Abstract
1. The patch clamp technique was used to investigate the K+ channels in the membranes of nerve terminals in thin slices prepared from the rat posterior pituitary. 2. Depolarization of the membrane produced a high density of K+ current. With a holding potential of -80 mV, test pulses to +50 mV activated a K+ current which was inactivated by 65% within 200 ms. Hyperpolarizing prepulses enhanced the transient K+ current, with half-maximal enhancement at -87 mV. Depolarizing prepulses reduced or eliminated the transient K+ current. 3. In cell-attached patches formed with pipettes containing 130 mM KCl, three types of K+ channel could be distinguished on the basis of single-channel properties. One channel had a conductance of 33 pS and was inactivated with a time constant of 18 ms. A second channel had a conductance of 134 pS and was inactivated with a time constant of 71 ms. A third channel had a conductance of 27 pS, was activated relatively slowly with a time constant of 65 ms, and was not inactivated during test pulses of up to one second in duration. 4. Inactivation of the whole-cell K+ current was a biphasic process with two exponential components. The fast component had a time constant of 22 ms (at +50 mV), corresponding well with the time constant of decay of average current in cell-attached patches containing only the rapidly inactivating K+ channel. The slow component of inactivation had a time constant of 104 ms (at +50 mV), which was similar to but slightly slower than the time constant of decay of the average current in cell-attached patches containing only the slowly inactivating