Abstract
1. Membrane potential was recorded with intracellular microelectrodes from the smooth muscle of coronary arteries of guinea-pigs, and the responses to endothelium-derived relaxants were studied under a variety of conditions. 2. Stimulation of the endothelium with brief applications of acetylcholine or substance P evoked concentration-dependent hyperpolarizations that were complex in nature. A transient component, which is likely to result from endothelium-derived hyperpolarizing factor (EDHF), was followed by a slow component that resulted from the production of nitric oxide (NO) and a prostaglandin. 3. The ability of exogenous and endogenous NO and prostacyclin to hyperpolarize the membrane depended upon the smooth muscle being under stretch. Unstretched preparations responded to acetylcholine with only the transient component of hyperpolarization; NO and prostacyclin were without effect. 4. In stretched preparations exogenous NO and prostacyclin, and its synthetic analogue methyl prostacyclin (Iloprost), evoked hyperpolarization, and the slow component of the response induced by acetylcholine appeared. The amplitudes of these responses reached maximum when the tissues were stretched to the equivalent of approximately 50 mmHg. 5. From a resting membrane potential of -61 +/- 0.6 mV, exogenous NO and Iloprost hyperpolarized the smooth muscle to around -80 mV. The EC50 values for NO- and Iloprost-induced hyperpolarization were 2.6 x 10(-6) and 1.3 x 10(-8) M, respectively. 