Abstract
1. An inward current carried by Ca2+ was recorded from single smooth muscle cells of rabbit oesophageal muscularis mucosae using a whole-cell gigaseal technique with physiological (2 mM) external calcium concentration ([Ca2+]o) in the presence of intracellular Cs+ ([Cs+]i 130 mM). Only one type of Ca2+ current could be identified. The threshold for its activation was approximately -30 mV and maximum inward current (approximately 300 pA) was recorded at 0 mV. 2. This inward current was blocked by Co2+ (4 mM), Cd2+ (0.5 mM) and nifedipine (1 microM) and was enhanced by Bay K 8644 (5 microM). We therefore classify it as a L-type Ca2+ current and denote it ICa. 3. Steady-state inactivation data were well-fitted by a Boltzmann distribution, indicating that inactivation of the Ca2+ current is strongly modulated by membrane potential. However, the inactivation of ICa slowed significantly and became less complete when BaCl2 replaced CaCl2 in the Tyrode solution suggesting that the inactivation of ICa may also be dependent on [Ca2+]i. The steady-state activation and inactivation curves for ICa overlap between -40 and 0 mV indicating that there may be a Ca2+ window current in this range of potentials. 4. When EGTA was omitted from the pipette-filling solution, depolarizations positive to -10 mV resulted in a transient as opposed to a maintained inward Ca2+ current which was followed by a relatively large outward current. Under these conditions, slowly decaying inward tail currents were also recorded upon repolarization to the holding potential, -60 mV. However, when EGTA was omitted from the pipette, marked 'run-down' of the Ca2+ current occurred within 10 min after starting the whole-cell recording. 