K+ channel. Inactivation of the slow transient K+ current became more rapid with increasing depolarization. 5. The low-conductance rapidly inactivating K+ channel had a lower voltage threshold for activation than the other two K+ channels. 6. Both inactivating K+ channels were enhanced in a similar manner by prior hyperpolarization. There was no difference with regard to voltage mid-point or steepness. 7. The large-conductance slowly inactivating K+ channel was activated by Ca2+ at the inner membrane surface. The resting intracellular Ca2+ was sufficiently high to produce significant activation of this channel without depolarization-induced Ca2+ entry. 8. Removal of Ca2+ from the bathing solution produced a -10 mV shift in the voltage dependence of enhancement of both transient K+ currents by prior hyperpolarization. This could be explained as a surface charge effect.(ABSTRACT TRUNCATED AT 400 WORDS)
Full text
PDF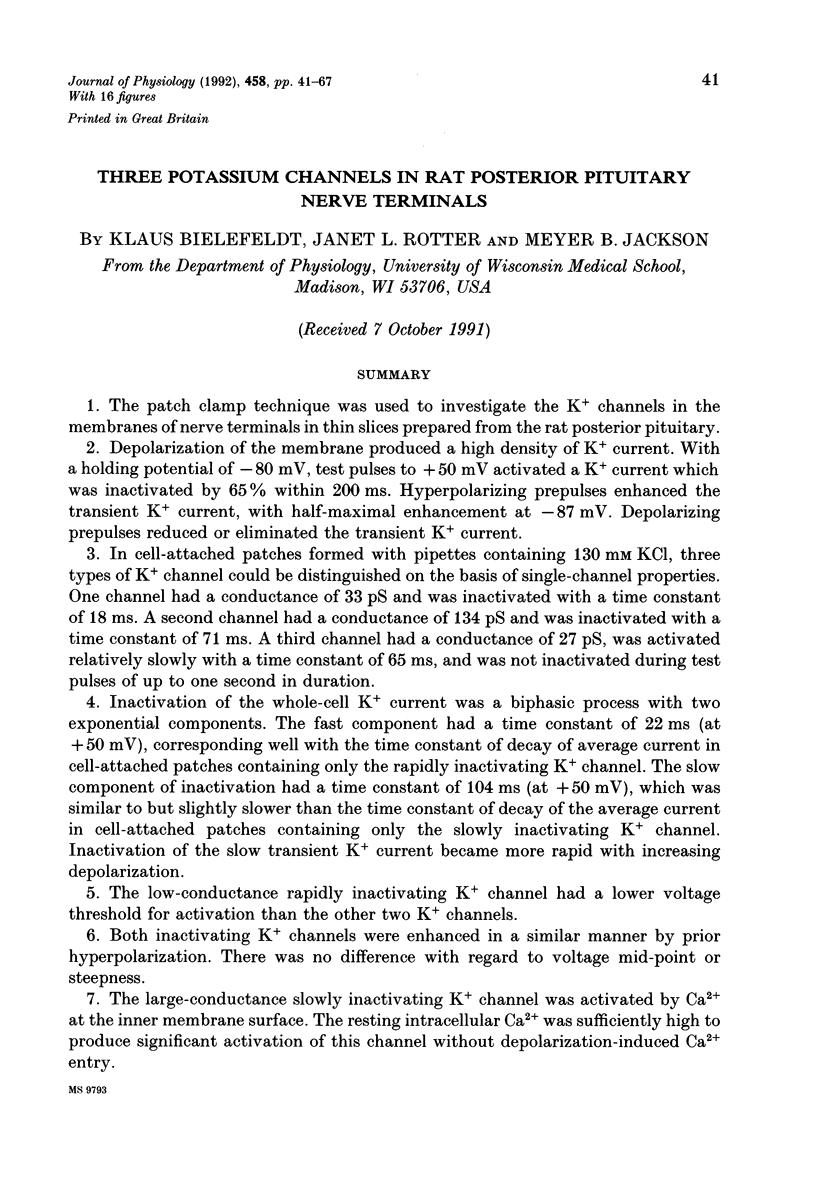
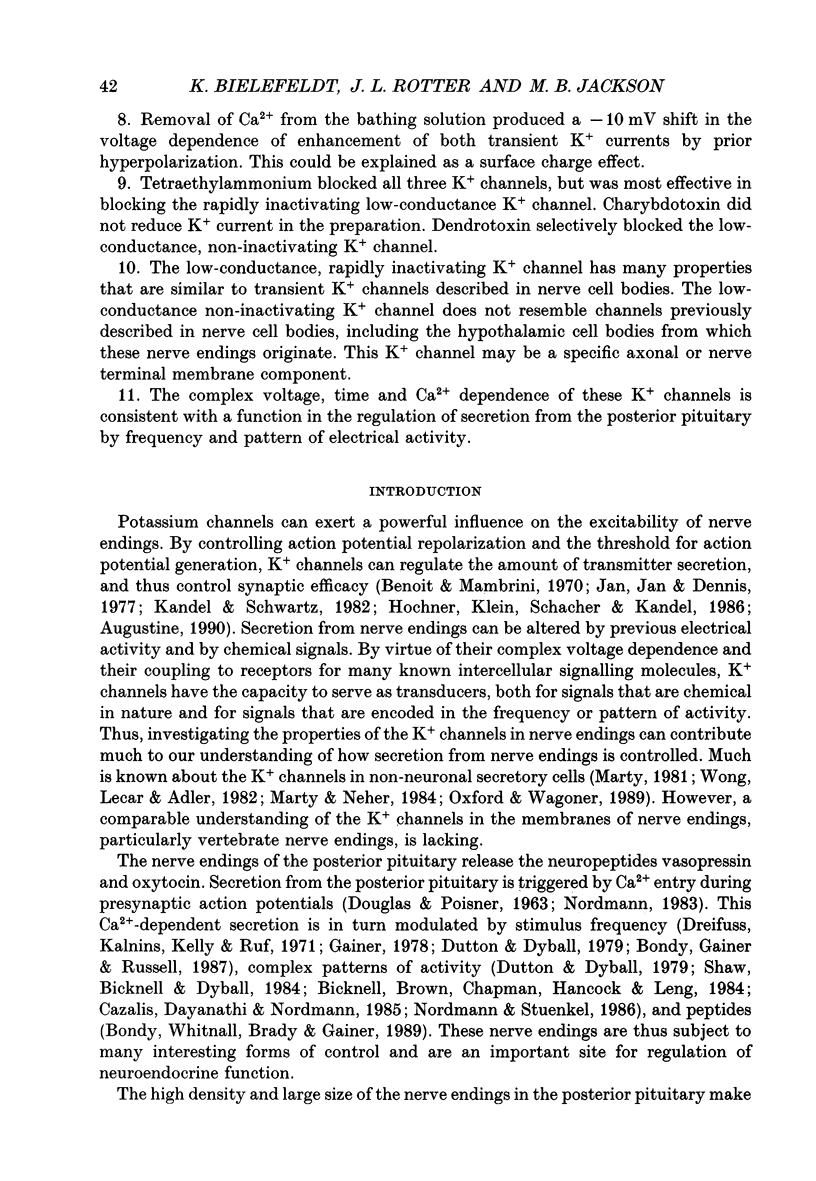
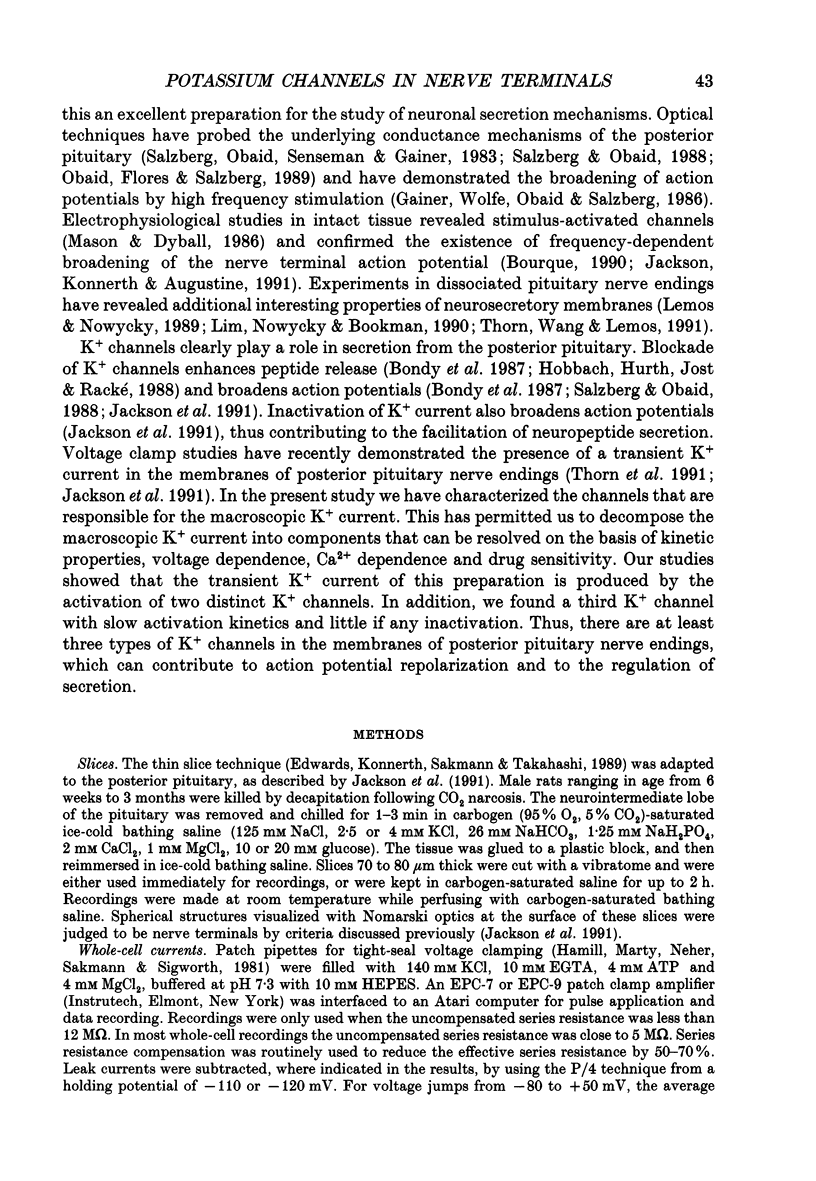
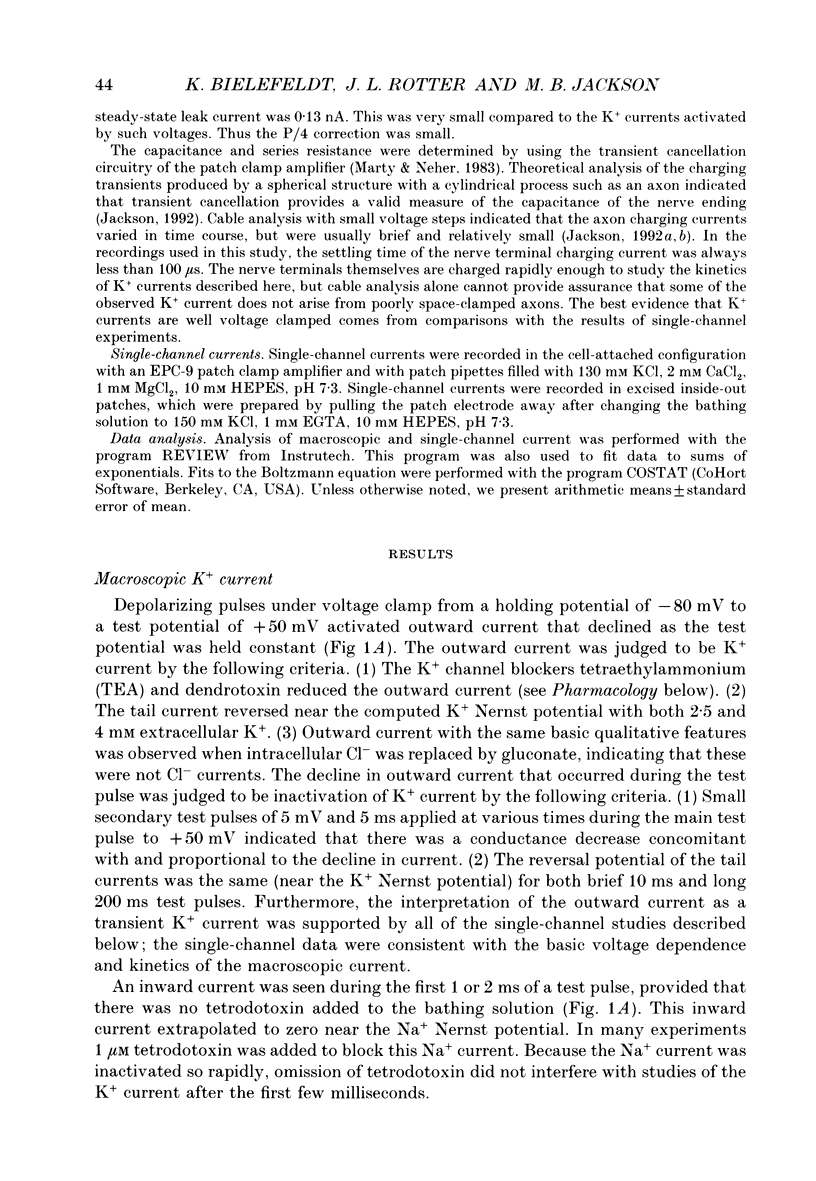
