Abstract
1. Intracellular free calcium concentration ([Ca2+]i) in isolated non-identified Helix pomatia neurones has been clamped at different physiologically significant levels by a feedback system between the fluorescent signal of fura-2 probe loaded into the cell and ionophoretic injection of Ca2+ ions through a CaCl2-loaded microelectrode. The membrane potential of the neurone has also been clamped using a conventional two-microelectrode method. 2. Special measurements have shown that the transport indices of injecting microelectrodes filled with 50 mM CaCl2 are quite variable (0.11 +/- 0.06, mean +/- S.D.). However, for each electrode the transport indices remained stable during several injection trials into a solution drop having the size of a neurone. The spread of calcium ions from the tip of the microelectrode across the cytosol of the neurone terminated within 2-4 s. The spatial difference in [Ca2+]i at this time did not exceed 10%. 3. Clamping of [Ca2+]i at a new increased level was accompanied by a transient of the Ca(2+)-injecting current. To increase [Ca2+]i by 0.1 microM, the amount of calcium ions injected during this stage had to be 36 +/- 20 microM Ca2+ per cell volume. Obviously, this transient represents the filling of a fast cytosolic buffer which has to be saturated to reach a new increased level of [Ca2+]i. It was followed by a steady component of Ca(2+)-injecting current, which was quite low (corresponding to injection of 0.39 +/- 0.20 microM s-1 for a 0.1 microM change of [Ca2+]i). This may represent the functioning of Ca(2+)-eliminating systems and corresponds to a similar amount of Ca2+ extruded from the cytoplasm. 4. Changes in the injection current also developed when Ca2+ influx through the membrane was triggered by the activation of voltage-gated calcium channels. The amount of Ca2+ entering the cell during the first seconds of depolarization to--15 mV was equal to 0.59 +/- 0.31 microM s-1 per cell volume. 