Abstract
1. The effects of increased osmolality and ionic strength on the mechanism of Ca2+ release were examined in mechanically skinned skeletal muscle fibres of the toad at 23 degrees C. Ca2+ release was induced by depolarizing the transverse tubular (T-) system by ionic substitution. 2. Increasing the osmolality of the 'myoplasmic' solution about four times (to 955 mosmol/kg), by addition of 700 mM sucrose to the standard potassium (K-)HDTA solution (HDTA: hexamethylenediamine-tetraacetate), only depressed the depolarization-induced response by about 46%. Much of this decrease could be attributed to a reduction in the Ca(2+)-sensitivity of the contractile proteins at this high osmolality. 3. Addition of > 400 mM sucrose itself often induced substantial Ca2+ release and a transient tension response. This 'spontaneous' release was (a) greatly enhanced when the sarcoplasmic reticulum (SR) had been heavily loaded with Ca2+, (b) little affected by inactivation of the voltage sensors by prolonged or permanent depolarization of the T-system and (c) blocked by Ruthenium Red (10 microM). 4. When both the osmolality and ionic strength were increased, by increasing the K-HDTA concentration, the depolarization-induced force was greatly reduced (to 35% at 818 mosmol/kg and 5% at 1095 mosmol/kg). Most of this reduction could be directly attributed to the substantially reduced maximum force and Ca2+ sensitivity of the contractile apparatus. 