Abstract
1. The establishment of the whole-cell patch-clamp recording configuration (WCR) revealed a type of inhibition to which L-type Ca2+ channels were subject in static rat ventricular myocytes before obtaining the WCR. 2. Immediately after membrane disruption (< 10 s), the Ca2+ current (ICa) was absent but gradually increased in amplitude to reach its final waveform (amplitude and kinetics) 2-3 min after the WCR was reached. 3. Three distinct phases (P) were identified. First, no inward but an outward current, blocked (1-2 min) by Cs+ dialysing from the patch pipette (P1), was recorded. Second, overlapping with (P1), ICa increased dramatically to reach a maximum peak amplitude within 2-3 min (P2). Concomitantly, its rate of decay, initially monoexponential and slow, became biexponential owing to the appearance of a fast component of inactivation (P3). Complete interconversion between slow and fast components sometimes occurred. 4. Changes in current waveform were not related to voltage loss or series resistance variation, and suppression of an outward current (P1) was unlikely to account for P2 and P3. 5. The run-up of ICa was independent of the nature of the permeating ions, the membrane holding potential, depolarization, rate of stimulation, the intracellular Ca2+, ATP, Mg2+, Cs+ and the pH of the pipette solution. Since large Ca2+ currents were recorded using the perforated patch technique, the run-up of ICa is not explained by the wash-out of an inhibitory endogenous macromolecule during cell-pipette exchanges. 6. Pharmacological manipulations, including the use of Ca(2+)-Ba(2+)-EGTA and exposure of the cells to isoprenaline and/or Bay K 8644 prior to recording, did not alter the mechanism primarily responsible for build-up. Unrepriming of channel activity was required before these modulations could be effective. 7. Currents could however be instantly augmented when cells were extracellularly superfused during the run-up step. The wash-out of an inhibitory agent originating in the