Abstract
1. Intracellular recordings were made from the lateral nucleus of the amygdala in tissue slices cut from rat brain and maintained in vitro. 2. Three types of neurones were distinguished according to the after-potential that followed an action potential. Type 1 cells (44%, n = 225) had depolarizing after-potentials, resulting from a calcium-dependent chloride conductance. Type 2 cells (48%) had long-lasting (> 250 ms) hyperpolarizing after-potentials and type 3 cells (8%) had shorter hyperpolarizing after-potentials. The average resting potentials of the three cell types were -78, -69 and -62 mV respectively. Intracellular labelling with biocytin showed that type 1 cells were pyramidal neurones; type 2 and type 3 cells were non-pyramidal. 3. Experiments with receptor antagonists identified synaptic potentials mediated by excitatory amino acids and by GABA (acting at GABAA receptors) in all three cell types. A longer duration inhibitory synaptic potential resulting from activation of GABAB receptors was present in type 1 (pyramidal) and type 2 cells. 4. Cholecystokinin (100 nM to 1 microM) depolarized type 2 and type 3 cells but had no effect on type 1 (pyramidal) cells. Baclofen (1-3 microM) hyperpolarized type 1 and type 2, but not type 3 cells. [Met5]enkephalin (1-10 microM) hyperpolarized only type 2 cells. 