Abstract
1. Three to eight micromolar external Mg2+ produces discrete block of the unitary inward currents through the L-type Ca2+ channel carried by 300 mM external Li+. Like the Ca2+ block, increasing Li+ concentration decreases the Mg2+ on-rate and increases the Mg2+ off-rate. 2. These kinetic changes are saturating and the apparent dissociation constant (Kd) for the on-rates in 75 mM Li+ (in activity), the same as that in the case of Ca2+ block. This suggests that Mg2+ and Ca2+ produce the discrete block at the same site. The apparent Kd for the off-rates is 300 mM, much smaller than that in the case of Ca2+ block. This indicates that Mg2+ exerts much less repulsion on the Li+ ion in the neighbouring (enhancement) site than Ca2+, although Mg2+ and Ca2+ both have two charges. The theoretical fits to the off-rates also suggest that Mg2+ can exit the blocking sites at a rate of several hundred per second in the absence of any enhancement effect. 3. Seventeen to forty-eight micromolar internal Mg2+ produces discrete block of the outward unitary currents carried by 300 mM internal Li+. The off-rates are in general approximately 20 times faster as compared to the Mg2+ off-rates in the inward currents. This finding suggests that Mg2+ in the high-affinity sites can much more easily exit to the outside than to the inside, implying significantly higher energy barriers on the inner side of the high-affinity sites for Mg2+. 4. At least 5-10 mM internal Mg2+ is needed to produce discrete block of the inward unitary currents carried by 215 mM external Na+. The off-rates in such experiments are generally the same as those in the case of external Mg2+ block of inward currents. This suggests that internal and external Mg2+ both reach the same site, namely the high-affinity Ca2+ binding sites in the pore, to produce the discrete block. 