5. This run-down of ICa was reduced significantly when the nystatin perforated patch technique was used. Under these conditions stable ICa records could be obtained for over 1 h. Outward currents and slow decaying inward tail currents similar to those recorded with no EGTA in the pipette were also obtained consistently using the nystatin recording technique. 6. In nystatin perforated patch recordings, CoCl2 (2 mM) completely abolished the Ca2+ current, the outward currents and the slow inward tails. These findings suggest that the outward currents and slow inward tails are activated by a transmembrane influx of Ca2+. 7. Ion replacement and pharmacological tests provided evidence that both the outward currents and the slow inward tails are due to Ca(2+)-activated Cl- current (ICl(Ca)).(ABSTRACT TRUNCATED AT 400 WORDS)
Full text
PDF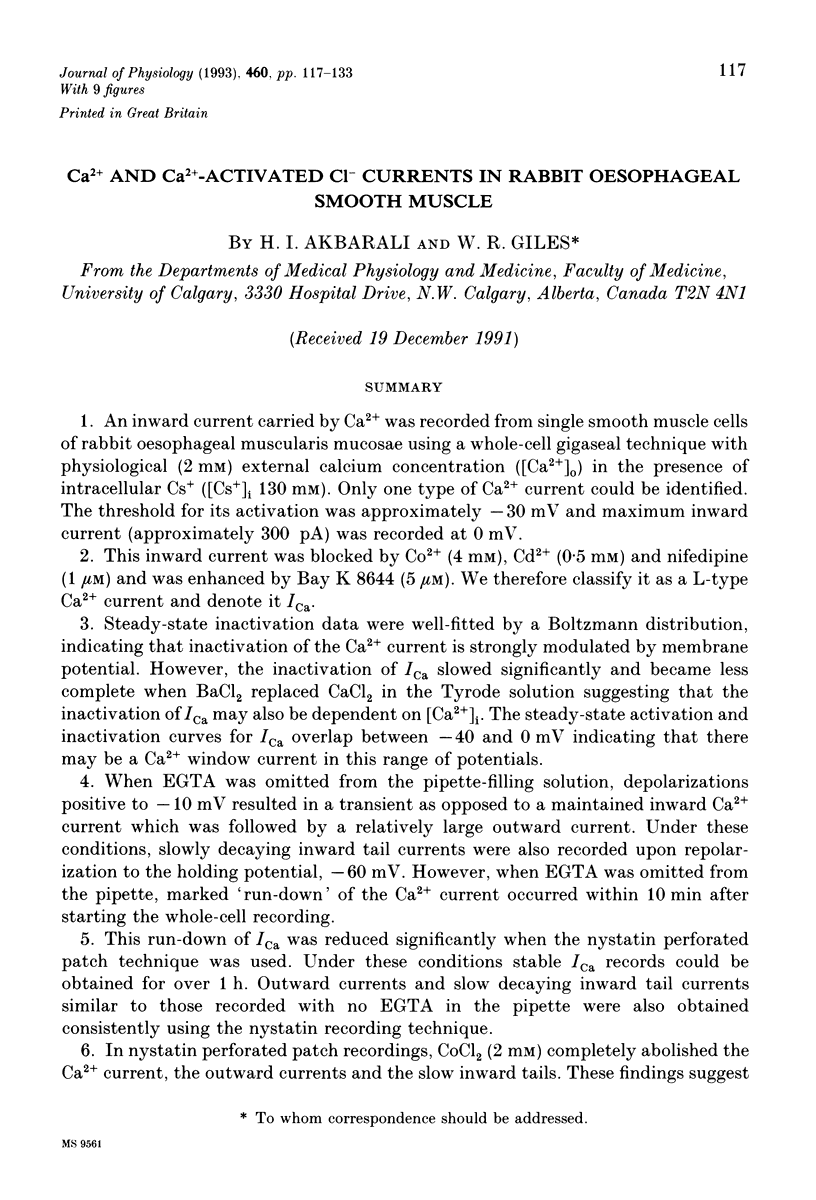
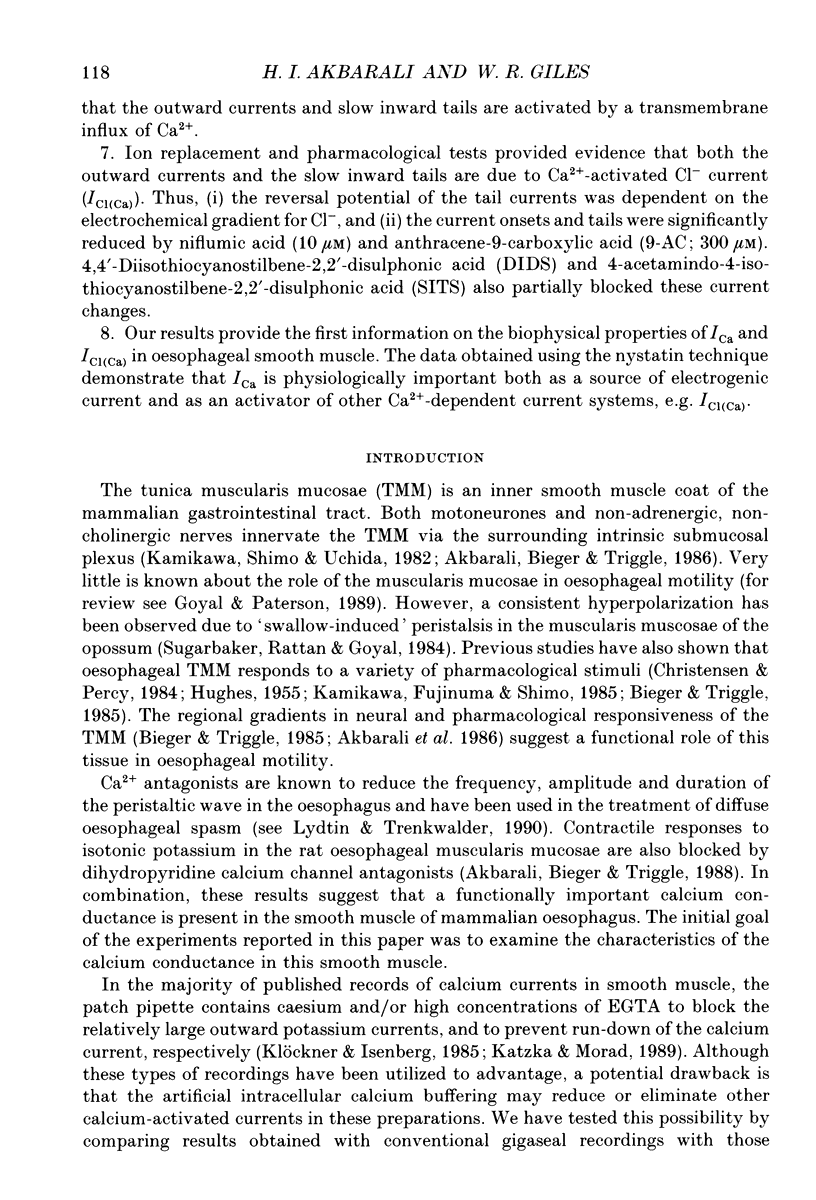
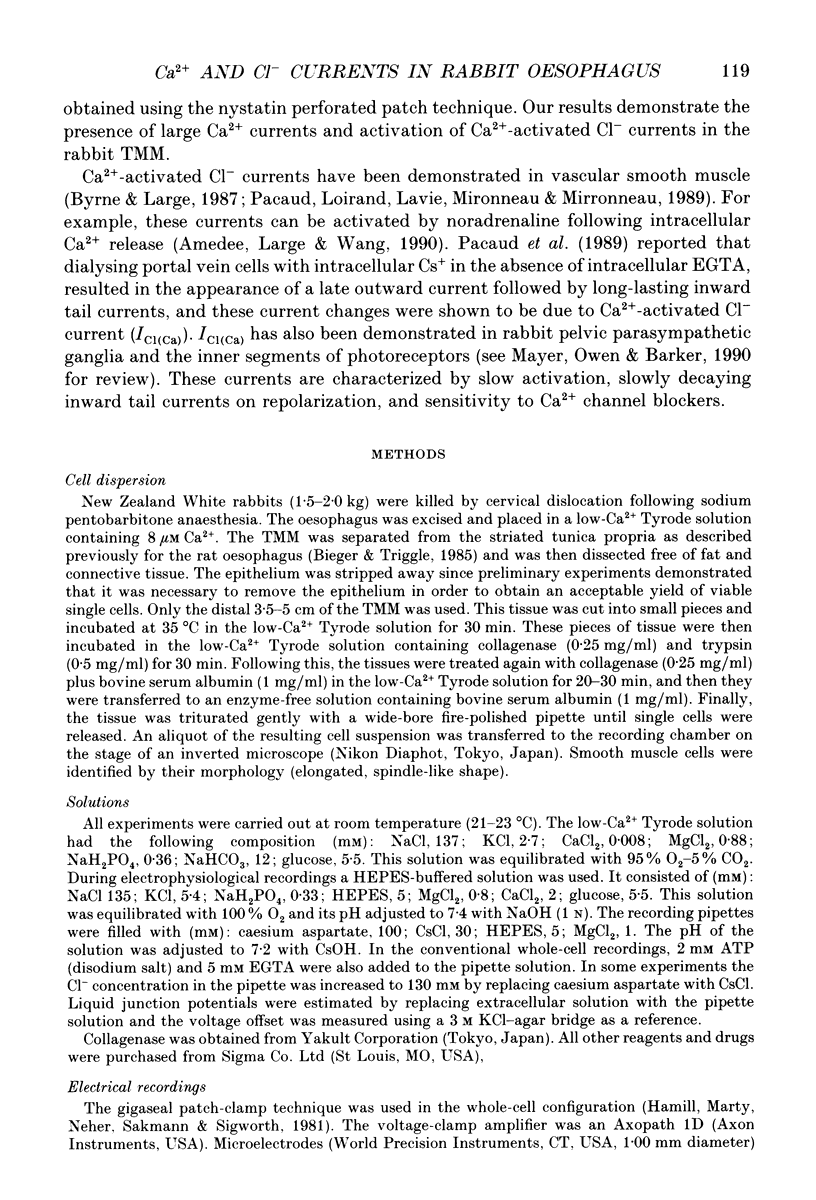
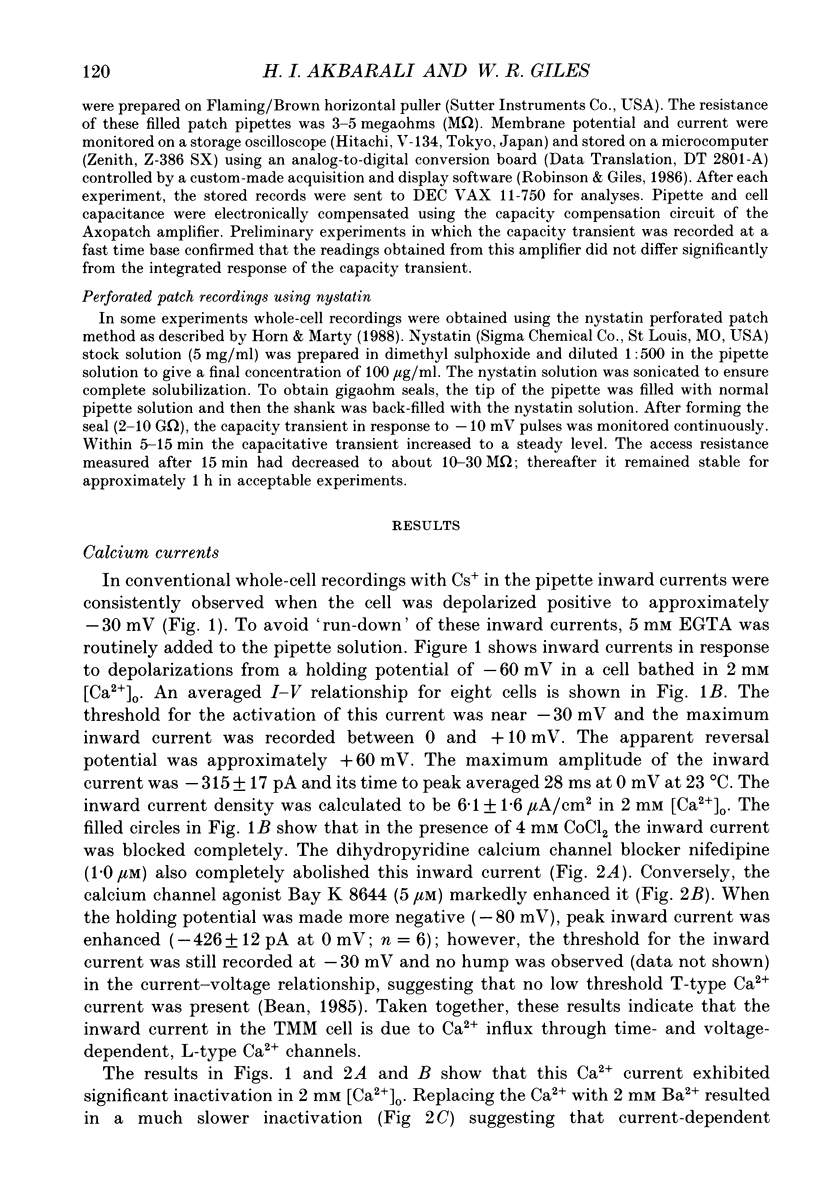
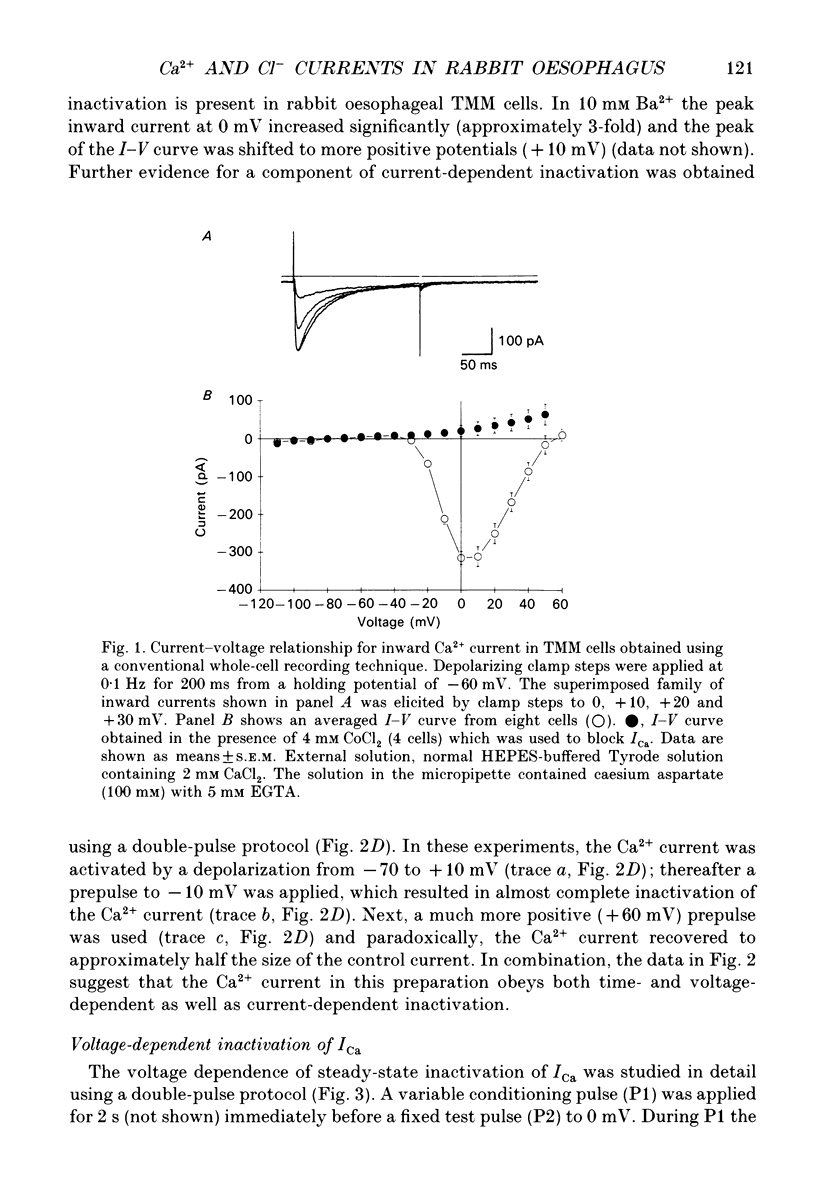
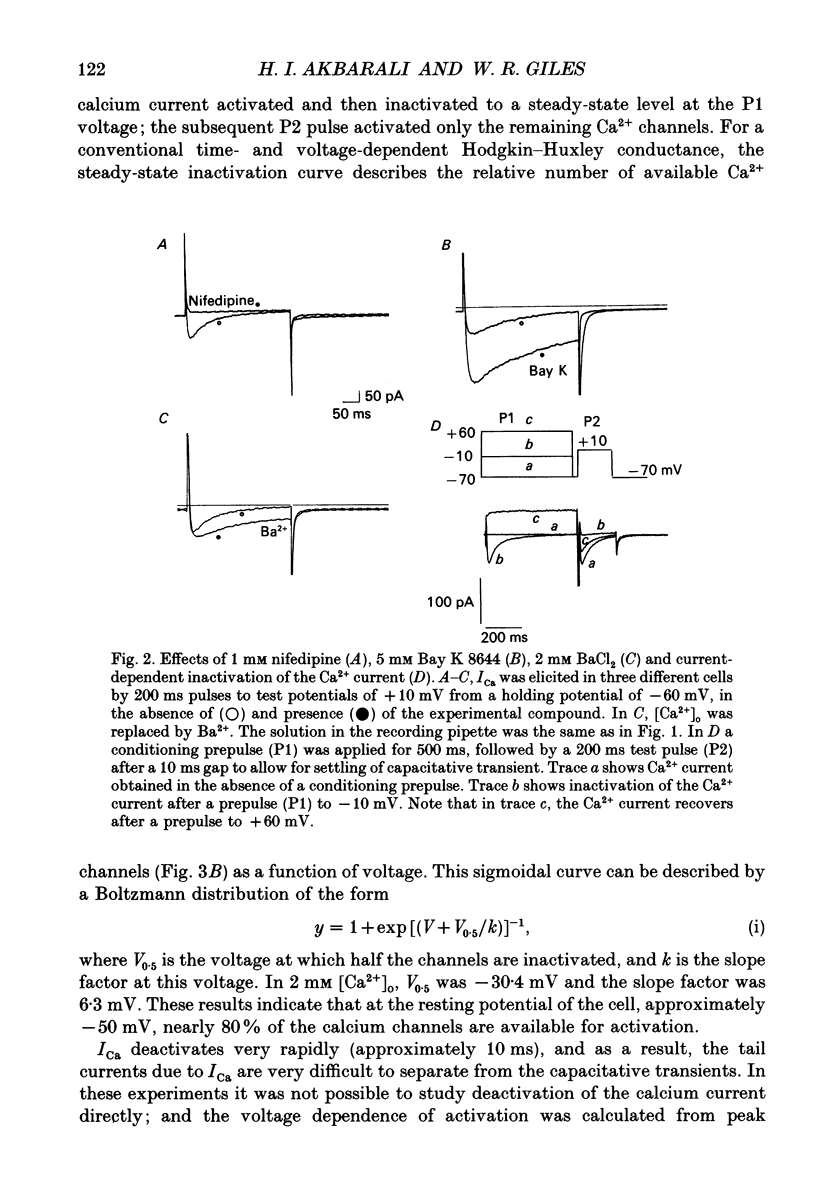
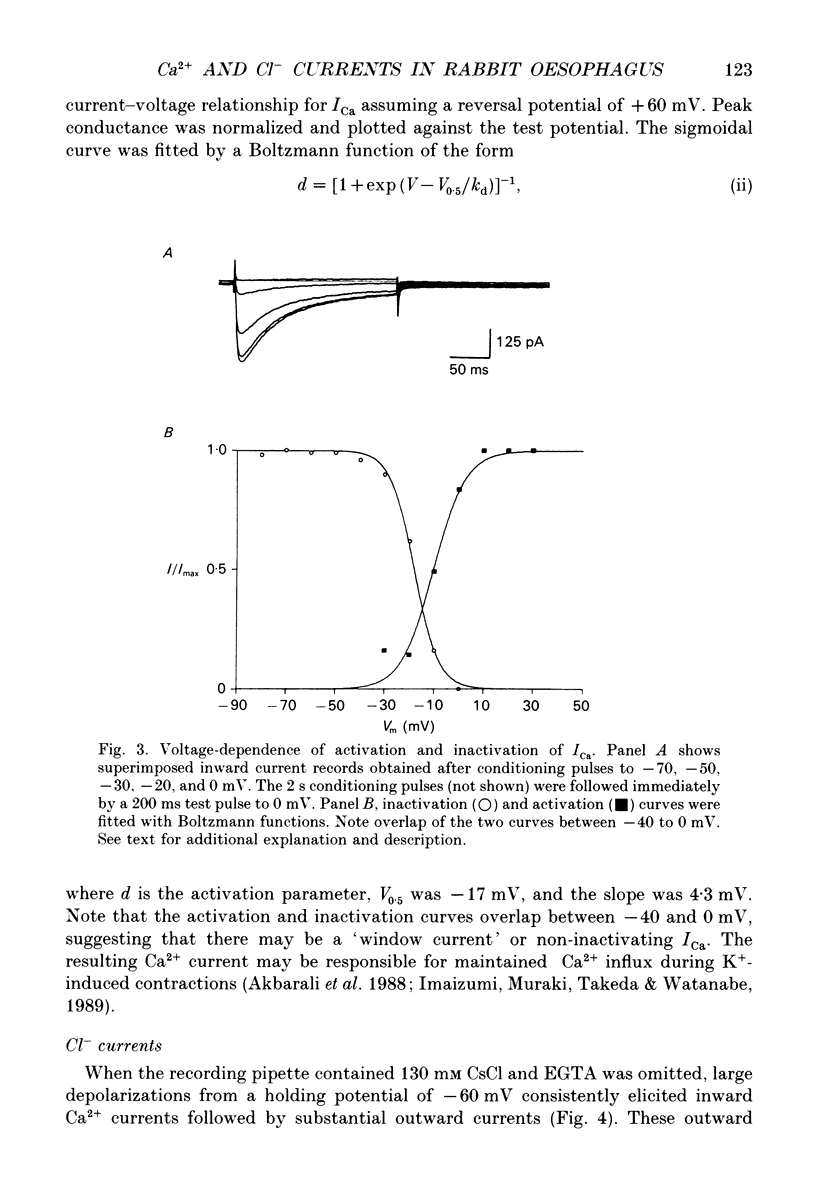
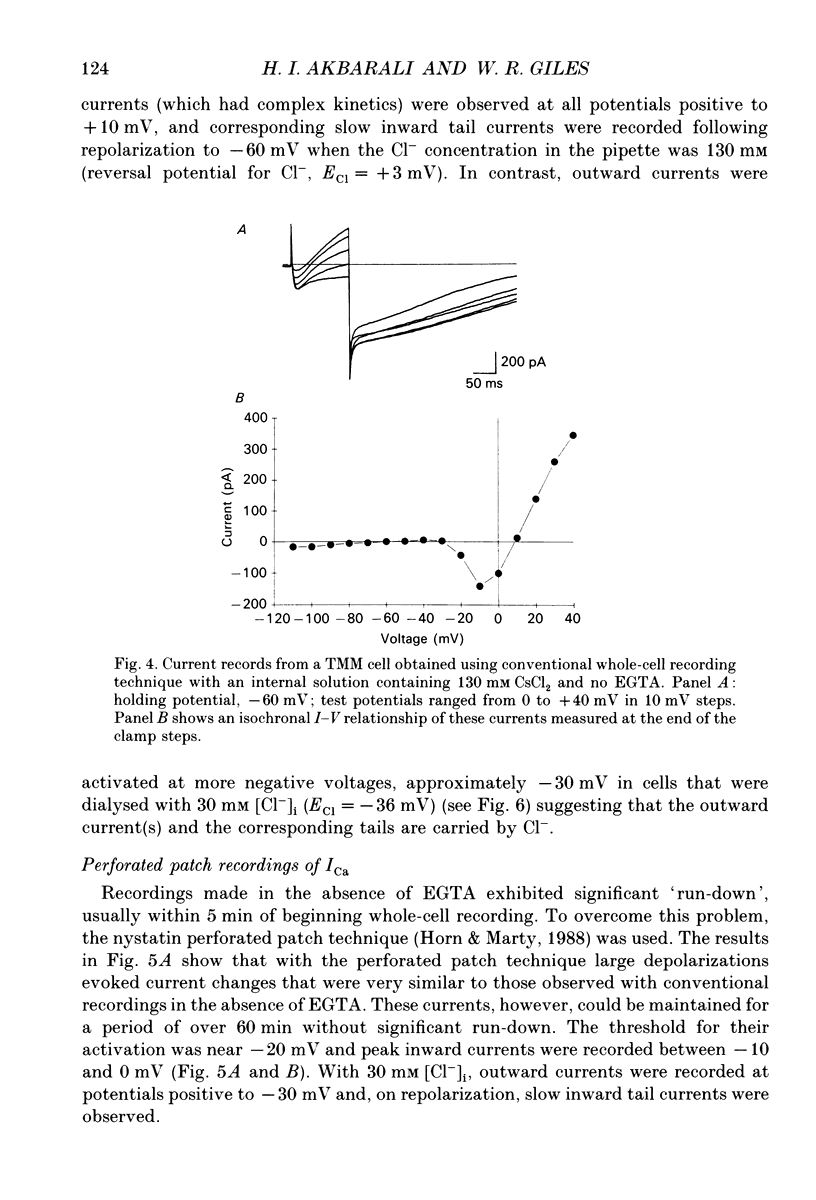
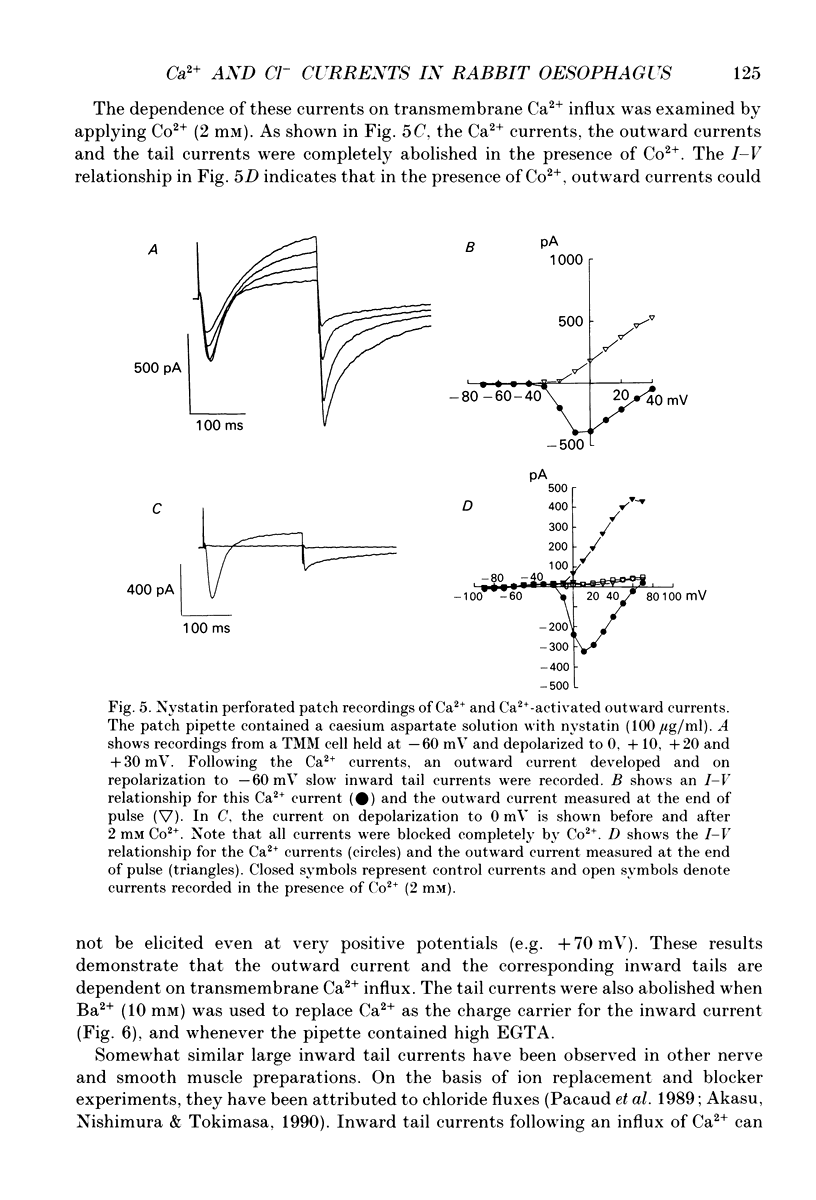
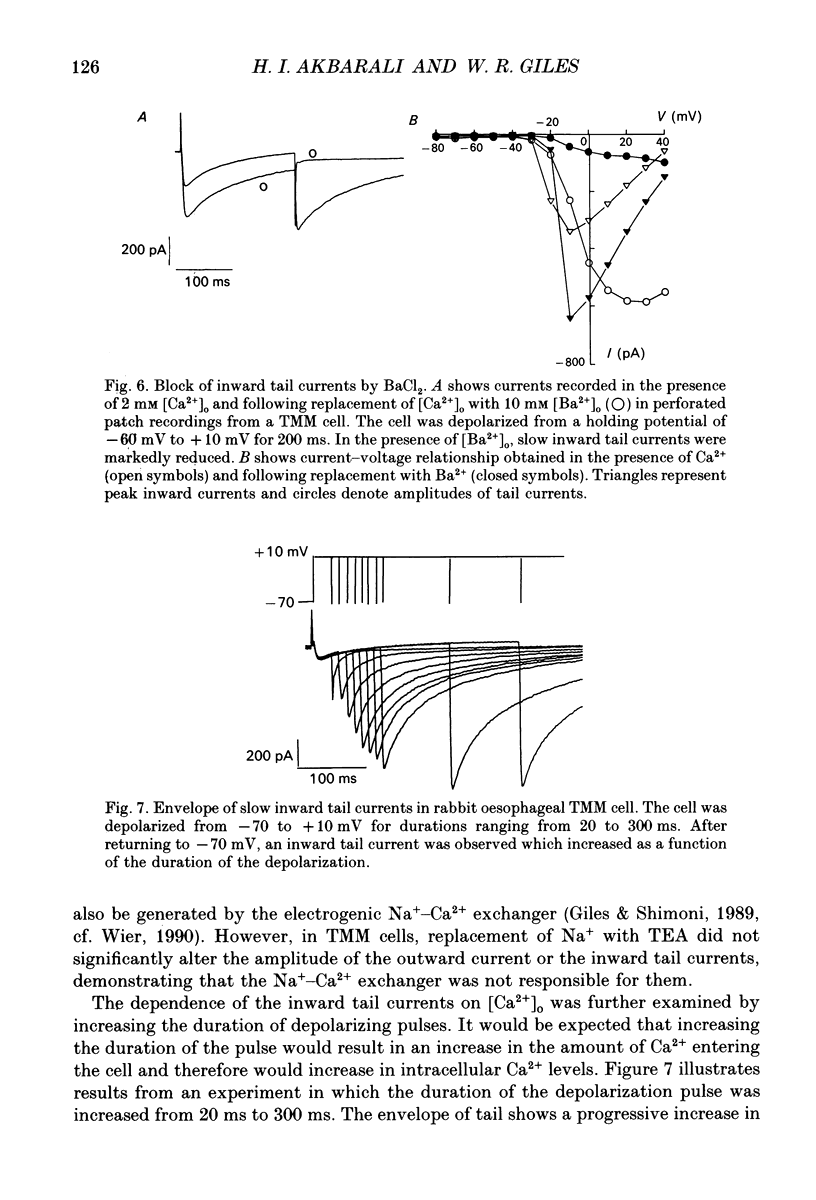
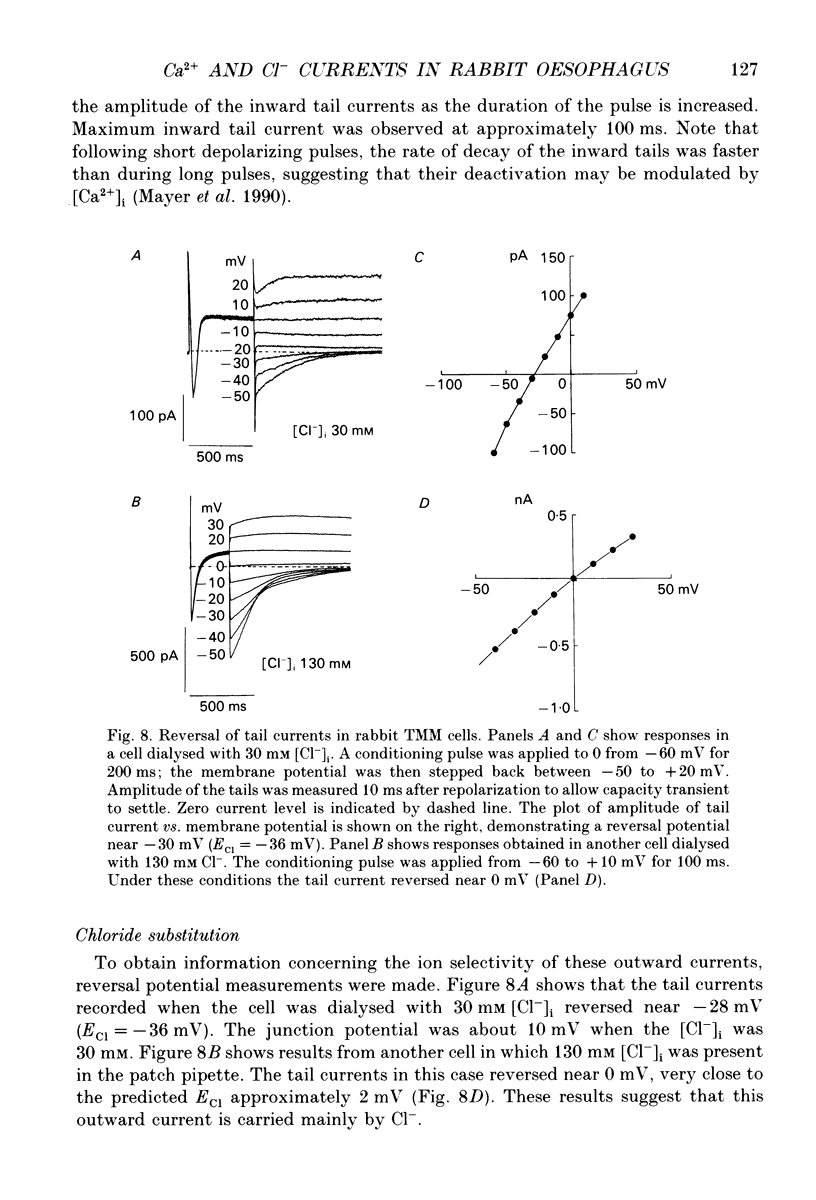
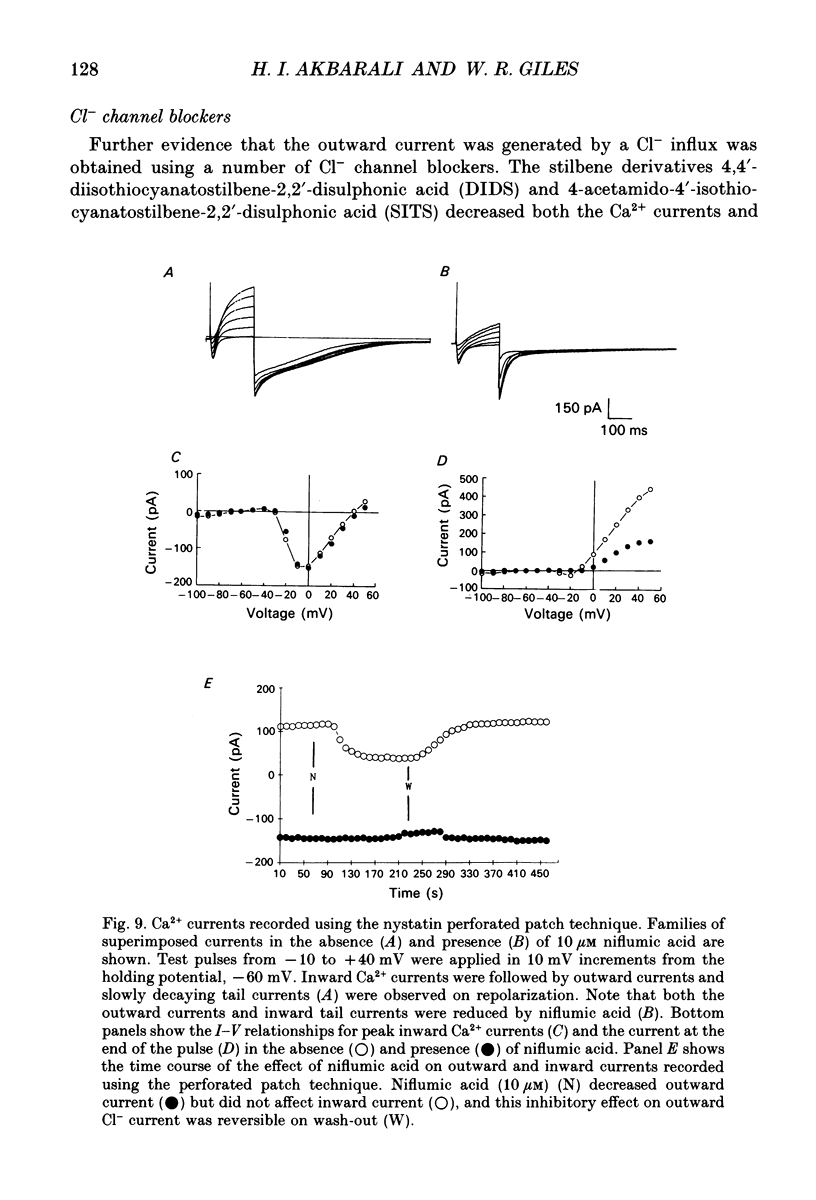
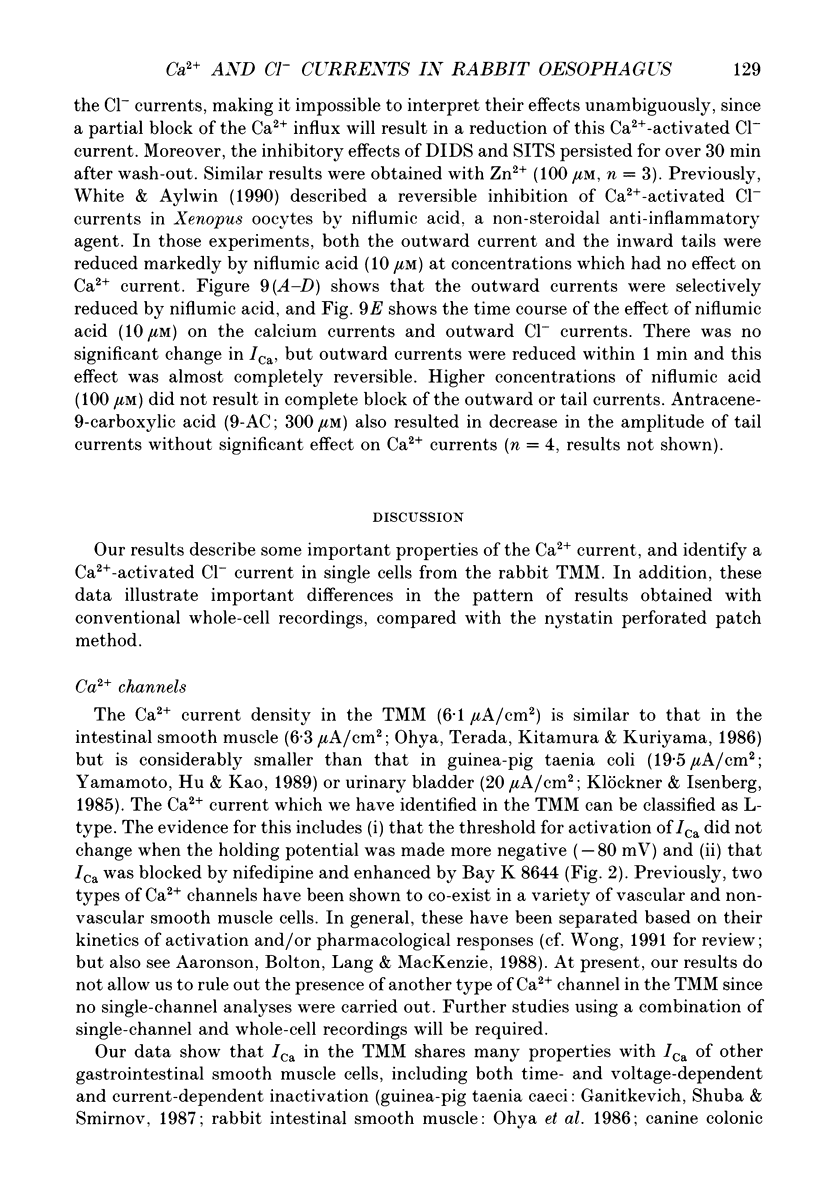