5. It is concluded that the lateral nucleus of the amygdala contains pyramidal neurones and two types of non-pyramidal neurone; these can be differentiated by membrane properties, synaptic inputs and sensitivities to transmitters.
Full text
PDF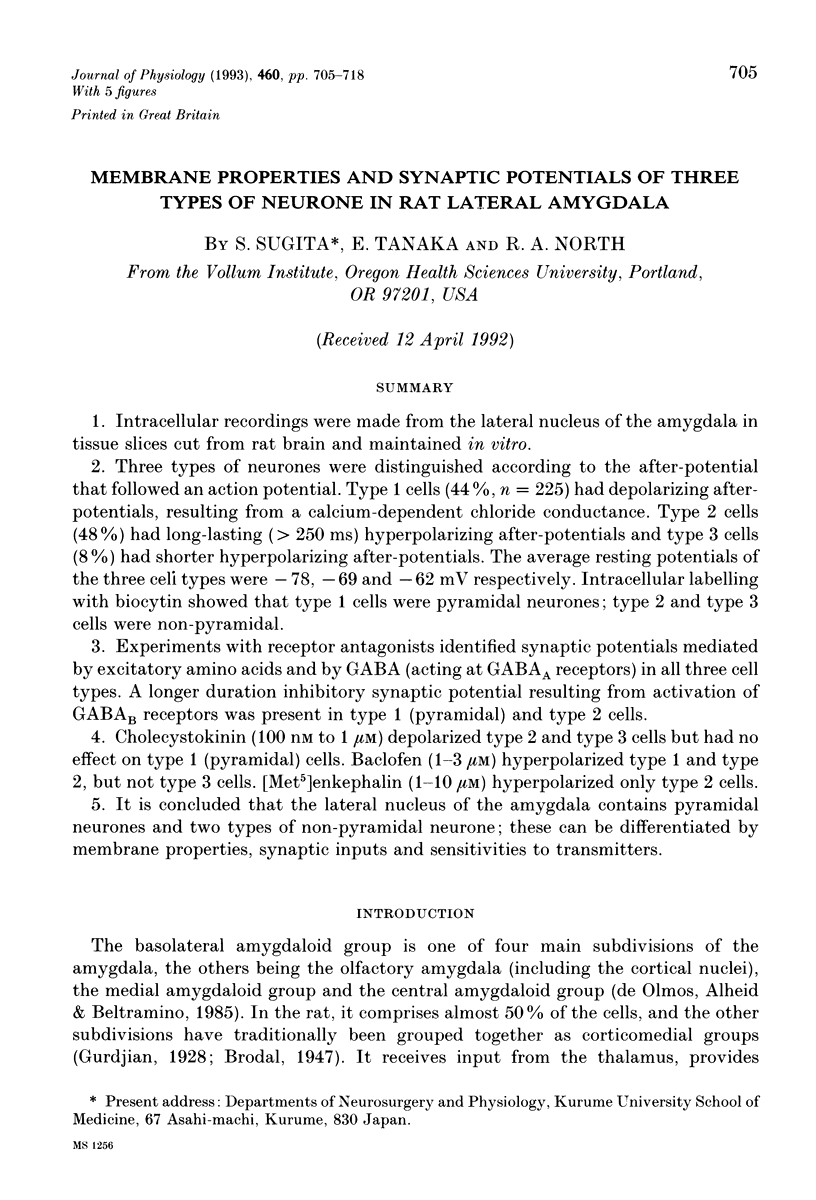