5. The small amount of releasable Ca2+ remaining in the SR after a single depolarization in a high-HDTA solution with 1 mM EGTA (to chelate the released Ca2+), indicated that depolarization could still elicit massive Ca2+ release at high ionic strength and osmolality (at 1 mM free Mg2+). 6. In contrast, when the total Mg2+ and ATP concentrations were raised about threefold (free [Mg2+] increased 2.7-fold) along with the osmolality and ionic strength, the ability of depolarization to elicit Ca2+ release was greatly hindered. 7. Osmotic compression of the skinned fibres to their in situ diameter by addition of 4% polyvinylpyrrolidone (PVP-40), substantially potentiated the depolarization-induced force responses, due partly to an increase in the Ca(2+)-sensitivity of the contractile apparatus. 8. These results indicate how increased intracellular osmolality, ionic strength and [Mg2+] produce the transient contraction and subsequent inhibition of tetanic tension in intact muscle fibres exposed to hypertonic solutions.
Full text
PDF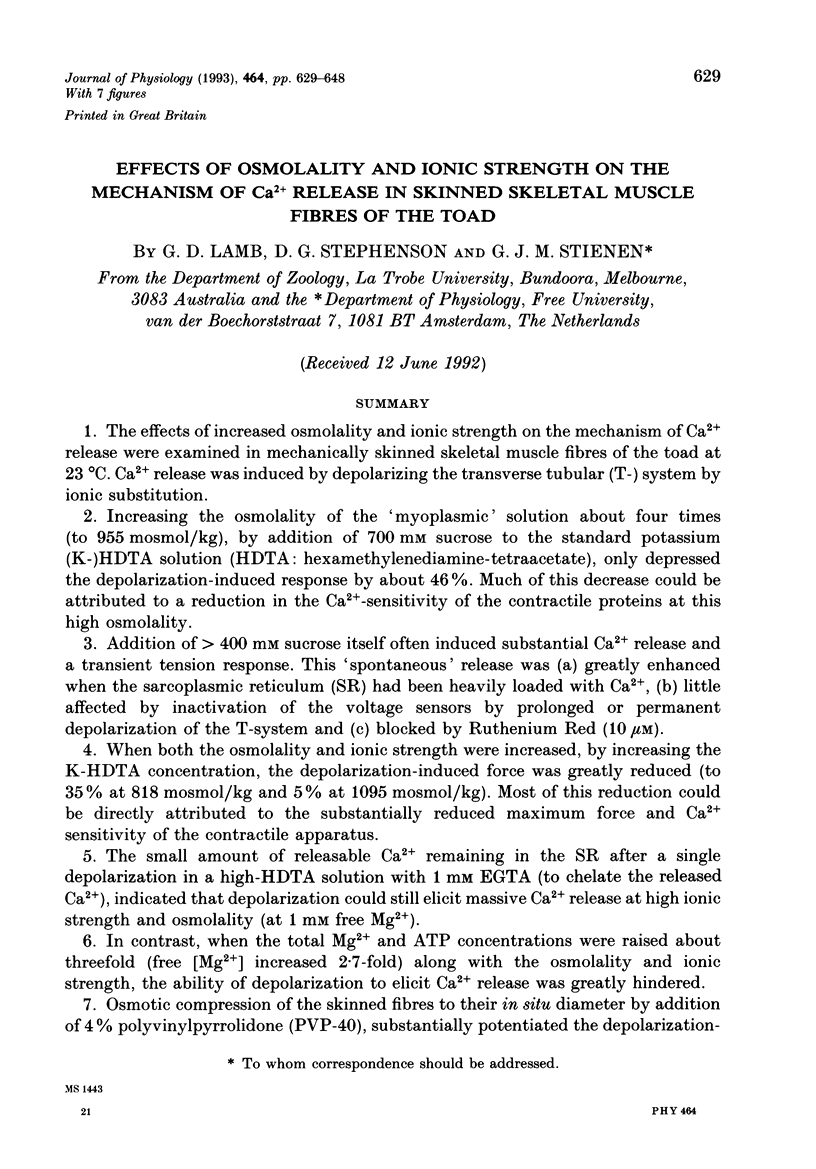
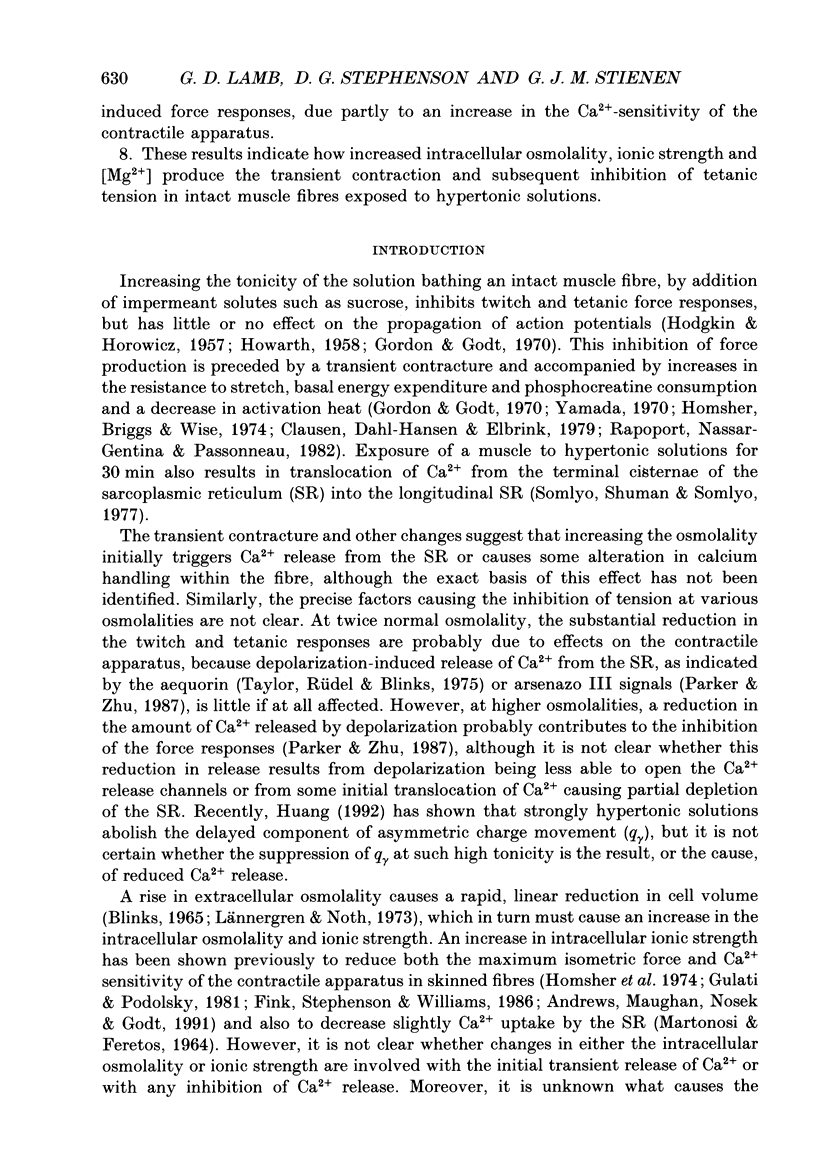
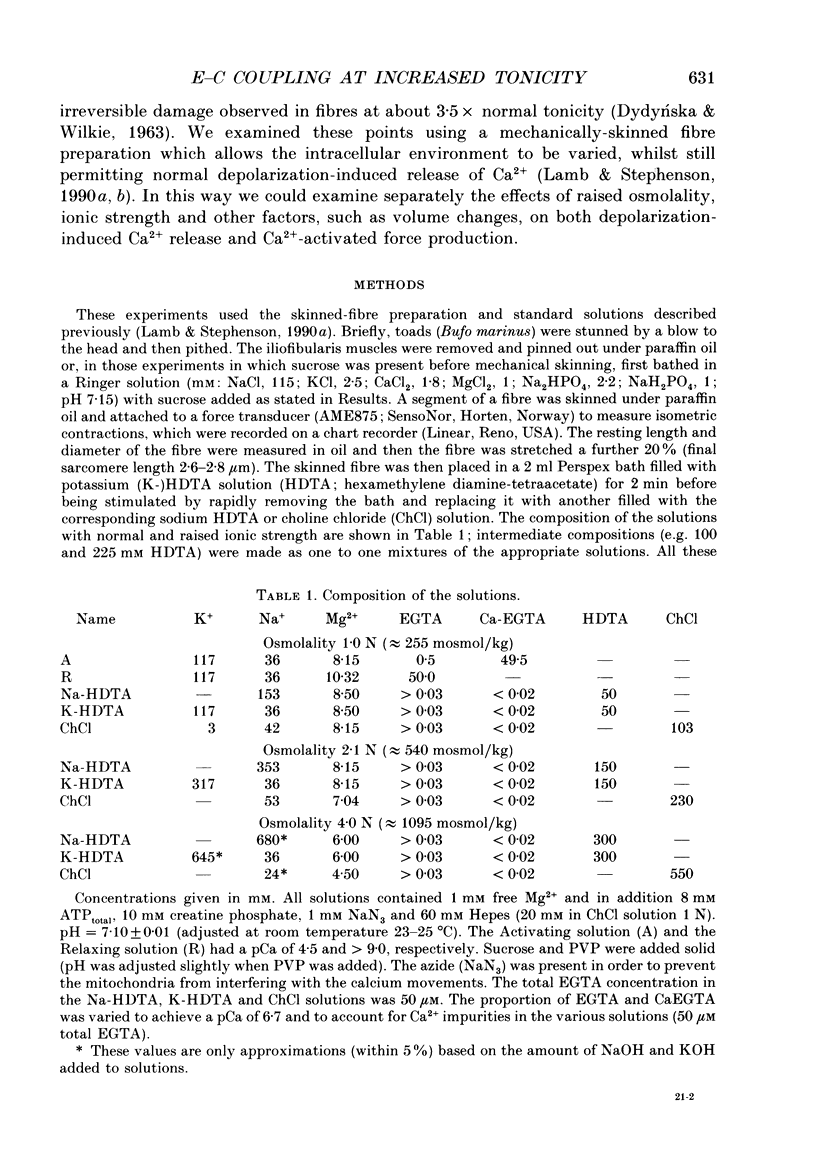
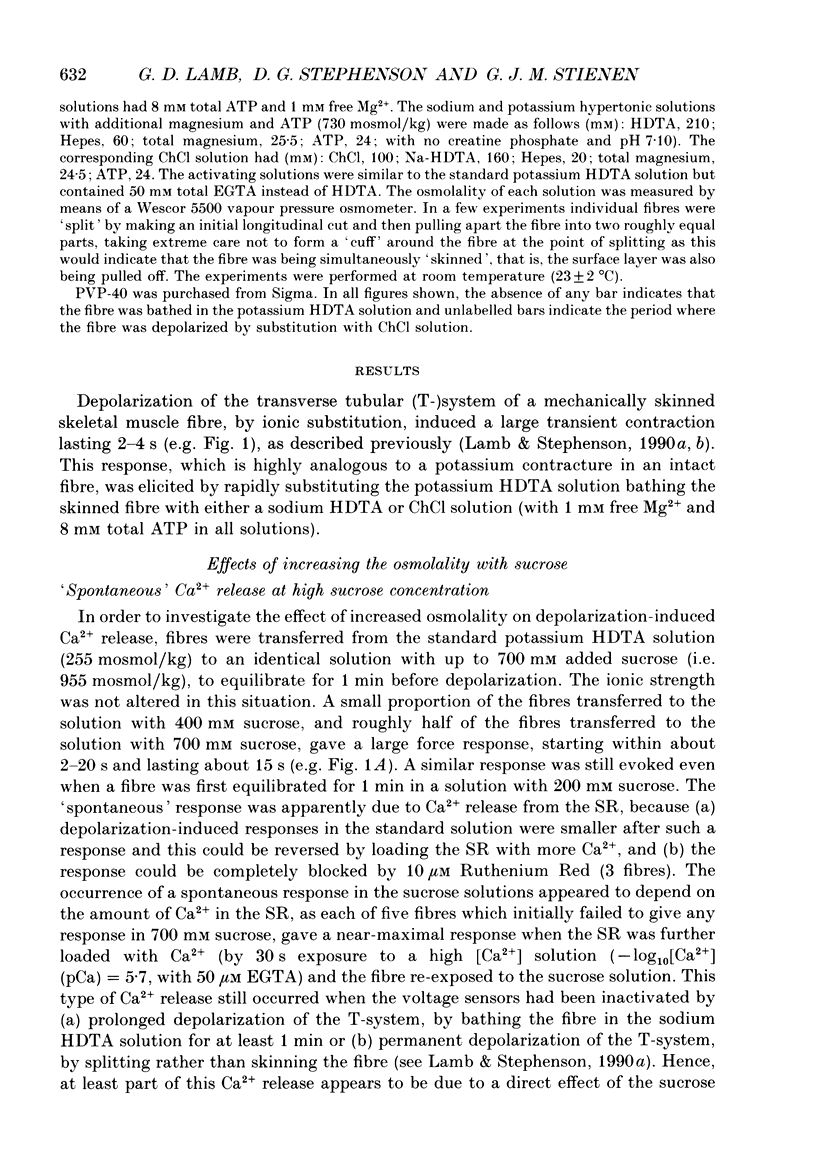
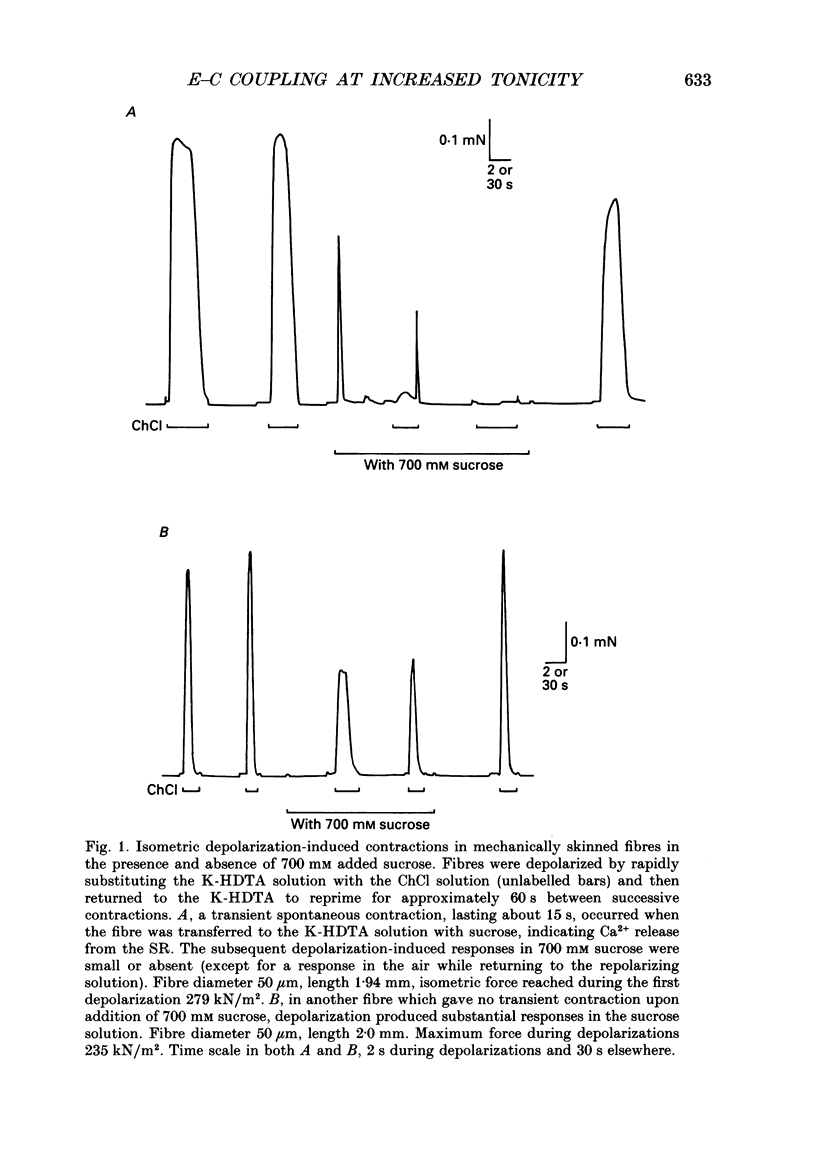
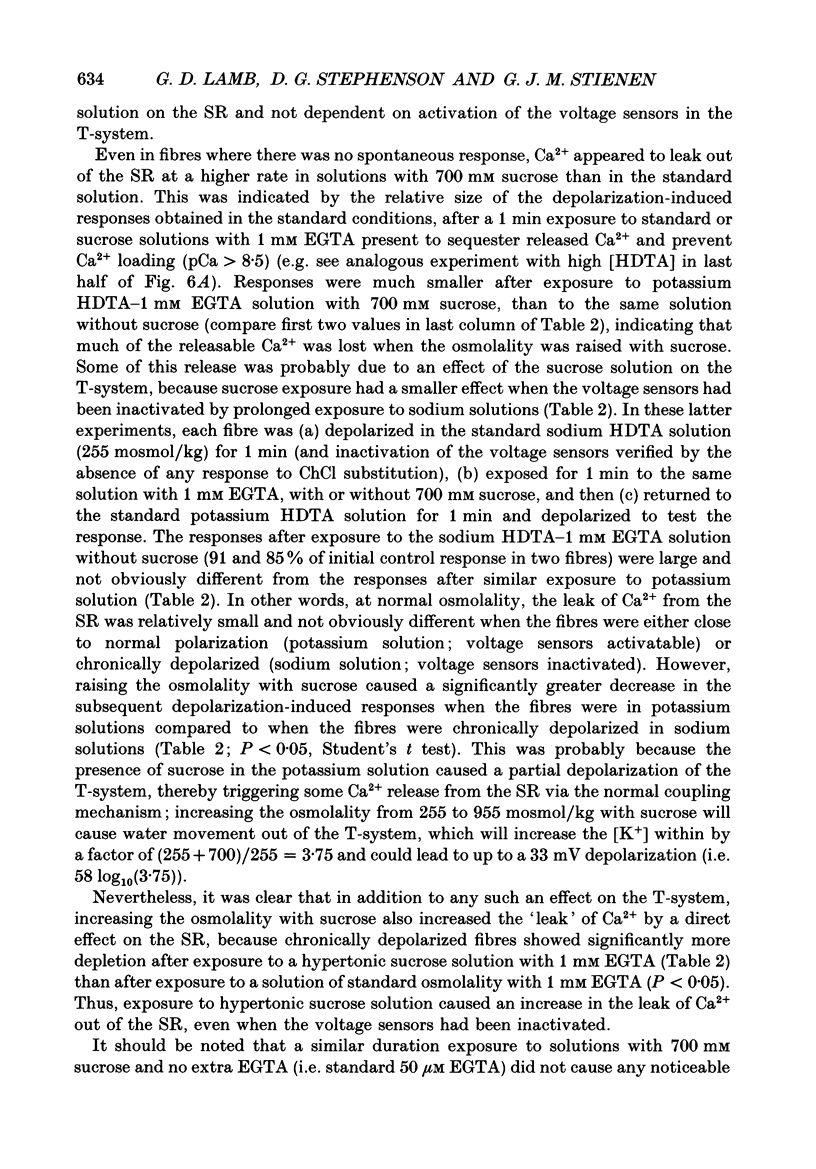
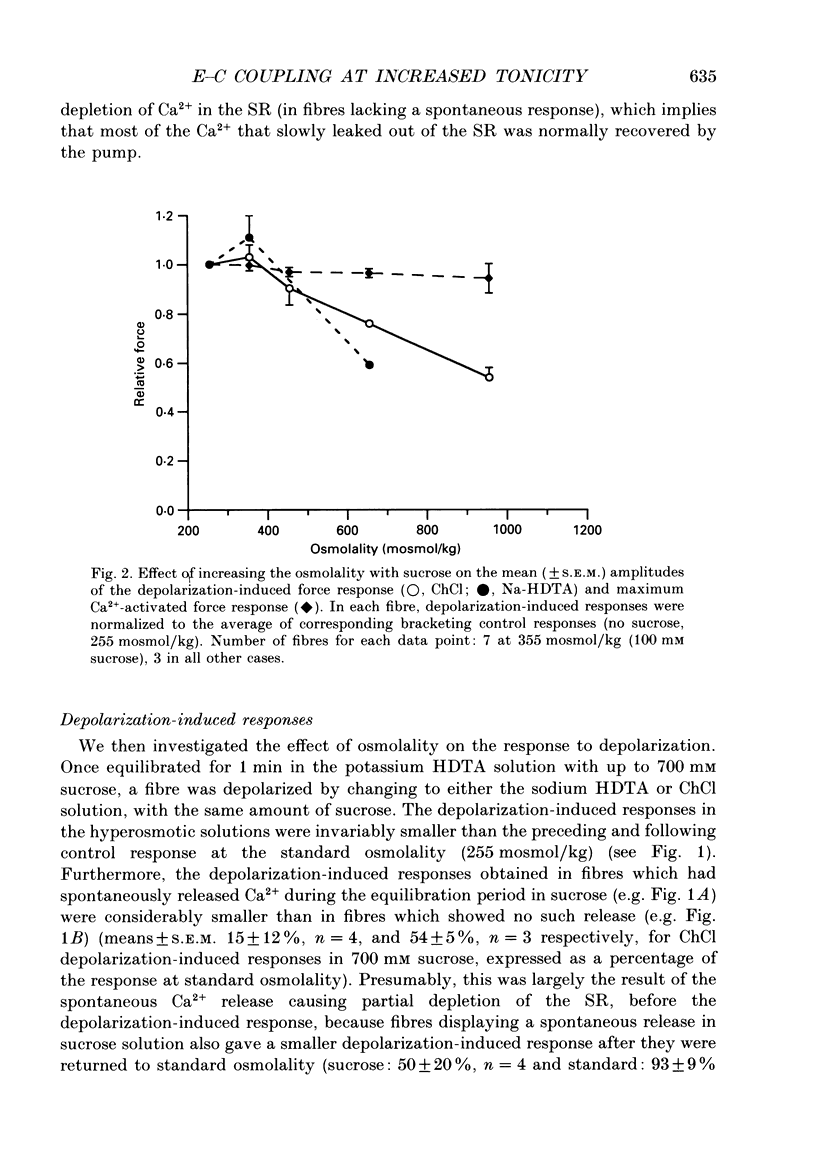
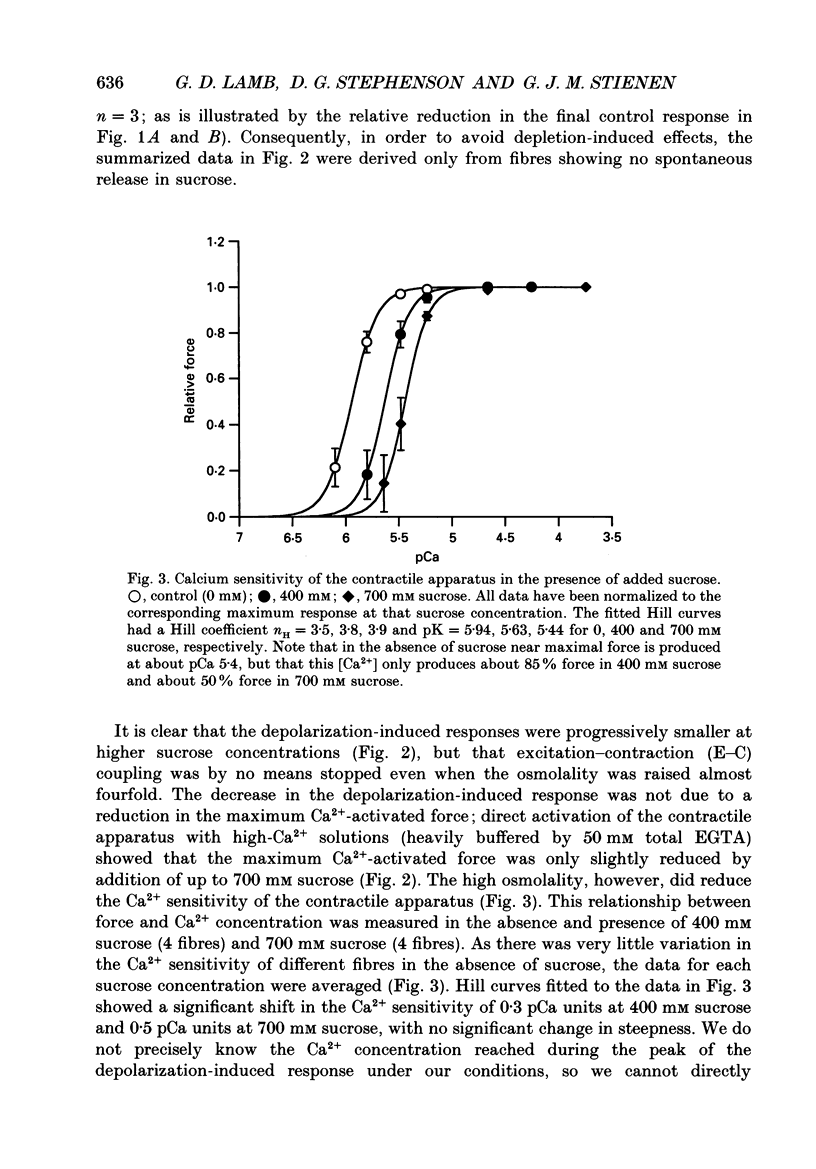
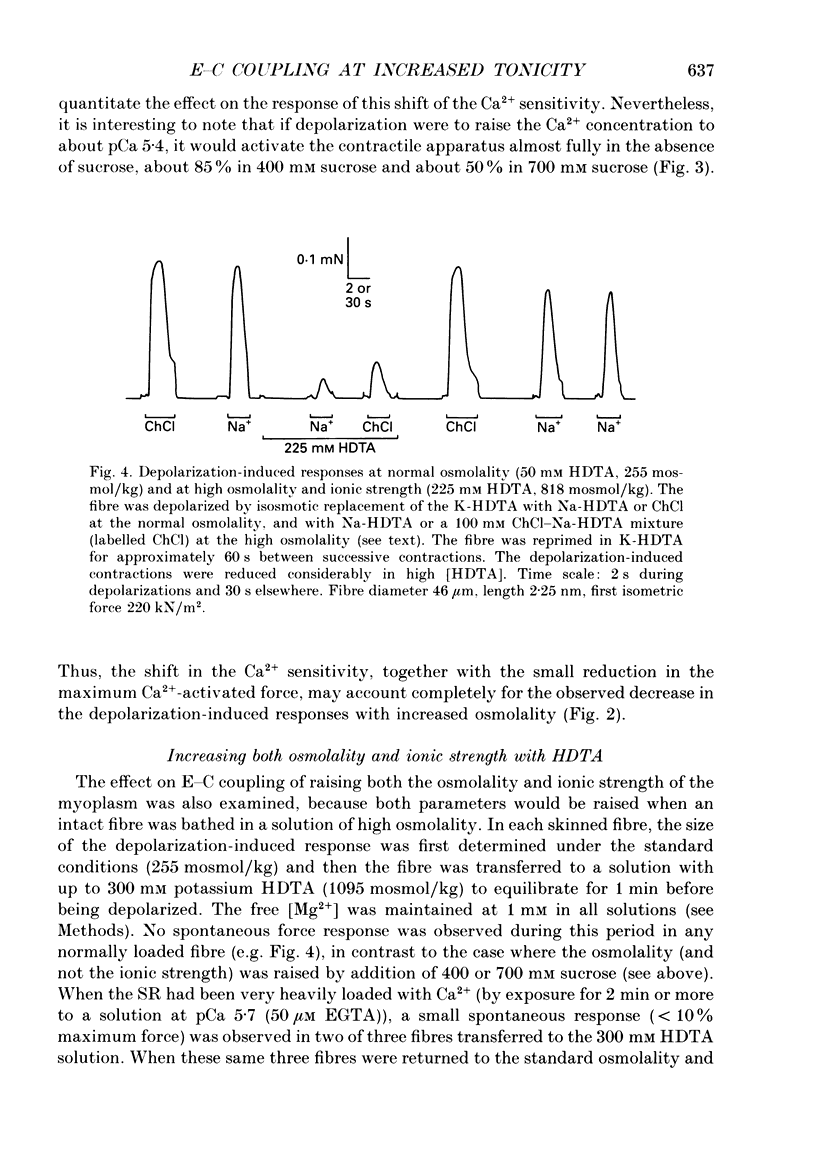
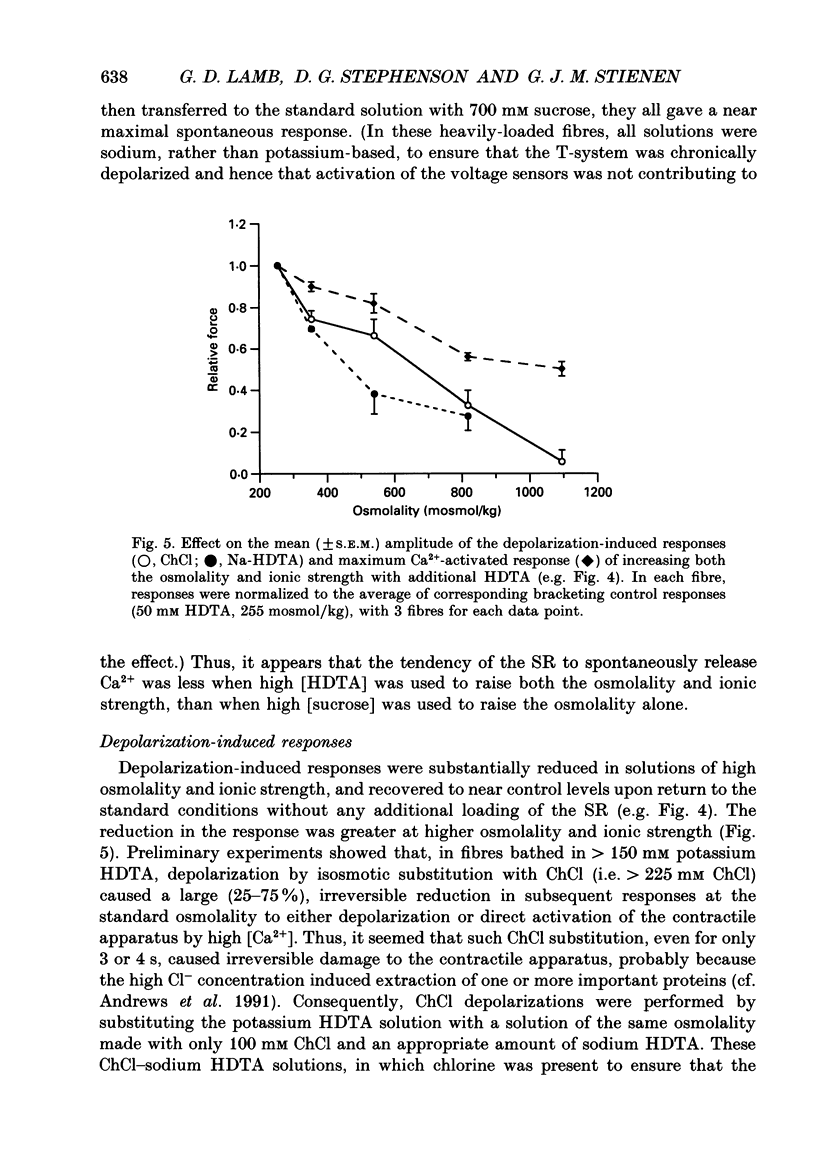
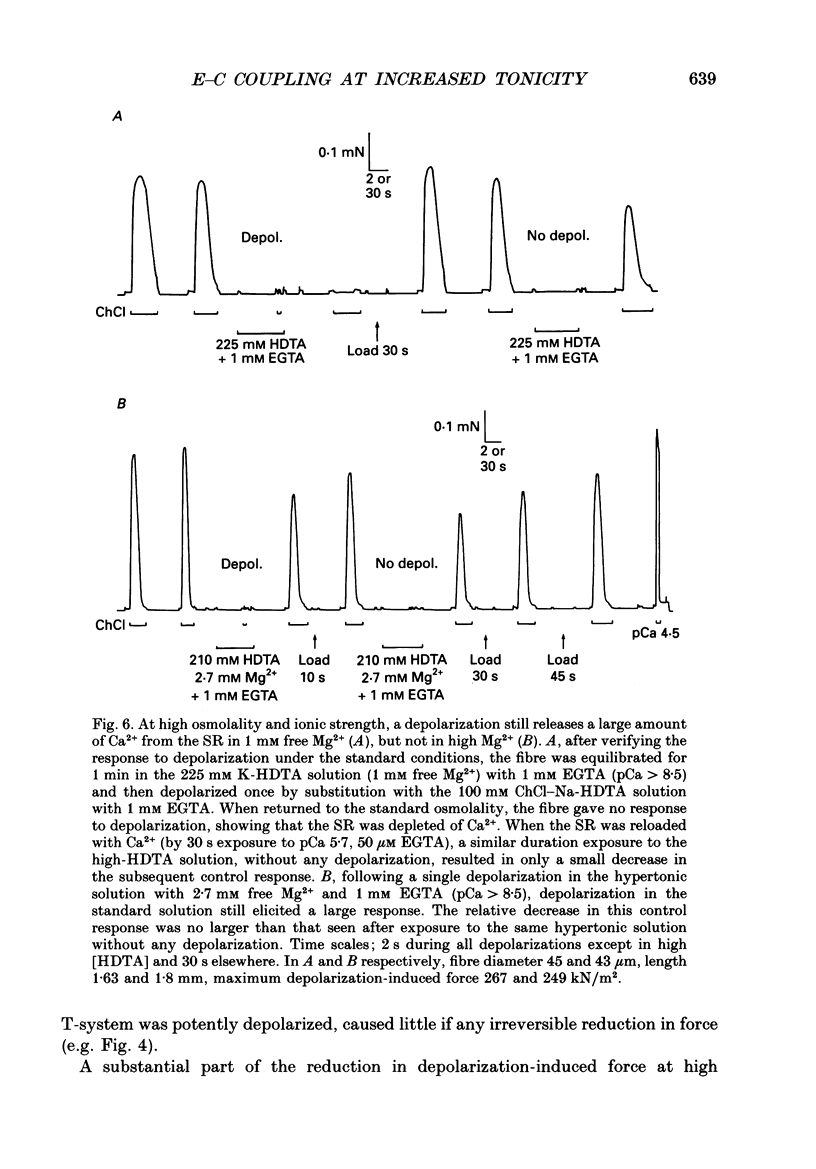
