Abstract
1. Lactate and H+ efflux from skeletal muscles were studied with the one-legged knee extension model under conditions in which blood flow, arterial lactate and the muscle-blood lactate concentration gradient were altered. Subjects exercised one leg twice to exhaustion (EX1, EX2), separated by a 10 min recovery and a period of intense intermittent exercise. After 1 h of recovery the exercise protocol was repeated with the other leg. Low-intensity exercise was performed with one leg during the recovery periods, while the other leg was passive during its recovery periods. 2. Prior to, and immediately after, EX1 and EX2 and then 3 and 10 min after EX1, a biopsy was taken from the vastus lateralis of the exercised leg for lactate, pH, muscle water and fibre-type determinations. Measurements of leg blood flow and venous-arterial differences for lactate (whole blood and plasma), pH, partial pressure of CO2 (PCO2), haemoglobin, saturation and base excess (BE) were performed at the end of exercise and regularly during the recovery period after EX1. 3. The lactate release was linearly related (r = 0.96; P < 0.05) to the muscle lactate gradient over a range of muscle lactate from 0 to 45 mmol (kg wet wt)-1. The muscle lactate transport was evaluated from the net femoral venous-arterial differences (V-Adiff) for lactate. This rose with increases in the muscle lactate gradients, but as the gradient reached higher levels the V-Adiff lactate responded less