Abstract
1. Both inward and outward unitary Li+ currents through the L-type Ca2+ channel and discrete block of such currents by either internal or external Ca2+ are recorded. Detailed kinetic analyses are obtained for all of the four experimental configurations (internal or external Ca2+ block of either inward or outward Li+ currents). 2. No matter from which side the blocking Ca2+ ion comes, the exit (unblocking) rates are always the same at the same potential in the same direction of Li+ current flow. This indicates that the high-affinity Ca2+ binding site (the blocking site) is in the pore, and internal and external Ca2+ both go to the same site to produce the block. In other words, there can only be one high-affinity Ca2+ binding site or one set of such sites (sites separated by insignificant barriers) in the pore. Furthermore, the direction of exit of the blocking Ca2+ ion is always with, not against, the Li+ current flow. This suggests ion-ion interaction (the 'long pore effect') in the high-affinity sites. Therefore there must be more than one high-affinity site in the pore. Overall it is concluded that the pore must contain a set of high-affinity Ca2+ binding sites separated by insignificant energy barriers. 3. The voltage dependence of the off- (exit) rates is very similar in amplitude for all the four experimental configurations (e-fold change per approximately 25 mV depolarization or hyperpolarization). This strong voltage dependence in every configuration cannot be explained by any Ca2+ energy