6. Coronary arterial smooth muscles from rats, rabbits and sheep also hyperpolarized in response to exogenous NO, although their sensitivities were less than those of preparations obtained from guinea-pigs. Iloprost hyperpolarized tissues from rabbits and sheep but not those obtained from rats. 7. It is concluded that the endothelial lining of coronary arteries can release three factors, EDHF, NO and prostacyclin, all of which can hyperpolarize the membrane of the smooth muscle. The relative proportions and significance of each factor depends on the amount of stretch, on the artery and on the species of animal.
Full text
PDF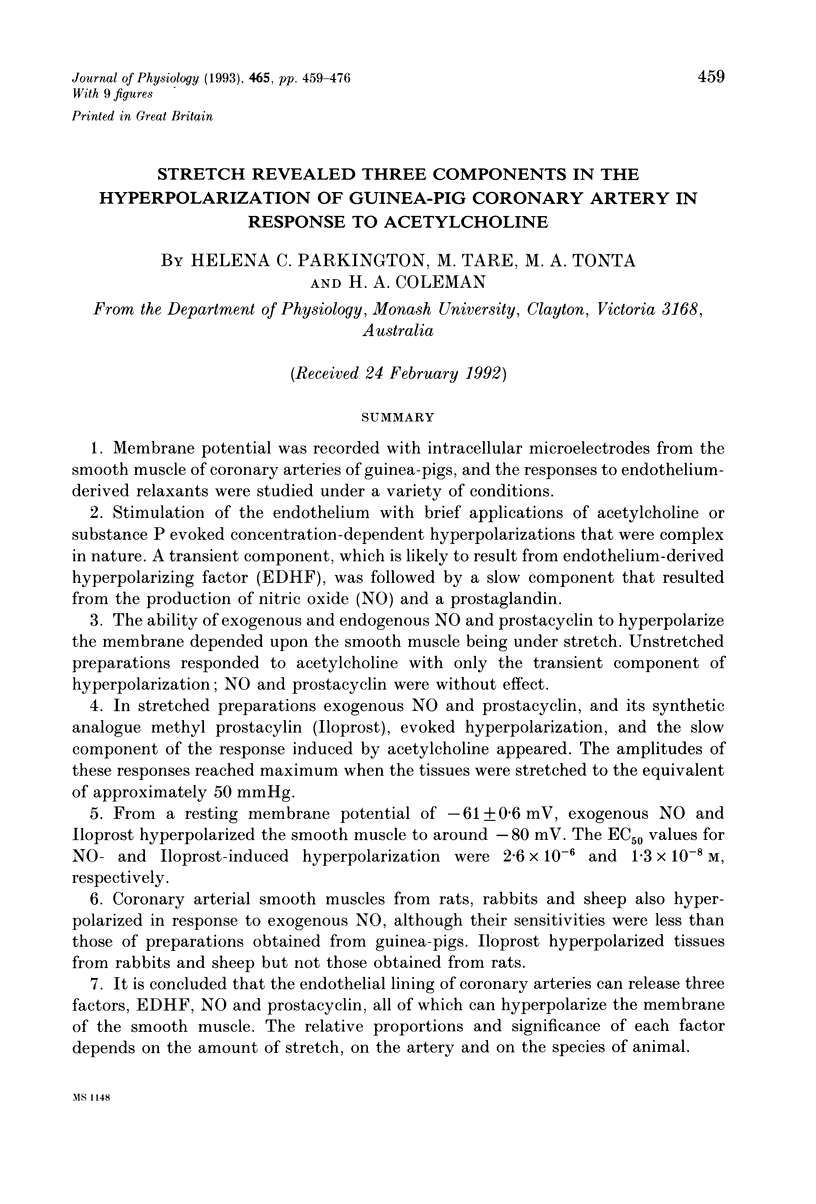
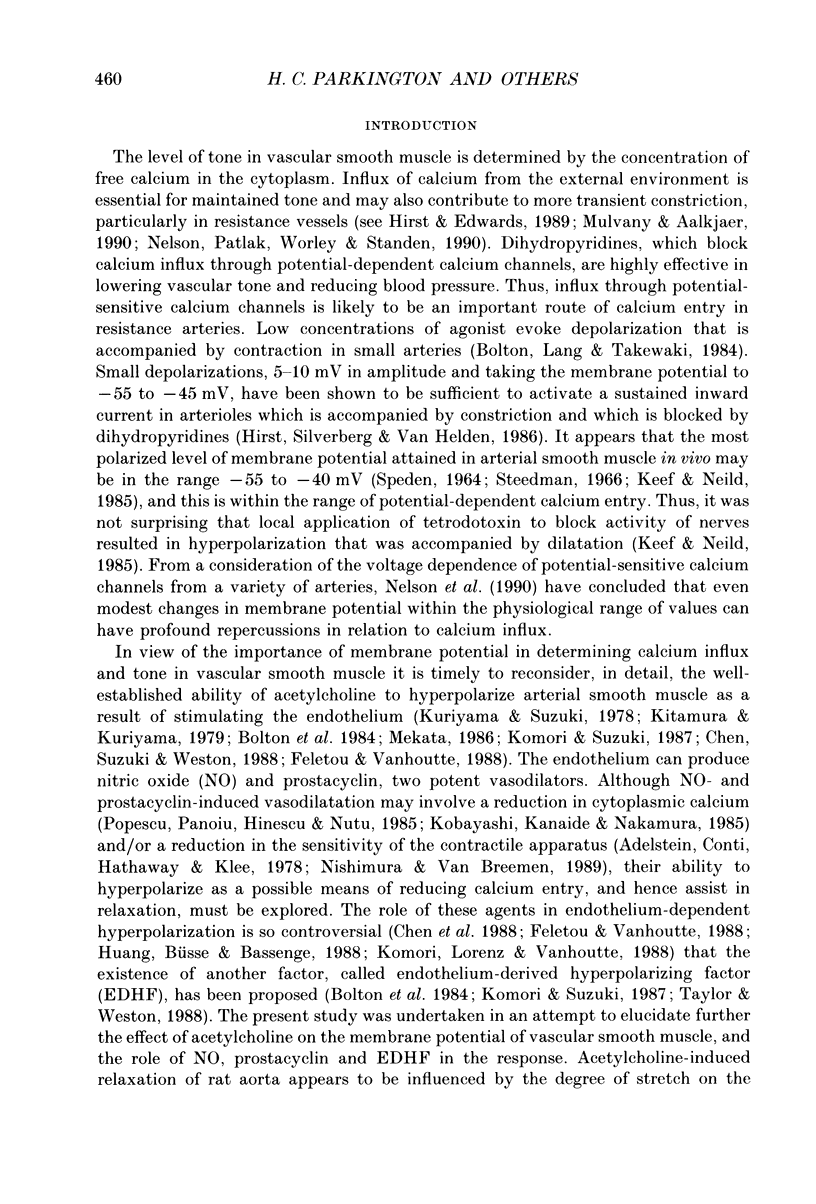
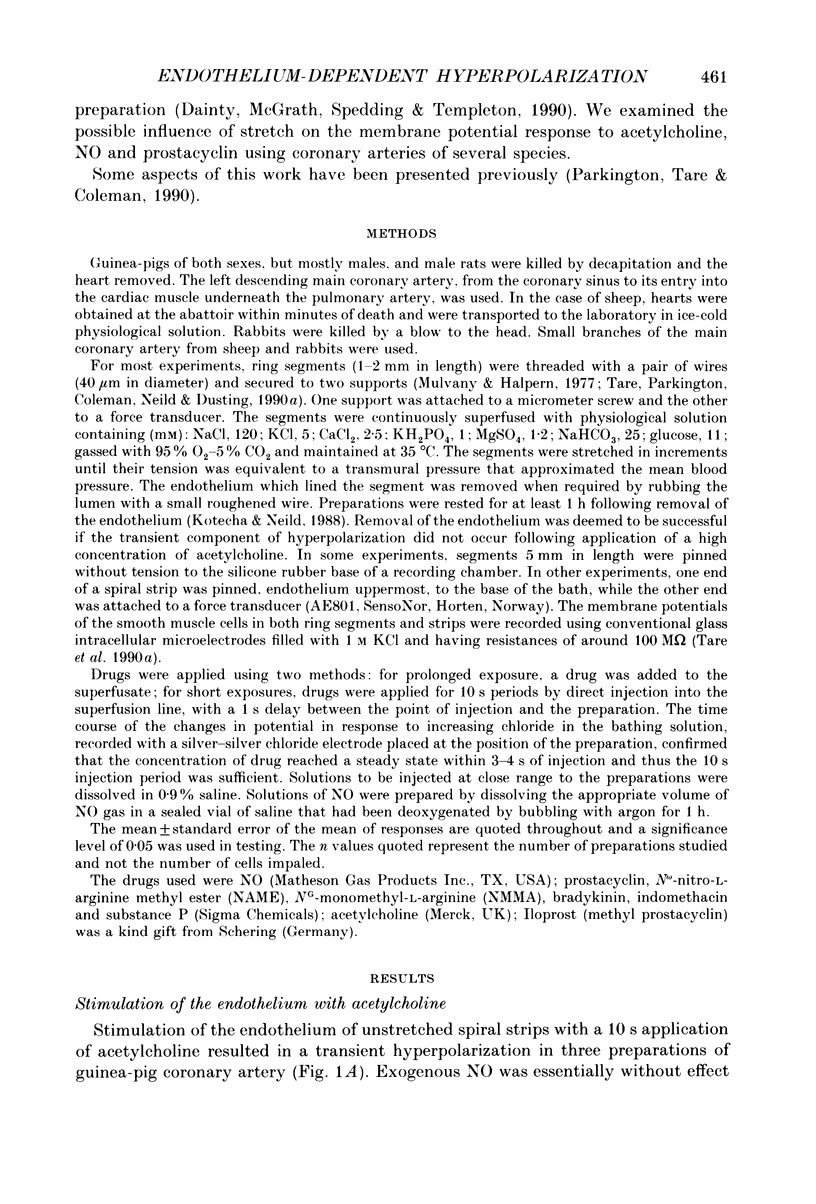
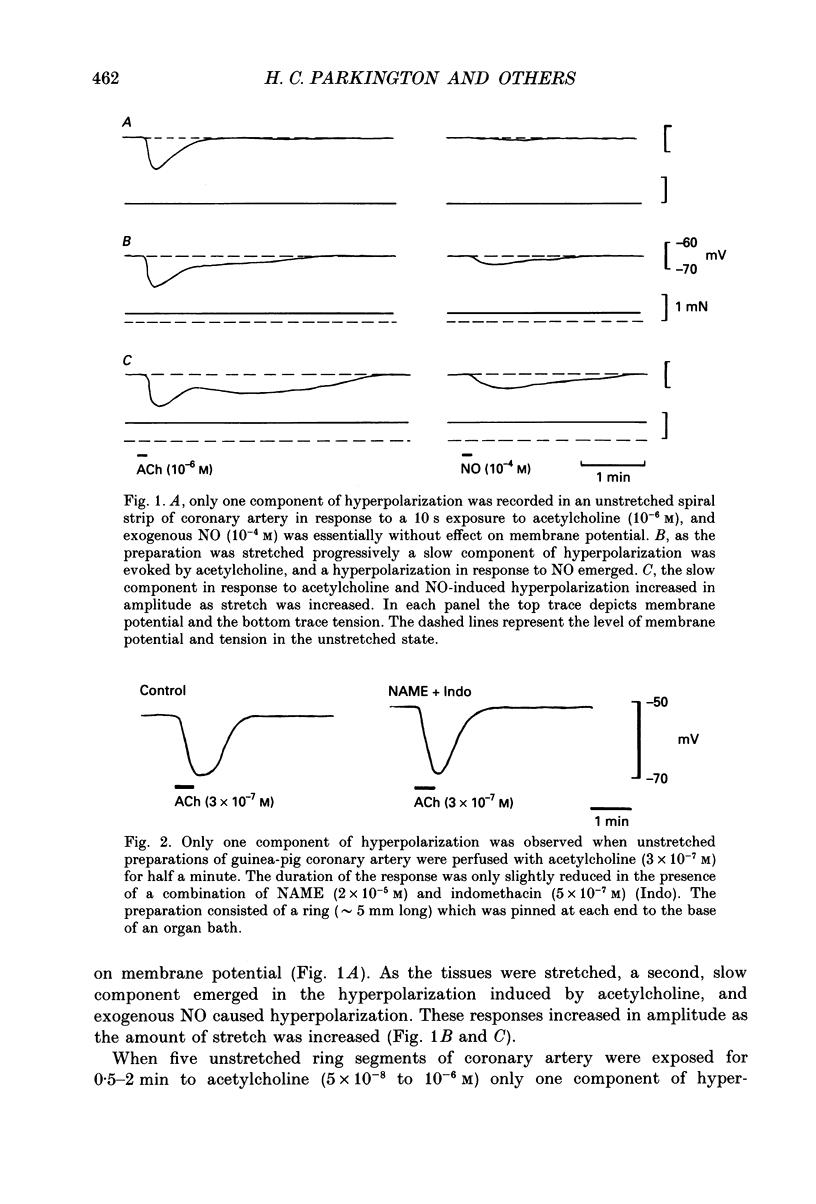
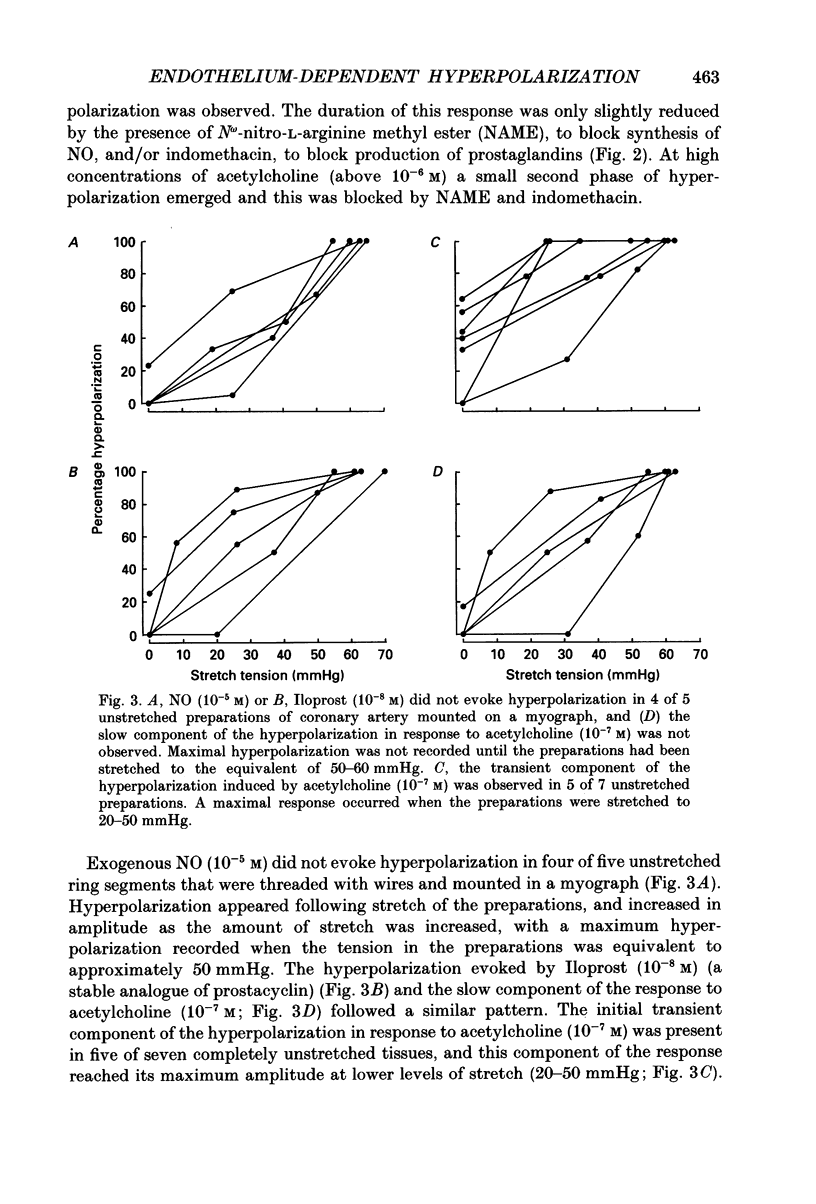
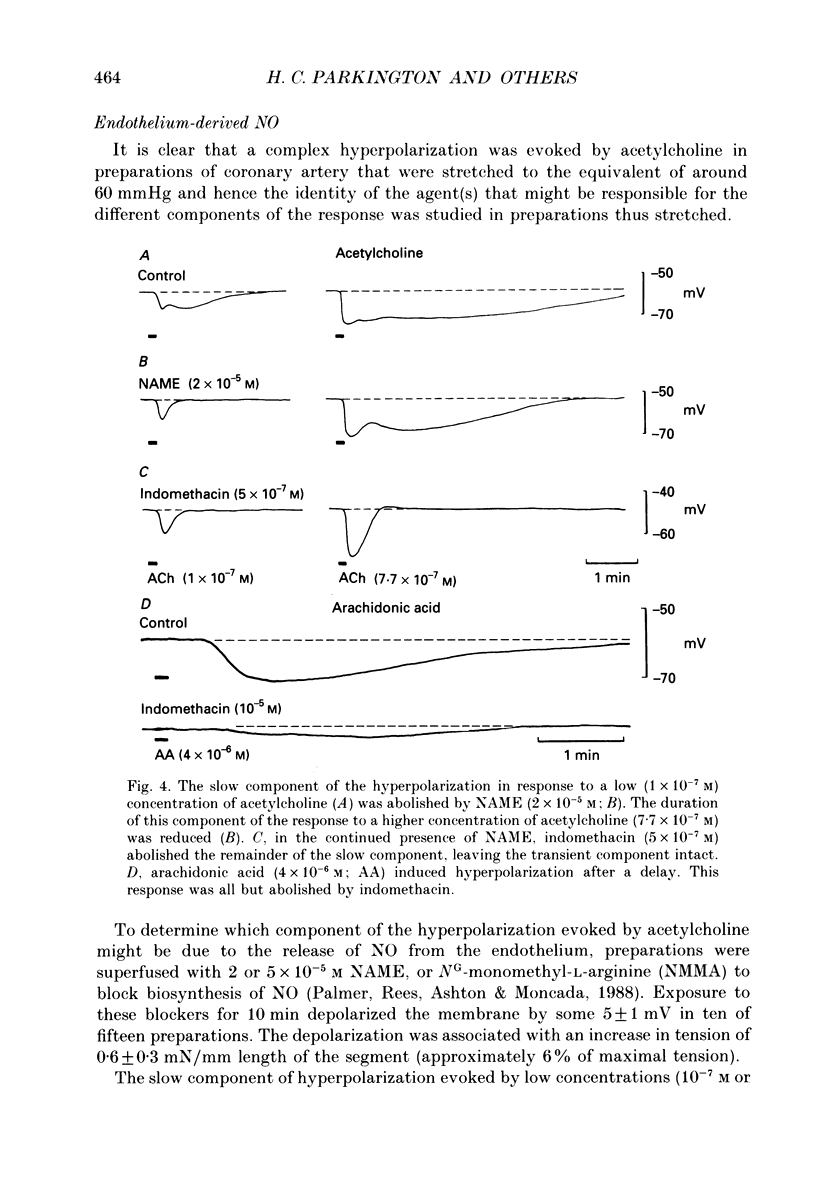
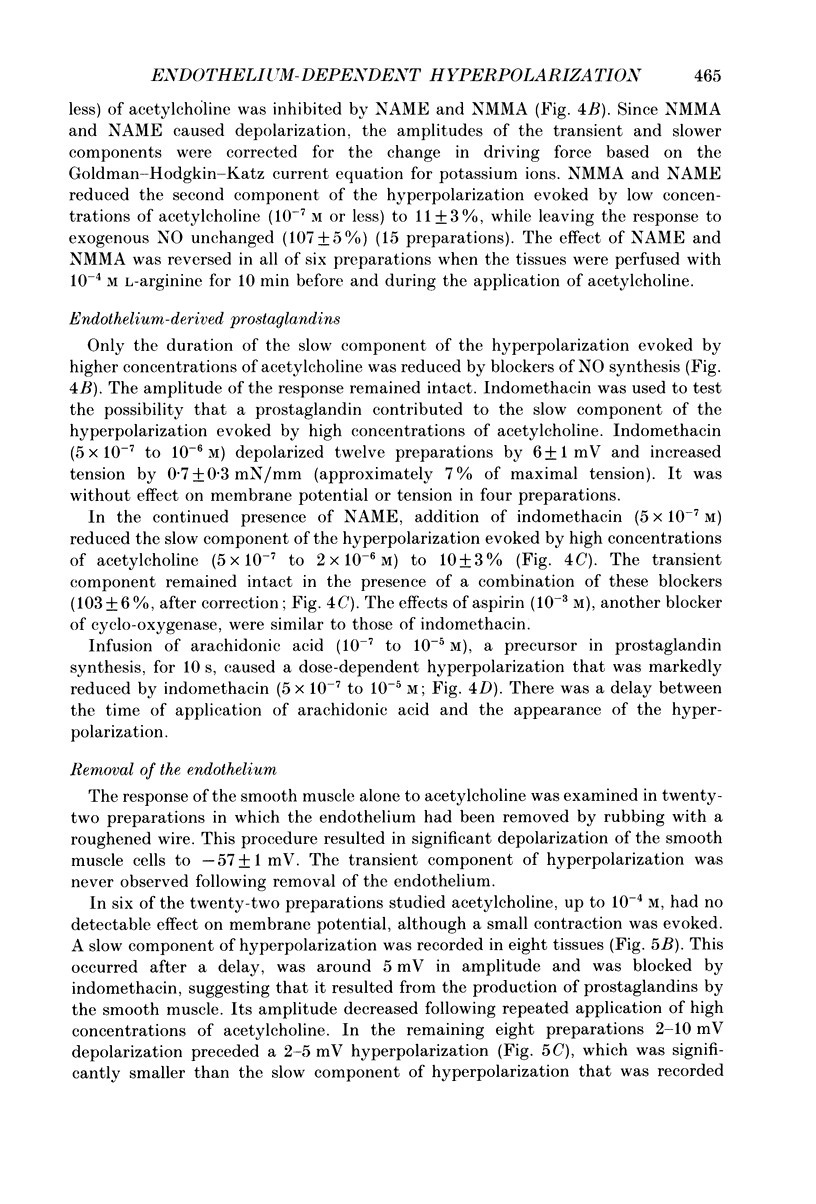
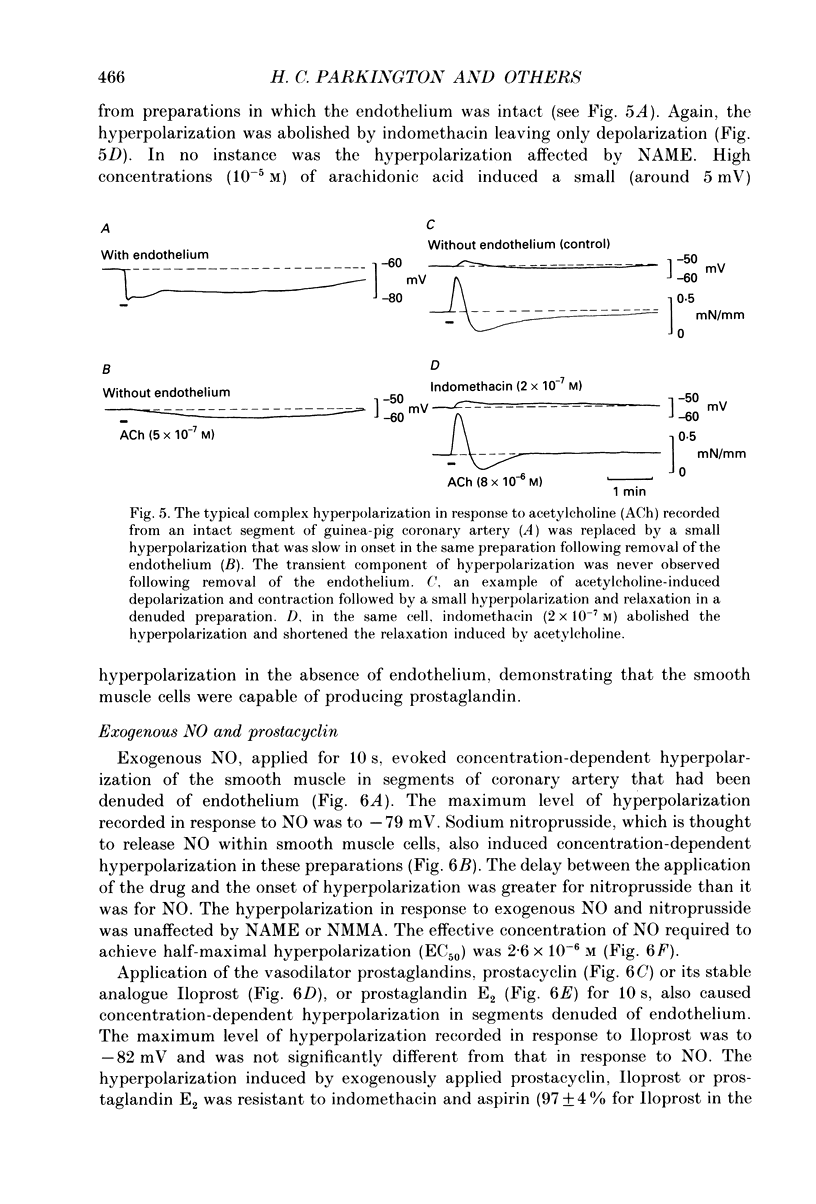
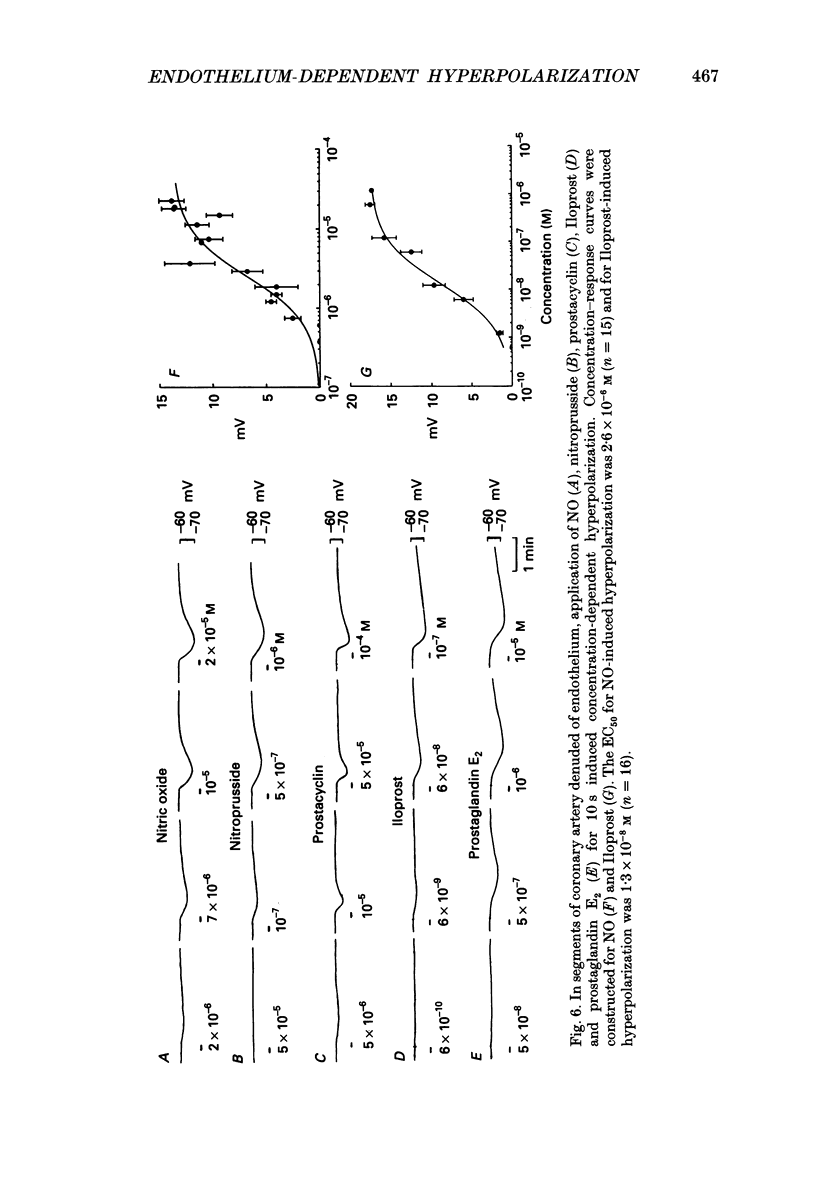
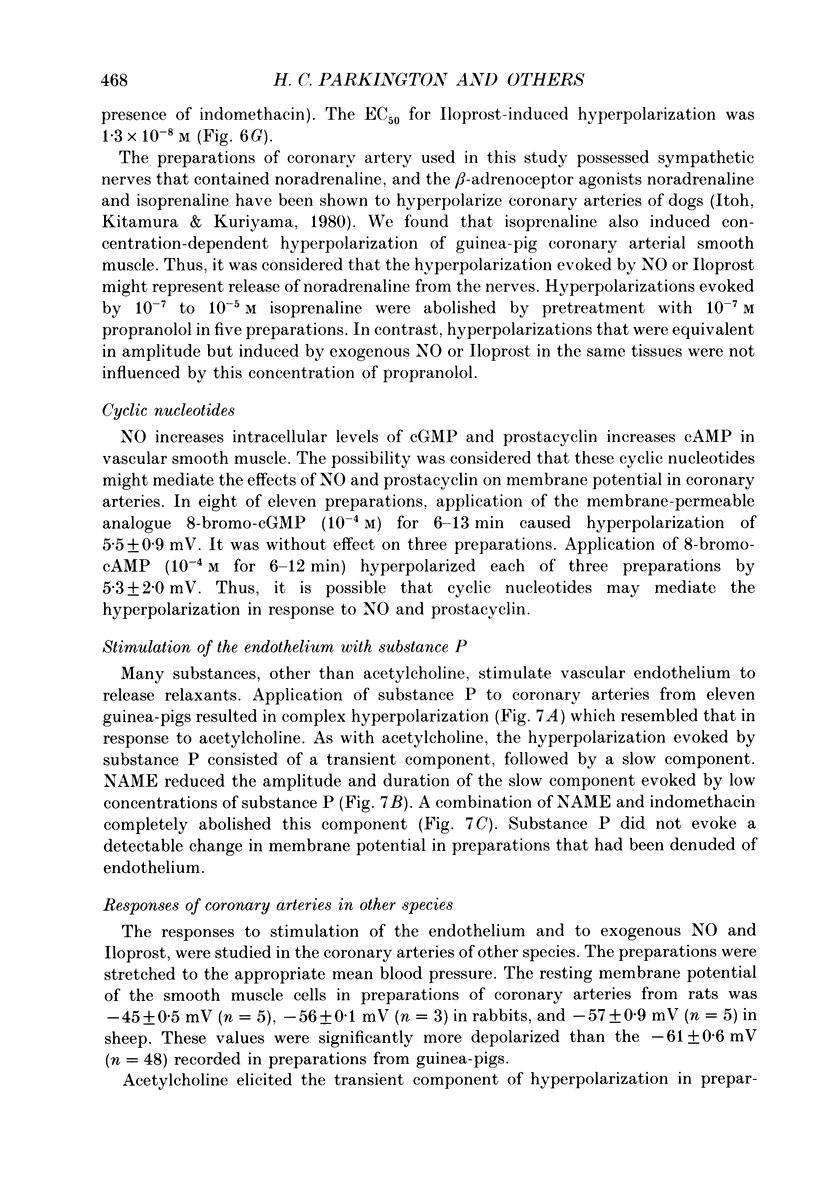
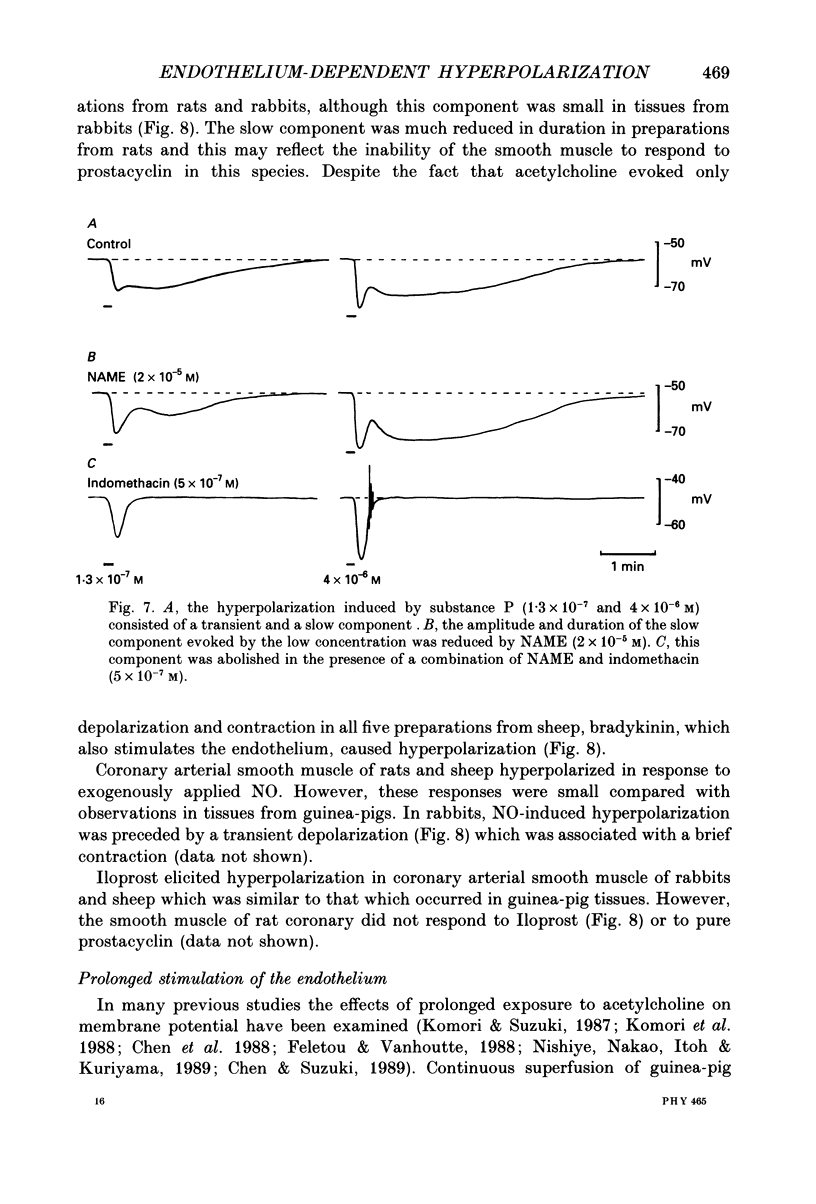
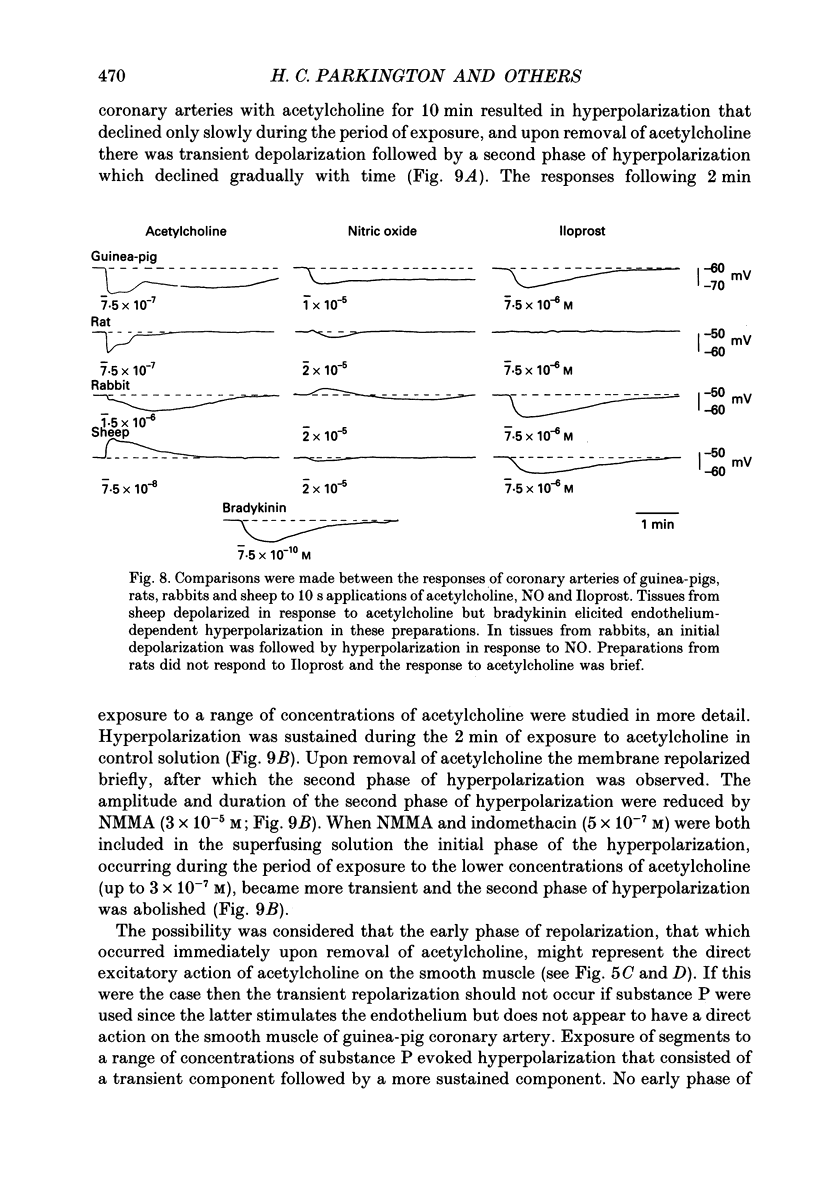
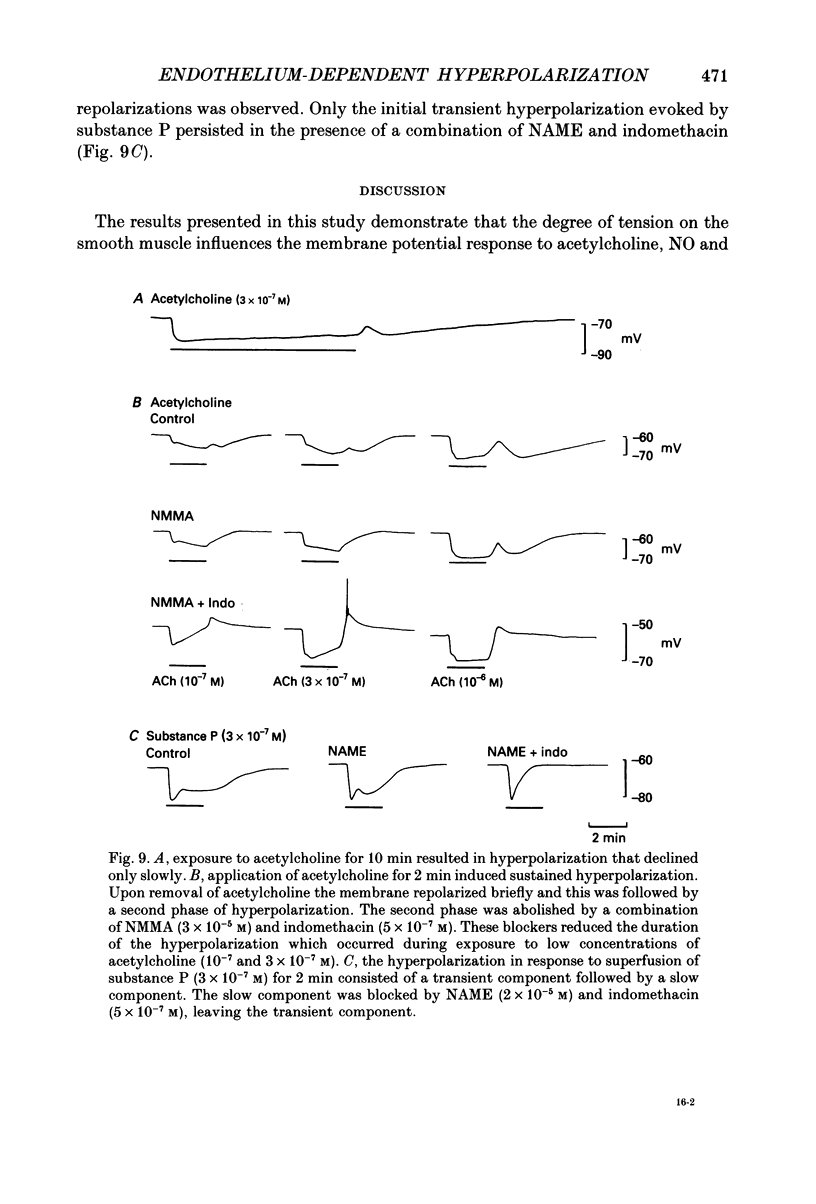
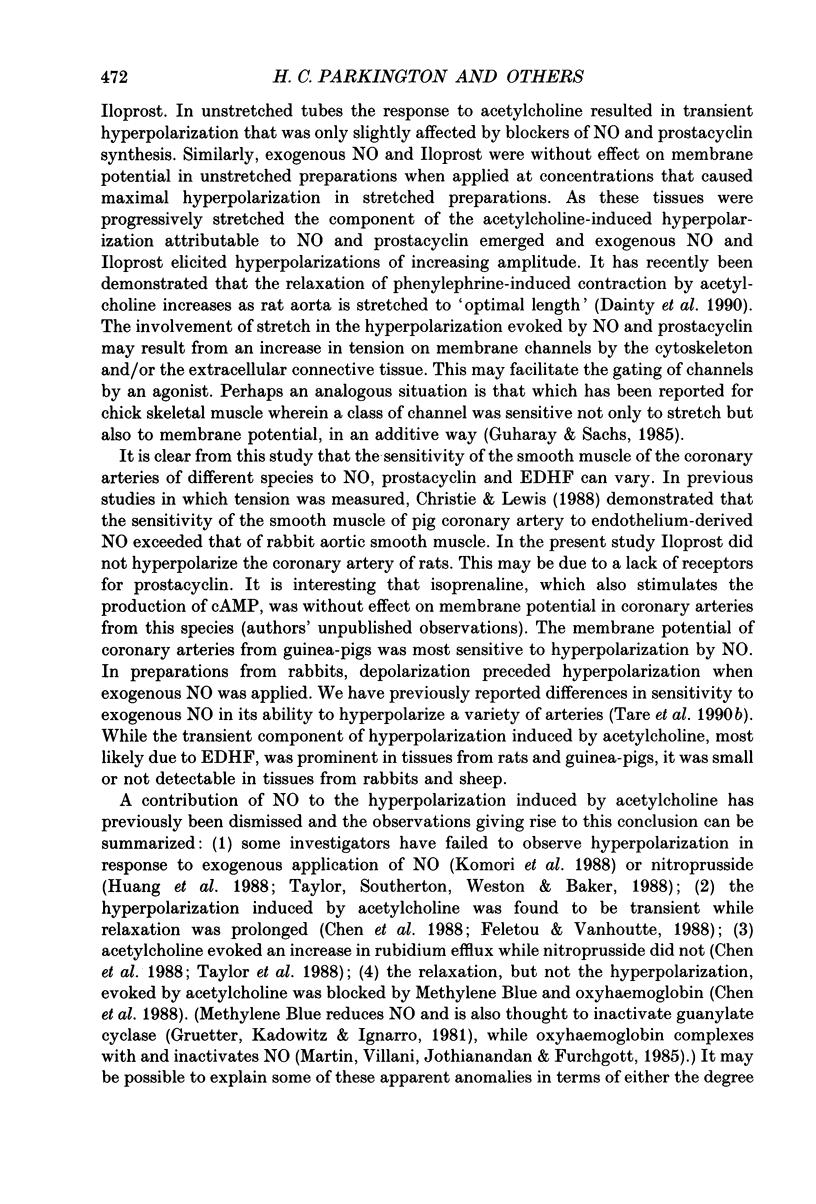
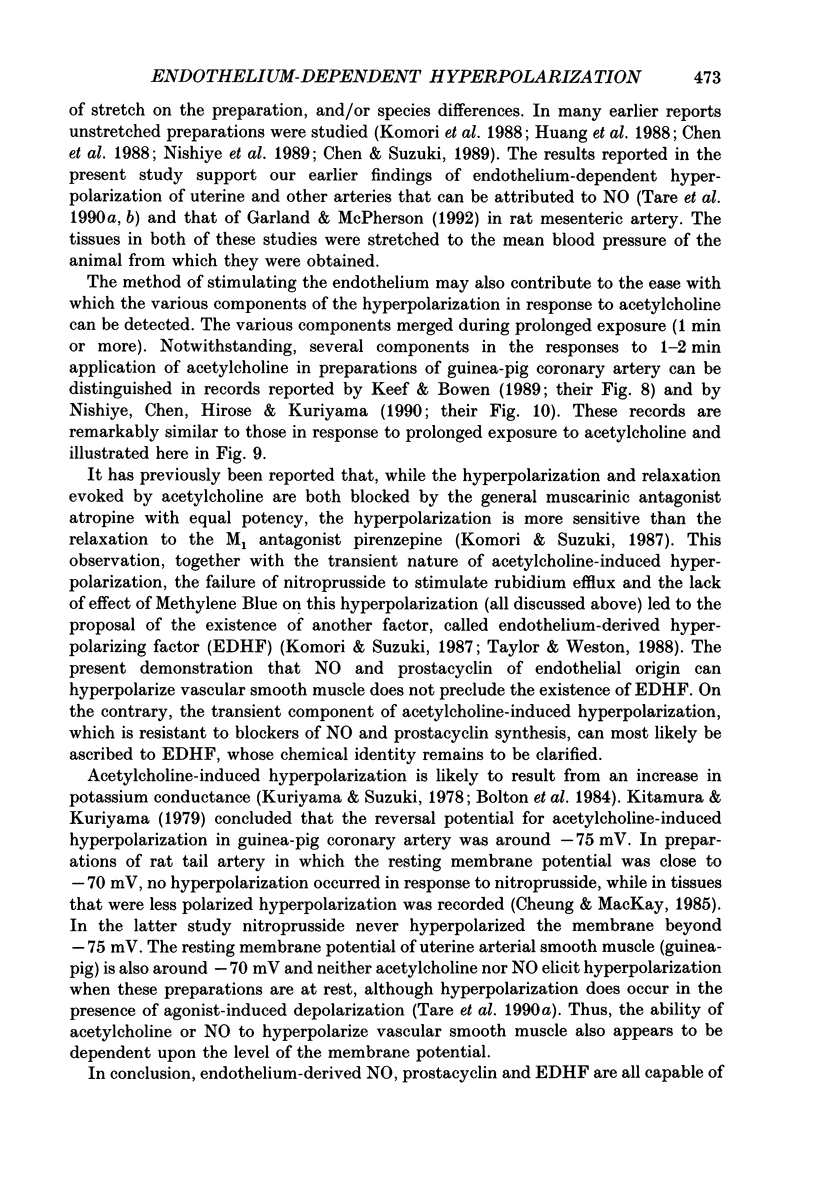
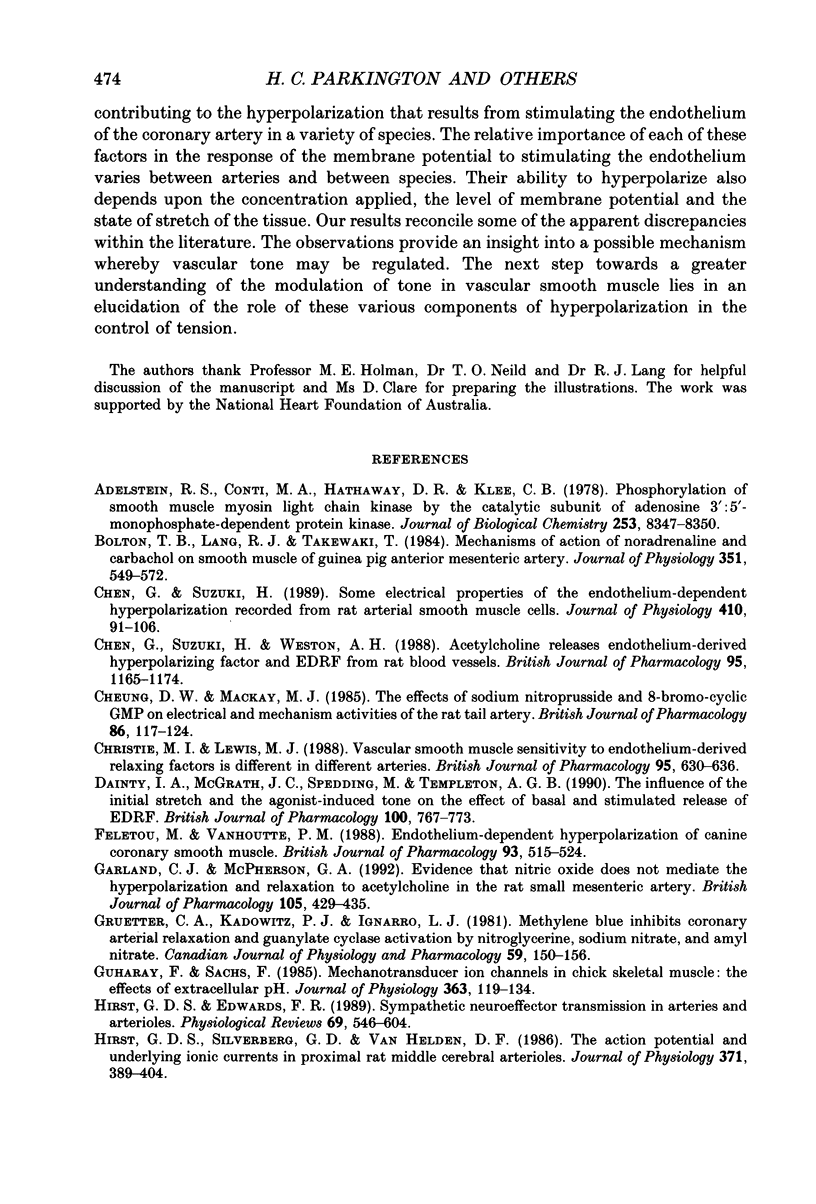
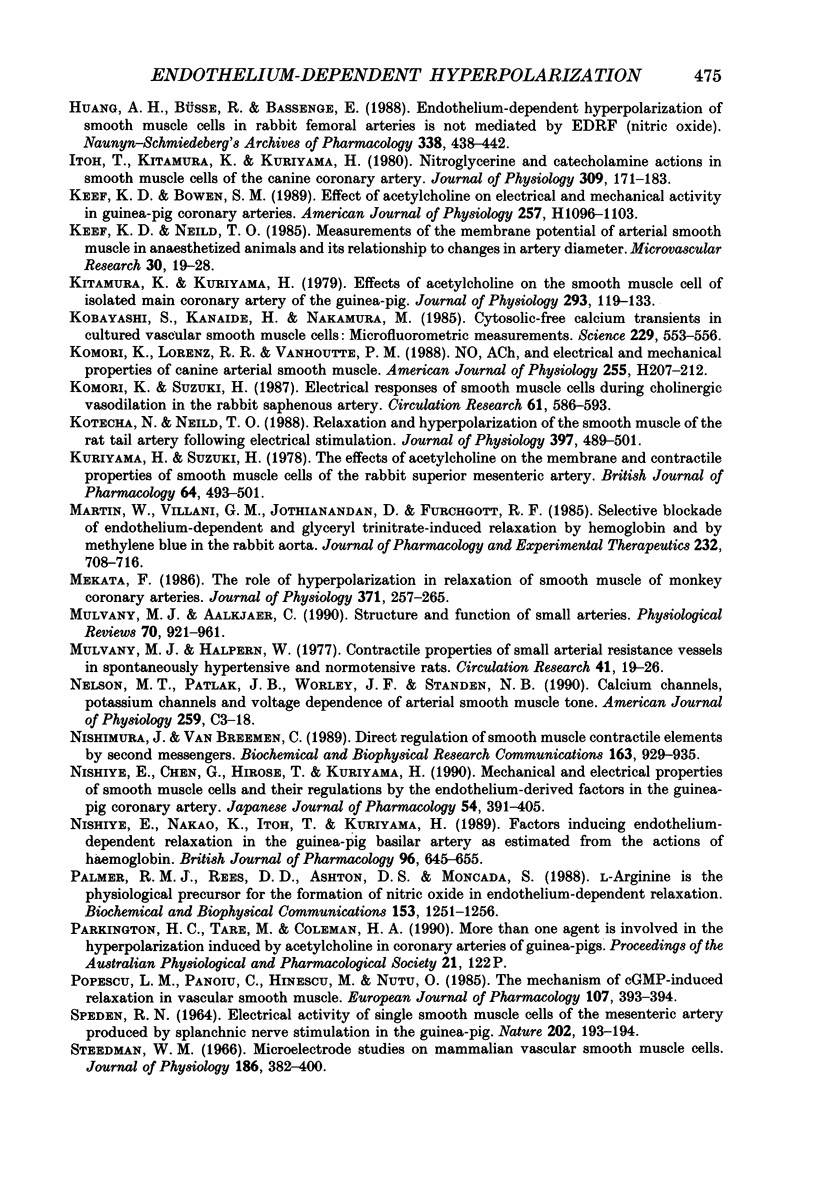
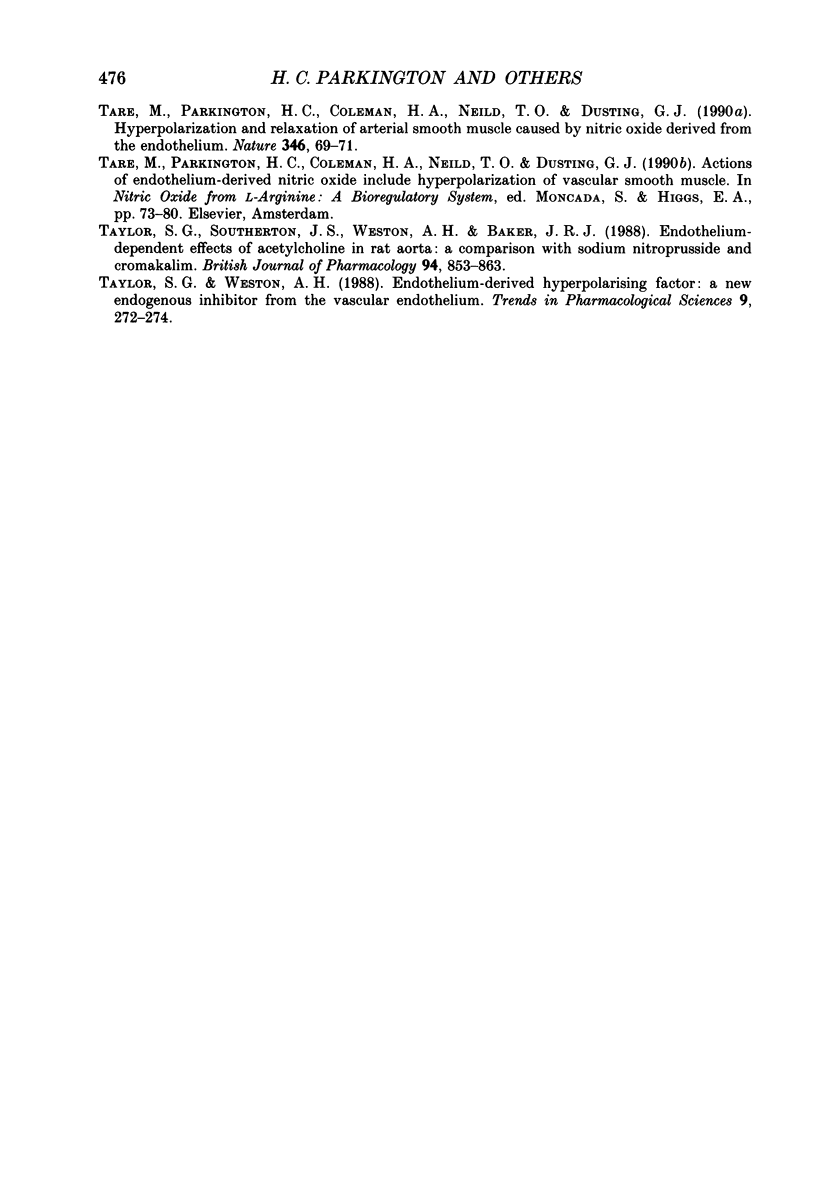
Selected References
These references are in PubMed. This may not be the complete list of references from this article.
- Adelstein R. S., Conti M. A., Hathaway D. R., Klee C. B. Phosphorylation of smooth muscle myosin light chain kinase by the catalytic subunit of adenosine 3': 5'-monophosphate-dependent protein kinase. J Biol Chem. 1978 Dec 10;253(23):8347–8350. [PubMed] [Google Scholar]