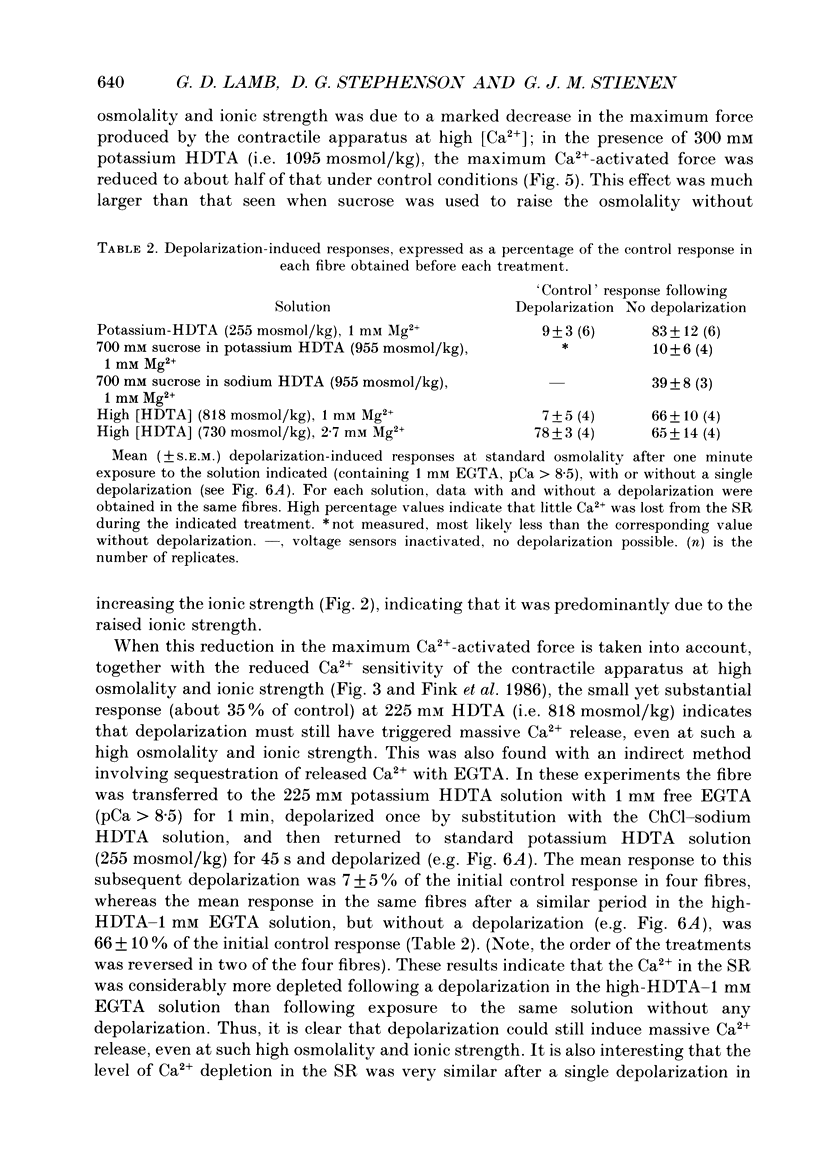
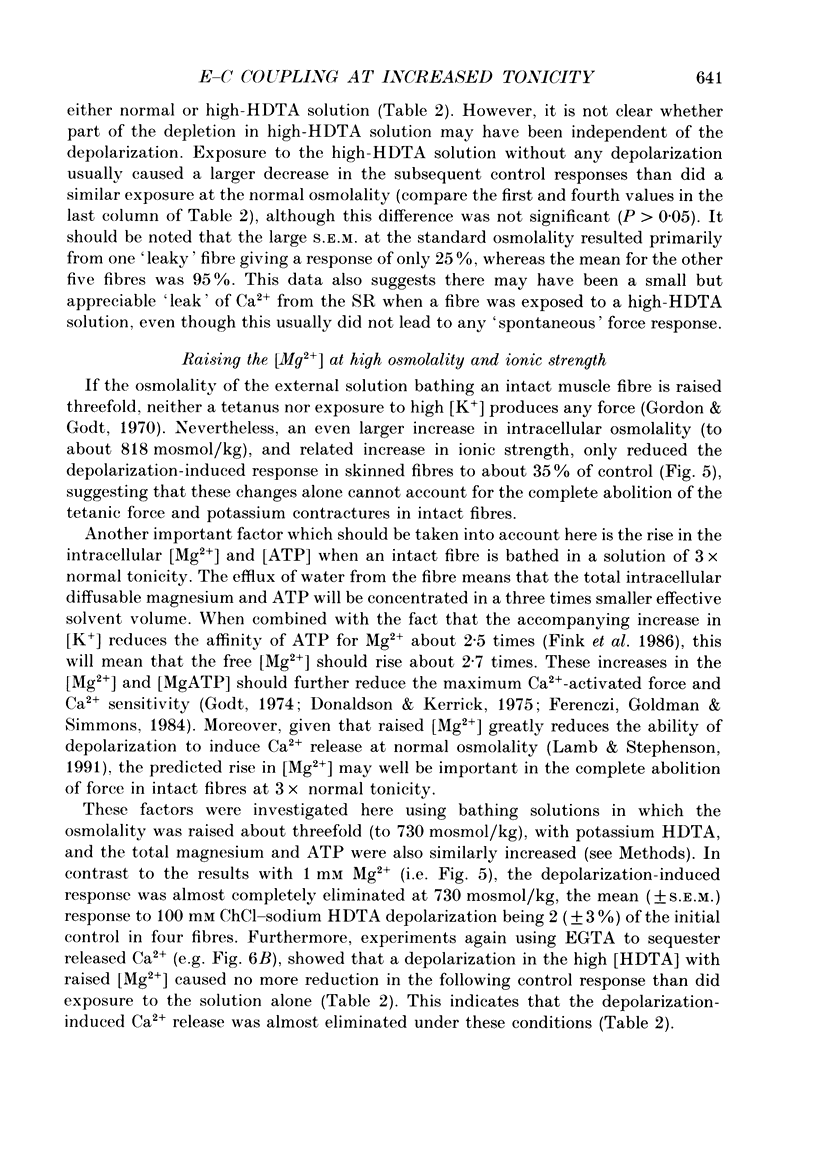
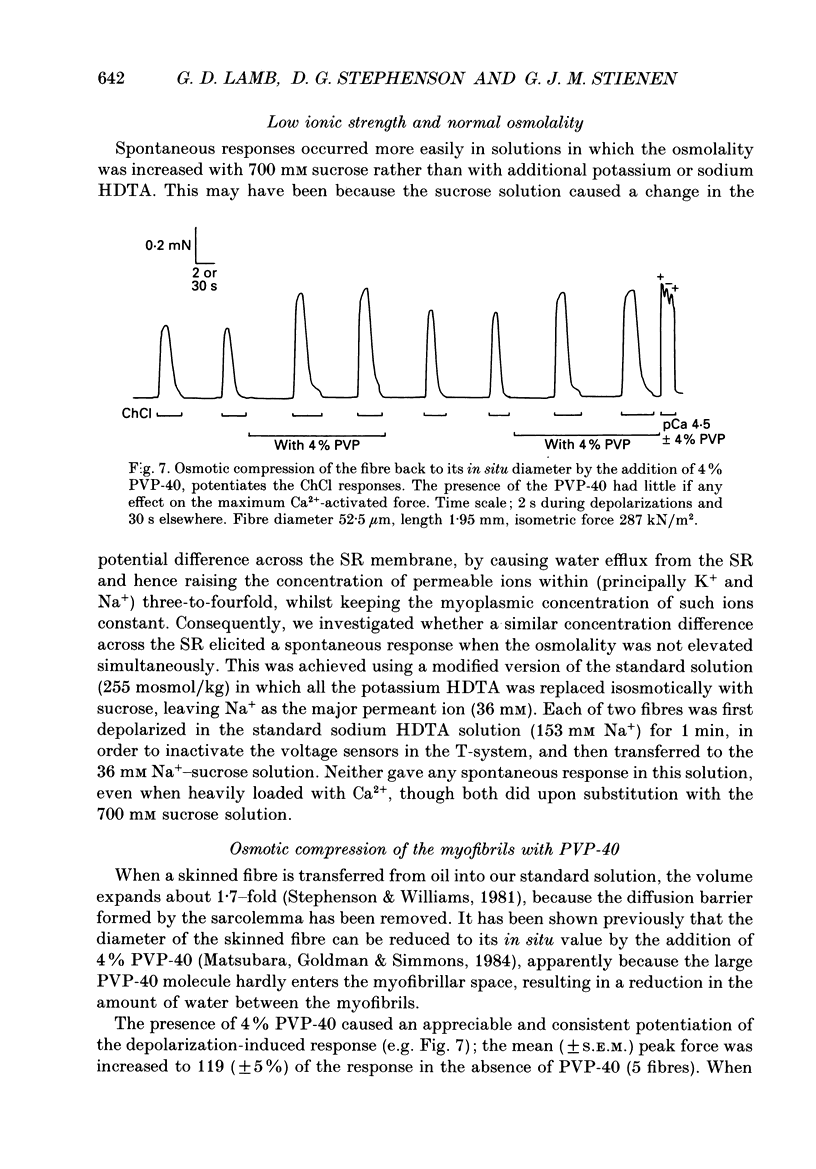
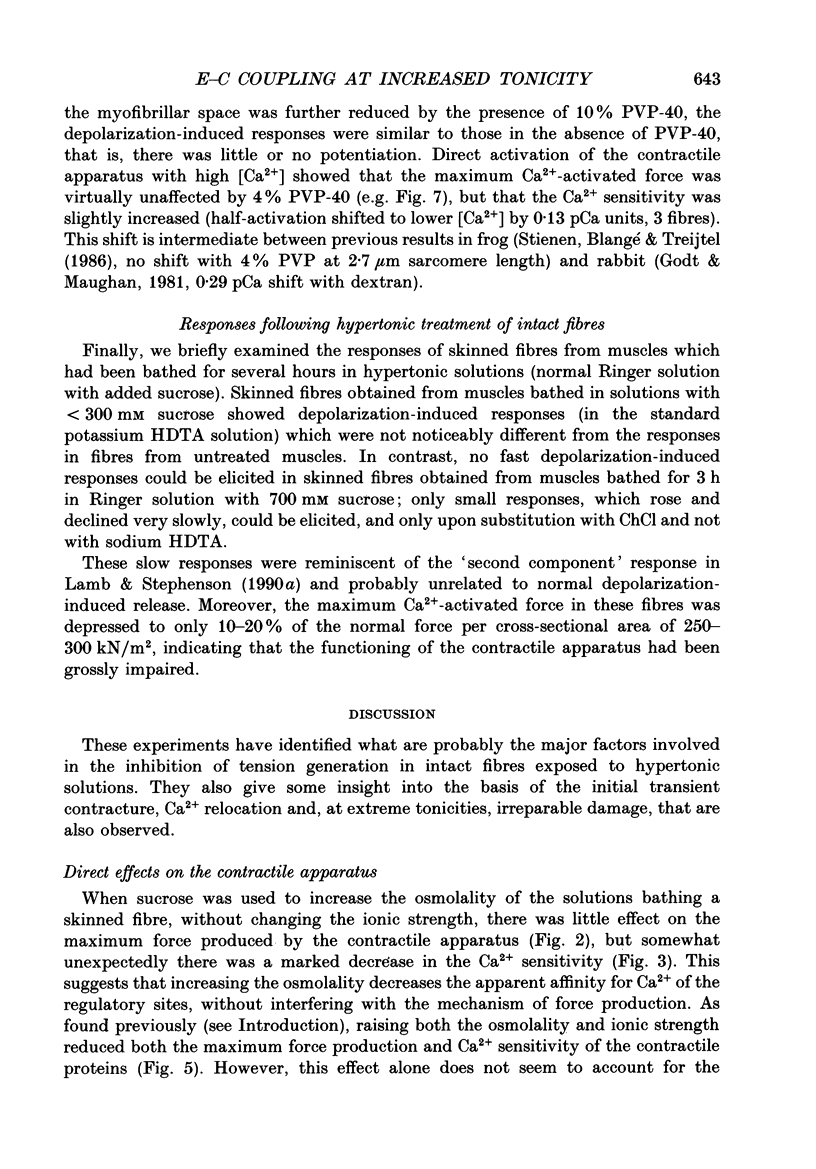
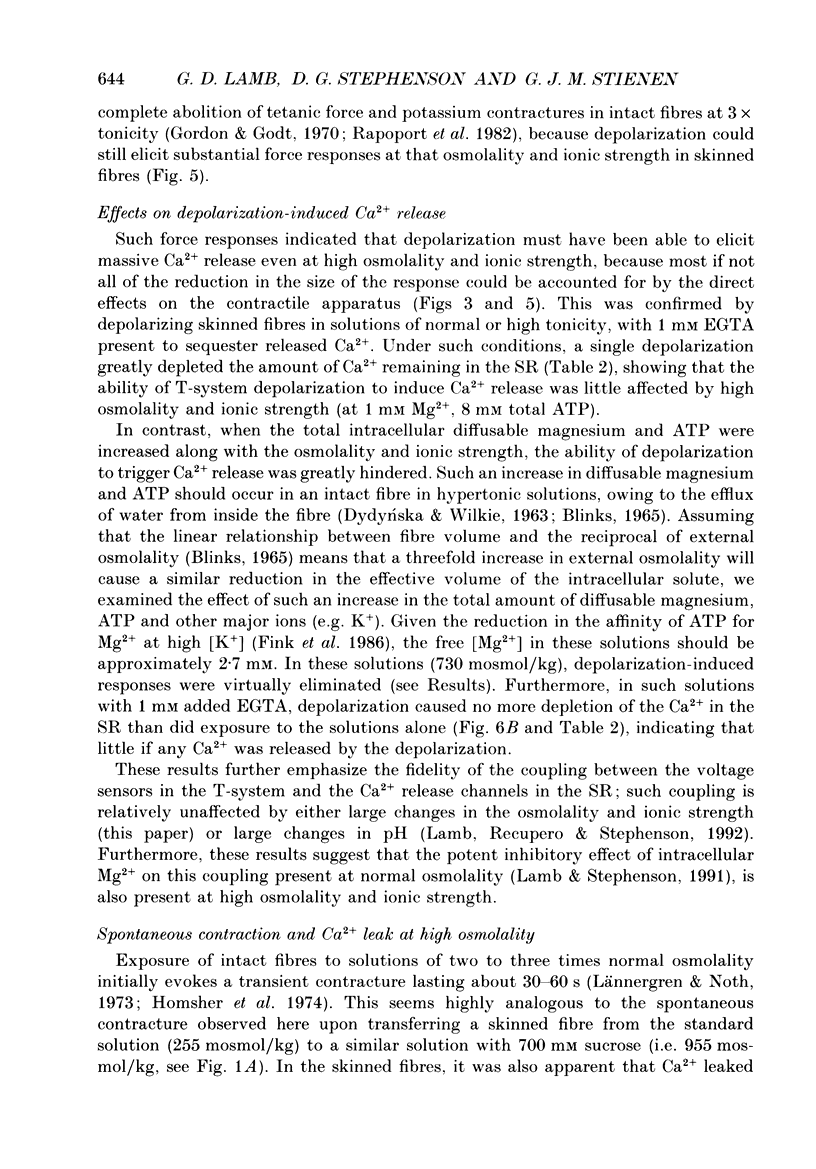
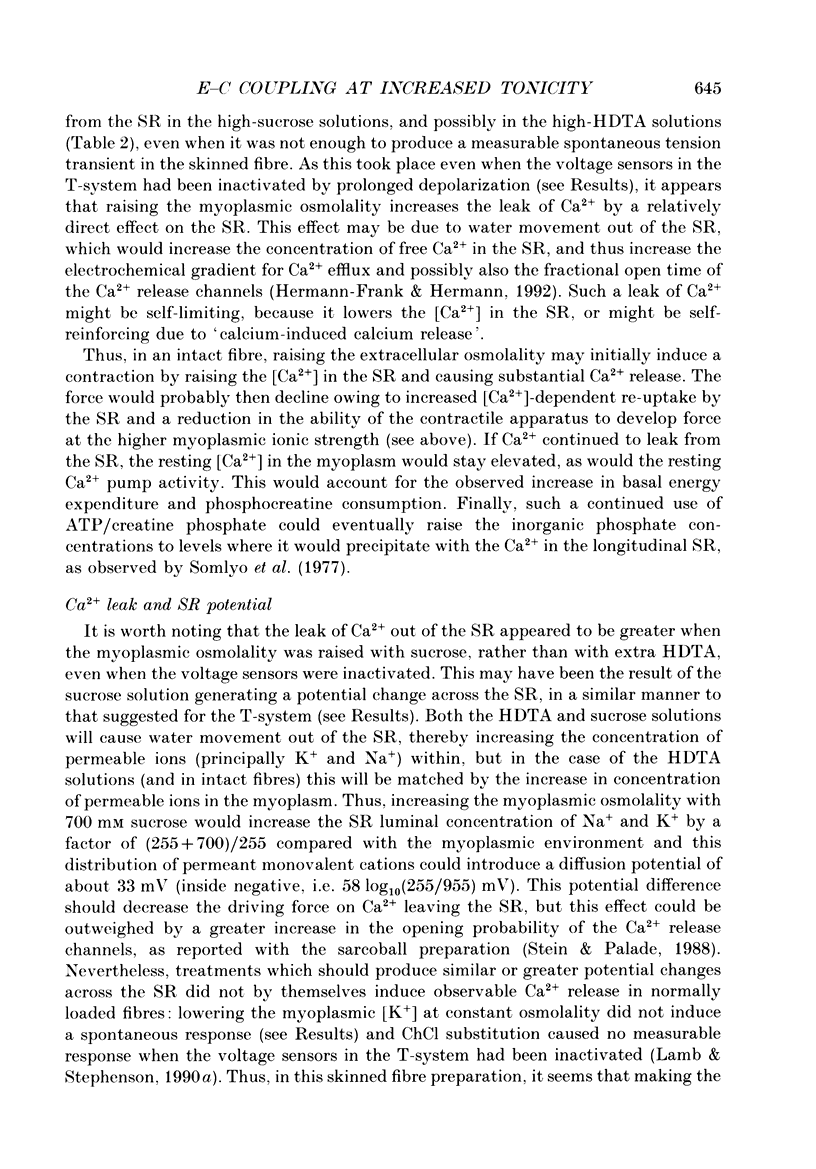
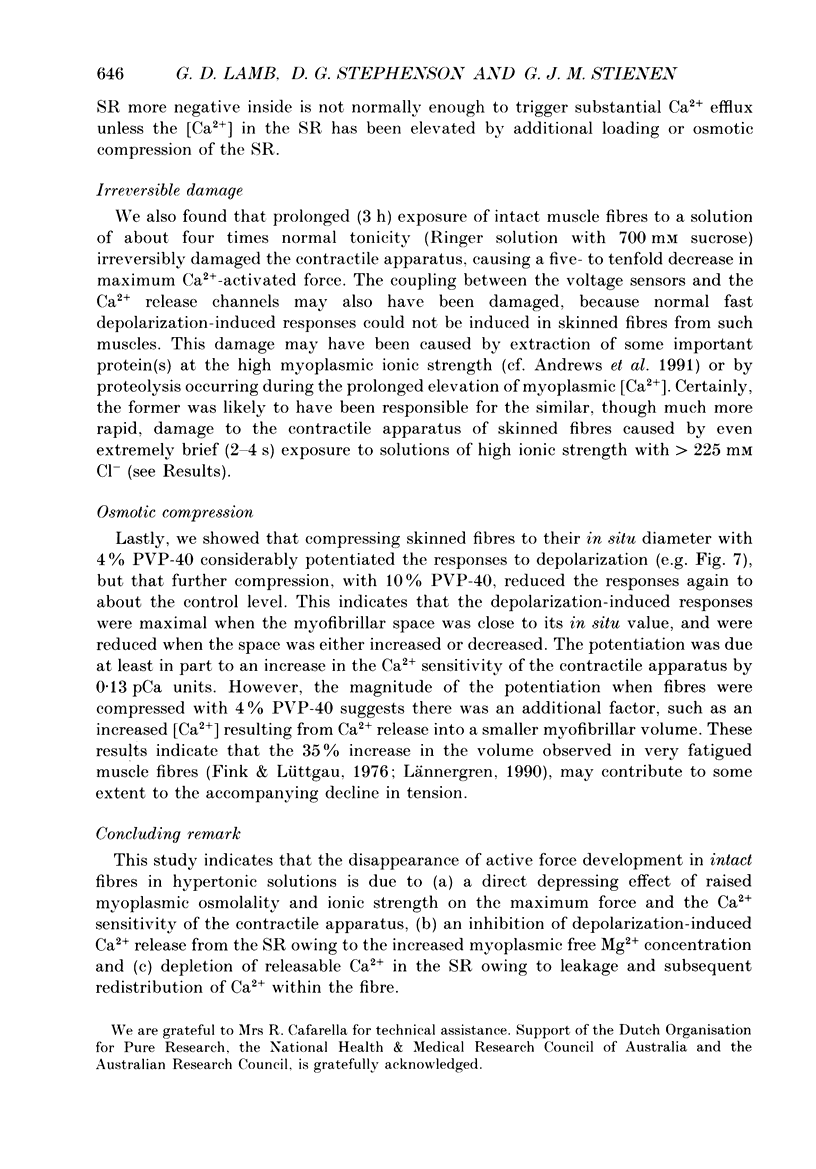
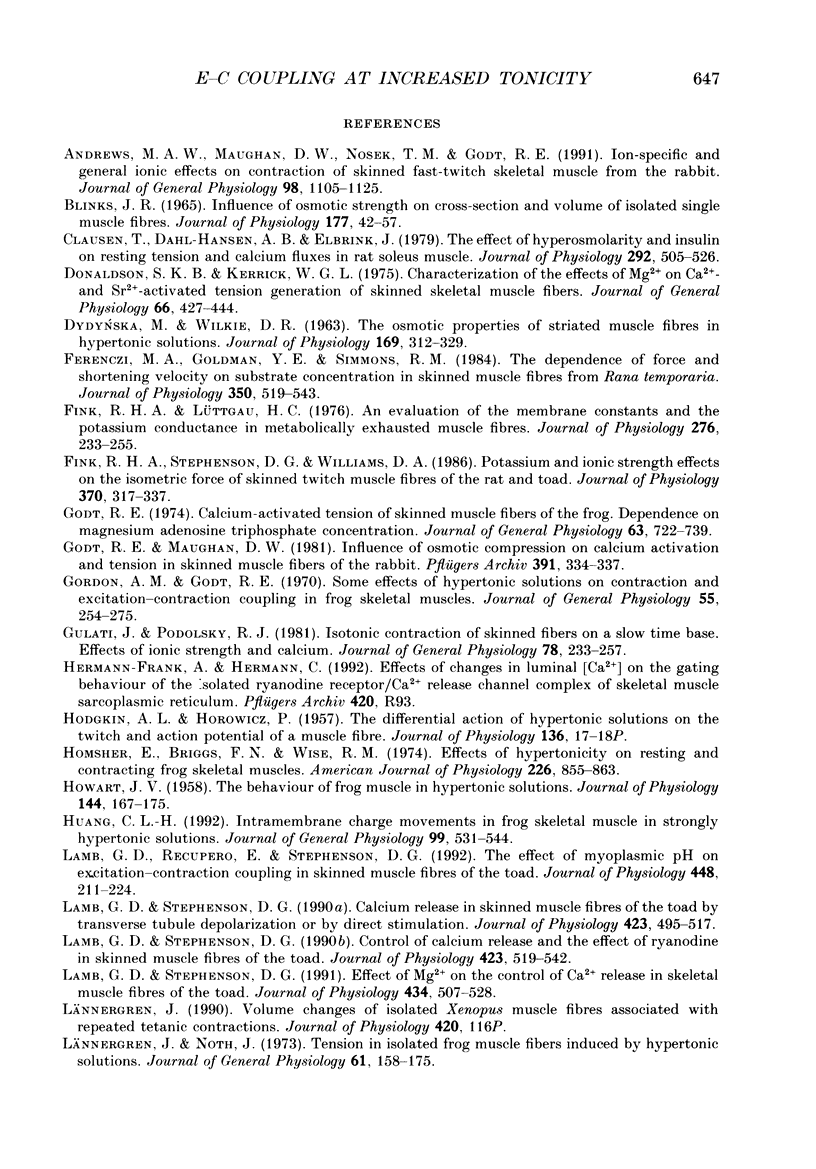
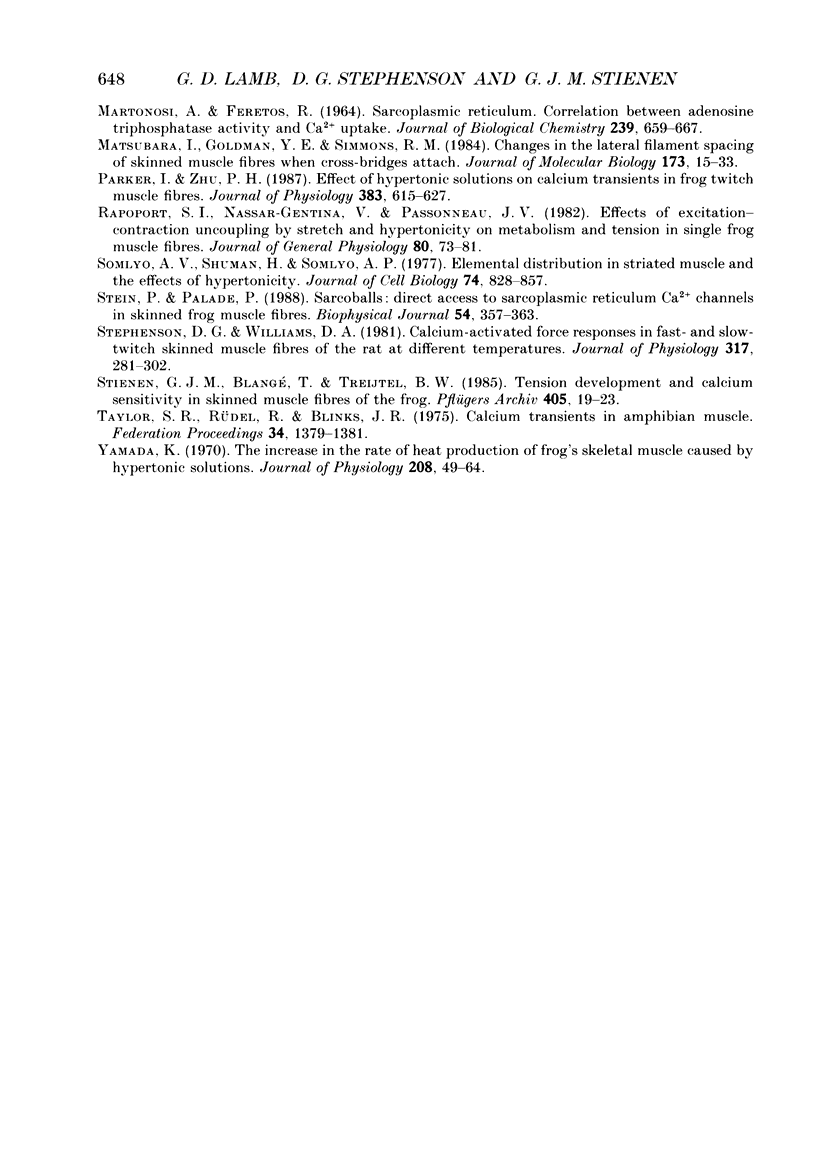
Selected References
These references are in PubMed. This may not be the complete list of references from this article.