profile alone and must include a certain contribution from Li+. The mechanism underlying such a contribution seems to reside in the enhancement effect of Li+ on the exist of Ca2+. 4. The on-rates (blocking rates) for external Ca2+ are always fast no matter whether the Li+ currents are outward or inward. In certain cases the rates even approach the diffusion-controlled limit (approximately 10(9) M-1 S-1). This suggests that the high-affinity sites are very easily accessible from the outside, and probably there is no other ionic site located between the external pore mouth and the high-affinity sites. 5. The on-rates for internal Ca2+ are fast and voltage independent in outward Li+ currents, but are very slow and strongly voltage dependent in inward Li+ currents.(ABSTRACT TRUNCATED AT 400 WORDS)
Full text
PDF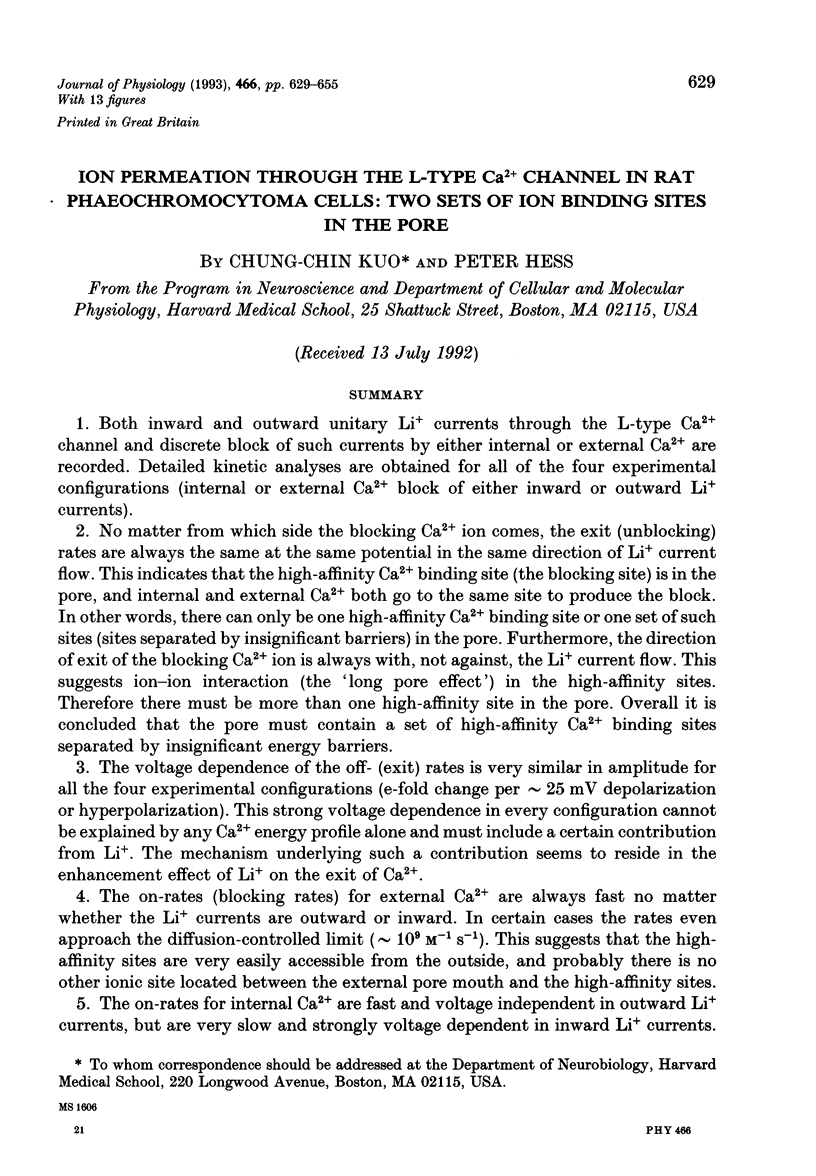
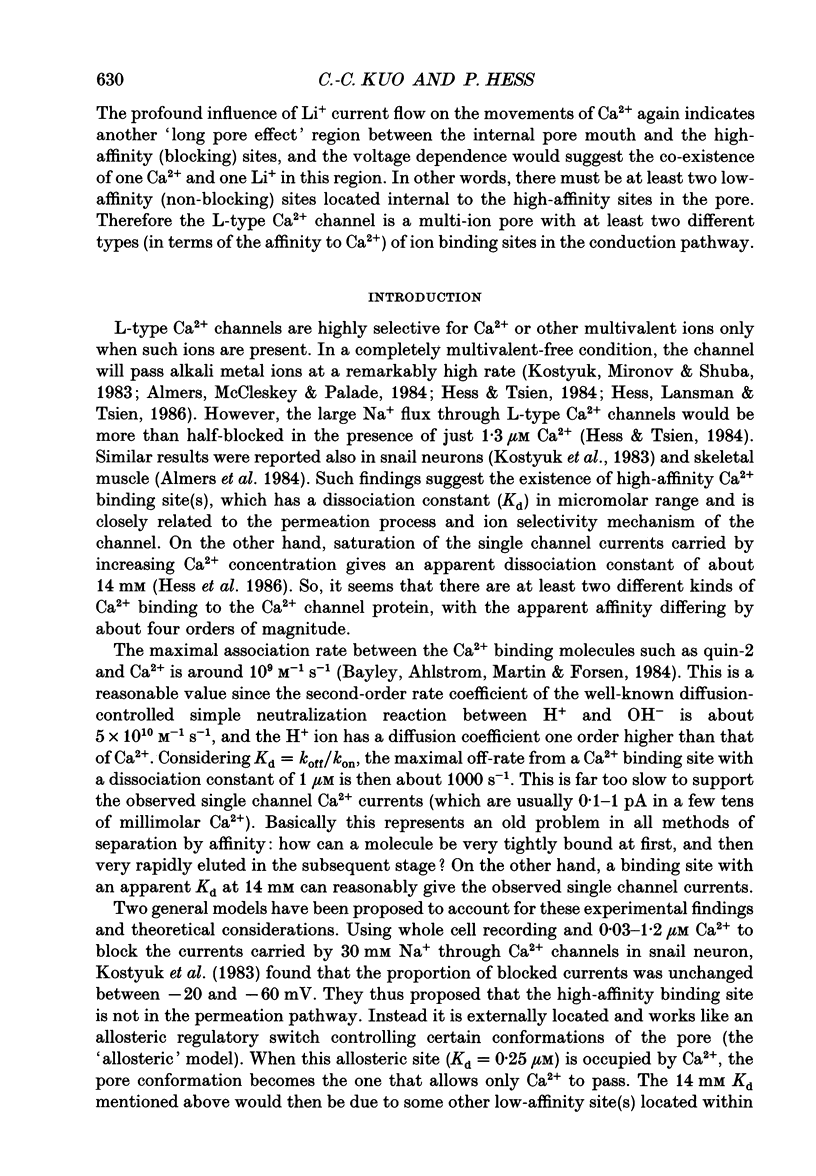
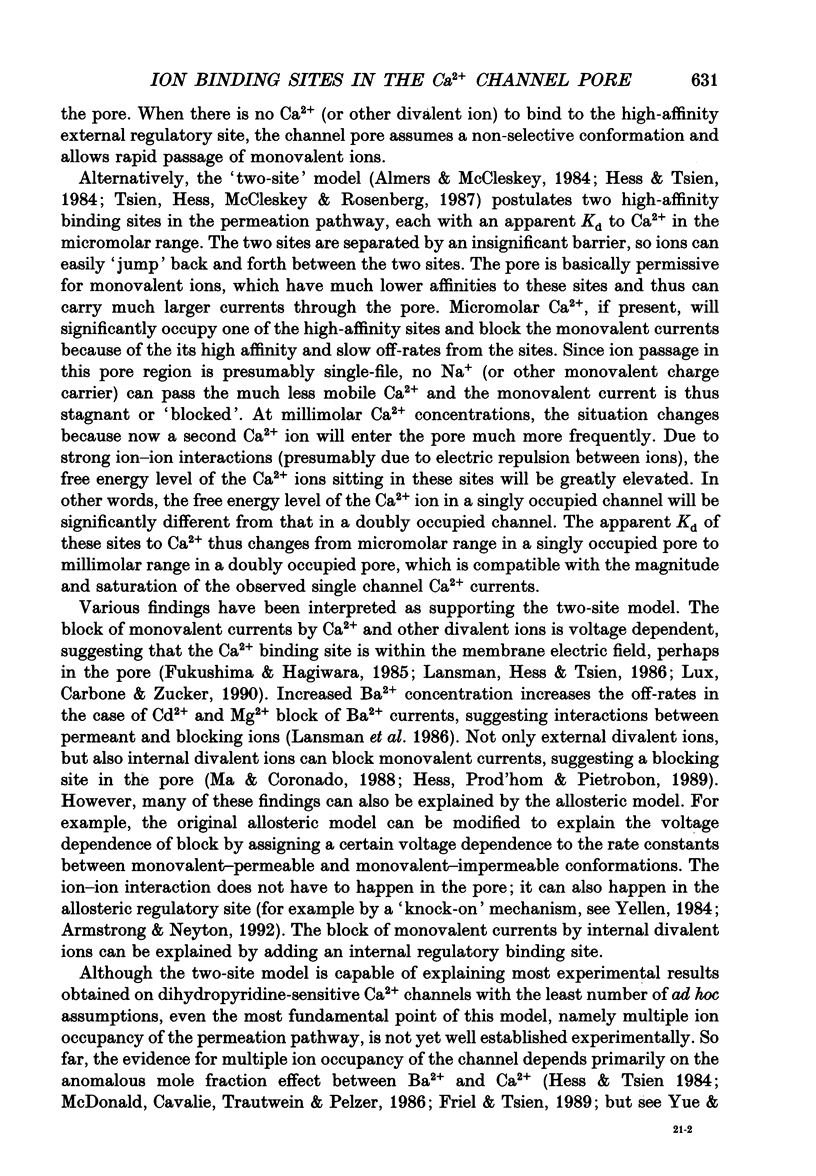
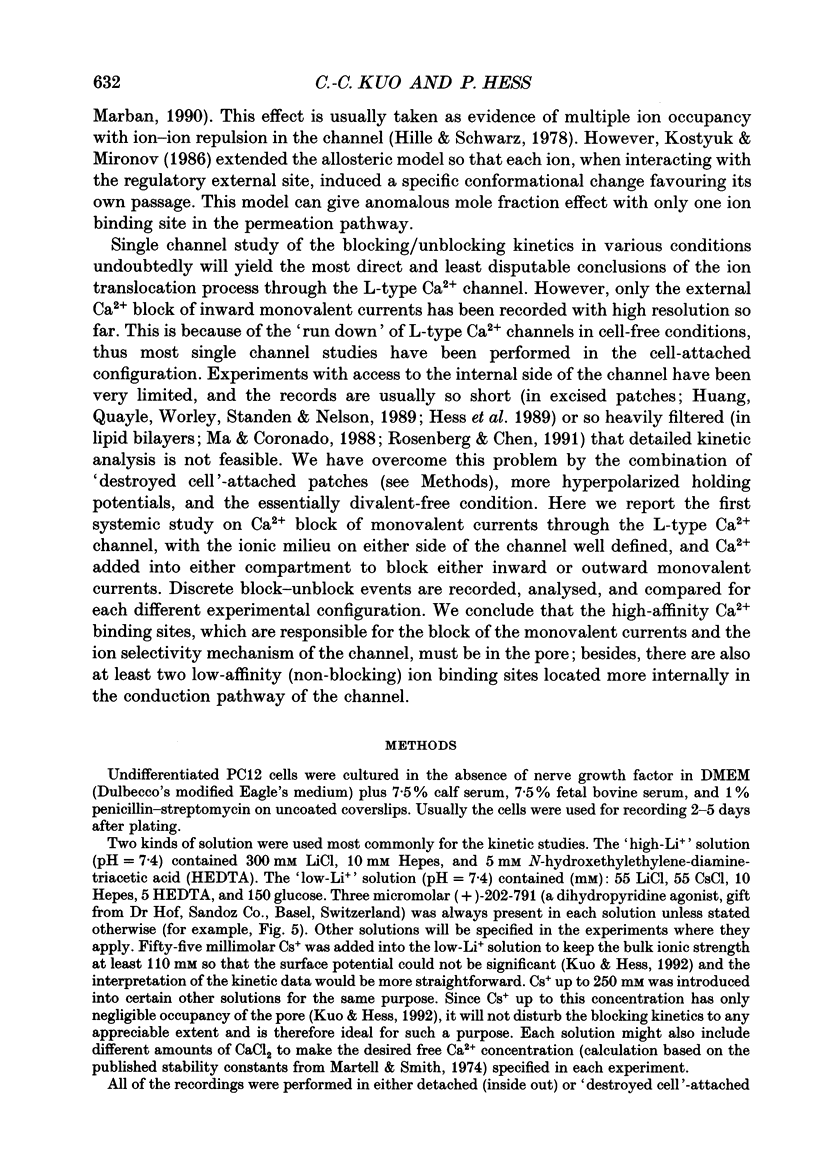
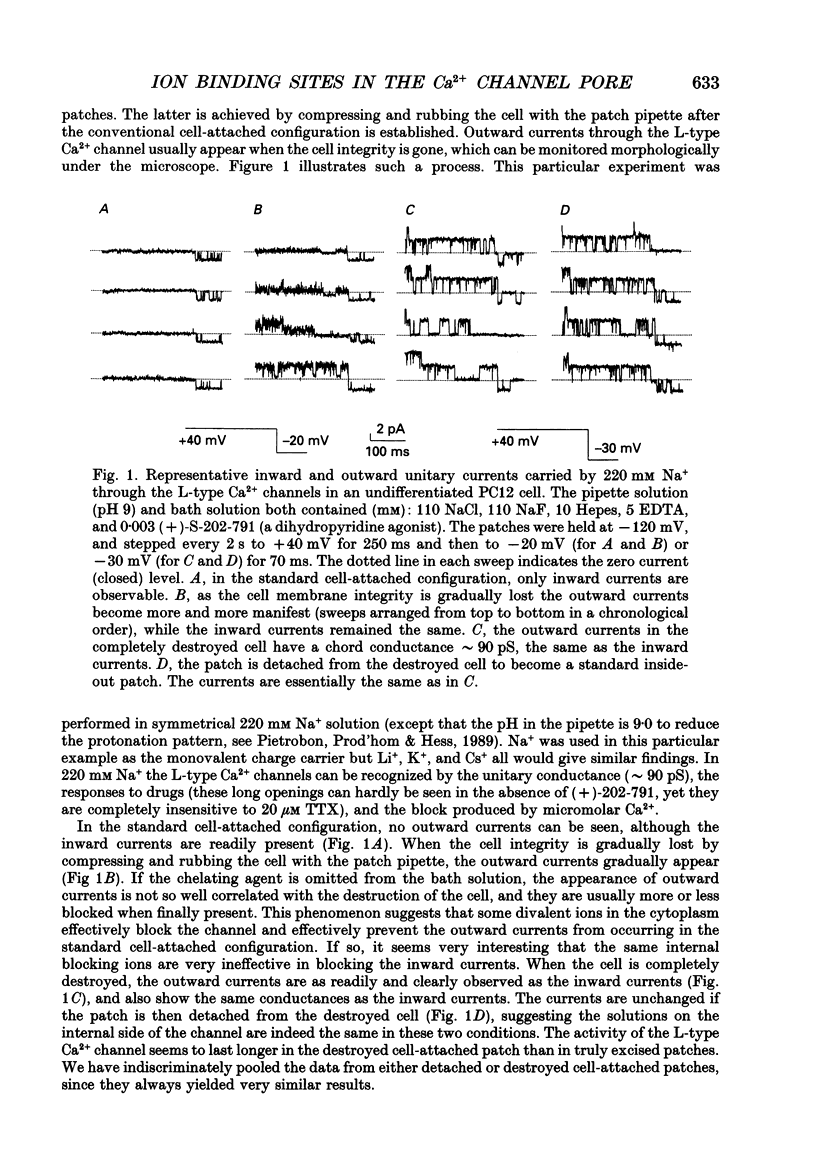