- Bolton T. B., Lang R. J., Takewaki T. Mechanisms of action of noradrenaline and carbachol on smooth muscle of guinea-pig anterior mesenteric artery. J Physiol. 1984 Jun;351:549–572. doi: 10.1113/jphysiol.1984.sp015262. [DOI] [PMC free article] [PubMed] [Google Scholar]
- Chen G., Suzuki H. Some electrical properties of the endothelium-dependent hyperpolarization recorded from rat arterial smooth muscle cells. J Physiol. 1989 Mar;410:91–106. doi: 10.1113/jphysiol.1989.sp017522. [DOI] [PMC free article] [PubMed] [Google Scholar]
- Chen G., Suzuki H., Weston A. H. Acetylcholine releases endothelium-derived hyperpolarizing factor and EDRF from rat blood vessels. Br J Pharmacol. 1988 Dec;95(4):1165–1174. doi: 10.1111/j.1476-5381.1988.tb11752.x. [DOI] [PMC free article] [PubMed] [Google Scholar]
- Cheung D. W., MacKay M. J. The effects of sodium nitroprusside and 8-bromo-cyclic GMP on electrical and mechanical activities of the rat tail artery. Br J Pharmacol. 1985 Sep;86(1):117–124. doi: 10.1111/j.1476-5381.1985.tb09441.x. [DOI] [PMC free article] [PubMed] [Google Scholar]
- Christie M. I., Lewis M. J. Vascular smooth muscle sensitivity to endothelium-derived relaxing factor is different in different arteries. Br J Pharmacol. 1988 Oct;95(2):630–636. doi: 10.1111/j.1476-5381.1988.tb11685.x. [DOI] [PMC free article] [PubMed] [Google Scholar]