than at smaller gradients. Thus, the lactate transport over the muscle membrane appears to be partly saturated at high muscle lactate concentrations. 4. The percentage of slow twitch (%ST) fibres was inversely related to the muscle lactate gradient, but it was not correlated to the lactate release at the end of the exercises. In spite of a significantly higher blood flow during active recovery, the lactate release was the same whether the leg was resting or performed low-intensity exercise in the recovery periods. In several other conditions the muscle lactate and H+ gradients would have predicted that the V-Adiff lactate would have been greater than it actually was. Thus, a variety of factors affect muscle lactate transport, including arterial lactate concentration, muscle perfusion, muscle contraction pattern and muscle morphology. 5. The muscle and femoral venous pH declined during EX1 to 6.73 and 7.14-7.15, respectively, and they increased to resting levels during 10 min of either passive or active recovery.(ABSTRACT TRUNCATED AT 400 WORDS)
Full text
PDF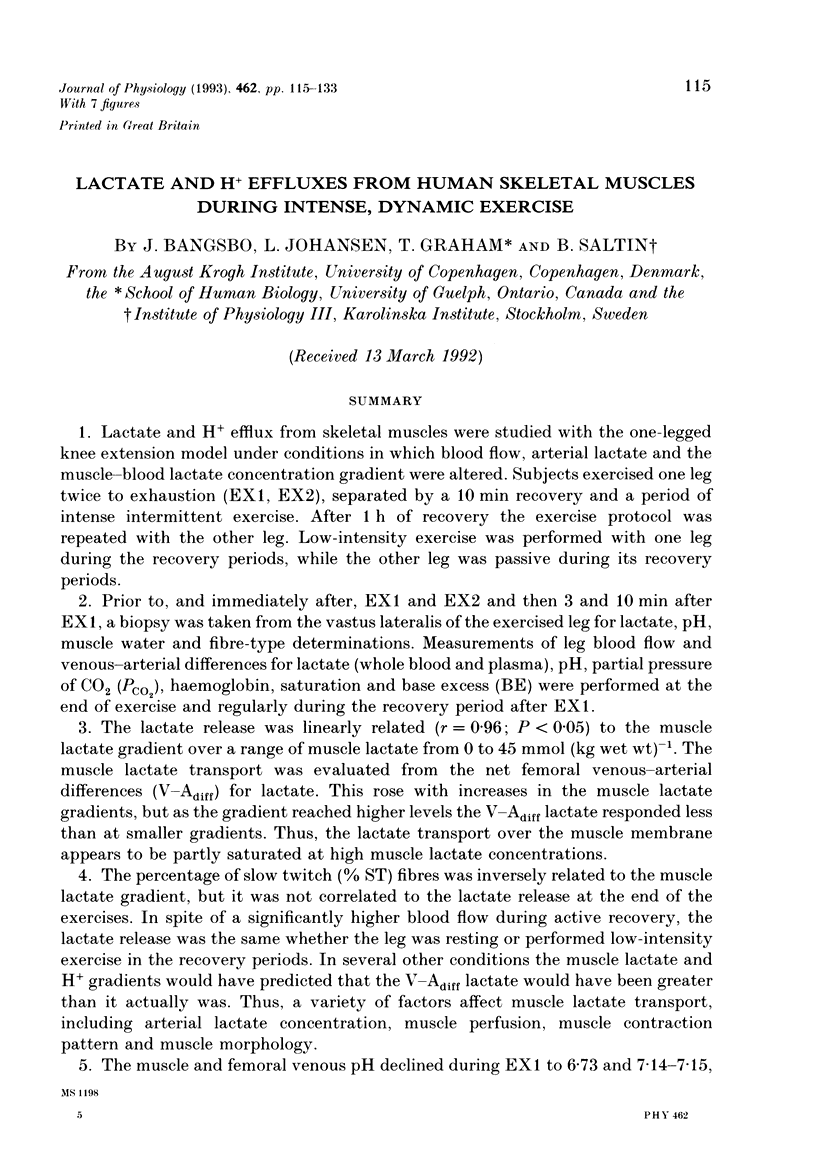
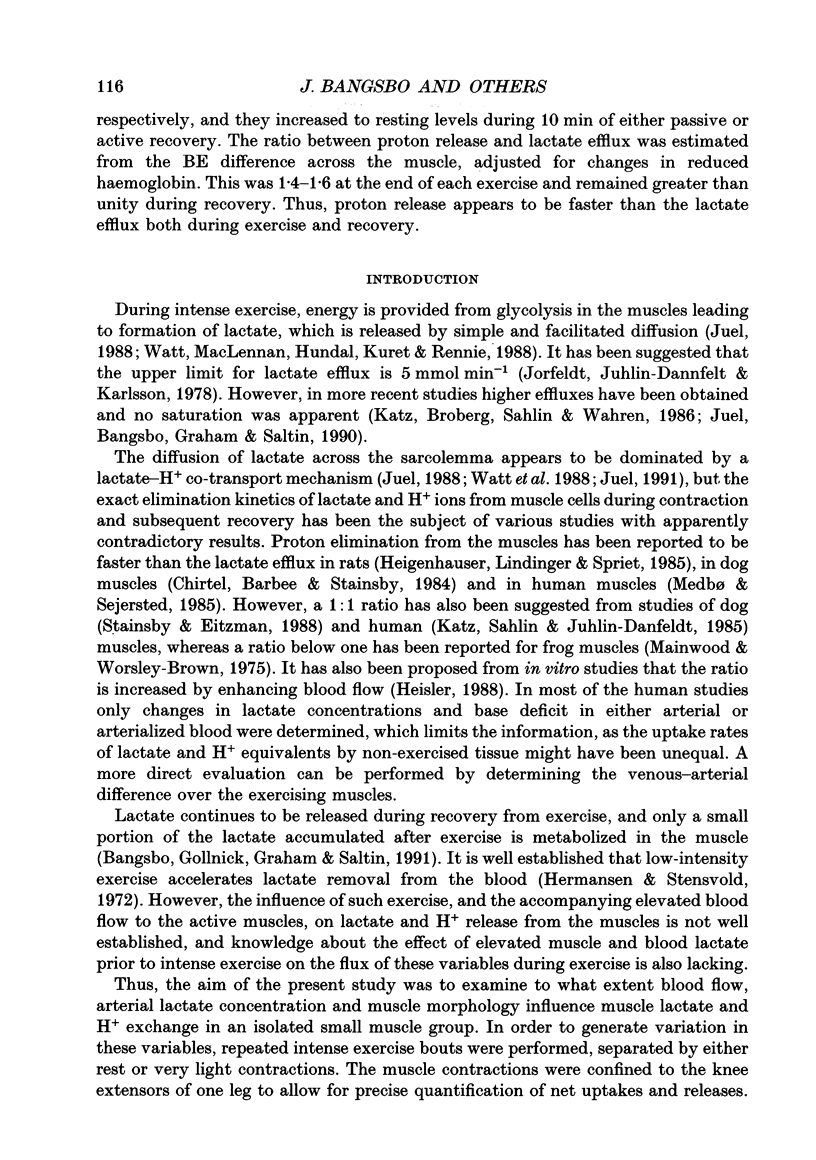
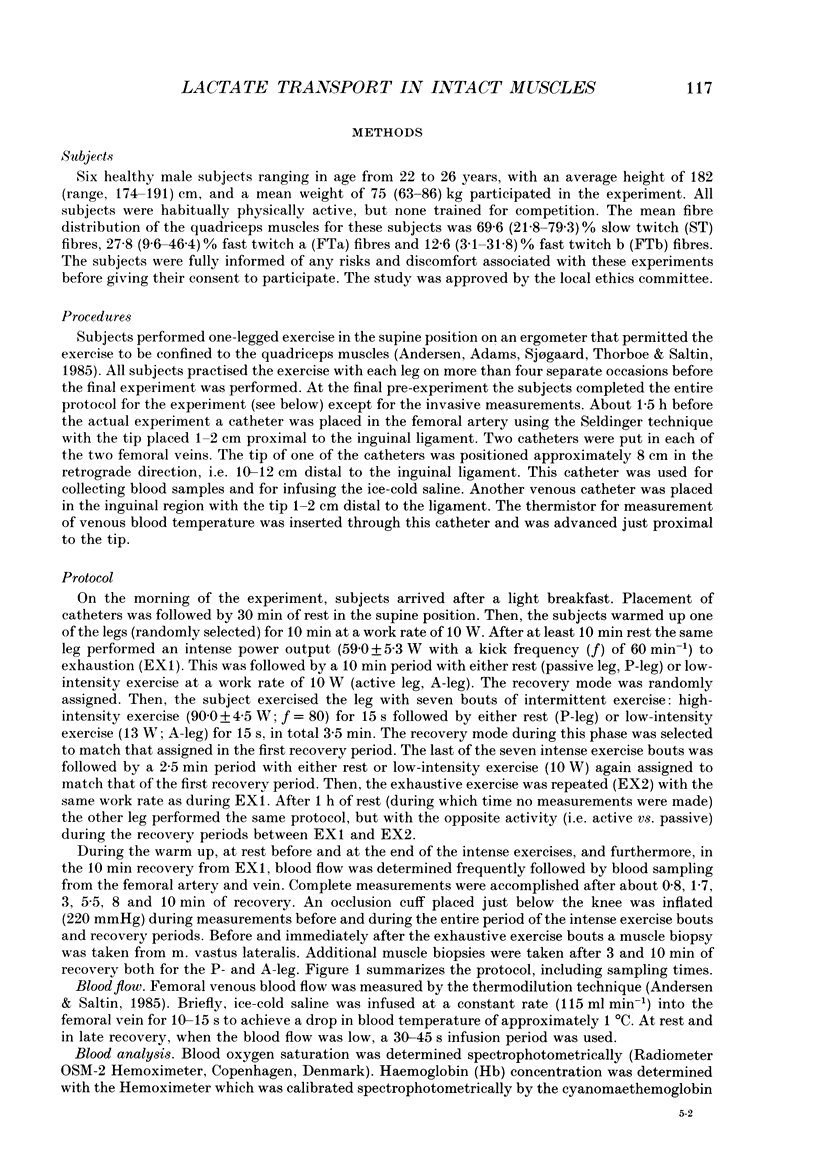
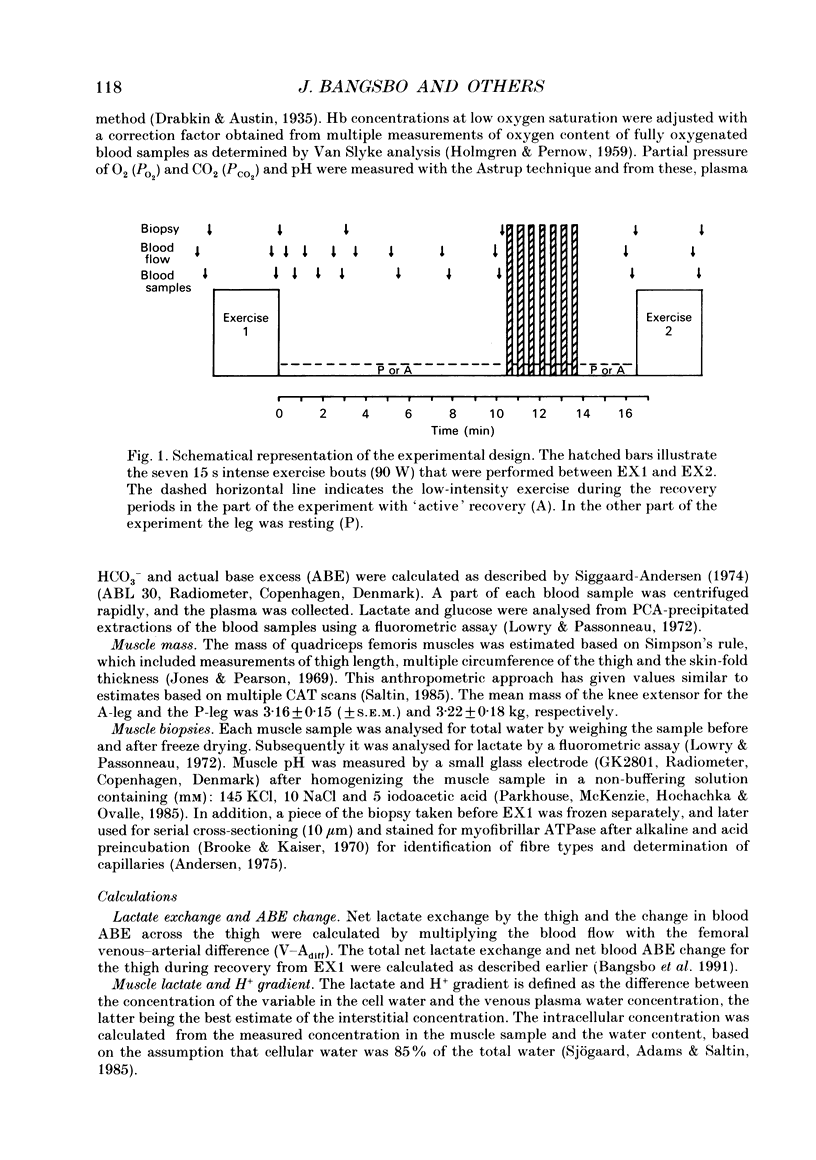
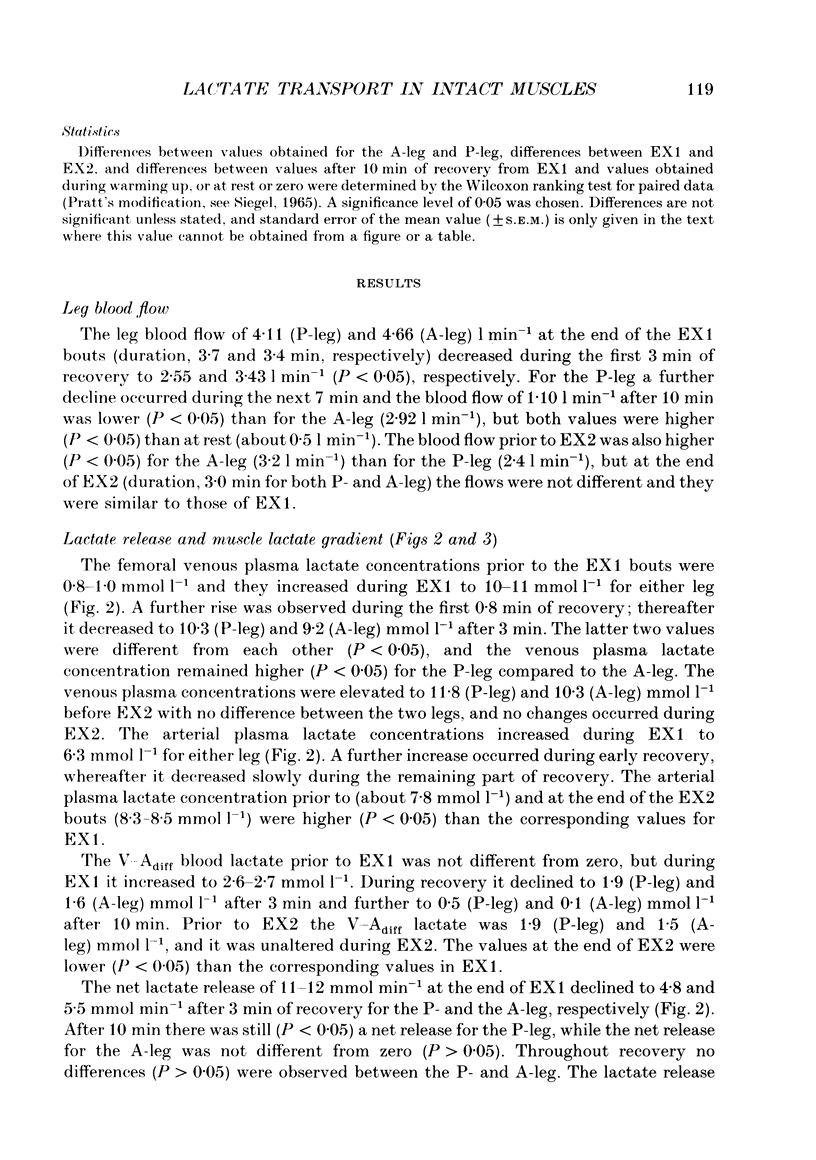
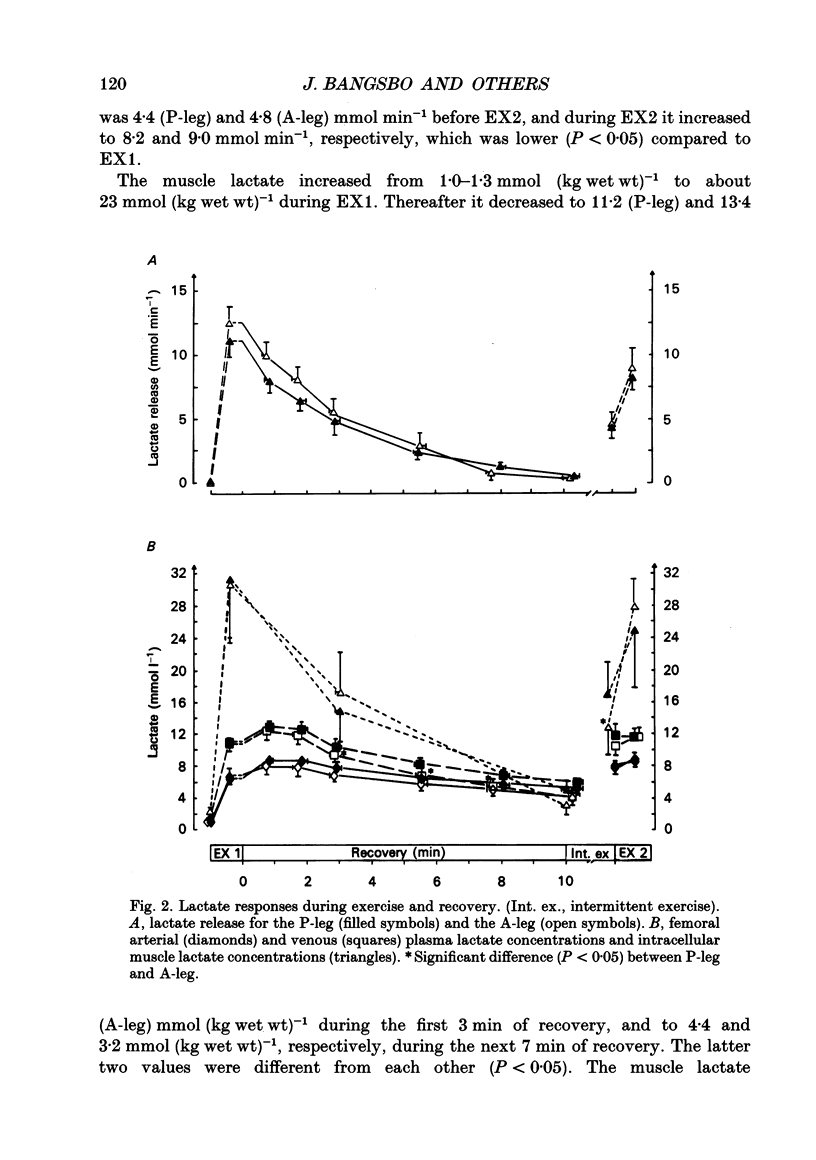
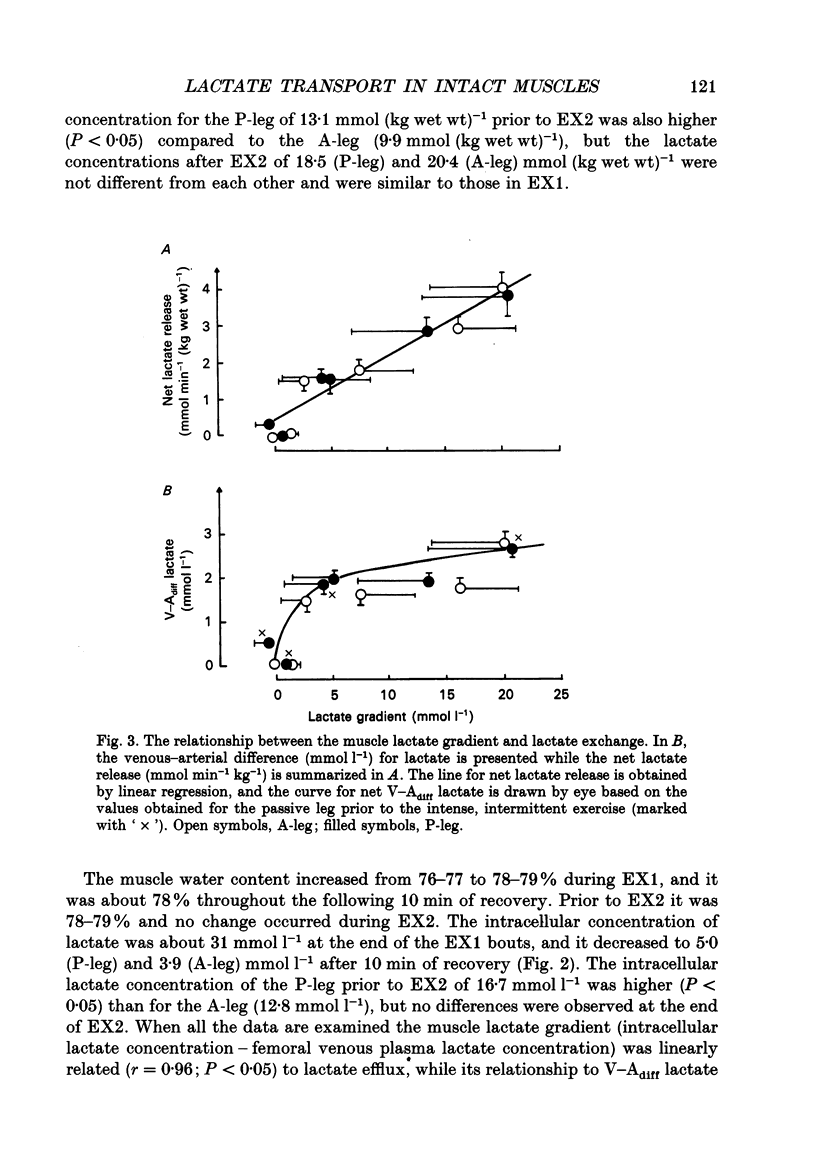
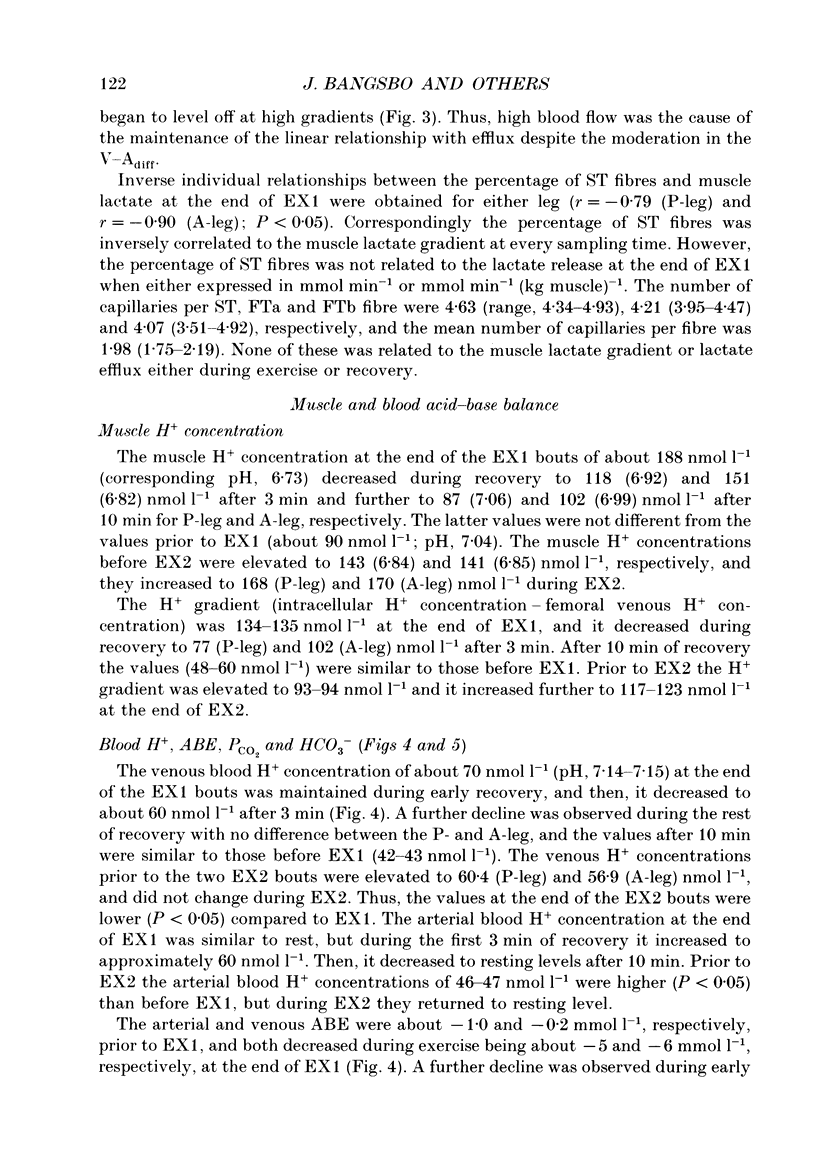
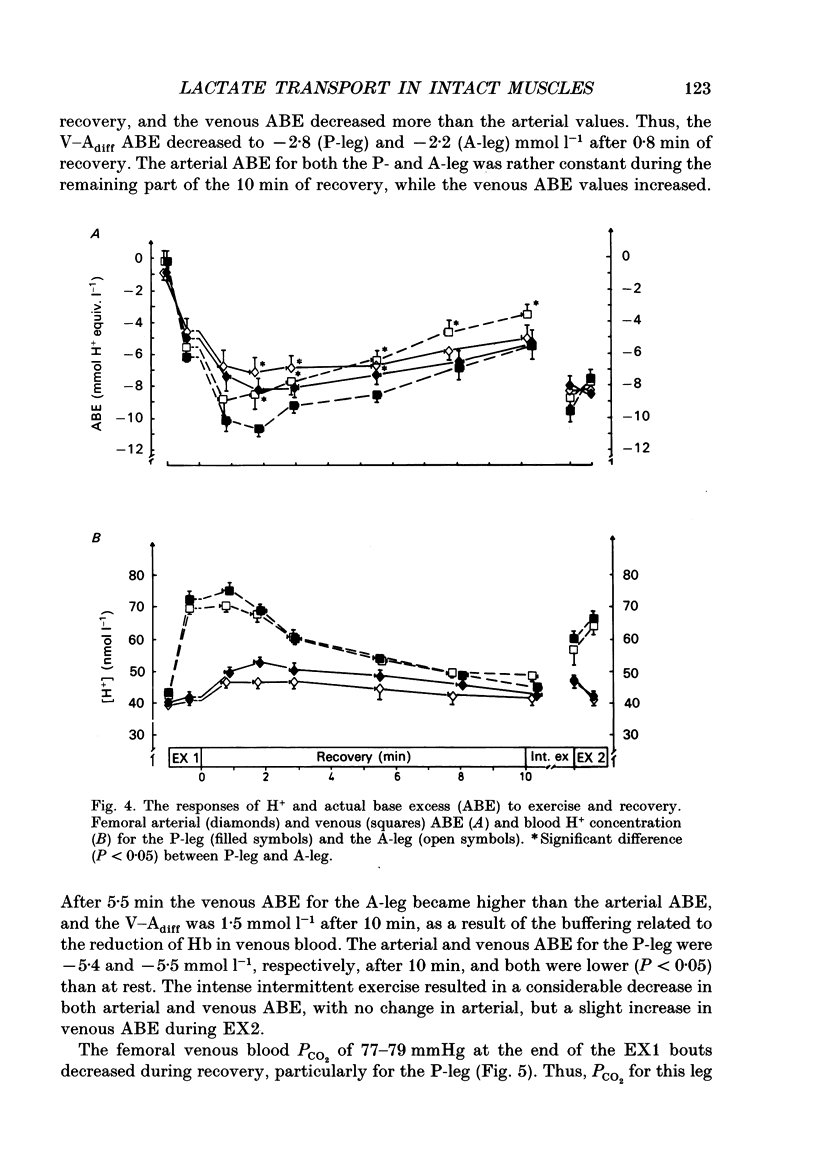
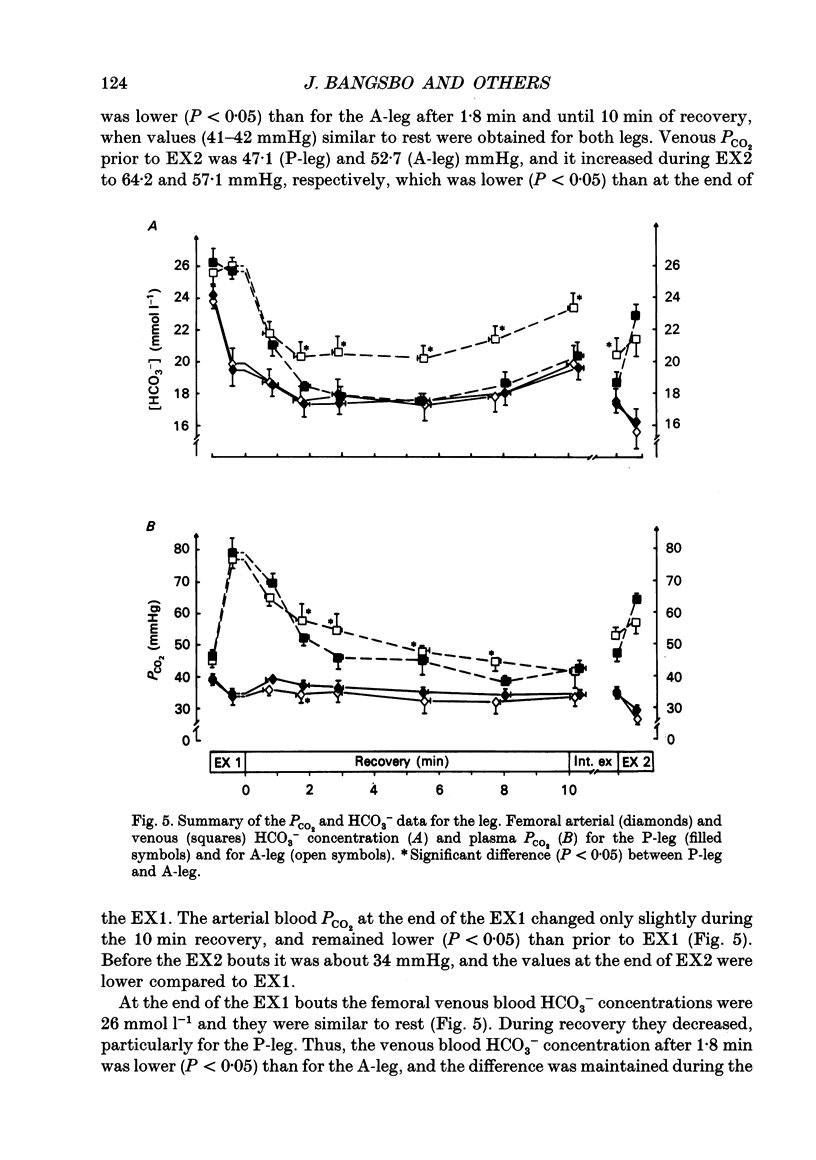