cell itself (such as H+, NH4+ and lactate) and accumulating in the extracellular microenvironment of the cells seems unlikely. Rather, we suggest that pressure-induced mechanostimulation may be involved in the restoration of Ca2+ channel activity.
Full text
PDF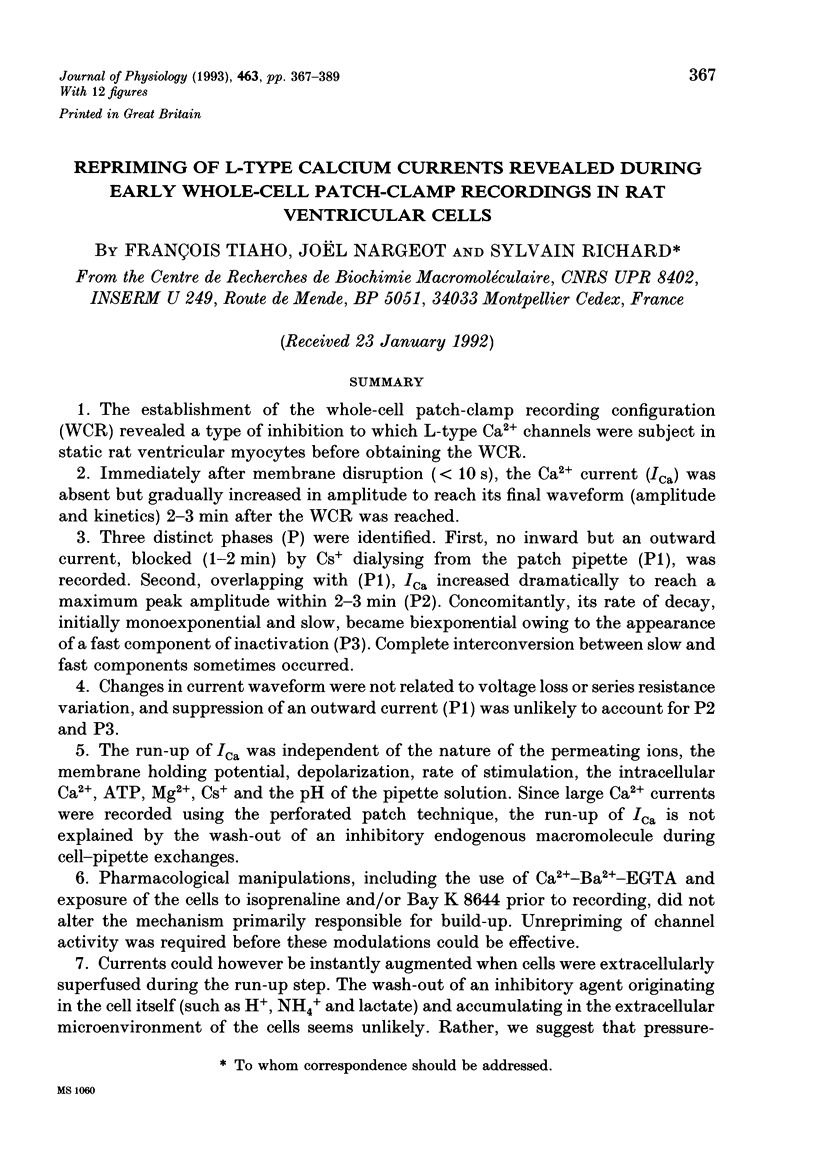
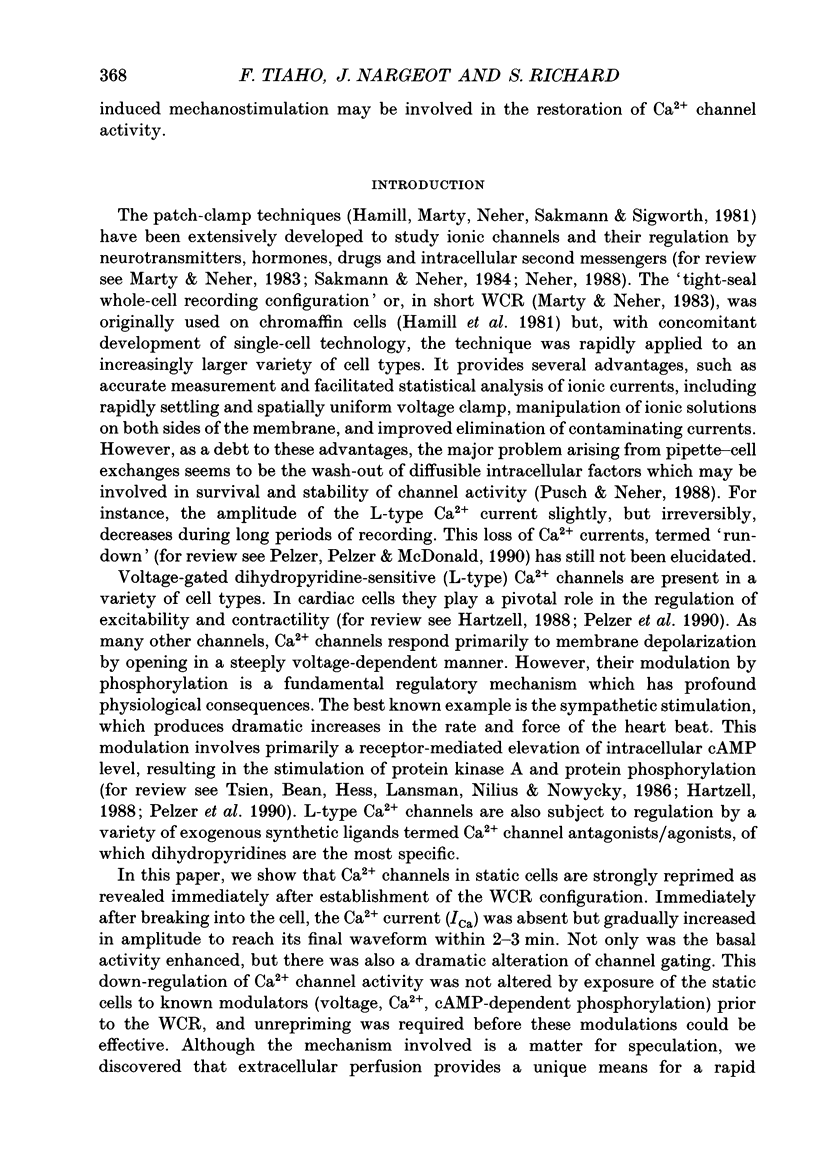
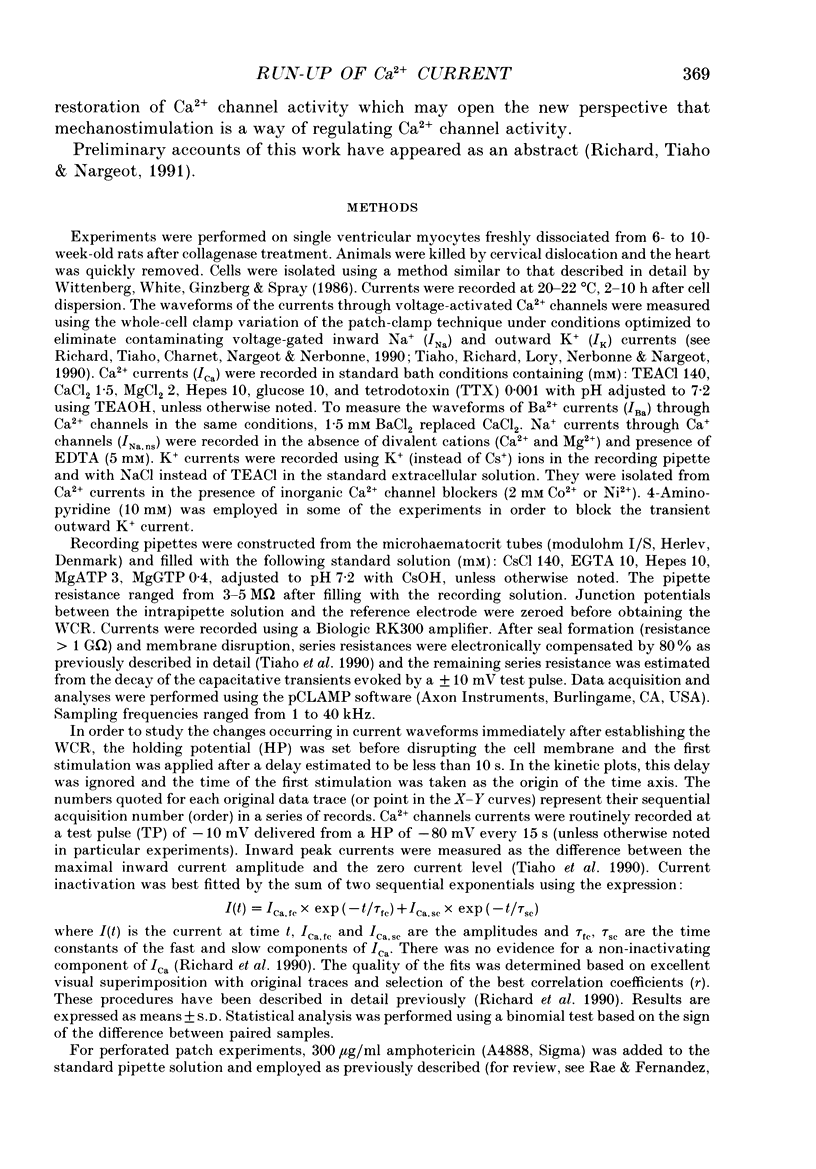
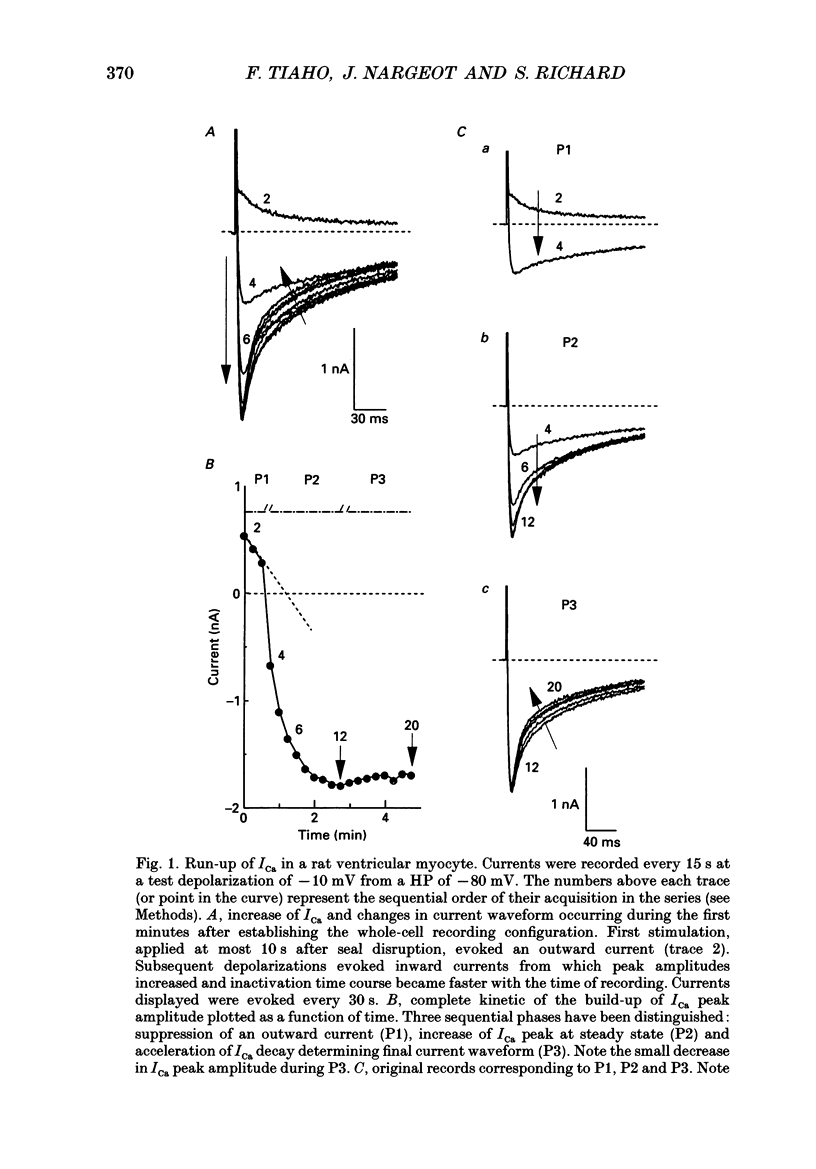
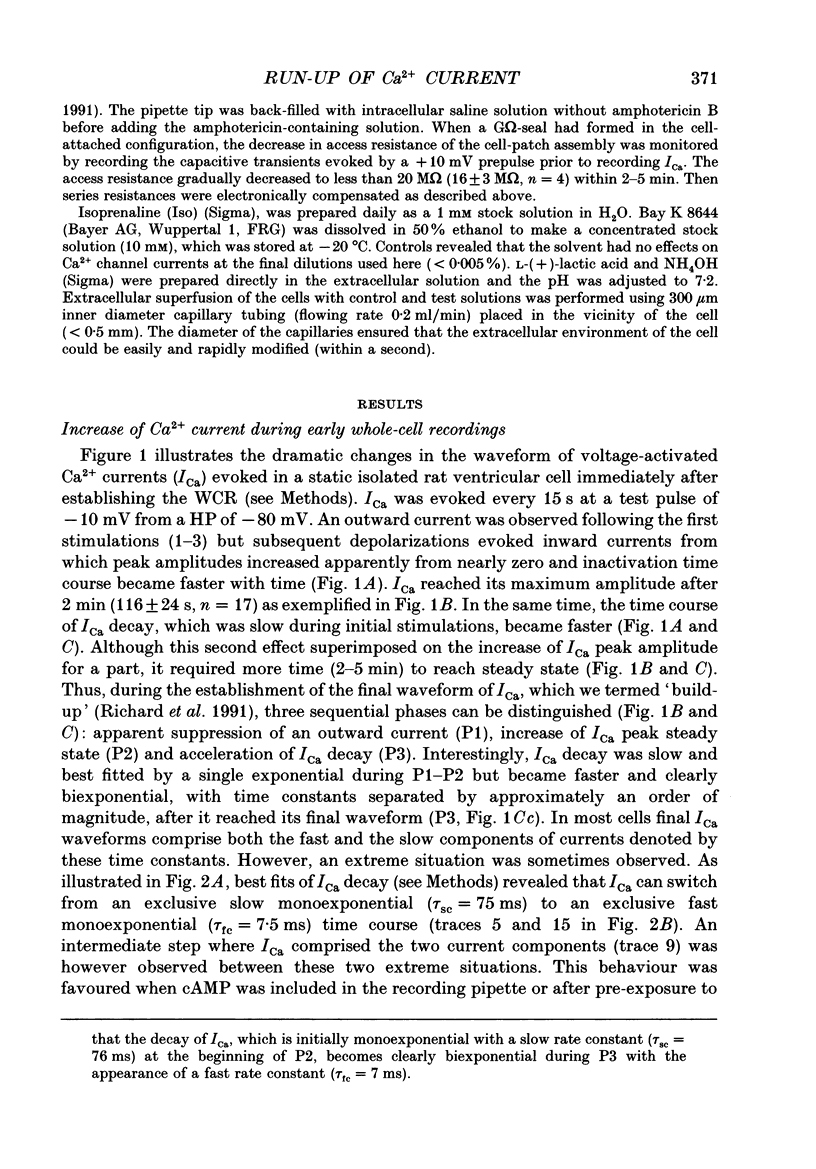
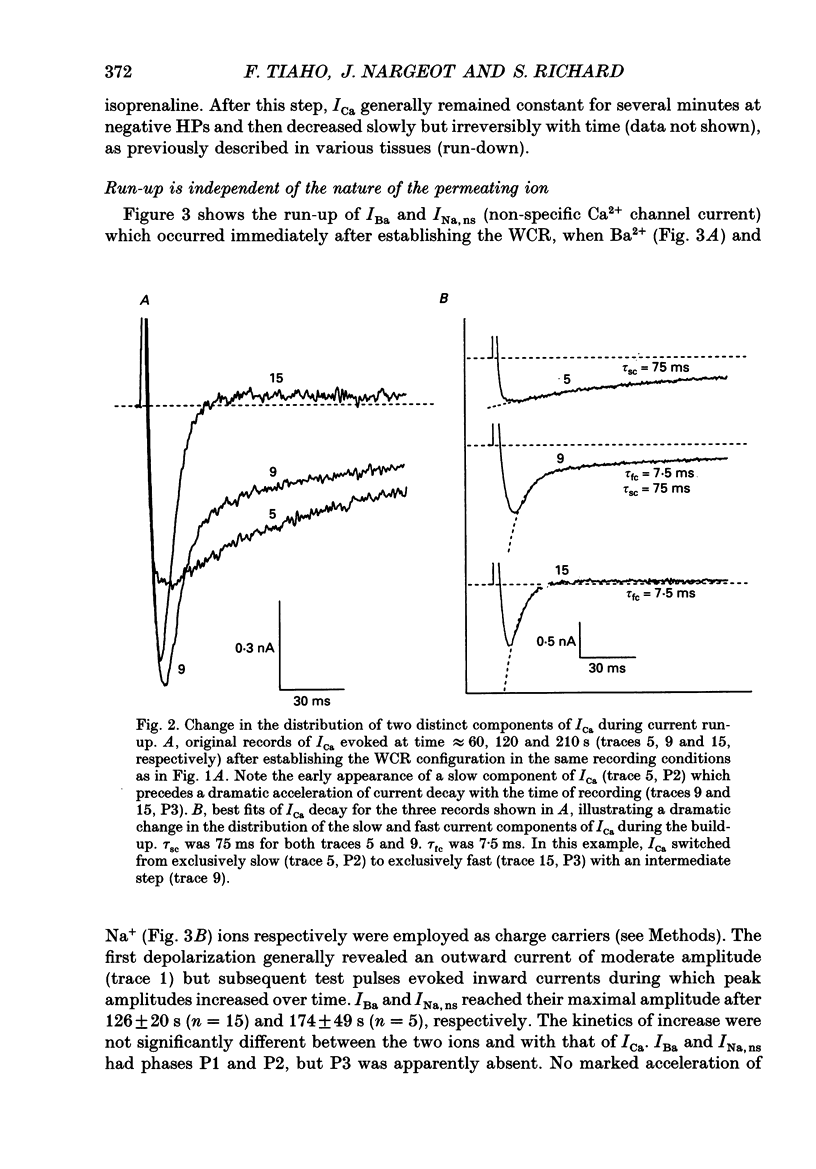
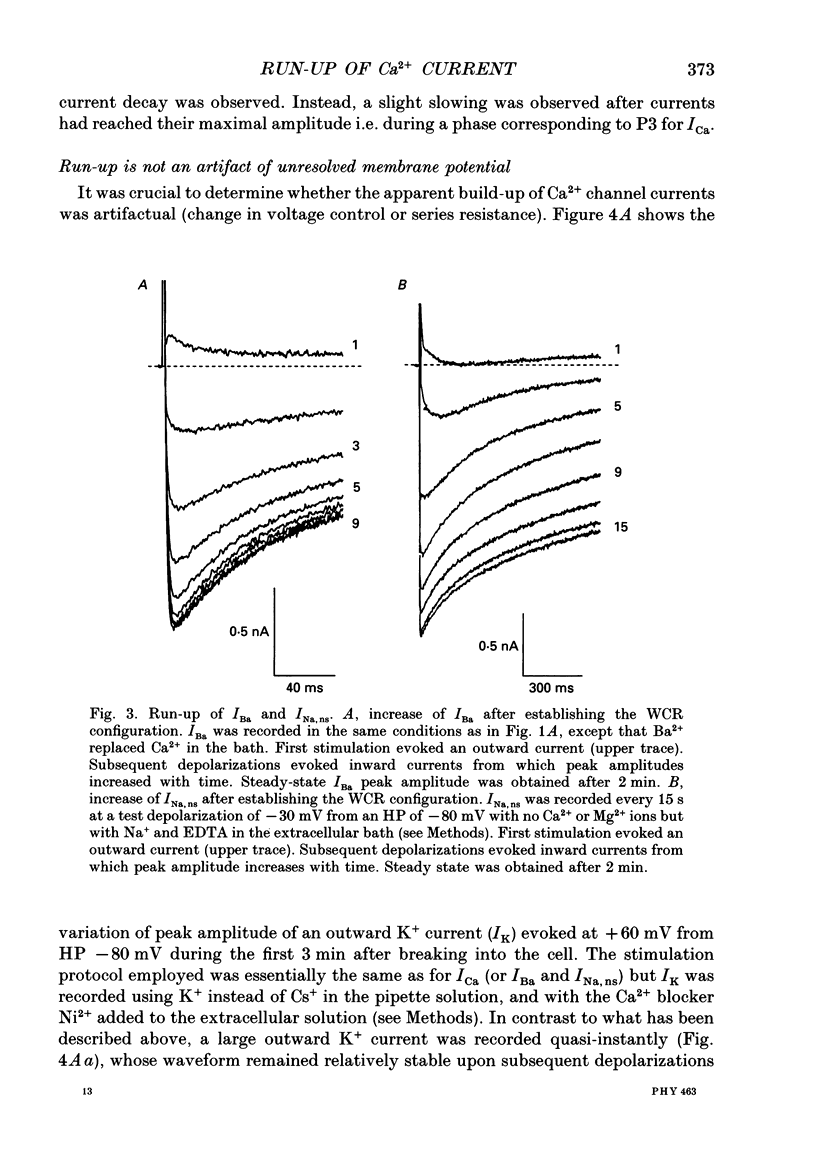
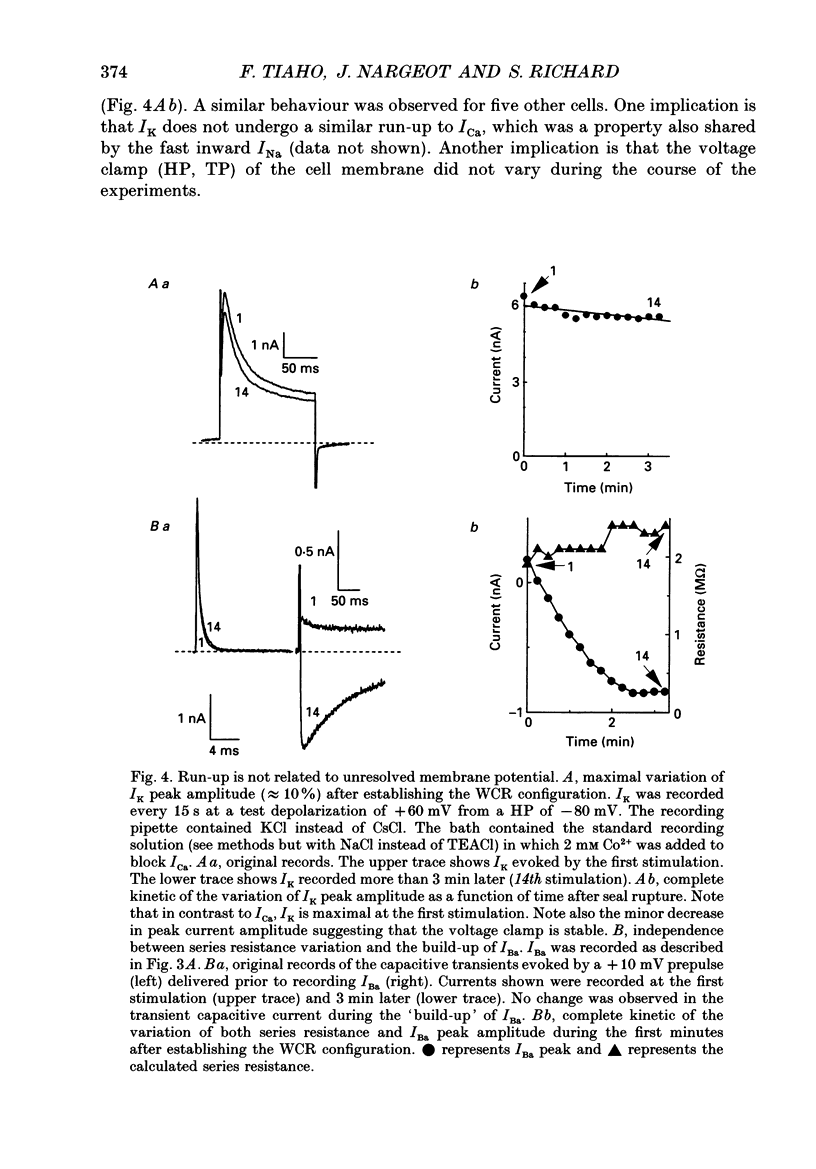
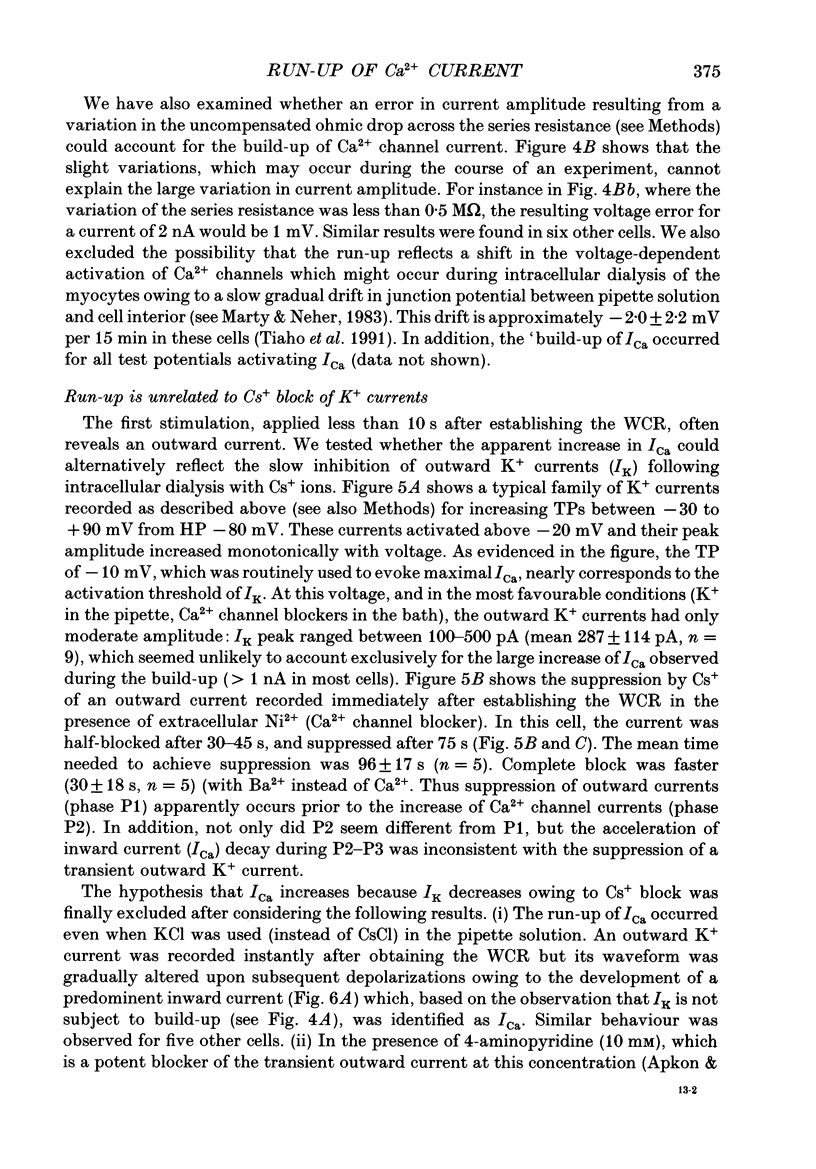
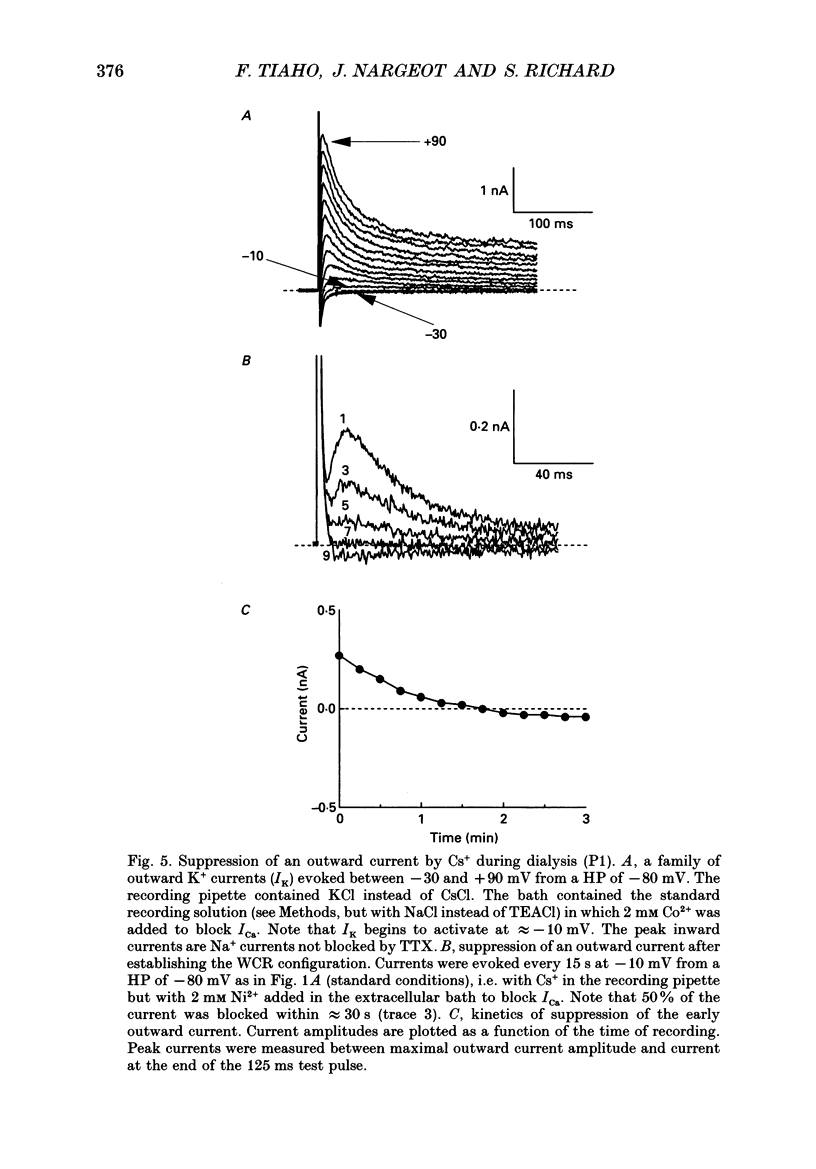
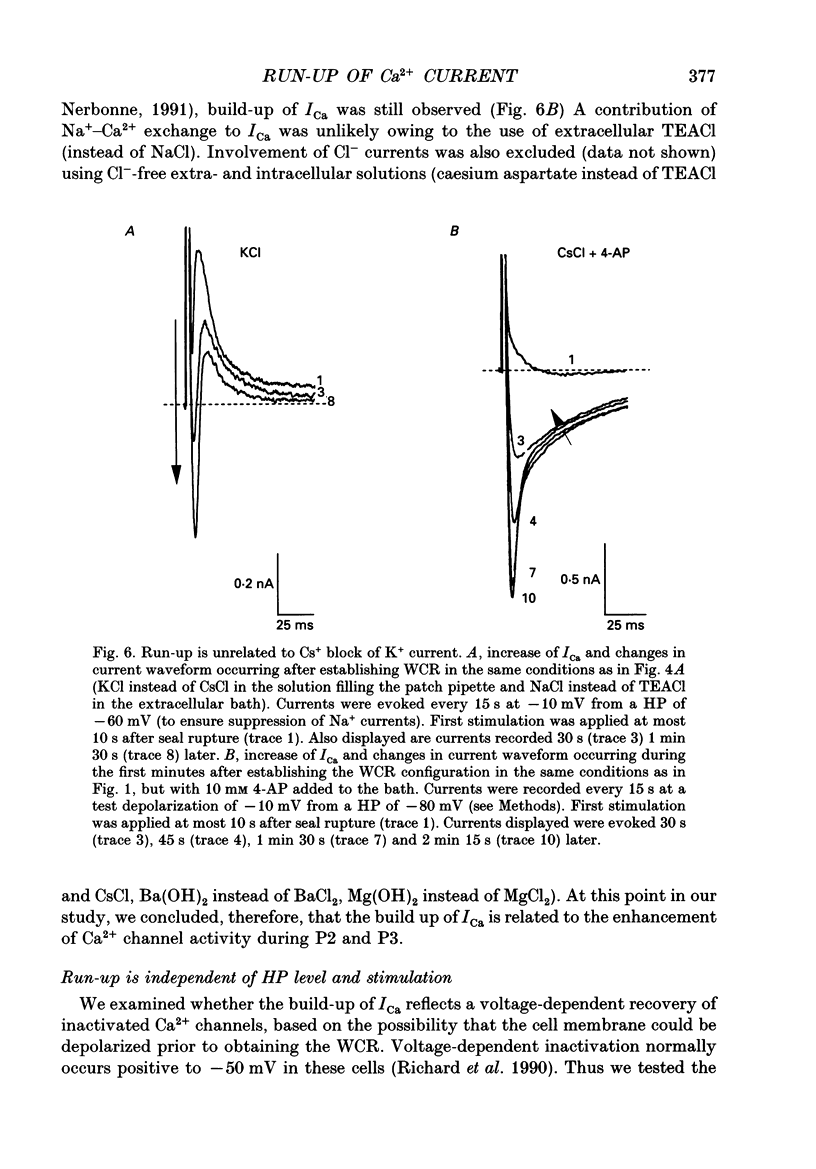
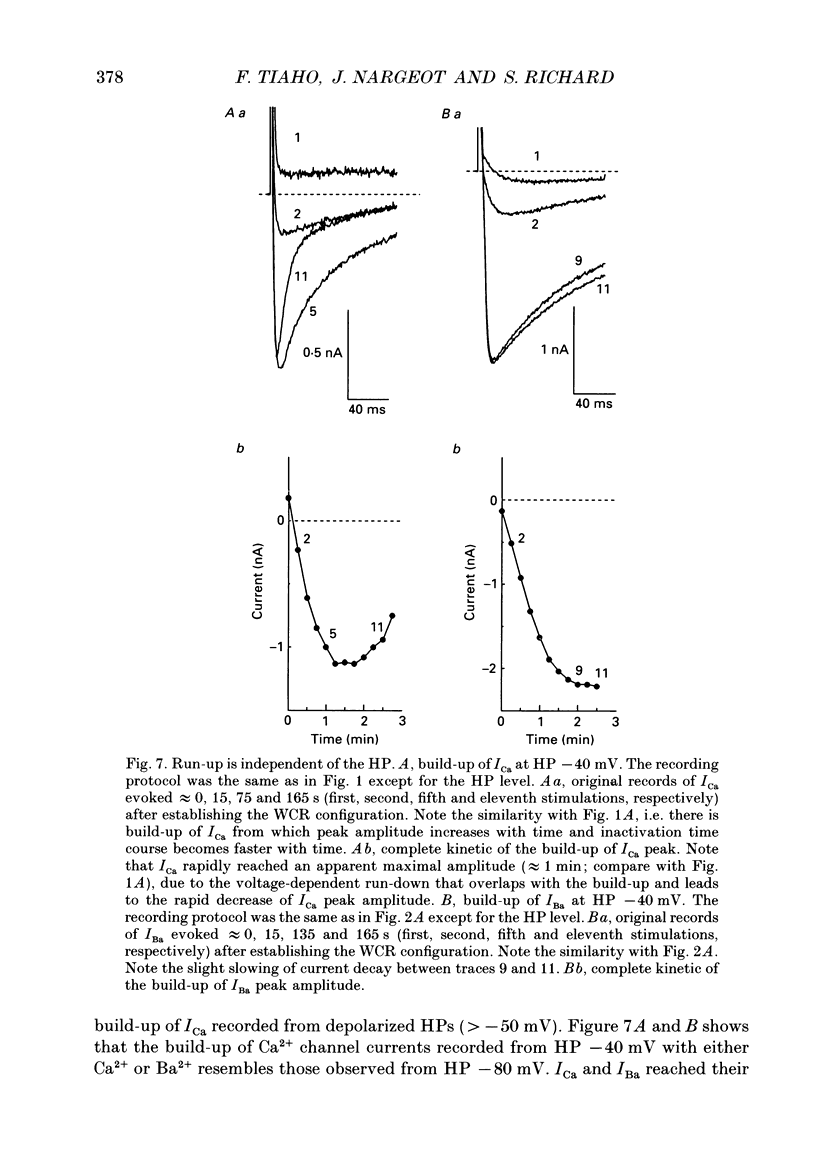
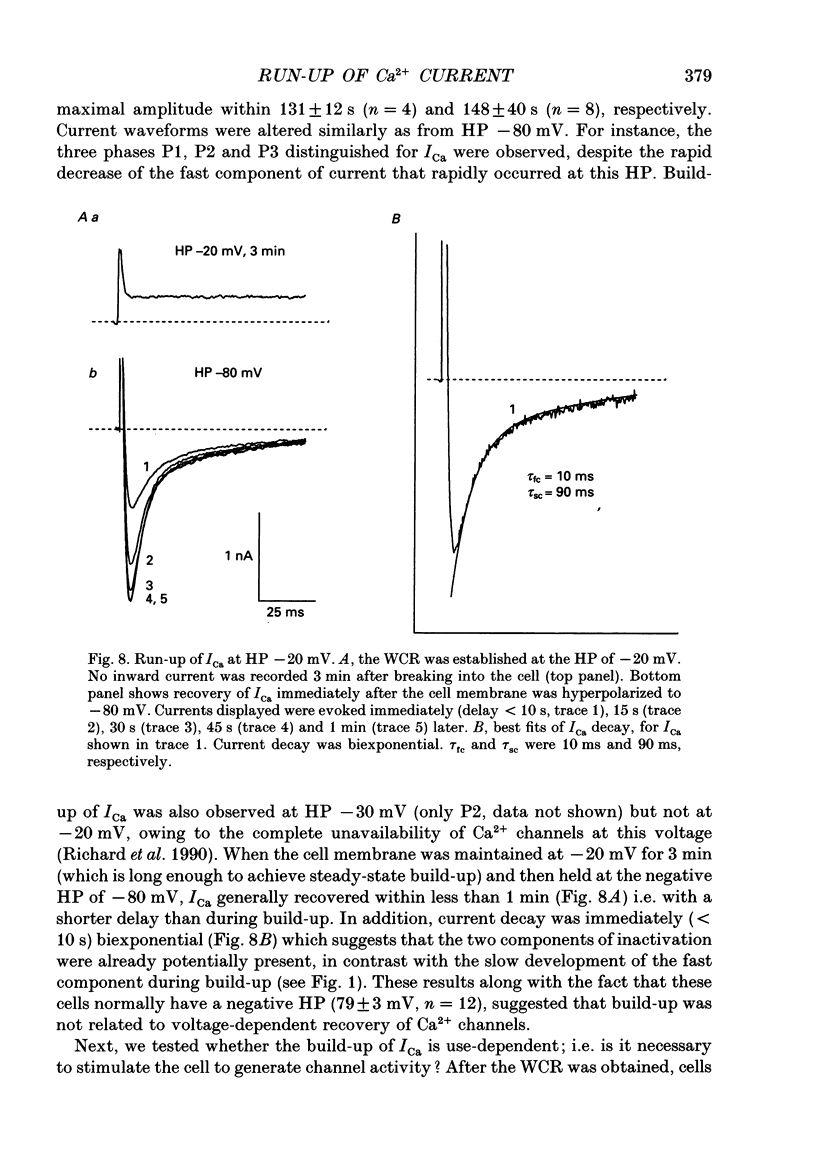
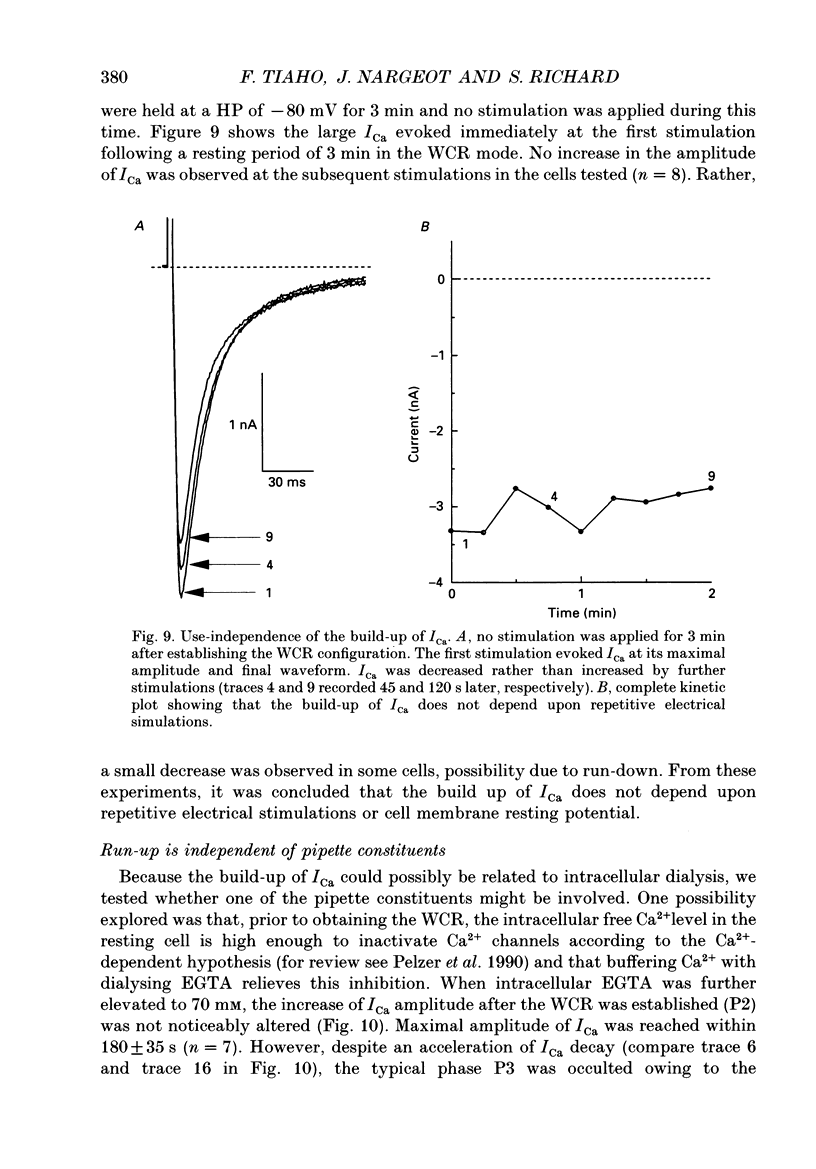
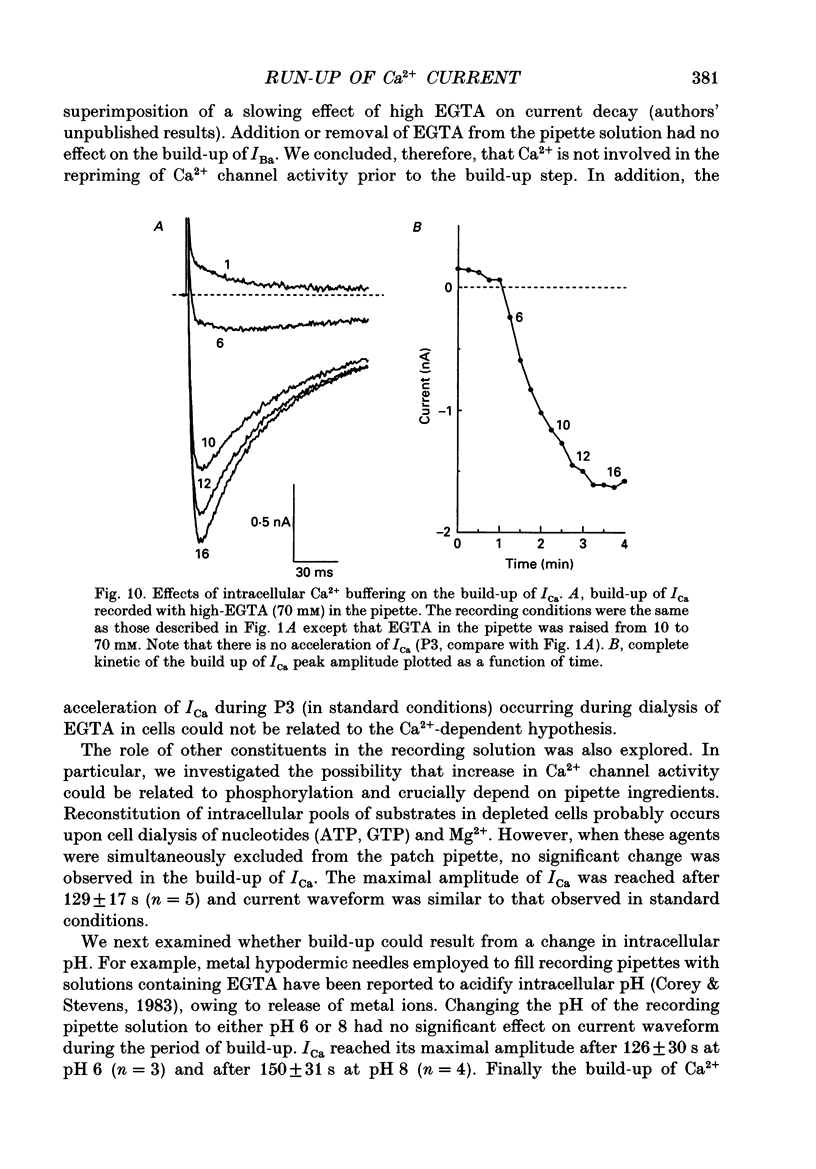
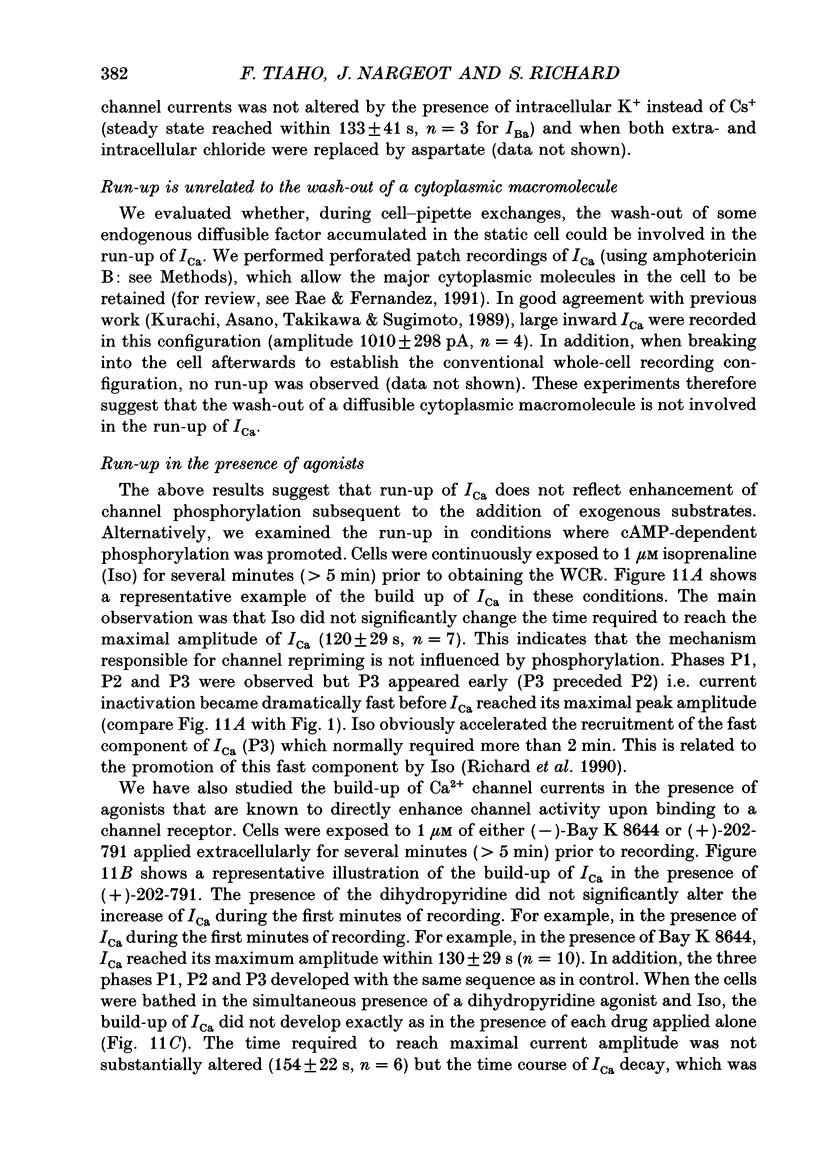
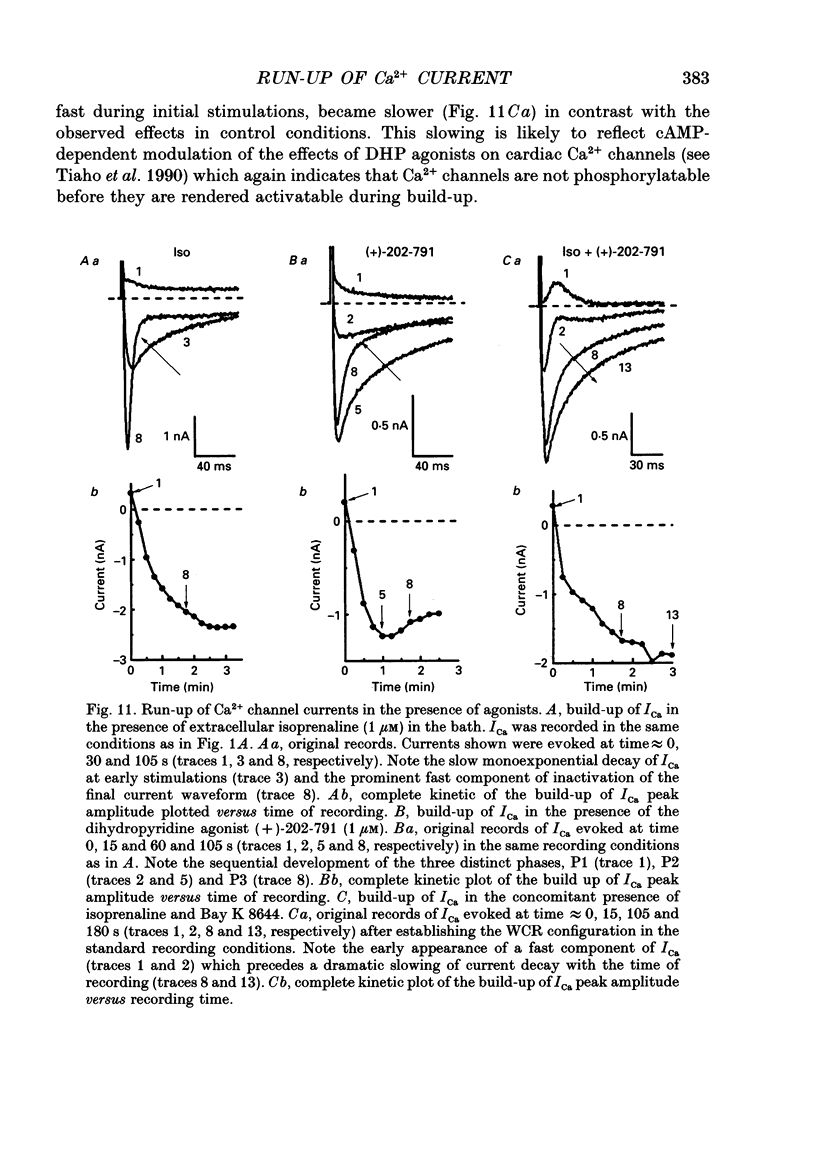
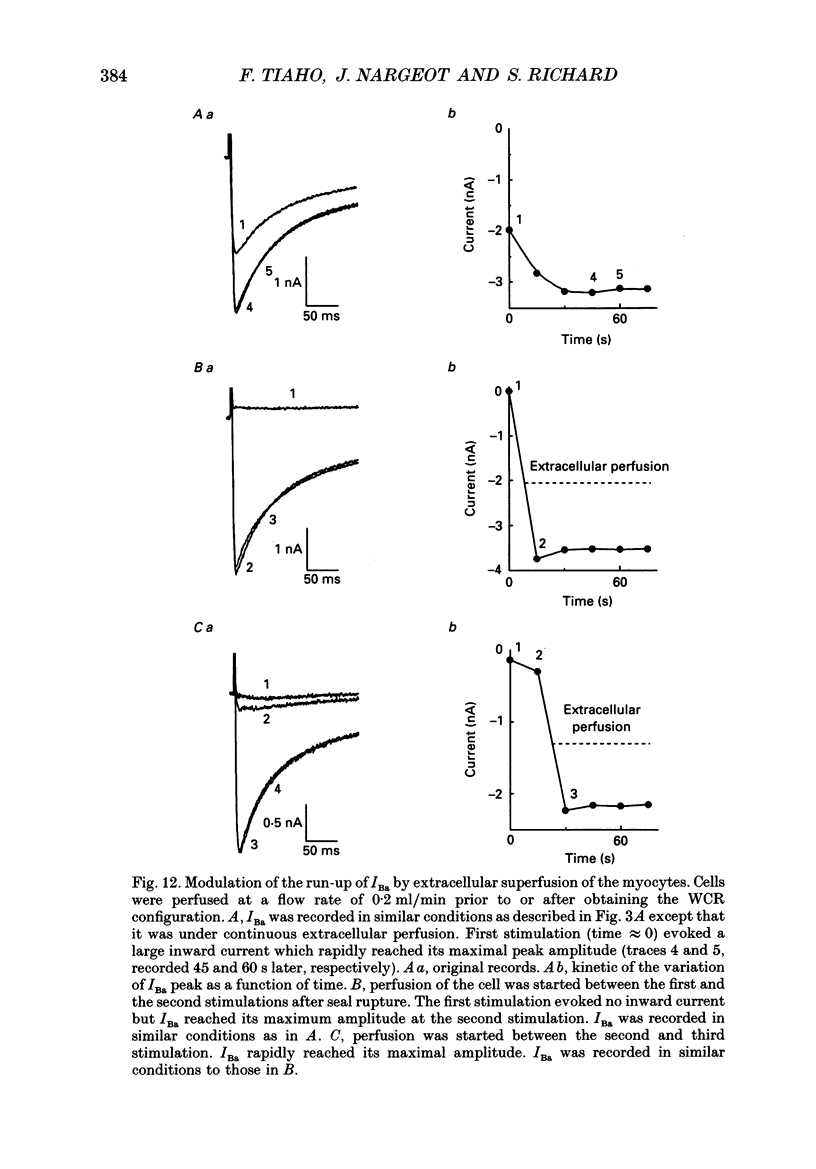