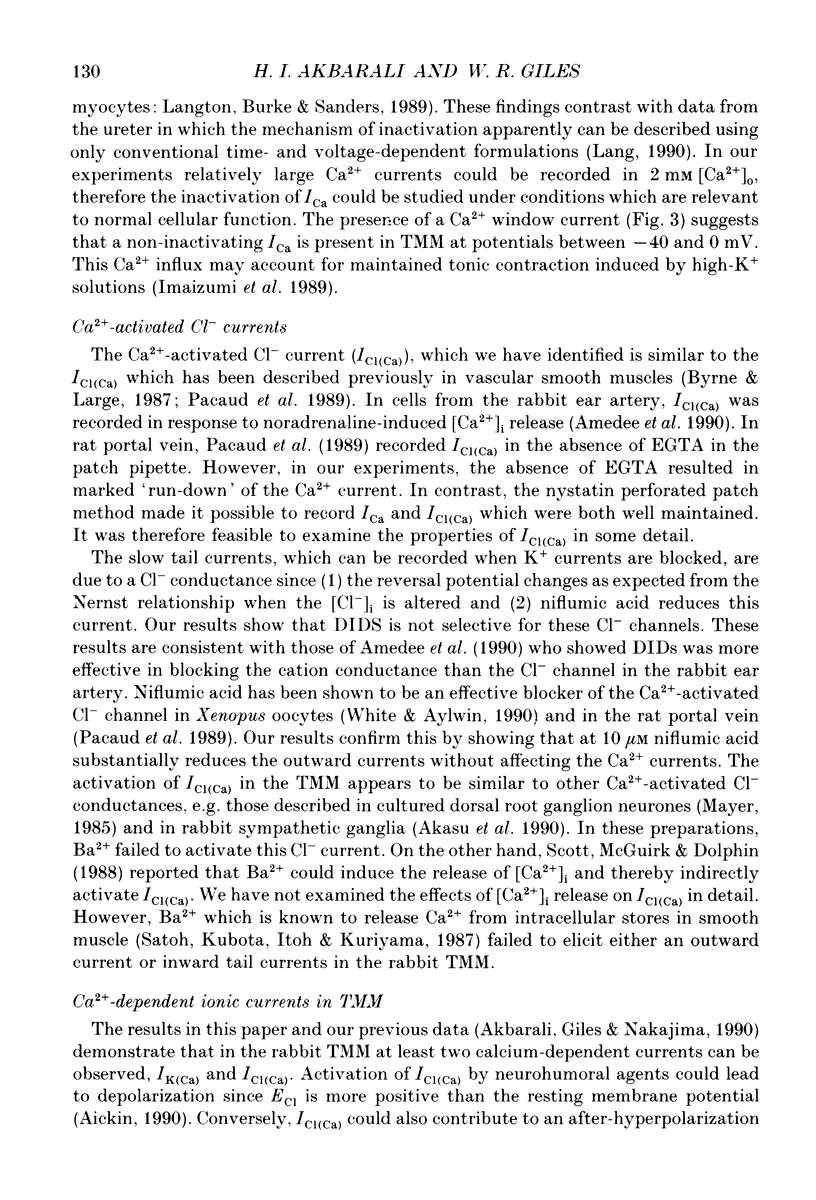
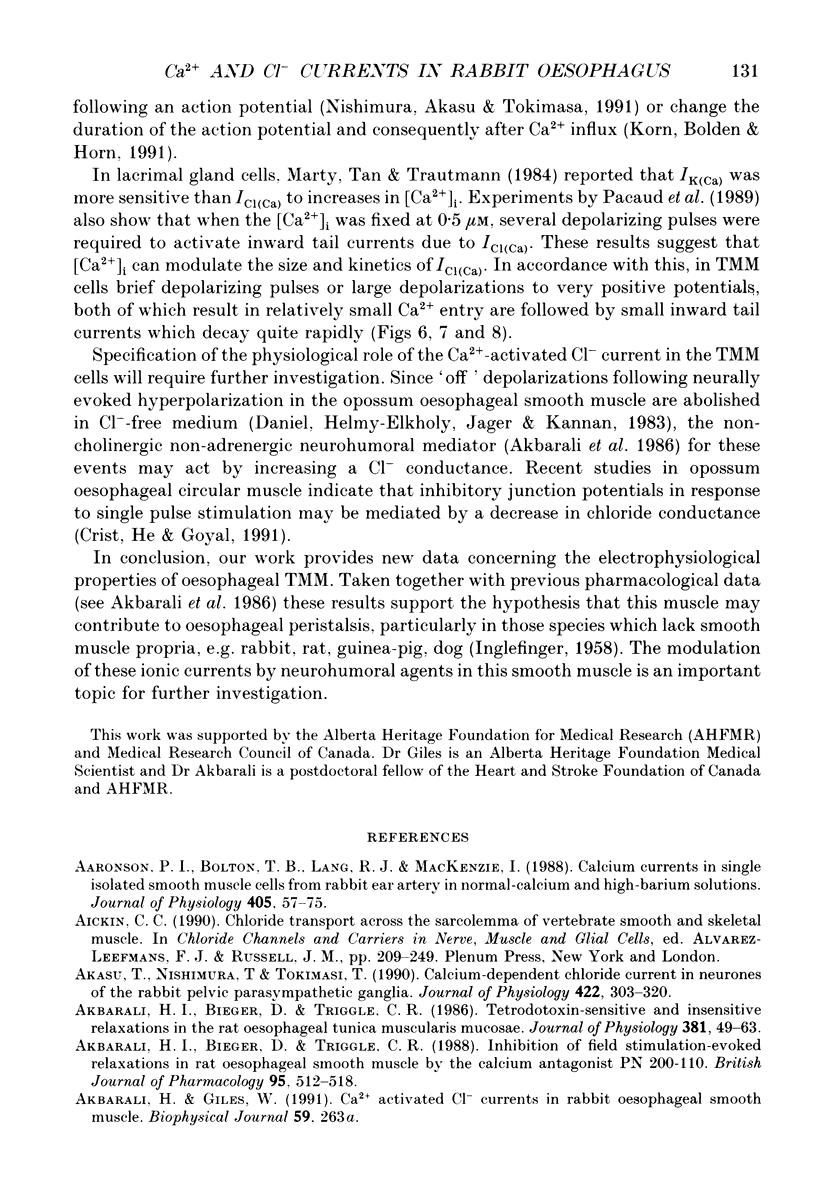
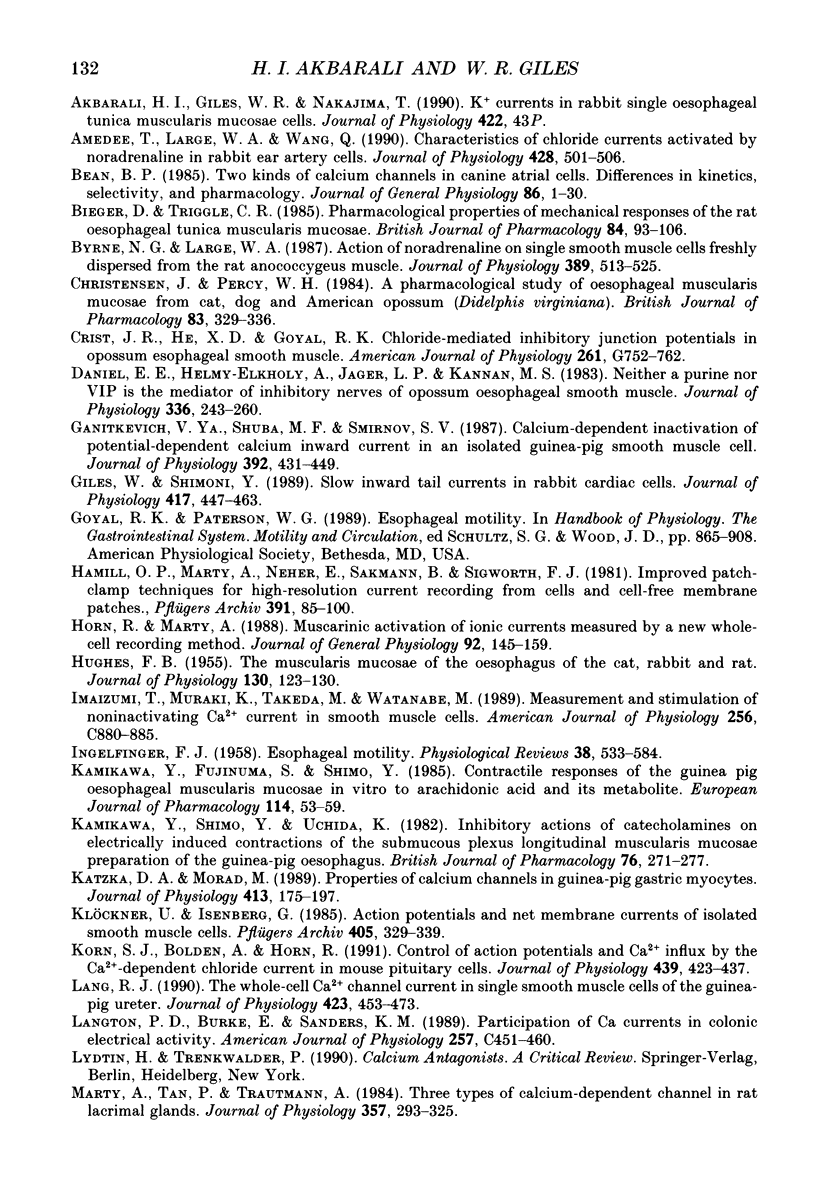
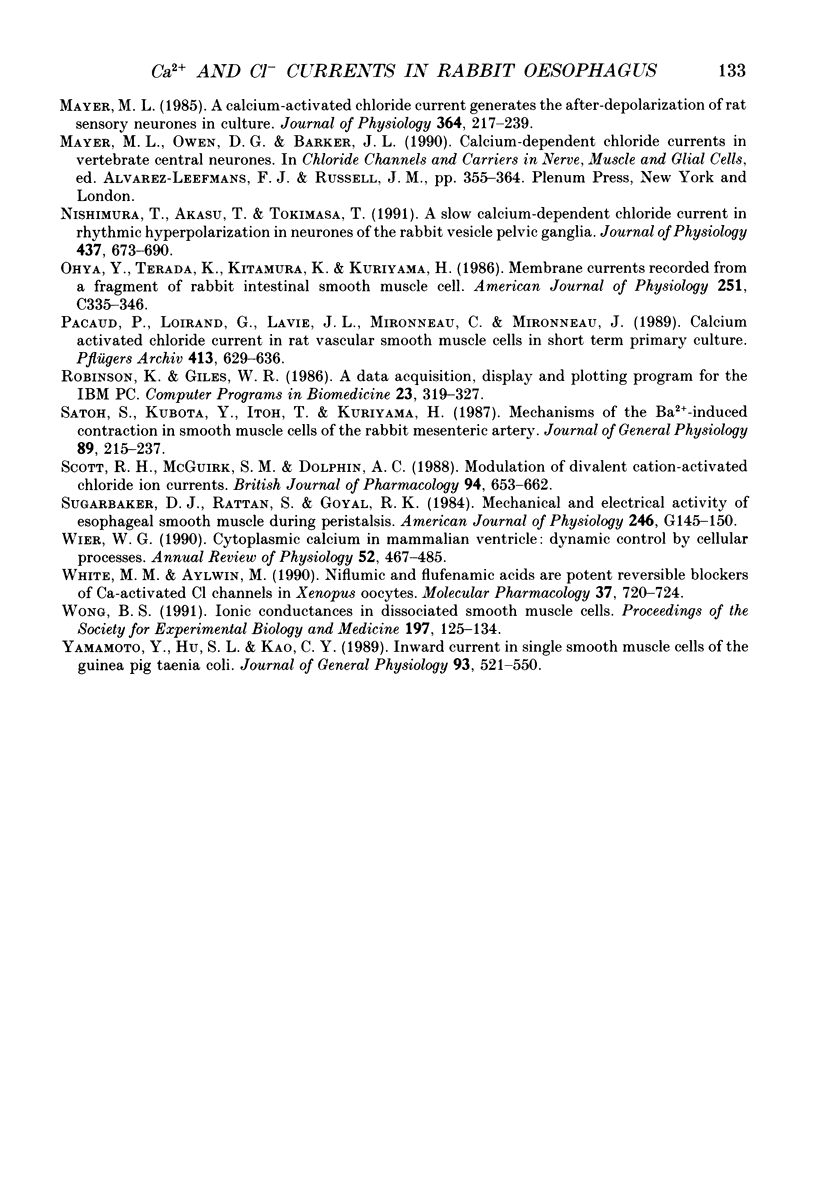
Selected References
These references are in PubMed. This may not be the complete list of references from this article.
- Aaronson P. I., Bolton T. B., Lang R. J., MacKenzie I. Calcium currents in single isolated smooth muscle cells from the rabbit ear artery in normal-calcium and high-barium solutions. J Physiol. 1988 Nov;405:57–75. doi: 10.1113/jphysiol.1988.sp017321. [DOI] [PMC free article] [PubMed] [Google Scholar]
- Akasu T., Nishimura T., Tokimasa T. Calcium-dependent chloride current in neurones of the rabbit pelvic parasympathetic ganglia. J Physiol. 1990 Mar;422:303–320. doi: 10.1113/jphysiol.1990.sp017985. [DOI] [PMC free article] [PubMed] [Google Scholar]
- Akbarali H. I., Bieger D., Triggle C. R. Inhibition of field stimulation-evoked relaxations in rat oesophageal smooth muscle by the calcium antagonist PN 200-110. Br J Pharmacol. 1988 Oct;95(2):512–518. doi: 10.1111/j.1476-5381.1988.tb11671.x. [DOI] [PMC free article] [PubMed] [Google Scholar]
- Akbarali H. I., Bieger D., Triggle C. R. Tetrodotoxin-sensitive and -insensitive relaxations in the rat oesophageal tunica muscularis mucosae. J Physiol. 1986 Dec;381:49–63. doi: 10.1113/jphysiol.1986.sp016312. [DOI] [PMC free article] [PubMed] [Google Scholar]
- Amédée T., Large W. A., Wang Q. Characteristics of chloride currents activated by noradrenaline in rabbit ear artery cells. J Physiol. 1990 Sep;428:501–516. doi: 10.1113/jphysiol.1990.sp018224. [DOI] [PMC free article] [PubMed] [Google Scholar]