5. Other than discrete block, 5-10 mM internal Mg2+ also decreases the size of the inward unitary current. This is most probably due to a fast block at the more internally located low-affinity sites in the pore. The fractional decrease of the currents is voltage dependent and can be fitted by a rectangular hyperbola to calculate the apparent Kd, which increases e-fold per 45 mV hyperpolarization, indicating an electrical distance of 0.3 between the low-affinity sites and the internal pore mouth.(ABSTRACT TRUNCATED AT 400 WORDS)
Full text
PDF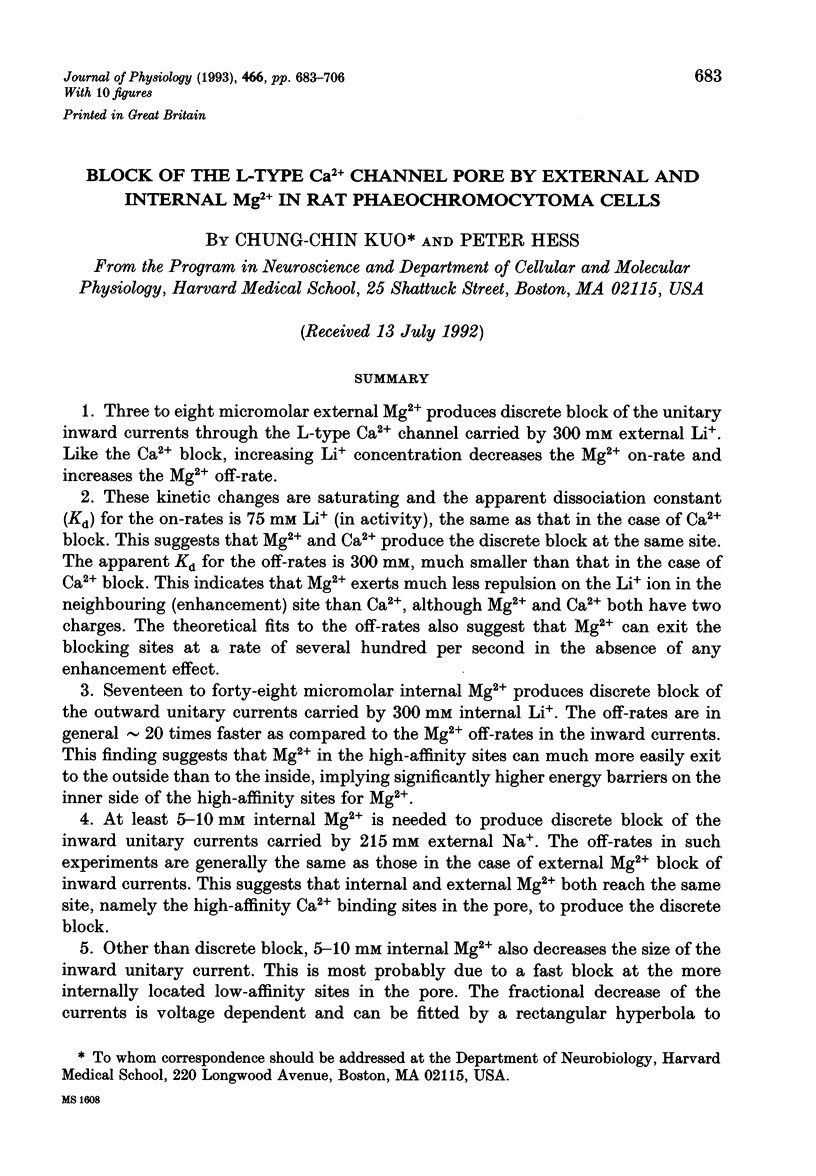
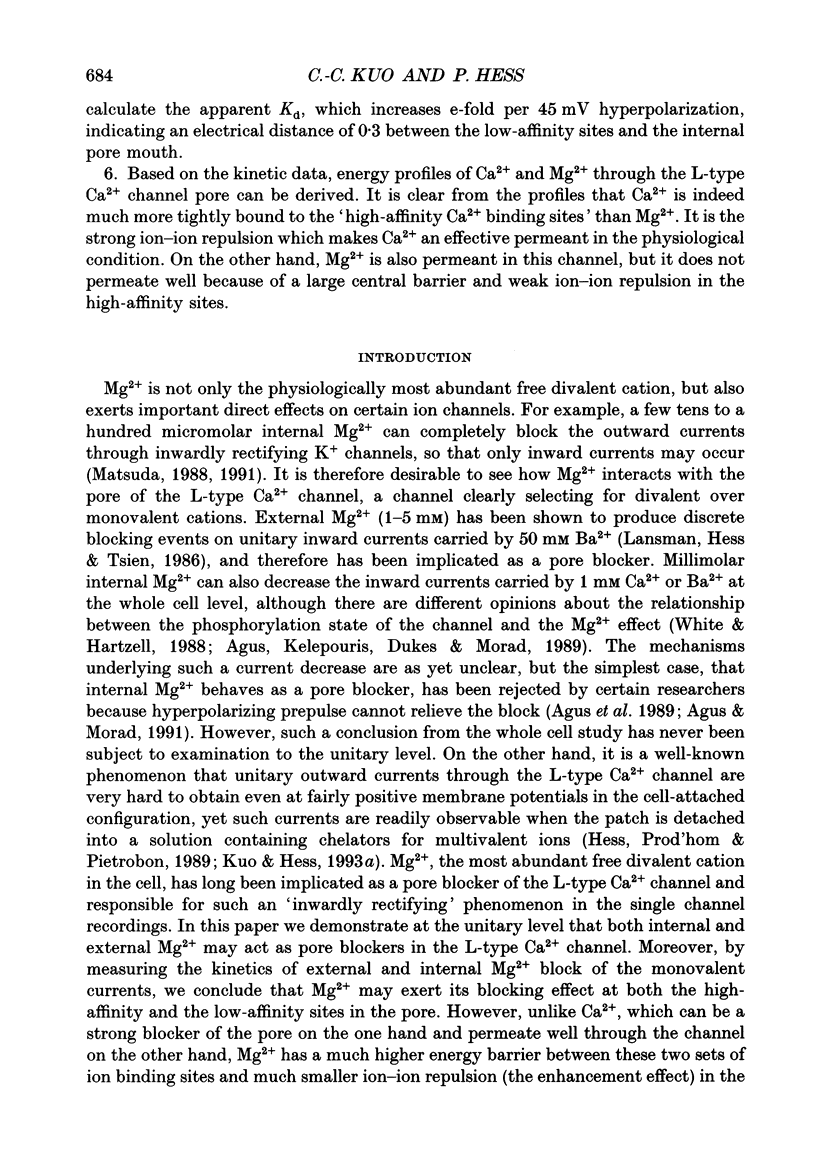
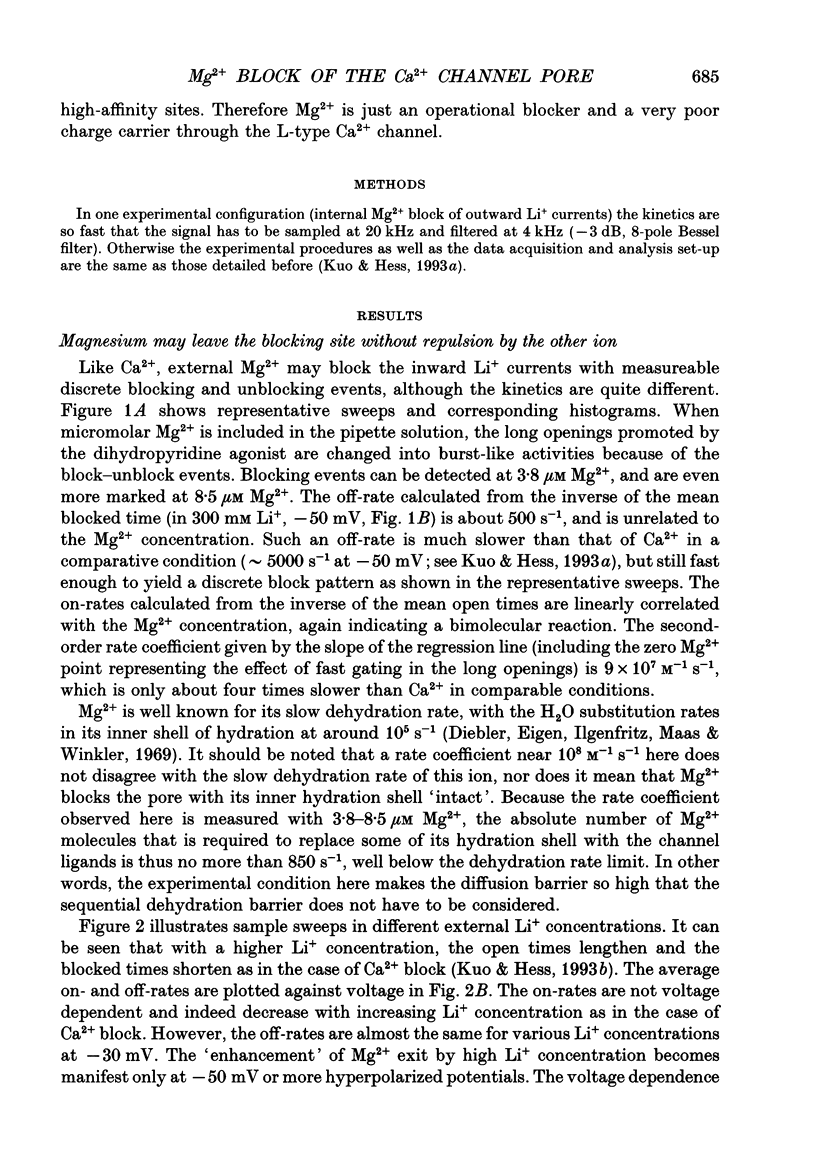
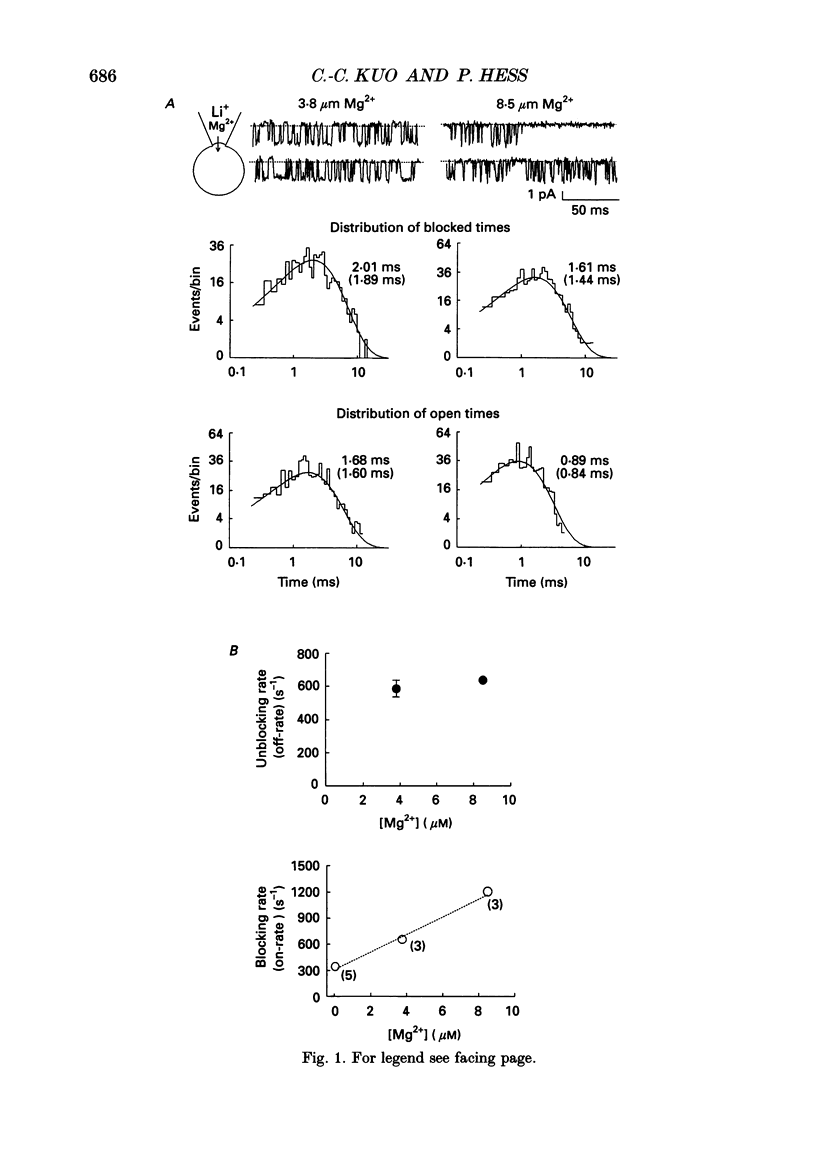
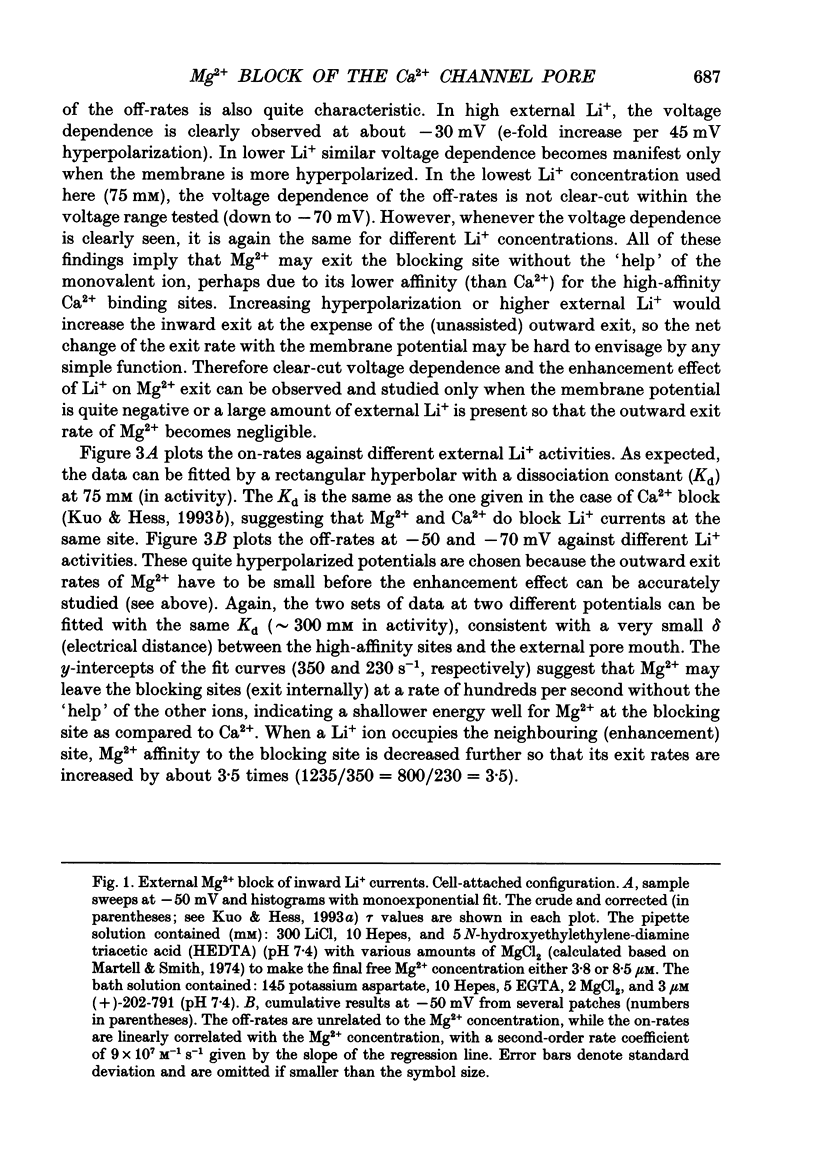
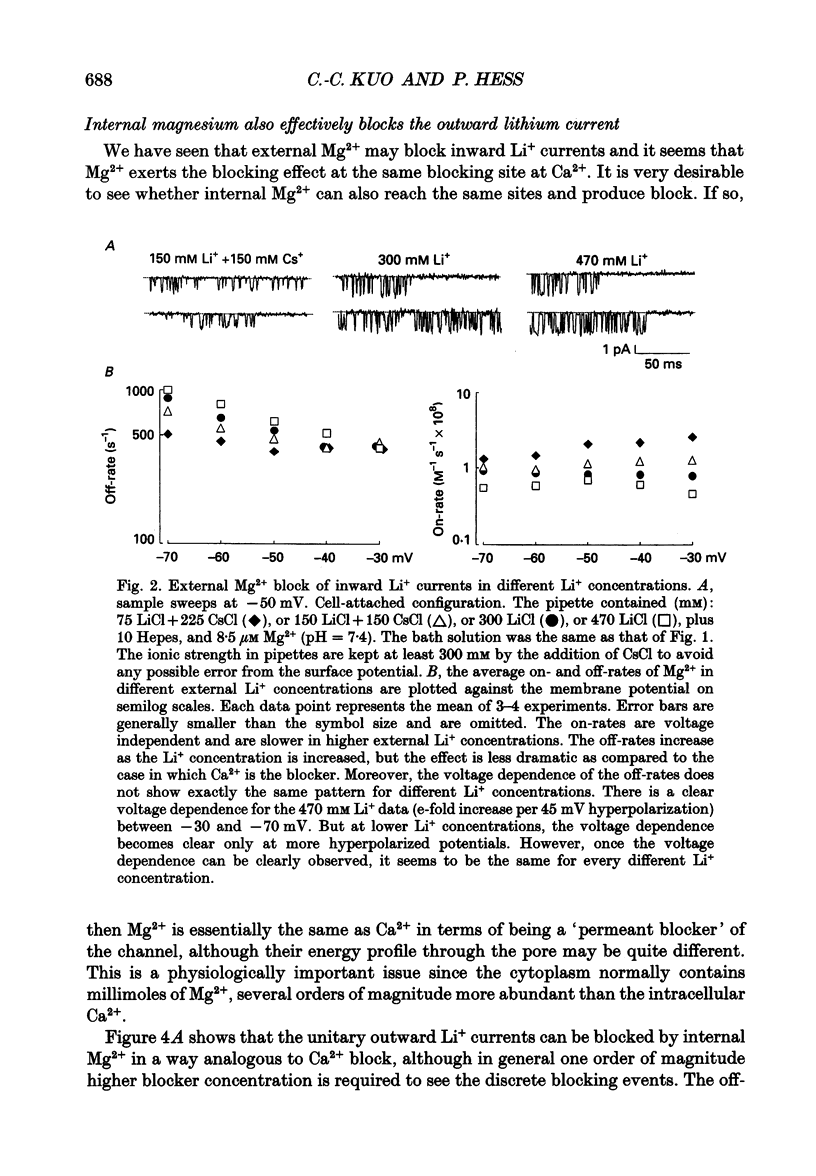
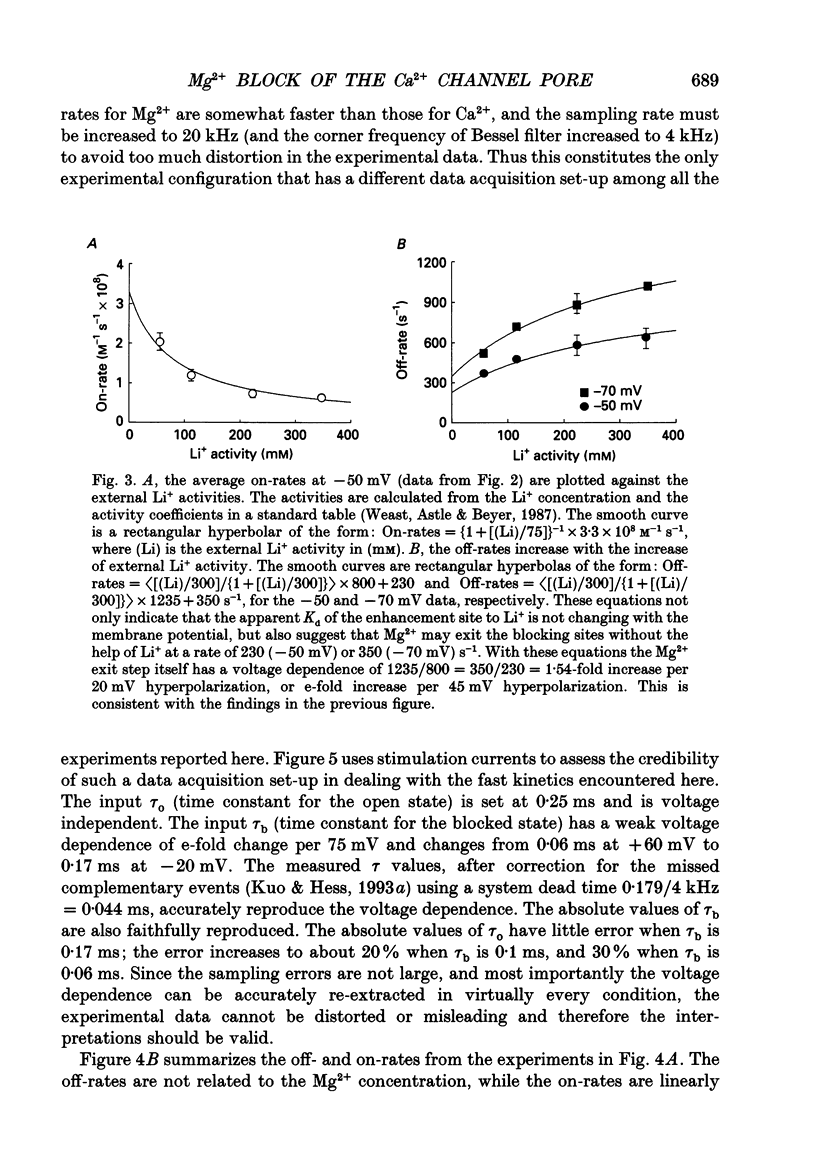
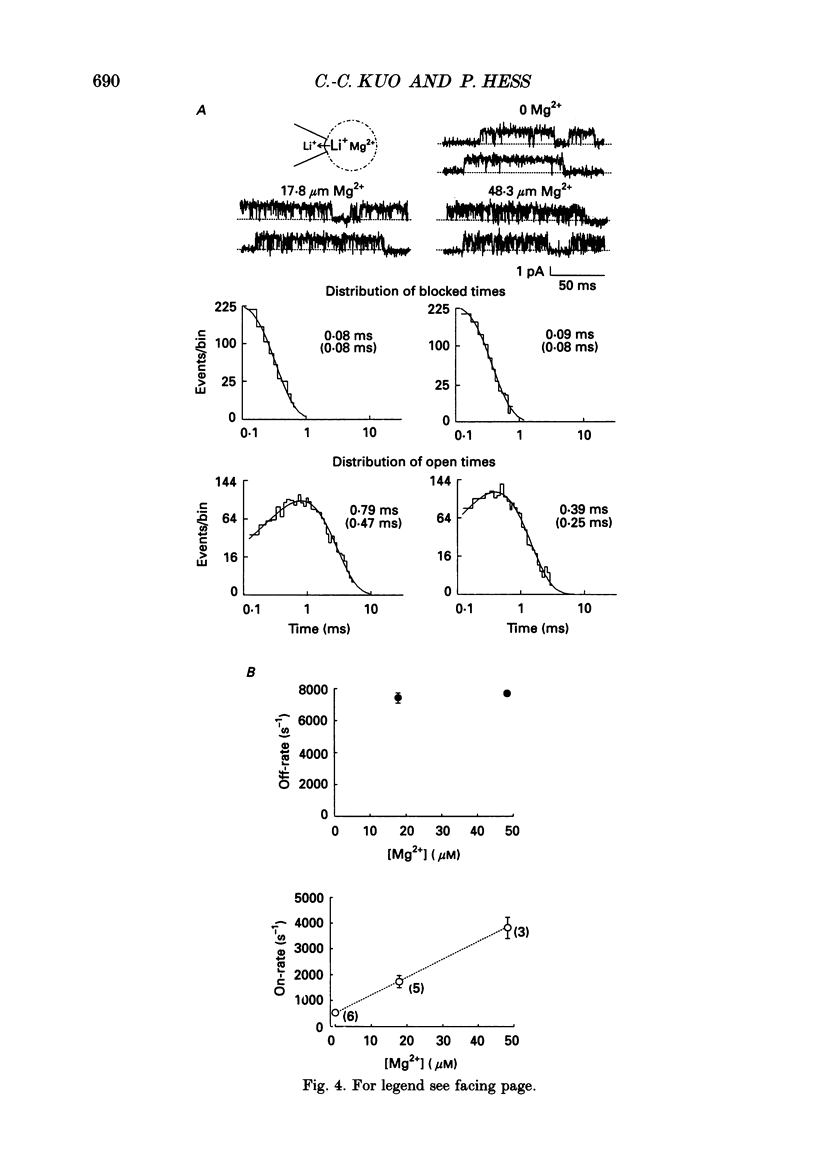
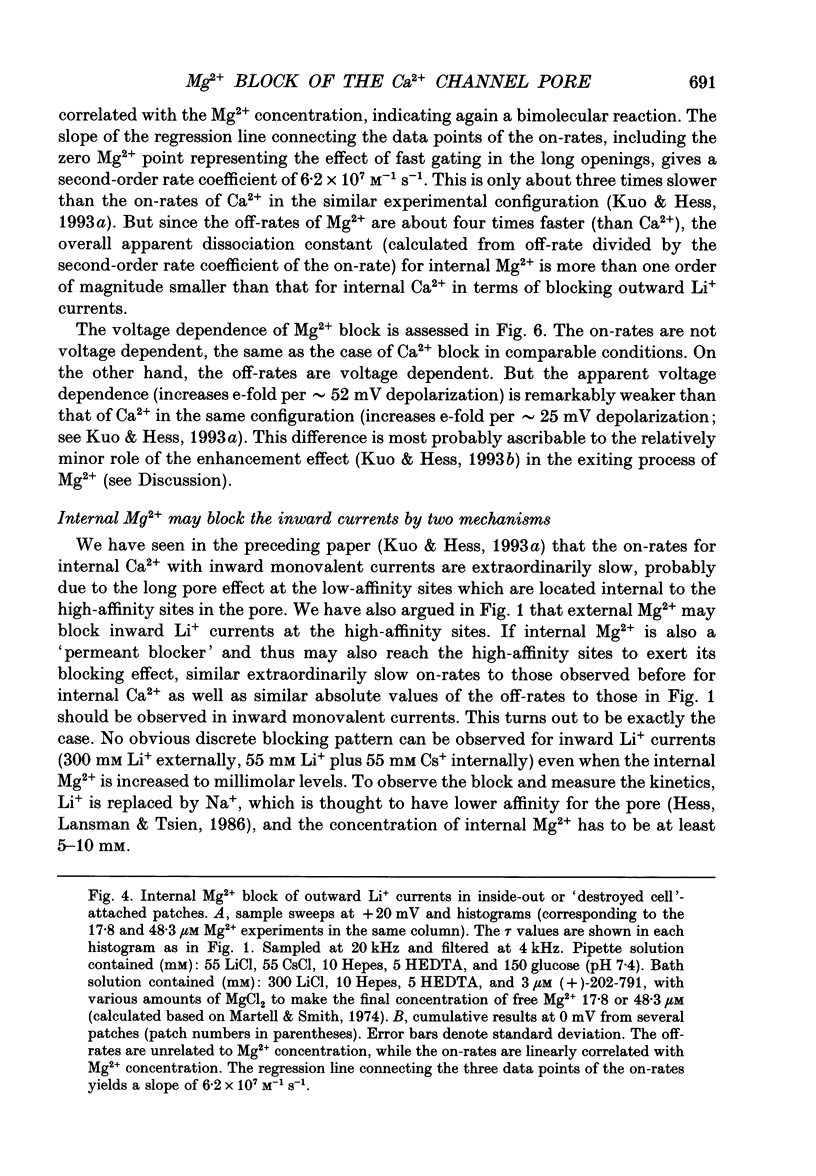
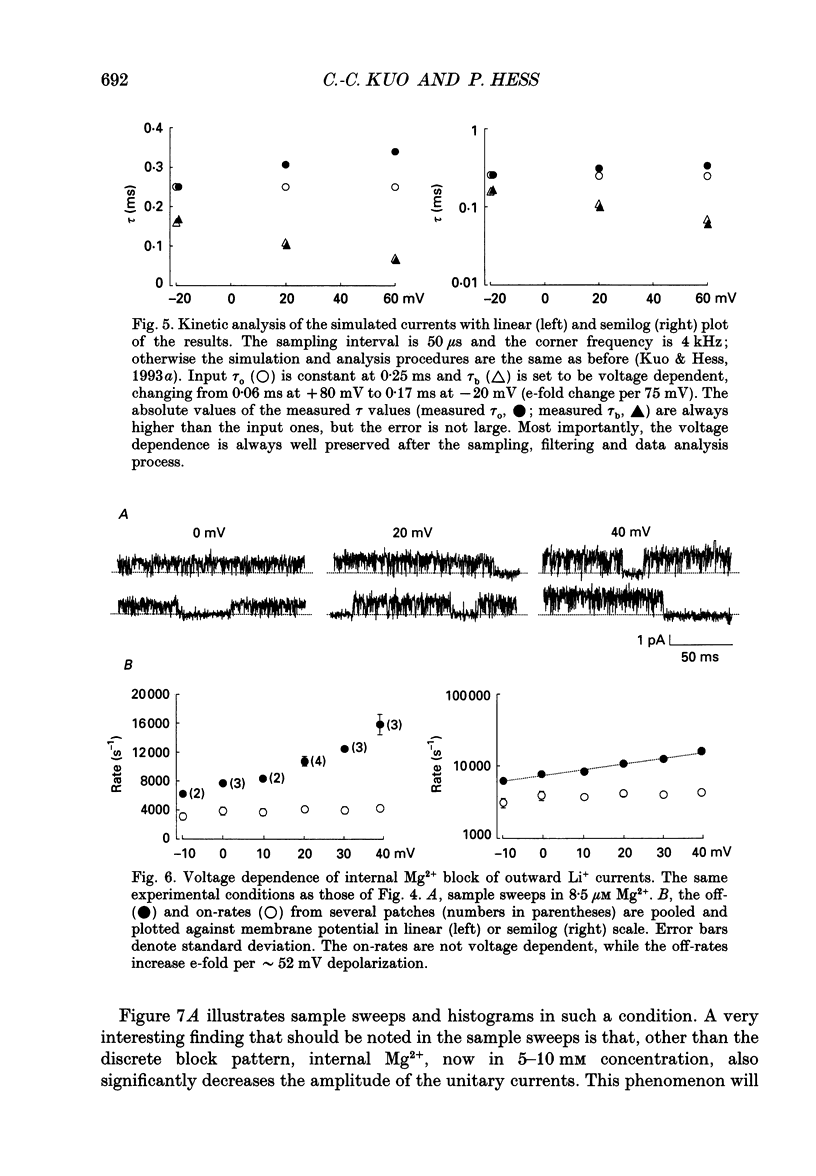
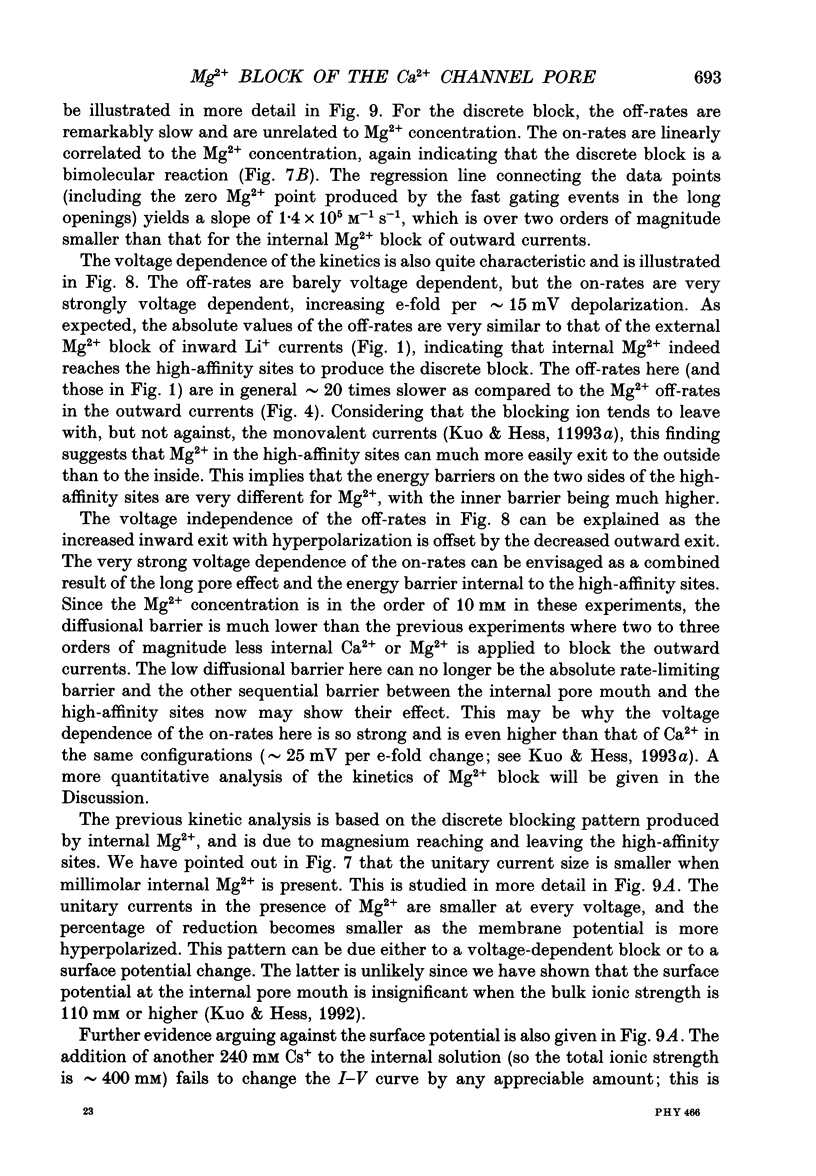
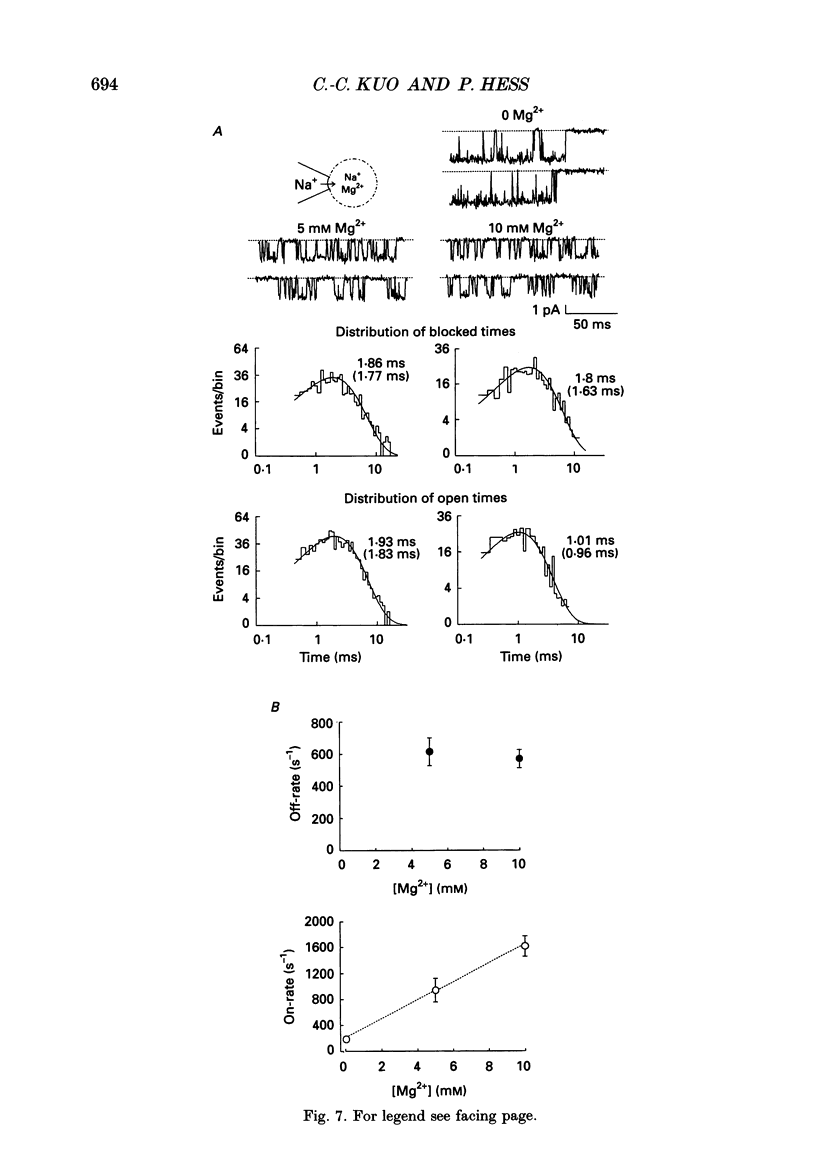
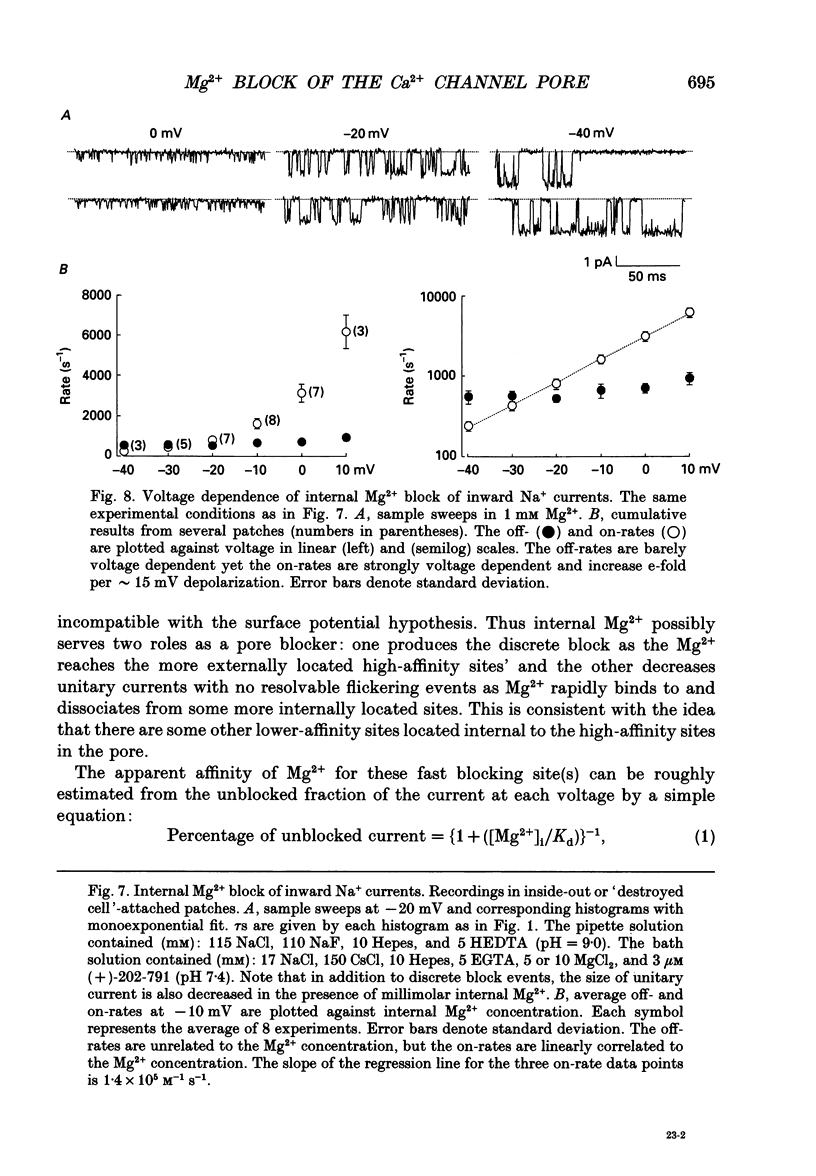
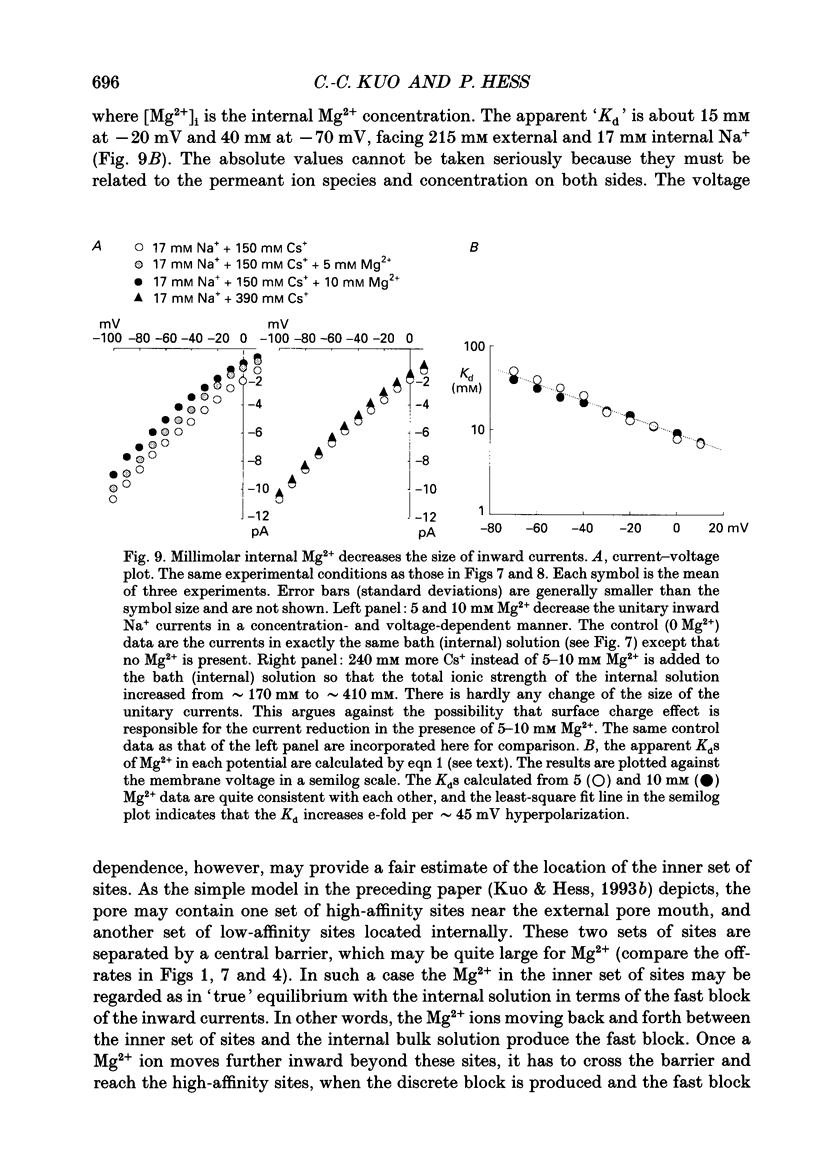