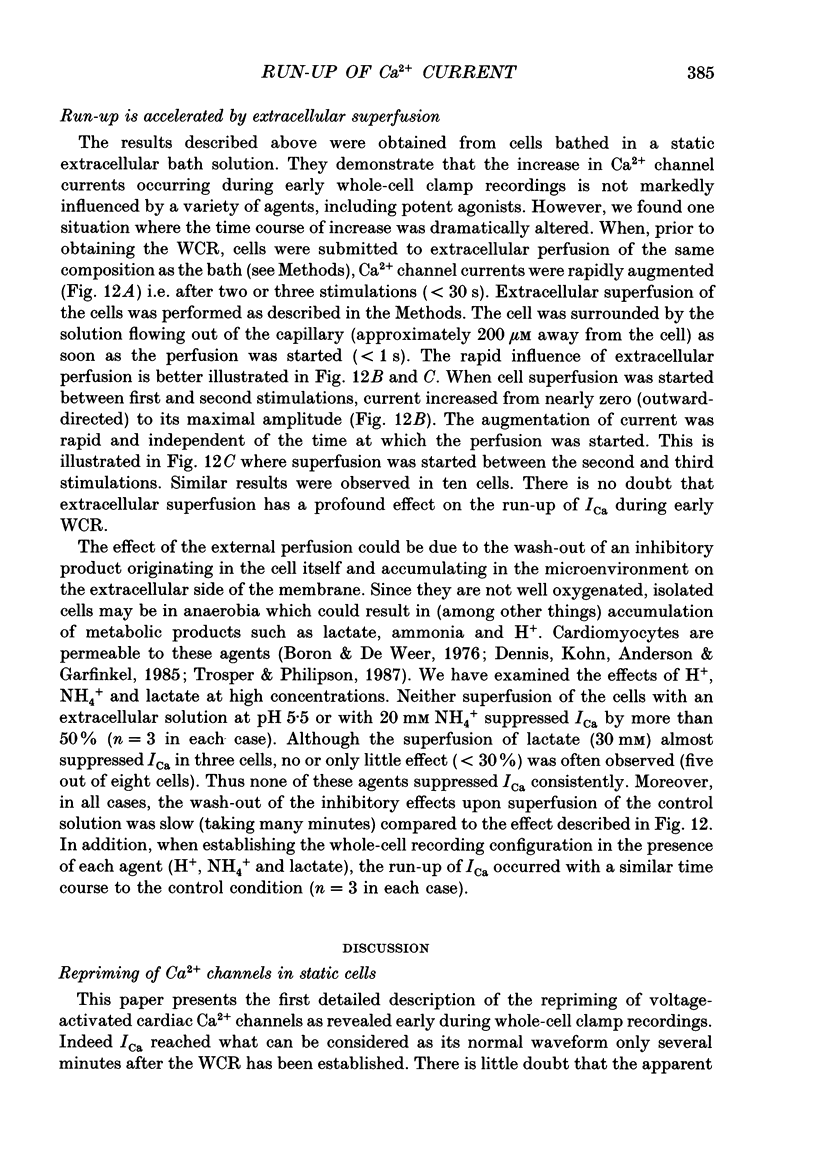
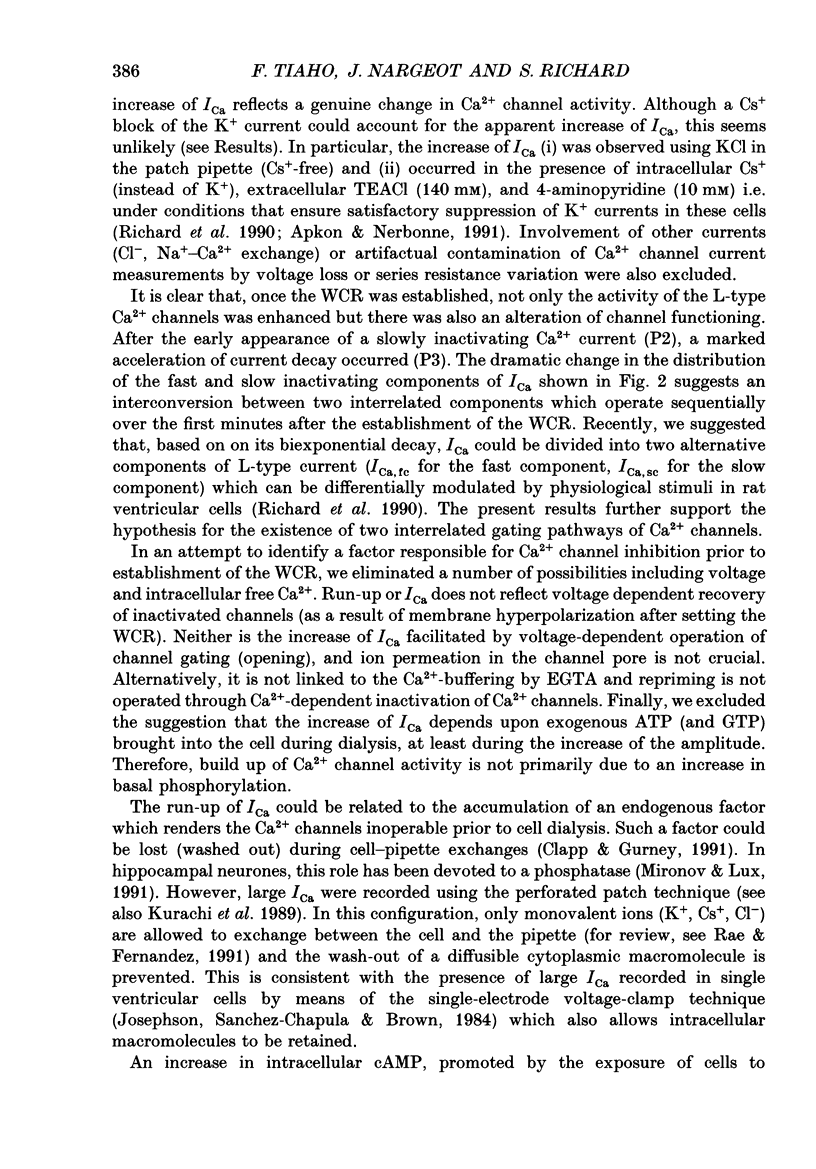
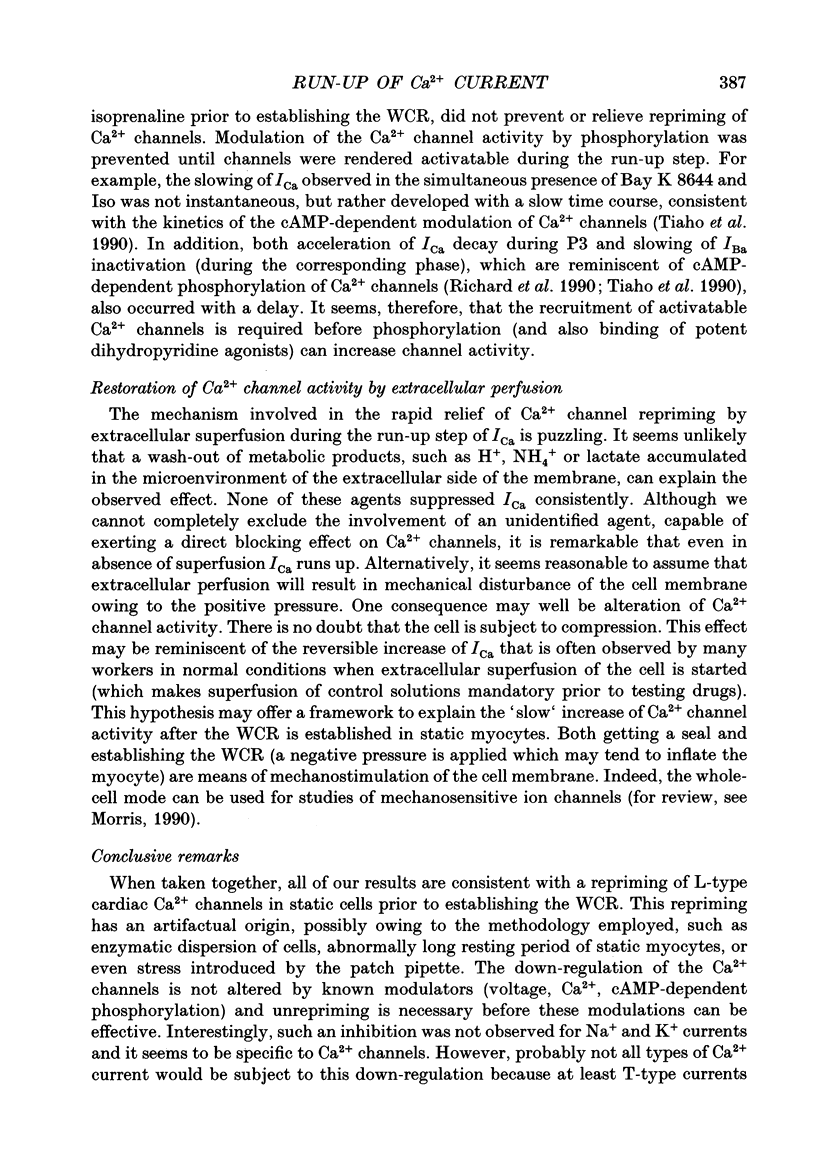
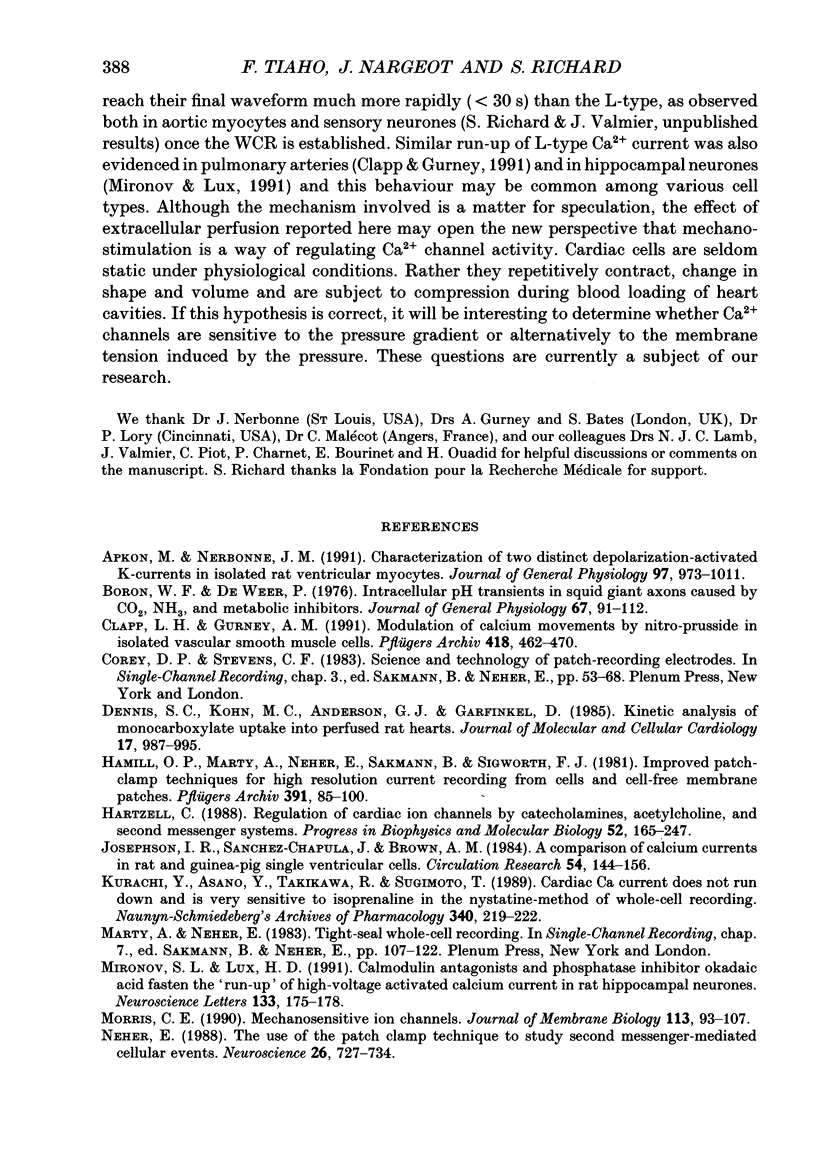
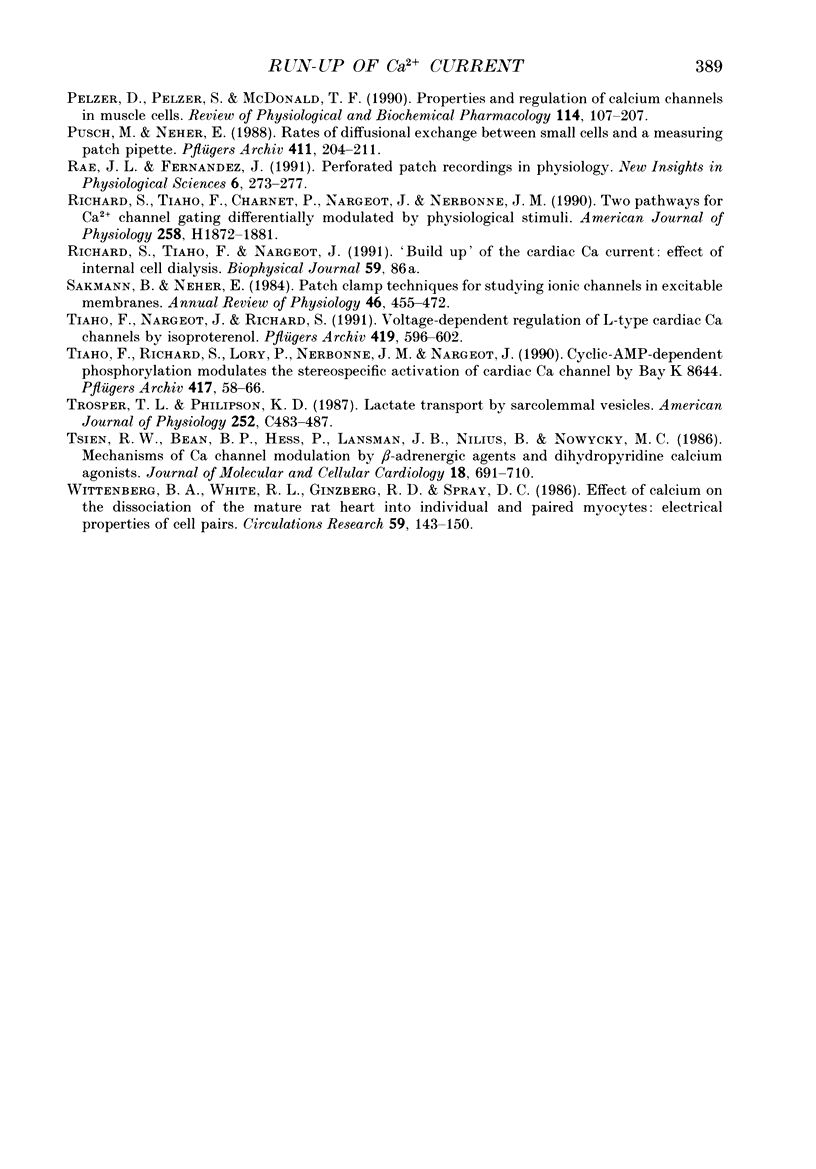
Selected References
These references are in PubMed. This may not be the complete list of references from this article.
- Apkon M., Nerbonne J. M. Characterization of two distinct depolarization-activated K+ currents in isolated adult rat ventricular myocytes. J Gen Physiol. 1991 May;97(5):973–1011. doi: 10.1085/jgp.97.5.973. [DOI] [PMC free article] [PubMed] [Google Scholar]
- Boron W. F., De Weer P. Intracellular pH transients in squid giant axons caused by CO2, NH3, and metabolic inhibitors. J Gen Physiol. 1976 Jan;67(1):91–112. doi: 10.1085/jgp.67.1.91. [DOI] [PMC free article] [PubMed] [Google Scholar]
- Clapp L. H., Gurney A. M. Modulation of calcium movements by nitroprusside in isolated vascular smooth muscle cells. Pflugers Arch. 1991 Jun;418(5):462–470. doi: 10.1007/BF00497774. [DOI] [PubMed] [Google Scholar]