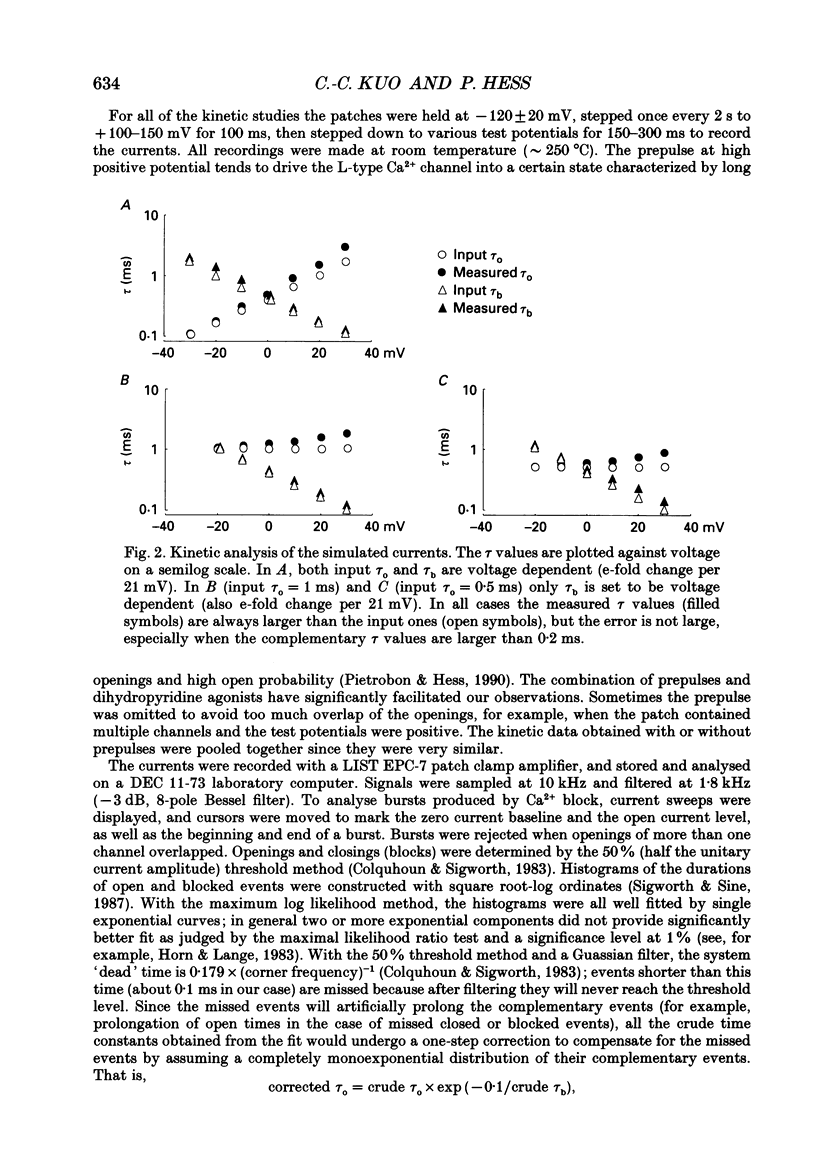
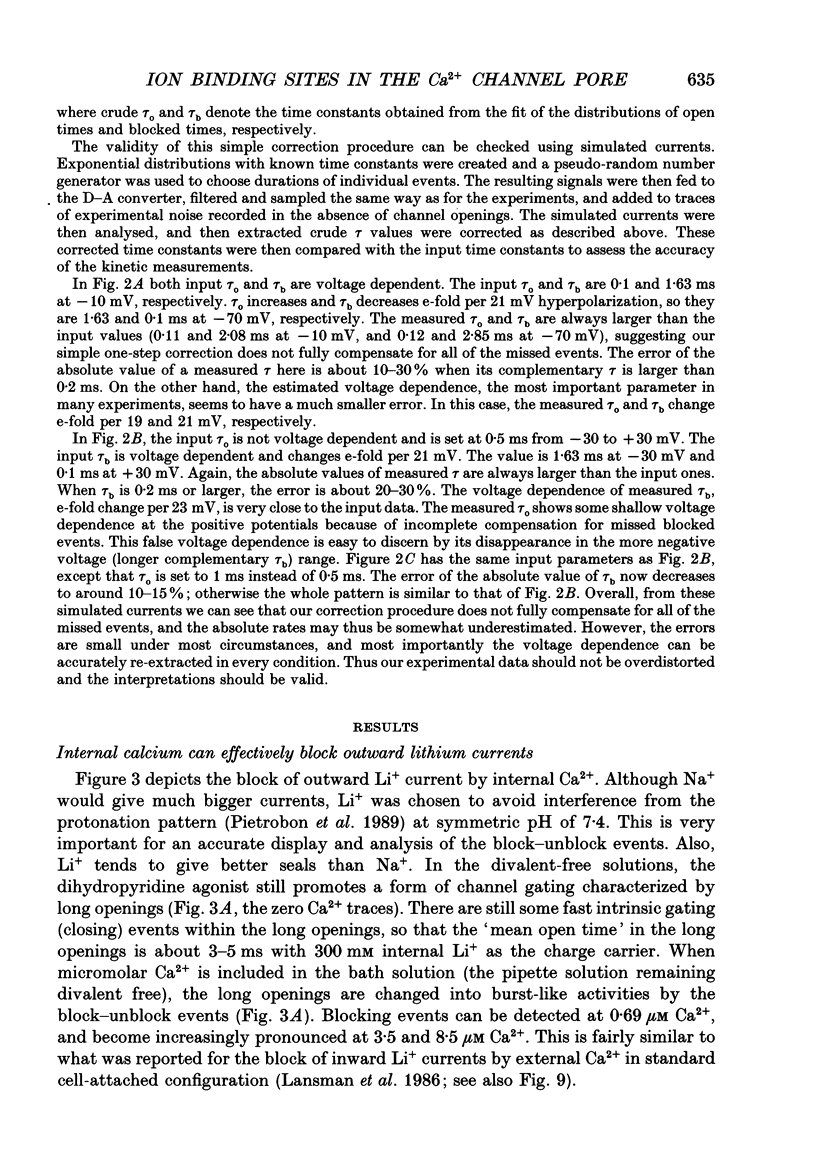
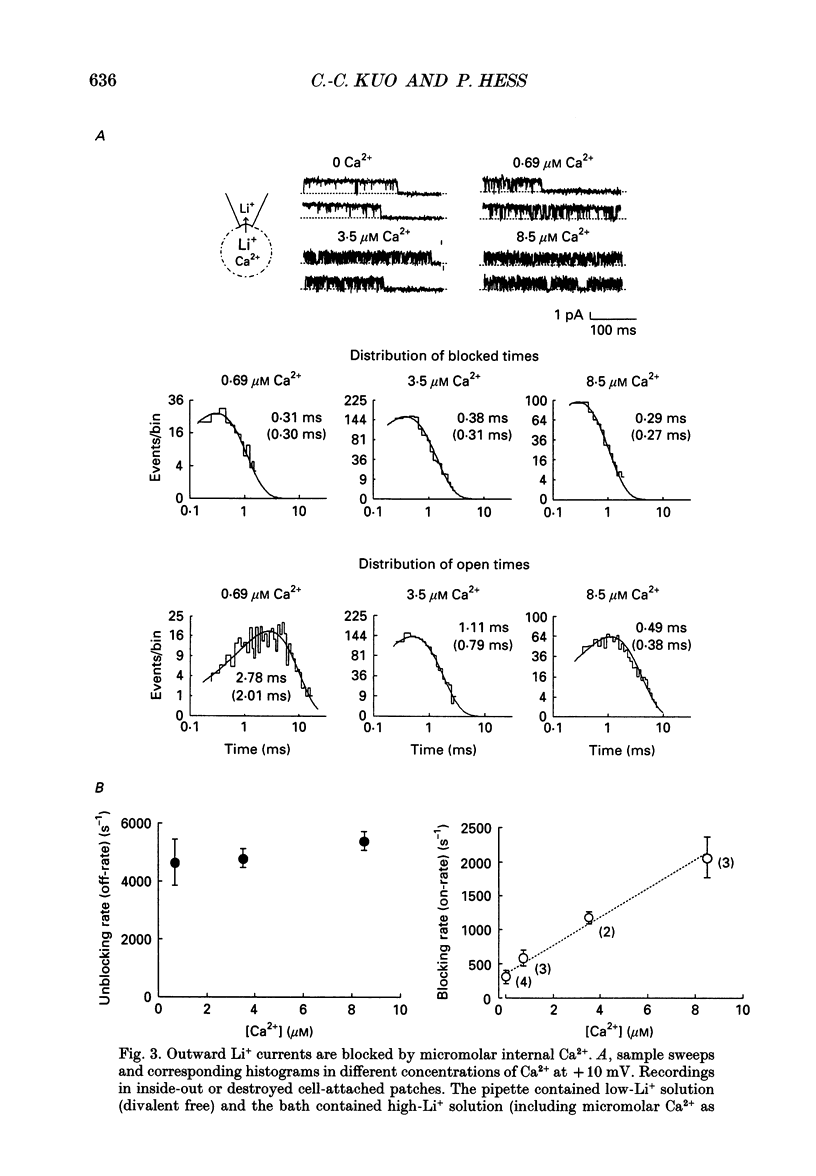
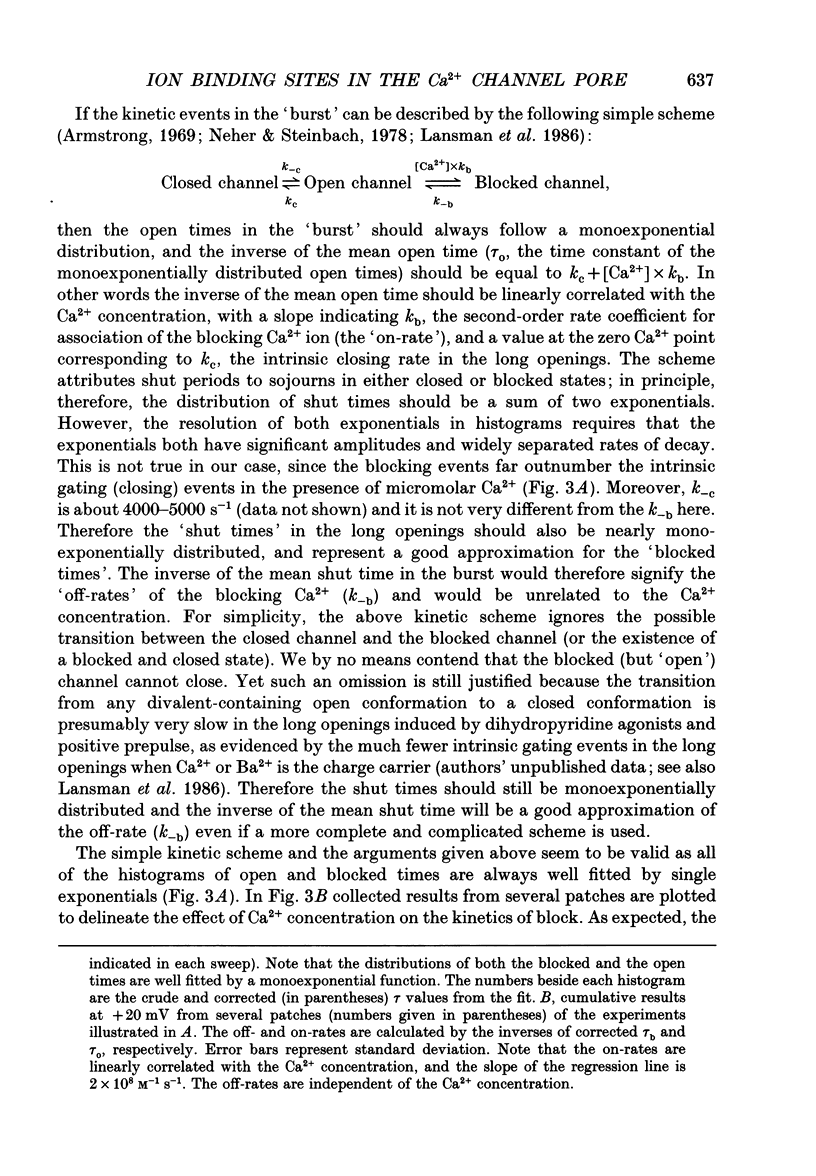
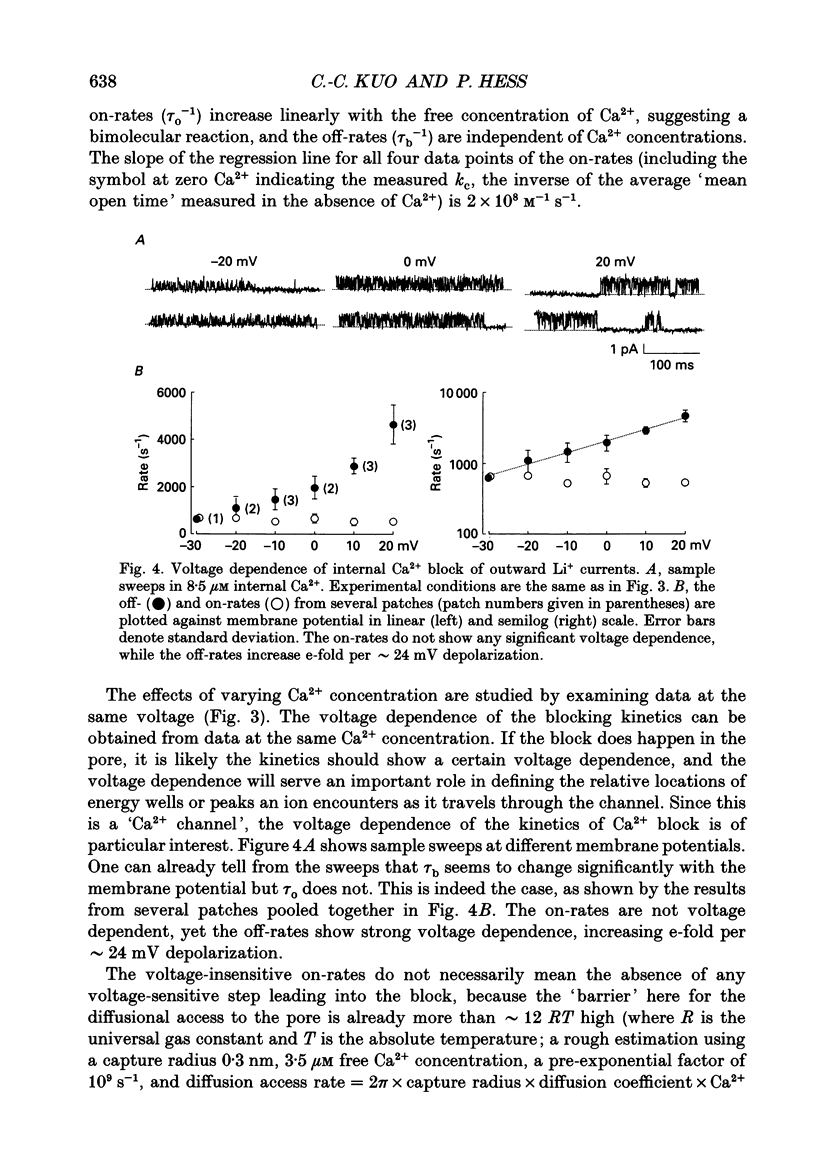
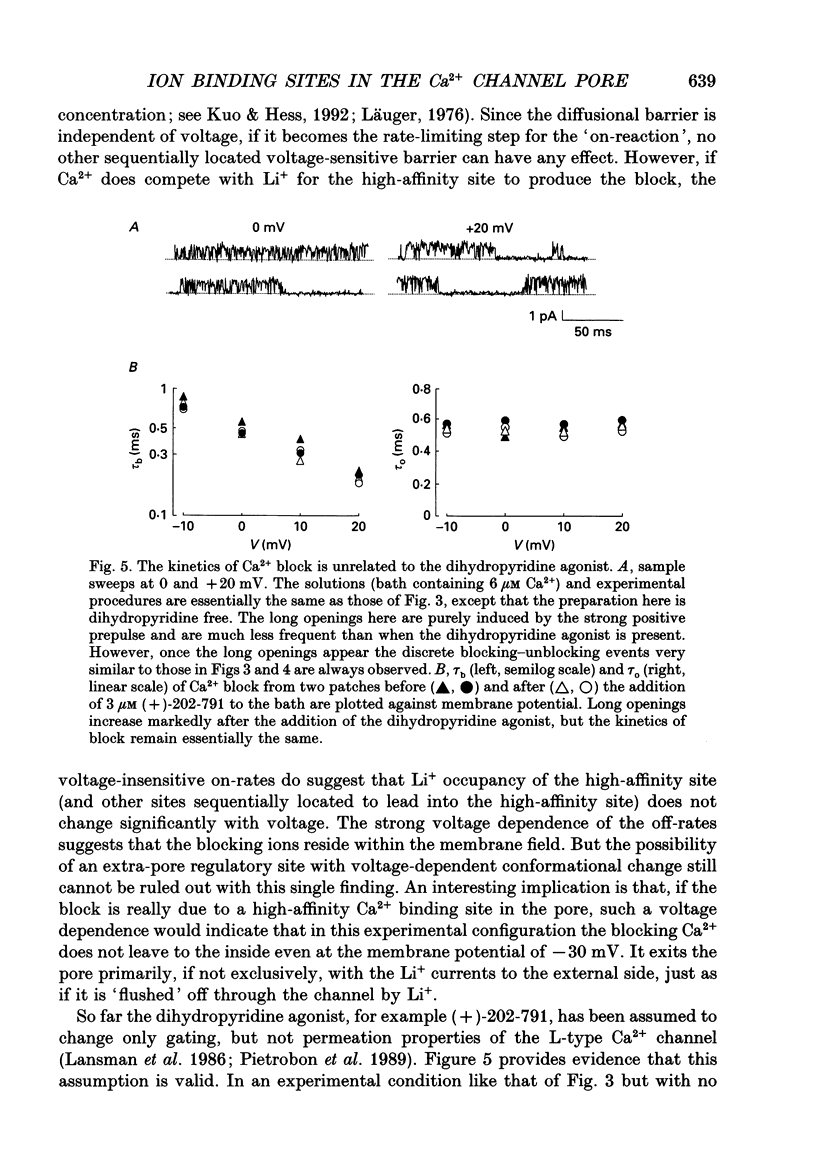
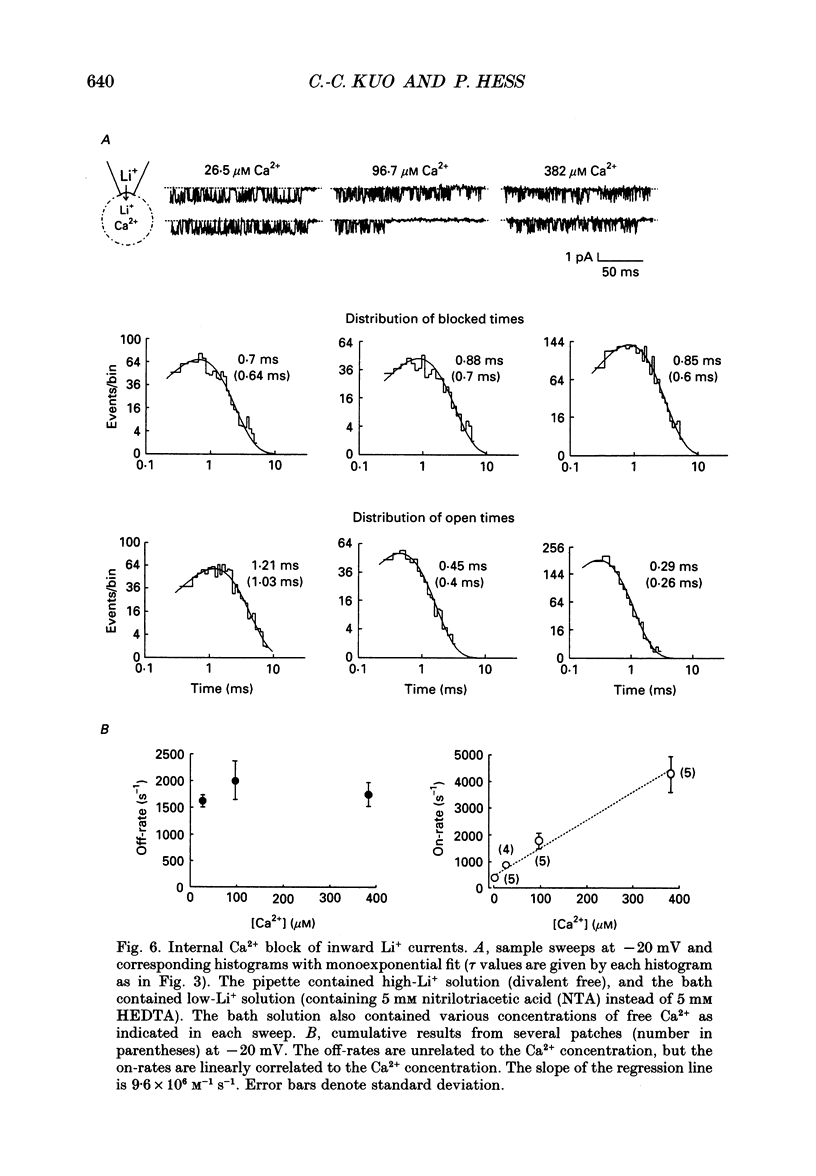
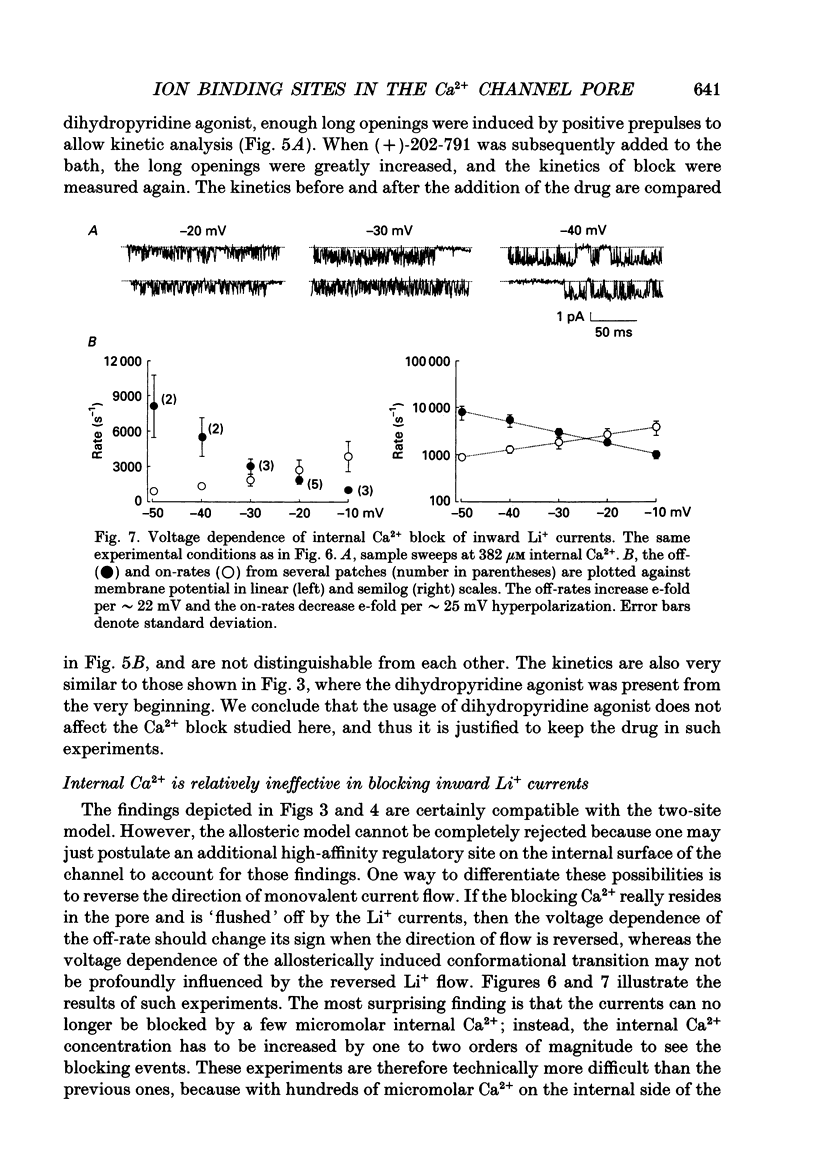
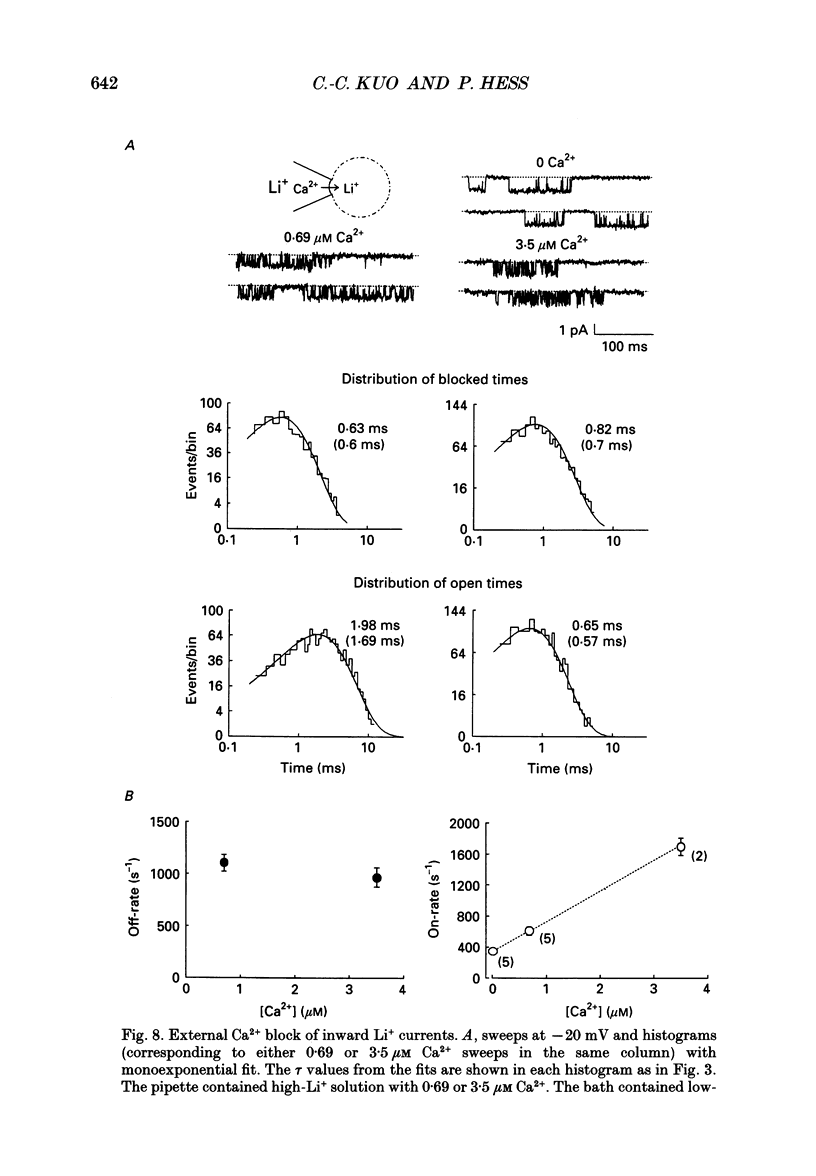
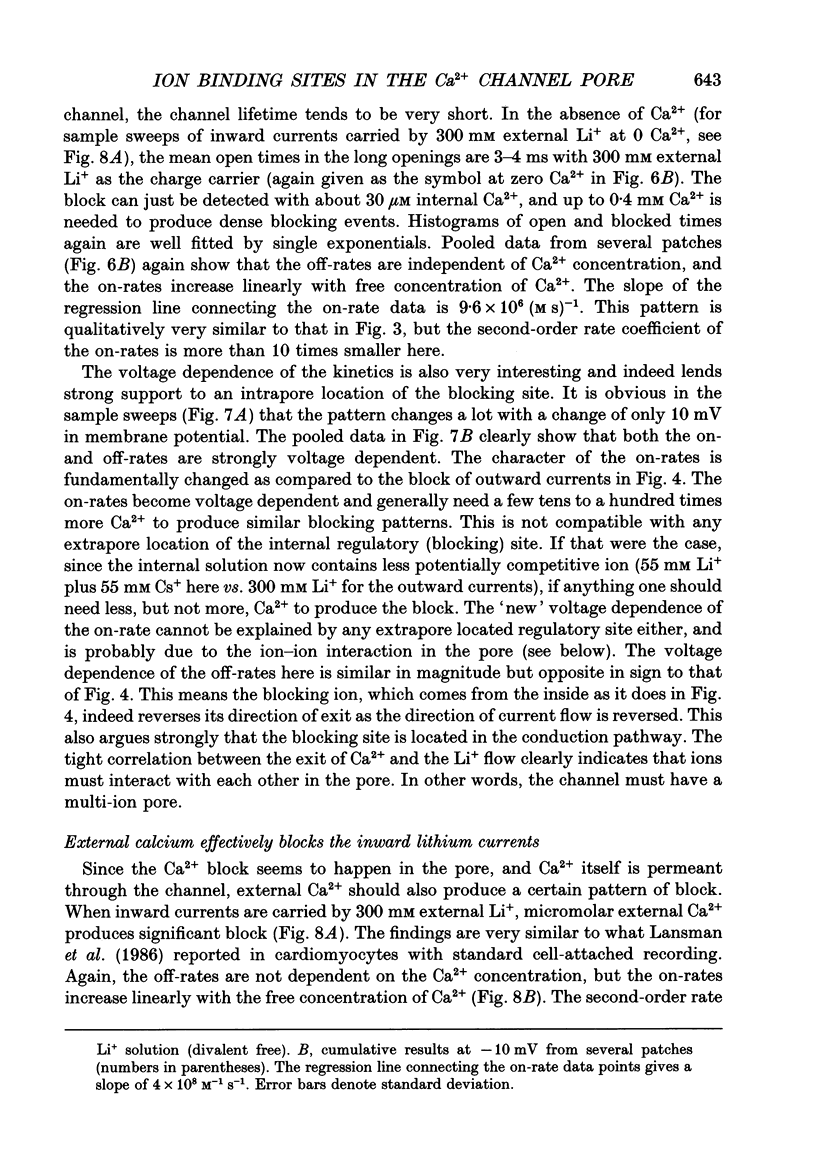
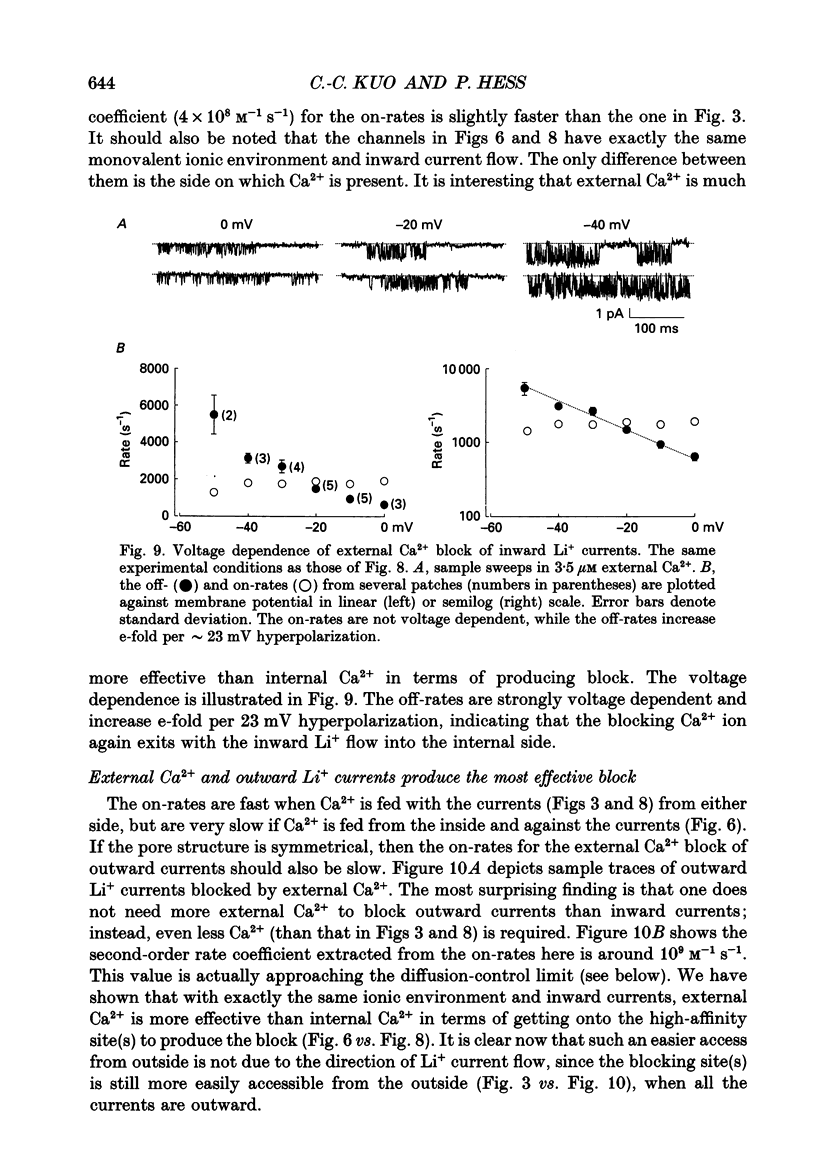
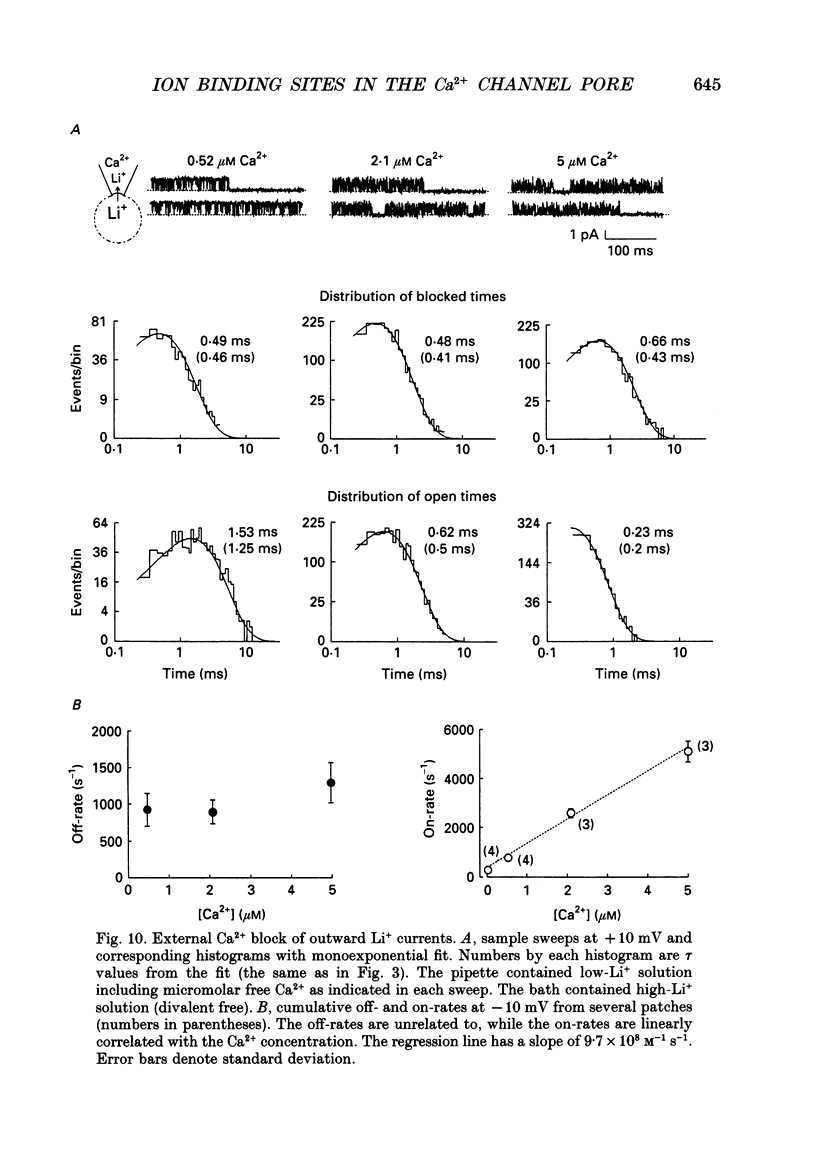