- Dainty I. A., McGrath J. C., Spedding M., Templeton A. G. The influence of the initial stretch and the agonist-induced tone on the effect of basal and stimulated release of EDRF. Br J Pharmacol. 1990 Aug;100(4):767–773. doi: 10.1111/j.1476-5381.1990.tb14090.x. [DOI] [PMC free article] [PubMed] [Google Scholar]
- Feletou M., Vanhoutte P. M. Endothelium-dependent hyperpolarization of canine coronary smooth muscle. Br J Pharmacol. 1988 Mar;93(3):515–524. doi: 10.1111/j.1476-5381.1988.tb10306.x. [DOI] [PMC free article] [PubMed] [Google Scholar]
- Garland C. J., McPherson G. A. Evidence that nitric oxide does not mediate the hyperpolarization and relaxation to acetylcholine in the rat small mesenteric artery. Br J Pharmacol. 1992 Feb;105(2):429–435. doi: 10.1111/j.1476-5381.1992.tb14270.x. [DOI] [PMC free article] [PubMed] [Google Scholar]
- Gruetter C. A., Kadowitz P. J., Ignarro L. J. Methylene blue inhibits coronary arterial relaxation and guanylate cyclase activation by nitroglycerin, sodium nitrite, and amyl nitrite. Can J Physiol Pharmacol. 1981 Feb;59(2):150–156. doi: 10.1139/y81-025. [DOI] [PubMed] [Google Scholar]
- Guharay F., Sachs F. Mechanotransducer ion channels in chick skeletal muscle: the effects of extracellular pH. J Physiol. 1985 Jun;363:119–134. doi: 10.1113/jphysiol.1985.sp015699. [DOI] [PMC free article] [PubMed] [Google Scholar]
- Hirst G. D., Edwards F. R. Sympathetic neuroeffector transmission in arteries and arterioles. Physiol Rev. 1989 Apr;69(2):546–604. doi: 10.1152/physrev.1989.69.2.546. [DOI] [PubMed] [Google Scholar]