- Bean B. P. Two kinds of calcium channels in canine atrial cells. Differences in kinetics, selectivity, and pharmacology. J Gen Physiol. 1985 Jul;86(1):1–30. doi: 10.1085/jgp.86.1.1. [DOI] [PMC free article] [PubMed] [Google Scholar]
- Bieger D., Triggle C. Pharmacological properties of mechanical responses of the rat oesophageal muscularis mucosae to vagal and field stimulation. Br J Pharmacol. 1985 Jan;84(1):93–106. [PMC free article] [PubMed] [Google Scholar]
- Byrne N. G., Large W. A. Action of noradrenaline on single smooth muscle cells freshly dispersed from the rat anococcygeus muscle. J Physiol. 1987 Aug;389:513–525. doi: 10.1113/jphysiol.1987.sp016669. [DOI] [PMC free article] [PubMed] [Google Scholar]
- Christensen J., Percy W. H. A pharmacological study of oesophageal muscularis mucosae from the cat, dog and American opossum (Didelphis virginiana). Br J Pharmacol. 1984 Oct;83(2):329–336. doi: 10.1111/j.1476-5381.1984.tb16492.x. [DOI] [PMC free article] [PubMed] [Google Scholar]
- Daniel E. E., Helmy-Elkholy A., Jager L. P., Kannan M. S. Neither a purine nor VIP is the mediator of inhibitory nerves of opossum oesophageal smooth muscle. J Physiol. 1983 Mar;336:243–260. doi: 10.1113/jphysiol.1983.sp014579. [DOI] [PMC free article] [PubMed] [Google Scholar]
- Ganitkevich VYa, Shuba M. F., Smirnov S. V. Calcium-dependent inactivation of potential-dependent calcium inward current in an isolated guinea-pig smooth muscle cell. J Physiol. 1987 Nov;392:431–449. doi: 10.1113/jphysiol.1987.sp016789. [DOI] [PMC free article] [PubMed] [Google Scholar]
