Abstract
1. Regulation of glycogenolysis in frog skeletal muscle at rest and following contraction was studied by measuring the concentration of phosphate-containing metabolites and the intracellular pH (pHi) in CN-treated muscles, in which oxidative phosphorylation was inhibited by NaCN, using the 31P nuclear magnetic resonance (NMR) technique. 2. When CN-treated muscles were kept at rest, the phosphocreatine (PCr) concentration very slowly decreased with time with a corresponding increase of the inorganic phosphate (Pi) concentration, while the ATP concentration remained unchanged. The pHi changed in the alkaline direction for the first 3 h, and then started to change in the acidic direction. 3. When CN-treated muscles were tetanized for 10 s, the PCr concentration decreased with a corresponding increase of the Pi concentration and acidification of pHi, while the ATP concentration remained unchanged. 4. When CN-treated muscles were tetanized repeatedly (each for 2 s) at constant intervals, the pHi changed in the alkaline direction following the first and the second tetani, and then changed in the acidic direction following the subsequent tetani, indicating that the consumed ATP is first replenished by the Lohmann reaction, while glycogenolysis starts only when the total amount of contractile activity exceeds a critical value. 5. Irrespective of whether CN-treated muscles were kept at rest or tetanized repeatedly, the Pi concentration increased to about 8 mM (mmol/kg wet muscle) when