- Huang A. H., Busse R., Bassenge E. Endothelium-dependent hyperpolarization of smooth muscle cells in rabbit femoral arteries is not mediated by EDRF (nitric oxide). Naunyn Schmiedebergs Arch Pharmacol. 1988 Oct;338(4):438–442. doi: 10.1007/BF00172124. [DOI] [PubMed] [Google Scholar]
- Ito Y., Kitamura K., Kuriyama H. Nitroglycerine and catecholamine actions on smooth muscle cells of the canine coronary artery. J Physiol. 1980 Dec;309:171–183. doi: 10.1113/jphysiol.1980.sp013502. [DOI] [PMC free article] [PubMed] [Google Scholar]
- Keef K. D., Bowen S. M. Effect of ACh on electrical and mechanical activity in guinea pig coronary arteries. Am J Physiol. 1989 Oct;257(4 Pt 2):H1096–H1103. doi: 10.1152/ajpheart.1989.257.4.H1096. [DOI] [PubMed] [Google Scholar]
- Kitamura K., Kuriyama H. Effects of acetylcholine on the smooth muscle cell of isolated main coronary artery of the guinea-pig. J Physiol. 1979 Aug;293:119–133. doi: 10.1113/jphysiol.1979.sp012881. [DOI] [PMC free article] [PubMed] [Google Scholar]
- Kobayashi S., Kanaide H., Nakamura M. Cytosolic-free calcium transients in cultured vascular smooth muscle cells: microfluorometric measurements. Science. 1985 Aug 9;229(4713):553–556. doi: 10.1126/science.3927484. [DOI] [PubMed] [Google Scholar]
- Komori K., Lorenz R. R., Vanhoutte P. M. Nitric oxide, ACh, and electrical and mechanical properties of canine arterial smooth muscle. Am J Physiol. 1988 Jul;255(1 Pt 2):H207–H212. doi: 10.1152/ajpheart.1988.255.1.H207. [DOI] [PubMed] [Google Scholar]
- Komori K., Suzuki H. Electrical responses of smooth muscle cells during cholinergic vasodilation in the rabbit saphenous artery. Circ Res. 1987 Oct;61(4):586–593. doi: 10.1161/01.res.61.4.586. [DOI] [PubMed] [Google Scholar]