- Giles W., Shimoni Y. Slow inward tail currents in rabbit cardiac cells. J Physiol. 1989 Oct;417:447–463. doi: 10.1113/jphysiol.1989.sp017812. [DOI] [PMC free article] [PubMed] [Google Scholar]
- HUGHES F. B. The muscularis mucosae of the oesophagus of the cat, rabbit and rat. J Physiol. 1955 Oct 28;130(1):123–130. doi: 10.1113/jphysiol.1955.sp005398. [DOI] [PMC free article] [PubMed] [Google Scholar]
- Hamill O. P., Marty A., Neher E., Sakmann B., Sigworth F. J. Improved patch-clamp techniques for high-resolution current recording from cells and cell-free membrane patches. Pflugers Arch. 1981 Aug;391(2):85–100. doi: 10.1007/BF00656997. [DOI] [PubMed] [Google Scholar]
- Horn R., Marty A. Muscarinic activation of ionic currents measured by a new whole-cell recording method. J Gen Physiol. 1988 Aug;92(2):145–159. doi: 10.1085/jgp.92.2.145. [DOI] [PMC free article] [PubMed] [Google Scholar]
- INGELFINGER F. J. Esophageal motility. Physiol Rev. 1958 Oct;38(4):533–584. doi: 10.1152/physrev.1958.38.4.533. [DOI] [PubMed] [Google Scholar]
- Imaizumi Y., Muraki K., Takeda M., Watanabe M. Measurement and simulation of noninactivating Ca current in smooth muscle cells. Am J Physiol. 1989 Apr;256(4 Pt 1):C880–C885. doi: 10.1152/ajpcell.1989.256.4.C880. [DOI] [PubMed] [Google Scholar]
- Kamikawa Y., Fujinuma S., Shimo Y. Contractile responses of the guinea-pig esophageal muscularis mucosae in vitro to arachidonic acid and its metabolites. Eur J Pharmacol. 1985 Aug 7;114(1):53–59. doi: 10.1016/0014-2999(85)90519-9. [DOI] [PubMed] [Google Scholar]