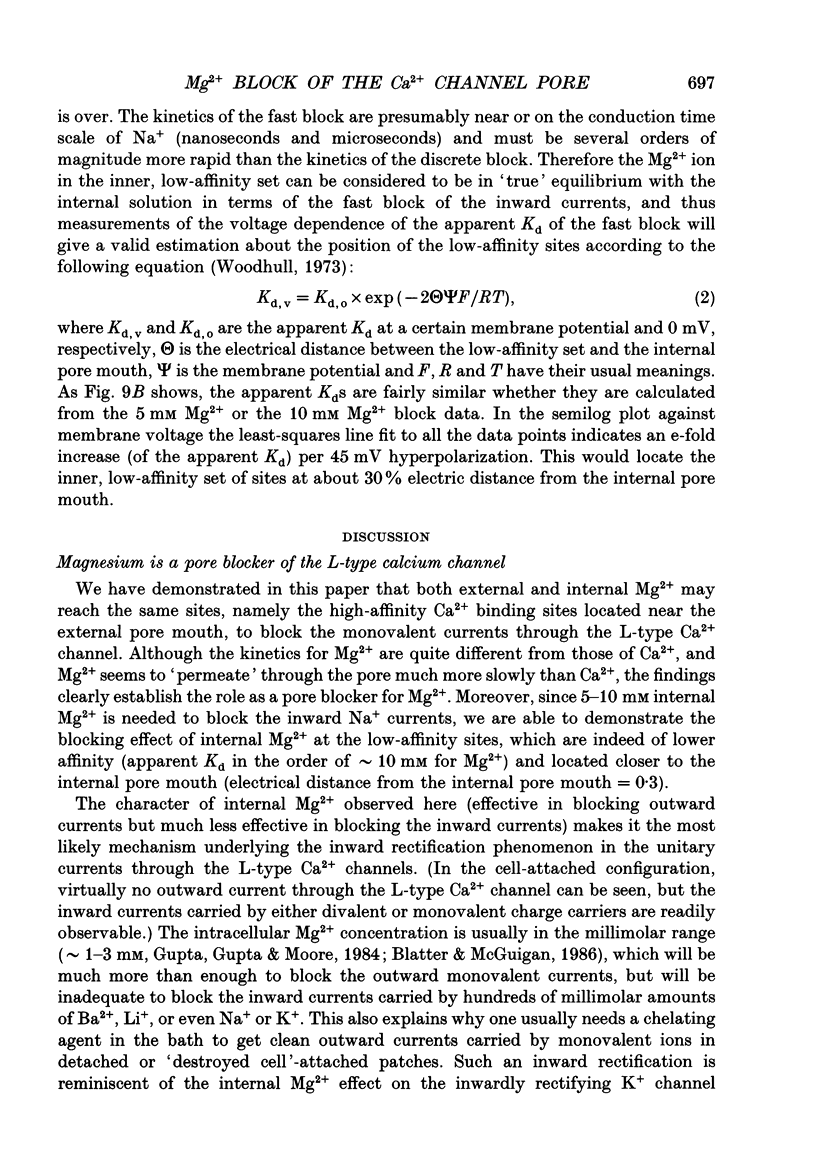
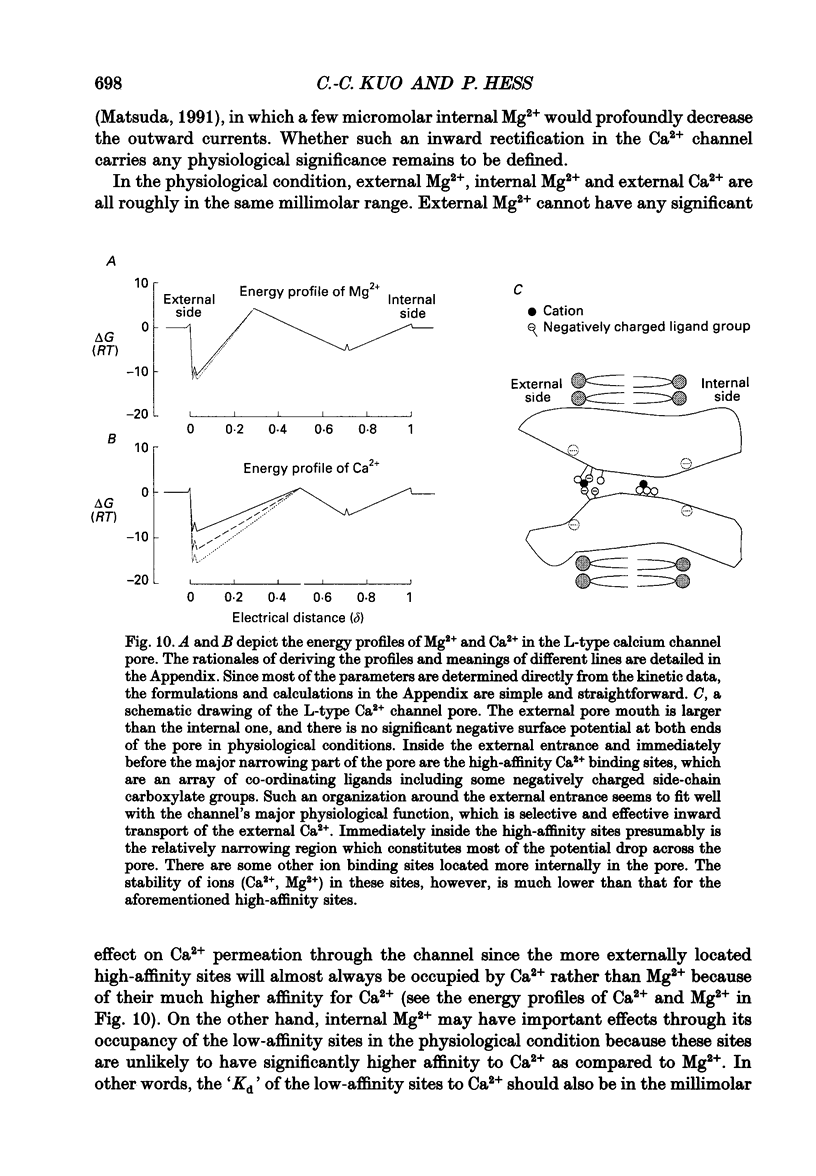
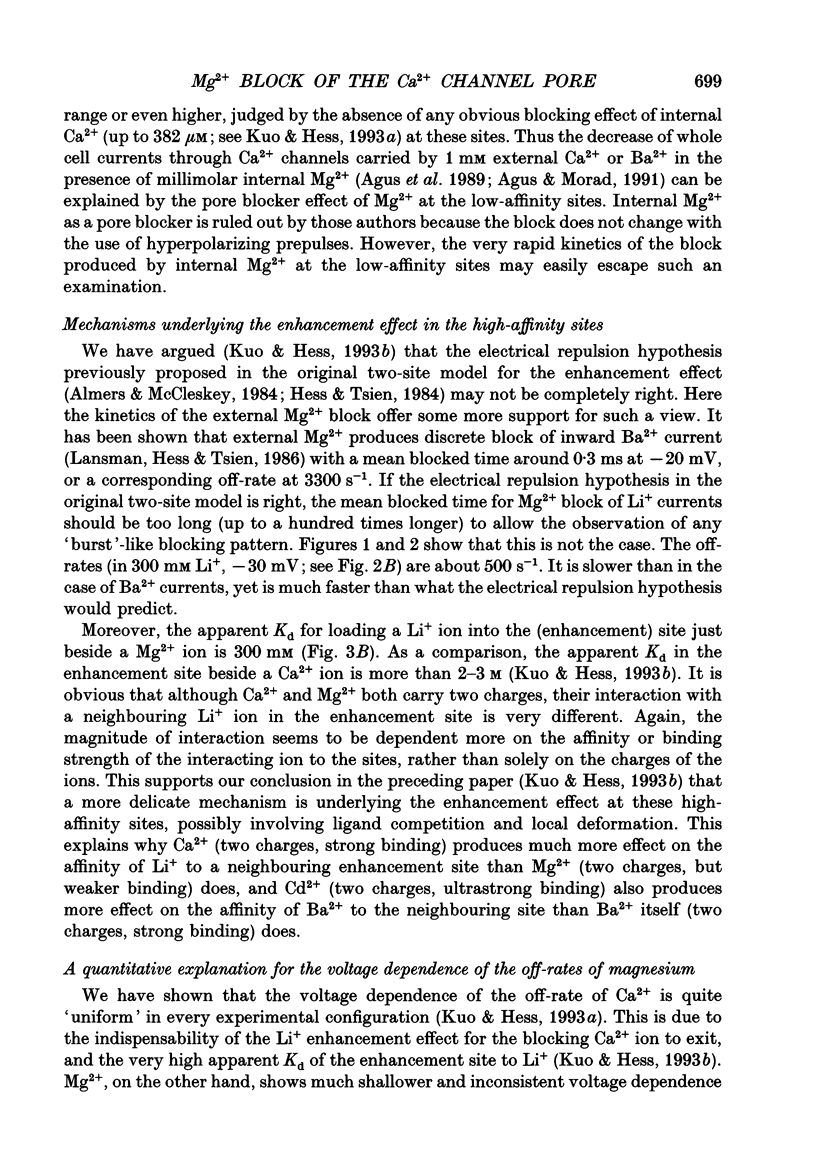
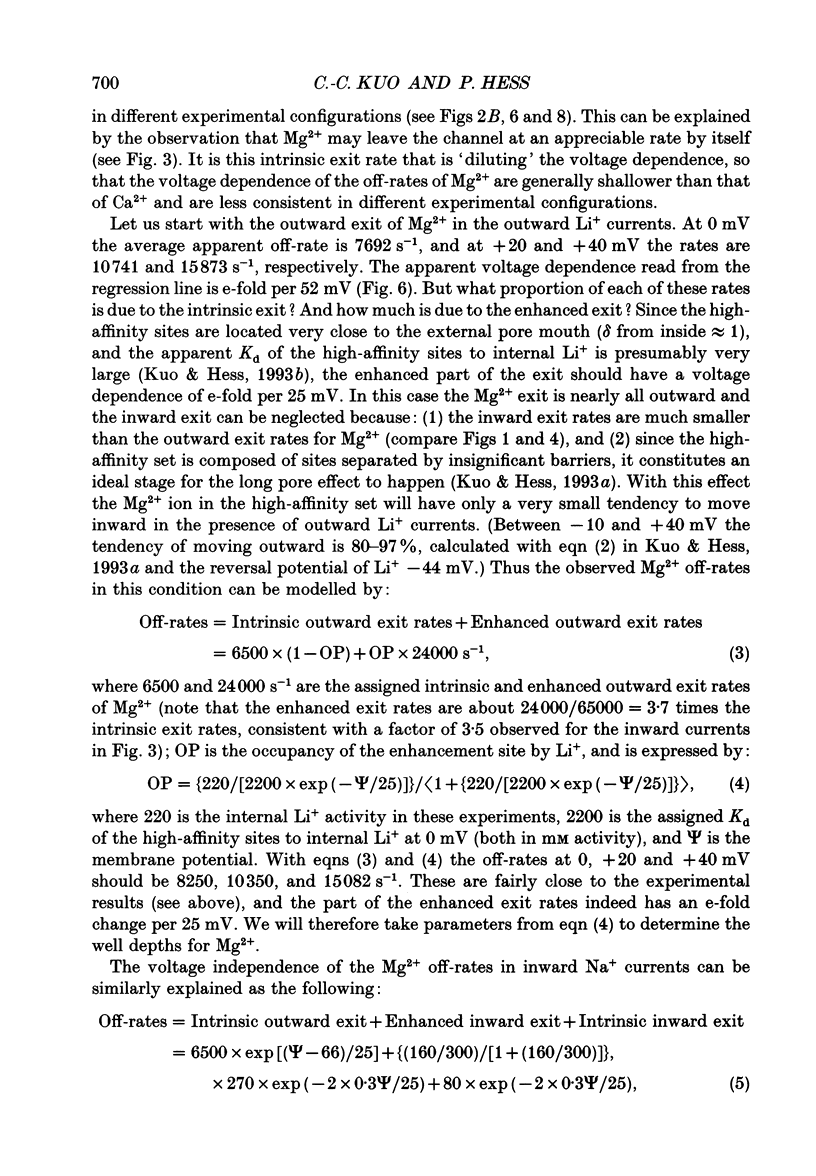
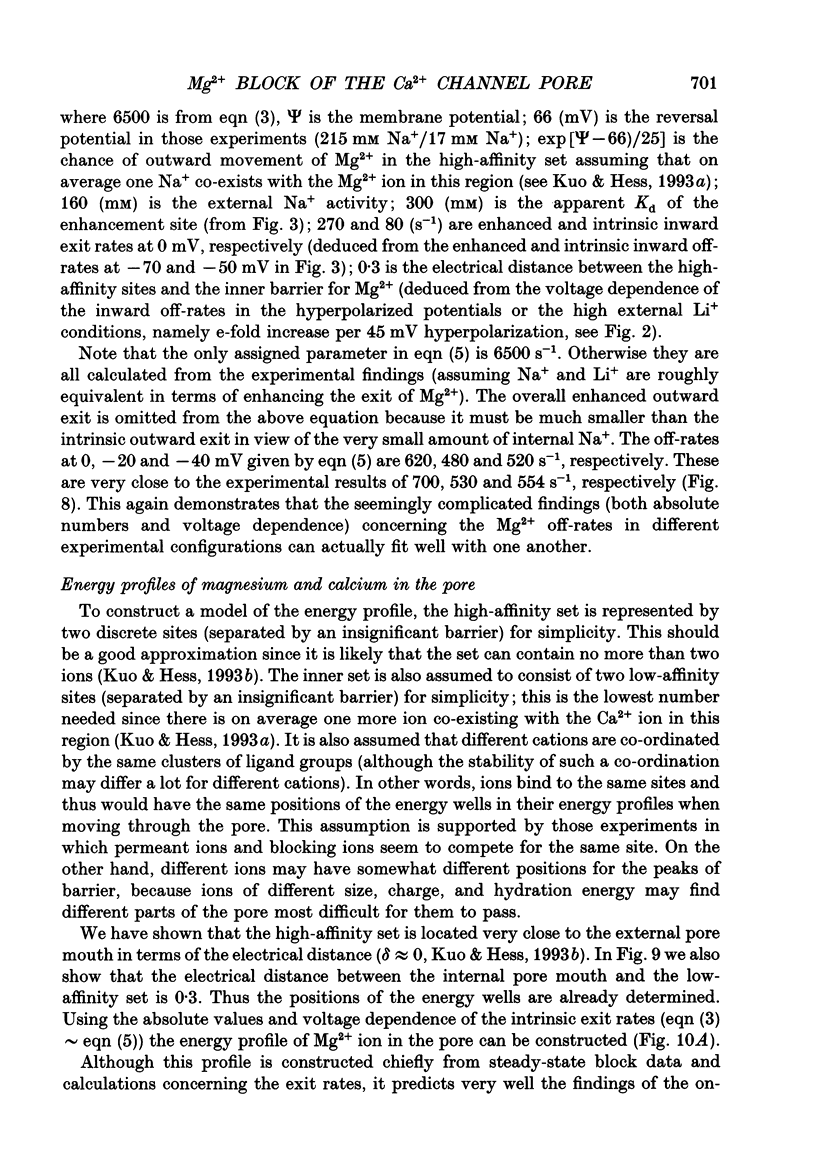
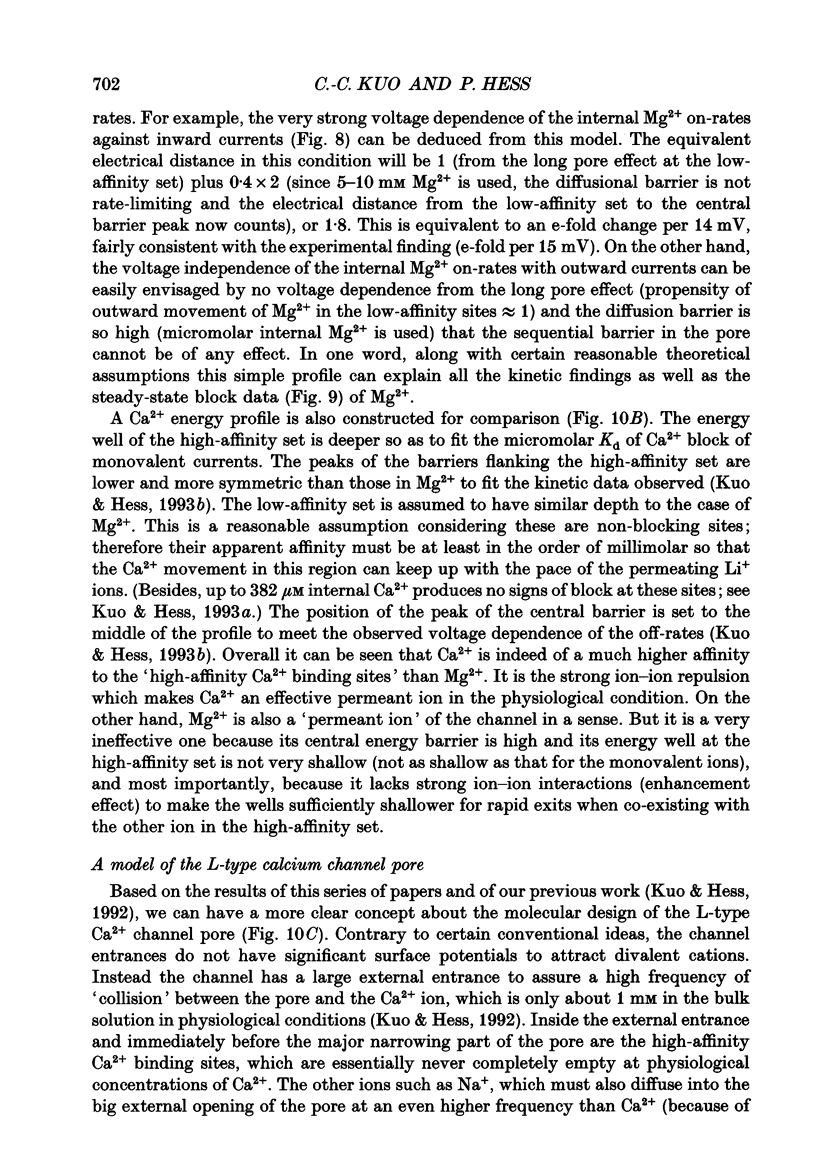
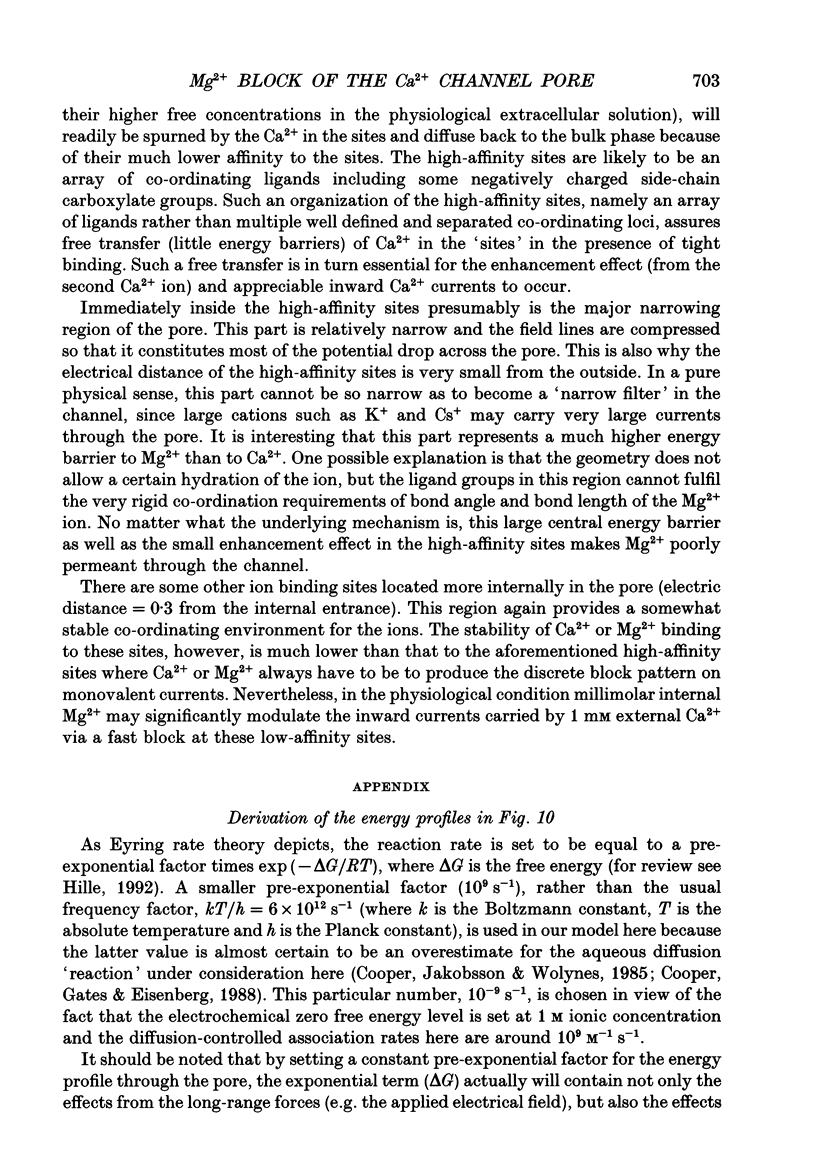
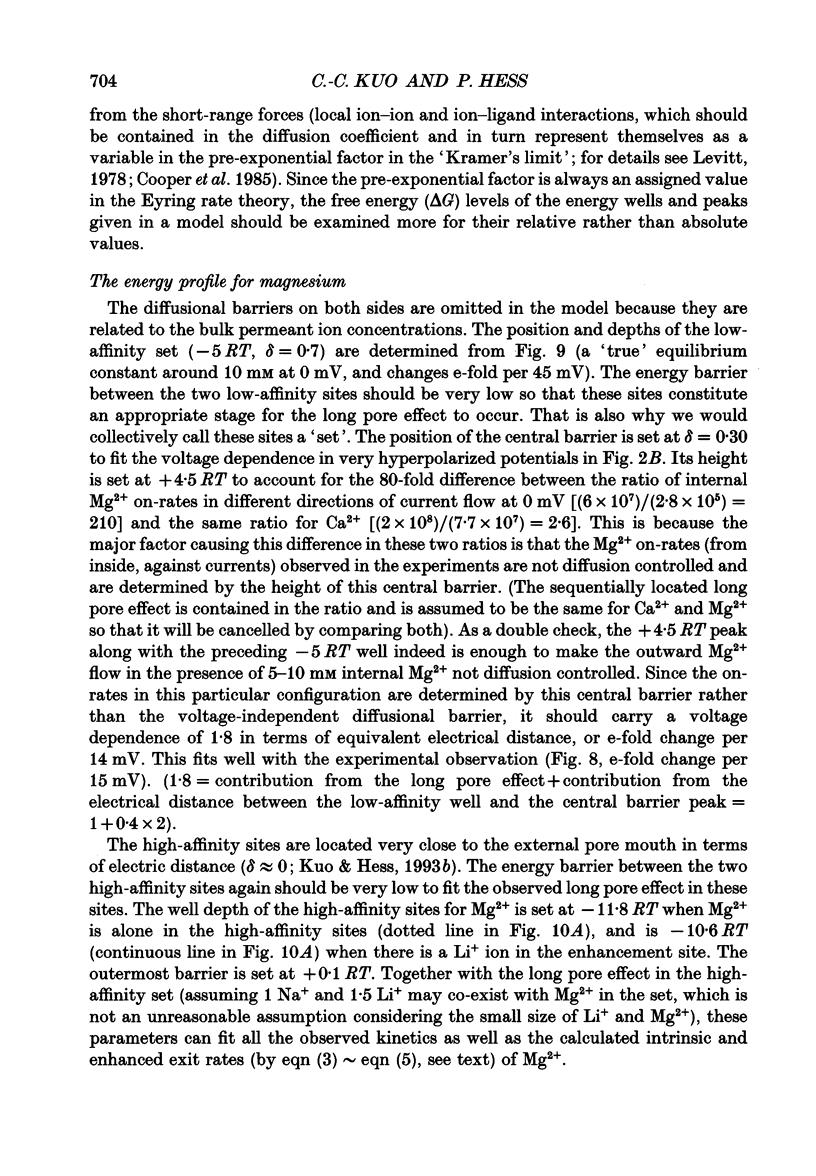
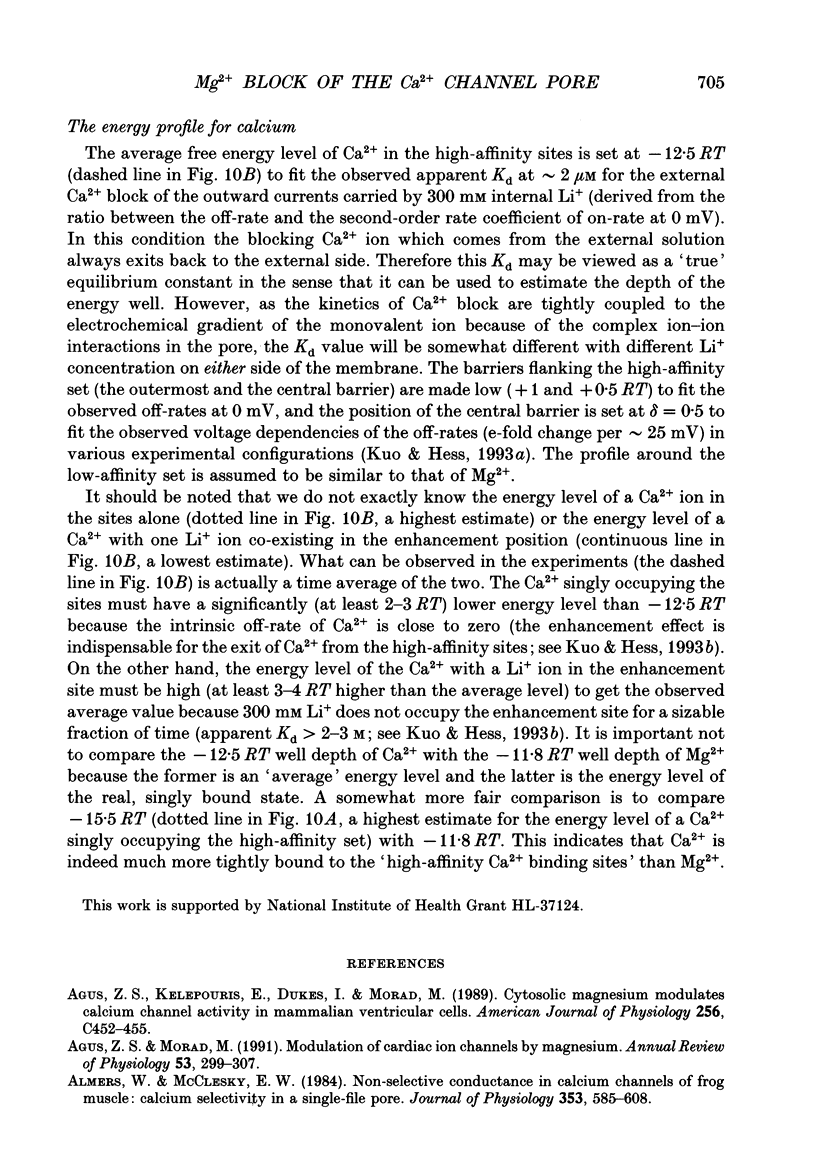
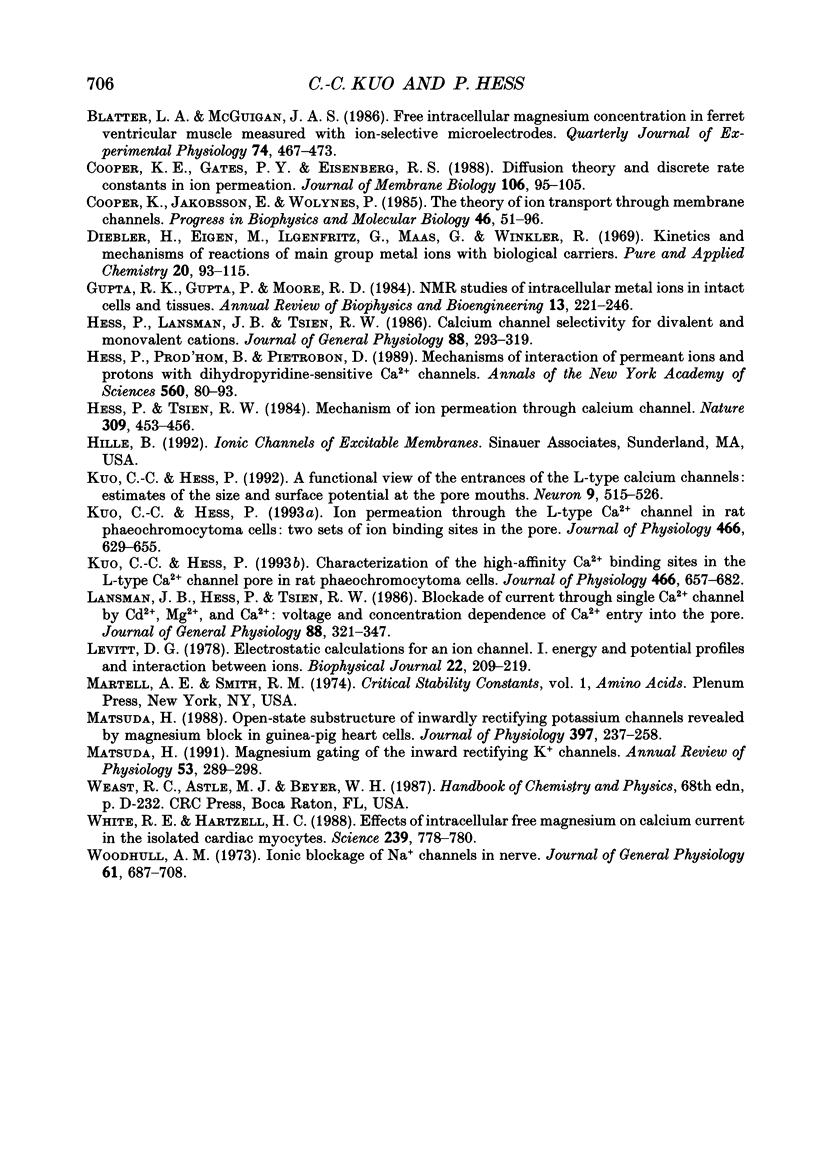
Selected References
These references are in PubMed. This may not be the complete list of references from this article.
- Agus Z. S., Kelepouris E., Dukes I., Morad M. Cytosolic magnesium modulates calcium channel activity in mammalian ventricular cells. Am J Physiol. 1989 Feb;256(2 Pt 1):C452–C455. doi: 10.1152/ajpcell.1989.256.2.C452. [DOI] [PubMed] [Google Scholar]
- Agus Z. S., Morad M. Modulation of cardiac ion channels by magnesium. Annu Rev Physiol. 1991;53:299–307. doi: 10.1146/annurev.ph.53.030191.001503. [DOI] [PubMed] [Google Scholar]