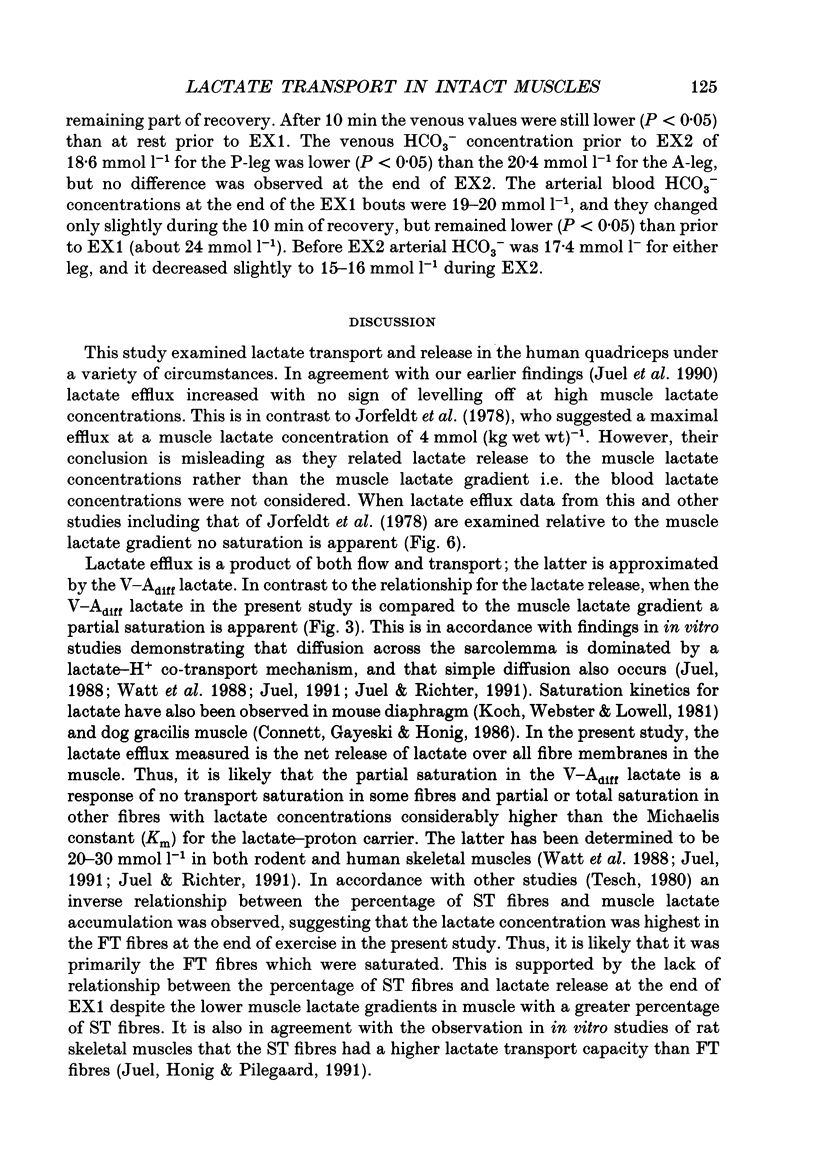
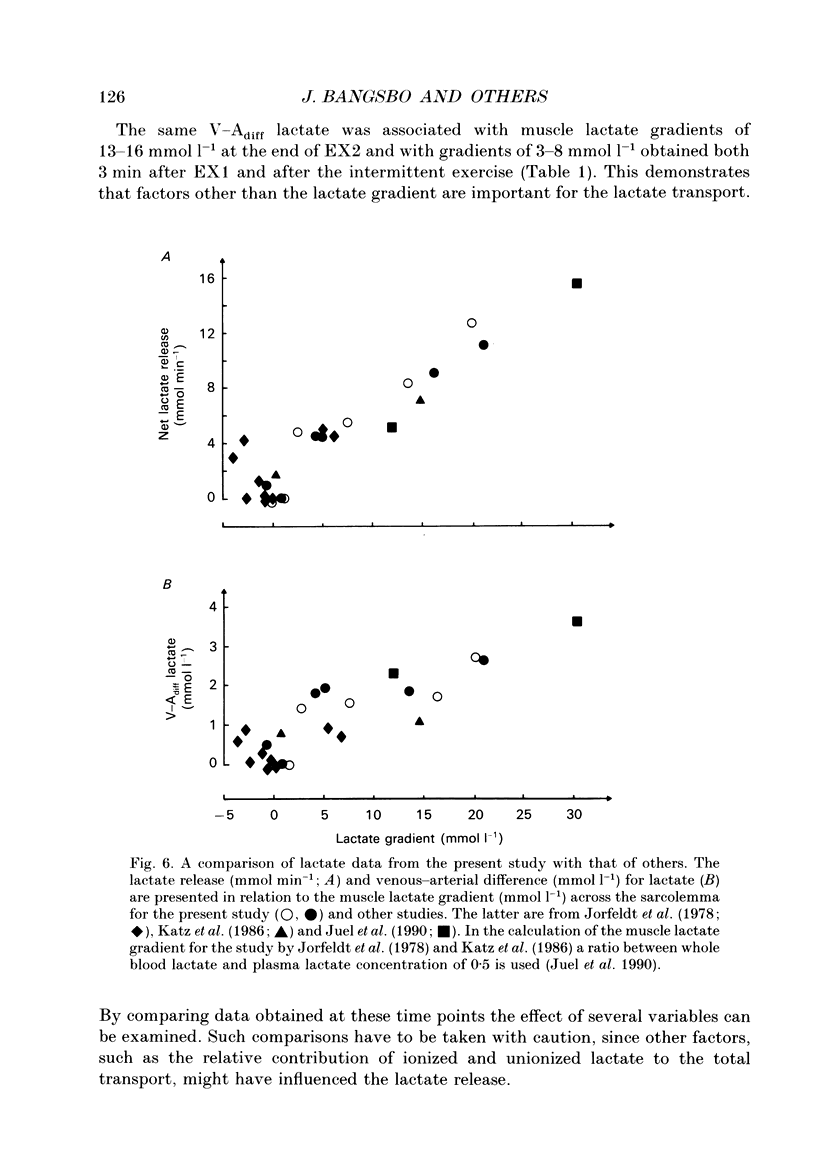
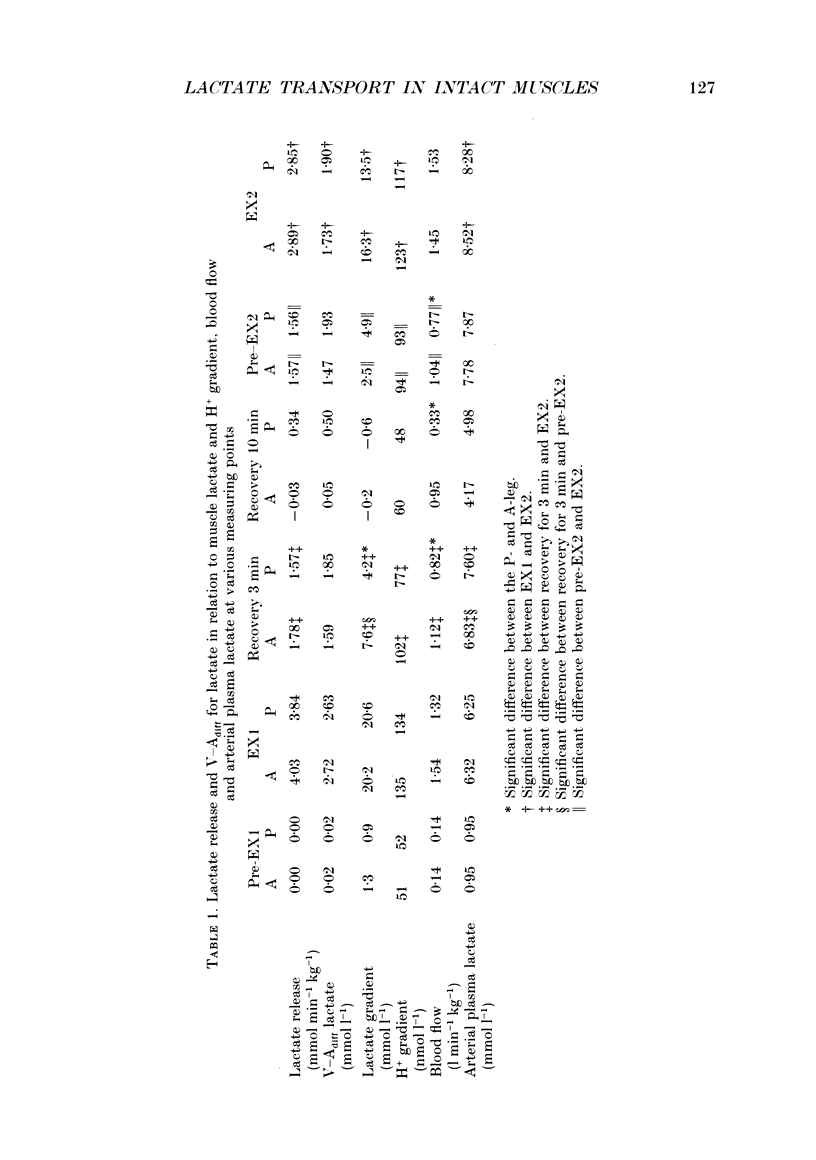
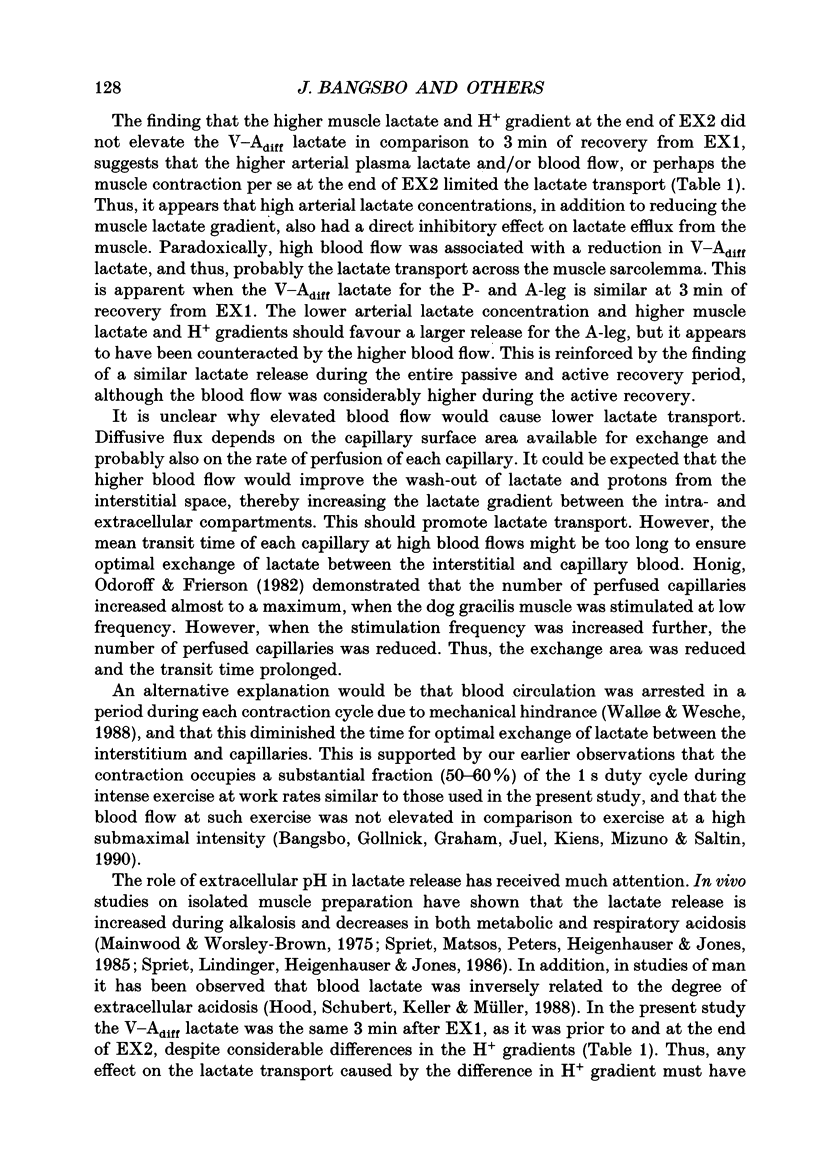
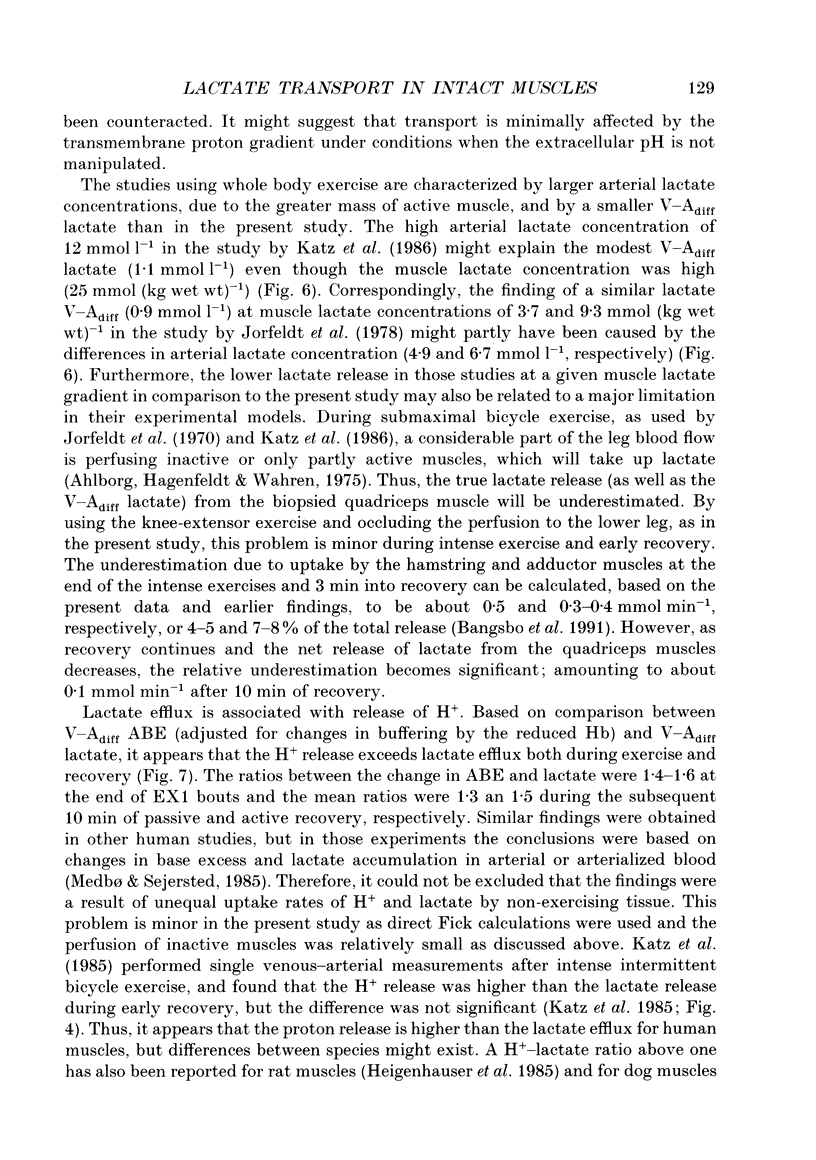
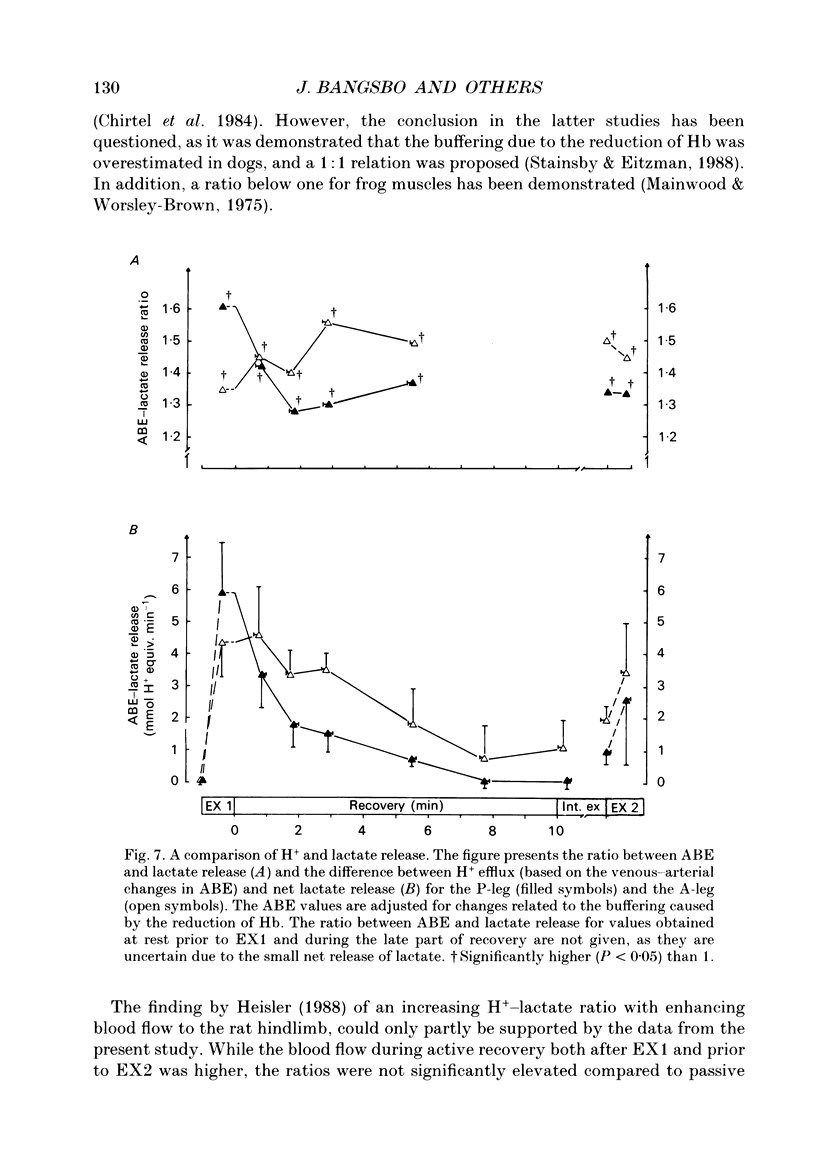
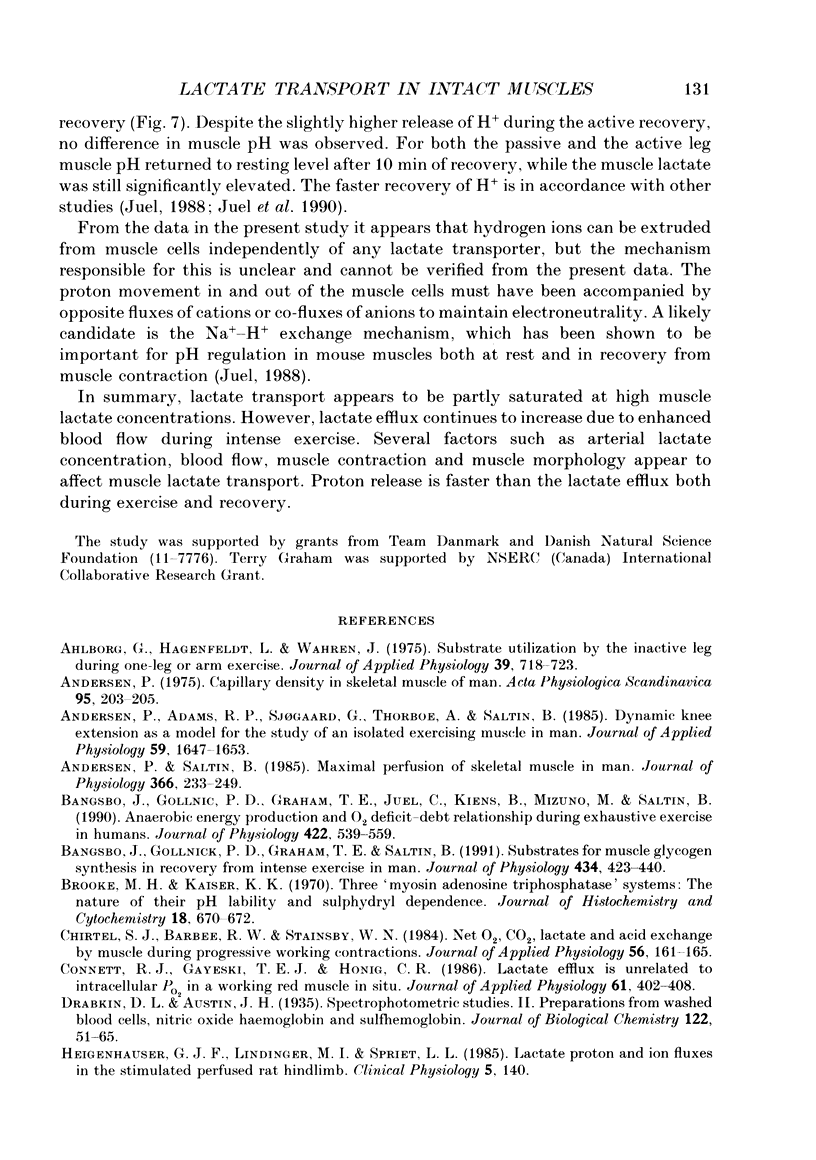
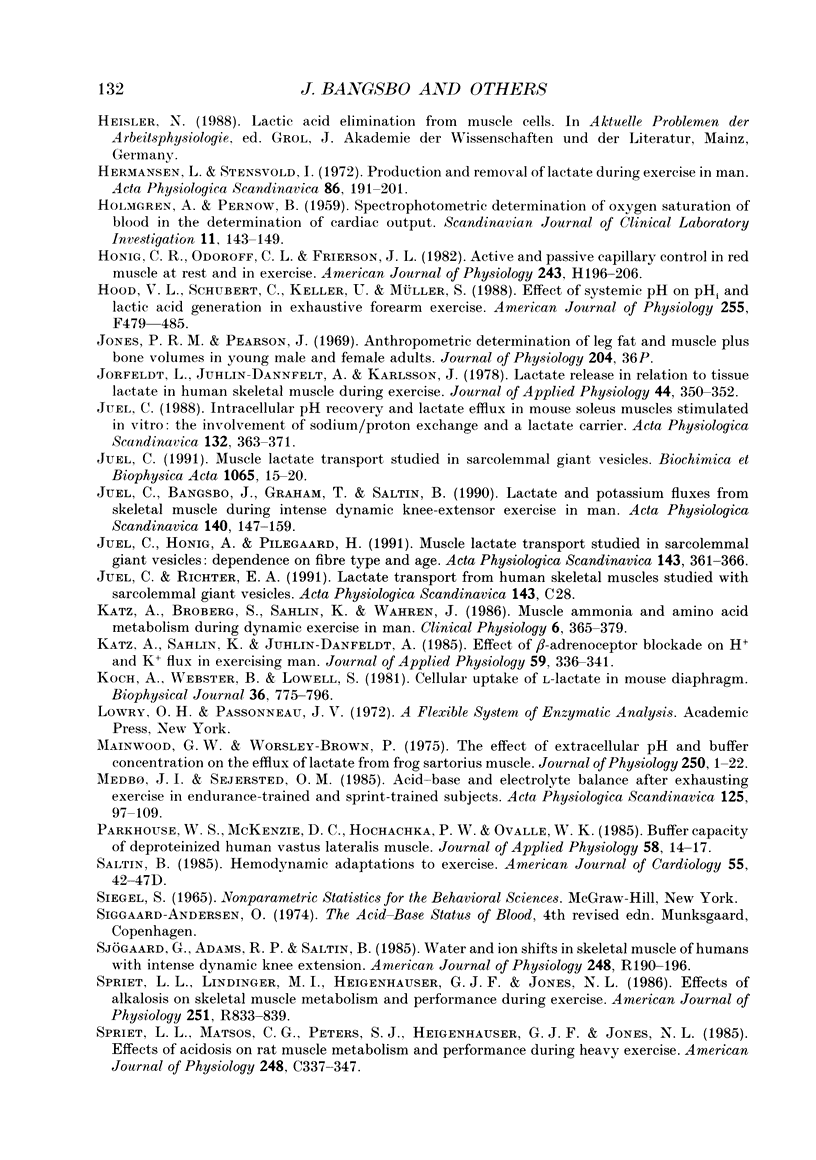
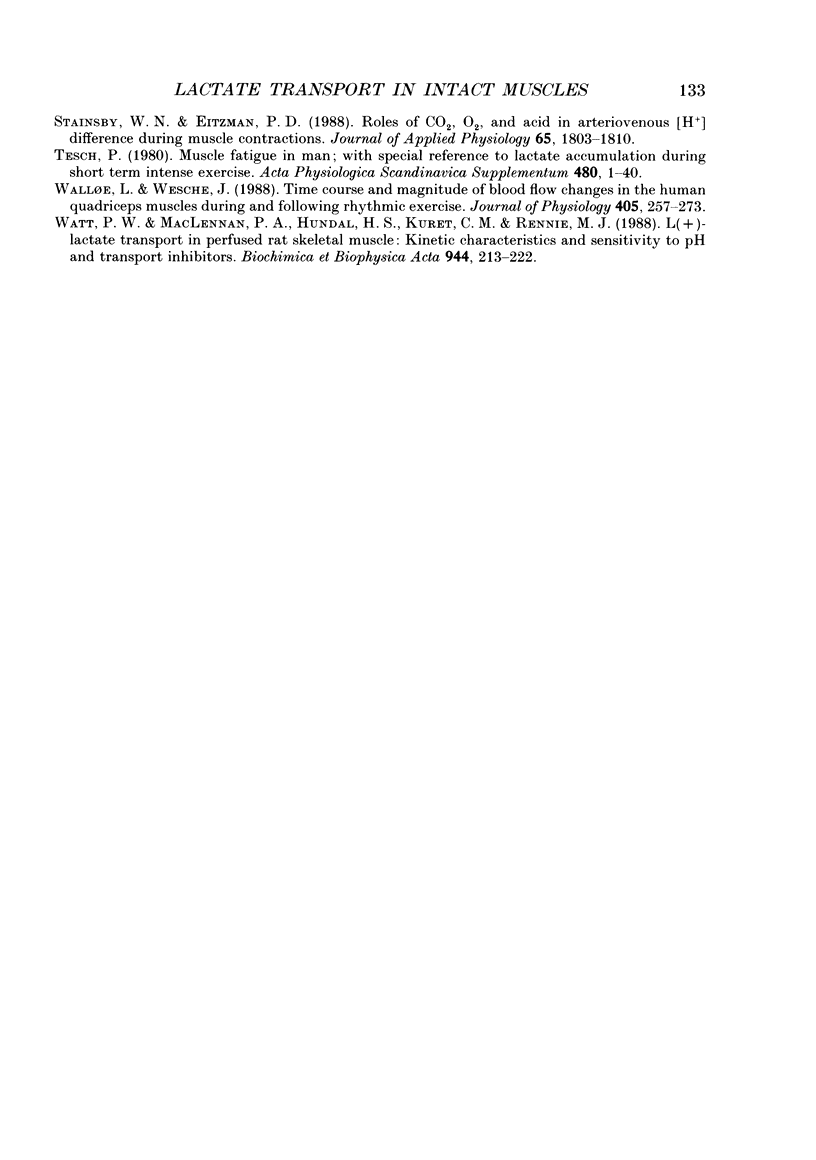
Selected References
These references are in PubMed. This may not be the complete list of references from this article.