glycogenolysis started, suggesting that the onset of glycogenolysis in CN-treated muscles is regulated by the Pi concentration. 6. The 'internal' buffering power of muscle cytosol was estimated to be 35 mM H+/pH unit in anaerobic muscles and 25 mM H+/pH unit in CN- and iodoacetic acid (IAA)-treated muscles. The 'internal' buffering power contains a contribution due to flux of carbon dioxide and lactic acid across the cell membrane. Evidence indicated that lactic acid flux is small.
Full text
PDF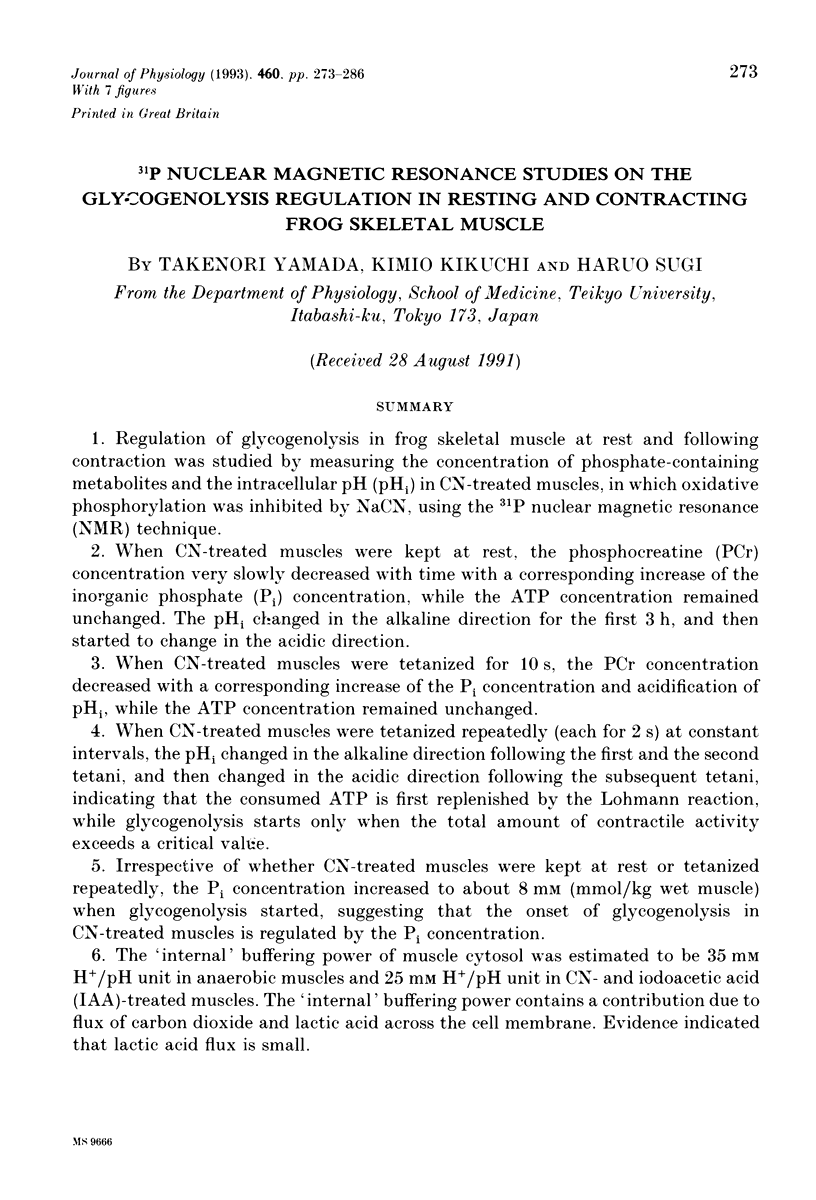
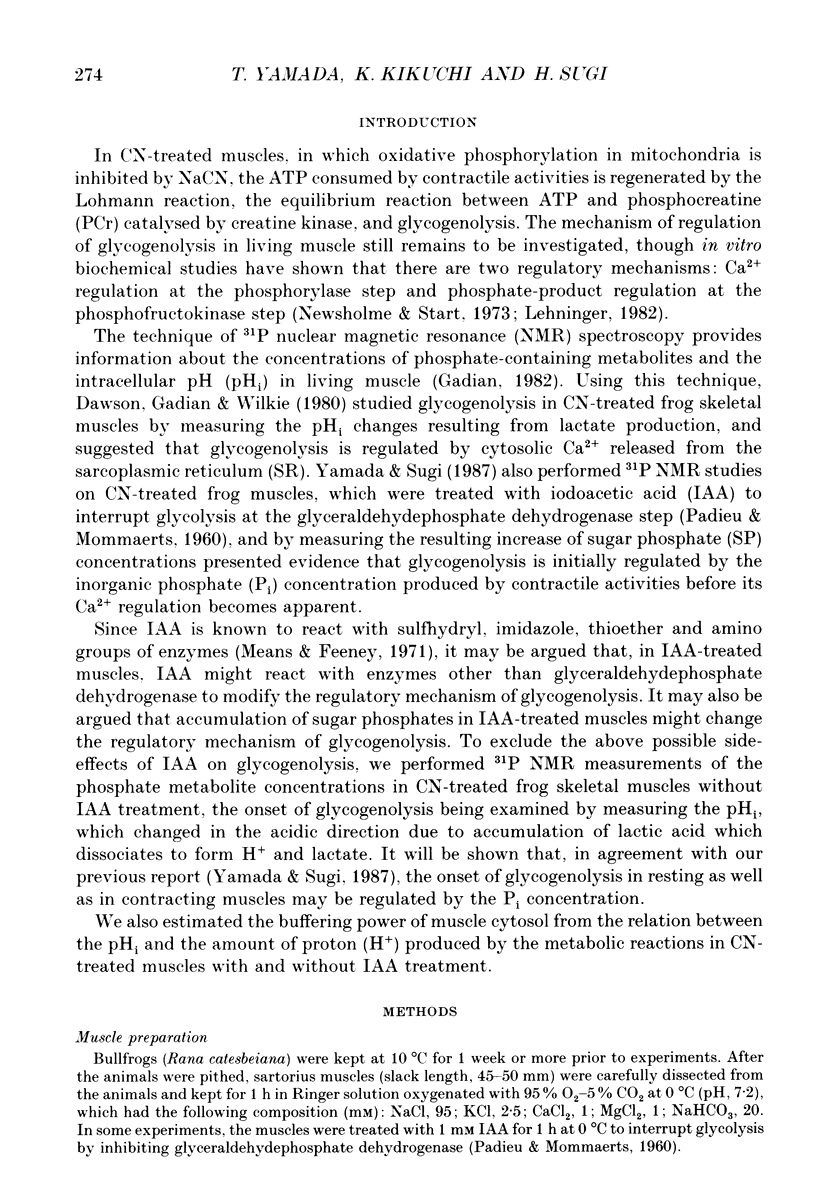
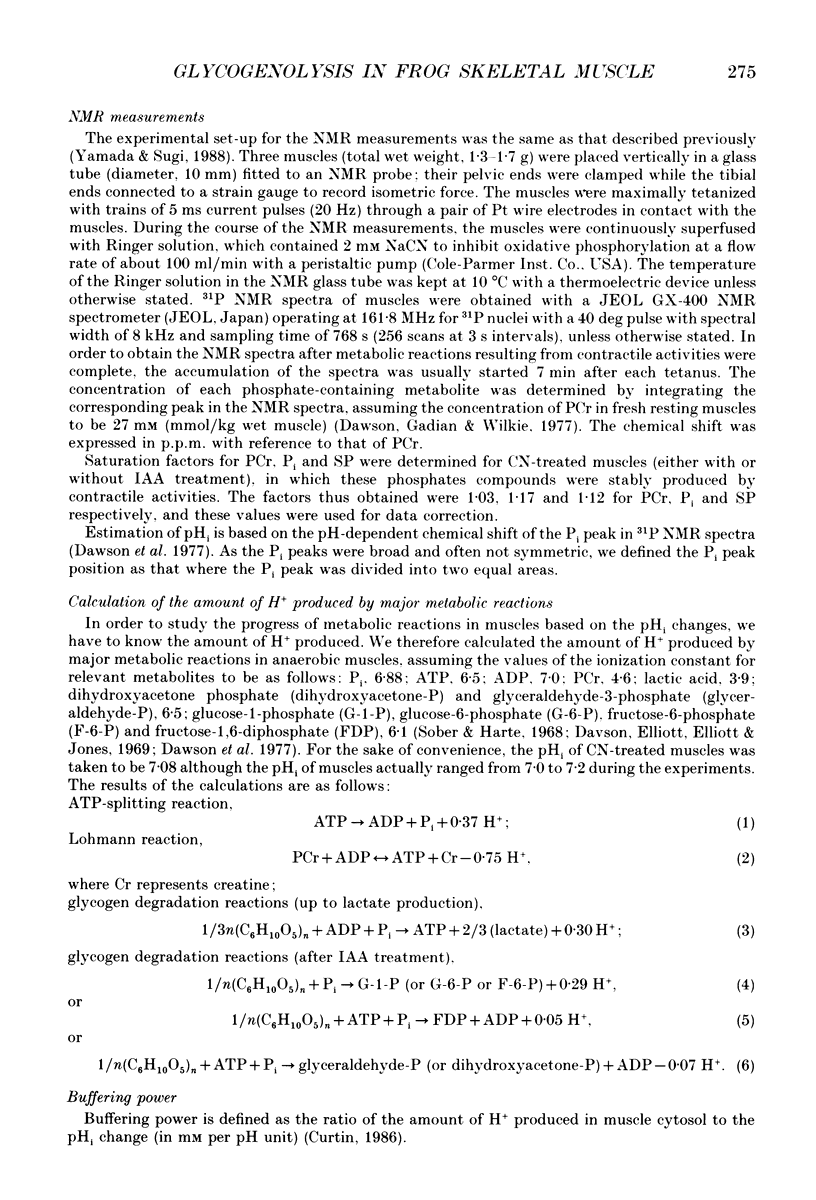
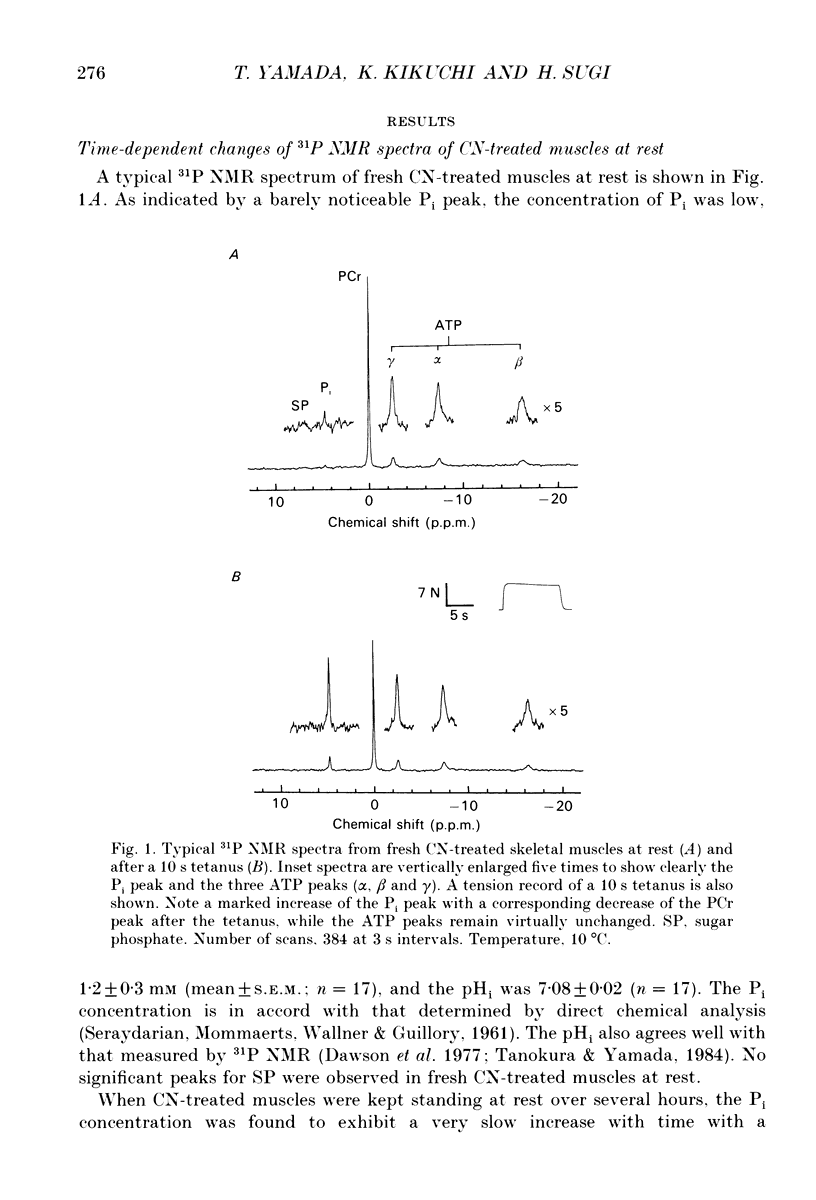
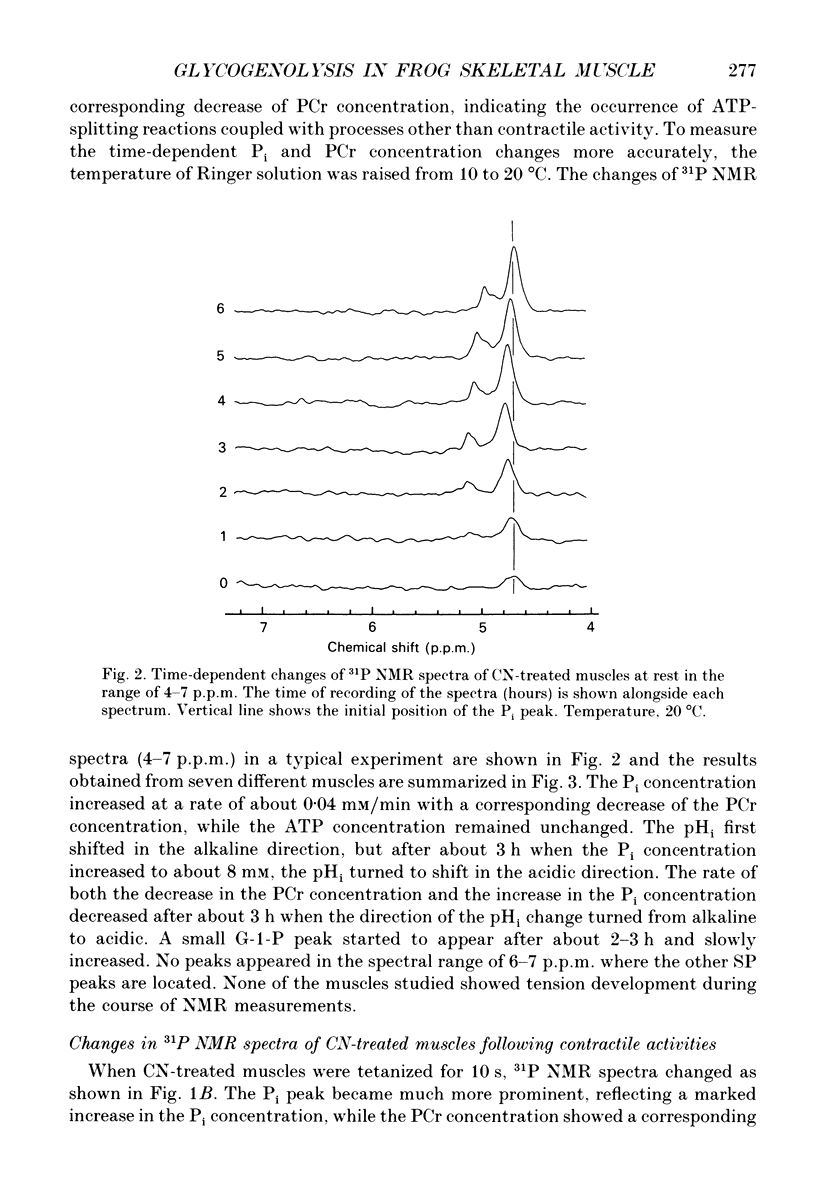
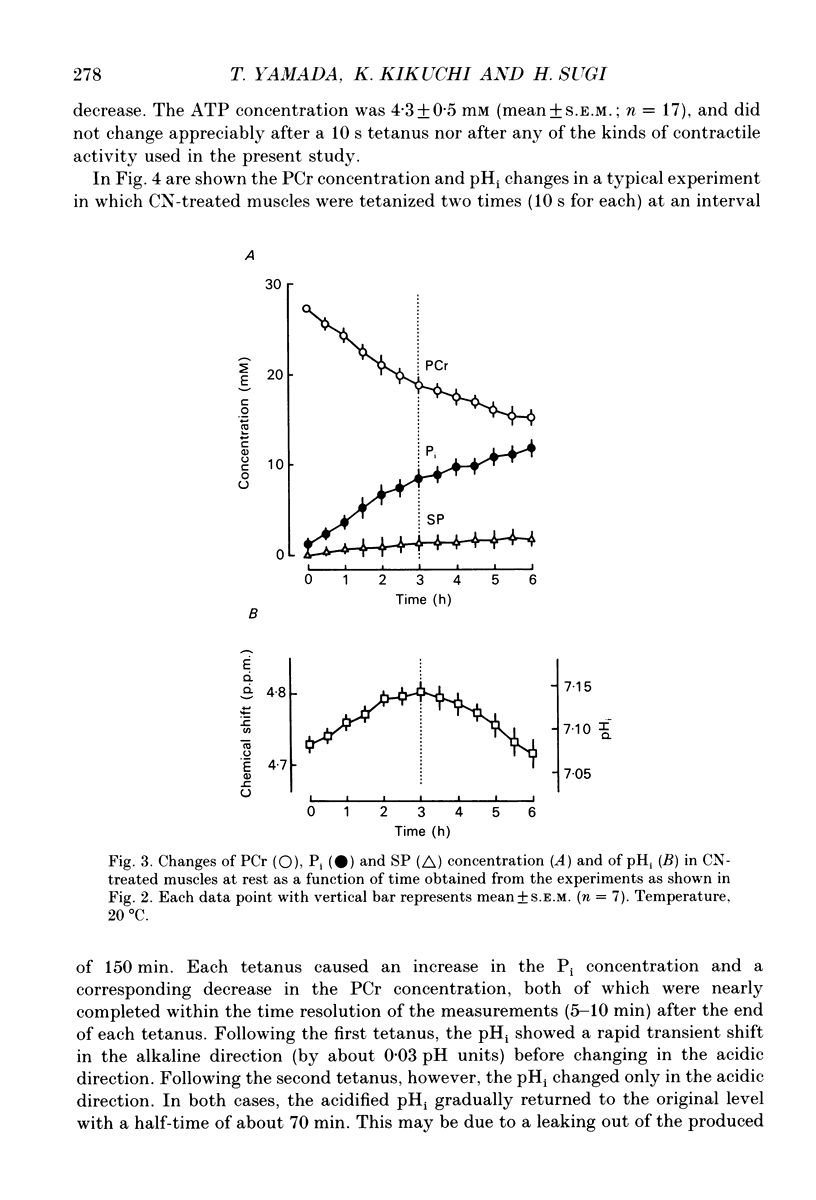