- Kamikawa Y., Shimo Y., Uchida K. Inhibitory actions of catecholamines on electrically induced contractions of the submucous plexus-longitudinal muscularis mucosae preparation of the guinea-pig oesophagus. Br J Pharmacol. 1982 Jun;76(2):271–277. doi: 10.1111/j.1476-5381.1982.tb09217.x. [DOI] [PMC free article] [PubMed] [Google Scholar]
- Katzka D. A., Morad M. Properties of calcium channels in guinea-pig gastric myocytes. J Physiol. 1989 Jun;413:175–197. doi: 10.1113/jphysiol.1989.sp017648. [DOI] [PMC free article] [PubMed] [Google Scholar]
- Klöckner U., Isenberg G. Action potentials and net membrane currents of isolated smooth muscle cells (urinary bladder of the guinea-pig). Pflugers Arch. 1985 Dec;405(4):329–339. doi: 10.1007/BF00595685. [DOI] [PubMed] [Google Scholar]
- Korn S. J., Bolden A., Horn R. Control of action potentials and Ca2+ influx by the Ca(2+)-dependent chloride current in mouse pituitary cells. J Physiol. 1991 Aug;439:423–437. doi: 10.1113/jphysiol.1991.sp018674. [DOI] [PMC free article] [PubMed] [Google Scholar]
- Lang R. J. The whole-cell Ca2+ channel current in single smooth muscle cells of the guinea-pig ureter. J Physiol. 1990 Apr;423:453–473. doi: 10.1113/jphysiol.1990.sp018033. [DOI] [PMC free article] [PubMed] [Google Scholar]
- Langton P. D., Burke E. P., Sanders K. M. Participation of Ca currents in colonic electrical activity. Am J Physiol. 1989 Sep;257(3 Pt 1):C451–C460. doi: 10.1152/ajpcell.1989.257.3.C451. [DOI] [PubMed] [Google Scholar]
