activity of phosphoglyceric acid mutase purified from human erythrocytes. Arch Biochem Biophys. 1970 May;138(1):208–219. doi: 10.1016/0003-9861(70)90300-0. [DOI] [PubMed] [Google Scholar]
- Heinrich R., Rapoport T. A. A linear steady-state treatment of enzymatic chains. General properties, control and effector strength. Eur J Biochem. 1974 Feb 15;42(1):89–95. doi: 10.1111/j.1432-1033.1974.tb03318.x. [DOI] [PubMed] [Google Scholar]
- Heinrich R., Rapoport T. A. Mathematical analysis of multienzyme systems. II. Steady state and transient control. Biosystems. 1975 Jul;7(1):130–136. doi: 10.1016/0303-2647(75)90050-7. [DOI] [PubMed] [Google Scholar]
- Jacobasch G. Einfluss des Phosphats und des Magnesiums auf die Regulation der Glykolyse. Folia Haematol Int Mag Klin Morphol Blutforsch. 1968;89(4):376–391. [PubMed] [Google Scholar]
- Kosow D. P., Oski F. A., Warms J. V., Rose I. A. Regulation of mammalian hexokinase: regulatory differences between isoenzyme I and II. Arch Biochem Biophys. 1973 Jul;157(1):114–124. doi: 10.1016/0003-9861(73)90396-2. [DOI] [PubMed] [Google Scholar]
- Kühn B., Jacobasch G., Gerth C., Rapoport S. M. Kinetic properties of the phosphofructokinase from erythrocytes of rats and rabbits. 2. The influence of effectors under nearly cellular conditions. Eur J Biochem. 1974 Apr 16;43(3):443–450. doi: 10.1111/j.1432-1033.1974.tb03430.x. [DOI] [PubMed] [Google Scholar]
- LOWY B. A., RAMOT B., LONDON I. M. Adenosine triphosphate metabolism in the rabbit erythrocyte in vivo. Nature. 1958 Feb 1;181(4605):324–326. doi: 10.1038/181324a0. [DOI] [PubMed] [Google Scholar]
- Oski F. A., Bowman H. A low Km phosphoenolpyruvate mutant in the Amish with red cell pyruvate kinase deficiency. Br J Haematol. 1969 Sep;17(3):289–297. doi: 10.1111/j.1365-2141.1969.tb01375.x. [DOI] [PubMed] [Google Scholar]
- Otto M., Heinrich R., KUHN B., Jacobasch G. A mathematical model for the influence of fructose 6-phosphate, ATP, potassium, ammonium and magnesium on the phosphofructokinase from rat erythrocytes;. Eur J Biochem. 1974 Nov 1;49(1):169–178. doi: 10.1111/j.1432-1033.1974.tb03822.x. [DOI] [PubMed] [Google Scholar]
- Park D. J. The hierarchical structure of metabolic networks and the construction of efficient metabolic simulators. J Theor Biol. 1974 Jul;46(1):31–74. doi: 10.1016/0022-5193(74)90139-8. [DOI] [PubMed] [Google Scholar]
- RAPOPORT S., LUEBERING J. Glycerate-2,3-diphosphatase. J Biol Chem. 1951 Apr;189(2):683–694. [PubMed] [Google Scholar]
- REINAUER H., BRUNS F. H. LIMITIERENDE FAKTOREN DER GLYKOLYSE IN ROTEN BLUTZELLEN DES MENSCHEN. ZUGLEICH EIN BEITRAG ZUM MECHANISMUS DER HB(3)-REDUKTION. Biochem Z. 1964 Nov 6;340:503–521. [PubMed] [Google Scholar]
- ROSE I. A., O'CONNELL E. L. THE ROLE OF GLUCOSE 6-PHOSPHATE IN THE REGULATION OF GLUCOSE METABOLISM IN HUMAN ERYTHROCYTES. J Biol Chem. 1964 Jan;239:12–17. [PubMed] [Google Scholar]
- Rapoport T. A., Heinrich R., Jacobasch G., Rapoport S. A linear steady-state treatment of enzymatic chains. A mathematical model of glycolysis of human erythrocytes. Eur J Biochem. 1974 Feb 15;42(1):107–120. doi: 10.1111/j.1432-1033.1974.tb03320.x. [DOI] [PubMed] [Google Scholar]
- Rose I. A., Warms J. V. Control of red cell glycolysis. The cause of triose phosphate accumulation. J Biol Chem. 1970 Aug 25;245(16):4009–4015. [PubMed] [Google Scholar]
- Rose Z. B. Effects of salts and pH on the rate of erythrocyte diphosphoglycerate mutase. Arch Biochem Biophys. 1973 Oct;158(2):903–910. doi: 10.1016/0003-9861(73)90585-7. [DOI] [PubMed] [Google Scholar]
- Rose Z. B., Liebowitz J. 2,3-diphosphoglycerate phosphatase from human erythrocytes. General properties and activation by anions. J Biol Chem. 1970 Jun;245(12):3232–3241. [PubMed] [Google Scholar]
- Rose Z. B. The purification and properties of diphosphoglycerate mutase from human erythrocytes. J Biol Chem. 1968 Sep 25;243(18):4810–4820. [PubMed] [Google Scholar]
- Sel'kov E. E. Self-oscillations in glycolysis. 1. A simple kinetic model. Eur J Biochem. 1968 Mar;4(1):79–86. doi: 10.1111/j.1432-1033.1968.tb00175.x. [DOI] [PubMed] [Google Scholar]