- Almers W., McCleskey E. W. Non-selective conductance in calcium channels of frog muscle: calcium selectivity in a single-file pore. J Physiol. 1984 Aug;353:585–608. doi: 10.1113/jphysiol.1984.sp015352. [DOI] [PMC free article] [PubMed] [Google Scholar]
- Blatter L. A., McGuigan J. A. Free intracellular magnesium concentration in ferret ventricular muscle measured with ion selective micro-electrodes. Q J Exp Physiol. 1986 Jul;71(3):467–473. doi: 10.1113/expphysiol.1986.sp003005. [DOI] [PubMed] [Google Scholar]
- Cooper K. E., Gates P. Y., Eisenberg R. S. Diffusion theory and discrete rate constants in ion permeation. J Membr Biol. 1988 Dec;106(2):95–105. doi: 10.1007/BF01871391. [DOI] [PubMed] [Google Scholar]
- Cooper K., Jakobsson E., Wolynes P. The theory of ion transport through membrane channels. Prog Biophys Mol Biol. 1985;46(1):51–96. doi: 10.1016/0079-6107(85)90012-4. [DOI] [PubMed] [Google Scholar]
- Gupta R. K., Gupta P., Moore R. D. NMR studies of intracellular metal ions in intact cells and tissues. Annu Rev Biophys Bioeng. 1984;13:221–246. doi: 10.1146/annurev.bb.13.060184.001253. [DOI] [PubMed] [Google Scholar]
- Hess P., Lansman J. B., Tsien R. W. Calcium channel selectivity for divalent and monovalent cations. Voltage and concentration dependence of single channel current in ventricular heart cells. J Gen Physiol. 1986 Sep;88(3):293–319. doi: 10.1085/jgp.88.3.293. [DOI] [PMC free article] [PubMed] [Google Scholar]
- Hess P., Prod'Hom B., Pietrobon D. Mechanisms of interaction of permeant ions and protons with dihydropyridine-sensitive calcium channels. Ann N Y Acad Sci. 1989;560:80–93. doi: 10.1111/j.1749-6632.1989.tb24082.x. [DOI] [PubMed] [Google Scholar]
- Hess P., Tsien R. W. Mechanism of ion permeation through calcium channels. 1984 May 31-Jun 6Nature. 309(5967):453–456. doi: 10.1038/309453a0. [DOI] [PubMed] [Google Scholar]