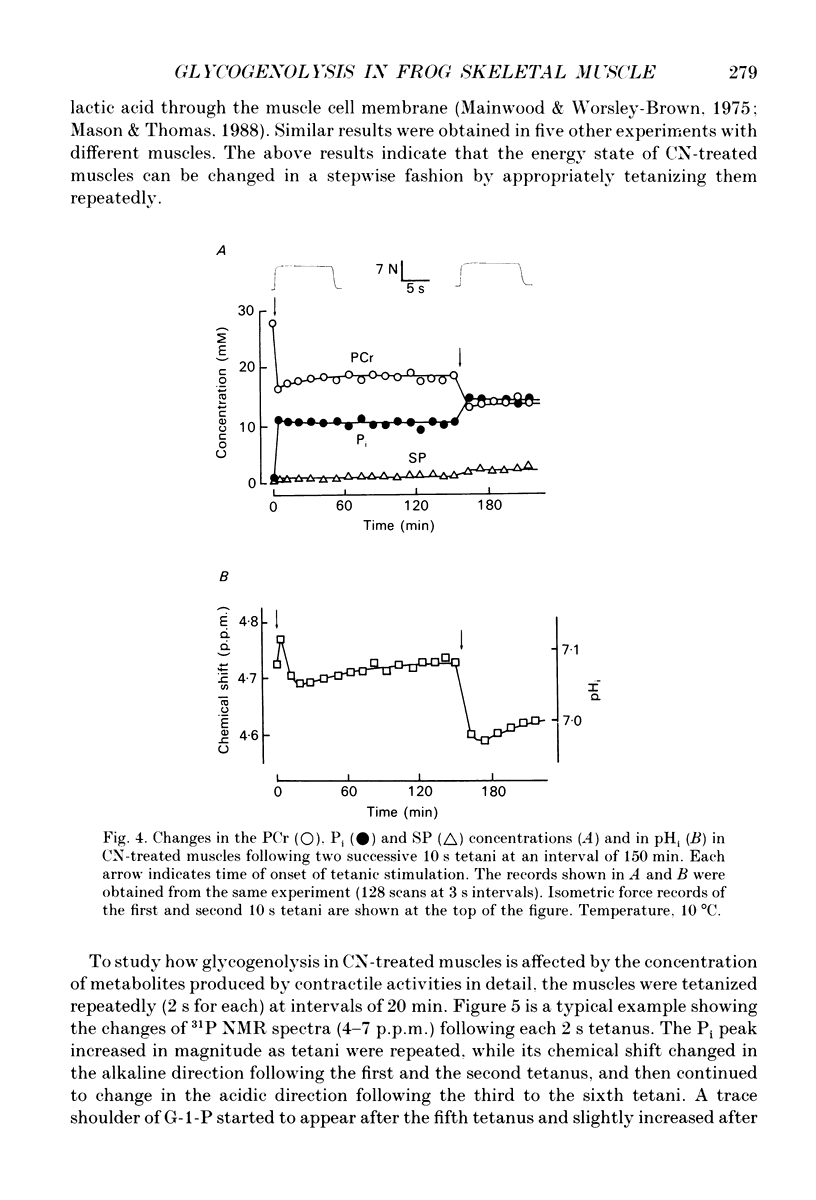
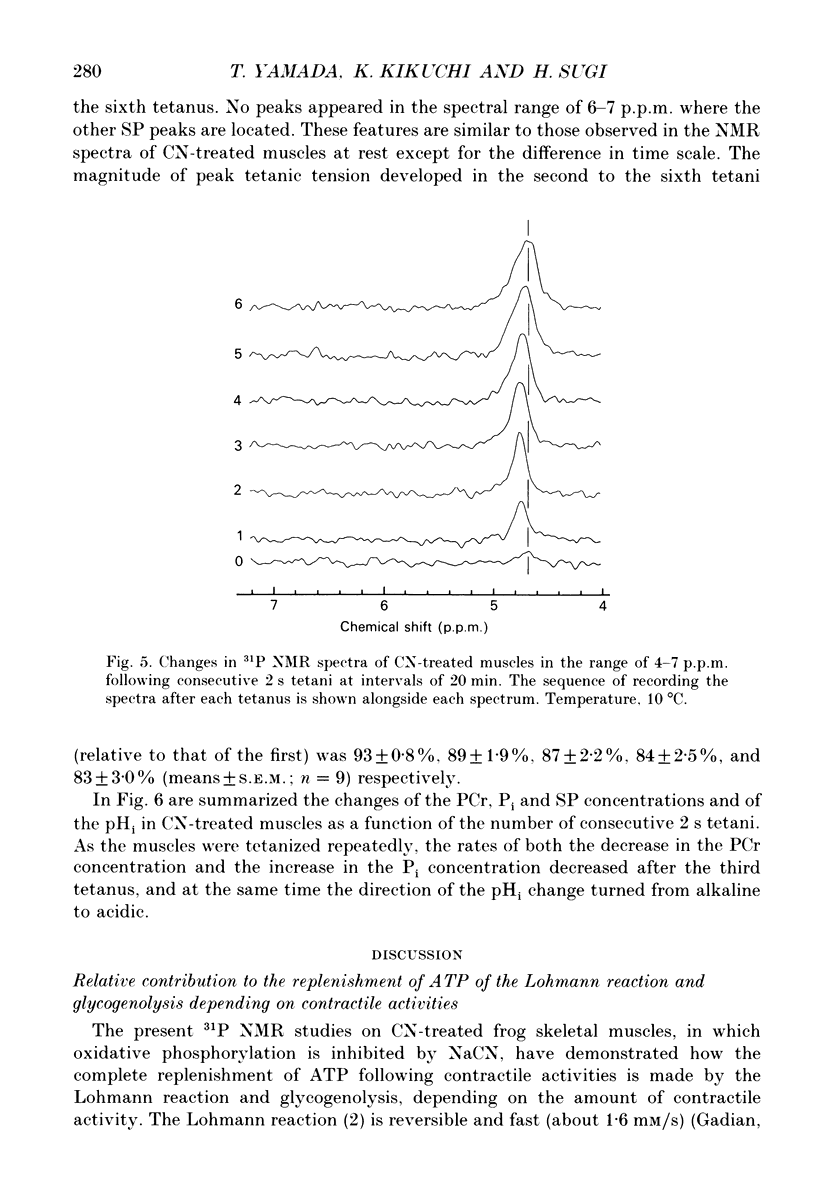
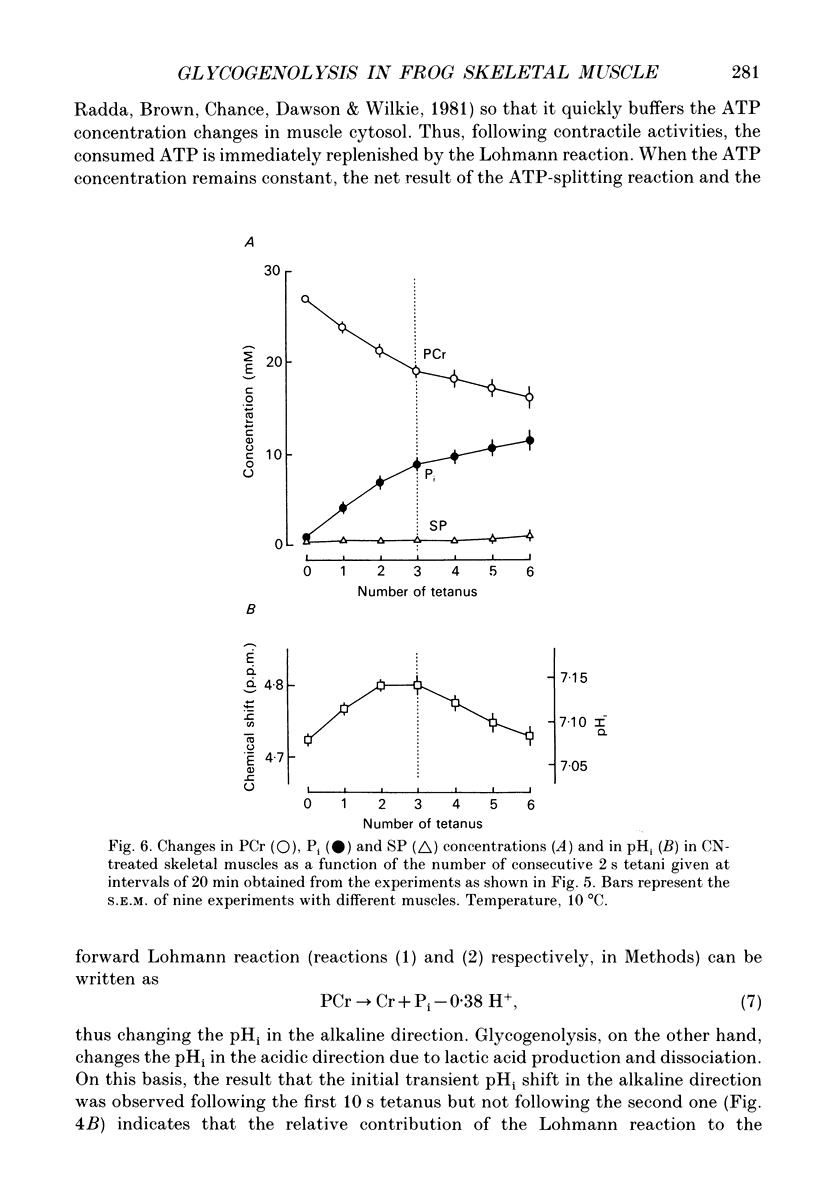
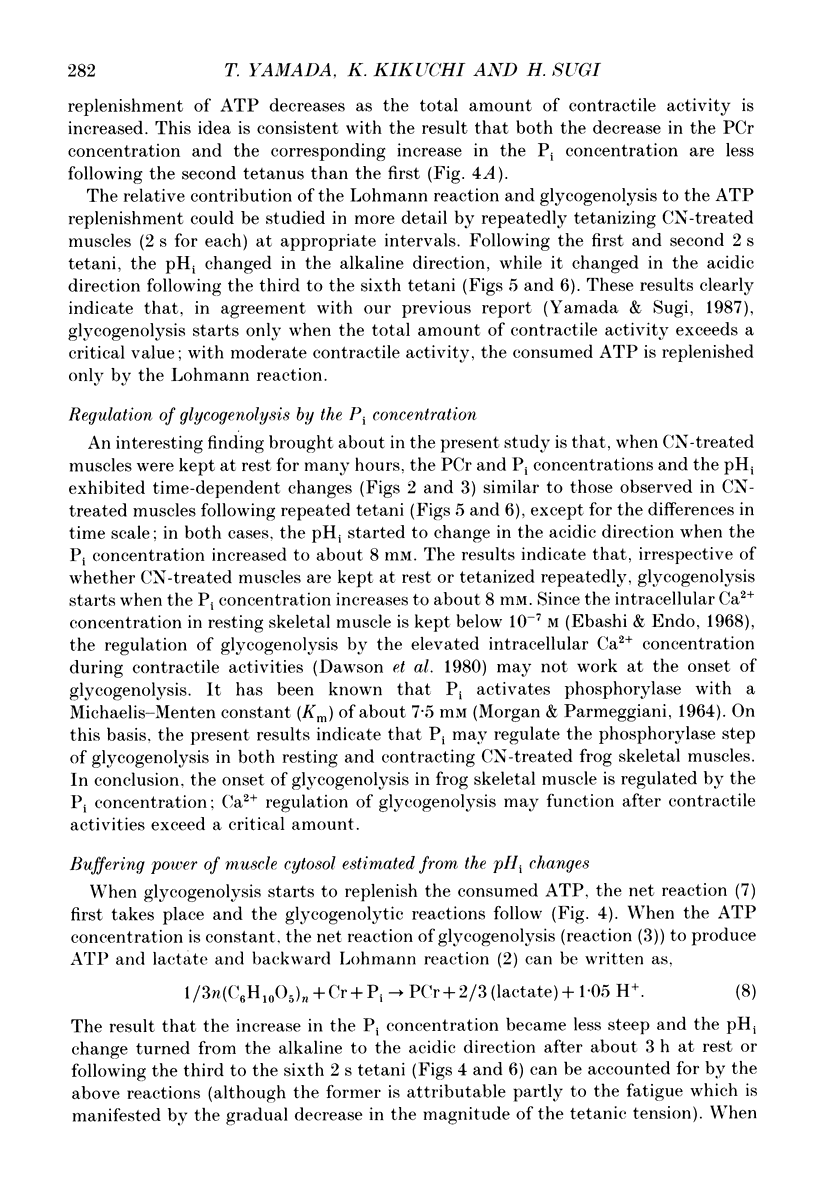
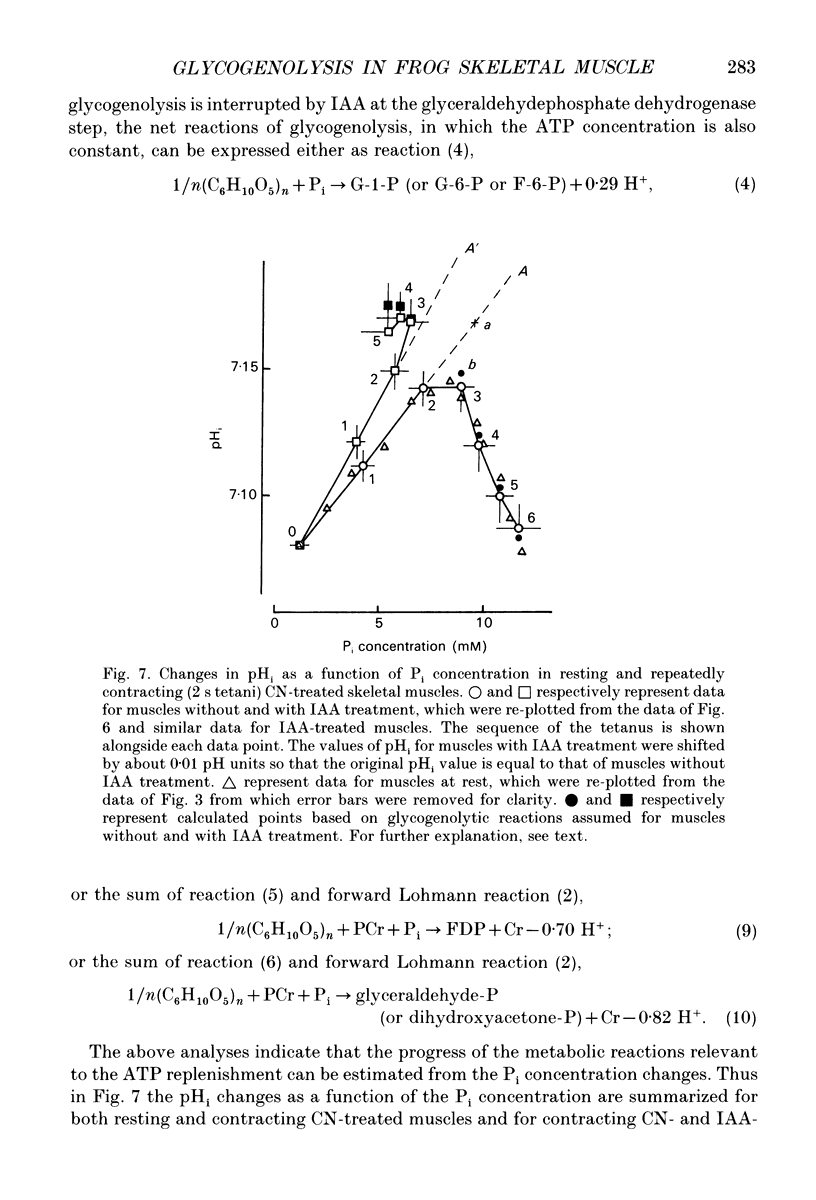
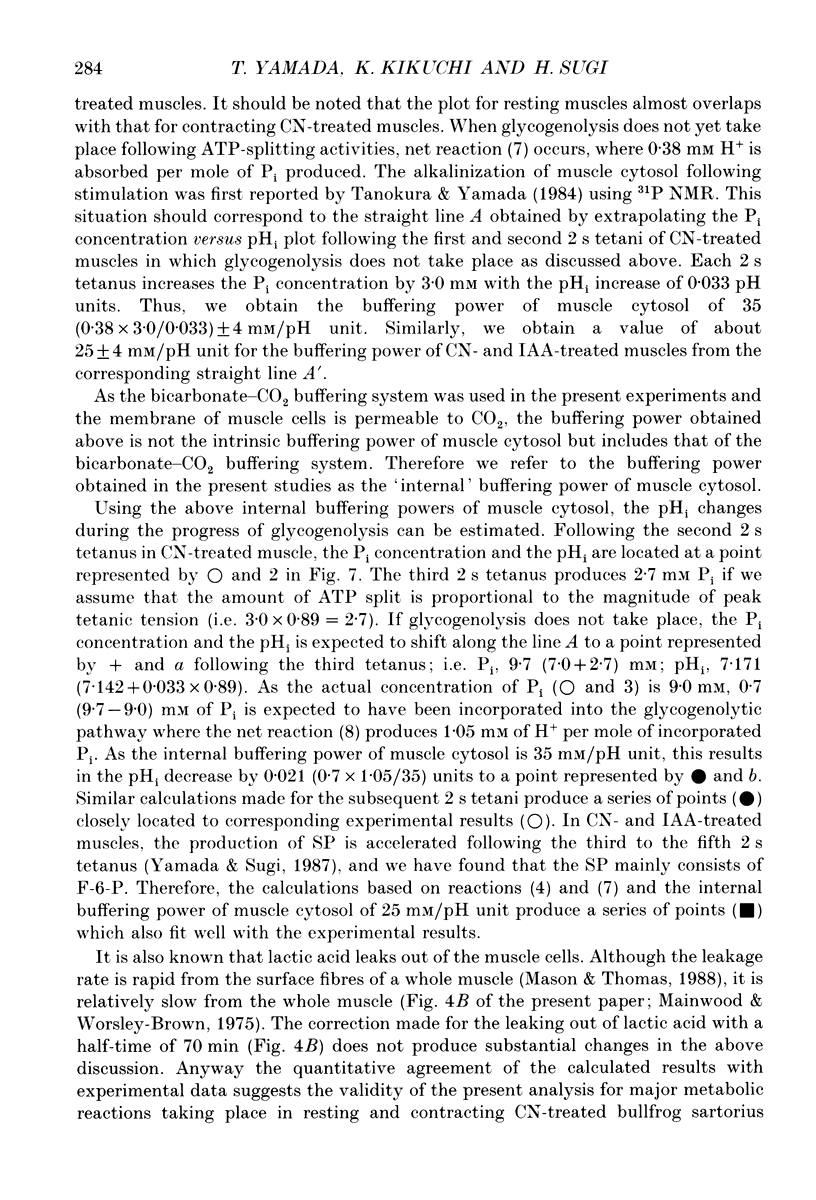
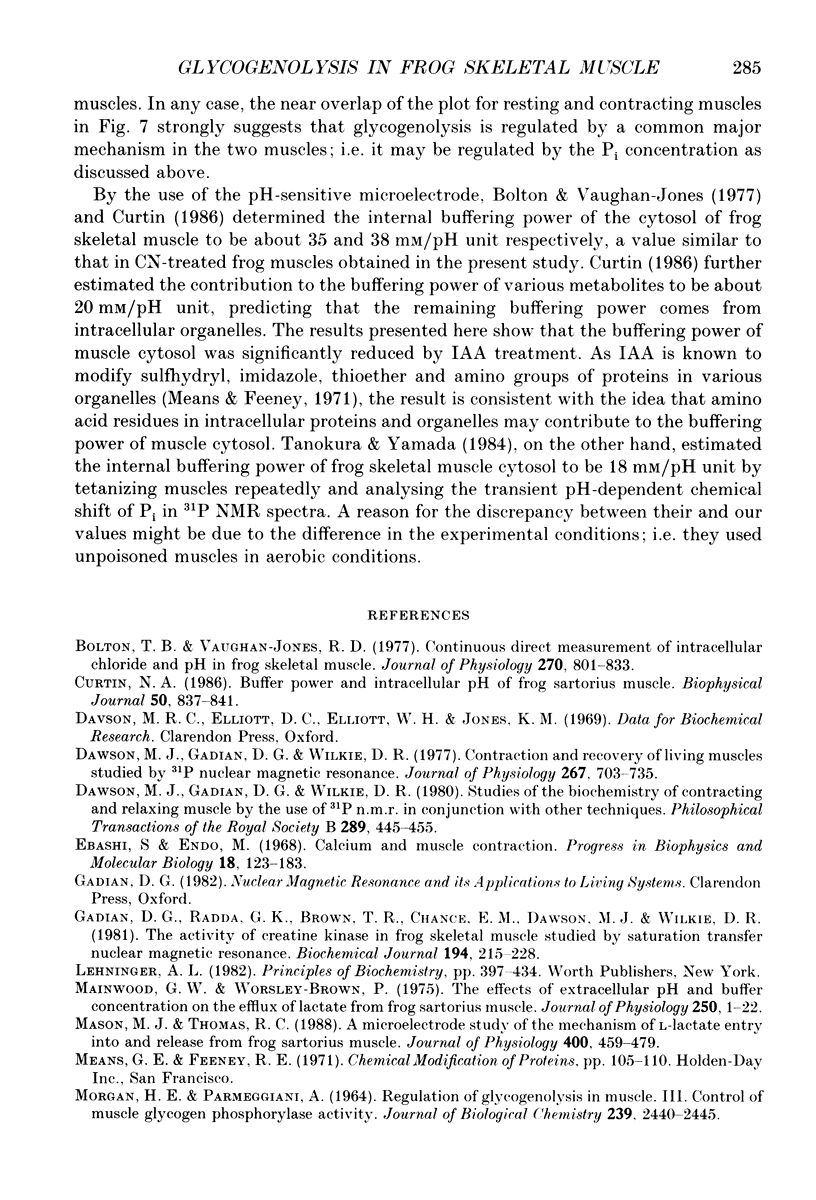
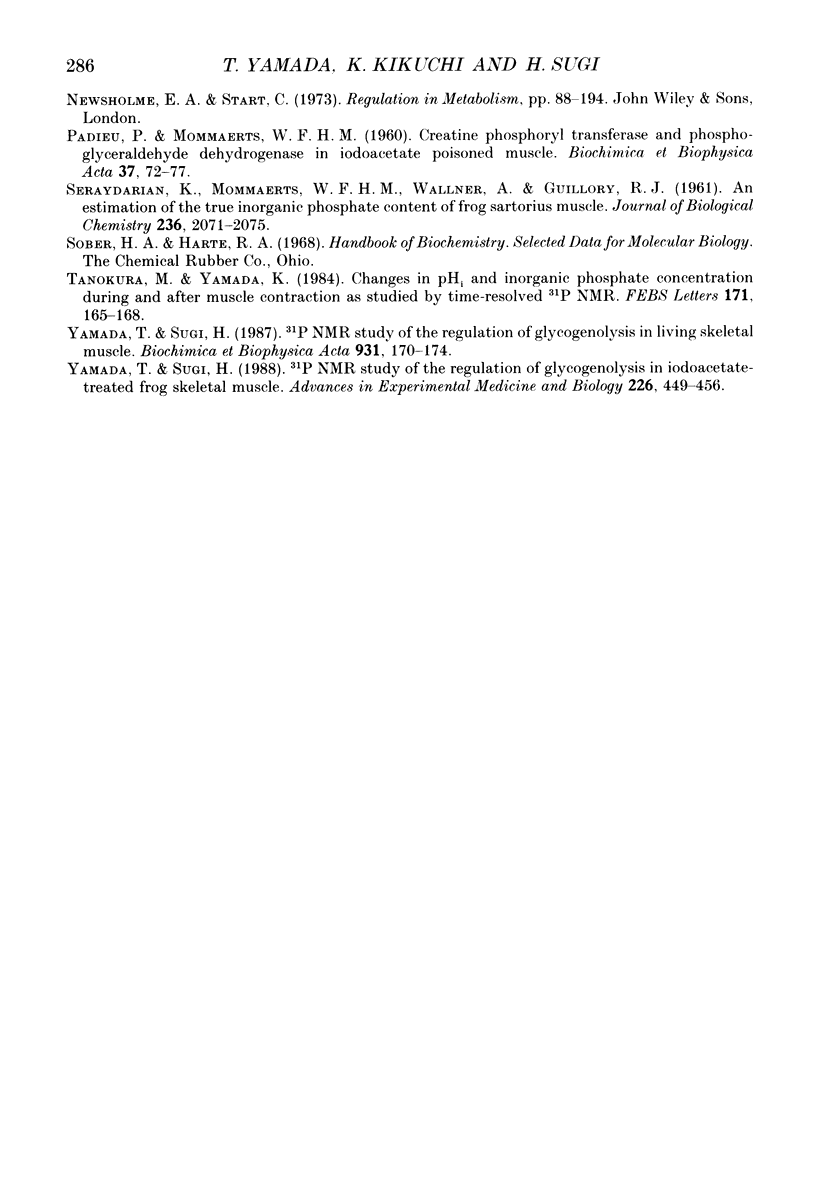
Selected References
These references are in PubMed. This may not be the complete list of references from this article.
- Bolton T. B., Vaughan-Jones R. D. Continuous direct measurement of intracellular chloride and pH in frog skeletal muscle. J Physiol. 1977 Sep;270(3):801–833. doi: 10.1113/jphysiol.1977.sp011983. [DOI] [PMC free article] [PubMed] [Google Scholar]