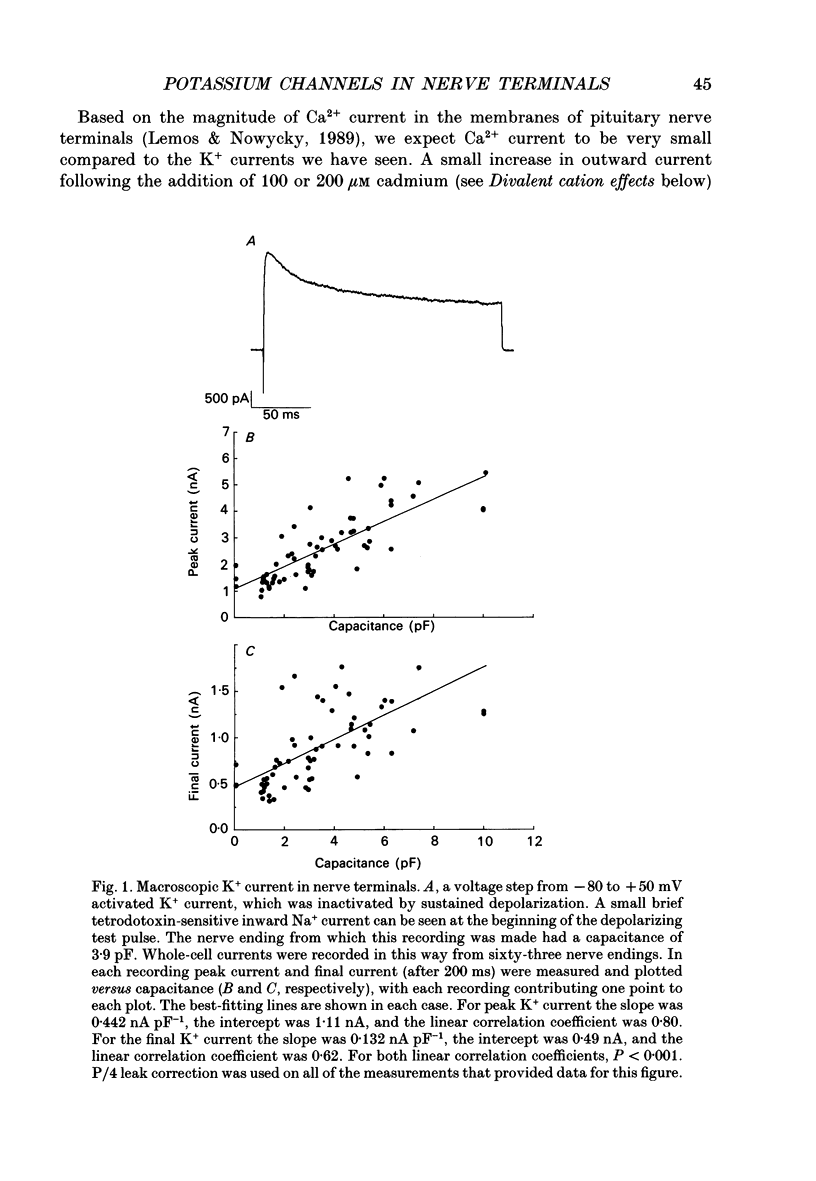
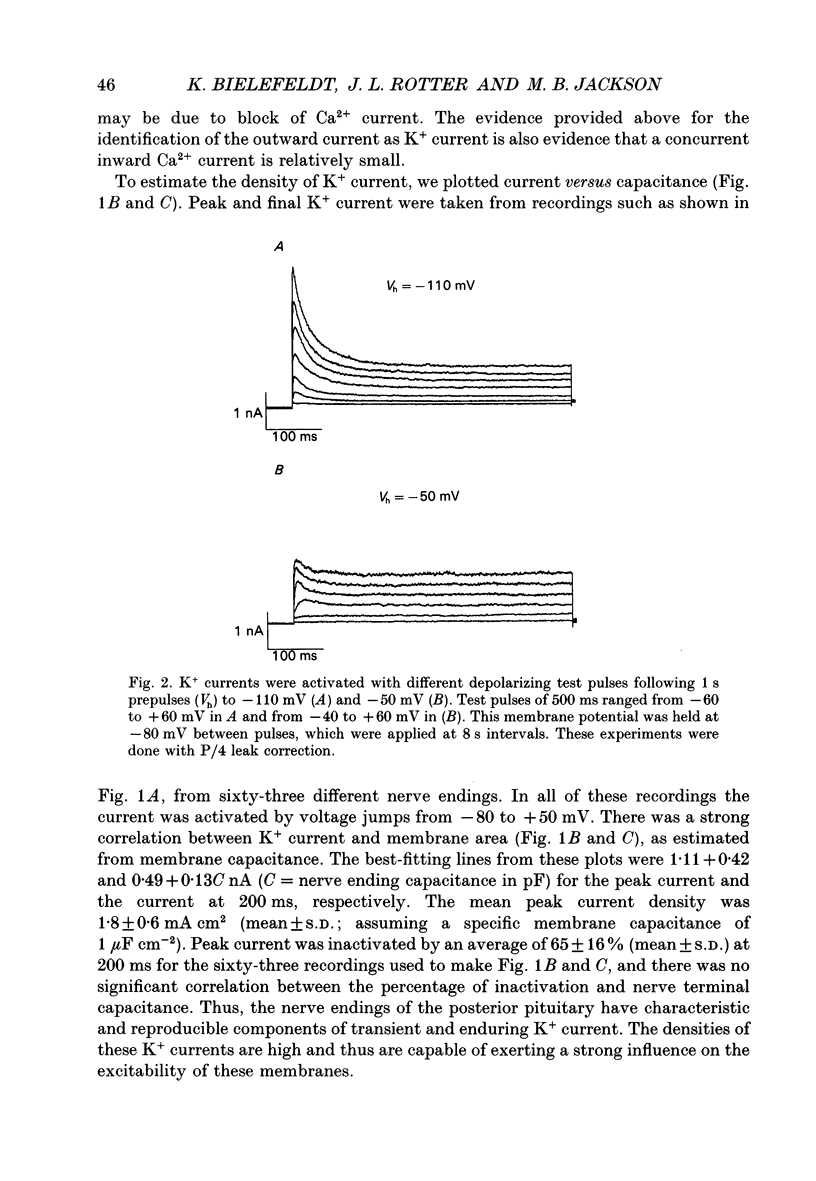
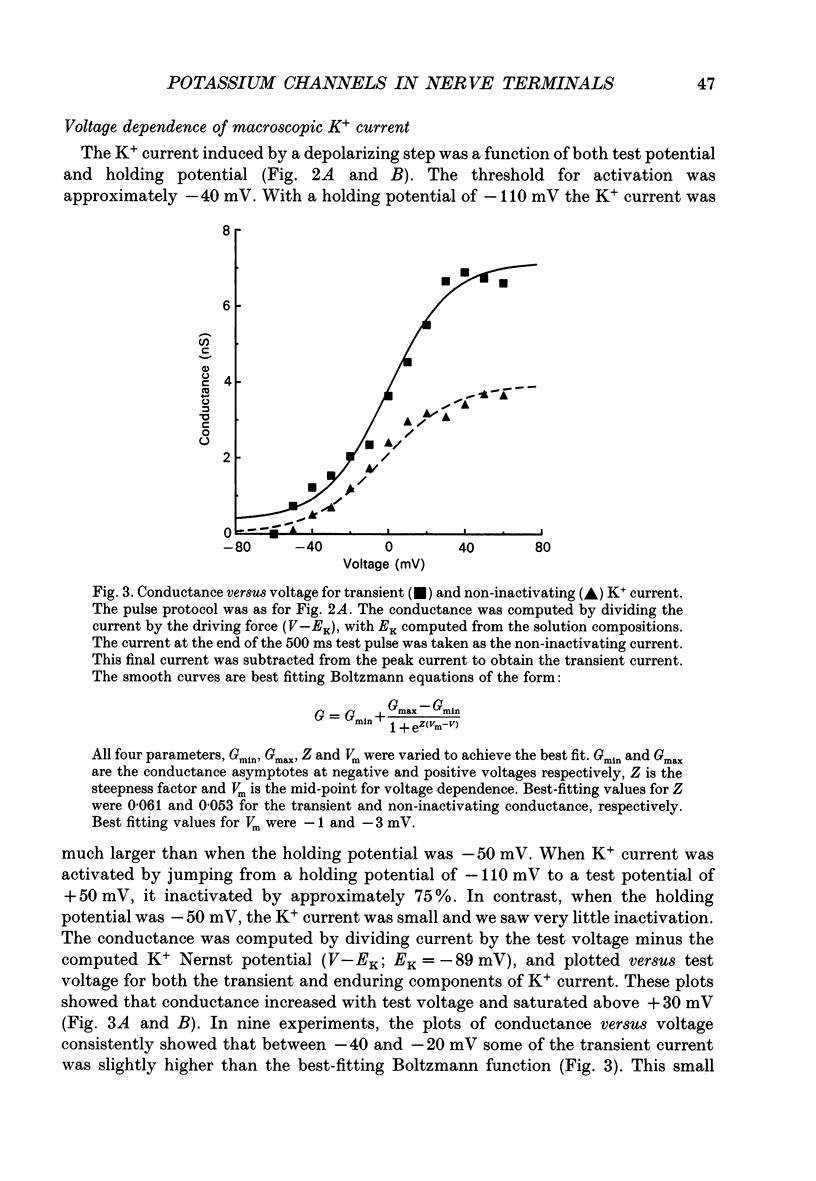
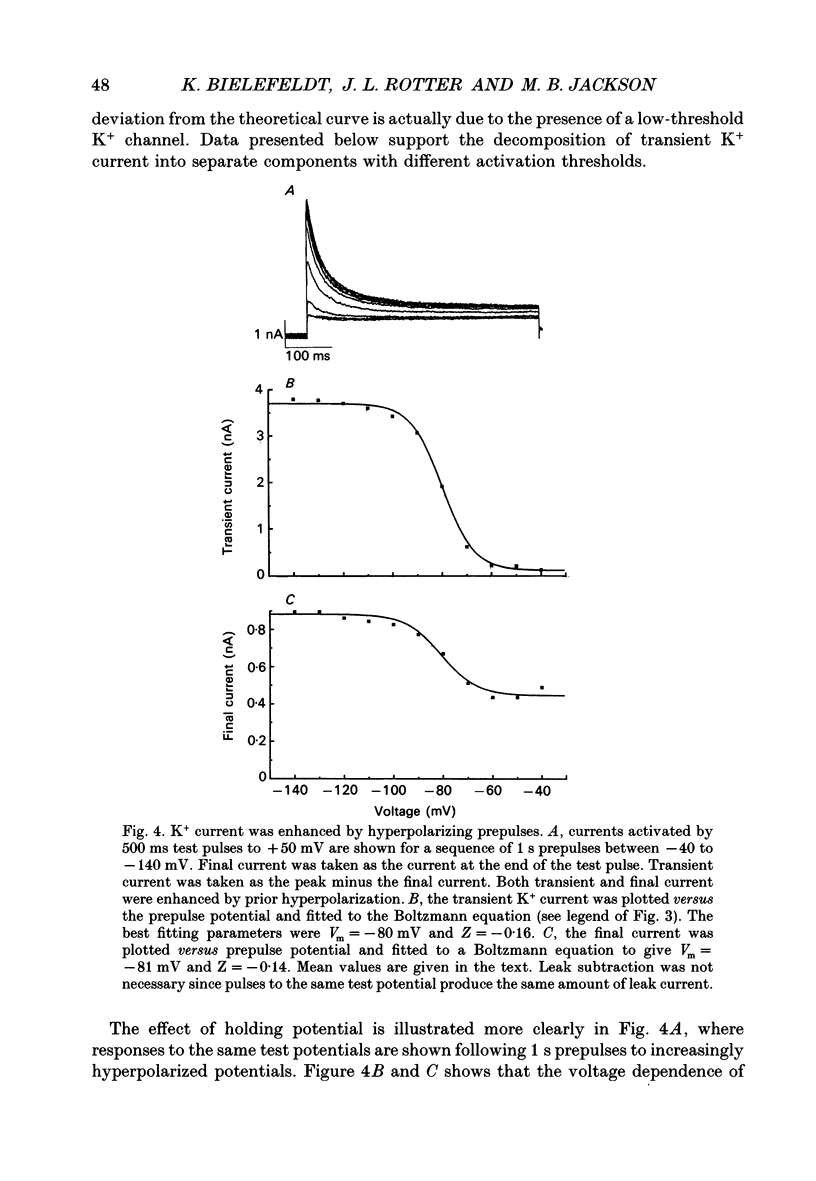
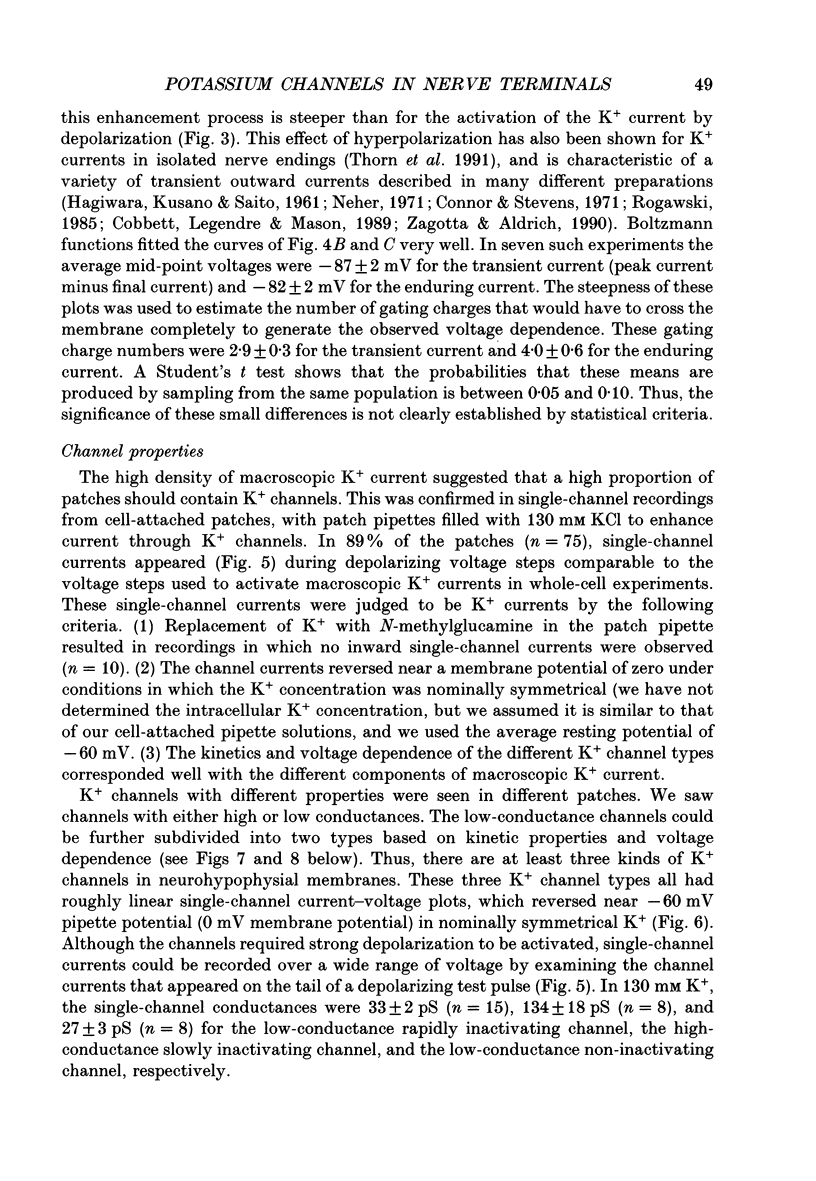
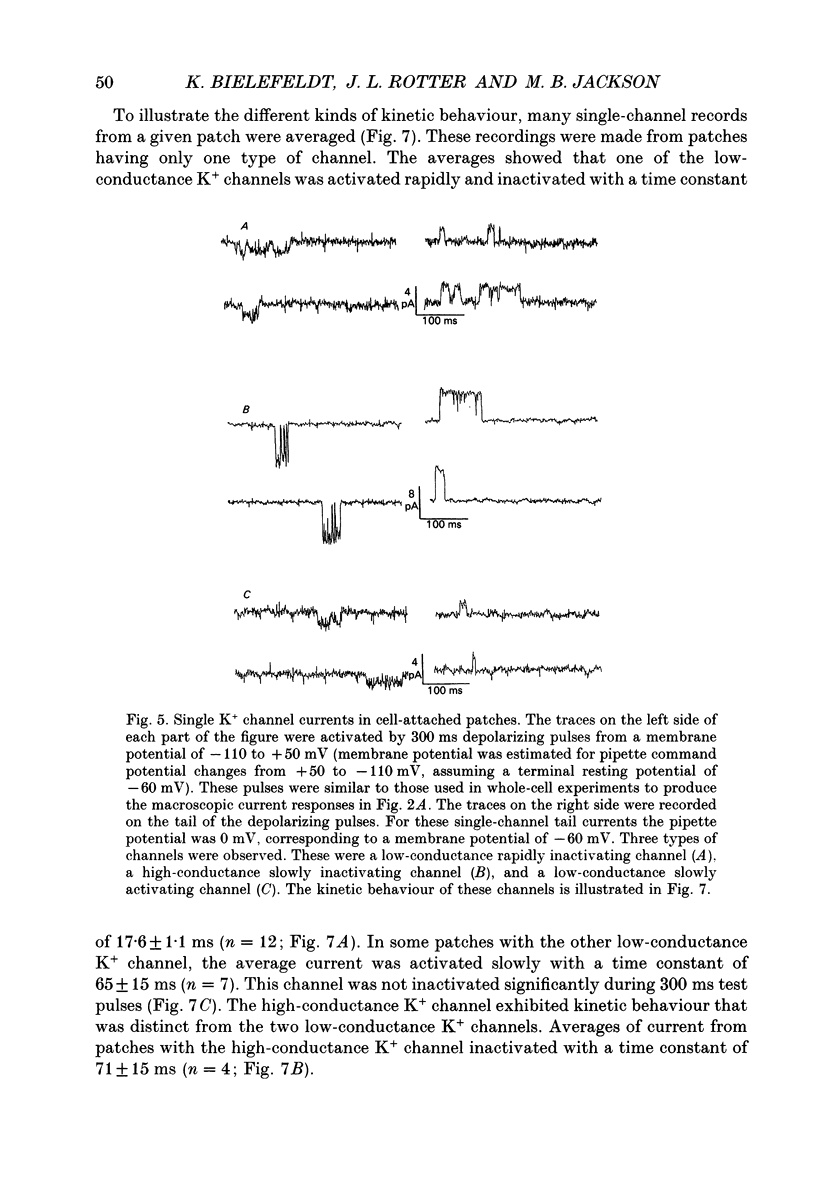
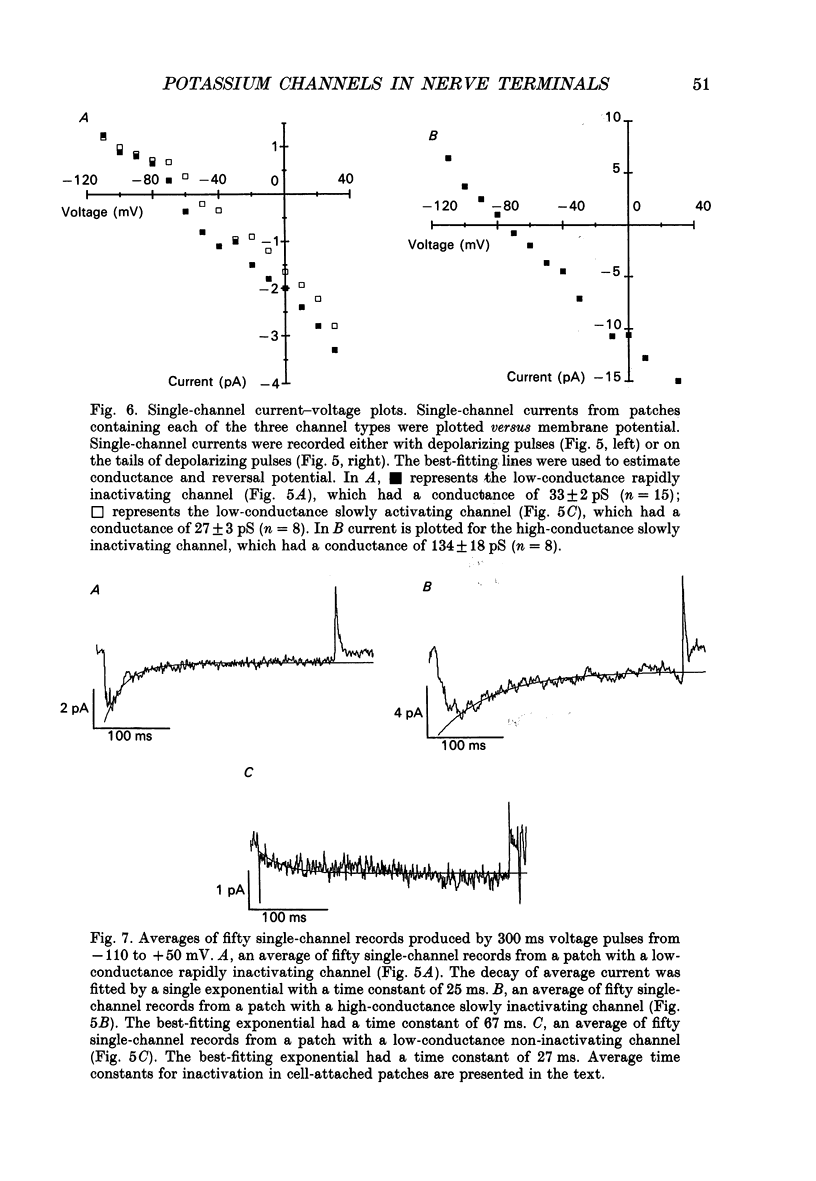
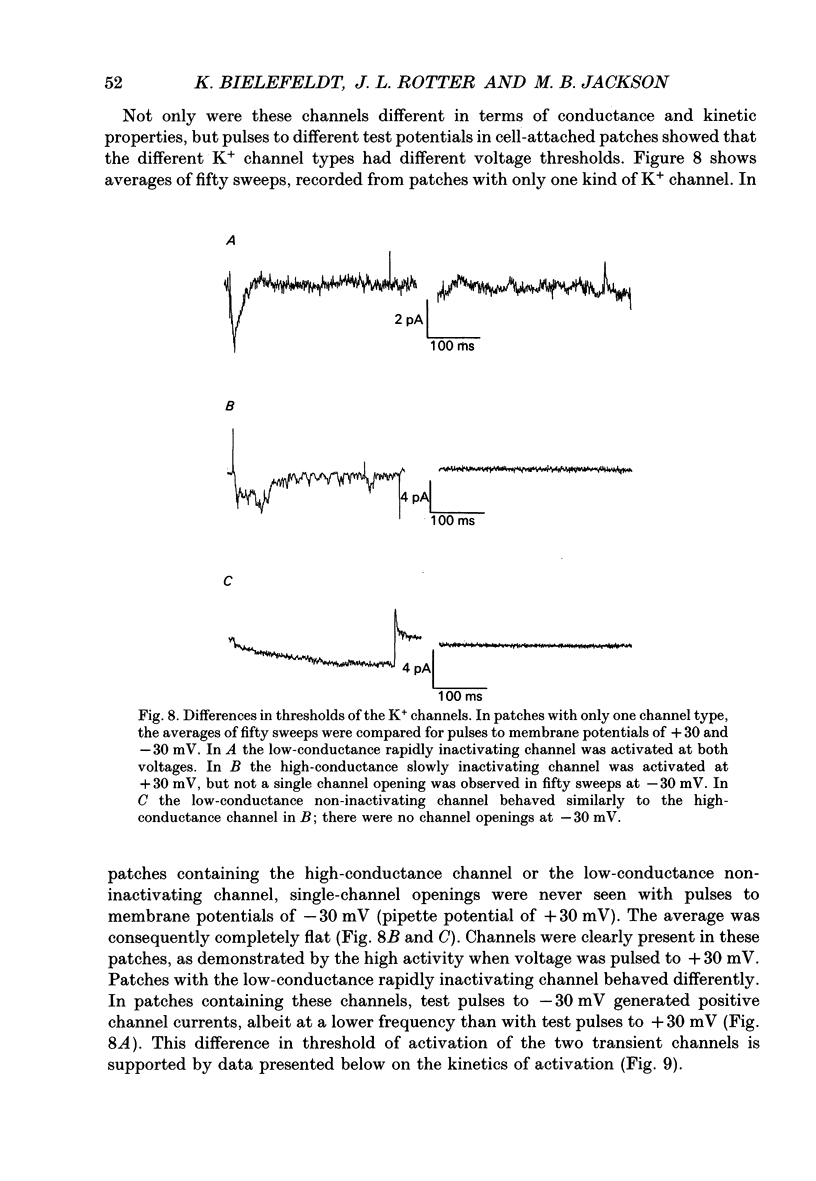
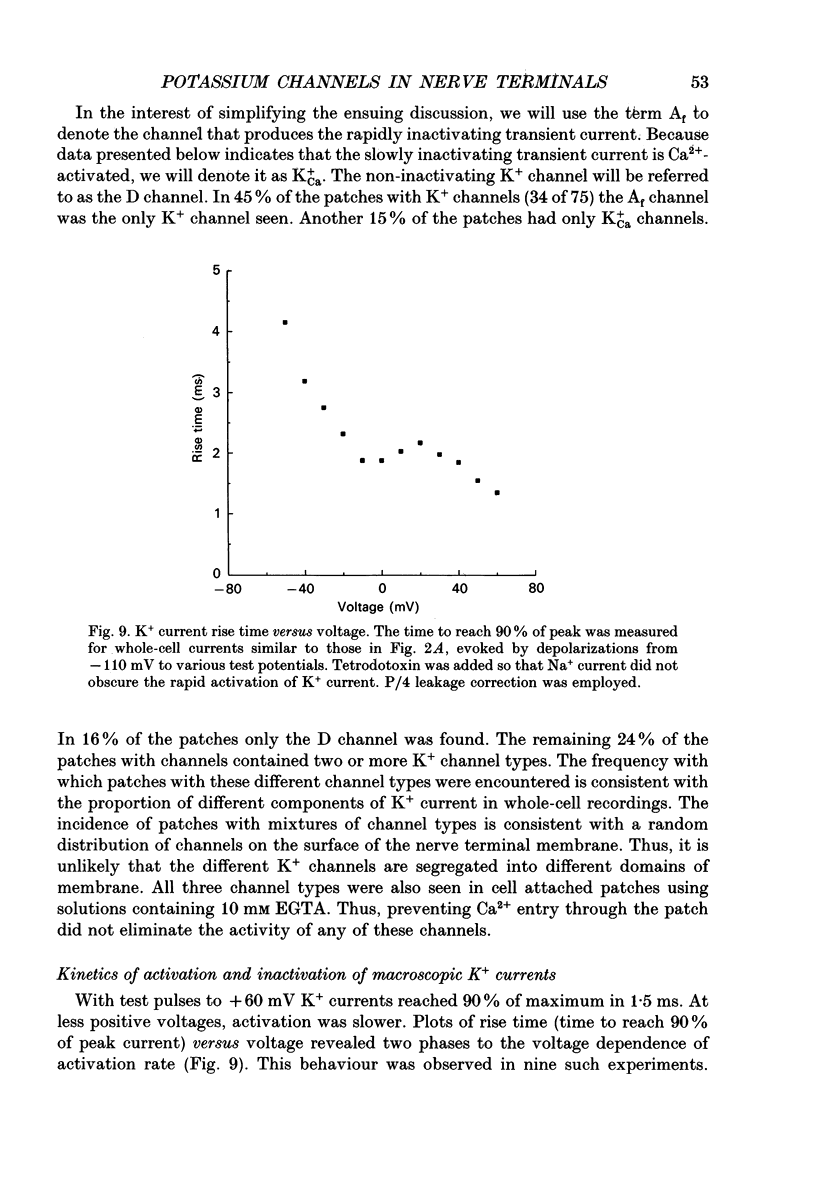
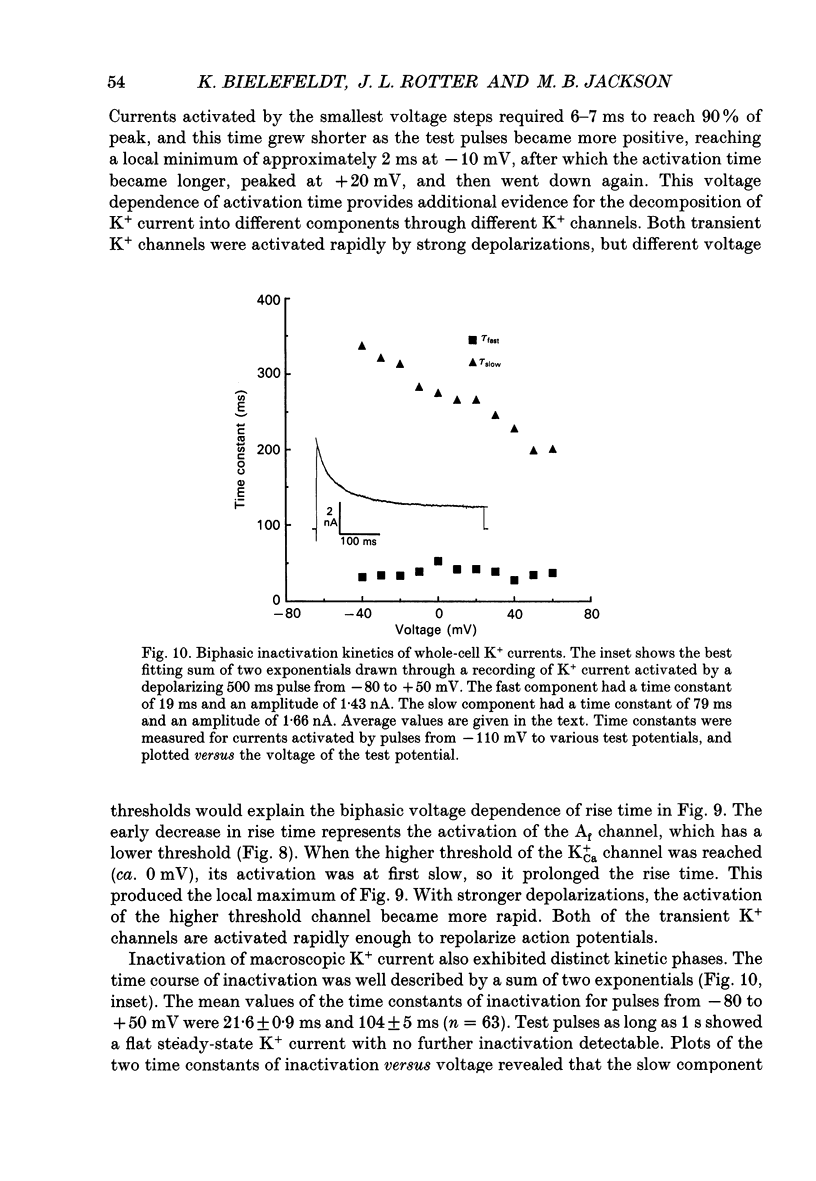
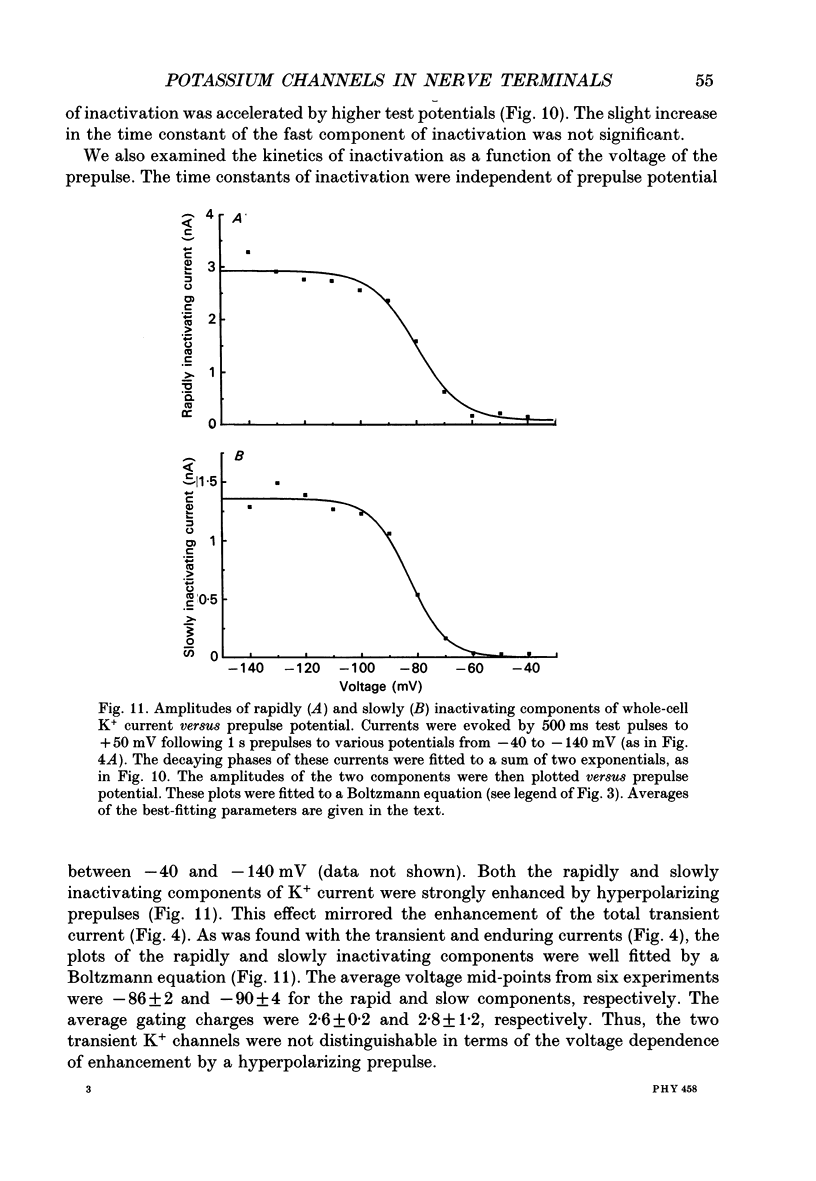
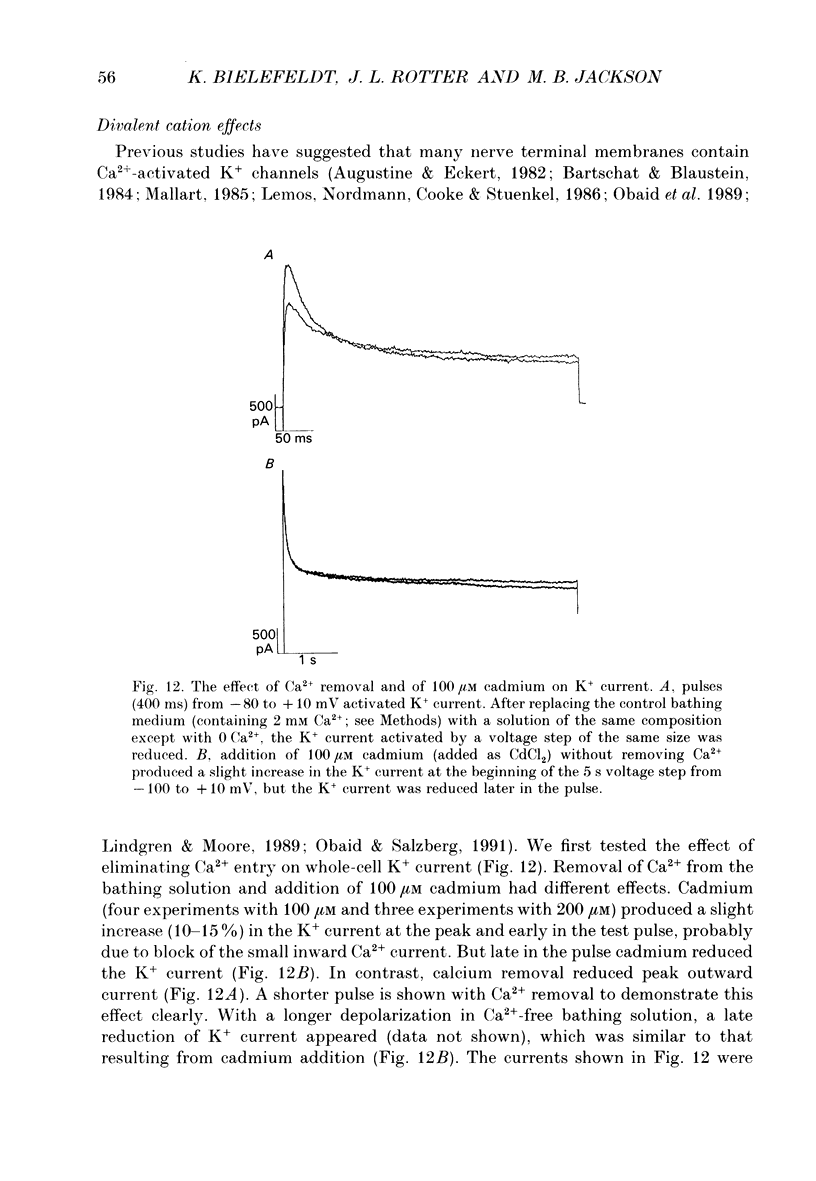
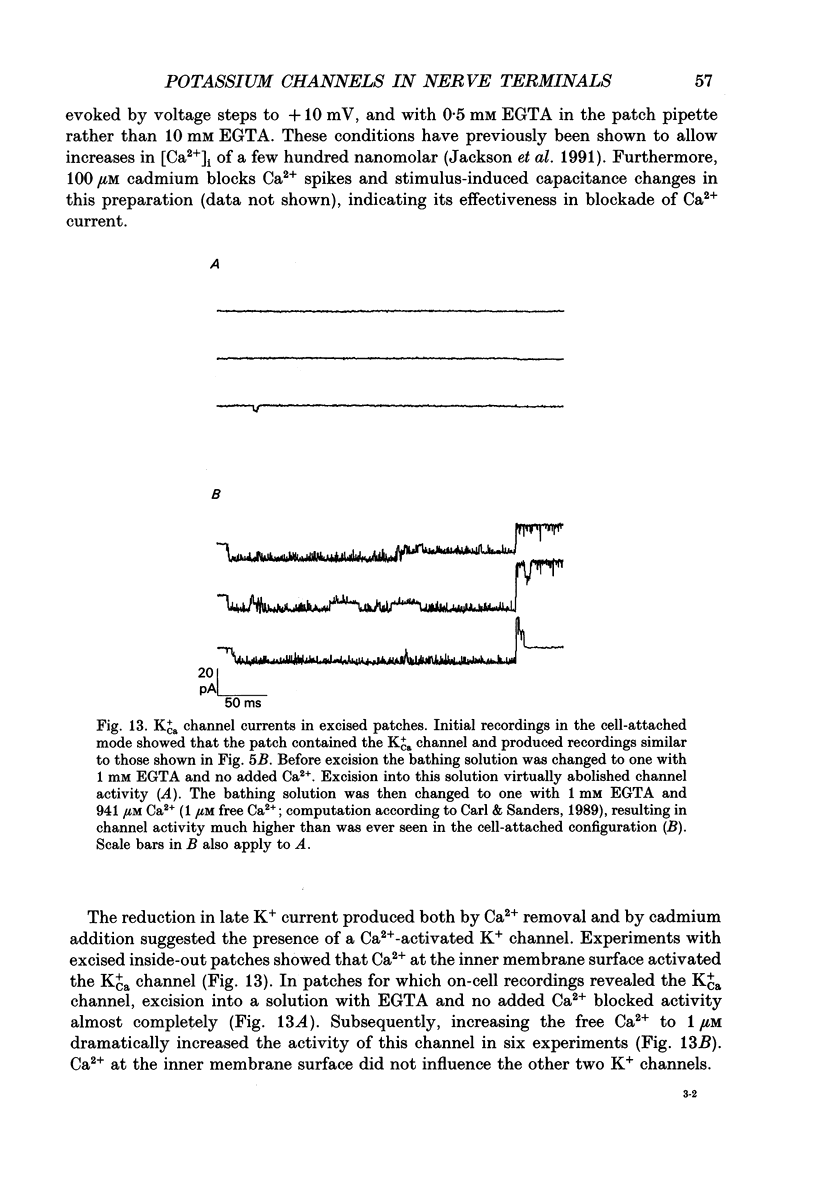