5. No activation of Ca(2+)-dependent potassium current was observed during the changes in [Ca2+]i to levels exceeding the basal one by several times. Obviously, to activate this current, a much stronger increase in [Ca2+]i is needed in the immediate vicinity of the corresponding channels.
Full text
PDF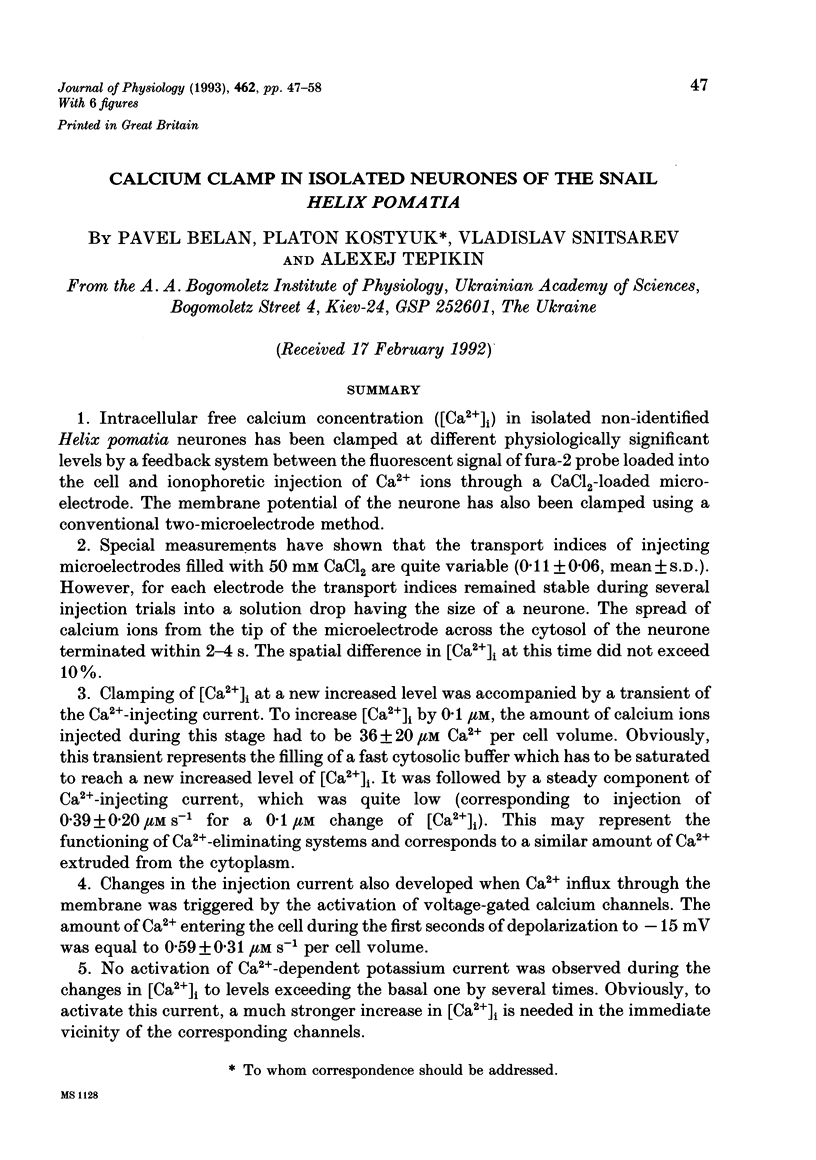
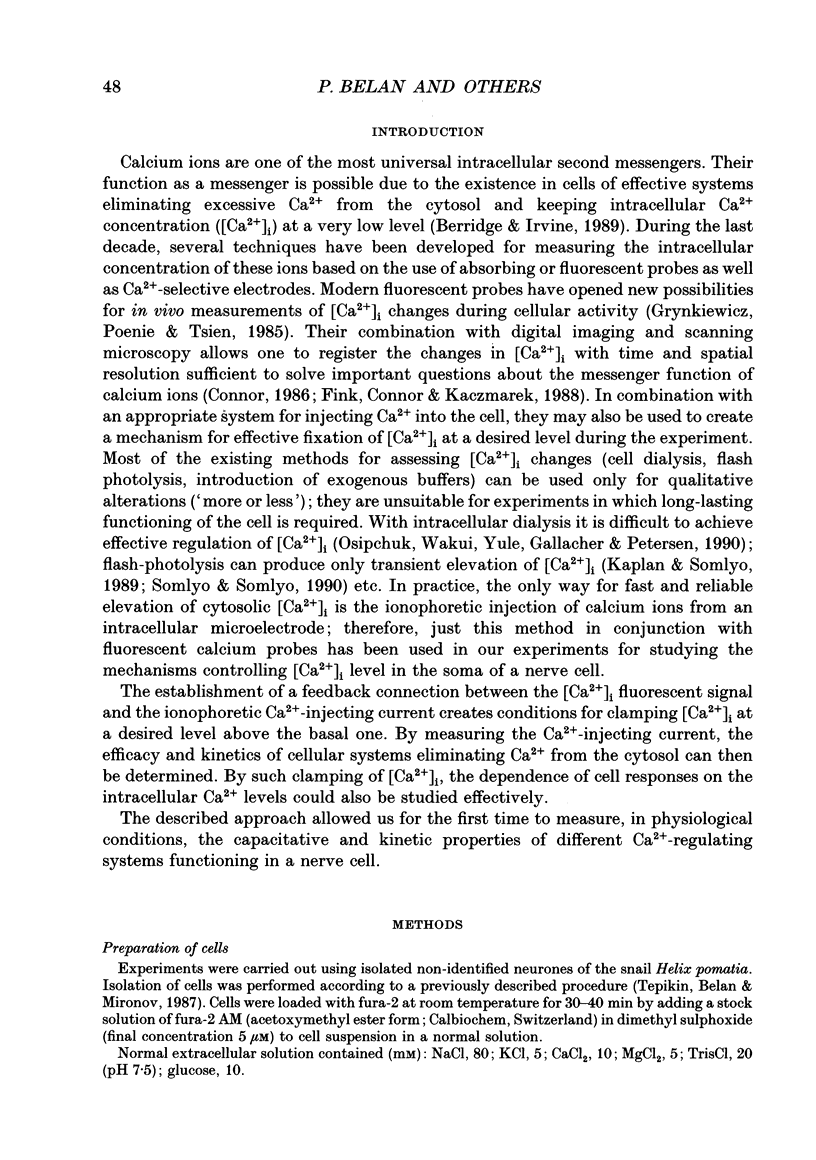
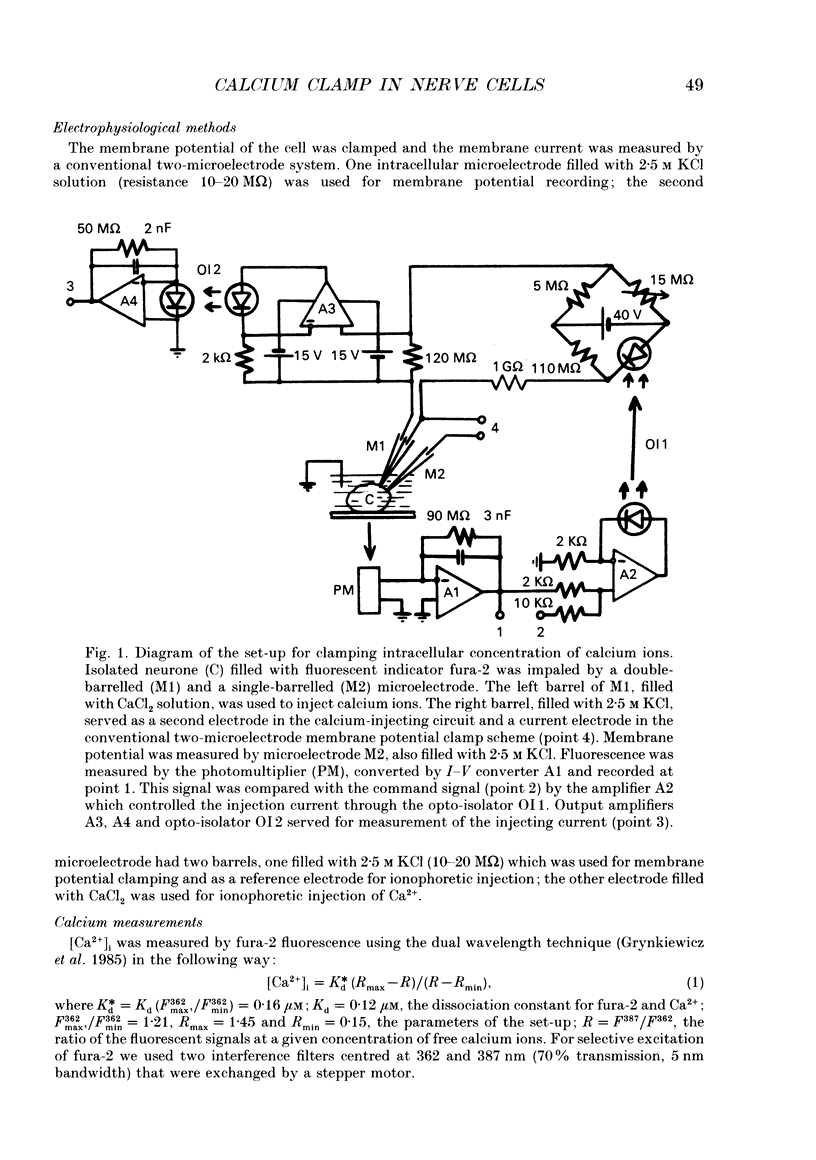
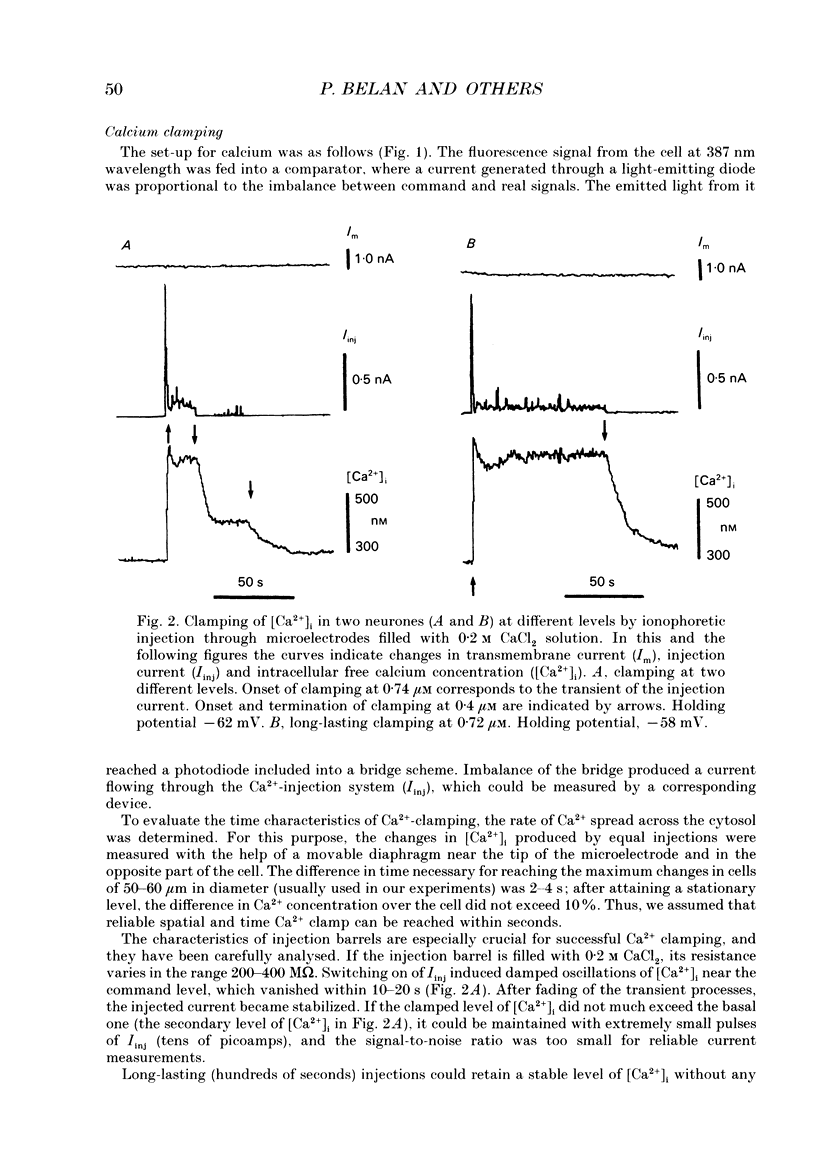
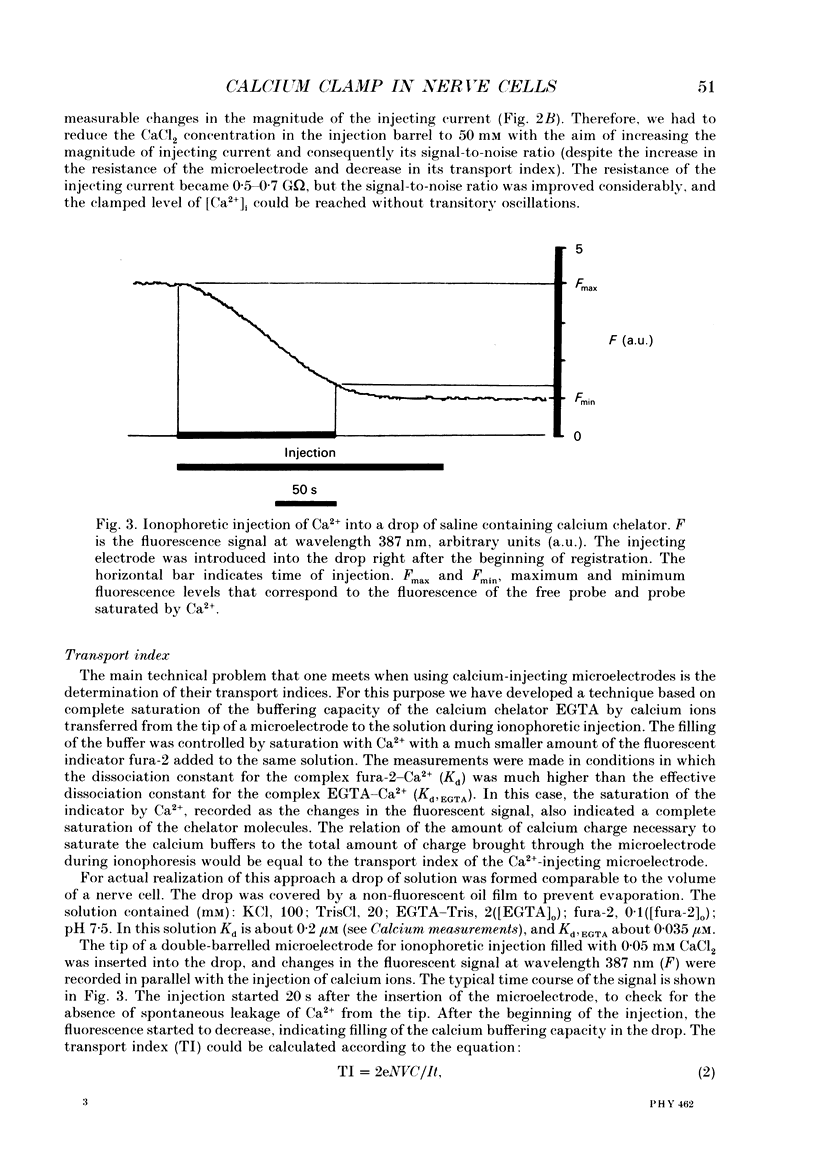
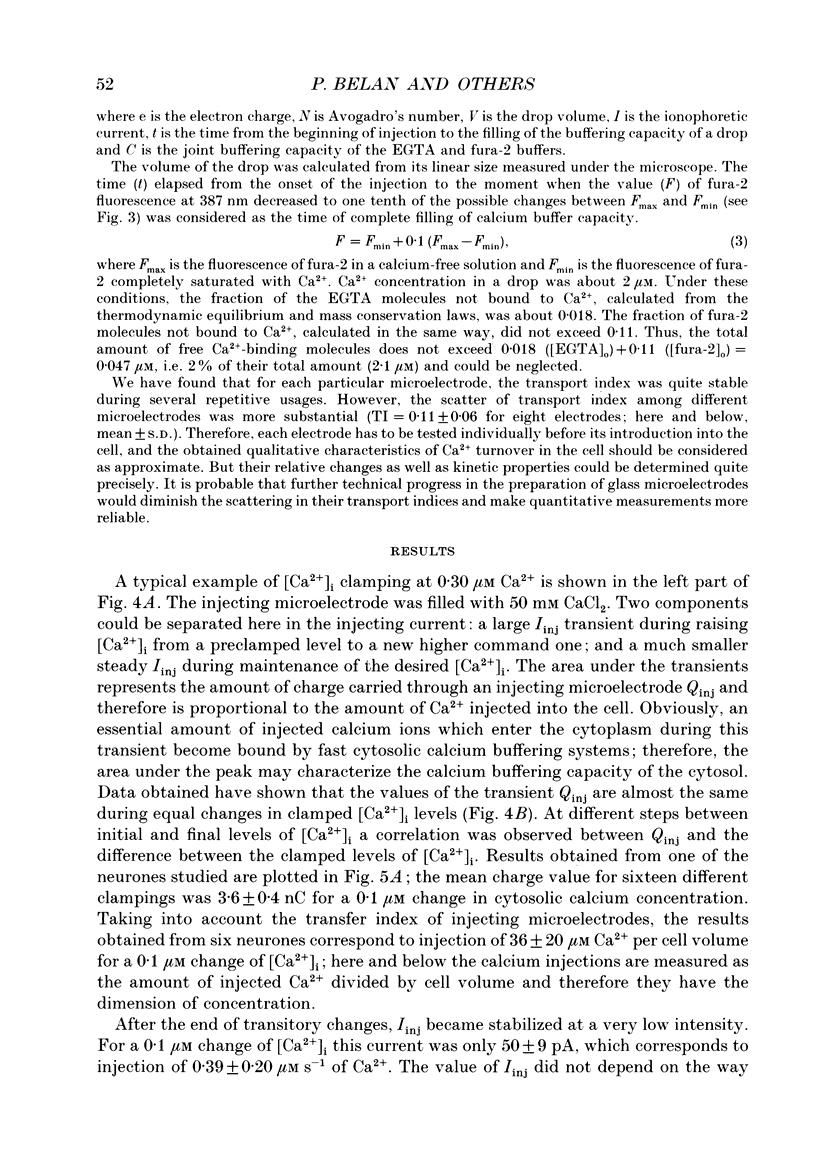
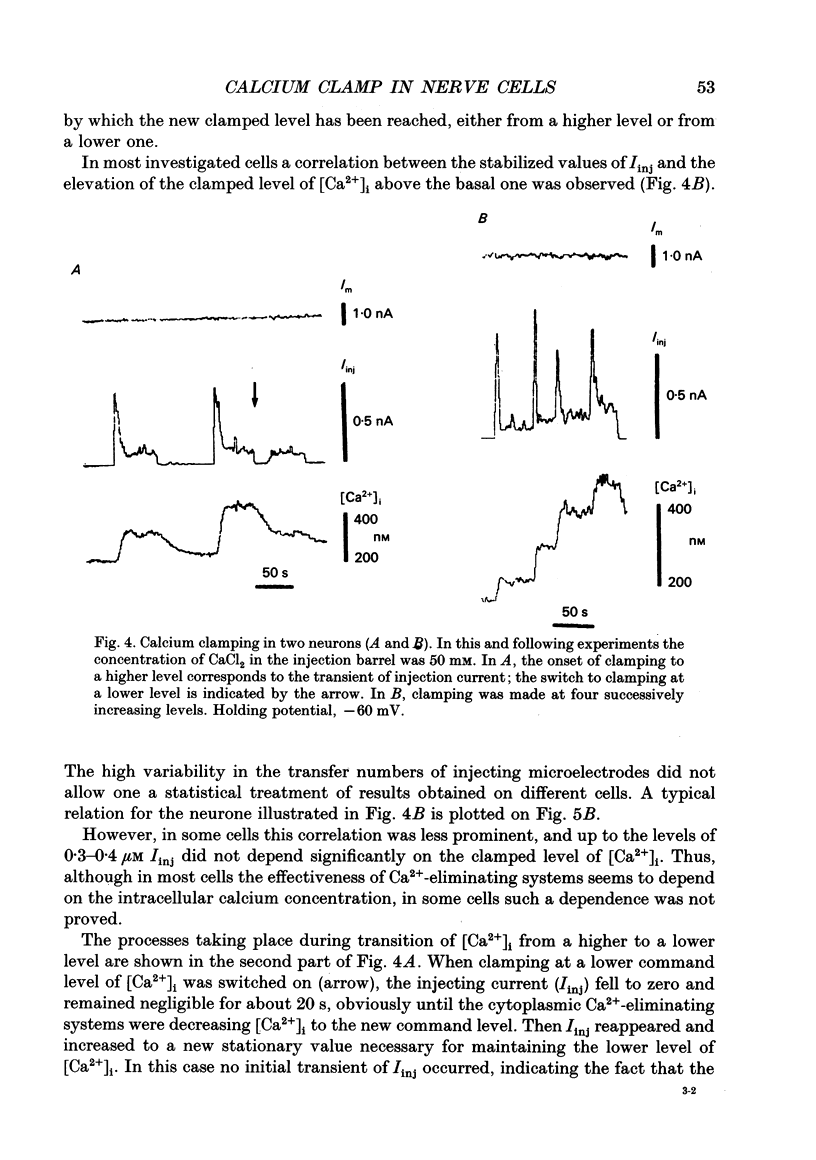
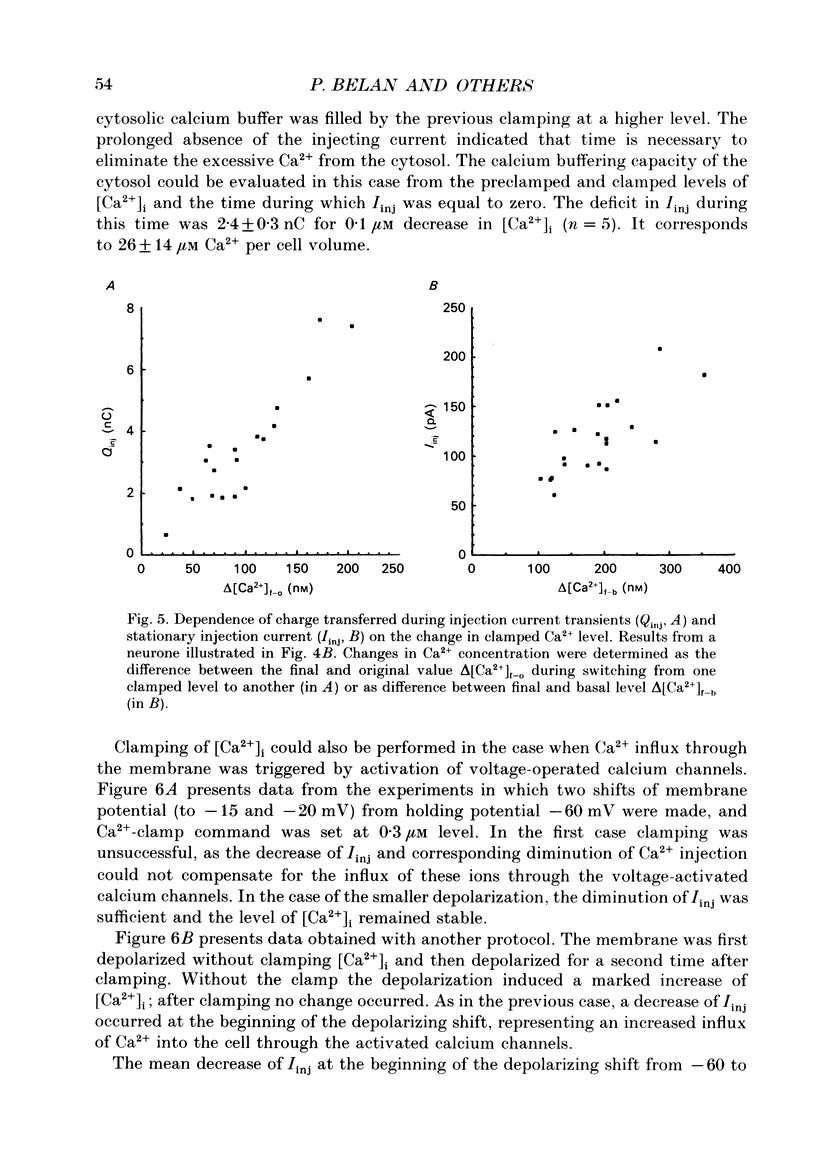
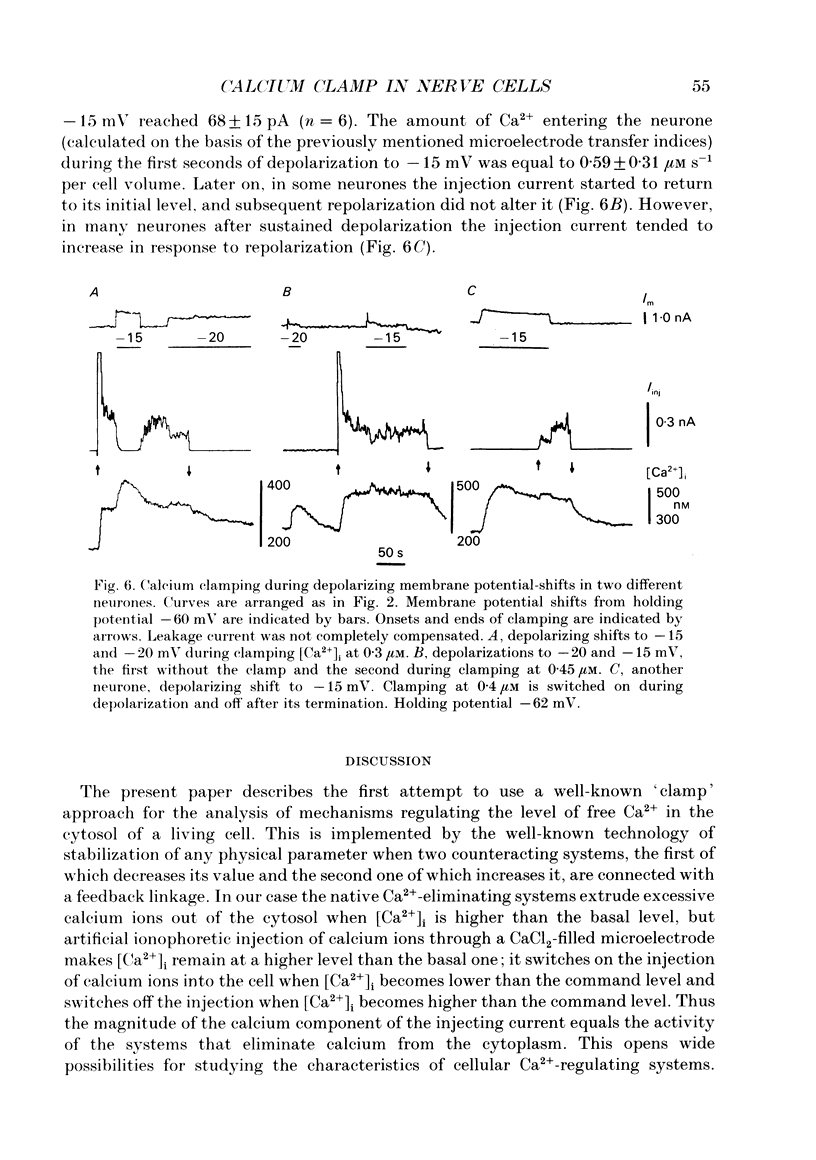
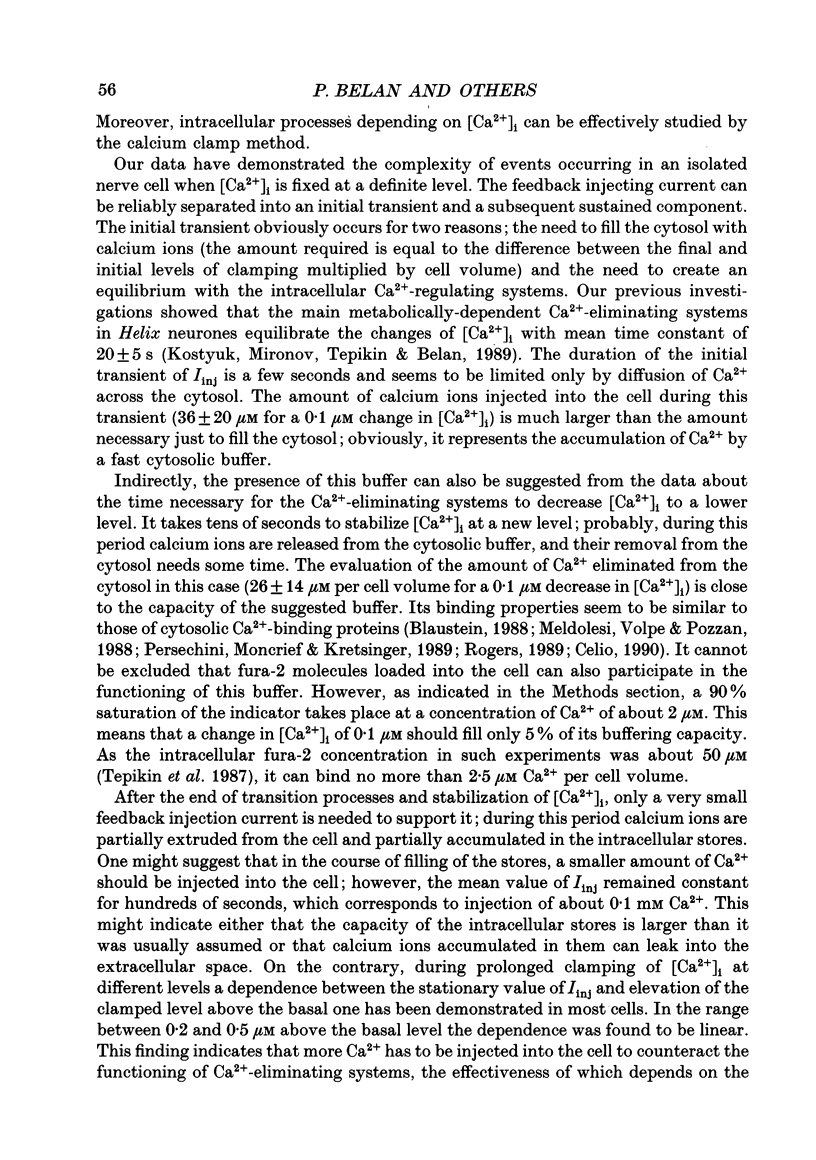
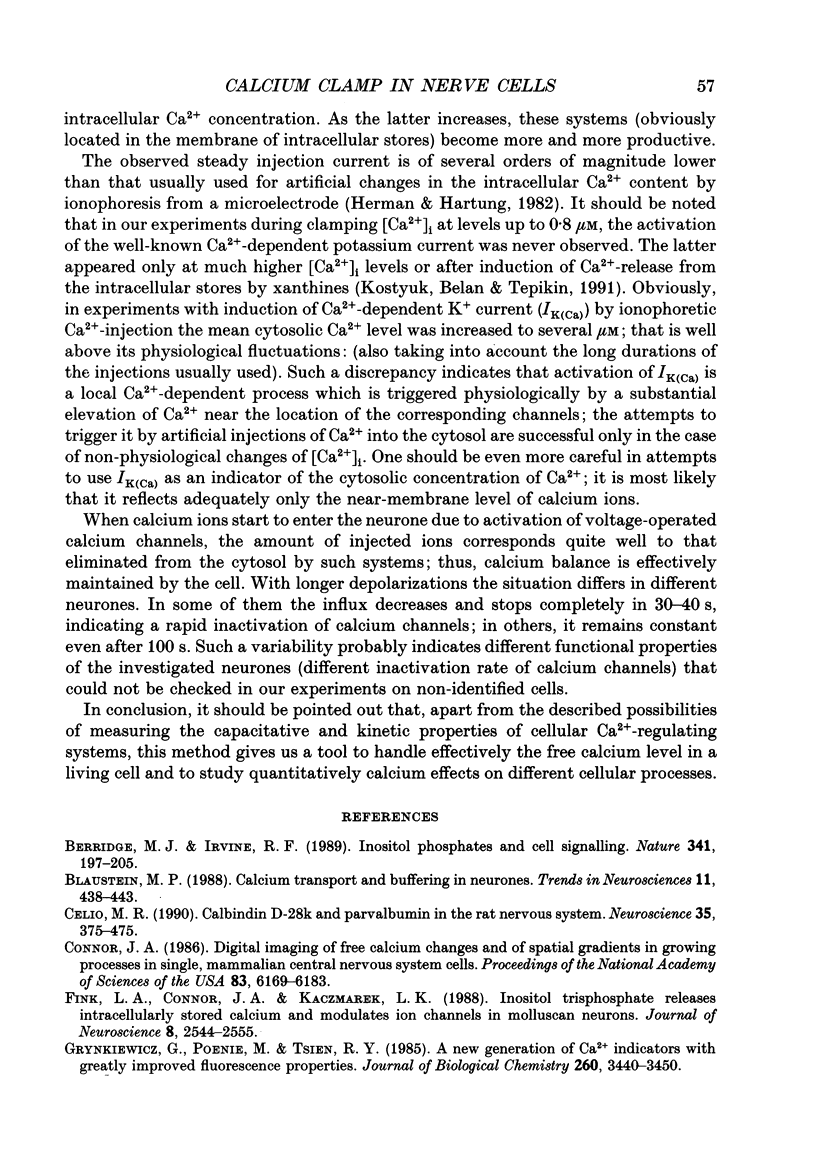
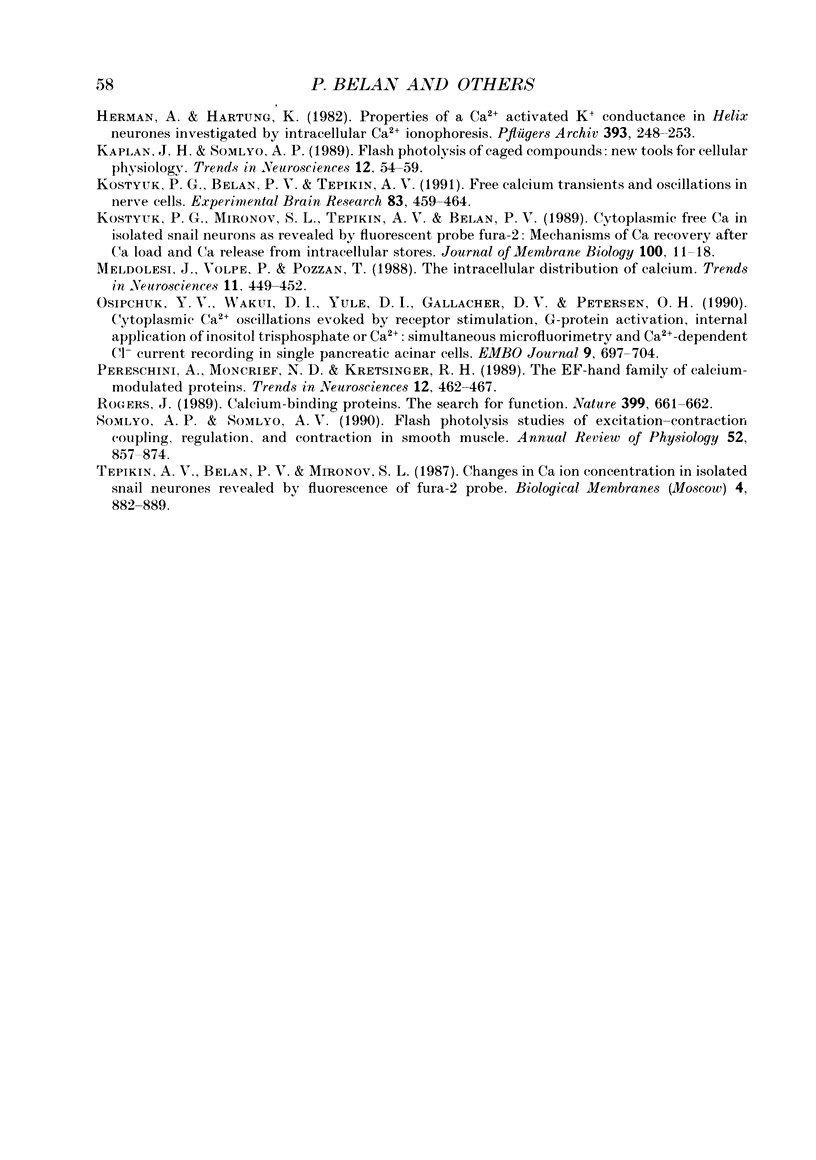
