Abstract
1. During just-suprathreshold synaptic activation of CA1 pyramidal cells in rat hippocampal slices in vitro the action potential begins as a slow depolarizing ramp, superimposed on the underlying EPSP and forming an integral part of the action potential. We call this ramp a synaptic prepotential (SyPP). 2. In order to examine the SyPP, a procedure for subtraction of the underlying EPSP was necessary. Because action potentials were only elicited by a subset of EPSPs with larger than average amplitude, a subtraction of the mean subthreshold EPSP would not give valid results. Instead, an EPSP to be subtracted was selected from an assemblage of subthreshold EPSPs, so that its amplitude matched the initial part of the spike-generating EPSP. 3. Virtually all action potentials started with a SyPP. Using an amplitude criterion of 1 S.D. of the mean of the matching subthreshold EPSPs, just-suprathreshold EPSPs gave prepotentials in 72-100% of all action potentials from fifteen randomly selected cells. With a criterion of 2 S.D.S, the frequency of occurrence ranged from 36 to 100%. 4. With a constant stimulus strength, there was a certain variability of the spike latencies. Shorter latency spikes had steeper, but smaller SyPPs than later spikes, suggesting that the slope of SyPP influenced the timing of the cell discharge. 5. The SyPP was best fitted by a single, exponentially rising curve, and was both smaller and slower than the large amplitude action potential. Its amplitude was 1-6 mV and the time constant 1-5 ms, which was 10-50 times slower than that of the upstroke of the action potential. 