Abstract
1. Single fibres isolated from the anterior tibialis muscle of Rana temporaria were allowed to shorten against a high load during a 2.5-4.0 s fused tetanus (1-3 degrees C) and the maximum force produced at the short length was compared with that recorded during a fixed-end tetanus at the same overall fibre length. Changes in length of marked, consecutive segments (ca 0.5 mm in length) along the fibre were measured throughout the tetanus using a photoelectric recording system. 2. Loaded shortening (load ca 3/4 of maximum tetanic force) starting from approximately 2.55 microns sarcomere length and ending near slack fibre length depressed the tetanic force by 13 +/- 2% (mean +/- S.E.M., n = 10) and caused a marked redistribution of sarcomere length along the fibre. Unloaded shortening over the same range caused no force deficit and did not lead to increased dispersion of sarcomere length. 3. Loaded shortening below slack length produced less force depression and less non-uniformity of sarcomere length than did a corresponding intervention above slack length. 4. The force deficit after loaded shortening, both above and below slack fibre length, was positively correlated (P < 0.005) to the coefficient of variation of the sarcomere length along the fibre. 5. The decrease in active force after loaded shortening, and its relation to increased dispersion of sarcomere length along the fibre, could be simulated closely by a computer model in which the muscle fibre was assumed to consist of eleven discrete segments acting in series with a passive elastic element. 6. Experiments were performed in which the length of an individual segment of the intact muscle fibre was strictly controlled throughout a tetanus. Loaded shortening of such a 'length-clamped' segment caused no force