- Curtin N. A. Buffer power and intracellular pH of frog sartorius muscle. Biophys J. 1986 Nov;50(5):837–841. doi: 10.1016/S0006-3495(86)83524-X. [DOI] [PMC free article] [PubMed] [Google Scholar]
- Dawson M. J., Gadian D. G., Wilkie D. R. Contraction and recovery of living muscles studies by 31P nuclear magnetic resonance. J Physiol. 1977 Jun;267(3):703–735. doi: 10.1113/jphysiol.1977.sp011835. [DOI] [PMC free article] [PubMed] [Google Scholar]
- Dawson M. J., Gadian D. G., Wilkie D. R. Studies of the biochemistry of contracting and relaxing muscle by the use of 31P n.m.r. in conjunction with other techniques. Philos Trans R Soc Lond B Biol Sci. 1980 Jun 25;289(1037):445–455. doi: 10.1098/rstb.1980.0062. [DOI] [PubMed] [Google Scholar]
- Ebashi S., Endo M. Calcium ion and muscle contraction. Prog Biophys Mol Biol. 1968;18:123–183. doi: 10.1016/0079-6107(68)90023-0. [DOI] [PubMed] [Google Scholar]
- Gadian D. G., Radda G. K., Brown T. R., Chance E. M., Dawson M. J., Wilkie D. R. The activity of creatine kinase in frog skeletal muscle studied by saturation-transfer nuclear magnetic resonance. Biochem J. 1981 Jan 15;194(1):215–228. doi: 10.1042/bj1940215. [DOI] [PMC free article] [PubMed] [Google Scholar]
- MORGAN H. E., PARMEGGIANI A. REGULATION OF GLYCOGENOLYSIS IN MUSCLE. 3. CONTROL OF MUSCLE GLYCOGEN PHOSPHORYLASE ACTIVITY. J Biol Chem. 1964 Aug;239:2440–2445. [PubMed] [Google Scholar]
- Mainwood G. W., Worsley-Brown P. The effects of extracellular pH and buffer concentration on the efflux of lactate from frog sartorius muscle. J Physiol. 