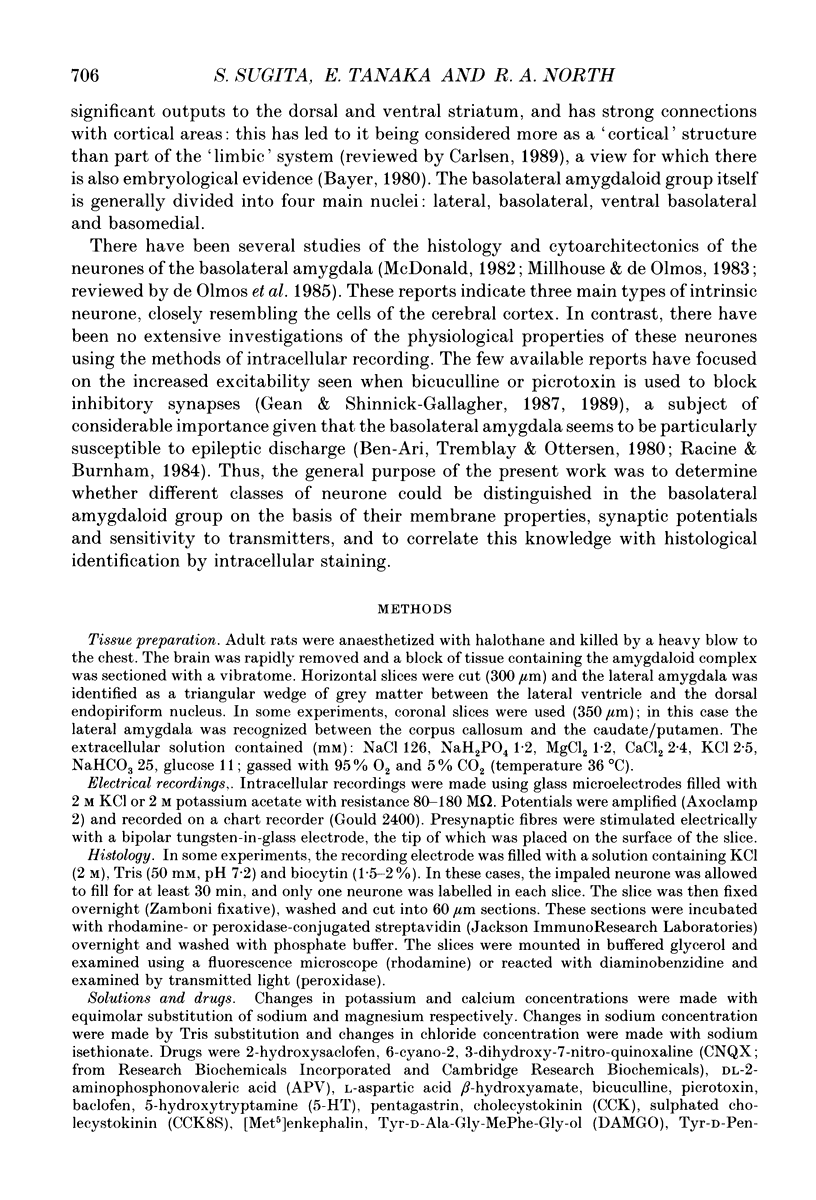
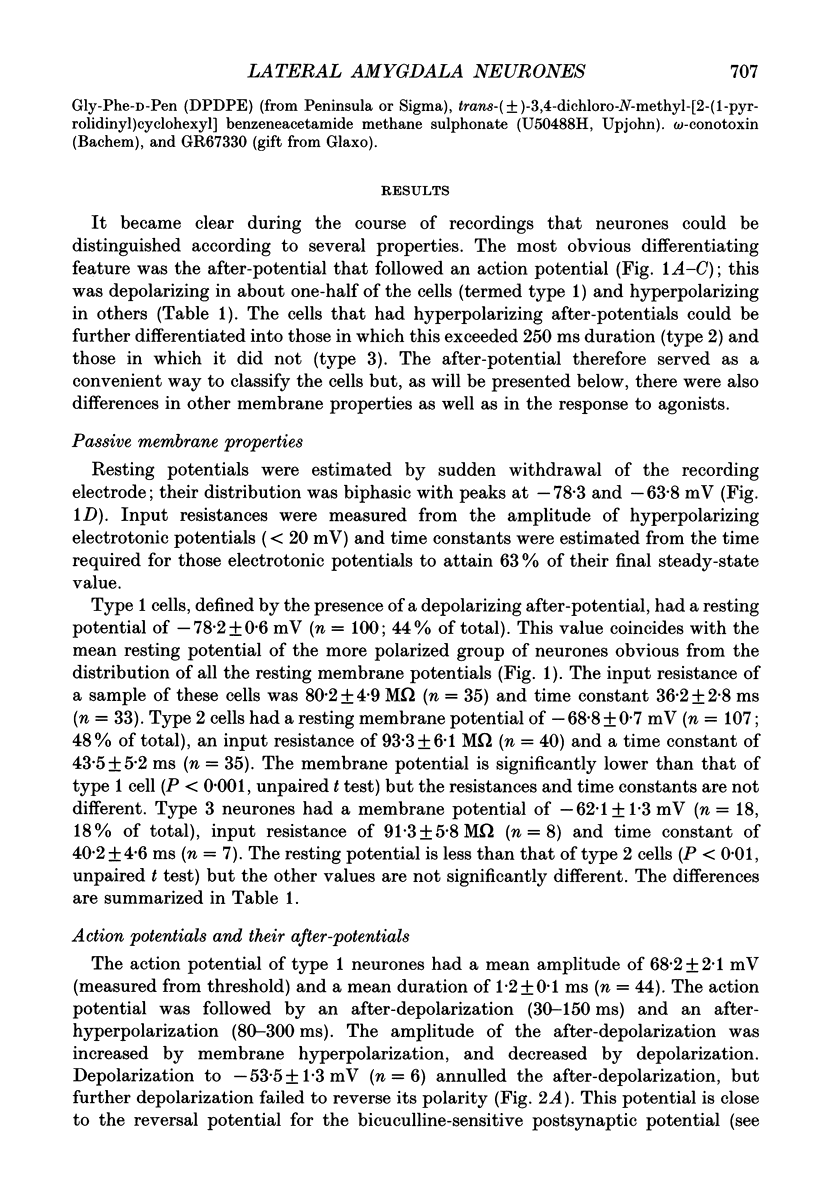
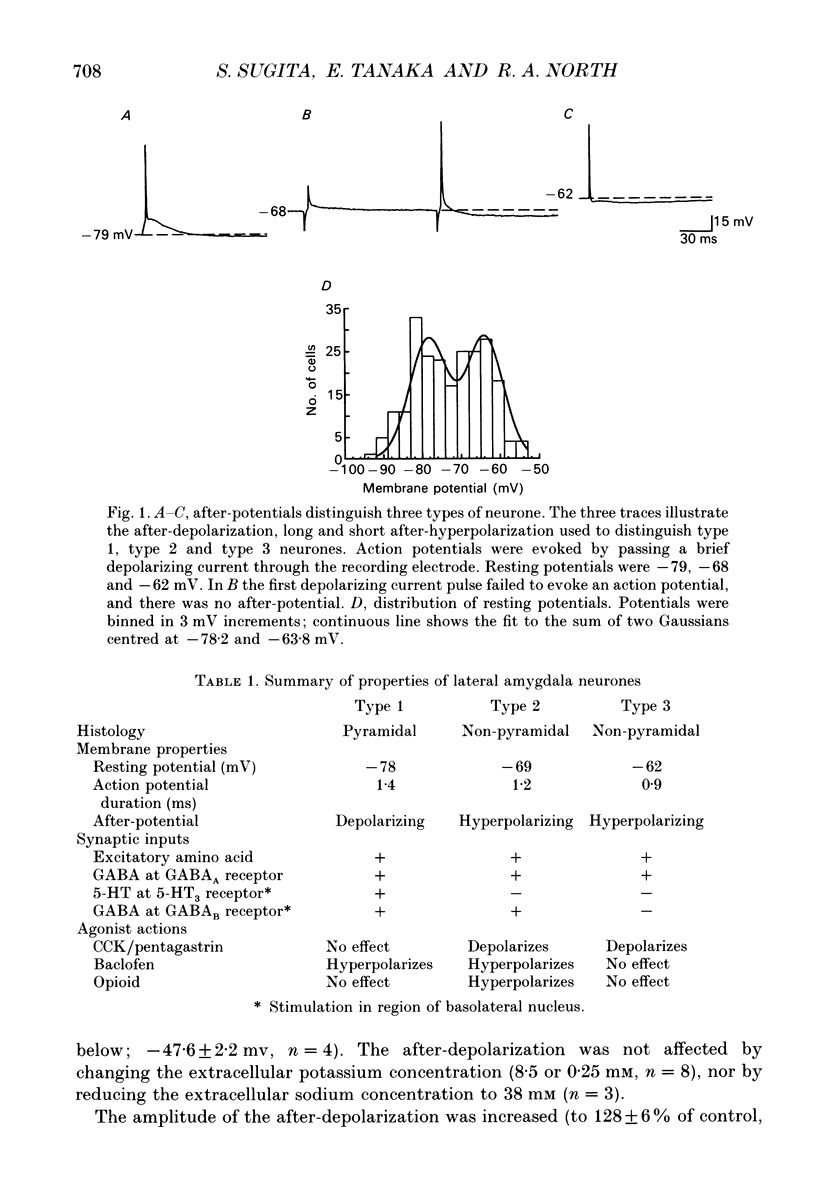
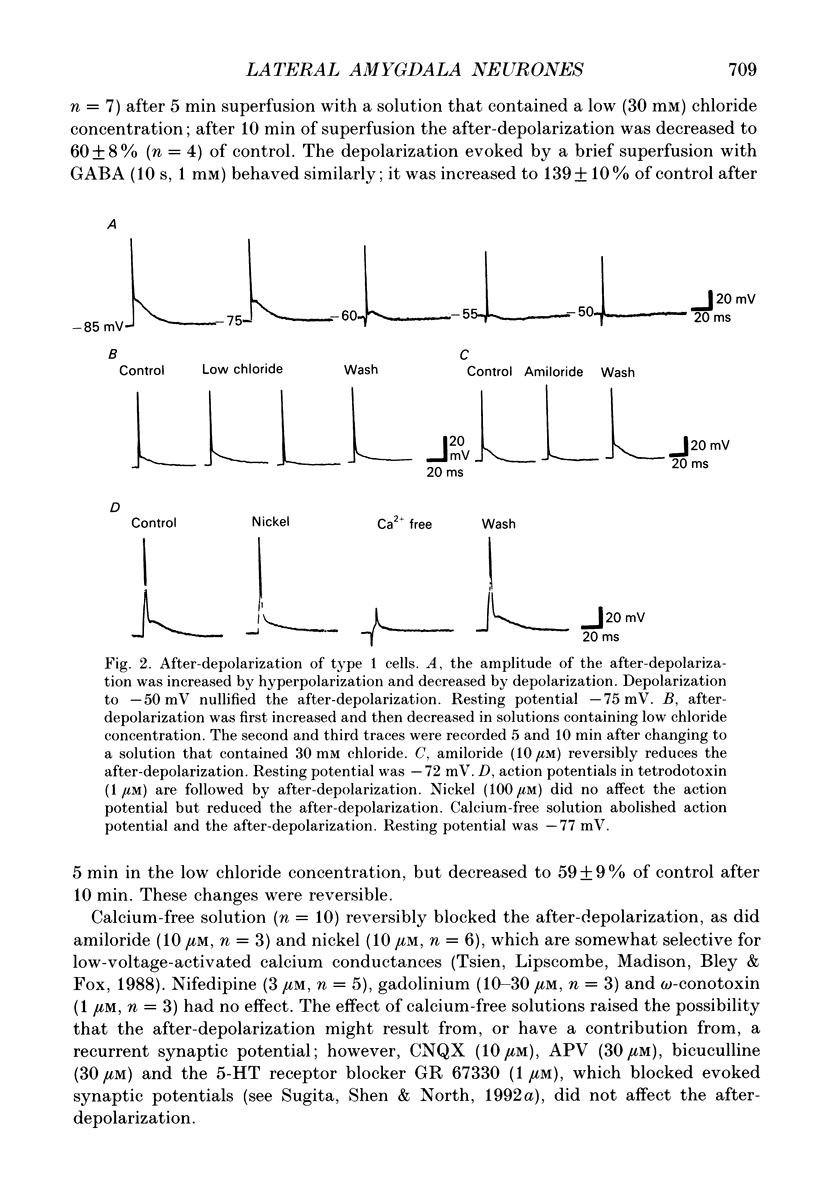
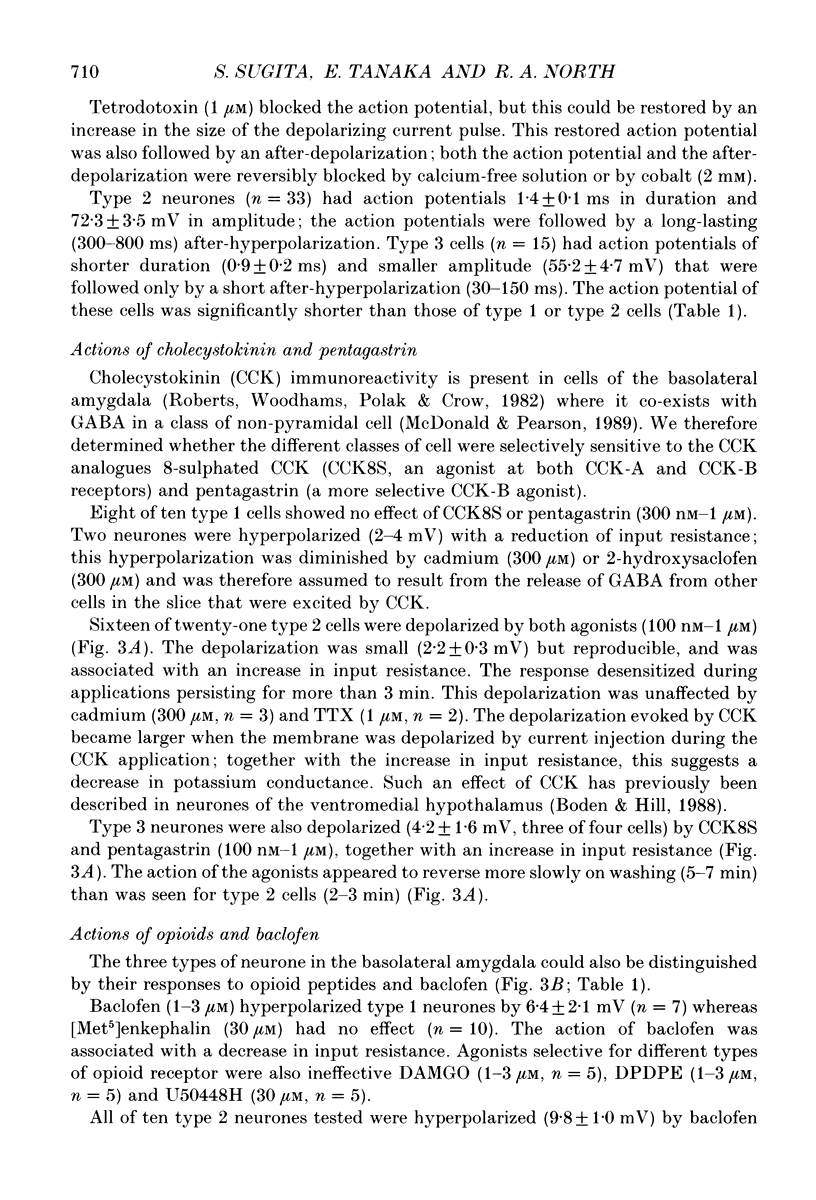
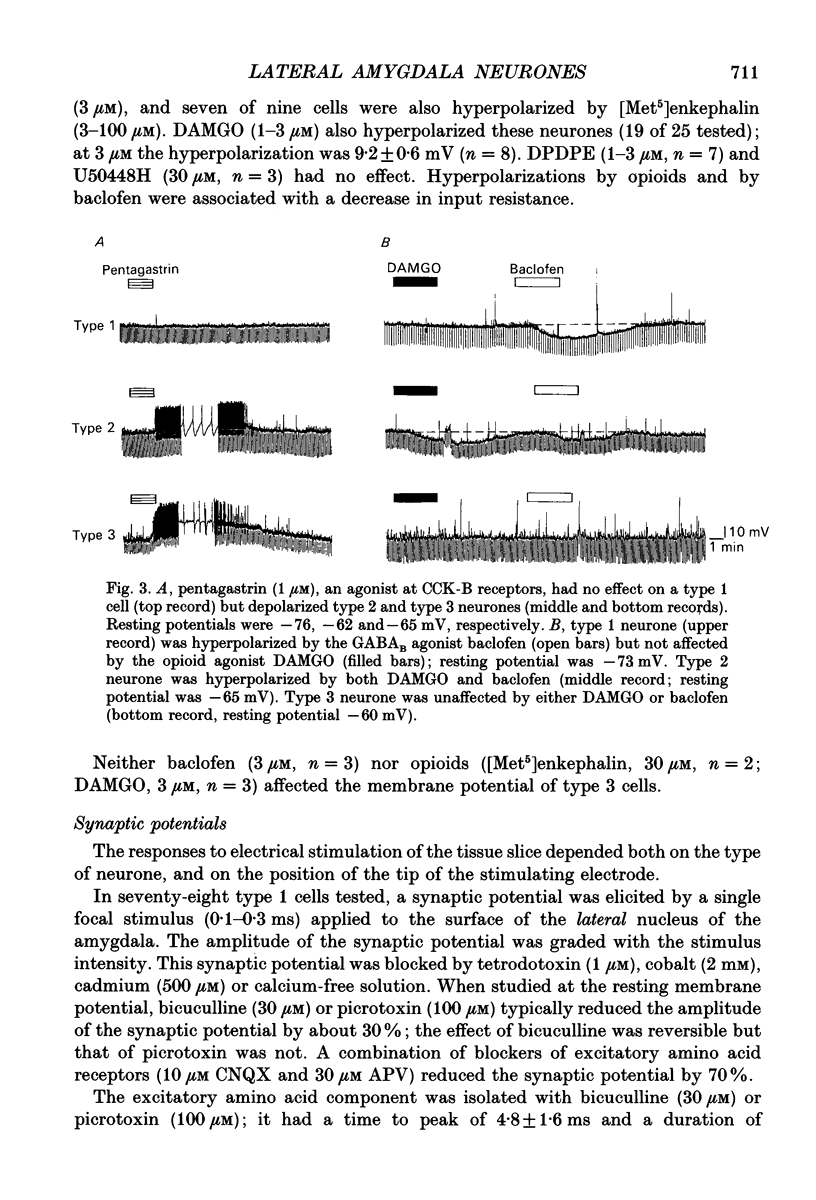
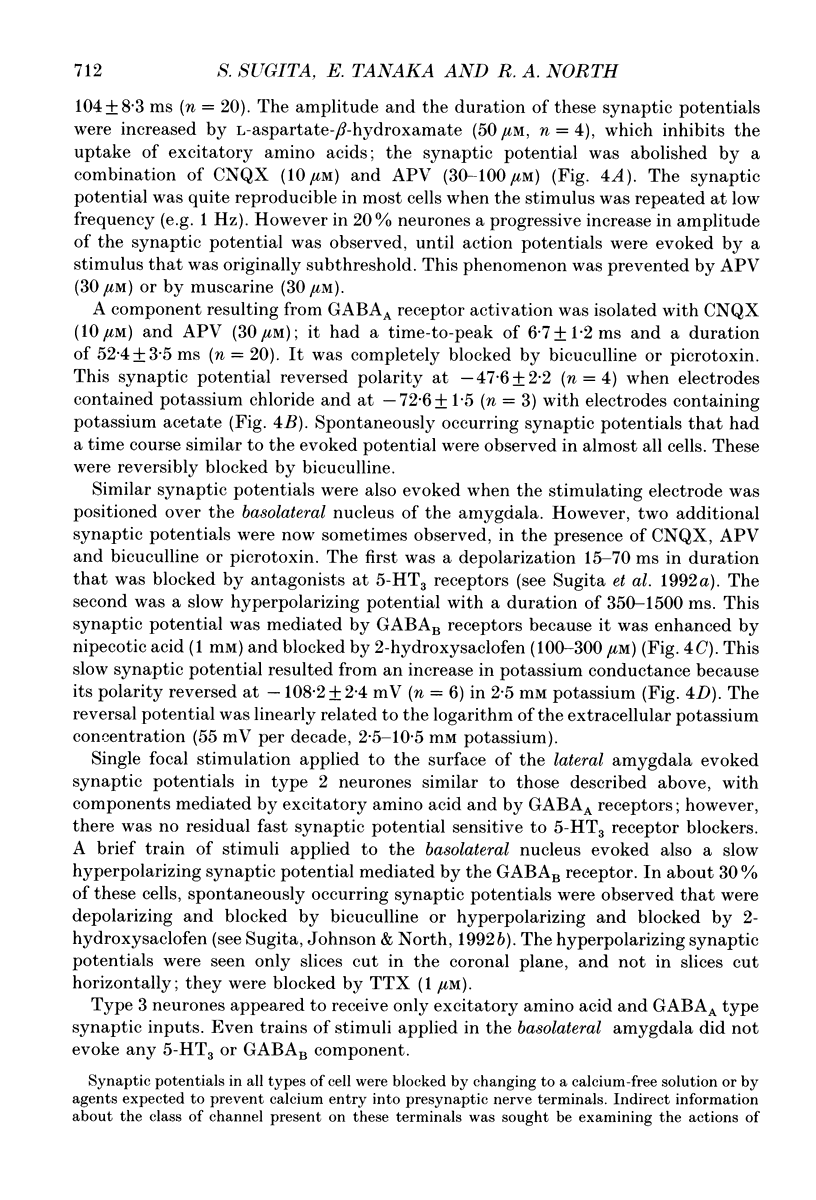
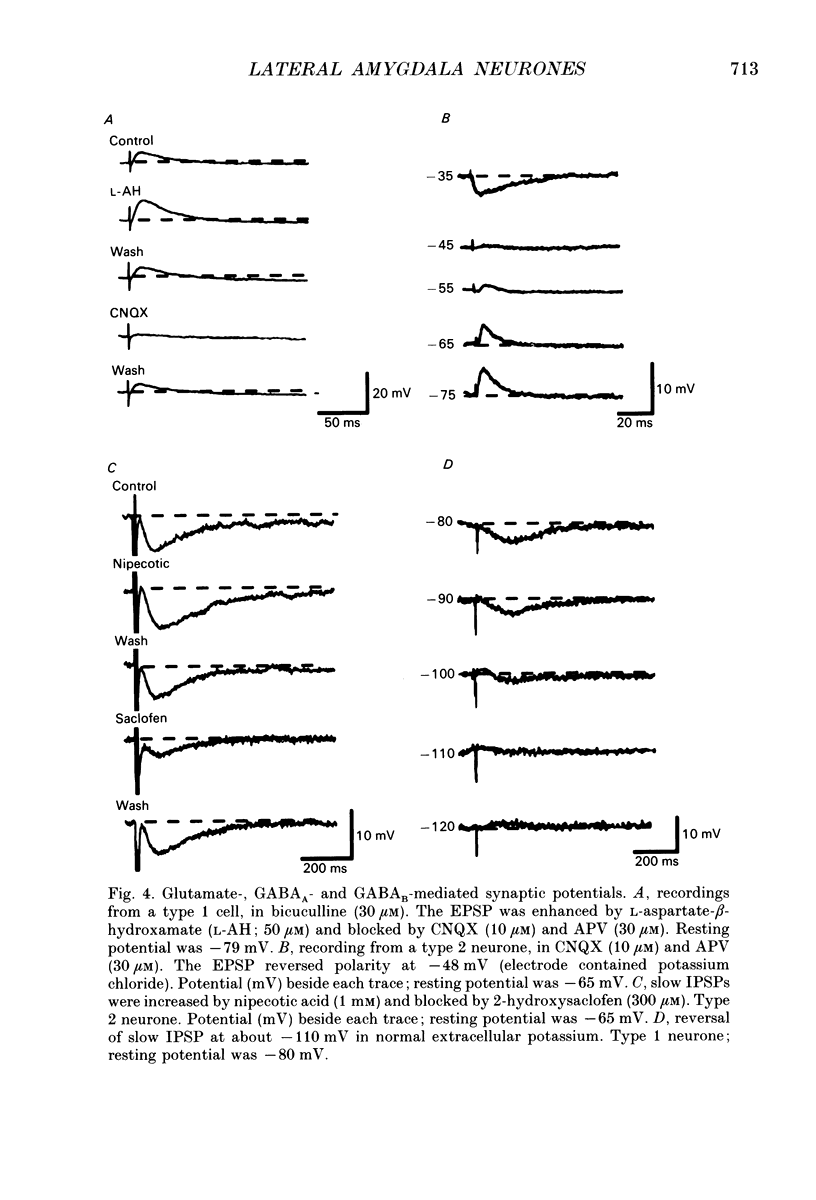