depression during the subsequent isometric phase either above or below slack fibre length. 7. The results suggest strongly that force depression after loaded shortening of a single muscle fibre is attributable to non-uniform sarcomere behaviour along the fibre. The experimental evidence supports the view that: (i) the myosin cross-bridges act as independent force generators; and (ii) their steady-state performance during a tetanus is unaffected by the preceding contractile activity.
Full text
PDF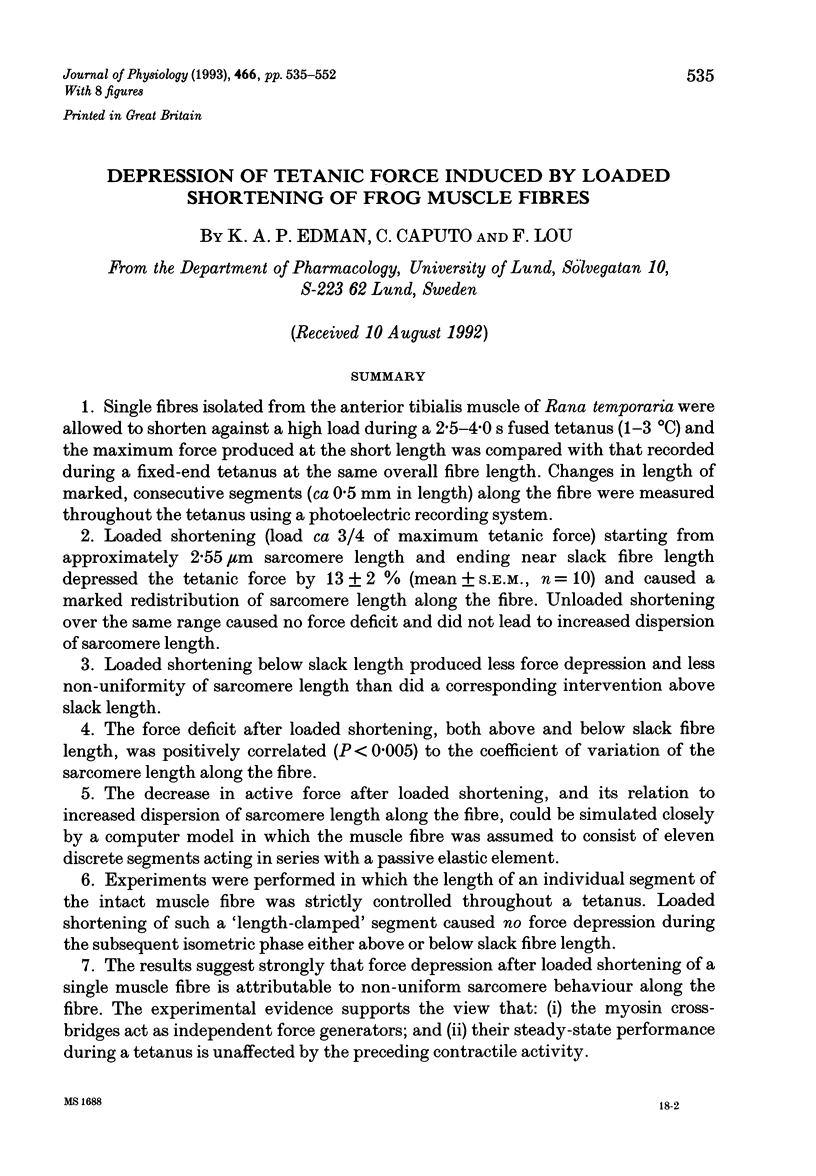
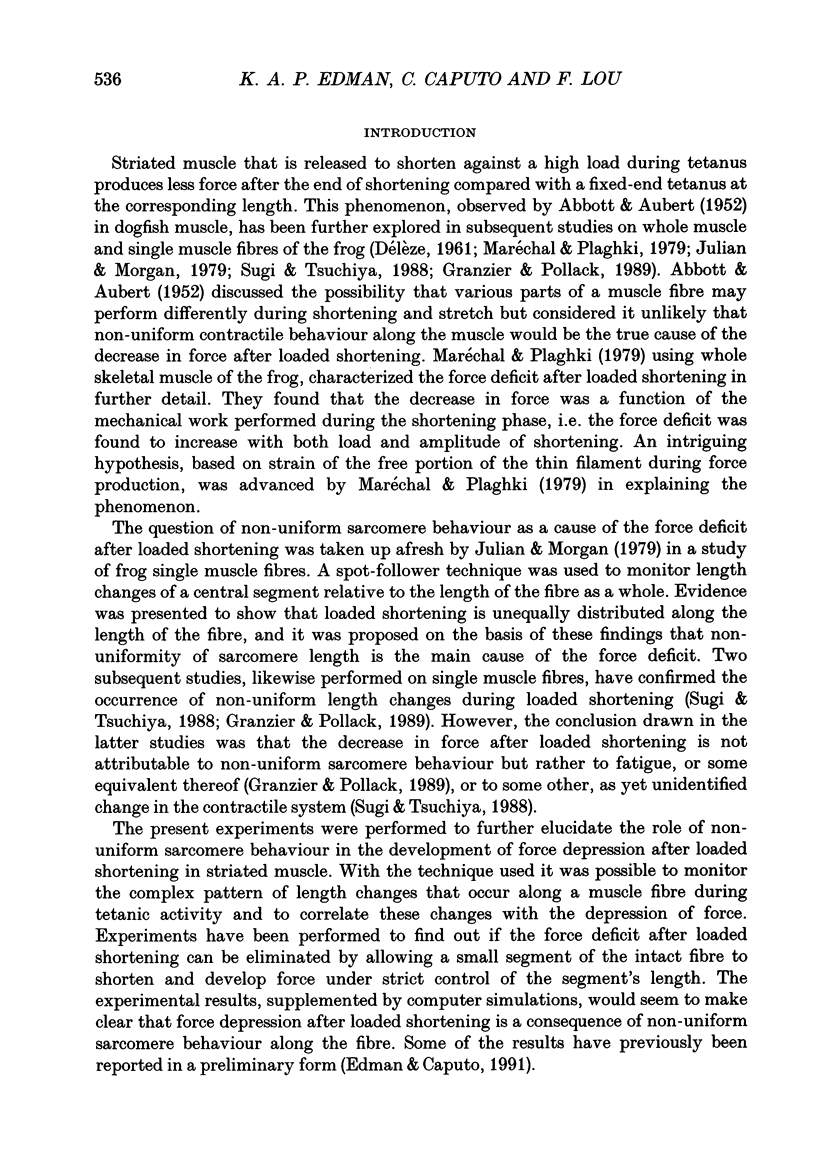
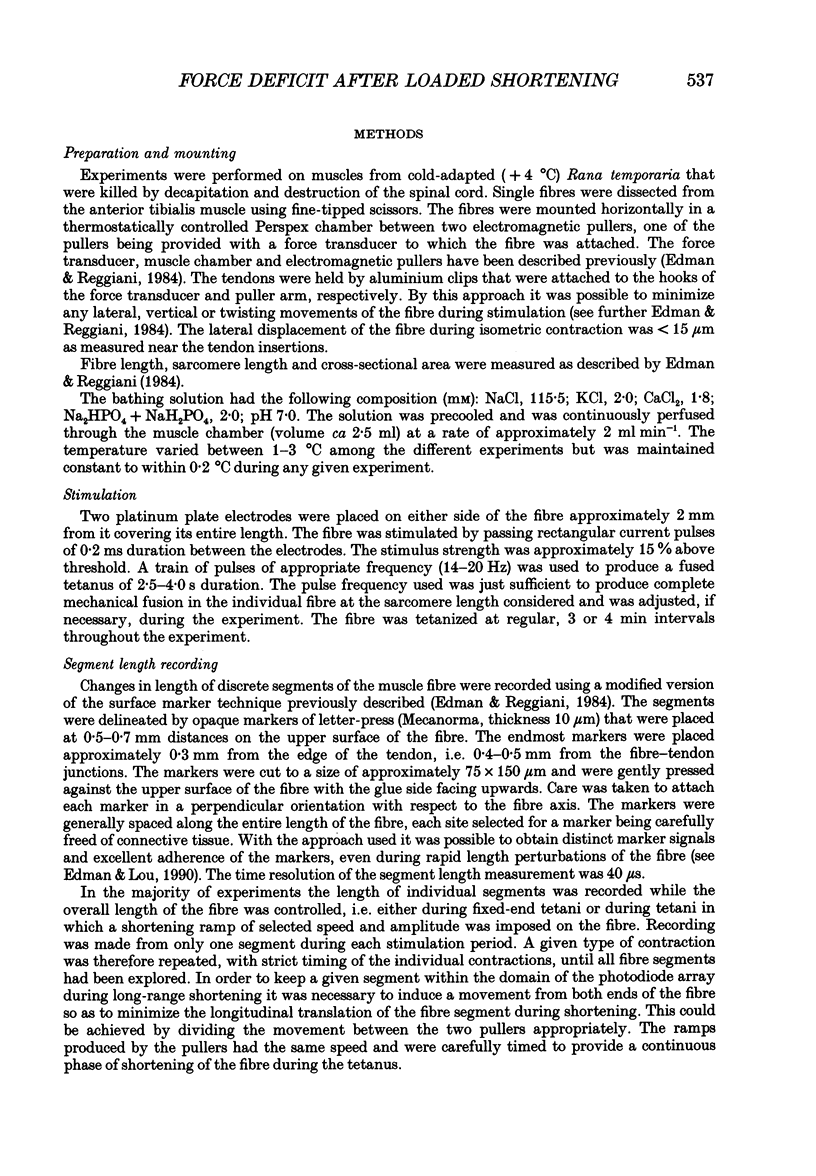
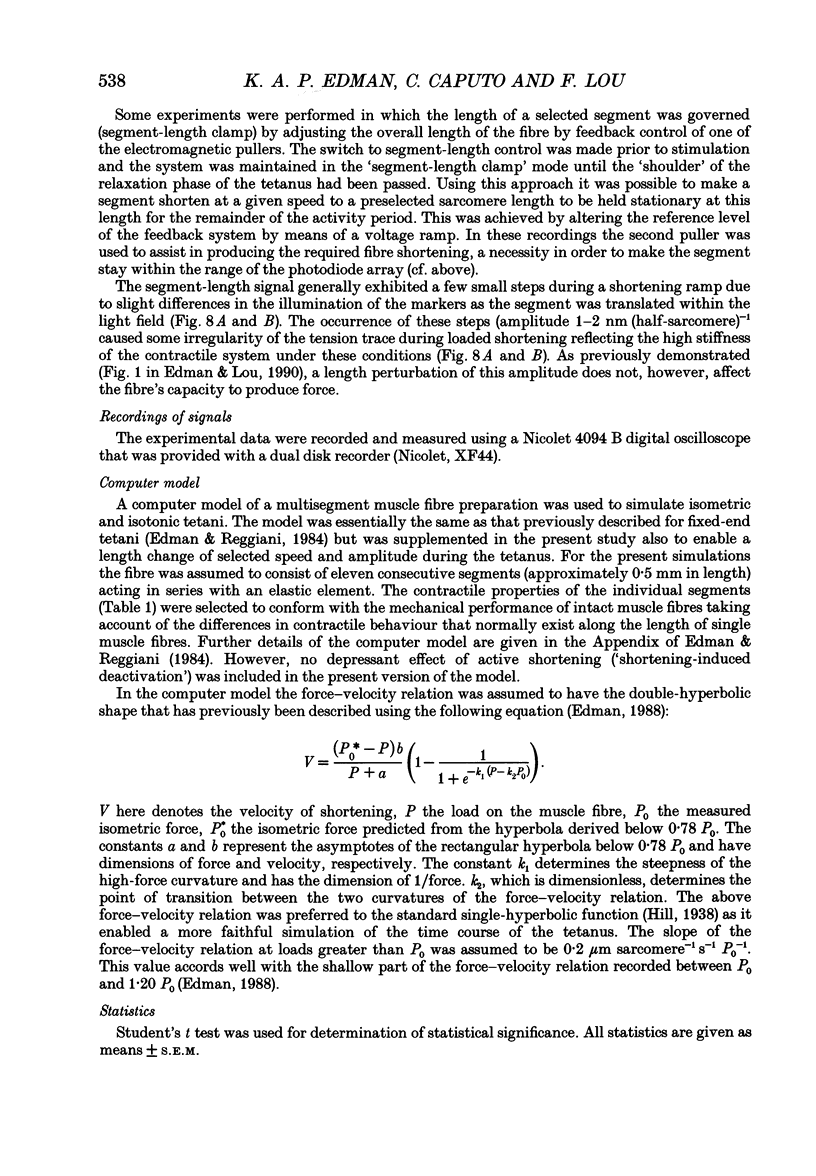
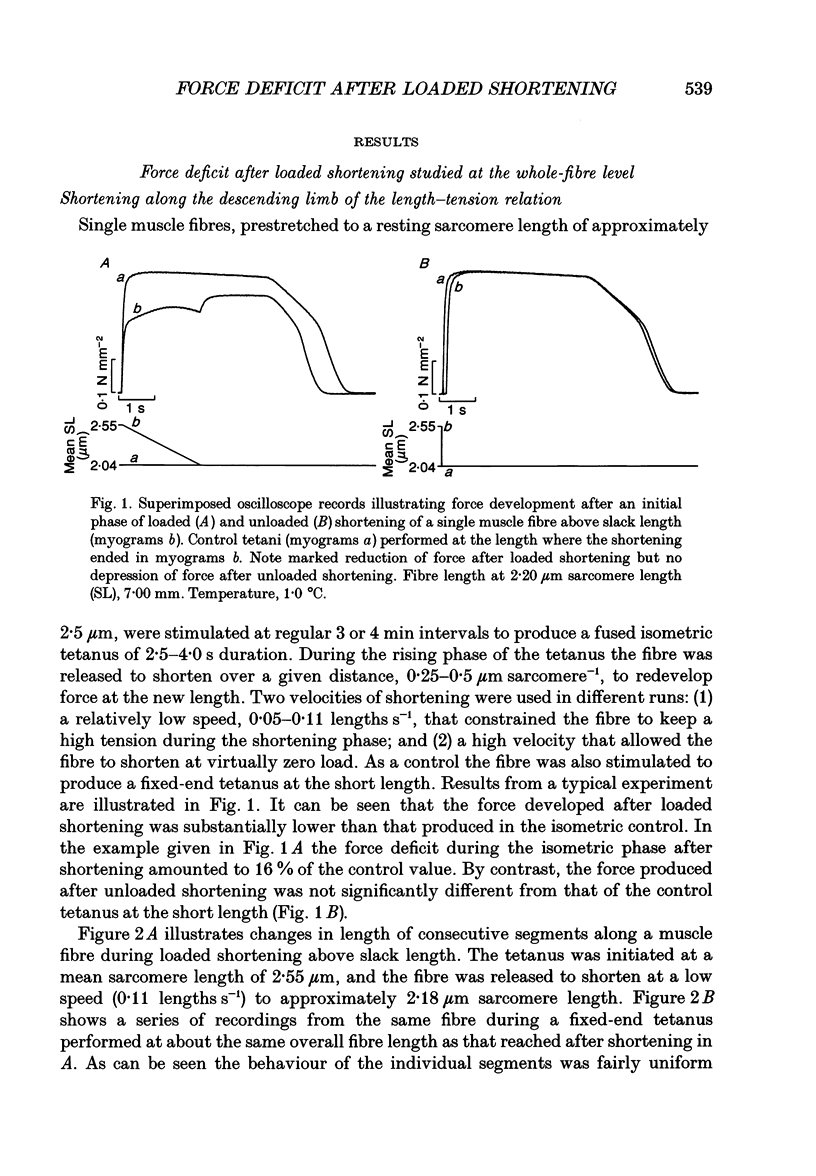
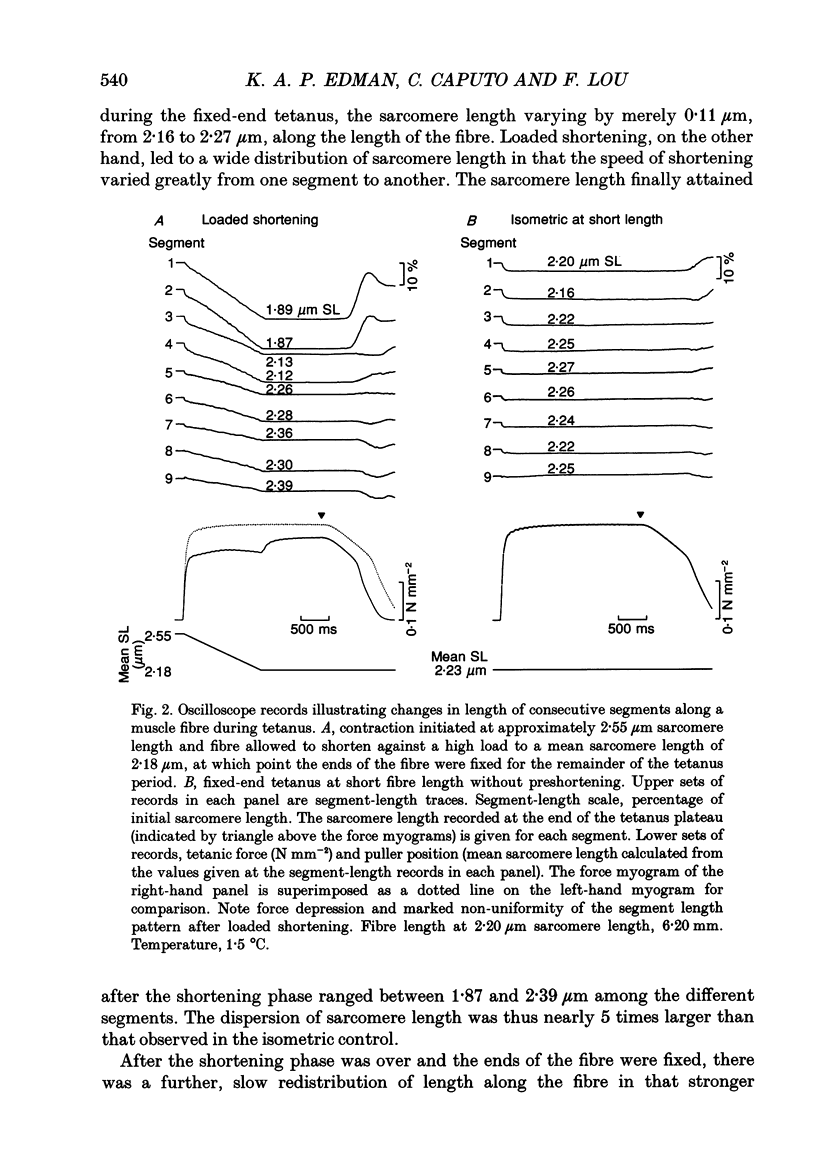
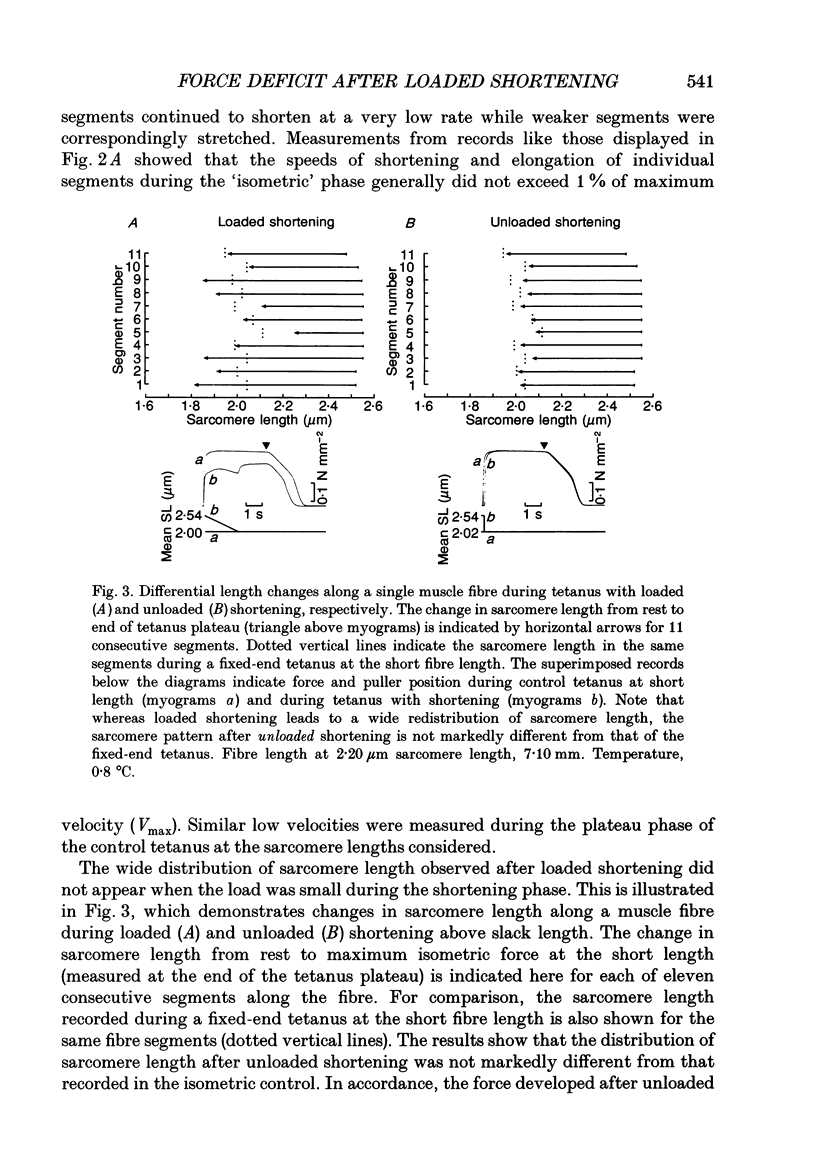
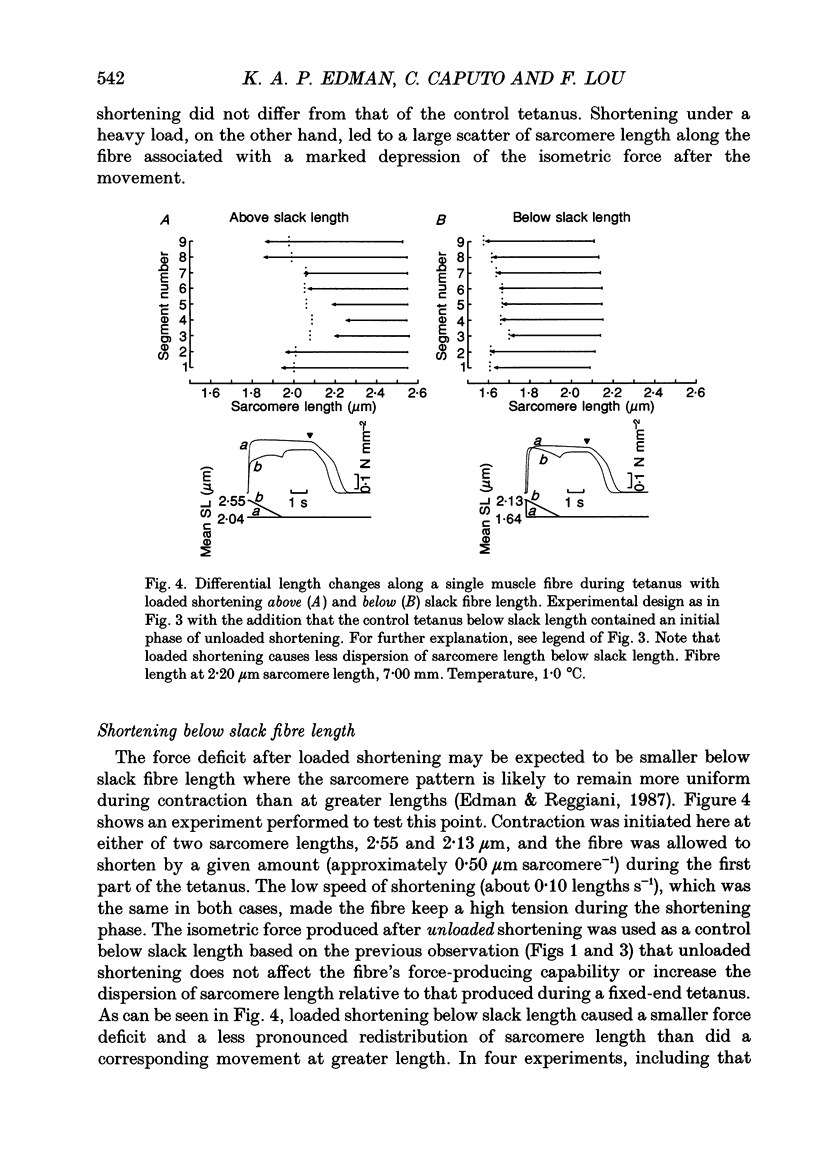
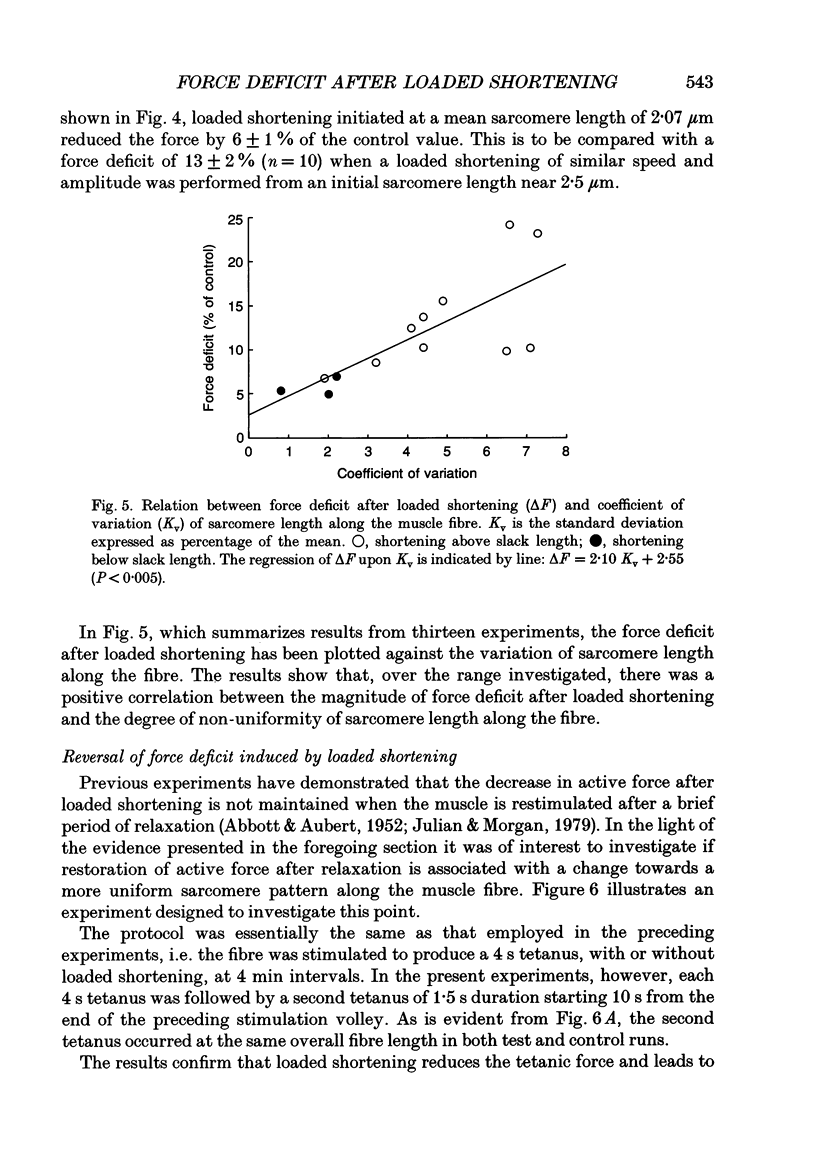
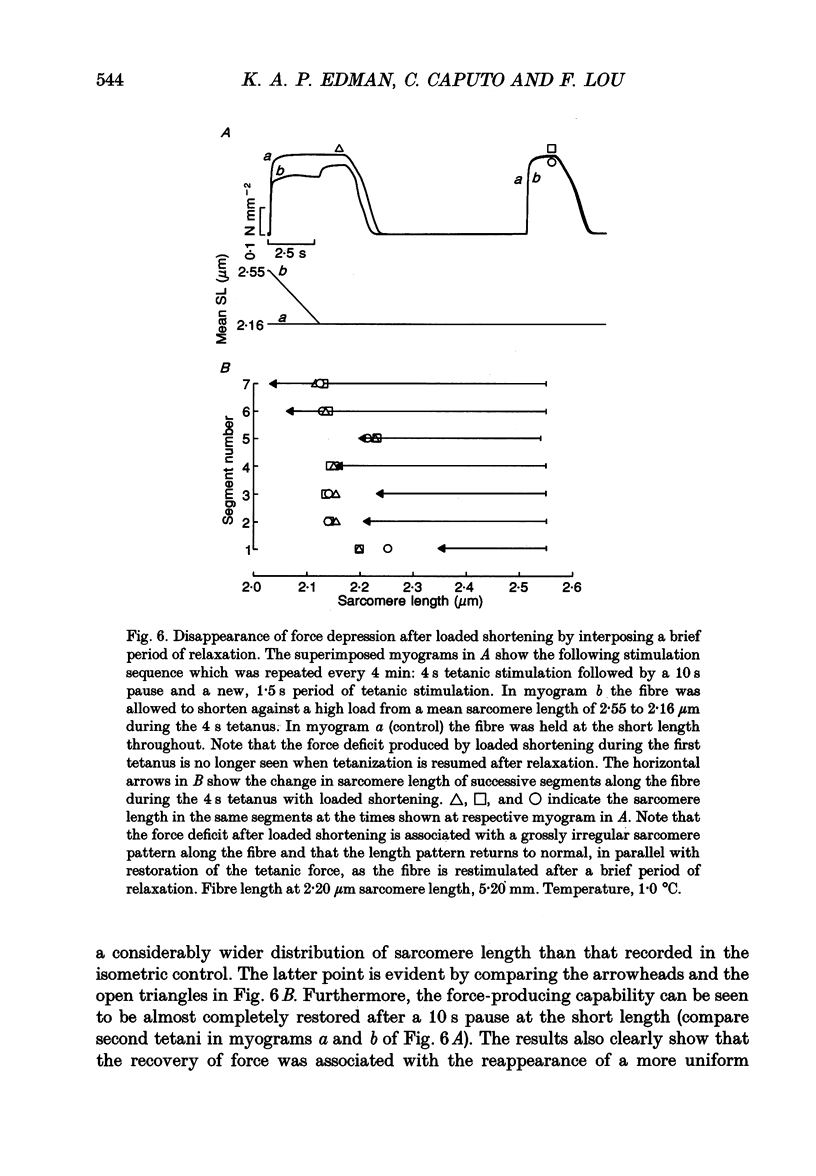