- Marty A., Tan Y. P., Trautmann A. Three types of calcium-dependent channel in rat lacrimal glands. J Physiol. 1984 Dec;357:293–325. doi: 10.1113/jphysiol.1984.sp015501. [DOI] [PMC free article] [PubMed] [Google Scholar]
- Mayer M. L. A calcium-activated chloride current generates the after-depolarization of rat sensory neurones in culture. J Physiol. 1985 Jul;364:217–239. doi: 10.1113/jphysiol.1985.sp015740. [DOI] [PMC free article] [PubMed] [Google Scholar]
- Nishimura T., Akasu T., Tokimasa T. A slow calcium-dependent chloride current in rhythmic hyperpolarization in neurones of the rabbit vesical pelvic ganglia. J Physiol. 1991 Jun;437:673–690. doi: 10.1113/jphysiol.1991.sp018618. [DOI] [PMC free article] [PubMed] [Google Scholar]
- Ohya Y., Terada K., Kitamura K., Kuriyama H. Membrane currents recorded from a fragment of rabbit intestinal smooth muscle cell. Am J Physiol. 1986 Sep;251(3 Pt 1):C335–C346. doi: 10.1152/ajpcell.1986.251.3.C335. [DOI] [PubMed] [Google Scholar]
- Pacaud P., Loirand G., Lavie J. L., Mironneau C., Mironneau J. Calcium-activated chloride current in rat vascular smooth muscle cells in short-term primary culture. Pflugers Arch. 1989 Apr;413(6):629–636. doi: 10.1007/BF00581813. [DOI] [PubMed] [Google Scholar]
- Robinson K., Giles W. A data acquisition, display and plotting program for the IBM PC. Comput Methods Programs Biomed. 1986 Dec;23(3):319–327. doi: 10.1016/0169-2607(86)90067-2. [DOI] [PubMed] [Google Scholar]