- Ahlborg G., Hagenfeldt L., Wahren J. Substrate utilization by the inactive leg during one-leg or arm exercise. J Appl Physiol. 1975 Nov;39(5):718–723. doi: 10.1152/jappl.1975.39.5.718. [DOI] [PubMed] [Google Scholar]
- Andersen P., Adams R. P., Sjøgaard G., Thorboe A., Saltin B. Dynamic knee extension as model for study of isolated exercising muscle in humans. J Appl Physiol (1985) 1985 Nov;59(5):1647–1653. doi: 10.1152/jappl.1985.59.5.1647. [DOI] [PubMed] [Google Scholar]
- Andersen P. Capillary density in skeletal muscle of man. Acta Physiol Scand. 1975 Oct;95(2):203–205. doi: 10.1111/j.1748-1716.1975.tb10043.x. [DOI] [PubMed] [Google Scholar]
- Andersen P., Saltin B. Maximal perfusion of skeletal muscle in man. J Physiol. 1985 Sep;366:233–249. doi: 10.1113/jphysiol.1985.sp015794. [DOI] [PMC free article] [PubMed] [Google Scholar]
- Bangsbo J., Gollnick P. D., Graham T. E., Juel C., Kiens B., Mizuno M., Saltin B. Anaerobic energy production and O2 deficit-debt relationship during exhaustive exercise in humans. J Physiol. 1990 Mar;422:539–559. doi: 10.1113/jphysiol.1990.sp018000. [DOI] [PMC free article] [PubMed] [Google Scholar]
- Bangsbo J., Gollnick P. D., Graham T. E., Saltin B. Substrates for muscle glycogen synthesis in recovery from intense exercise in man. J Physiol. 1991 Mar;434:423–440. doi: 10.1113/jphysiol.1991.sp018478. [DOI] [PMC free article] [PubMed] [Google Scholar]
- Brooke M. H., Kaiser K. K. Three "myosin adenosine triphosphatase" systems: the nature of their pH lability and sulfhydryl dependence. J Histochem Cytochem. 1970 Sep;18(9):670–672. doi: 10.1177/18.9.670. [DOI] [PubMed] [Google Scholar]
- Chirtel S. J., Barbee R. W., Stainsby W. N. Net O2, CO2, lactate, and acid exchange by muscle during progressive working contractions. J Appl Physiol Respir Environ Exerc Physiol. 1984 Jan;56(1):161–165. doi: 10.1152/jappl.1984.56.1.161. [DOI] [PubMed] [Google Scholar]
- Connett R. J., Gayeski T. E., Honig C. R. Lactate efflux is unrelated to intracellular PO2 in a working red muscle in situ. J Appl Physiol (1985) 1986 Aug;61(2):402–408. doi: 10.1152/jappl.1986.61.2.402. [DOI] [PubMed] [Google Scholar]
- Hermansen L., Stensvold I. Production and removal of lactate during exercise in man. Acta Physiol Scand. 1972 Oct;86(2):191–201. doi: 10.1111/j.1748-1716.1972.tb05325.x. [DOI] [PubMed] [Google Scholar]
- Honig C. R., Odoroff C. L., Frierson J. L. Active and passive capillary control in red muscle at rest and in exercise. Am J Physiol. 1982 Aug;243(2):H196–H206. doi: 10.1152/ajpheart.1982.243.2.H196. [DOI] [PubMed] [Google Scholar]
- Hood V. L., Schubert C., Keller U., Müller S. Effect of systemic pH on pHi and lactic acid generation in exhaustive forearm exercise. Am J Physiol. 1988 Sep;255(3 Pt 2):F479–F485. doi: 10.1152/ajprenal.1988.255.3.F479. [DOI] [PubMed] [Google Scholar]
- Jorfeldt L., Juhlin-Dannfelt A., Karlsson J. Lactate release in relation to tissue lactate in human skeletal muscle during exercise. J Appl Physiol Respir Environ Exerc Physiol. 1978 Mar;44(3):350–352. doi: 10.1152/jappl.1978.44.3.350. [DOI] [PubMed] [Google Scholar]
- Juel C., Bangsbo J., Graham T., Saltin B. Lactate and potassium fluxes from human skeletal muscle during and after intense, dynamic, knee extensor exercise. Acta Physiol Scand. 1990 Oct;140(2):147–159. doi: 10.1111/j.1748-1716.1990.tb08986.x. [DOI] [PubMed] [Google Scholar]
- Juel C., Honig A., Pilegaard H. Muscle lactate transport studied in sarcolemmal giant vesicles: dependence on fibre type and age. Acta Physiol Scand. 1991 Dec;143(4):361–365. doi: 10.1111/j.1748-1716.1991.tb09246.x. [DOI] [PubMed] [Google Scholar]
- Juel C. Intracellular pH recovery and lactate efflux in mouse soleus muscles stimulated in vitro: the involvement of sodium/proton exchange and a lactate carrier. Acta Physiol Scand. 1988 Mar;132(3):363–371. doi: 10.1111/j.1748-1716.1988.tb08340.x. [DOI] [PubMed] [Google Scholar]
- Juel C. Muscle lactate transport studied in sarcolemmal giant vesicles. Biochim Biophys Acta. 1991 May 31;1065(1):15–20. doi: 10.1016/0005-2736(91)90004-r. [DOI] [PubMed] [Google Scholar]
- Katz A., Broberg S., Sahlin K., Wahren J. Muscle ammonia and amino acid metabolism during dynamic exercise in man. Clin Physiol. 1986 Aug;6(4):365–379. doi: 10.1111/j.1475-097x.1986.tb00242.x. [DOI] [PubMed] [Google Scholar]
- Katz A., Sahlin K., Juhlin-Dannfelt A. Effect of beta-adrenoceptor blockade on H+ and K+ flux in exercising humans. J Appl Physiol (1985) 1985 Aug;59(2):336–341. doi: 10.1152/jappl.1985.59.2.336. [DOI] [PubMed] [Google Scholar]
- Koch A., Webster B., Lowell S. Cellular uptake of L-lactate in mouse diaphragm. Biophys J. 1981 Dec;36(3):775–796. doi: 10.1016/S0006-3495(81)84765-0. [DOI] [PMC free article] [PubMed] [Google Scholar]
- Mainwood G. W., Worsley-Brown P. The effects of extracellular pH and buffer concentration on the efflux of lactate from frog sartorius muscle. J Physiol. 1975 Aug;250(1):1–22. doi: 10.1113/jphysiol.1975.sp011040. [DOI] [PMC free article] [PubMed] [Google Scholar]
- Medbø J. I., Sejersted O. M. Acid-base and electrolyte balance after exhausting exercise in endurance-trained and sprint-trained subjects. Acta Physiol Scand. 1985 Sep;125(1):97–109. doi: 10.1111/j.1748-1716.1985.tb07696.x. [DOI] [PubMed] [Google Scholar]
- Parkhouse W. S., McKenzie D. C., Hochachka P. W., Ovalle W. K. Buffering capacity of deproteinized human vastus lateralis muscle. J Appl Physiol (1985) 1985 Jan;58(1):14–17. doi: 10.1152/jappl.1985.58.1.14. [DOI] [PubMed] [Google Scholar]
- Sjøgaard G., Adams R. P., Saltin B. Water and ion shifts in skeletal muscle of humans with intense dynamic knee extension. Am J Physiol. 1985 Feb;248(2 Pt 2):R190–R196. doi: 10.1152/ajpregu.1985.248.2.R190. [DOI] [PubMed] [Google Scholar]
- Spriet L. L., Lindinger M. I., Heigenhauser G. J., Jones N. L. Effects of alkalosis on skeletal muscle metabolism and performance during exercise. Am J Physiol. 1986 Nov;251(5 Pt 2):R833–R839. doi: 10.1152/ajpregu.1986.251.5.R833. [DOI] [PubMed] [Google Scholar]
- Spriet L. L., Matsos C. G., Peters S. J., Heigenhauser G. J., Jones N. L. Effects of acidosis on rat muscle metabolism and performance during heavy exercise. Am J Physiol. 1985 Mar;248(3 Pt 1):C337–C347. doi: 10.1152/ajpcell.1985.248.3.C337. [DOI] [PubMed] [Google Scholar]
- Stainsby W. N., Eitzman P. D. Roles of CO2, O2, and acid in arteriovenous [H+] difference during muscle contractions. J Appl Physiol (1985) 1988 Oct;65(4):1803–1810. doi: 10.1152/jappl.1988.65.4.1803. [DOI] [PubMed] [Google Scholar]
- Tesch P. Muscle fatigue in man. With special reference to lactate accumulation during short term intense exercise. Acta Physiol Scand Suppl. 1980;480:1–40. [PubMed] [Google Scholar]
- Walløe L., Wesche J. Time course and magnitude of blood flow changes in the human quadriceps muscles during and following rhythmic exercise. J Physiol. 1988 Nov;405:257–273. doi: 10.1113/jphysiol.1988.sp017332. [DOI] [PMC free article] [PubMed] [Google Scholar]
- Watt P. W., MacLennan P. A., Hundal H. S., Kuret C. M., Rennie M. J. L(+)-lactate transport in perfused rat skeletal muscle: kinetic characteristics and sensitivity to pH and transport inhibitors. Biochim Biophys Acta. 1988 Oct 6;944(2):213–222. doi: 10.1016/0005-2736(88)90434-8. [DOI] [PubMed] [Google Scholar]