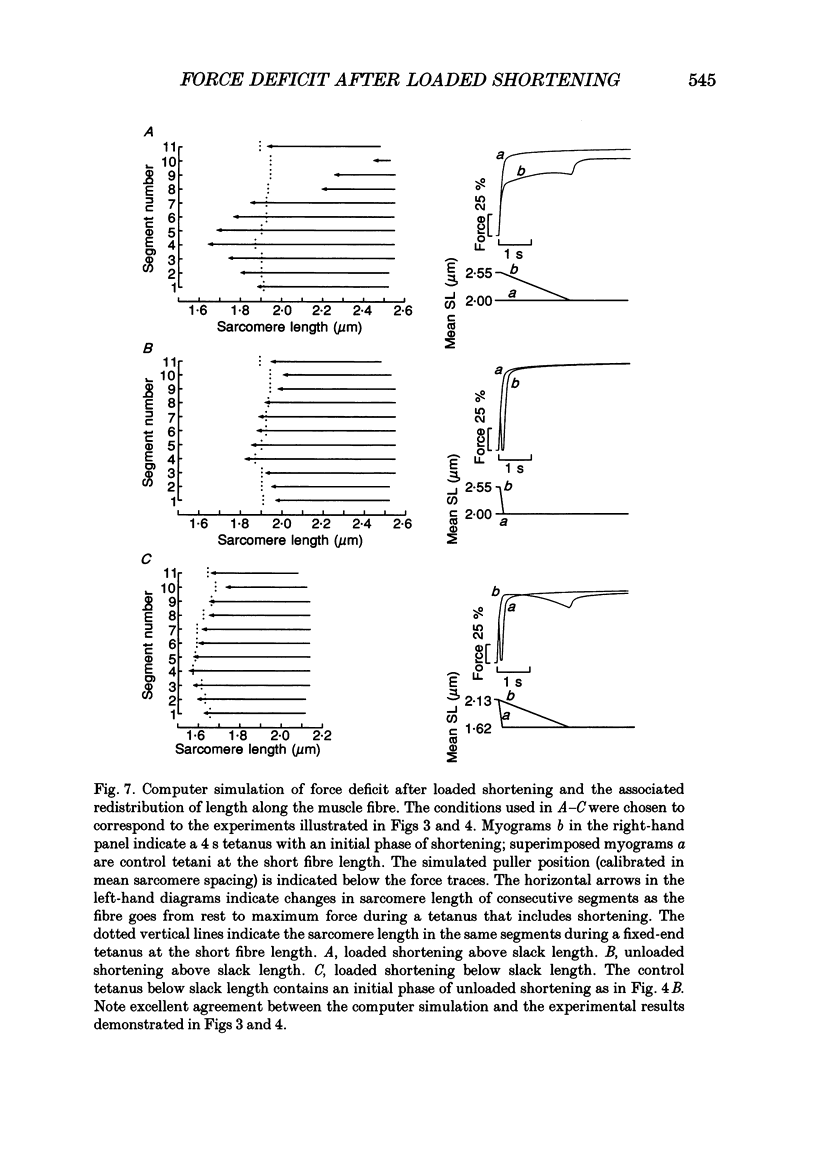
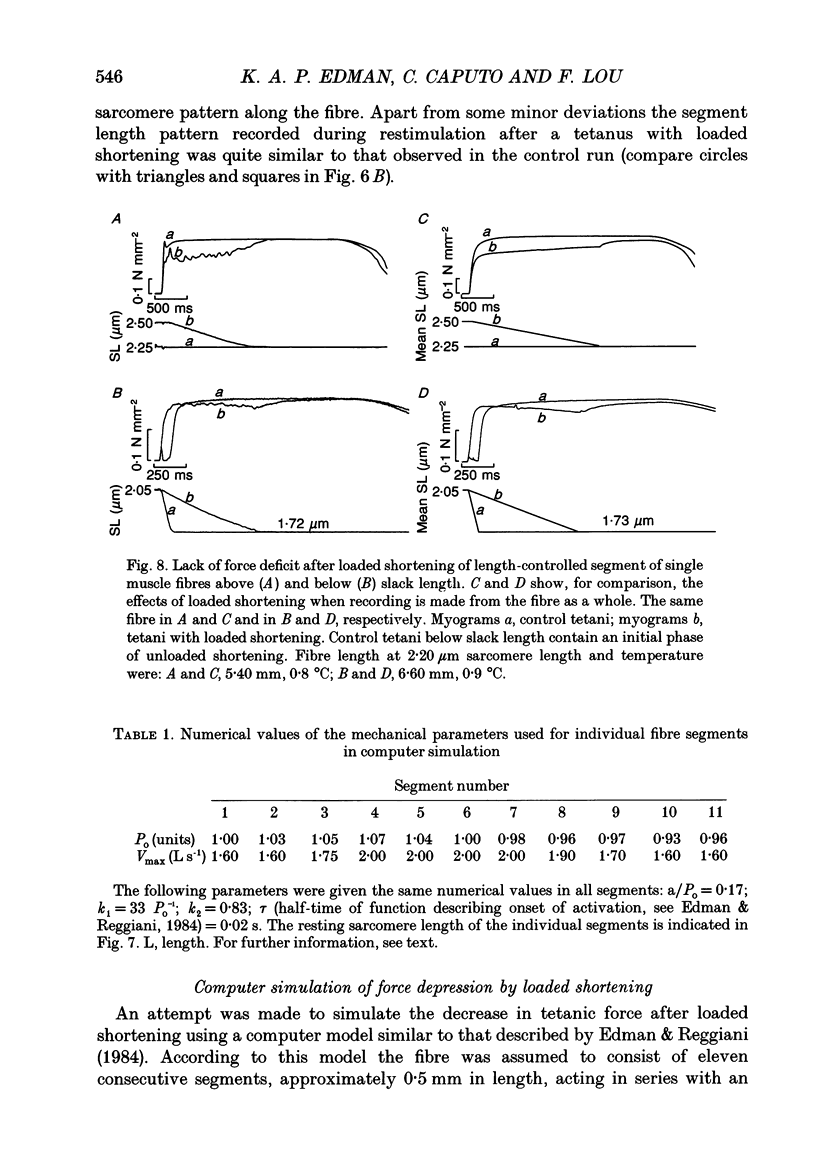
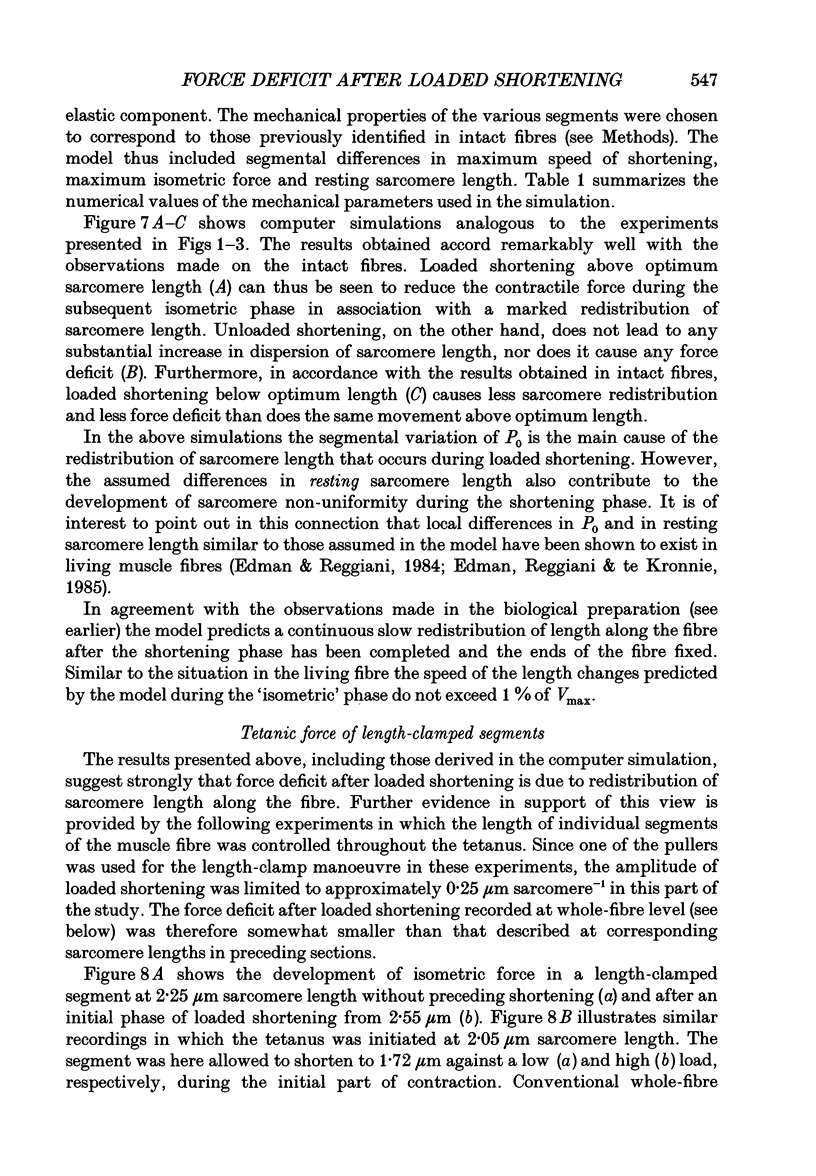
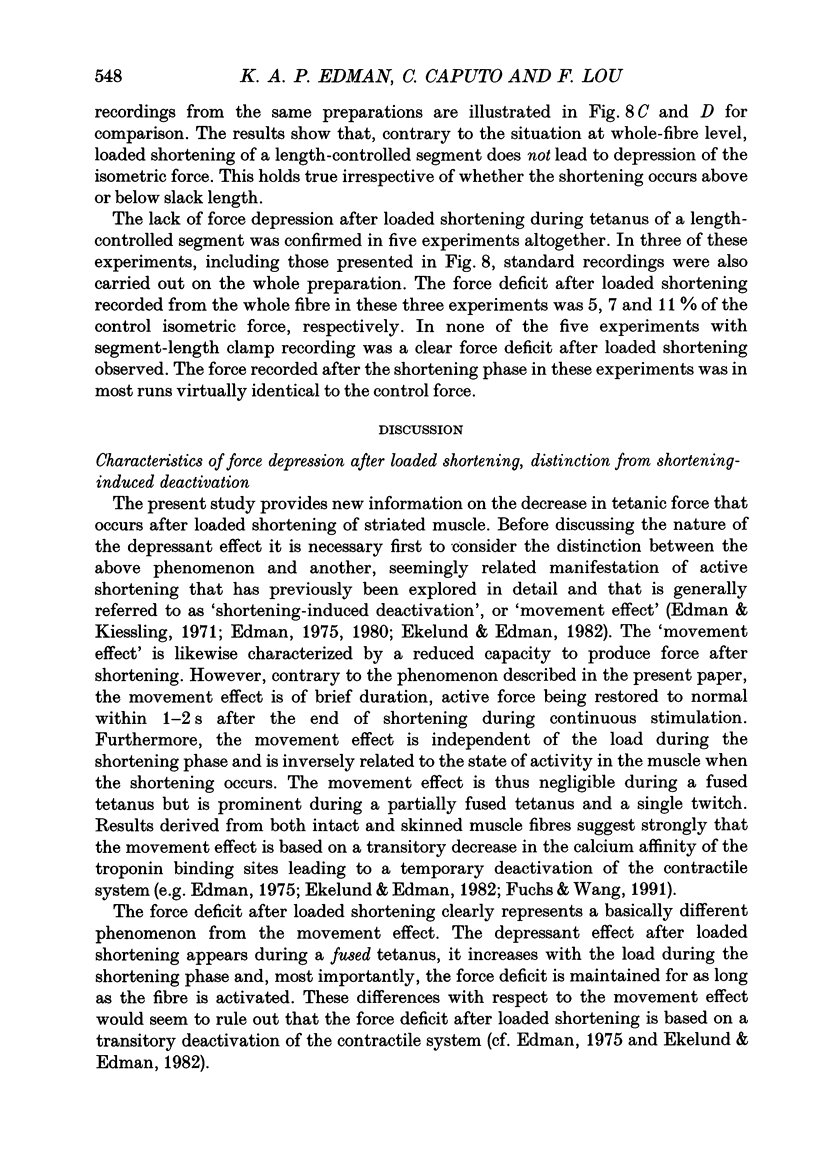
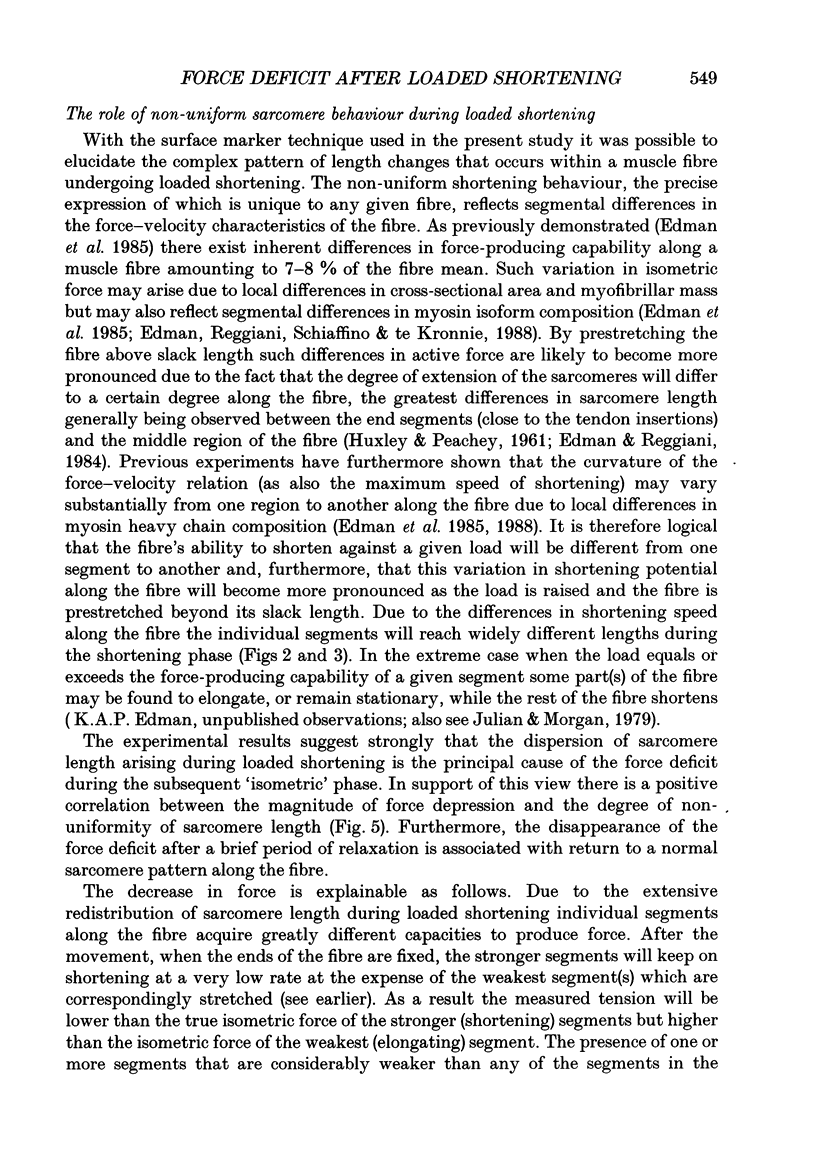
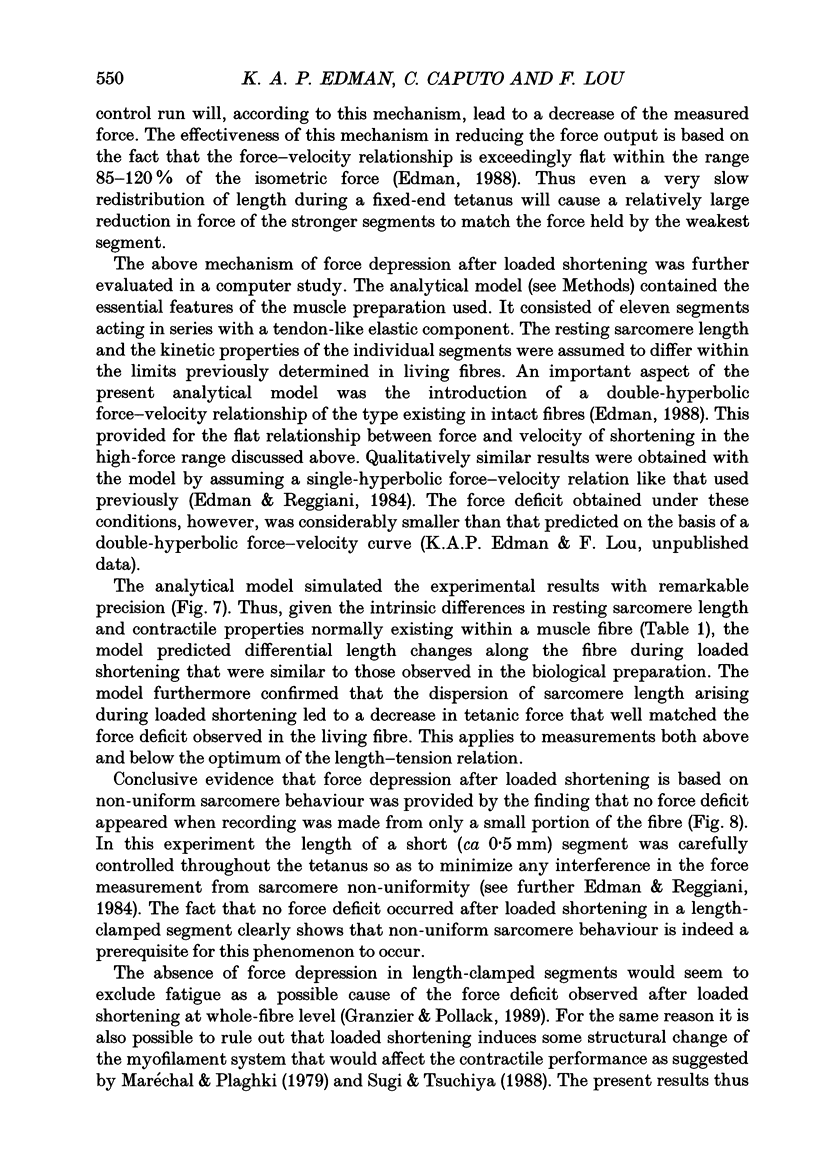
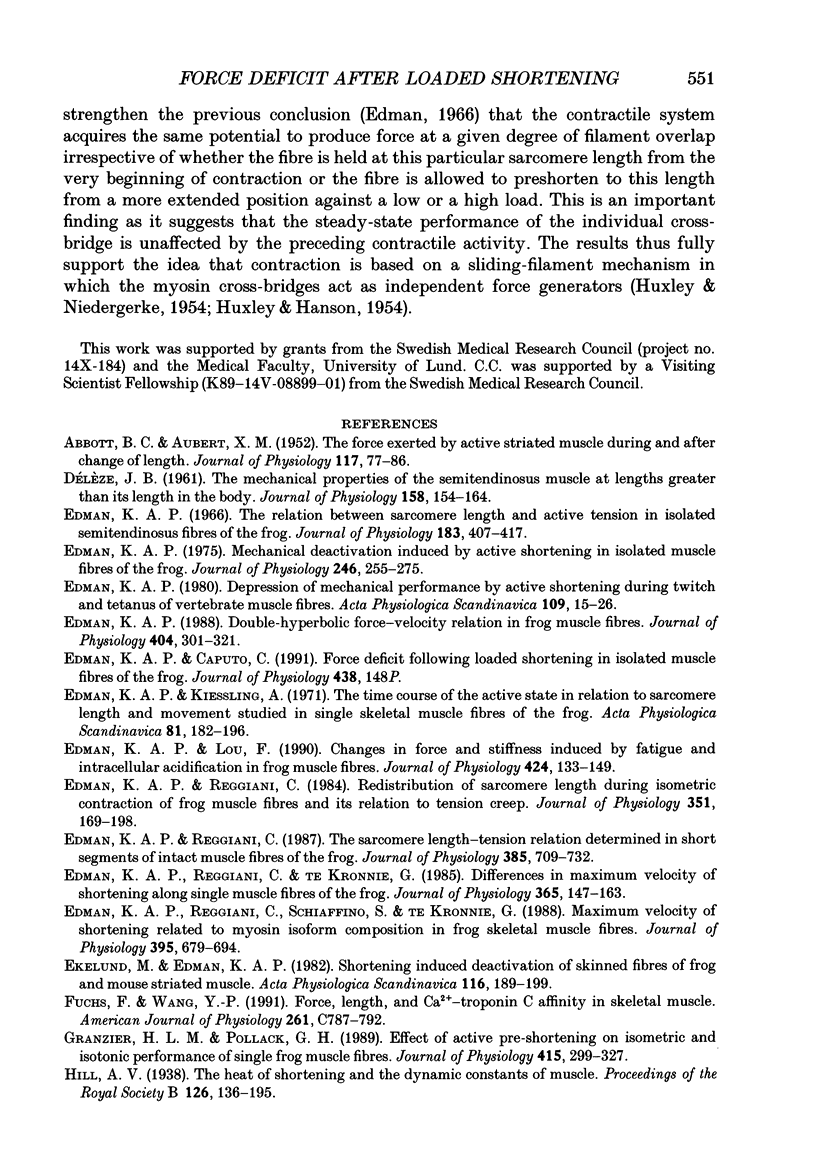
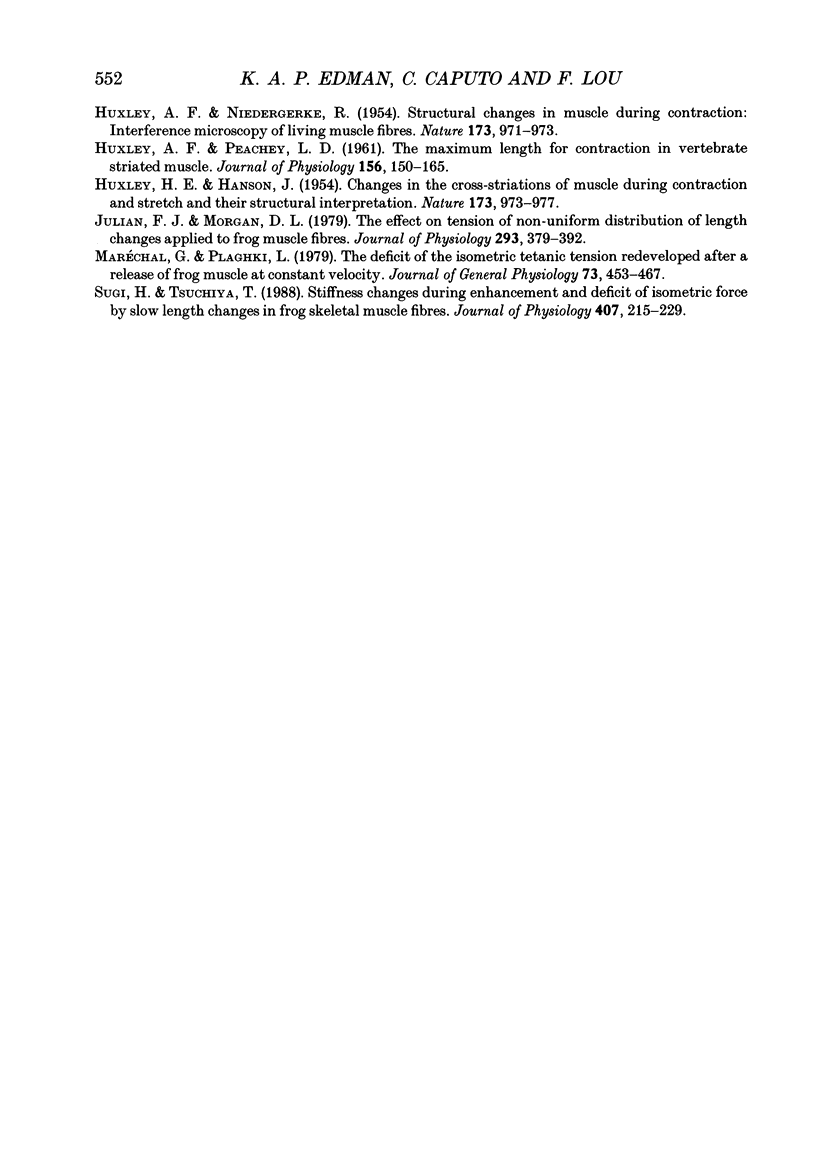
Selected References
These references are in PubMed. This may not be the complete list of references from this article.