6. A properly timed hyperpolarizing current pulse could block the large amplitude action potential, thereby unmasking the SyPP as an initial depolarizing ramp. 7. The SyPP was more sensitive than the large amplitude action potential to intracellular injection of QX-314, a lidocaine derivative. At the concentrations used (10 or 30 mM) no detectable changes were seen in the large amplitude action potential. 8. Droplet application of a specific N-methyl-D-aspartate receptor antagonist, DL-2-amino-5-phosphonovaleric acid (1 mM), reduced both the EPSP and the firing probability, but did not change the SyPP. 9. The SyPP amplitude and time course depended upon the membrane potential at which the cell was activated. Depolarization enhanced and prolonged the SyPP, while hyperpolarization gave opposite effects. In part, the depolarization-induced amplitude increase could be attributed to membrane accommodation. 10. Antidromically evoked action potentials never started with a prepotential.(ABSTRACT TRUNCATED AT 400 WORDS)
Full text
PDF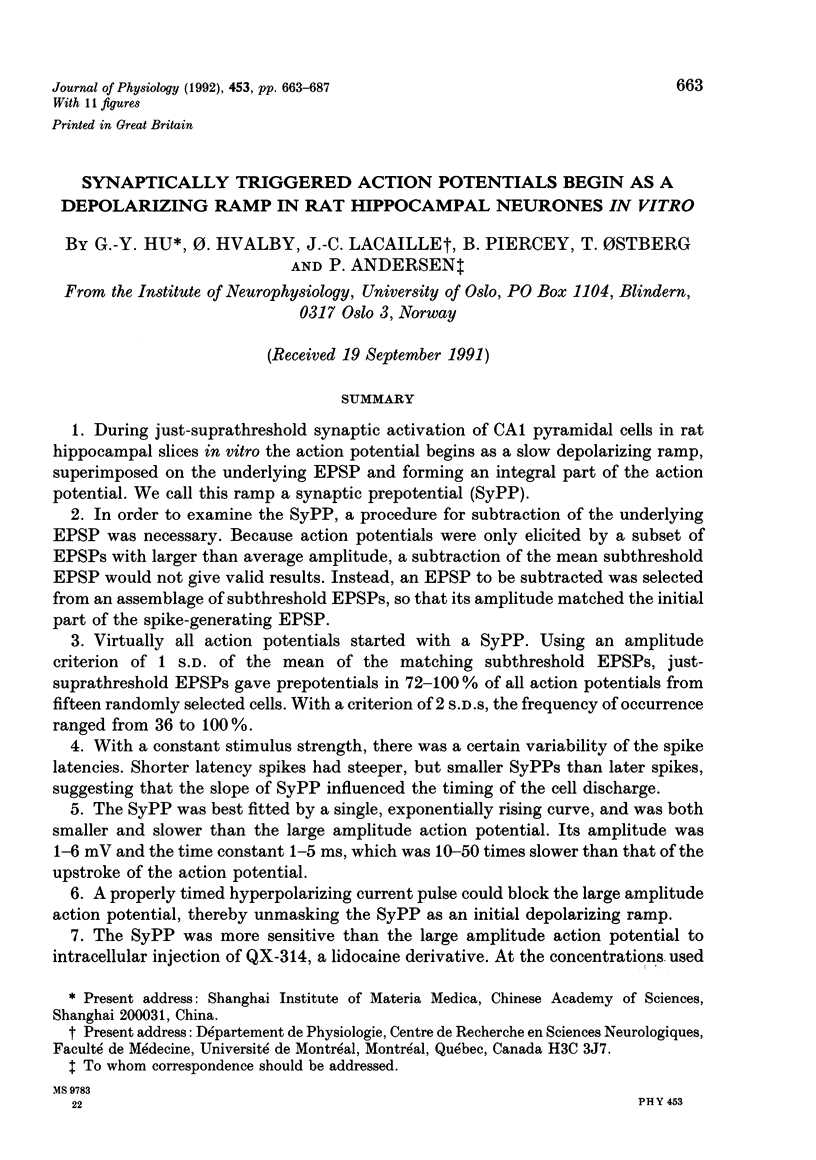
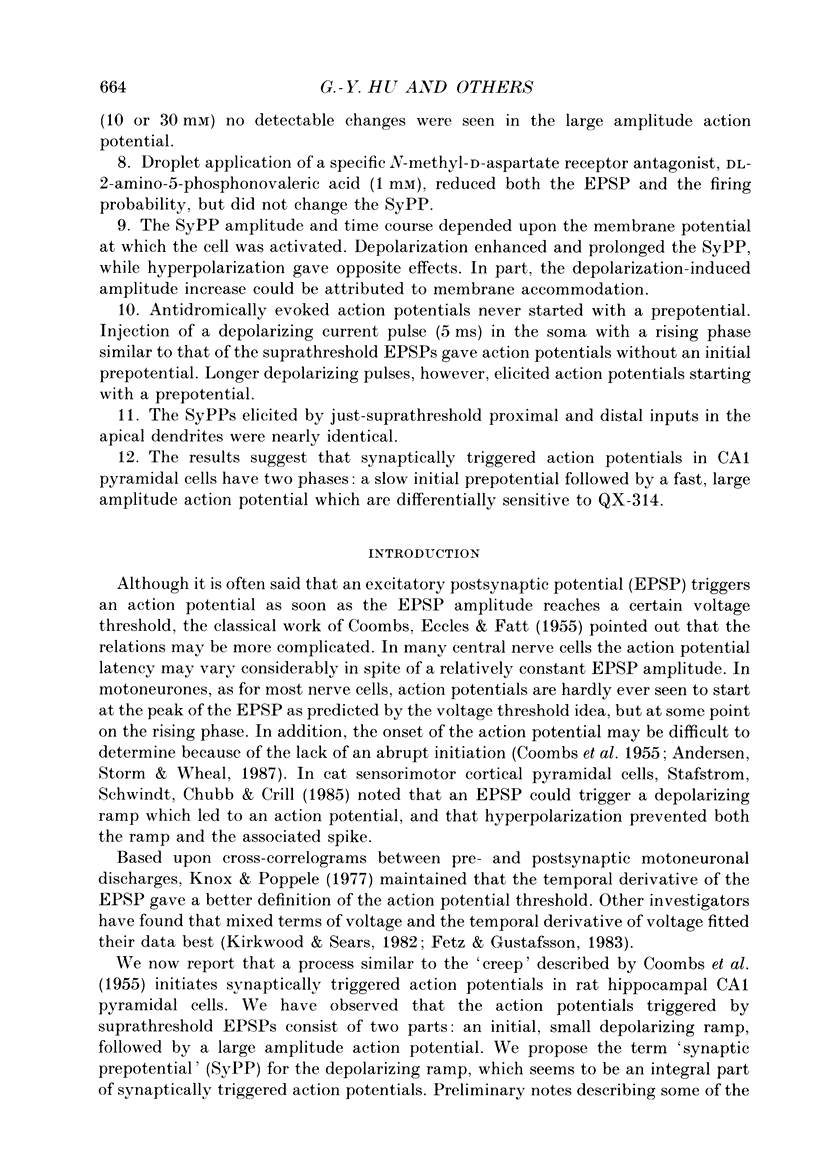
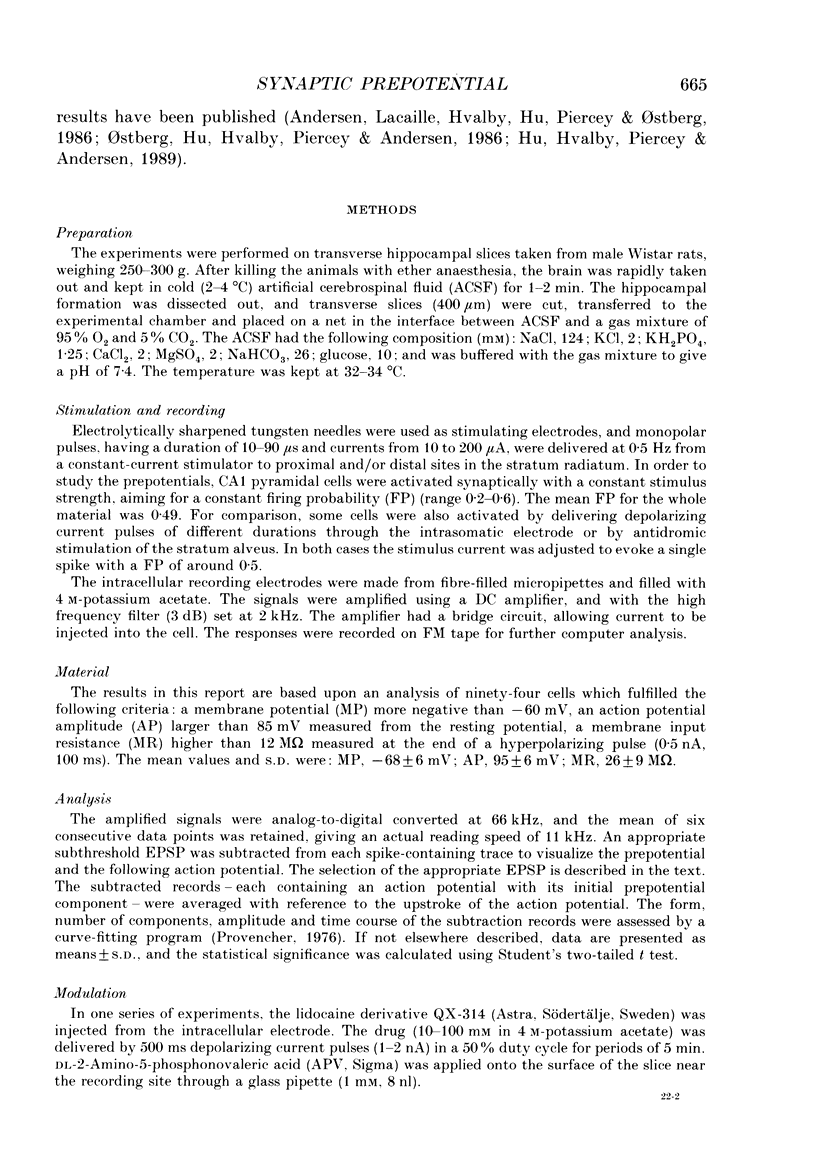