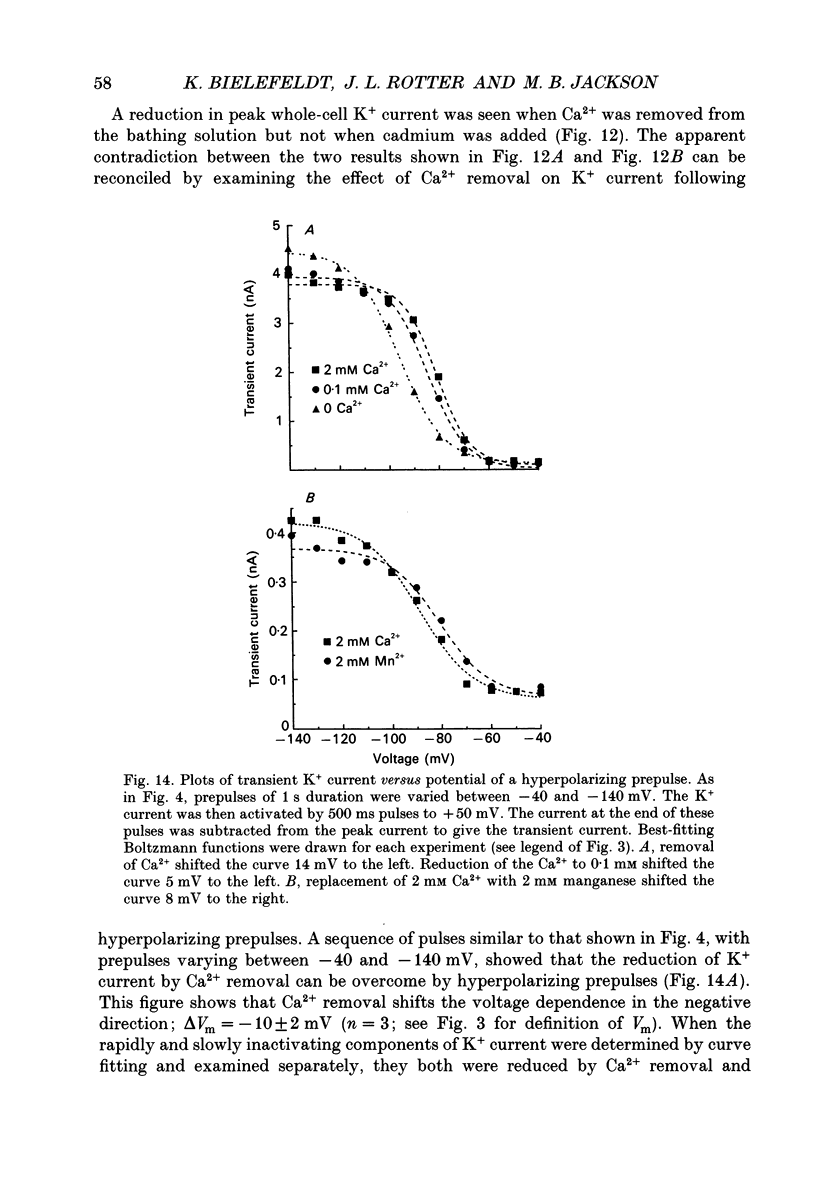
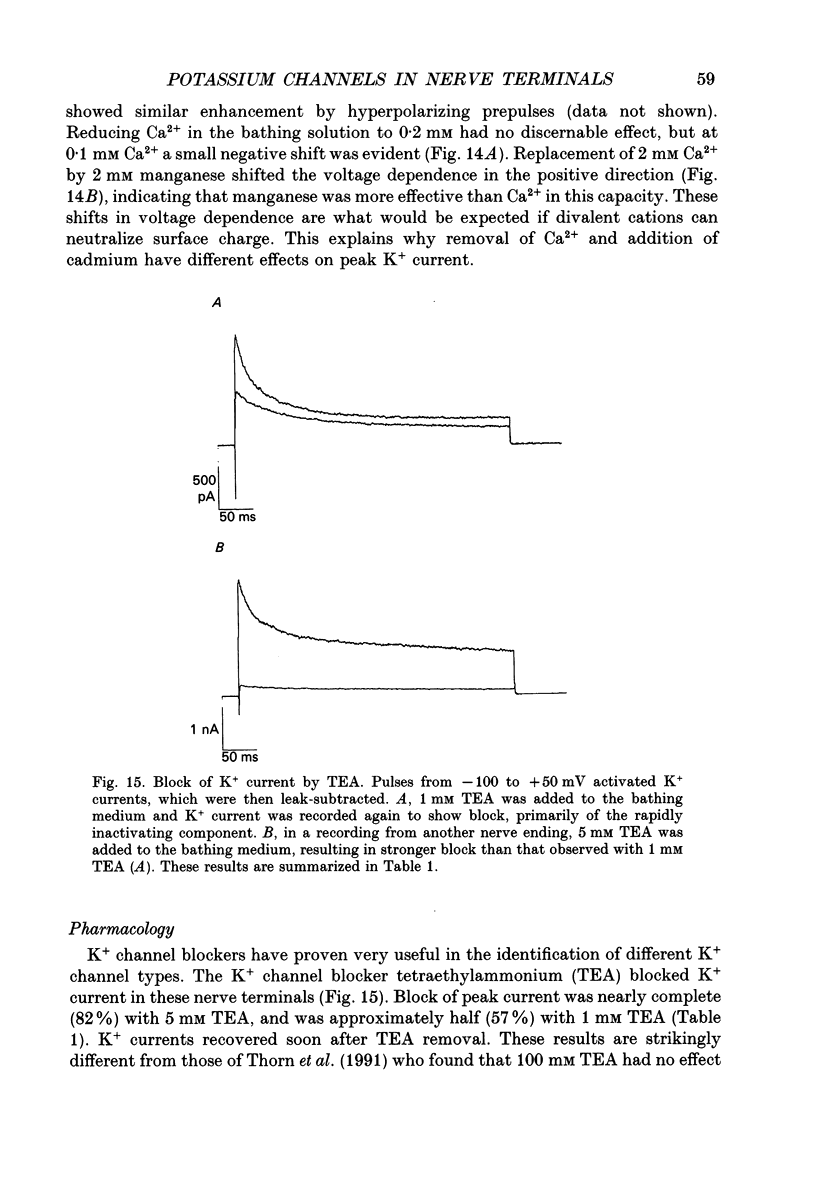
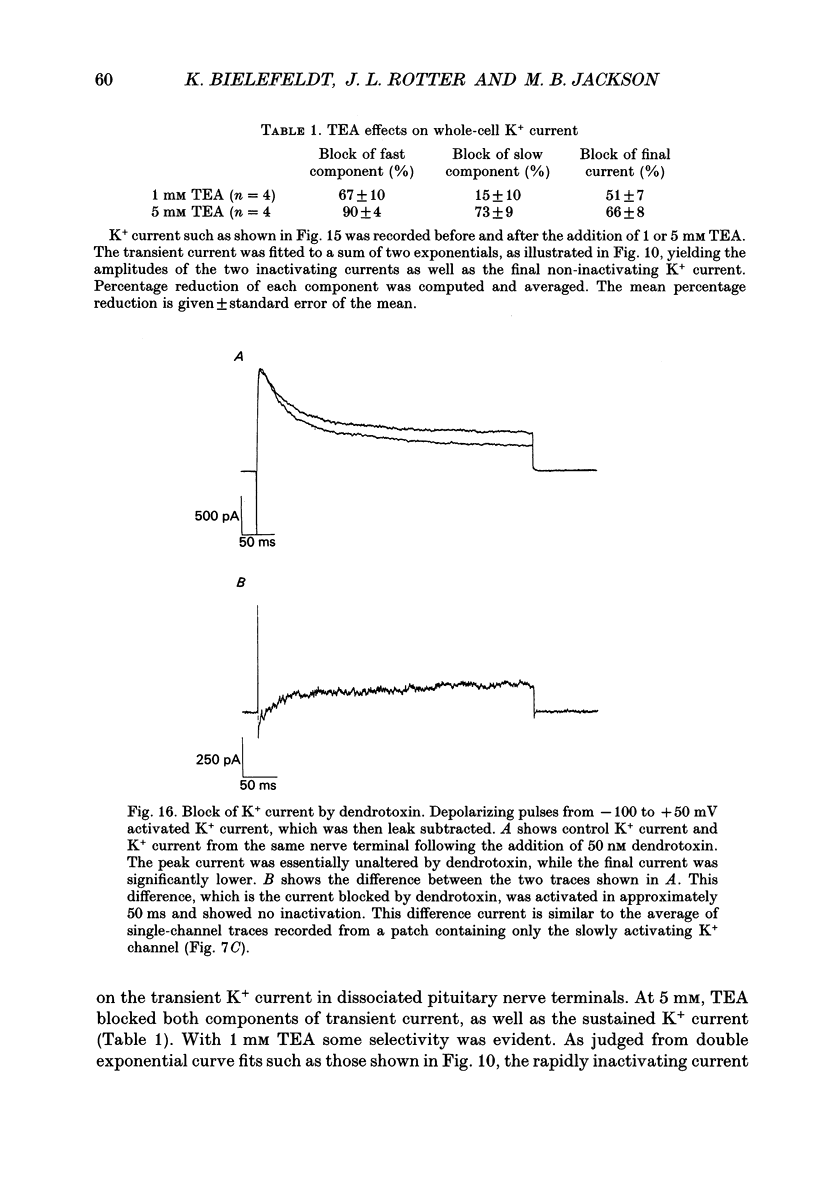
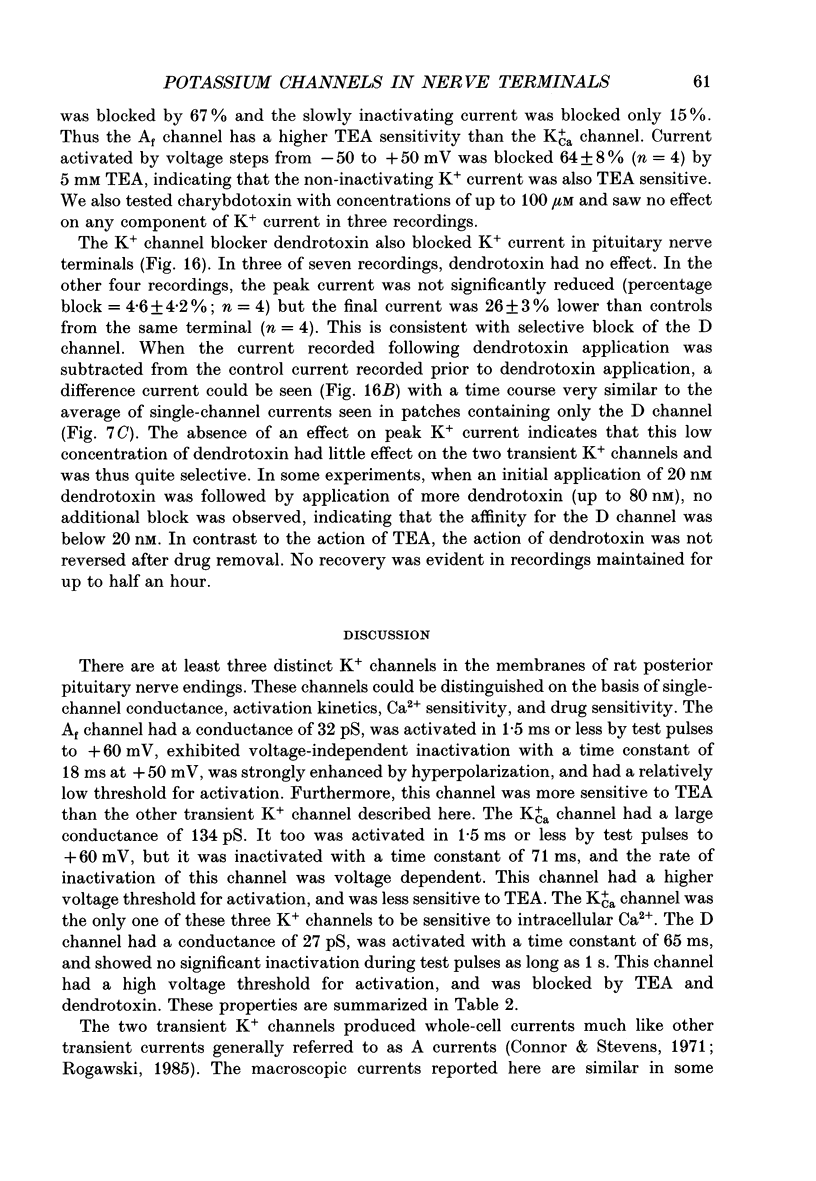
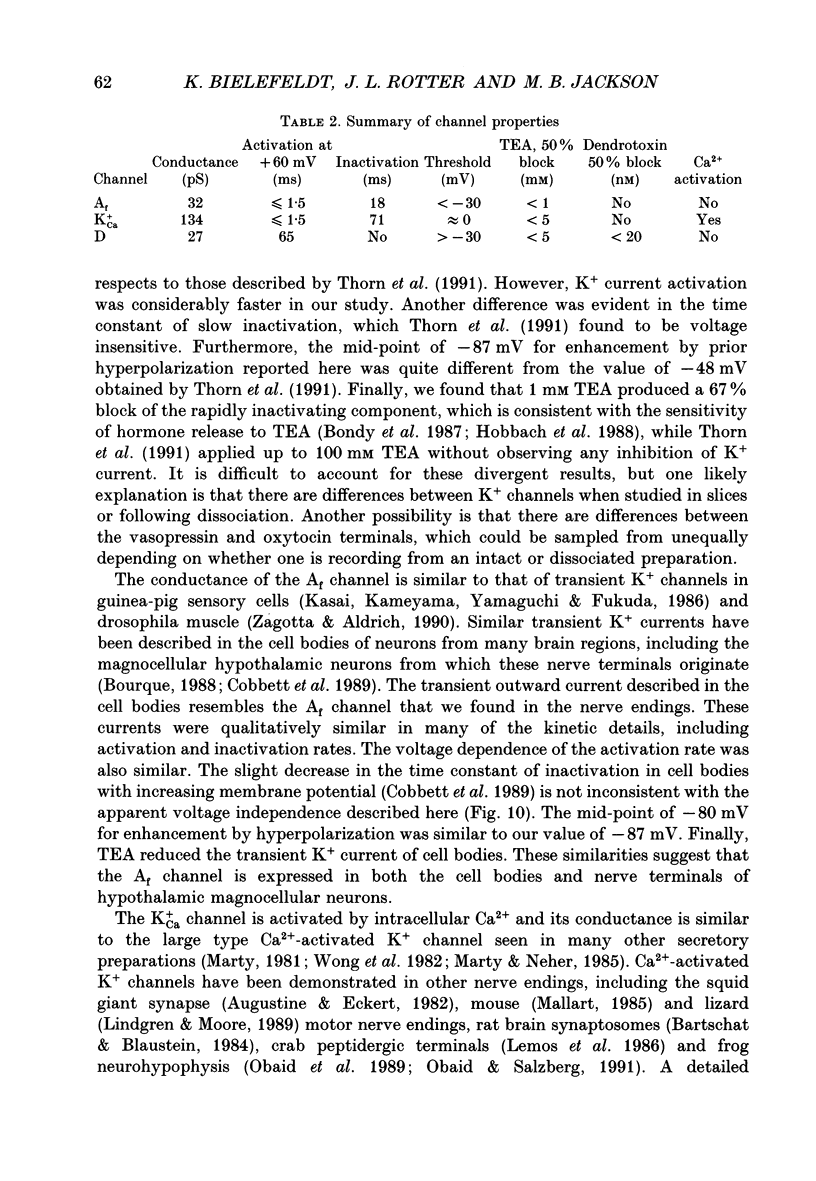
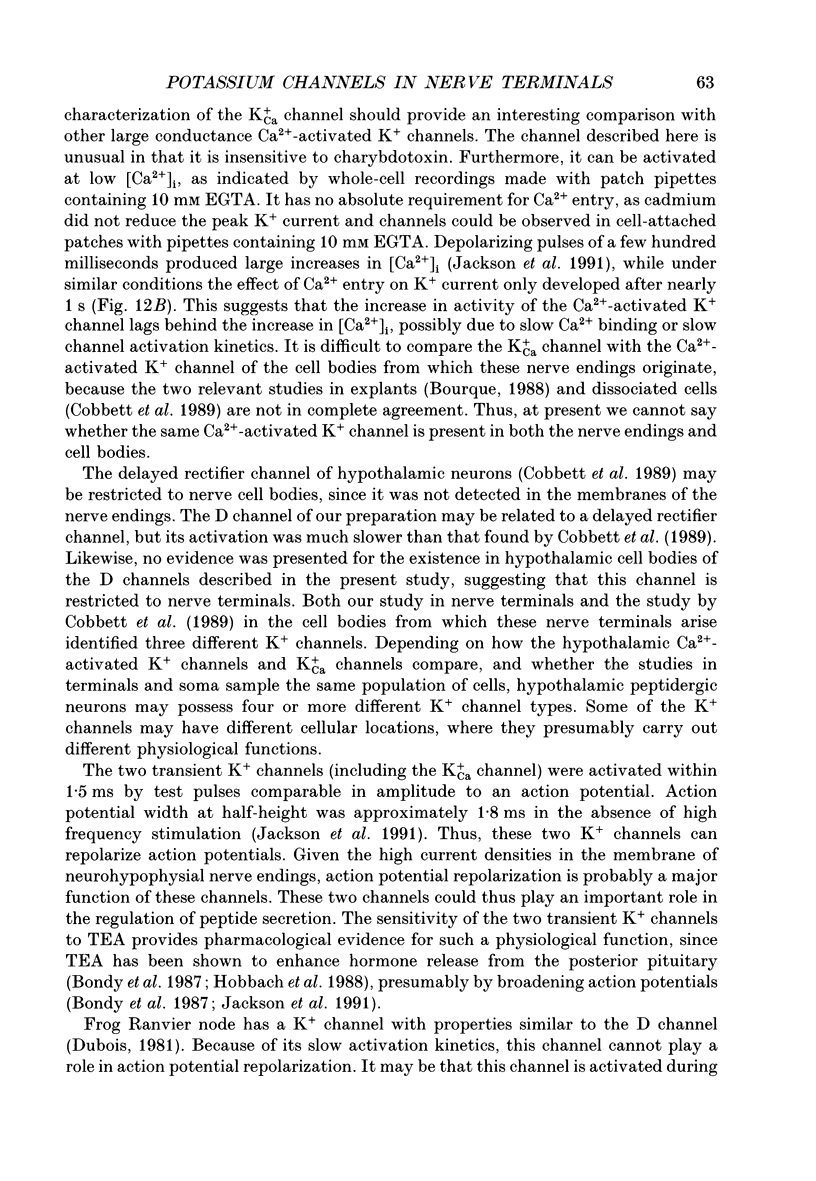
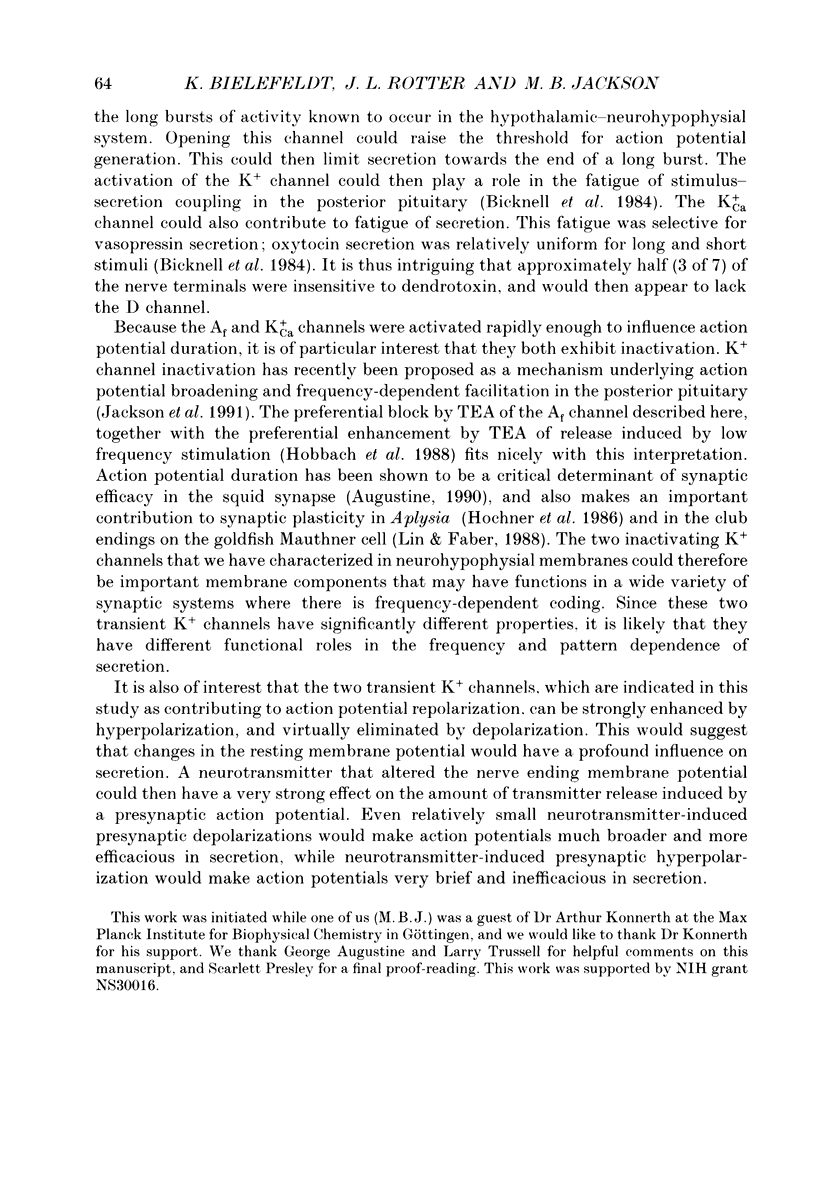
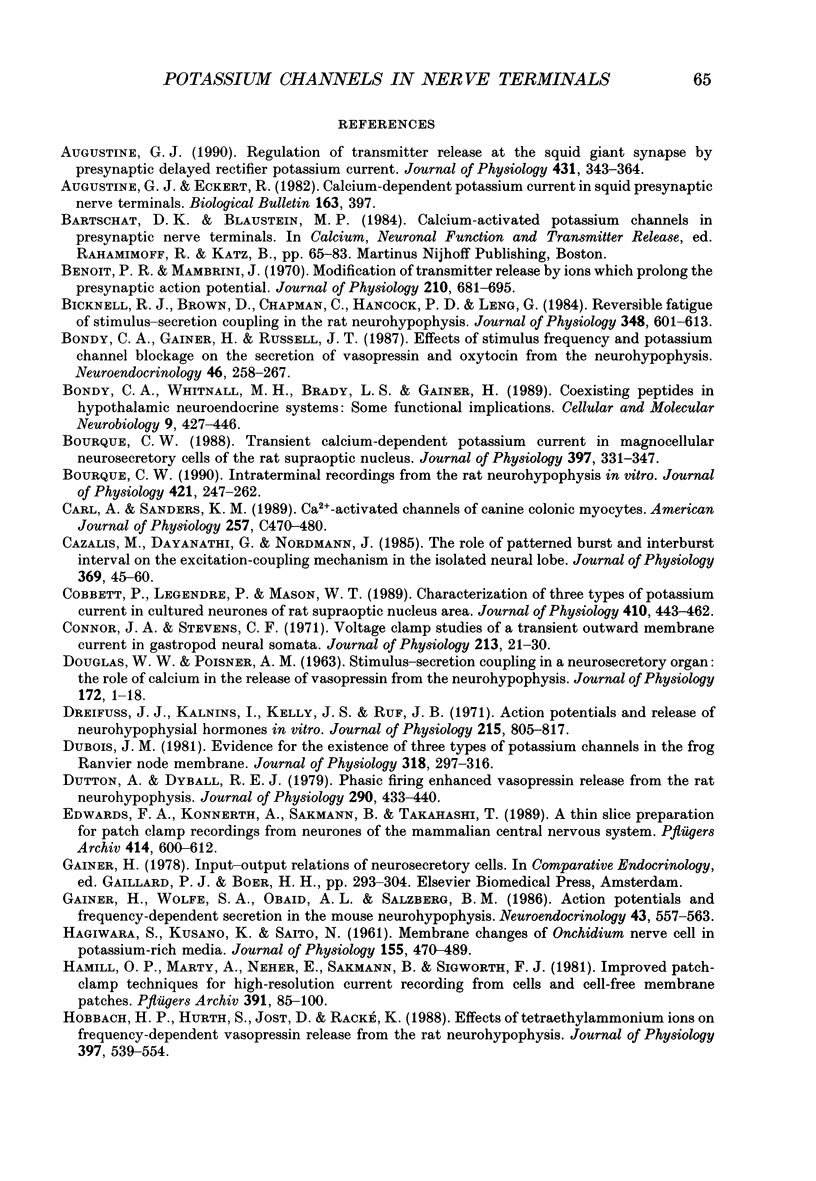
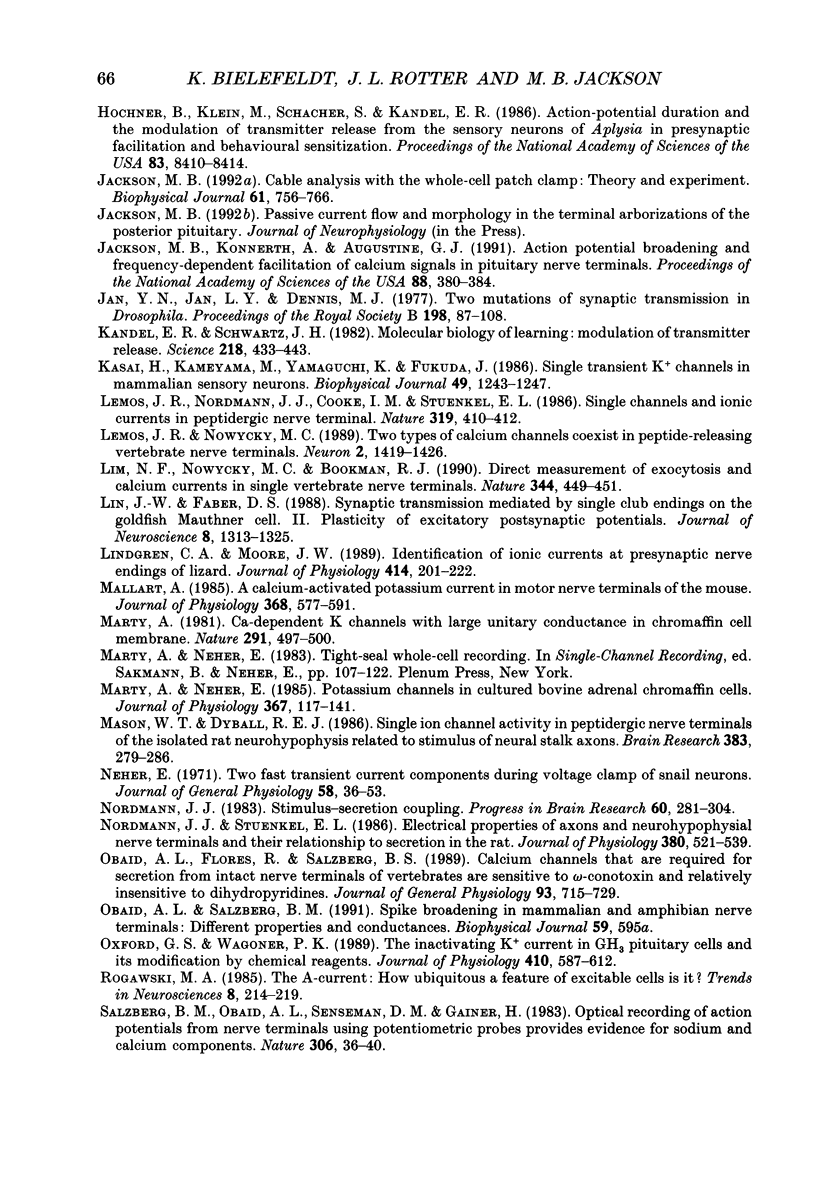
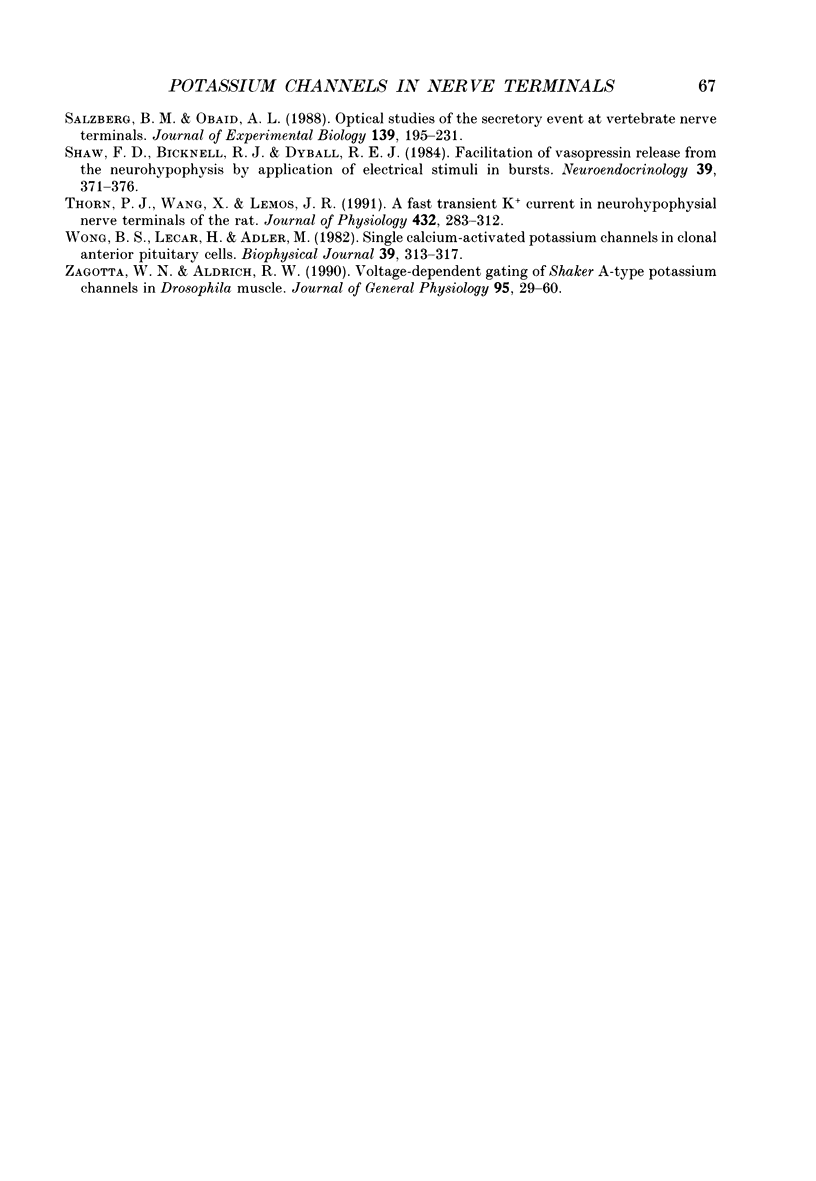
Selected References
These references are in PubMed. This may not be the complete list of references from this article.