- Satoh S., Kubota Y., Itoh T., Kuriyama H. Mechanisms of the Ba2+-induced contraction in smooth muscle cells of the rabbit mesenteric artery. J Gen Physiol. 1987 Feb;89(2):215–237. doi: 10.1085/jgp.89.2.215. [DOI] [PMC free article] [PubMed] [Google Scholar]
- Scott R. H., McGuirk S. M., Dolphin A. C. Modulation of divalent cation-activated chloride ion currents. Br J Pharmacol. 1988 Jul;94(3):653–662. doi: 10.1111/j.1476-5381.1988.tb11572.x. [DOI] [PMC free article] [PubMed] [Google Scholar]
- Sugarbaker D. J., Rattan S., Goyal R. K. Mechanical and electrical activity of esophageal smooth muscle during peristalsis. Am J Physiol. 1984 Feb;246(2 Pt 1):G145–G150. doi: 10.1152/ajpgi.1984.246.2.G145. [DOI] [PubMed] [Google Scholar]
- White M. M., Aylwin M. Niflumic and flufenamic acids are potent reversible blockers of Ca2(+)-activated Cl- channels in Xenopus oocytes. Mol Pharmacol. 1990 May;37(5):720–724. [PubMed] [Google Scholar]
- Wier W. G. Cytoplasmic [Ca2+] in mammalian ventricle: dynamic control by cellular processes. Annu Rev Physiol. 1990;52:467–485. doi: 10.1146/annurev.ph.52.030190.002343. [DOI] [PubMed] [Google Scholar]
- Wong B. S. Ionic conductances in dissociated smooth muscle cells. Proc Soc Exp Biol Med. 1991 Jun;197(2):125–134. doi: 10.3181/00379727-197-43234. [DOI] [PubMed] [Google Scholar]
- Yamamoto Y., Hu S. L., Kao C. Y. Inward current in single smooth muscle cells of the guinea pig taenia coli. J Gen Physiol. 1989 Mar;93(3):521–550. doi: 10.1085/jgp.93.3.521. [DOI] [PMC free article] [PubMed] [Google Scholar]