1975 Aug;250(1):1–22. doi: 10.1113/jphysiol.1975.sp011040. [DOI] [PMC free article] [PubMed] [Google Scholar]
- Mason M. J., Thomas R. C. A microelectrode study of the mechanisms of L-lactate entry into and release from frog sartorius muscle. J Physiol. 1988 Jun;400:459–479. doi: 10.1113/jphysiol.1988.sp017132. [DOI] [PMC free article] [PubMed] [Google Scholar]
- PADIEU P., MOMMAERTS W. F. Creatine phosphoryl transferase and phosphoglyceraldehyde dehydrogenase in iodoacetate poisoned muscle. Biochim Biophys Acta. 1960 Jan 1;37:72–77. doi: 10.1016/0006-3002(60)90080-9. [DOI] [PubMed] [Google Scholar]
- SERAYDARIAN K., MOMMAERTS W. F., WALLNER A., GUILLORY R. J. An estimation of the true inorganic phosphate content of frog sartorius muscle. J Biol Chem. 1961 Jul;236:2071–2075. [PubMed] [Google Scholar]
- Tanokura M., Yamada K. Changes in intracellular pH and inorganic phosphate concentration during and after muscle contraction as studied by time-resolved 31P-NMR. Alkalinization by contraction. FEBS Lett. 1984 Jun 11;171(2):165–168. doi: 10.1016/0014-5793(84)80480-9. [DOI] [PubMed] [Google Scholar]
- Yamada T., Sugi H. 31P NMR study of the regulation of glycogenolysis in iodoacetate-treated skeletal muscle. Adv Exp Med Biol. 1988;226:449–456. [PubMed] [Google Scholar]
- Yamada T., Sugi H. 31P-NMR study of the regulation of glycogenolysis in living skeletal muscle. Biochim Biophys Acta. 1987 Nov 12;931(2):170–174. doi: 10.1016/0167-4889(87)90203-5. [DOI] [PubMed] [Google Scholar]