- Kotecha N., Neild T. O. Relaxation and hyperpolarization of the smooth muscle of the rat tail artery following electrical stimulation. J Physiol. 1988 Mar;397:489–501. doi: 10.1113/jphysiol.1988.sp017014. [DOI] [PMC free article] [PubMed] [Google Scholar]
- Kuriyama H., Suzuki H. The effects of acetylcholine on the membrane and contractile properties of smooth muscle cells of the rabbit superior mesenteric artery. Br J Pharmacol. 1978 Dec;64(4):493–501. doi: 10.1111/j.1476-5381.1978.tb17310.x. [DOI] [PMC free article] [PubMed] [Google Scholar]
- Martin W., Villani G. M., Jothianandan D., Furchgott R. F. Selective blockade of endothelium-dependent and glyceryl trinitrate-induced relaxation by hemoglobin and by methylene blue in the rabbit aorta. J Pharmacol Exp Ther. 1985 Mar;232(3):708–716. [PubMed] [Google Scholar]
- Mekata F. The role of hyperpolarization in the relaxation of smooth muscle of monkey coronary artery. J Physiol. 1986 Feb;371:257–265. doi: 10.1113/jphysiol.1986.sp015972. [DOI] [PMC free article] [PubMed] [Google Scholar]
- Mulvany M. J., Aalkjaer C. Structure and function of small arteries. Physiol Rev. 1990 Oct;70(4):921–961. doi: 10.1152/physrev.1990.70.4.921. [DOI] [PubMed] [Google Scholar]
- Mulvany M. J., Halpern W. Contractile properties of small arterial resistance vessels in spontaneously hypertensive and normotensive rats. Circ Res. 1977 Jul;41(1):19–26. doi: 10.1161/01.res.41.1.19. [DOI] [PubMed] [Google Scholar]