- ABBOTT B. C., AUBERT X. M. The force exerted by active striated muscle during and after change of length. J Physiol. 1952 May;117(1):77–86. [PMC free article] [PubMed] [Google Scholar]
- DELEZE J. B. The mechanical properties of the semitendinosus muscle at lengths greater than its length in the body. J Physiol. 1961 Sep;158:154–164. [PMC free article] [PubMed] [Google Scholar]
- Edman K. A. Depression of mechanical performance by active shortening during twitch and tetanus of vertebrate muscle fibres. Acta Physiol Scand. 1980 May;109(1):15–26. doi: 10.1111/j.1748-1716.1980.tb06559.x. [DOI] [PubMed] [Google Scholar]
- Edman K. A. Double-hyperbolic force-velocity relation in frog muscle fibres. J Physiol. 1988 Oct;404:301–321. doi: 10.1113/jphysiol.1988.sp017291. [DOI] [PMC free article] [PubMed] [Google Scholar]
- Edman K. A., Kiessling A. The time course of the active state in relation to sarcomere length and movement studied in single skeletal muscle fibres of the frog. Acta Physiol Scand. 1971 Feb;81(2):182–196. doi: 10.1111/j.1748-1716.1971.tb04891.x. [DOI] [PubMed] [Google Scholar]
- Edman K. A., Lou F. Changes in force and stiffness induced by fatigue and intracellular acidification in frog muscle fibres. J Physiol. 1990 May;424:133–149. doi: 10.1113/jphysiol.1990.sp018059. [DOI] [PMC free article] [PubMed] [Google Scholar]
- Edman K. A. Mechanical deactivation induced by active shortening in isolated muscle fibres of the frog. J Physiol. 1975 Mar;246(1):255–275. doi: 10.1113/jphysiol.1975.sp010889. [DOI] [PMC free article] [PubMed] [Google Scholar]
- Edman K. A., Reggiani C. Redistribution of sarcomere length during isometric contraction of frog muscle fibres and its relation to tension creep. J Physiol. 1984 Jun;351:169–198. doi: 10.1113/jphysiol.1984.sp015240. [DOI] [PMC free article] [PubMed] [Google Scholar]
- Edman K. A., Reggiani C., Schiaffino S., te Kronnie G. Maximum velocity of shortening related to myosin isoform composition in frog skeletal muscle fibres. J Physiol. 1988 Jan;395:679–694. doi: 10.1113/jphysiol.1988.sp016941. [DOI] [PMC free article] [PubMed] [Google Scholar]
- Edman K. A., Reggiani C. The sarcomere length-tension relation determined in short segments of intact muscle fibres of the frog. J Physiol. 1987 Apr;385:709–732. doi: 10.1113/jphysiol.1987.sp016516. [DOI] [PMC free article] [PubMed] [Google Scholar]
- Edman K. A., Reggiani C., te Kronnie G. Differences in maximum velocity of shortening along single muscle fibres of the frog. J Physiol. 1985 Aug;365:147–163. doi: 10.1113/jphysiol.1985.sp015764. [DOI] [PMC free article] [PubMed] [Google Scholar]
- Edman K. A. The relation between sarcomere length and active tension in isolated semitendinosus fibres of the frog. J Physiol. 1966 Mar;183(2):407–417. doi: 10.1113/jphysiol.1966.sp007873. [DOI] [PMC free article] [PubMed] [Google Scholar]
- Ekelund M. C., Edman K. A. Shortening induced deactivation of skinned fibres of frog and mouse striated muscle. Acta Physiol Scand. 1982 Oct;116(2):189–199. doi: 10.1111/j.1748-1716.1982.tb07129.x. [DOI] [PubMed] [Google Scholar]
- Fuchs F., Wang Y. P. Force, length, and Ca(2+)-troponin C affinity in skeletal muscle. Am J Physiol. 1991 Nov;261(5 Pt 1):C787–C792. doi: 10.1152/ajpcell.1991.261.5.C787. [DOI] [PubMed] [Google Scholar]
- Granzier H. L., Pollack G. H. Effect of active pre-shortening on isometric and isotonic performance of single frog muscle fibres. J Physiol. 1989 Aug;415:299–327. doi: 10.1113/jphysiol.1989.sp017723. [DOI] [PMC free article] [PubMed] [Google Scholar]
- HUXLEY A. F., NIEDERGERKE R. Structural changes in muscle during contraction; interference microscopy of living muscle fibres. Nature. 1954 May 22;173(4412):971–973. doi: 10.1038/173971a0. [DOI] [PubMed] [Google Scholar]
- HUXLEY A. F., PEACHEY L. D. The maximum length for contraction in vertebrate straiated muscle. J Physiol. 1961 Apr;156:150–165. doi: 10.1113/jphysiol.1961.sp006665. [DOI] [PMC free article] [PubMed] [Google Scholar]
- HUXLEY H., HANSON J. Changes in the cross-striations of muscle during contraction and stretch and their structural interpretation. Nature. 1954 May 22;173(4412):973–976. doi: 10.1038/173973a0. [DOI] [PubMed] [Google Scholar]
- Julian F. J., Morgan D. L. The effect on tension of non-uniform distribution of length changes applied to frog muscle fibres. J Physiol. 1979 Aug;293:379–392. doi: 10.1113/jphysiol.1979.sp012895. [DOI] [PMC free article] [PubMed] [Google Scholar]
- Maréchal G., Plaghki L. The deficit of the isometric tetanic tension redeveloped after a release of frog muscle at a constant velocity. J Gen Physiol. 1979 Apr;73(4):453–467. doi: 10.1085/jgp.73.4.453. [DOI] [PMC free article] [PubMed] [Google Scholar]
- Sugi H., Tsuchiya T. Stiffness changes during enhancement and deficit of isometric force by slow length changes in frog skeletal muscle fibres. J Physiol. 1988 Dec;407:215–229. doi: 10.1113/jphysiol.1988.sp017411. [DOI] [PMC free article] [PubMed] [Google Scholar]