Selected References
These references are in PubMed. This may not be the complete list of references from this article.
- Berridge M. J., Irvine R. F. Inositol phosphates and cell signalling. Nature. 1989 Sep 21;341(6239):197–205. doi: 10.1038/341197a0. [DOI] [PubMed] [Google Scholar]
- Celio M. R. Calbindin D-28k and parvalbumin in the rat nervous system. Neuroscience. 1990;35(2):375–475. doi: 10.1016/0306-4522(90)90091-h. [DOI] [PubMed] [Google Scholar]
- Connor J. A. Digital imaging of free calcium changes and of spatial gradients in growing processes in single, mammalian central nervous system cells. Proc Natl Acad Sci U S A. 1986 Aug;83(16):6179–6183. doi: 10.1073/pnas.83.16.6179. [DOI] [PMC free article] [PubMed] [Google Scholar]
- Fink L. A., Connor J. A., Kaczmarek L. K. Inositol trisphosphate releases intracellularly stored calcium and modulates ion channels in molluscan neurons. J Neurosci. 1988 Jul;8(7):2544–2555. doi: 10.1523/JNEUROSCI.08-07-02544.1988. [DOI] [PMC free article] [PubMed] [Google Scholar]
- Grynkiewicz G., Poenie M., Tsien R. Y. A new generation of Ca2+ indicators with greatly improved fluorescence properties. J Biol Chem. 1985 Mar 25;260(6):3440–3450. [PubMed] [Google Scholar]
- Hermann A., Hartung K. Properties of a Ca2+ activated K+ conductance in Helix neurones investigated by intracellular Ca2+ ionophoresis. Pflugers Arch. 1982 May;393(3):248–253. doi: 10.1007/BF00584078. [DOI] [PubMed] [Google Scholar]