- Andrews M. A., Maughan D. W., Nosek T. M., Godt R. E. Ion-specific and general ionic effects on contraction of skinned fast-twitch skeletal muscle from the rabbit. J Gen Physiol. 1991 Dec;98(6):1105–1125. doi: 10.1085/jgp.98.6.1105. [DOI] [PMC free article] [PubMed] [Google Scholar]
- BLINKS J. R. INFLUENCE OF OSMOTIC STRENGTH ON CROSS-SECTION AND VOLUME OF ISOLATED SINGLE MUSCLE FIBRES. J Physiol. 1965 Mar;177:42–57. doi: 10.1113/jphysiol.1965.sp007574. [DOI] [PMC free article] [PubMed] [Google Scholar]
- Clausen T., Dahl-Hansen A. B., Elbrink J. The effect of hyperosmolarity and insulin on resting tension and calcium fluxes in rat soleus muscle. J Physiol. 1979 Jul;292:505–526. doi: 10.1113/jphysiol.1979.sp012868. [DOI] [PMC free article] [PubMed] [Google Scholar]
- DYDYNSKA M., WILKIE D. R. THE OSMOTIC PROPERTIES OF STRIATED MUSCLE FIBERS IN HYPERTONIC SOLUTIONS. J Physiol. 1963 Nov;169:312–329. doi: 10.1113/jphysiol.1963.sp007258. [DOI] [PMC free article] [PubMed] [Google Scholar]
- Donaldson S. K., Kerrick W. G. Characterization of the effects of Mg2+ on Ca2+- and Sr2+-activated tension generation of skinned skeletal muscle fibers. J Gen Physiol. 1975 Oct;66(4):427–444. doi: 10.1085/jgp.66.4.427. [DOI] [PMC free article] [PubMed] [Google Scholar]
- Ferenczi M. A., Goldman Y. E., Simmons R. M. The dependence of force and shortening velocity on substrate concentration in skinned muscle fibres from Rana temporaria. J Physiol. 1984 May;350:519–543. doi: 10.1113/jphysiol.1984.sp015216. [DOI] [PMC free article] [PubMed] [Google Scholar]
- Fink R. H., Stephenson D. G., Williams D. A. Potassium and ionic strength effects on the isometric force of skinned twitch muscle fibres of the rat and toad. J Physiol. 1986 Jan;370:317–337. doi: 10.1113/jphysiol.1986.sp015937. [DOI] [PMC free article] [PubMed] [Google Scholar]
- Godt R. E. Calcium-activated tension of skinned muscle fibers of the frog. Dependence on magnesium adenosine triphosphate concentration. J Gen Physiol. 1974 Jun;63(6):722–739. doi: 10.1085/jgp.63.6.722. [DOI] [PMC free article] [PubMed] [Google Scholar]
- Godt R. E., Maughan D. W. Influence of osmotic compression on calcium activation and tension in skinned muscle fibers of the rabbit. Pflugers Arch. 1981 Oct;391(4):334–337. doi: 10.1007/BF00581519. [DOI] [PubMed] [Google Scholar]
- Gordon A. M., Godt R. E. Some effects of hypertonic solutions on contraction and excitation-contraction coupling in frog skeletal muscles. J Gen Physiol. 1970 Feb;55(2):254–275. doi: 10.1085/jgp.55.2.254. [DOI] [PMC free article] [PubMed] [Google Scholar]
- Gulati J., Podolsky R. J. Isotonic contraction of skinned muscle fibers on a slow time base: effects of ionic strength and calcium. J Gen Physiol. 1981 Sep;78(3):233–257. doi: 10.1085/jgp.78.3.233. [DOI] [PMC free article] [PubMed] [Google Scholar]
- HOWARTH J. V. The behaviour of frog muscle in hypertonic solutions. J Physiol. 1958 Nov 10;144(1):167–175. doi: 10.1113/jphysiol.1958.sp006093. [DOI] [PMC free article] [PubMed] [Google Scholar]
- Homsher E., Briggs F. N., Wise R. M. Effects of hypertonicity on resting and contracting frog skeletal muscles. Am J Physiol. 1974 Apr;226(4):855–863. doi: 10.1152/ajplegacy.1974.226.4.855. [DOI] [PubMed] [Google Scholar]
- Huang C. L. Intramembrane charge movements in frog skeletal muscle in strongly hypertonic solutions. J Gen Physiol. 1992 Apr;99(4):531–544. doi: 10.1085/jgp.99.4.531. [DOI] [PMC free article] [PubMed] [Google Scholar]
- Lamb G. D., Recupero E., Stephenson D. G. Effect of myoplasmic pH on excitation-contraction coupling in skeletal muscle fibres of the toad. J Physiol. 1992 Mar;448:211–224. doi: 10.1113/jphysiol.1992.sp019037. [DOI] [PMC free article] [PubMed] [Google Scholar]
- Lamb G. D., Stephenson D. G. Calcium release in skinned muscle fibres of the toad by transverse tubule depolarization or by direct stimulation. J Physiol. 1990 Apr;423:495–517. doi: 10.1113/jphysiol.1990.sp018036. [DOI] [PMC free article] [PubMed] [Google Scholar]
- Lamb G. D., Stephenson D. G. Control of calcium release and the effect of ryanodine in skinned muscle fibres of the toad. J Physiol. 1990 Apr;423:519–542. doi: 10.1113/jphysiol.1990.sp018037. [DOI] [PMC free article] [PubMed] [Google Scholar]
- Lamb G. D., Stephenson D. G. Effect of Mg2+ on the control of Ca2+ release in skeletal muscle fibres of the toad. J Physiol. 1991 Mar;434:507–528. doi: 10.1113/jphysiol.1991.sp018483. [DOI] [PMC free article] [PubMed] [Google Scholar]
- Lännergren J., Noth J. Tension in isolated frog muscle fibers induced by hypertonic solutions. J Gen Physiol. 1973 Feb;61(2):158–175. doi: 10.1085/jgp.61.2.158. [DOI] [PMC free article] [PubMed] [Google Scholar]
- MARTONOSI A., FERETOS R. SARCOPLASMIC RETICULUM. II. CORRELATION BETWEEN ADENOSINE TRIPHOSPHATASE ACTIVITY AND CA++ UPTAKE. J Biol Chem. 1964 Feb;239:659–668. [PubMed] [Google Scholar]
- Matsubara I., Goldman Y. E., Simmons R. M. Changes in the lateral filament spacing of skinned muscle fibres when cross-bridges attach. J Mol Biol. 1984 Feb 15;173(1):15–33. doi: 10.1016/0022-2836(84)90401-7. [DOI] [PubMed] [Google Scholar]
- Parker I., Zhu P. H. Effects of hypertonic solutions on calcium transients in frog twitch muscle fibres. J Physiol. 1987 Feb;383:615–627. doi: 10.1113/jphysiol.1987.sp016432. [DOI] [PMC free article] [PubMed] [Google Scholar]
- Rapoport S. I., Nassar-Gentina V., Passonneau J. V. Effects of excitation-contraction uncoupling by stretch and hypertonicity on metabolism and tension in single frog muscle fibers. J Gen Physiol. 1982 Jul;80(1):73–81. doi: 10.1085/jgp.80.1.73. [DOI] [PMC free article] [PubMed] [Google Scholar]
- Somlyo A. V., Shuman H., Somlyo A. P. Elemental distribution in striated muscle and the effects of hypertonicity. Electron probe analysis of cryo sections. J Cell Biol. 1977 Sep;74(3):828–857. doi: 10.1083/jcb.74.3.828. [DOI] [PMC free article] [PubMed] [Google Scholar]
- Stein P., Palade P. Sarcoballs: direct access to sarcoplasmic reticulum Ca2+-channels in skinned frog muscle fibers. Biophys J. 1988 Aug;54(2):357–363. doi: 10.1016/S0006-3495(88)82967-9. [DOI] [PMC free article] [PubMed] [Google Scholar]
- Stephenson D. G., Williams D. A. Calcium-activated force responses in fast- and slow-twitch skinned muscle fibres of the rat at different temperatures. J Physiol. 1981 Aug;317:281–302. doi: 10.1113/jphysiol.1981.sp013825. [DOI] [PMC free article] [PubMed] [Google Scholar]
- Stienen G. J., Blangé T., Treijtel B. W. Tension development and calcium sensitivity in skinned muscle fibres of the frog. Pflugers Arch. 1985 Sep;405(1):19–23. doi: 10.1007/BF00591092. [DOI] [PubMed] [Google Scholar]
- Taylor S. R., Rüdel R., Blinks J. R. Calcium transients in amphibian muscle. Fed Proc. 1975 Apr;34(5):1379–1381. [PubMed] [Google Scholar]
- Yamada K. The increase in the rate of heat production of frog's skeletal muscle caused by hypertonic solutions. J Physiol. 1970 May;208(1):49–64. doi: 10.1113/jphysiol.1970.sp009105. [DOI] [PMC free article] [PubMed] [Google Scholar]