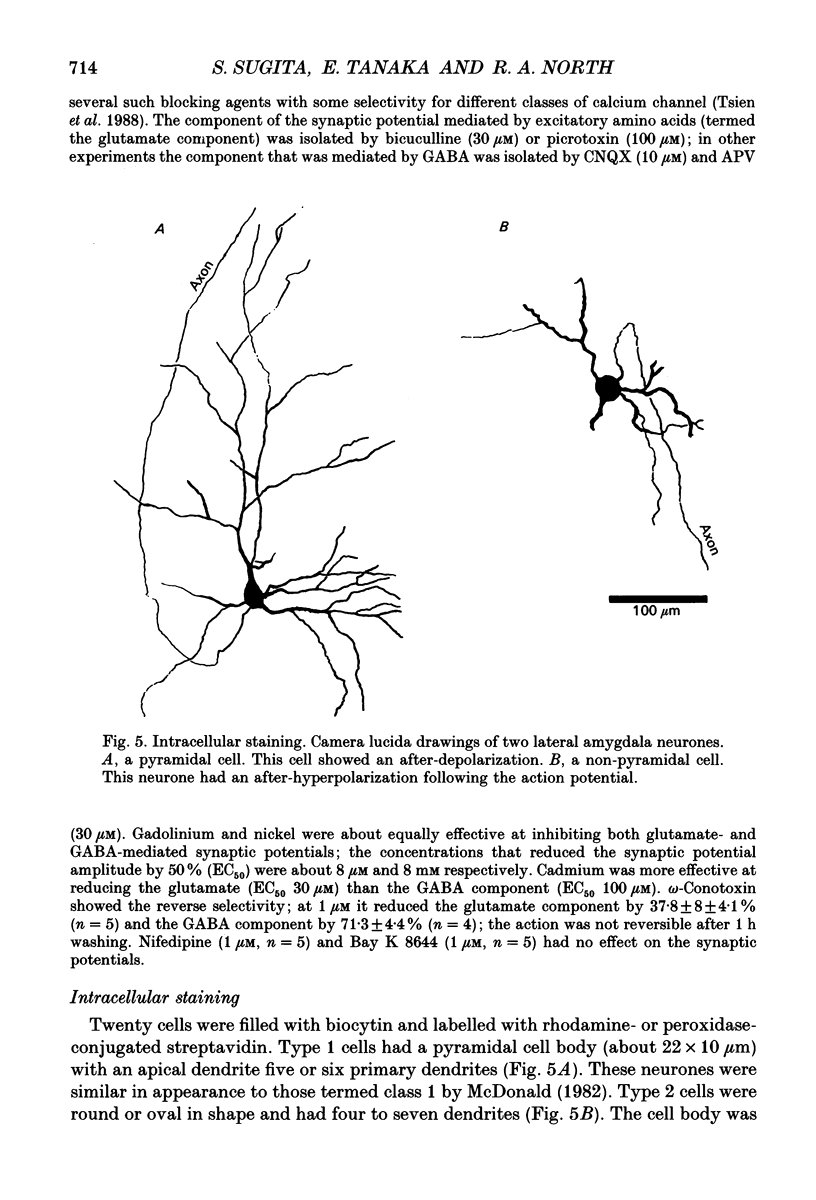
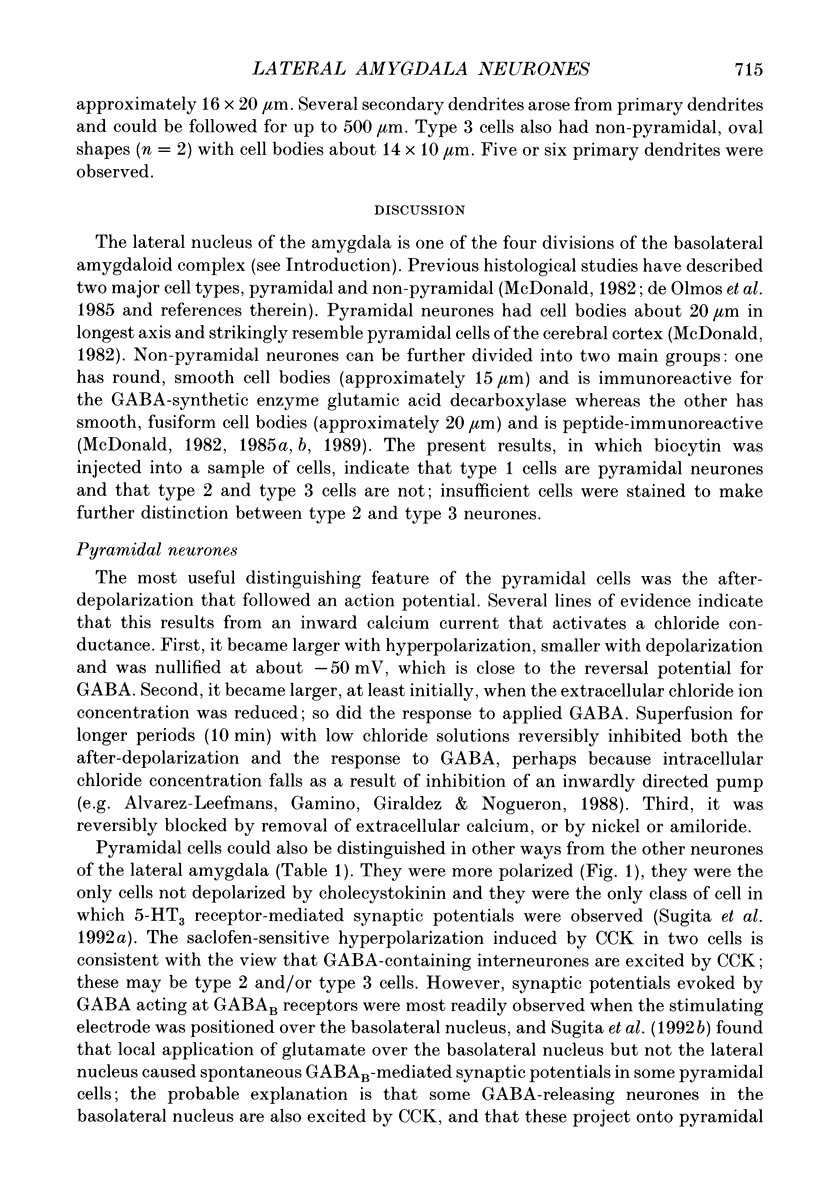
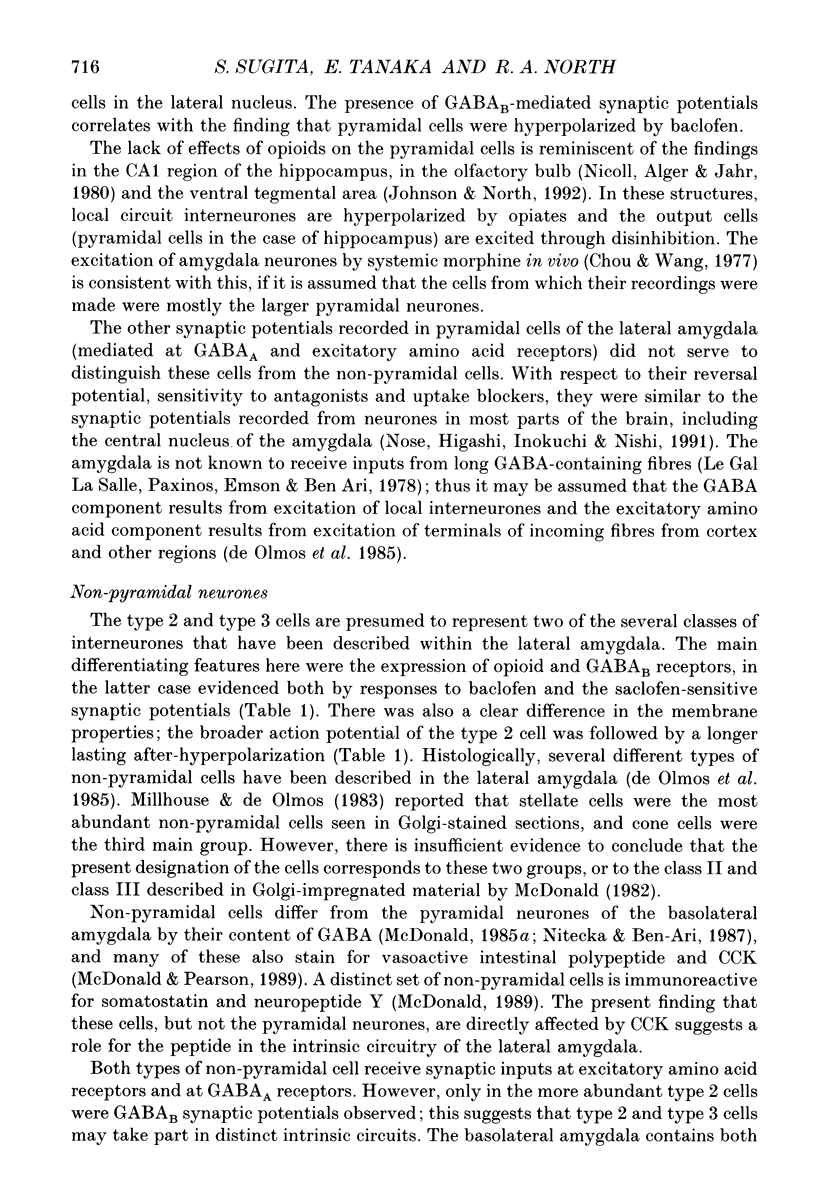
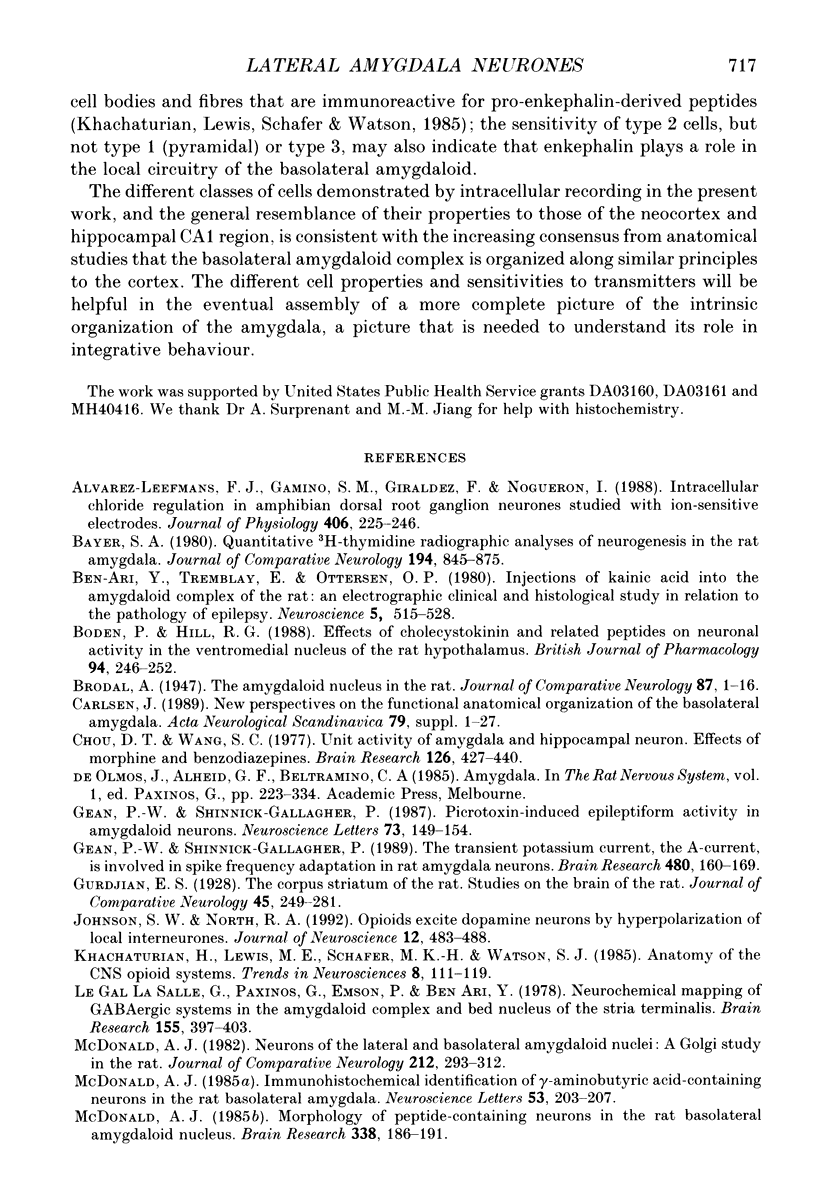
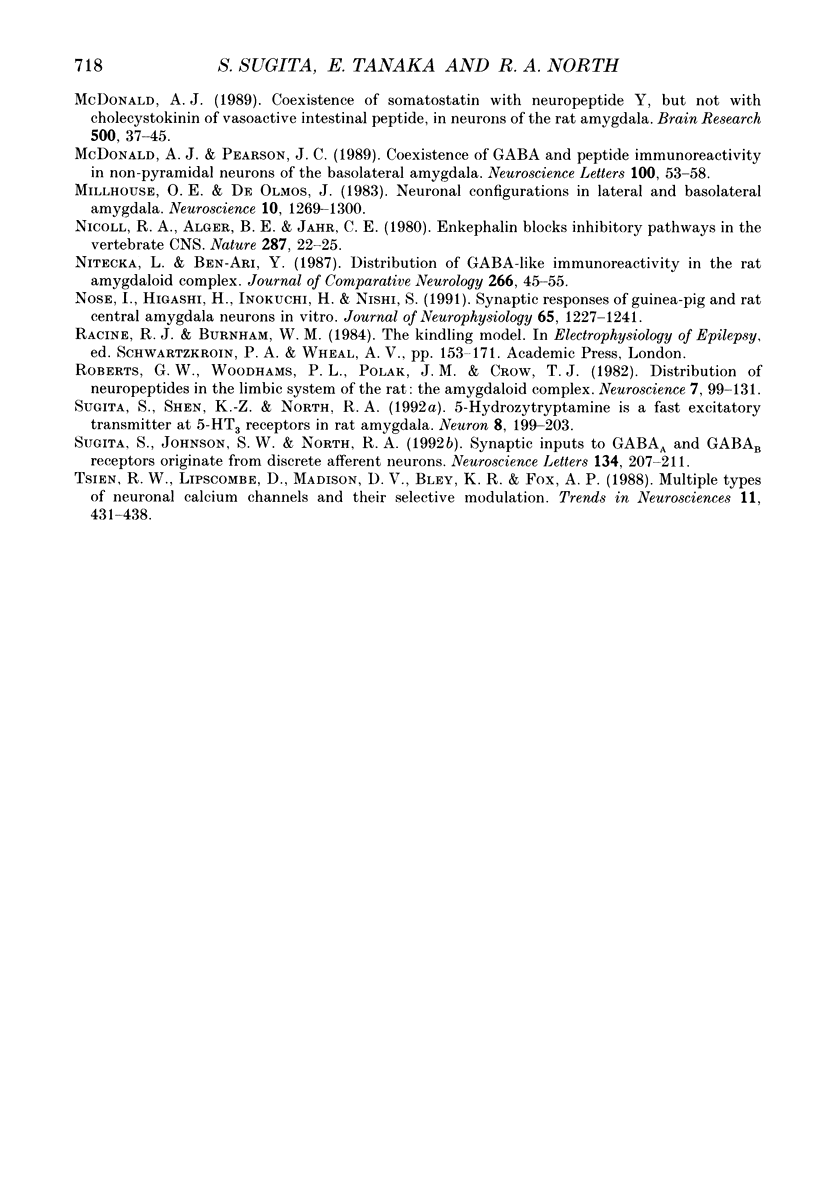
Selected References
These references are in PubMed. This may not be the complete list of references from this article.