- Neild T. O., Keef K. Measurements of the membrane potential of arterial smooth muscle in anesthetized animals and its relationship to changes in artery diameter. Microvasc Res. 1985 Jul;30(1):19–28. doi: 10.1016/0026-2862(85)90034-2. [DOI] [PubMed] [Google Scholar]
- Nelson M. T., Patlak J. B., Worley J. F., Standen N. B. Calcium channels, potassium channels, and voltage dependence of arterial smooth muscle tone. Am J Physiol. 1990 Jul;259(1 Pt 1):C3–18. doi: 10.1152/ajpcell.1990.259.1.C3. [DOI] [PubMed] [Google Scholar]
- Nishimura J., van Breemen C. Direct regulation of smooth muscle contractile elements by second messengers. Biochem Biophys Res Commun. 1989 Sep 15;163(2):929–935. doi: 10.1016/0006-291x(89)92311-5. [DOI] [PubMed] [Google Scholar]
- Nishiye E., Chen G. F., Hirose T., Kuriyama H. Mechanical and electrical properties of smooth muscle cells and their regulations by endothelium-derived factors in the guinea pig coronary artery. Jpn J Pharmacol. 1990 Dec;54(4):391–405. doi: 10.1254/jjp.54.391. [DOI] [PubMed] [Google Scholar]
- Nishiye E., Nakao K., Itoh T., Kuriyama H. Factors inducing endothelium-dependent relaxation in the guinea-pig basilar artery as estimated from the actions of haemoglobin. Br J Pharmacol. 1989 Mar;96(3):645–655. doi: 10.1111/j.1476-5381.1989.tb11864.x. [DOI] [PMC free article] [PubMed] [Google Scholar]
- Palmer R. M., Rees D. D., Ashton D. S., Moncada S. L-arginine is the physiological precursor for the formation of nitric oxide in endothelium-dependent relaxation. Biochem Biophys Res Commun. 1988 Jun 30;153(3):1251–1256. doi: 10.1016/s0006-291x(88)81362-7. [DOI] [PubMed] [Google Scholar]