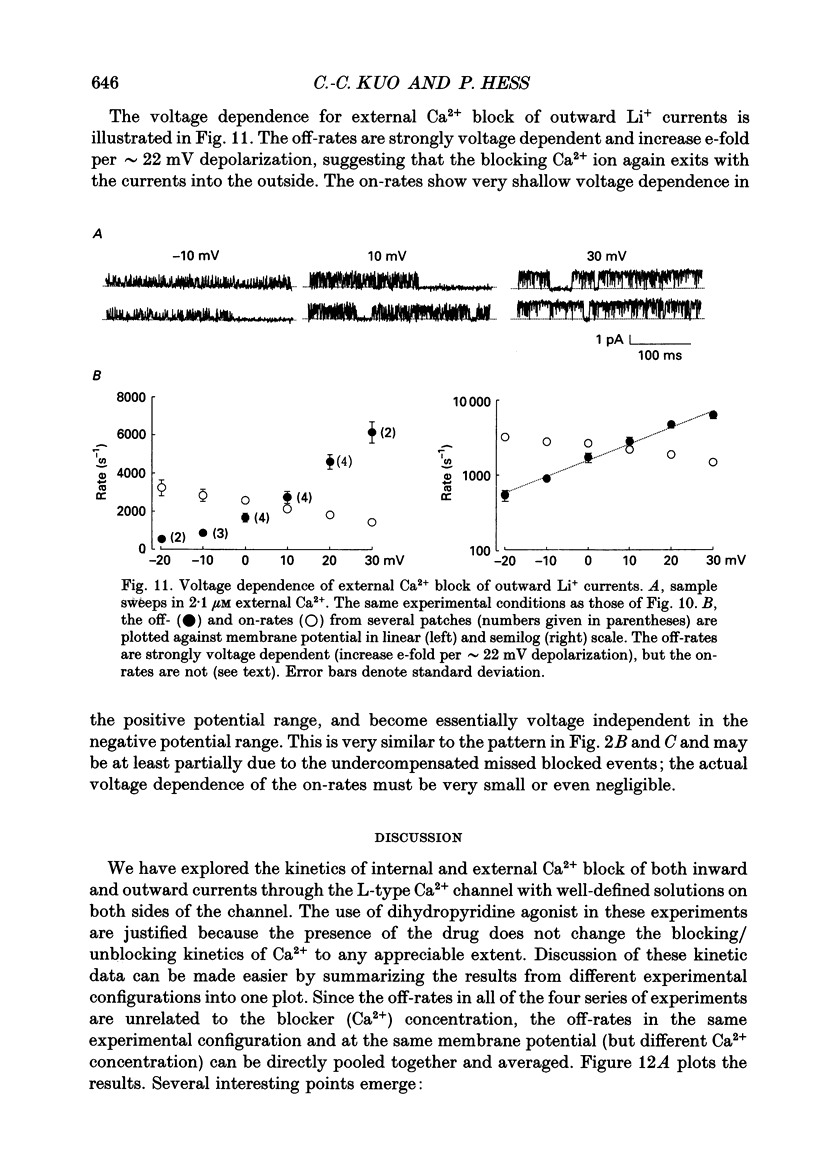
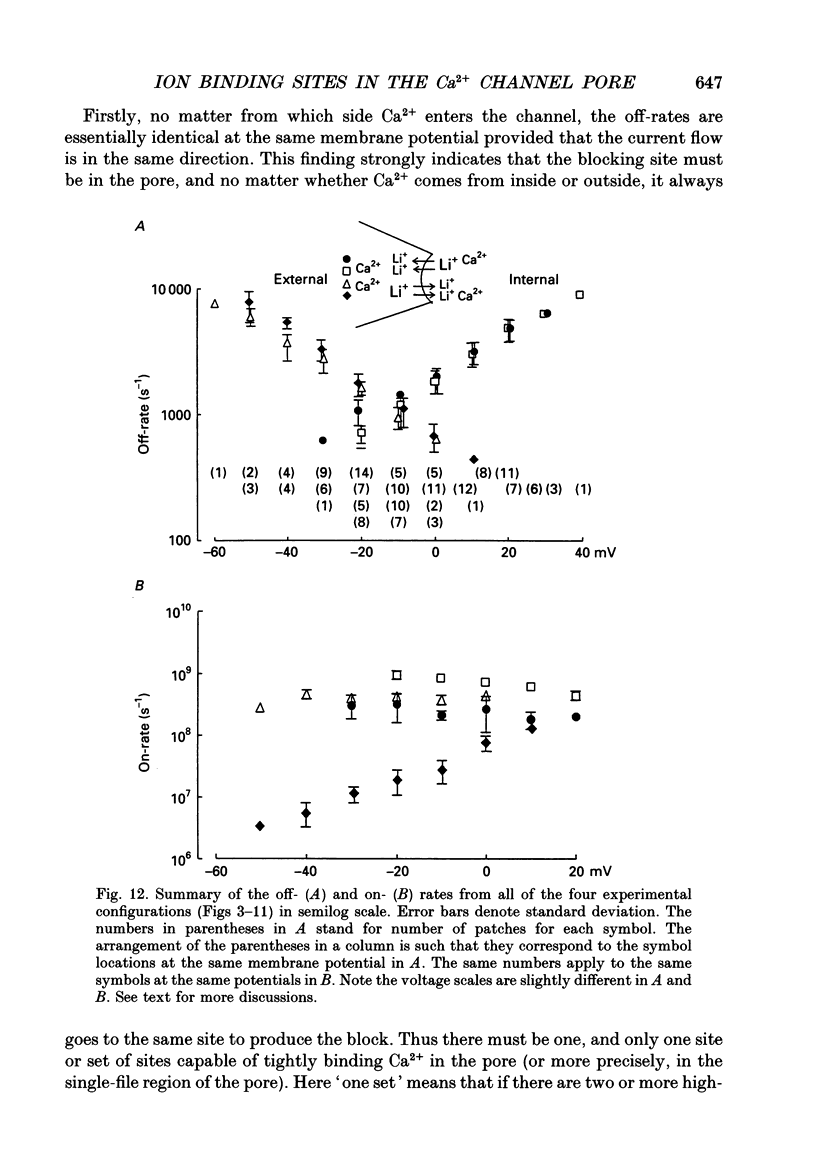
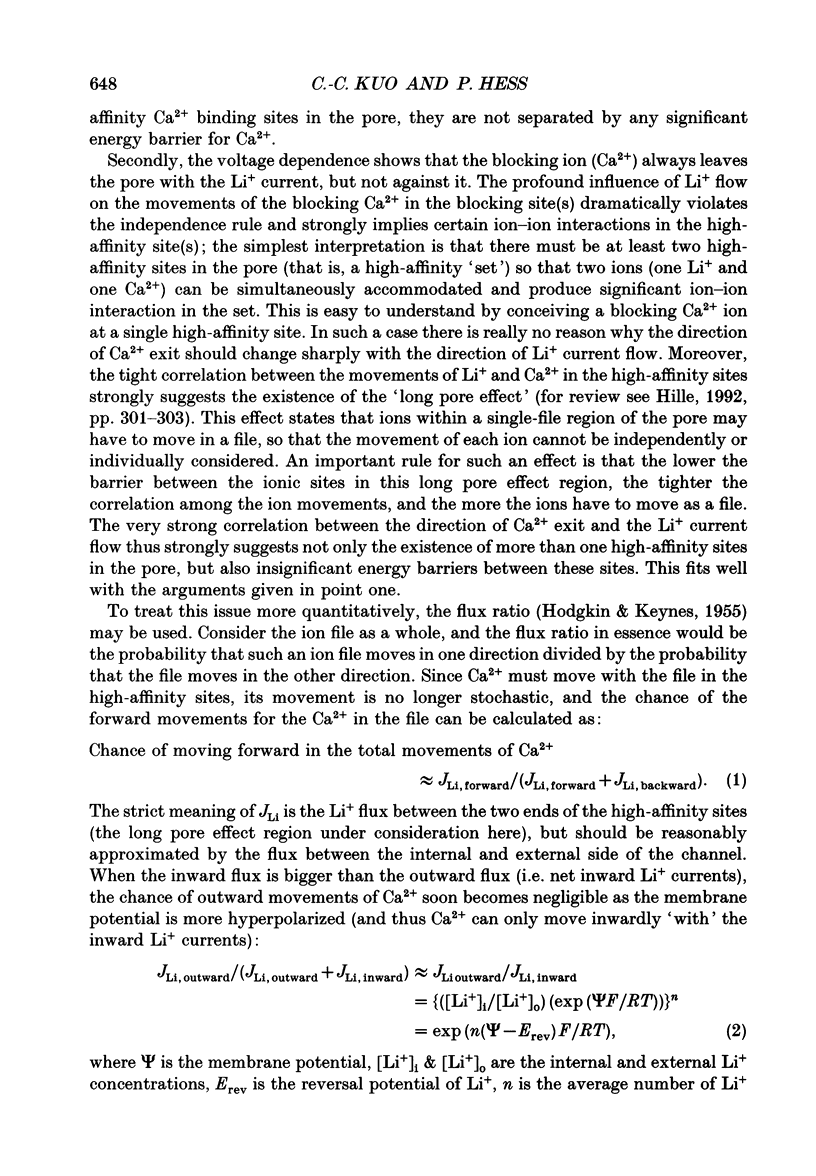
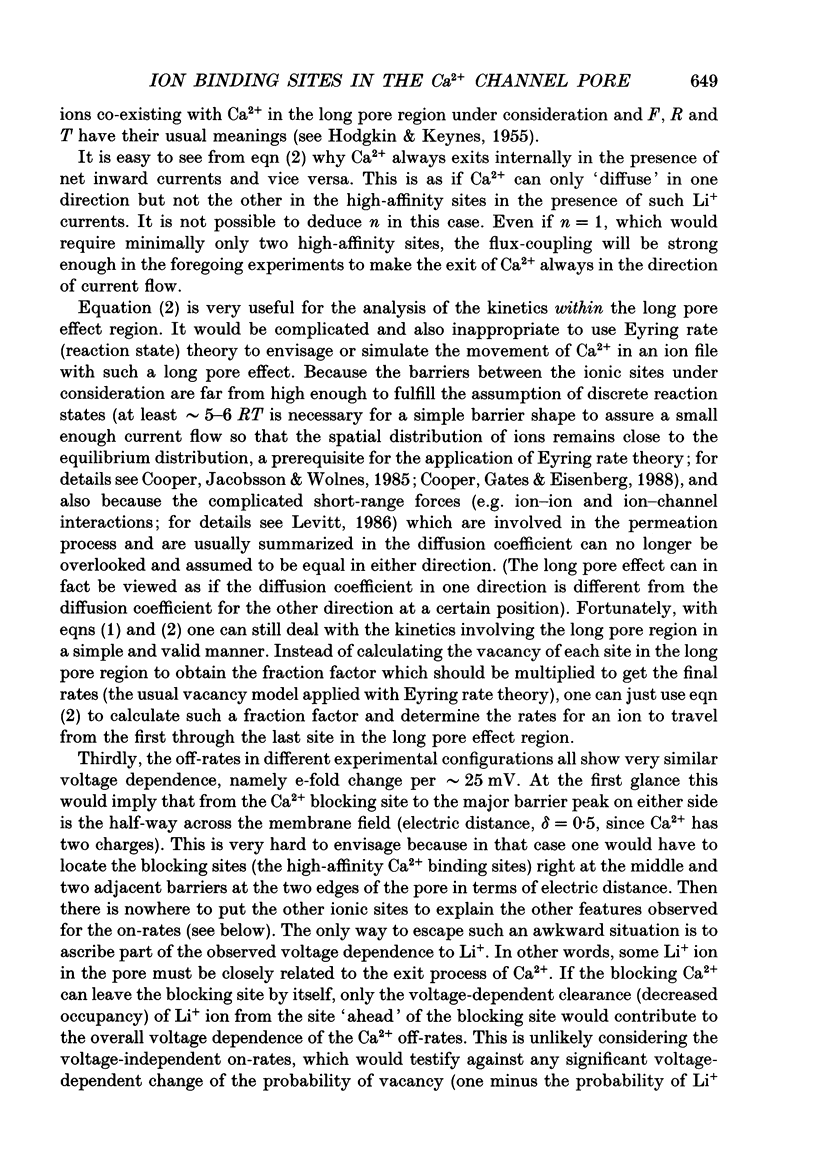
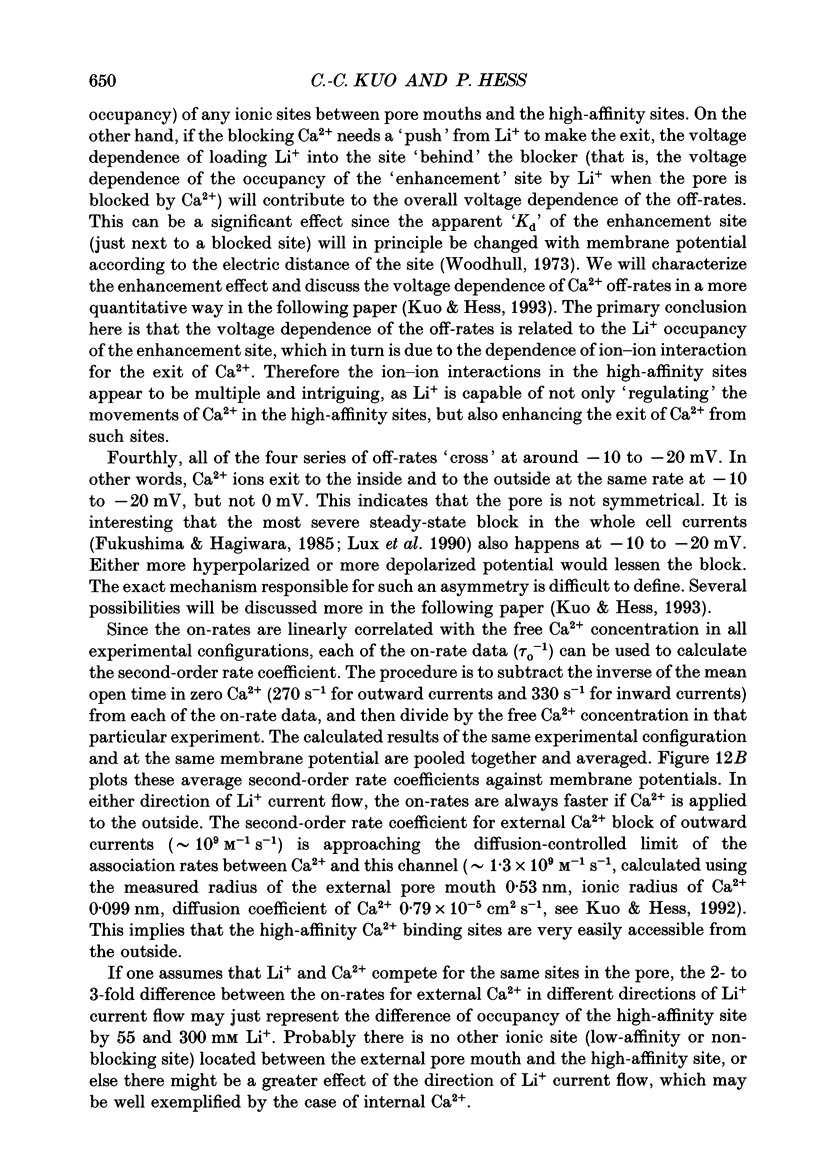
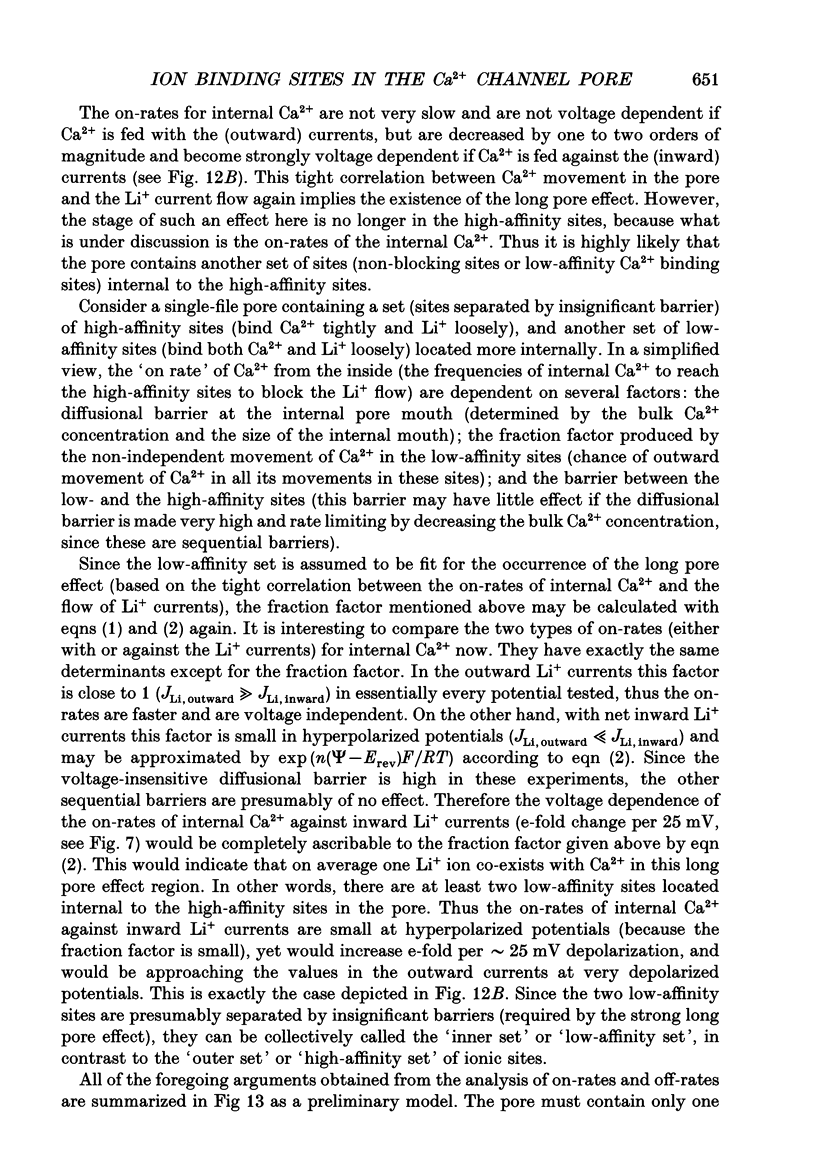
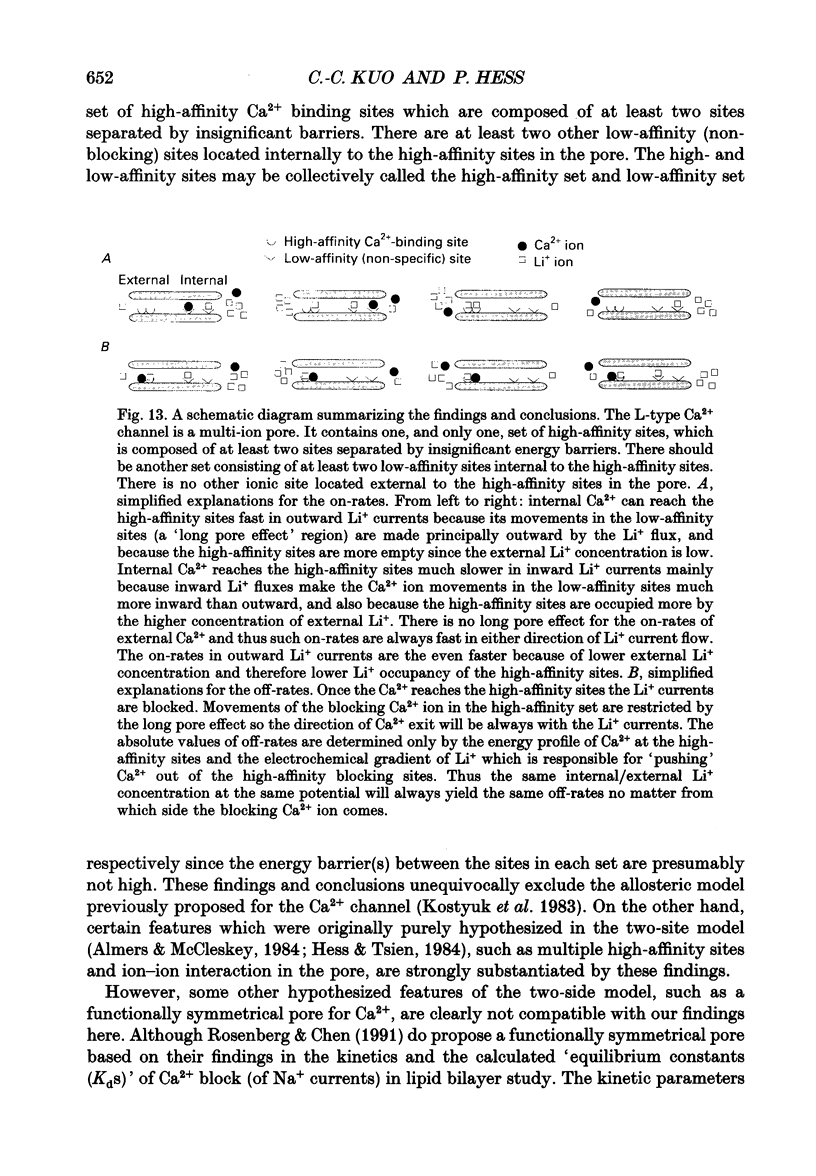
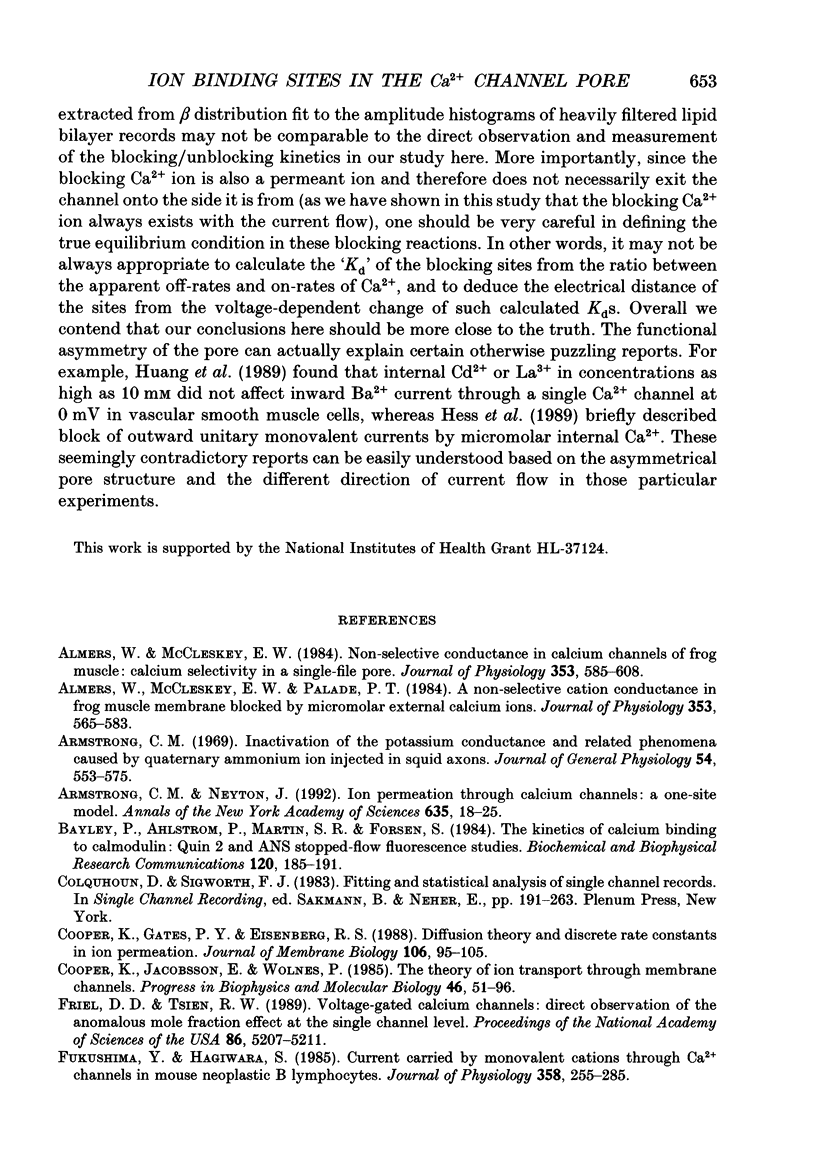
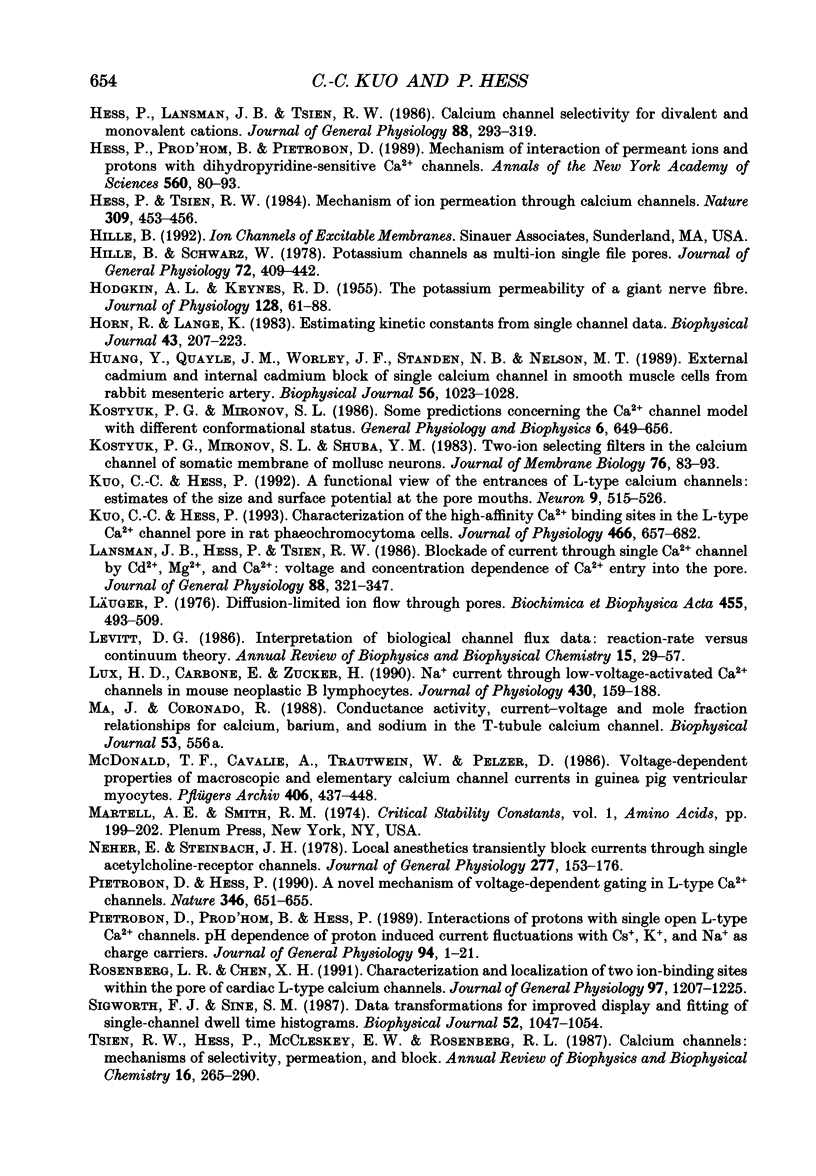
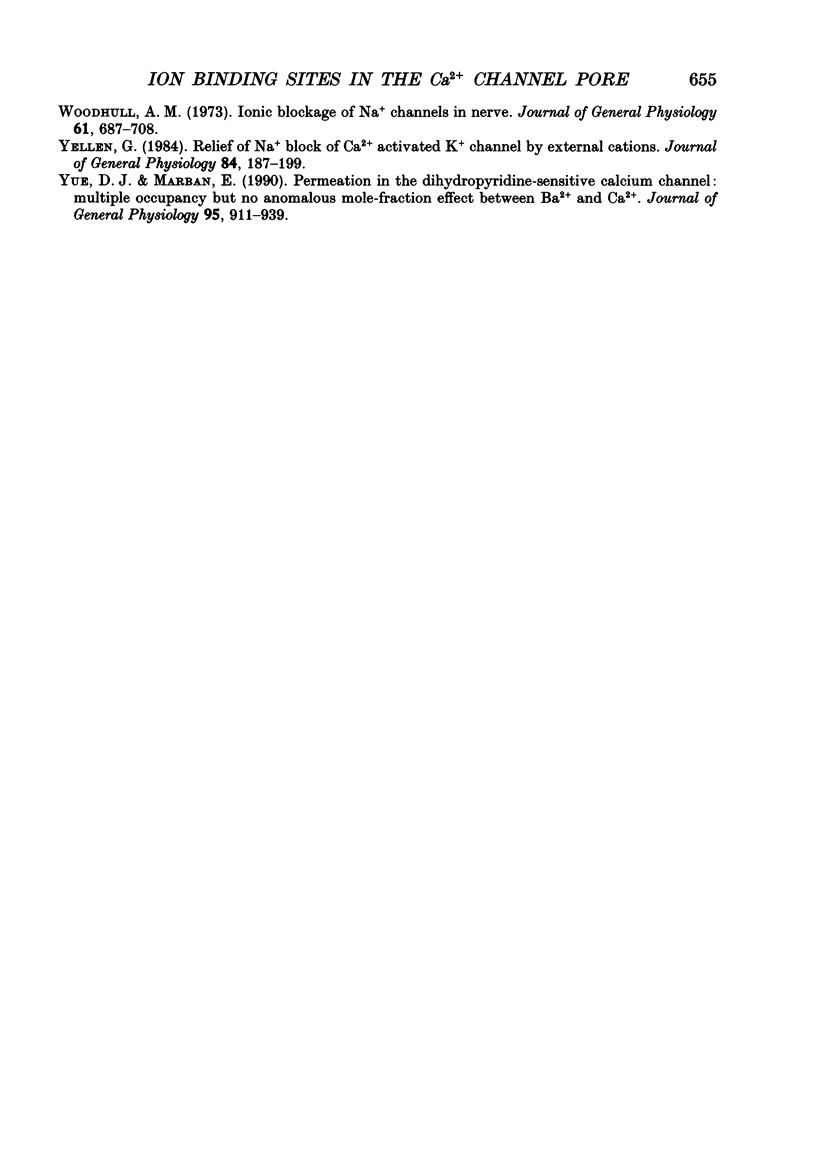
Selected References
These references are in PubMed. This may not be the complete list of references from this article.