- Alvarez-Leefmans F. J., Gamiño S. M., Giraldez F., Noguerón I. Intracellular chloride regulation in amphibian dorsal root ganglion neurones studied with ion-selective microelectrodes. J Physiol. 1988 Dec;406:225–246. doi: 10.1113/jphysiol.1988.sp017378. [DOI] [PMC free article] [PubMed] [Google Scholar]
- Bayer S. A. Quantitative 3H-thymidine radiographic analyses of neurogenesis in the rat amygdala. J Comp Neurol. 1980 Dec 15;194(4):845–875. doi: 10.1002/cne.901940409. [DOI] [PubMed] [Google Scholar]
- Ben-Ari Y., Tremblay E., Ottersen O. P. Injections of kainic acid into the amygdaloid complex of the rat: an electrographic, clinical and histological study in relation to the pathology of epilepsy. Neuroscience. 1980;5(3):515–528. doi: 10.1016/0306-4522(80)90049-4. [DOI] [PubMed] [Google Scholar]
- Boden P., Hill R. G. Effects of cholecystokinin and related peptides on neuronal activity in the ventromedial nucleus of the rat hypothalamus. Br J Pharmacol. 1988 May;94(1):246–252. doi: 10.1111/j.1476-5381.1988.tb11521.x. [DOI] [PMC free article] [PubMed] [Google Scholar]
- Carlsen J. New perspectives on the functional anatomical organization of the basolateral amygdala. Acta Neurol Scand Suppl. 1989;122:1–27. doi: 10.1111/j.1600-0404.1989.tb08018.x. [DOI] [PubMed] [Google Scholar]
- Chou D. T., Wang S. C. Unit activity of amygdala and hippocampal neurons: effects of morphine and benzodiazepines. Brain Res. 1977 May 13;126(3):427–440. doi: 10.1016/0006-8993(77)90595-9. [DOI] [PubMed] [Google Scholar]
- Gean P. W., Shinnick-Gallagher P. Picrotoxin induced epileptiform activity in amygdaloid neurons. Neurosci Lett. 1987 Jan 14;73(2):149–154. doi: 10.1016/0304-3940(87)90009-7. [DOI] [PubMed] [Google Scholar]
- Gean P. W., Shinnick-Gallagher P. The transient potassium current, the A-current, is involved in spike frequency adaptation in rat amygdala neurons. Brain Res. 1989 Feb 20;480(1-2):160–169. doi: 10.1016/0006-8993(89)91578-3. [DOI] [PubMed] [Google Scholar]
- Johnson S. W., North R. A. Opioids excite dopamine neurons by hyperpolarization of local interneurons. J Neurosci. 1992 Feb;12(2):483–488. doi: 10.1523/JNEUROSCI.12-02-00483.1992. [DOI] [PMC free article] [PubMed] [Google Scholar]
- Le Gal LaSalle G., Paxinos G., Ben-Ari Y. Neurochemical mapping of GABAergic systems in the amygdaloid complex and bed nucleus of the stria terminalis. Brain Res. 1978 Oct 27;155(2):397–403. doi: 10.1016/0006-8993(78)91037-5. [DOI] [PubMed] [Google Scholar]
- McDonald A. J. Coexistence of somatostatin with neuropeptide Y, but not with cholecystokinin or vasoactive intestinal peptide, in neurons of the rat amygdala. Brain Res. 1989 Oct 23;500(1-2):37–45. doi: 10.1016/0006-8993(89)90297-7. [DOI] [PubMed] [Google Scholar]
- McDonald A. J. Immunohistochemical identification of gamma-aminobutyric acid-containing neurons in the rat basolateral amygdala. Neurosci Lett. 1985 Jan 21;53(2):203–207. doi: 10.1016/0304-3940(85)90186-7. [DOI] [PubMed] [Google Scholar]
- McDonald A. J. Morphology of peptide-containing neurons in the rat basolateral amygdaloid nucleus. Brain Res. 1985 Jul 8;338(1):186–191. doi: 10.1016/0006-8993(85)90266-5. [DOI] [PubMed] [Google Scholar]
- McDonald A. J. Neurons of the lateral and basolateral amygdaloid nuclei: a Golgi study in the rat. J Comp Neurol. 1982 Dec 10;212(3):293–312. doi: 10.1002/cne.902120307. [DOI] [PubMed] [Google Scholar]
- McDonald A. J., Pearson J. C. Coexistence of GABA and peptide immunoreactivity in non-pyramidal neurons of the basolateral amygdala. Neurosci Lett. 1989 May 22;100(1-3):53–58. doi: 10.1016/0304-3940(89)90659-9. [DOI] [PubMed] [Google Scholar]
- Millhouse O. E., DeOlmos J. Neuronal configurations in lateral and basolateral amygdala. Neuroscience. 1983 Dec;10(4):1269–1300. doi: 10.1016/0306-4522(83)90112-4. [DOI] [PubMed] [Google Scholar]
- Nicoll R. A., Alger B. E., Jahr C. E. Enkephalin blocks inhibitory pathways in the vertebrate CNS. Nature. 1980 Sep 4;287(5777):22–25. doi: 10.1038/287022a0. [DOI] [PubMed] [Google Scholar]
- Nitecka L., Ben-Ari Y. Distribution of GABA-like immunoreactivity in the rat amygdaloid complex. J Comp Neurol. 1987 Dec 1;266(1):45–55. doi: 10.1002/cne.902660105. [DOI] [PubMed] [Google Scholar]
- Nose I., Higashi H., Inokuchi H., Nishi S. Synaptic responses of guinea pig and rat central amygdala neurons in vitro. J Neurophysiol. 1991 May;65(5):1227–1241. doi: 10.1152/jn.1991.65.5.1227. [DOI] [PubMed] [Google Scholar]
- Roberts G. W., Woodhams P. L., Polak J. M., Crow T. J. Distribution of neuropeptides in the limbic system of the rat: the amygdaloid complex. Neuroscience. 1982 Jan;7(1):99–131. doi: 10.1016/0306-4522(82)90156-7. [DOI] [PubMed] [Google Scholar]
- Sugita S., Johnson S. W., North R. A. Synaptic inputs to GABAA and GABAB receptors originate from discrete afferent neurons. Neurosci Lett. 1992 Jan 6;134(2):207–211. doi: 10.1016/0304-3940(92)90518-c. [DOI] [PubMed] [Google Scholar]
- Sugita S., Shen K. Z., North R. A. 5-hydroxytryptamine is a fast excitatory transmitter at 5-HT3 receptors in rat amygdala. Neuron. 1992 Jan;8(1):199–203. doi: 10.1016/0896-6273(92)90121-s. [DOI] [PubMed] [Google Scholar]
- Tsien R. W., Lipscombe D., Madison D. V., Bley K. R., Fox A. P. Multiple types of neuronal calcium channels and their selective modulation. Trends Neurosci. 1988 Oct;11(10):431–438. doi: 10.1016/0166-2236(88)90194-4. [DOI] [PubMed] [Google Scholar]