- Dennis S. C., Kohn M. C., Anderson G. J., Garfinkel D. Kinetic analysis of monocarboxylate uptake into perfused rat hearts. J Mol Cell Cardiol. 1985 Oct;17(10):987–995. doi: 10.1016/s0022-2828(85)80079-1. [DOI] [PubMed] [Google Scholar]
- Hamill O. P., Marty A., Neher E., Sakmann B., Sigworth F. J. Improved patch-clamp techniques for high-resolution current recording from cells and cell-free membrane patches. Pflugers Arch. 1981 Aug;391(2):85–100. doi: 10.1007/BF00656997. [DOI] [PubMed] [Google Scholar]
- Hartzell H. C. Regulation of cardiac ion channels by catecholamines, acetylcholine and second messenger systems. Prog Biophys Mol Biol. 1988;52(3):165–247. doi: 10.1016/0079-6107(88)90014-4. [DOI] [PubMed] [Google Scholar]
- Josephson I. R., Sanchez-Chapula J., Brown A. M. A comparison of calcium currents in rat and guinea pig single ventricular cells. Circ Res. 1984 Feb;54(2):144–156. doi: 10.1161/01.res.54.2.144. [DOI] [PubMed] [Google Scholar]
- Kurachi Y., Asano Y., Takikawa R., Sugimoto T. Cardiac Ca current does not run down and is very sensitive to isoprenaline in the nystatin-method of whole cell recording. Naunyn Schmiedebergs Arch Pharmacol. 1989 Aug;340(2):219–222. doi: 10.1007/BF00168972. [DOI] [PubMed] [Google Scholar]
- Mironov S. L., Lux H. D. Calmodulin antagonists and protein phosphatase inhibitor okadaic acid fasten the 'run-up' of high-voltage activated calcium current in rat hippocampal neurones. Neurosci Lett. 1991 Dec 9;133(2):175–178. doi: 10.1016/0304-3940(91)90563-9. [DOI] [PubMed] [Google Scholar]
- Morris C. E. Mechanosensitive ion channels. J Membr Biol. 1990 Feb;113(2):93–107. doi: 10.1007/BF01872883. [DOI] [PubMed] [Google Scholar]
- Neher E. The use of the patch clamp technique to study second messenger-mediated cellular events. Neuroscience. 1988 Sep;26(3):727–734. doi: 10.1016/0306-4522(88)90094-2. [DOI] [PubMed] [Google Scholar]
- Pelzer D., Pelzer S., McDonald T. F. Properties and regulation of calcium channels in muscle cells. Rev Physiol Biochem Pharmacol. 1990;114:107–207. doi: 10.1007/BFb0031019. [DOI] [PubMed] [Google Scholar]