- Augustine G. J. Regulation of transmitter release at the squid giant synapse by presynaptic delayed rectifier potassium current. J Physiol. 1990 Dec;431:343–364. doi: 10.1113/jphysiol.1990.sp018333. [DOI] [PMC free article] [PubMed] [Google Scholar]
- Benoit P. R., Mambrini J. Modification of transmitter release by ions which prolong the presynaptic action potential. J Physiol. 1970 Oct;210(3):681–695. doi: 10.1113/jphysiol.1970.sp009235. [DOI] [PMC free article] [PubMed] [Google Scholar]
- Bicknell R. J., Brown D., Chapman C., Hancock P. D., Leng G. Reversible fatigue of stimulus-secretion coupling in the rat neurohypophysis. J Physiol. 1984 Mar;348:601–613. doi: 10.1113/jphysiol.1984.sp015128. [DOI] [PMC free article] [PubMed] [Google Scholar]
- Bondy C. A., Gainer H., Russell J. T. Effects of stimulus frequency and potassium channel blockade on the secretion of vasopressin and oxytocin from the neurohypophysis. Neuroendocrinology. 1987 Sep;46(3):258–267. doi: 10.1159/000124829. [DOI] [PubMed] [Google Scholar]
- Bondy C. A., Whitnall M. H., Brady L. S., Gainer H. Coexisting peptides in hypothalamic neuroendocrine systems: some functional implications. Cell Mol Neurobiol. 1989 Dec;9(4):427–446. doi: 10.1007/BF00712791. [DOI] [PubMed] [Google Scholar]
- Bourque C. W. Intraterminal recordings from the rat neurohypophysis in vitro. J Physiol. 1990 Feb;421:247–262. doi: 10.1113/jphysiol.1990.sp017943. [DOI] [PMC free article] [PubMed] [Google Scholar]
- Bourque C. W. Transient calcium-dependent potassium current in magnocellular neurosecretory cells of the rat supraoptic nucleus. J Physiol. 1988 Mar;397:331–347. doi: 10.1113/jphysiol.1988.sp017004. [DOI] [PMC free article] [PubMed] [Google Scholar]
- Carl A., Sanders K. M. Ca2+-activated K channels of canine colonic myocytes. Am J Physiol. 1989 Sep;257(3 Pt 1):C470–C480. doi: 10.1152/ajpcell.1989.257.3.C470. [DOI] [PubMed] [Google Scholar]
- Cazalis M., Dayanithi G., Nordmann J. J. The role of patterned burst and interburst interval on the excitation-coupling mechanism in the isolated rat neural lobe. J Physiol. 1985 Dec;369:45–60. doi: 10.1113/jphysiol.1985.sp015887. [DOI] [PMC free article] [PubMed] [Google Scholar]
- Cleemann L., Morad M. Role of Ca2+ channel in cardiac excitation-contraction coupling in the rat: evidence from Ca2+ transients and contraction. J Physiol. 1991 Jan;432:283–312. doi: 10.1113/jphysiol.1991.sp018385. [DOI] [PMC free article] [PubMed] [Google Scholar]
- Cobbett P., Legendre P., Mason W. T. Characterization of three types of potassium current in cultured neurones of rat supraoptic nucleus area. J Physiol. 1989 Mar;410:443–462. doi: 10.1113/jphysiol.1989.sp017543. [DOI] [PMC free article] [PubMed] [Google Scholar]
- Connor J. A., Stevens C. F. Voltage clamp studies of a transient outward membrane current in gastropod neural somata. J Physiol. 1971 Feb;213(1):21–30. doi: 10.1113/jphysiol.1971.sp009365. [DOI] [PMC free article] [PubMed] [Google Scholar]
- DOUGLAS W. W., POISNER A. M. STIMULUS-SECRETION COUPLING IN A NEUROSECRETORY ORGAN: THE ROLE OF CALCIUM IN THE RELEASE OF VASOPRESSIN FROM THE NEUROHYPOPHYSIS. J Physiol. 1964 Jul;172:1–18. doi: 10.1113/jphysiol.1964.sp007399. [DOI] [PMC free article] [PubMed] [Google Scholar]
- Dreifuss J. J., Kalnins I., Kelly J. S., Ruf K. B. Action potentials and release of neurohypophysial hormones in vitro. J Physiol. 1971 Jul;215(3):805–817. doi: 10.1113/jphysiol.1971.sp009499. [DOI] [PMC free article] [PubMed] [Google Scholar]
- Dubois J. M. Evidence for the existence of three types of potassium channels in the frog Ranvier node membrane. J Physiol. 1981 Sep;318:297–316. doi: 10.1113/jphysiol.1981.sp013865. [DOI] [PMC free article] [PubMed] [Google Scholar]
- Dutton A., Dyball R. E. Phasic firing enhances vasopressin release from the rat neurohypophysis. J Physiol. 1979 May;290(2):433–440. doi: 10.1113/jphysiol.1979.sp012781. [DOI] [PMC free article] [PubMed] [Google Scholar]
- Edwards F. A., Konnerth A., Sakmann B., Takahashi T. A thin slice preparation for patch clamp recordings from neurones of the mammalian central nervous system. Pflugers Arch. 1989 Sep;414(5):600–612. doi: 10.1007/BF00580998. [DOI] [PubMed] [Google Scholar]
- Gainer H., Wolfe S. A., Jr, Obaid A. L., Salzberg B. M. Action potentials and frequency-dependent secretion in the mouse neurohypophysis. Neuroendocrinology. 1986;43(5):557–563. doi: 10.1159/000124582. [DOI] [PubMed] [Google Scholar]
- HAGIWARA S., KUSANO K., SAITO N. Membrane changes of Onchidium nerve cell in potassium-rich media. J Physiol. 1961 Mar;155:470–489. doi: 10.1113/jphysiol.1961.sp006640. [DOI] [PMC free article] [PubMed] [Google Scholar]
- Hamill O. P., Marty A., Neher E., Sakmann B., Sigworth F. J. Improved patch-clamp techniques for high-resolution current recording from cells and cell-free membrane patches. Pflugers Arch. 1981 Aug;391(2):85–100. doi: 10.1007/BF00656997. [DOI] [PubMed] [Google Scholar]
- Hobbach H. P., Hurth S., Jost D., Racké K. Effects of tetraethylammonium ions on frequency-dependent vasopressin release from the rat neurohypophysis. J Physiol. 1988 Mar;397:539–554. doi: 10.1113/jphysiol.1988.sp017018. [DOI] [PMC free article] [PubMed] [Google Scholar]
- Hochner B., Klein M., Schacher S., Kandel E. R. Action-potential duration and the modulation of transmitter release from the sensory neurons of Aplysia in presynaptic facilitation and behavioral sensitization. Proc Natl Acad Sci U S A. 1986 Nov;83(21):8410–8414. doi: 10.1073/pnas.83.21.8410. [DOI] [PMC free article] [PubMed] [Google Scholar]
- Jackson M. B. Cable analysis with the whole-cell patch clamp. Theory and experiment. Biophys J. 1992 Mar;61(3):756–766. doi: 10.1016/S0006-3495(92)81880-5. [DOI] [PMC free article] [PubMed] [Google Scholar]
- Jackson M. B., Konnerth A., Augustine G. J. Action potential broadening and frequency-dependent facilitation of calcium signals in pituitary nerve terminals. Proc Natl Acad Sci U S A. 1991 Jan 15;88(2):380–384. doi: 10.1073/pnas.88.2.380. [DOI] [PMC free article] [PubMed] [Google Scholar]
- Jan Y. N., Jan L. Y., Dennis M. J. Two mutations of synaptic transmission in Drosophila. Proc R Soc Lond B Biol Sci. 1977 Jul 28;198(1130):87–108. doi: 10.1098/rspb.1977.0087. [DOI] [PubMed] [Google Scholar]
- Kandel E. R., Schwartz J. H. Molecular biology of learning: modulation of transmitter release. Science. 1982 Oct 29;218(4571):433–443. doi: 10.1126/science.6289442. [DOI] [PubMed] [Google Scholar]
- Kasai H., Kameyama M., Yamaguchi K., Fukuda J. Single transient K channels in mammalian sensory neurons. Biophys J. 1986 Jun;49(6):1243–1247. doi: 10.1016/S0006-3495(86)83754-7. [DOI] [PMC free article] [PubMed] [Google Scholar]
- Lemos J. R., Nordmann J. J., Cooke I. M., Stuenkel E. L. Single channels and ionic currents in peptidergic nerve terminals. 1986 Jan 30-Feb 5Nature. 319(6052):410–412. doi: 10.1038/319410a0. [DOI] [PubMed] [Google Scholar]
- Lemos J. R., Nowycky M. C. Two types of calcium channels coexist in peptide-releasing vertebrate nerve terminals. Neuron. 1989 May;2(5):1419–1426. doi: 10.1016/0896-6273(89)90187-6. [DOI] [PubMed] [Google Scholar]
- Lim N. F., Nowycky M. C., Bookman R. J. Direct measurement of exocytosis and calcium currents in single vertebrate nerve terminals. Nature. 1990 Mar 29;344(6265):449–451. doi: 10.1038/344449a0. [DOI] [PubMed] [Google Scholar]
- Lin J. W., Faber D. S. Synaptic transmission mediated by single club endings on the goldfish Mauthner cell. II. Plasticity of excitatory postsynaptic potentials. J Neurosci. 1988 Apr;8(4):1313–1325. doi: 10.1523/JNEUROSCI.08-04-01313.1988. [DOI] [PMC free article] [PubMed] [Google Scholar]
- Lindgren C. A., Moore J. W. Identification of ionic currents at presynaptic nerve endings of the lizard. J Physiol. 1989 Jul;414:201–222. doi: 10.1113/jphysiol.1989.sp017684. [DOI] [PMC free article] [PubMed] [Google Scholar]
- Mallart A. A calcium-activated potassium current in motor nerve terminals of the mouse. J Physiol. 1985 Nov;368:577–591. doi: 10.1113/jphysiol.1985.sp015877. [DOI] [PMC free article] [PubMed] [Google Scholar]
- Marty A. Ca-dependent K channels with large unitary conductance in chromaffin cell membranes. Nature. 1981 Jun 11;291(5815):497–500. doi: 10.1038/291497a0. [DOI] [PubMed] [Google Scholar]
- Marty A., Neher E. Potassium channels in cultured bovine adrenal chromaffin cells. J Physiol. 1985 Oct;367:117–141. doi: 10.1113/jphysiol.1985.sp015817. [DOI] [PMC free article] [PubMed] [Google Scholar]
- Mason W. T., Dyball R. E. Single ion channel activity in peptidergic nerve terminals of the isolated rat neurohypophysis related to stimulation of neural stalk axons. Brain Res. 1986 Sep 24;383(1-2):279–286. doi: 10.1016/0006-8993(86)90026-0. [DOI] [PubMed] [Google Scholar]
- Neher E. Two fast transient current components during voltage clamp on snail neurons. J Gen Physiol. 1971 Jul;58(1):36–53. doi: 10.1085/jgp.58.1.36. [DOI] [PMC free article] [PubMed] [Google Scholar]
- Nordmann J. J. Stimulus-secretion coupling. Prog Brain Res. 1983;60:281–304. doi: 10.1016/S0079-6123(08)64397-6. [DOI] [PubMed] [Google Scholar]
- Nordmann J. J., Stuenkel E. L. Electrical properties of axons and neurohypophysial nerve terminals and their relationship to secretion in the rat. J Physiol. 1986 Nov;380:521–539. doi: 10.1113/jphysiol.1986.sp016300. [DOI] [PMC free article] [PubMed] [Google Scholar]
- Obaid A. L., Flores R., Salzberg B. M. Calcium channels that are required for secretion from intact nerve terminals of vertebrates are sensitive to omega-conotoxin and relatively insensitive to dihydropyridines. Optical studies with and without voltage-sensitive dyes. J Gen Physiol. 1989 Apr;93(4):715–729. doi: 10.1085/jgp.93.4.715. [DOI] [PMC free article] [PubMed] [Google Scholar]
- Oxford G. S., Wagoner P. K. The inactivating K+ current in GH3 pituitary cells and its modification by chemical reagents. J Physiol. 1989 Mar;410:587–612. doi: 10.1113/jphysiol.1989.sp017550. [DOI] [PMC free article] [PubMed] [Google Scholar]
- Salzberg B. M., Obaid A. L. Optical studies of the secretory event at vertebrate nerve terminals. J Exp Biol. 1988 Sep;139:195–231. doi: 10.1242/jeb.139.1.195. [DOI] [PubMed] [Google Scholar]
- Salzberg B. M., Obaid A. L., Senseman D. M., Gainer H. Optical recording of action potentials from vertebrate nerve terminals using potentiometric probes provides evidence for sodium and calcium components. Nature. 1983 Nov 3;306(5938):36–40. doi: 10.1038/306036a0. [DOI] [PubMed] [Google Scholar]
- Shaw F. D., Bicknell R. J., Dyball R. E. Facilitation of vasopressin release from the neurohypophysis by application of electrical stimuli in bursts. Relevant stimulation parameters. Neuroendocrinology. 1984 Oct;39(4):371–376. doi: 10.1159/000124007. [DOI] [PubMed] [Google Scholar]
- Wong B. S., Lecar H., Adler M. Single calcium-dependent potassium channels in clonal anterior pituitary cells. Biophys J. 1982 Sep;39(3):313–317. doi: 10.1016/S0006-3495(82)84522-0. [DOI] [PMC free article] [PubMed] [Google Scholar]
- Zagotta W. N., Aldrich R. W. Voltage-dependent gating of Shaker A-type potassium channels in Drosophila muscle. J Gen Physiol. 1990 Jan;95(1):29–60. doi: 10.1085/jgp.95.1.29. [DOI] [PMC free article] [PubMed] [Google Scholar]