- Kuo C. C., Hess P. A functional view of the entrances of L-type Ca2+ channels: estimates of the size and surface potential at the pore mouths. Neuron. 1992 Sep;9(3):515–526. doi: 10.1016/0896-6273(92)90189-k. [DOI] [PubMed] [Google Scholar]
- Kuo C. C., Hess P. Characterization of the high-affinity Ca2+ binding sites in the L-type Ca2+ channel pore in rat phaeochromocytoma cells. J Physiol. 1993 Jul;466:657–682. [PMC free article] [PubMed] [Google Scholar]
- Kuo C. C., Hess P. Ion permeation through the L-type Ca2+ channel in rat phaeochromocytoma cells: two sets of ion binding sites in the pore. J Physiol. 1993 Jul;466:629–655. [PMC free article] [PubMed] [Google Scholar]
- Lansman J. B., Hess P., Tsien R. W. Blockade of current through single calcium channels by Cd2+, Mg2+, and Ca2+. Voltage and concentration dependence of calcium entry into the pore. J Gen Physiol. 1986 Sep;88(3):321–347. doi: 10.1085/jgp.88.3.321. [DOI] [PMC free article] [PubMed] [Google Scholar]
- Levitt D. G. Electrostatic calculations for an ion channel. I. Energy and potential profiles and interactions between ions. Biophys J. 1978 May;22(2):209–219. doi: 10.1016/S0006-3495(78)85485-X. [DOI] [PMC free article] [PubMed] [Google Scholar]
- Matsuda H. Magnesium gating of the inwardly rectifying K+ channel. Annu Rev Physiol. 1991;53:289–298. doi: 10.1146/annurev.ph.53.030191.001445. [DOI] [PubMed] [Google Scholar]
- Matsuda H. Open-state substructure of inwardly rectifying potassium channels revealed by magnesium block in guinea-pig heart cells. J Physiol. 1988 Mar;397:237–258. doi: 10.1113/jphysiol.1988.sp016998. [DOI] [PMC free article] [PubMed] [Google Scholar]
- White R. E., Hartzell H. C. Effects of intracellular free magnesium on calcium current in isolated cardiac myocytes. Science. 1988 Feb 12;239(4841 Pt 1):778–780. doi: 10.1126/science.2448878. [DOI] [PubMed] [Google Scholar]
- Woodhull A. M. Ionic blockage of sodium channels in nerve. J Gen Physiol. 1973 Jun;61(6):687–708. doi: 10.1085/jgp.61.6.687. [DOI] [PMC free article] [PubMed] [Google Scholar]