- Pusch M., Neher E. Rates of diffusional exchange between small cells and a measuring patch pipette. Pflugers Arch. 1988 Feb;411(2):204–211. doi: 10.1007/BF00582316. [DOI] [PubMed] [Google Scholar]
- Richard S., Tiaho F., Charnet P., Nargeot J., Nerbonne J. M. Two pathways for Ca2+ channel gating differentially modulated by physiological stimuli. Am J Physiol. 1990 Jun;258(6 Pt 2):H1872–H1881. doi: 10.1152/ajpheart.1990.258.6.H1872. [DOI] [PubMed] [Google Scholar]
- Sakmann B., Neher E. Patch clamp techniques for studying ionic channels in excitable membranes. Annu Rev Physiol. 1984;46:455–472. doi: 10.1146/annurev.ph.46.030184.002323. [DOI] [PubMed] [Google Scholar]
- Tiaho F., Nargeot J., Richard S. Voltage-dependent regulation of L-type cardiac Ca channels by isoproterenol. Pflugers Arch. 1991 Dec;419(6):596–602. doi: 10.1007/BF00370301. [DOI] [PubMed] [Google Scholar]
- Tiaho F., Richard S., Lory P., Nerbonne J. M., Nargeot J. Cyclic-AMP-dependent phosphorylation modulates the stereospecific activation of cardiac Ca channels by Bay K 8644. Pflugers Arch. 1990 Sep;417(1):58–66. doi: 10.1007/BF00370769. [DOI] [PubMed] [Google Scholar]
- Trosper T. L., Philipson K. D. Lactate transport by cardiac sarcolemmal vesicles. Am J Physiol. 1987 May;252(5 Pt 1):C483–C489. doi: 10.1152/ajpcell.1987.252.5.C483. [DOI] [PubMed] [Google Scholar]
- Tsien R. W., Bean B. P., Hess P., Lansman J. B., Nilius B., Nowycky M. C. Mechanisms of calcium channel modulation by beta-adrenergic agents and dihydropyridine calcium agonists. J Mol Cell Cardiol. 1986 Jul;18(7):691–710. doi: 10.1016/s0022-2828(86)80941-5. [DOI] [PubMed] [Google Scholar]
- Wittenberg B. A., White R. L., Ginzberg R. D., Spray D. C. Effect of calcium on the dissociation of the mature rat heart into individual and paired myocytes: electrical properties of cell pairs. Circ Res. 1986 Aug;59(2):143–150. doi: 10.1161/01.res.59.2.143. [DOI] [PubMed] [Google Scholar]