- Popescu L. M., Panoiu C., Hinescu M., Nutu O. The mechanism of cGMP-induced relaxation in vascular smooth muscle. Eur J Pharmacol. 1985 Jan 8;107(3):393–394. doi: 10.1016/0014-2999(85)90269-9. [DOI] [PubMed] [Google Scholar]
- SPEDEN R. N. ELECTRICAL ACTIVITY OF SINGLE SMOOTH MUSCLE CELLS OF THE MESENTERIC ARTERY PRODUCED BY SPLANCHNIC NERVE STIMULATION IN THE GUINEA PIG. Nature. 1964 Apr 11;202:193–194. doi: 10.1038/202193a0. [DOI] [PubMed] [Google Scholar]
- Steedman W. M. Micro-electrode studies on mammalian vascular muscle. J Physiol. 1966 Oct;186(2):382–400. doi: 10.1113/jphysiol.1966.sp008041. [DOI] [PMC free article] [PubMed] [Google Scholar]
- Tare M., Parkington H. C., Coleman H. A., Neild T. O., Dusting G. J. Hyperpolarization and relaxation of arterial smooth muscle caused by nitric oxide derived from the endothelium. Nature. 1990 Jul 5;346(6279):69–71. doi: 10.1038/346069a0. [DOI] [PubMed] [Google Scholar]
- Taylor S. G., Southerton J. S., Weston A. H., Baker J. R. Endothelium-dependent effects of acetylcholine in rat aorta: a comparison with sodium nitroprusside and cromakalim. Br J Pharmacol. 1988 Jul;94(3):853–863. doi: 10.1111/j.1476-5381.1988.tb11597.x. [DOI] [PMC free article] [PubMed] [Google Scholar]
- Taylor S. G., Weston A. H. Endothelium-derived hyperpolarizing factor: a new endogenous inhibitor from the vascular endothelium. Trends Pharmacol Sci. 1988 Aug;9(8):272–274. doi: 10.1016/0165-6147(88)90003-x. [DOI] [PubMed] [Google Scholar]