- Kostyuk P. G., Belan P. V., Tepikin A. V. Free calcium transients and oscillations in nerve cells. Exp Brain Res. 1991;83(2):459–464. doi: 10.1007/BF00231173. [DOI] [PubMed] [Google Scholar]
- Meldolesi J., Volpe P., Pozzan T. The intracellular distribution of calcium. Trends Neurosci. 1988 Oct;11(10):449–452. doi: 10.1016/0166-2236(88)90197-x. [DOI] [PubMed] [Google Scholar]
- Osipchuk Y. V., Wakui M., Yule D. I., Gallacher D. V., Petersen O. H. Cytoplasmic Ca2+ oscillations evoked by receptor stimulation, G-protein activation, internal application of inositol trisphosphate or Ca2+: simultaneous microfluorimetry and Ca2+ dependent Cl- current recording in single pancreatic acinar cells. EMBO J. 1990 Mar;9(3):697–704. doi: 10.1002/j.1460-2075.1990.tb08162.x. [DOI] [PMC free article] [PubMed] [Google Scholar]
- Persechini A., Moncrief N. D., Kretsinger R. H. The EF-hand family of calcium-modulated proteins. Trends Neurosci. 1989 Nov;12(11):462–467. doi: 10.1016/0166-2236(89)90097-0. [DOI] [PubMed] [Google Scholar]
- Somlyo A. P., Somlyo A. V. Flash photolysis studies of excitation-contraction coupling, regulation, and contraction in smooth muscle. Annu Rev Physiol. 1990;52:857–874. doi: 10.1146/annurev.ph.52.030190.004233. [DOI] [PubMed] [Google Scholar]