- Almers W., McCleskey E. W. Non-selective conductance in calcium channels of frog muscle: calcium selectivity in a single-file pore. J Physiol. 1984 Aug;353:585–608. doi: 10.1113/jphysiol.1984.sp015352. [DOI] [PMC free article] [PubMed] [Google Scholar]
- Almers W., McCleskey E. W., Palade P. T. A non-selective cation conductance in frog muscle membrane blocked by micromolar external calcium ions. J Physiol. 1984 Aug;353:565–583. doi: 10.1113/jphysiol.1984.sp015351. [DOI] [PMC free article] [PubMed] [Google Scholar]
- Armstrong C. M. Inactivation of the potassium conductance and related phenomena caused by quaternary ammonium ion injection in squid axons. J Gen Physiol. 1969 Nov;54(5):553–575. doi: 10.1085/jgp.54.5.553. [DOI] [PMC free article] [PubMed] [Google Scholar]
- Armstrong C. M., Neyton J. Ion permeation through calcium channels. A one-site model. Ann N Y Acad Sci. 1991;635:18–25. doi: 10.1111/j.1749-6632.1991.tb36477.x. [DOI] [PubMed] [Google Scholar]
- Bayley P., Ahlström P., Martin S. R., Forsen S. The kinetics of calcium binding to calmodulin: Quin 2 and ANS stopped-flow fluorescence studies. Biochem Biophys Res Commun. 1984 Apr 16;120(1):185–191. doi: 10.1016/0006-291x(84)91431-1. [DOI] [PubMed] [Google Scholar]
- Cooper K. E., Gates P. Y., Eisenberg R. S. Diffusion theory and discrete rate constants in ion permeation. J Membr Biol. 1988 Dec;106(2):95–105. doi: 10.1007/BF01871391. [DOI] [PubMed] [Google Scholar]
- Cooper K., Jakobsson E., Wolynes P. The theory of ion transport through membrane channels. Prog Biophys Mol Biol. 1985;46(1):51–96. doi: 10.1016/0079-6107(85)90012-4. [DOI] [PubMed] [Google Scholar]
- Friel D. D., Tsien R. W. Voltage-gated calcium channels: direct observation of the anomalous mole fraction effect at the single-channel level. Proc Natl Acad Sci U S A. 1989 Jul;86(13):5207–5211. doi: 10.1073/pnas.86.13.5207. [DOI] [PMC free article] [PubMed] [Google Scholar]
- Fukushima Y., Hagiwara S. Currents carried by monovalent cations through calcium channels in mouse neoplastic B lymphocytes. J Physiol. 1985 Jan;358:255–284. doi: 10.1113/jphysiol.1985.sp015550. [DOI] [PMC free article] [PubMed] [Google Scholar]
- HODGKIN A. L., KEYNES R. D. The potassium permeability of a giant nerve fibre. J Physiol. 1955 Apr 28;128(1):61–88. doi: 10.1113/jphysiol.1955.sp005291. [DOI] [PMC free article] [PubMed] [Google Scholar]
- Hess P., Lansman J. B., Tsien R. W. Calcium channel selectivity for divalent and monovalent cations. Voltage and concentration dependence of single channel current in ventricular heart cells. J Gen Physiol. 1986 Sep;88(3):293–319. doi: 10.1085/jgp.88.3.293. [DOI] [PMC free article] [PubMed] [Google Scholar]
- Hess P., Prod'Hom B., Pietrobon D. Mechanisms of interaction of permeant ions and protons with dihydropyridine-sensitive calcium channels. Ann N Y Acad Sci. 1989;560:80–93. doi: 10.1111/j.1749-6632.1989.tb24082.x. [DOI] [PubMed] [Google Scholar]
- Hess P., Tsien R. W. Mechanism of ion permeation through calcium channels. 1984 May 31-Jun 6Nature. 309(5967):453–456. doi: 10.1038/309453a0. [DOI] [PubMed] [Google Scholar]
- Hille B., Schwarz W. Potassium channels as multi-ion single-file pores. J Gen Physiol. 1978 Oct;72(4):409–442. doi: 10.1085/jgp.72.4.409. [DOI] [PMC free article] [PubMed] [Google Scholar]
- Horn R., Lange K. Estimating kinetic constants from single channel data. Biophys J. 1983 Aug;43(2):207–223. doi: 10.1016/S0006-3495(83)84341-0. [DOI] [PMC free article] [PubMed] [Google Scholar]
- Huang Y., Quayle J. M., Worley J. F., Standen N. B., Nelson M. T. External cadmium and internal calcium block of single calcium channels in smooth muscle cells from rabbit mesenteric artery. Biophys J. 1989 Nov;56(5):1023–1028. doi: 10.1016/S0006-3495(89)82747-X. [DOI] [PMC free article] [PubMed] [Google Scholar]
- Kostyuk P. G., Mironov S. L. Some predictions concerning the calcium channel model with different conformational states. Gen Physiol Biophys. 1986 Dec;5(6):649–654. [PubMed] [Google Scholar]
- Kuo C. C., Hess P. A functional view of the entrances of L-type Ca2+ channels: estimates of the size and surface potential at the pore mouths. Neuron. 1992 Sep;9(3):515–526. doi: 10.1016/0896-6273(92)90189-k. [DOI] [PubMed] [Google Scholar]
- Kuo C. C., Hess P. Characterization of the high-affinity Ca2+ binding sites in the L-type Ca2+ channel pore in rat phaeochromocytoma cells. J Physiol. 1993 Jul;466:657–682. [PMC free article] [PubMed] [Google Scholar]
- Lansman J. B., Hess P., Tsien R. W. Blockade of current through single calcium channels by Cd2+, Mg2+, and Ca2+. Voltage and concentration dependence of calcium entry into the pore. J Gen Physiol. 1986 Sep;88(3):321–347. doi: 10.1085/jgp.88.3.321. [DOI] [PMC free article] [PubMed] [Google Scholar]
- Levitt D. G. Interpretation of biological ion channel flux data--reaction-rate versus continuum theory. Annu Rev Biophys Biophys Chem. 1986;15:29–57. doi: 10.1146/annurev.bb.15.060186.000333. [DOI] [PubMed] [Google Scholar]
- Lux H. D., Carbone E., Zucker H. Na+ currents through low-voltage-activated Ca2+ channels of chick sensory neurones: block by external Ca2+ and Mg2+. J Physiol. 1990 Nov;430:159–188. doi: 10.1113/jphysiol.1990.sp018287. [DOI] [PMC free article] [PubMed] [Google Scholar]
- Läuger P. Diffusion-limited ion flow through pores. Biochim Biophys Acta. 1976 Dec 2;455(2):493–509. doi: 10.1016/0005-2736(76)90320-5. [DOI] [PubMed] [Google Scholar]
- McDonald T. F., Cavalié A., Trautwein W., Pelzer D. Voltage-dependent properties of macroscopic and elementary calcium channel currents in guinea pig ventricular myocytes. Pflugers Arch. 1986 May;406(5):437–448. doi: 10.1007/BF00583365. [DOI] [PubMed] [Google Scholar]
- Neher E., Steinbach J. H. Local anaesthetics transiently block currents through single acetylcholine-receptor channels. J Physiol. 1978 Apr;277:153–176. doi: 10.1113/jphysiol.1978.sp012267. [DOI] [PMC free article] [PubMed] [Google Scholar]
- Pietrobon D., Hess P. Novel mechanism of voltage-dependent gating in L-type calcium channels. Nature. 1990 Aug 16;346(6285):651–655. doi: 10.1038/346651a0. [DOI] [PubMed] [Google Scholar]
- Pietrobon D., Prod'hom B., Hess P. Interactions of protons with single open L-type calcium channels. pH dependence of proton-induced current fluctuations with Cs+, K+, and Na+ as permeant ions. J Gen Physiol. 1989 Jul;94(1):1–21. doi: 10.1085/jgp.94.1.1. [DOI] [PMC free article] [PubMed] [Google Scholar]
- Rosenberg R. L., Chen X. H. Characterization and localization of two ion-binding sites within the pore of cardiac L-type calcium channels. J Gen Physiol. 1991 Jun;97(6):1207–1225. doi: 10.1085/jgp.97.6.1207. [DOI] [PMC free article] [PubMed] [Google Scholar]
- Sigworth F. J., Sine S. M. Data transformations for improved display and fitting of single-channel dwell time histograms. Biophys J. 1987 Dec;52(6):1047–1054. doi: 10.1016/S0006-3495(87)83298-8. [DOI] [PMC free article] [PubMed] [Google Scholar]
- Tsien R. W., Hess P., McCleskey E. W., Rosenberg R. L. Calcium channels: mechanisms of selectivity, permeation, and block. Annu Rev Biophys Biophys Chem. 1987;16:265–290. doi: 10.1146/annurev.bb.16.060187.001405. [DOI] [PubMed] [Google Scholar]
- Woodhull A. M. Ionic blockage of sodium channels in nerve. J Gen Physiol. 1973 Jun;61(6):687–708. doi: 10.1085/jgp.61.6.687. [DOI] [PMC free article] [PubMed] [Google Scholar]
- Yellen G. Relief of Na+ block of Ca2+-activated K+ channels by external cations. J Gen Physiol. 1984 Aug;84(2):187–199. doi: 10.1085/jgp.84.2.187. [DOI] [PMC free article] [PubMed] [Google Scholar]
- Yue D. T., Marban E. Permeation in the dihydropyridine-sensitive calcium channel. Multi-ion occupancy but no anomalous mole-fraction effect between Ba2+ and Ca2+. J Gen Physiol. 1990 May;95(5):911–939. doi: 10.1085/jgp.95.5.911. [DOI] [PMC free article] [PubMed] [Google Scholar]