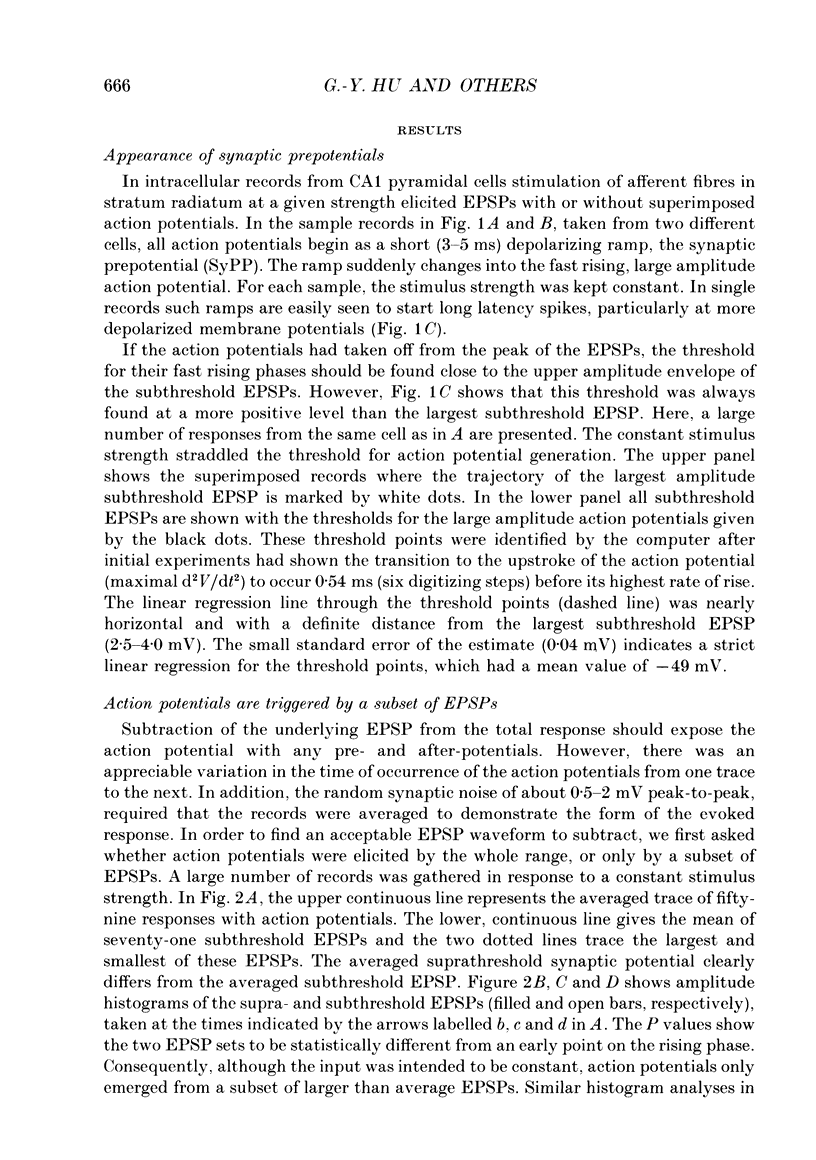
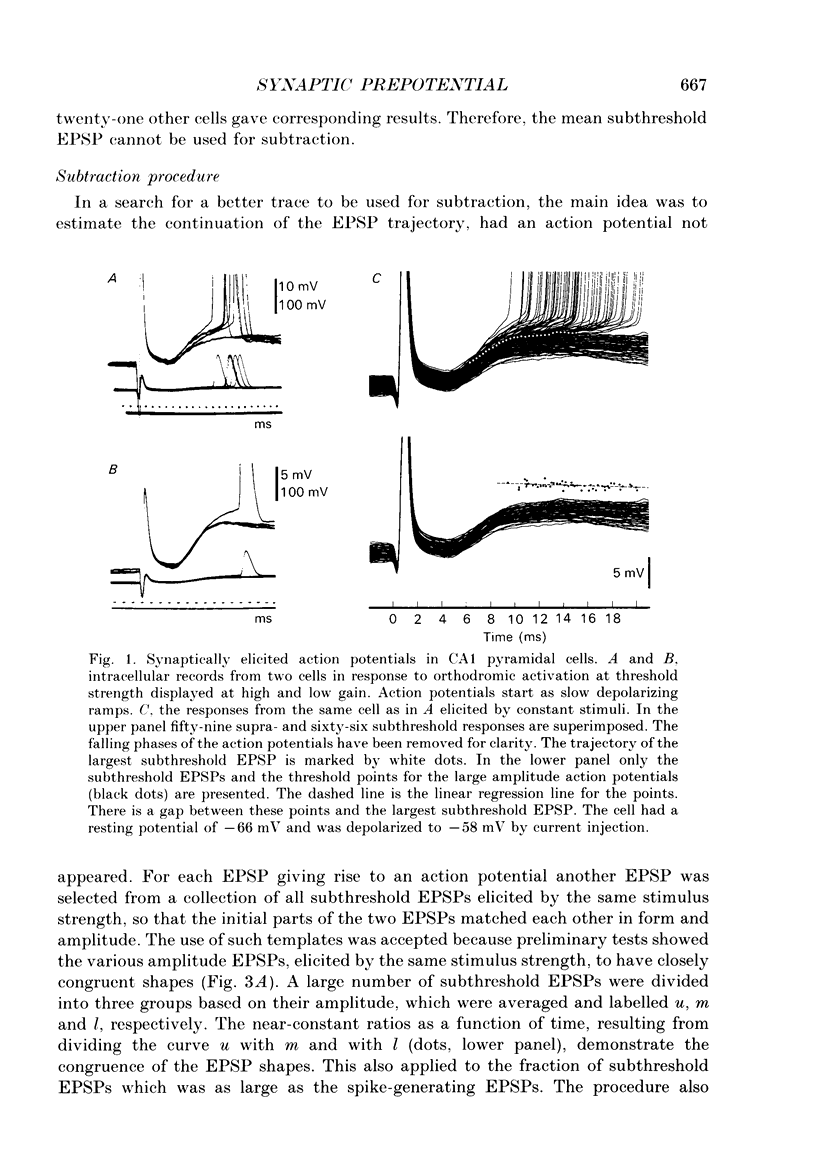
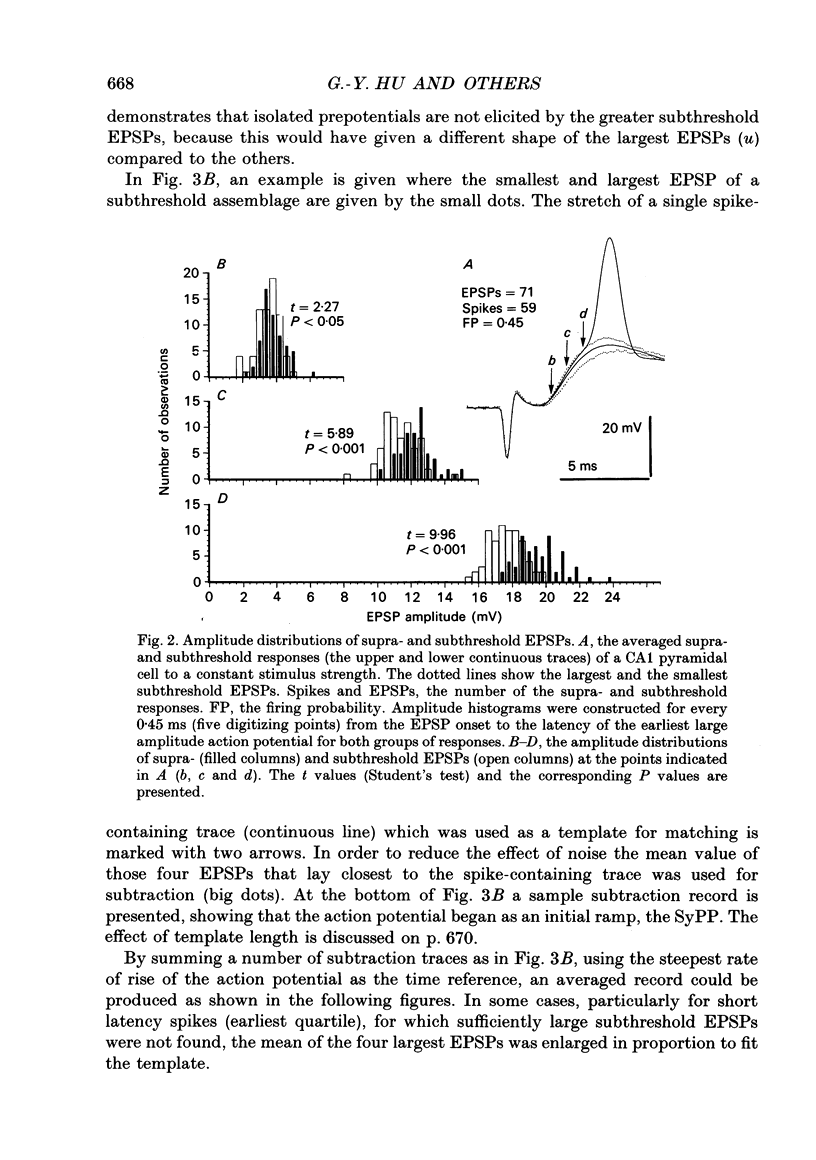
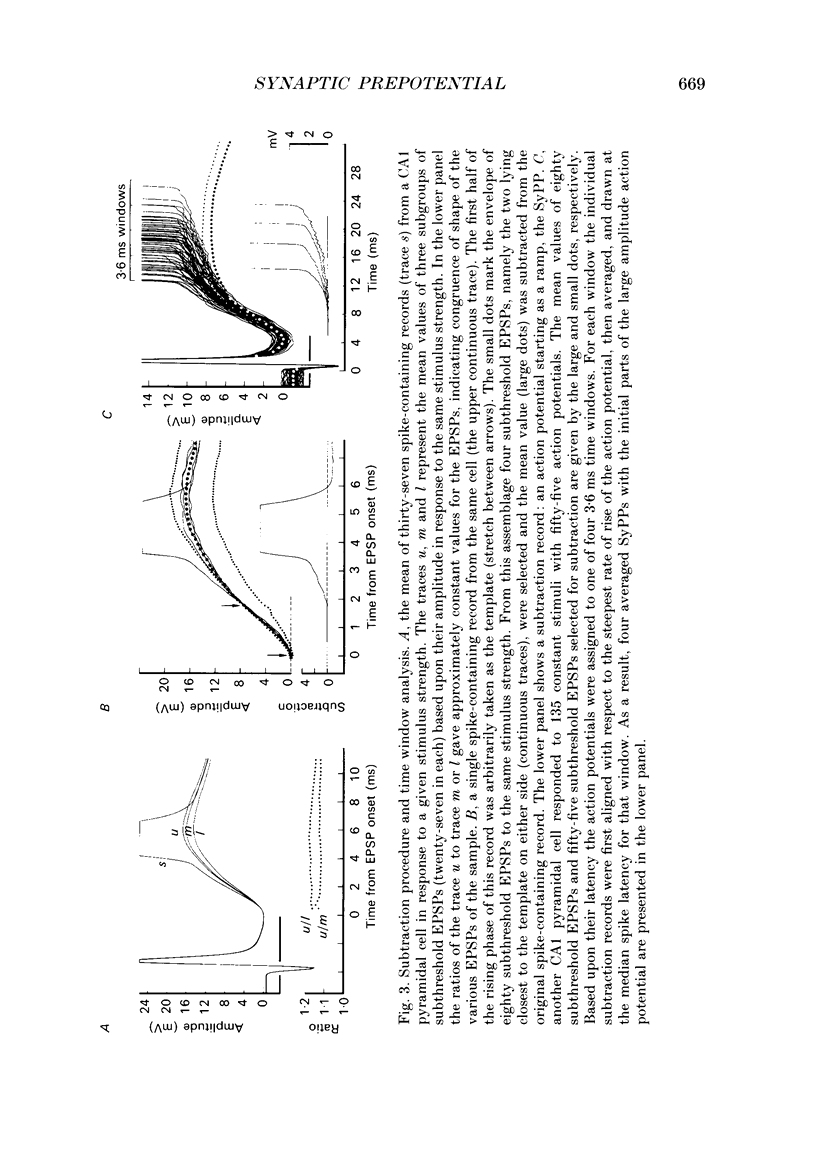
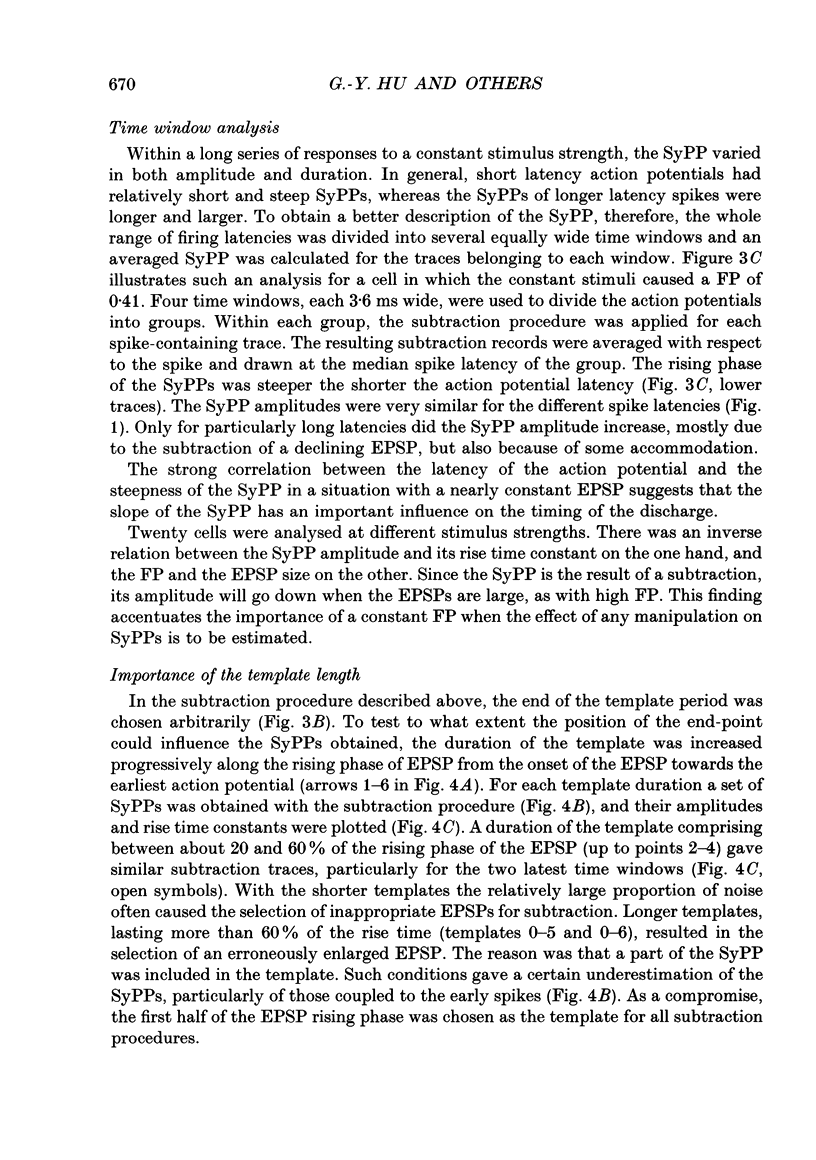
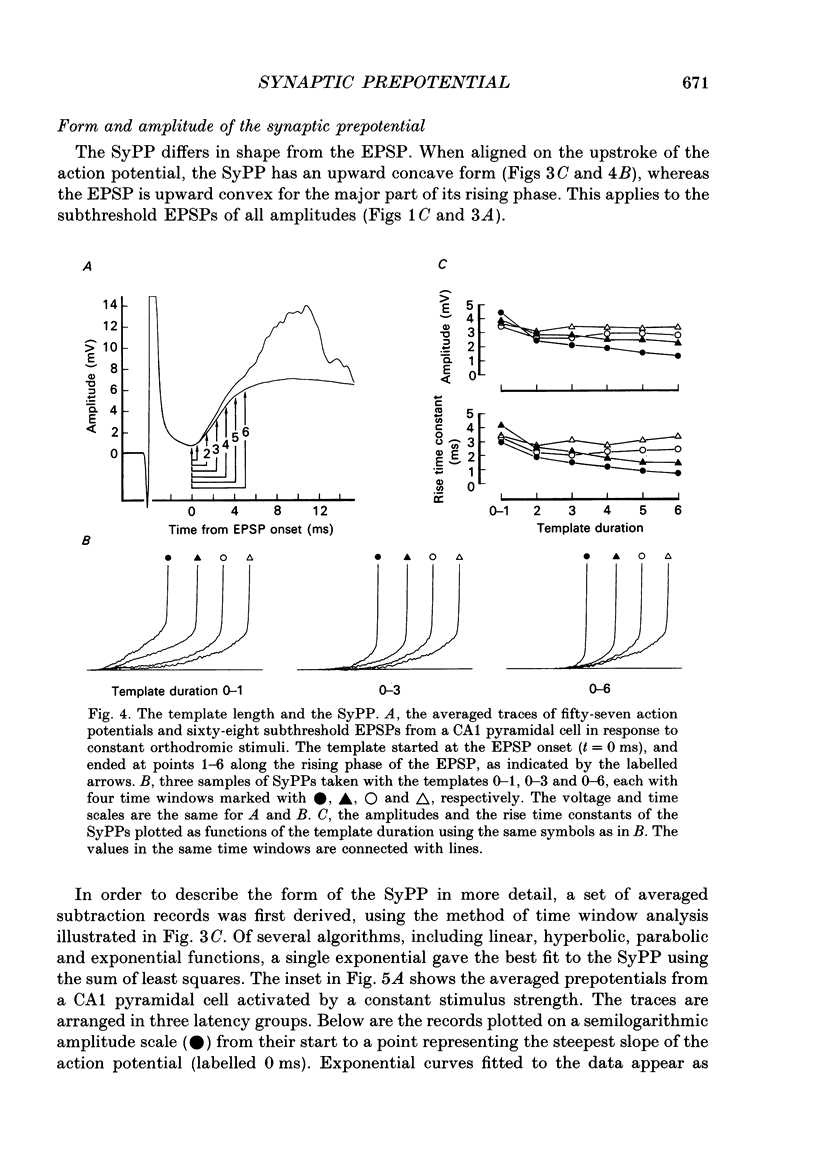
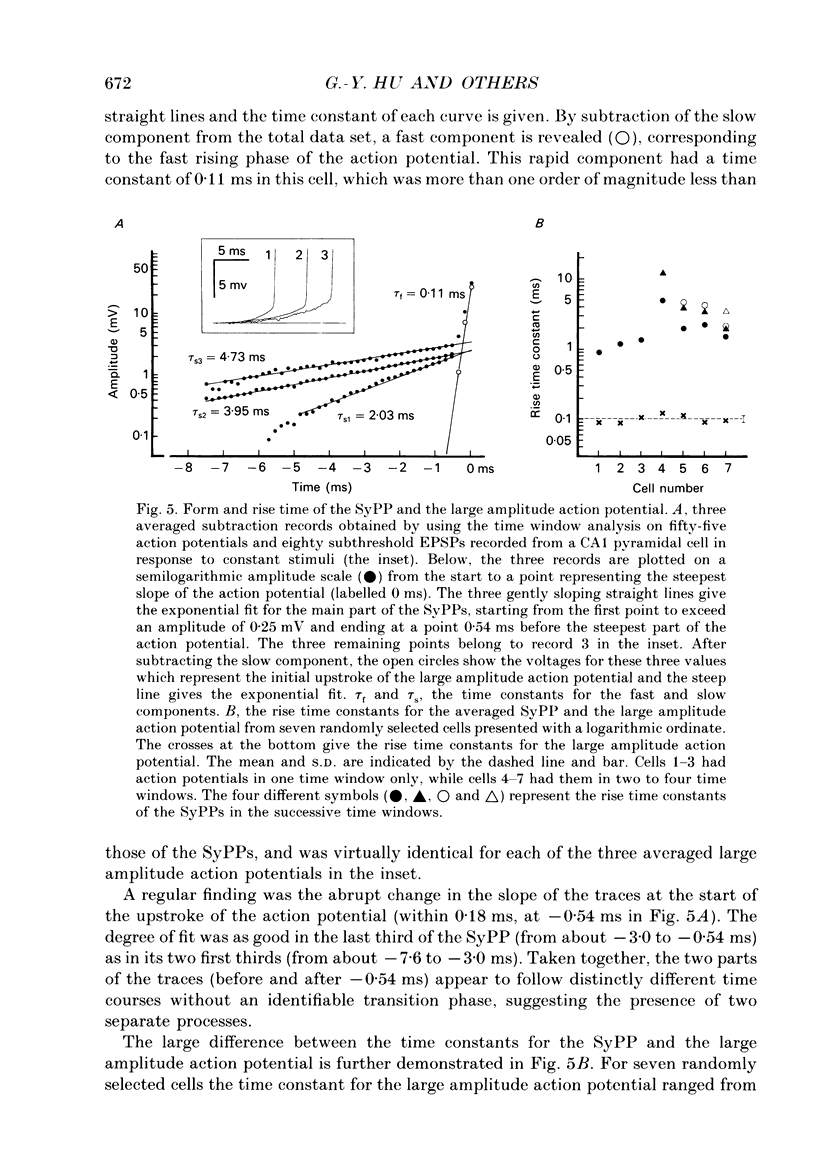
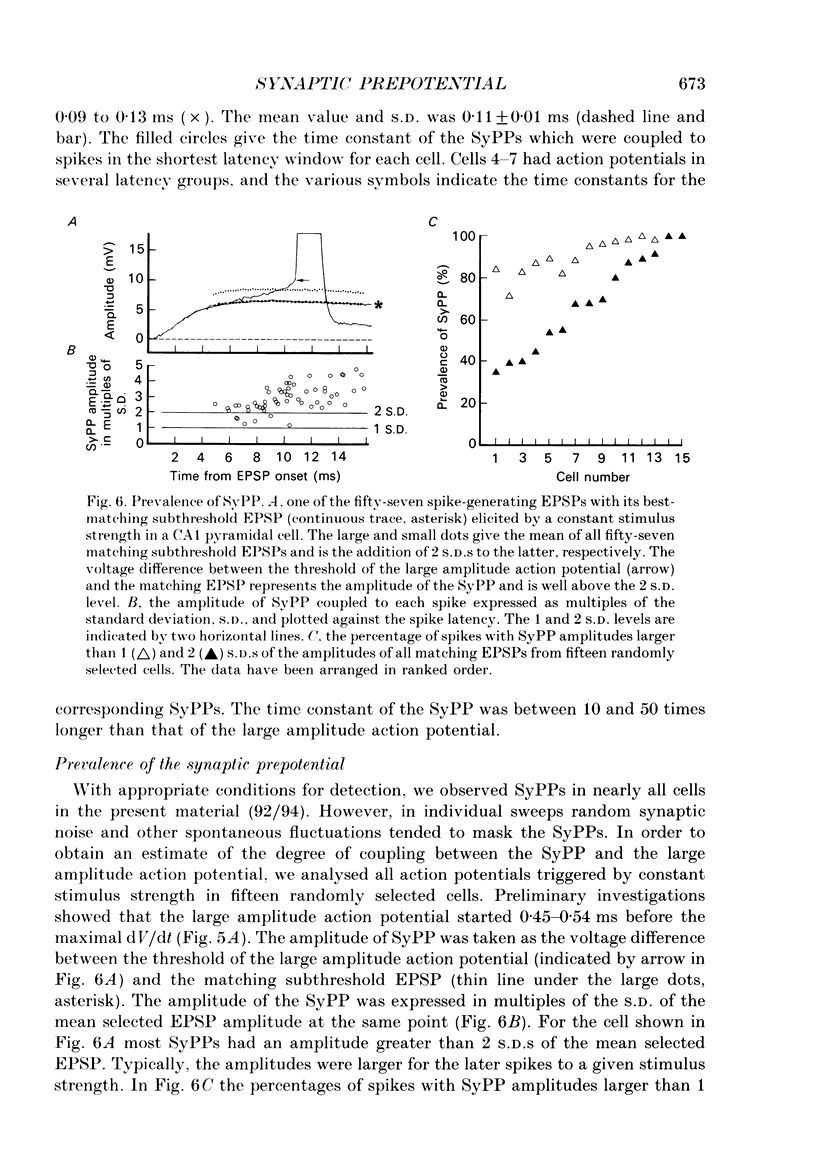
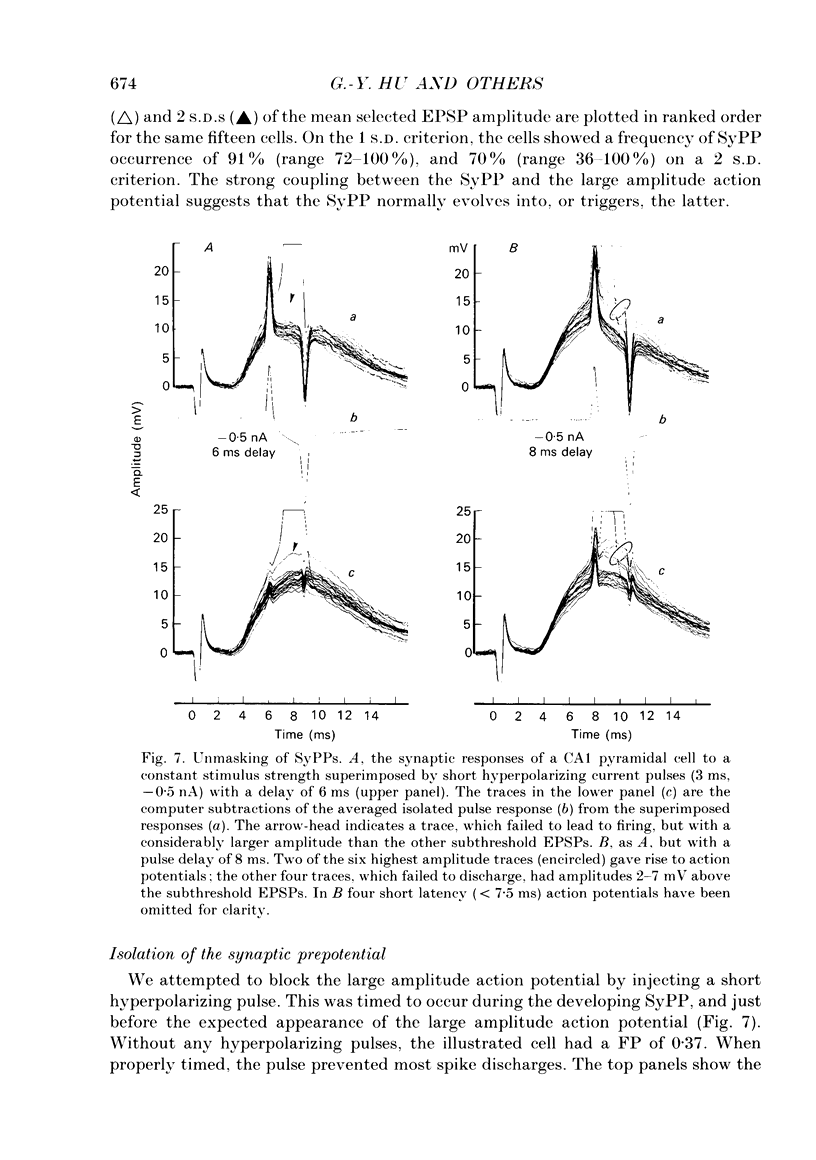
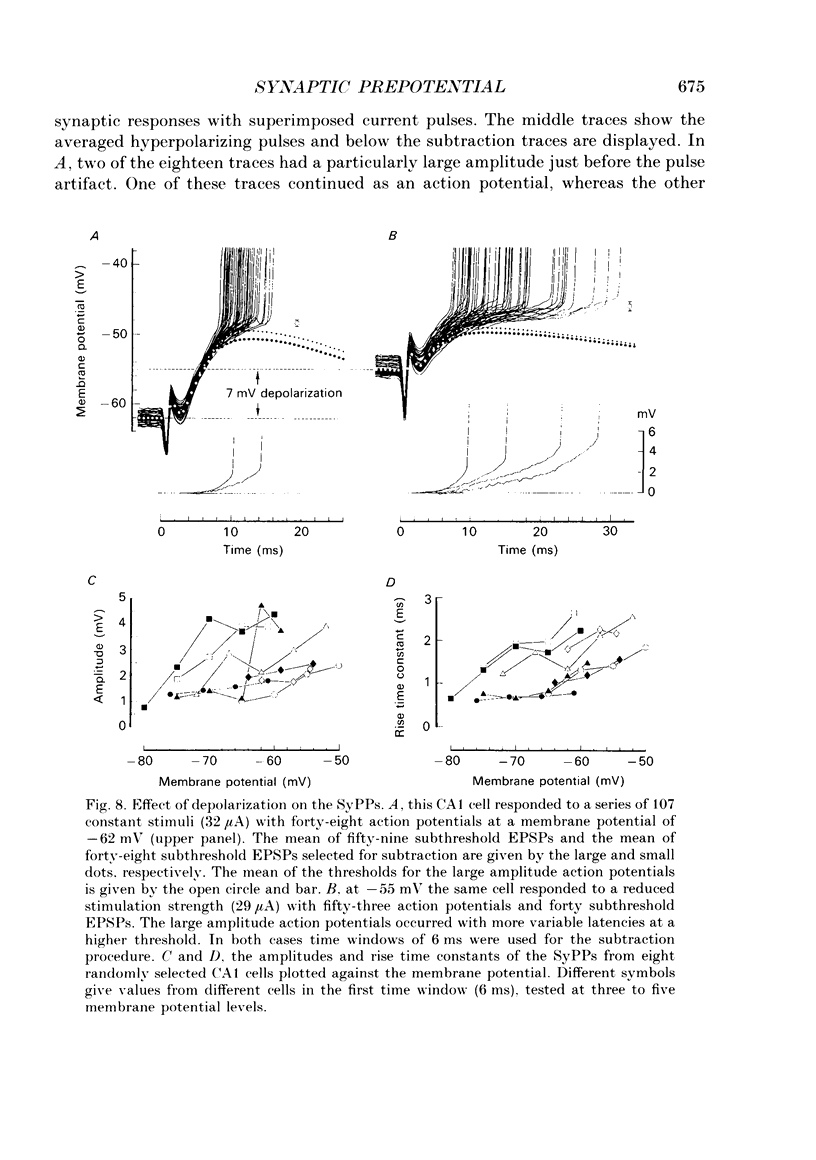
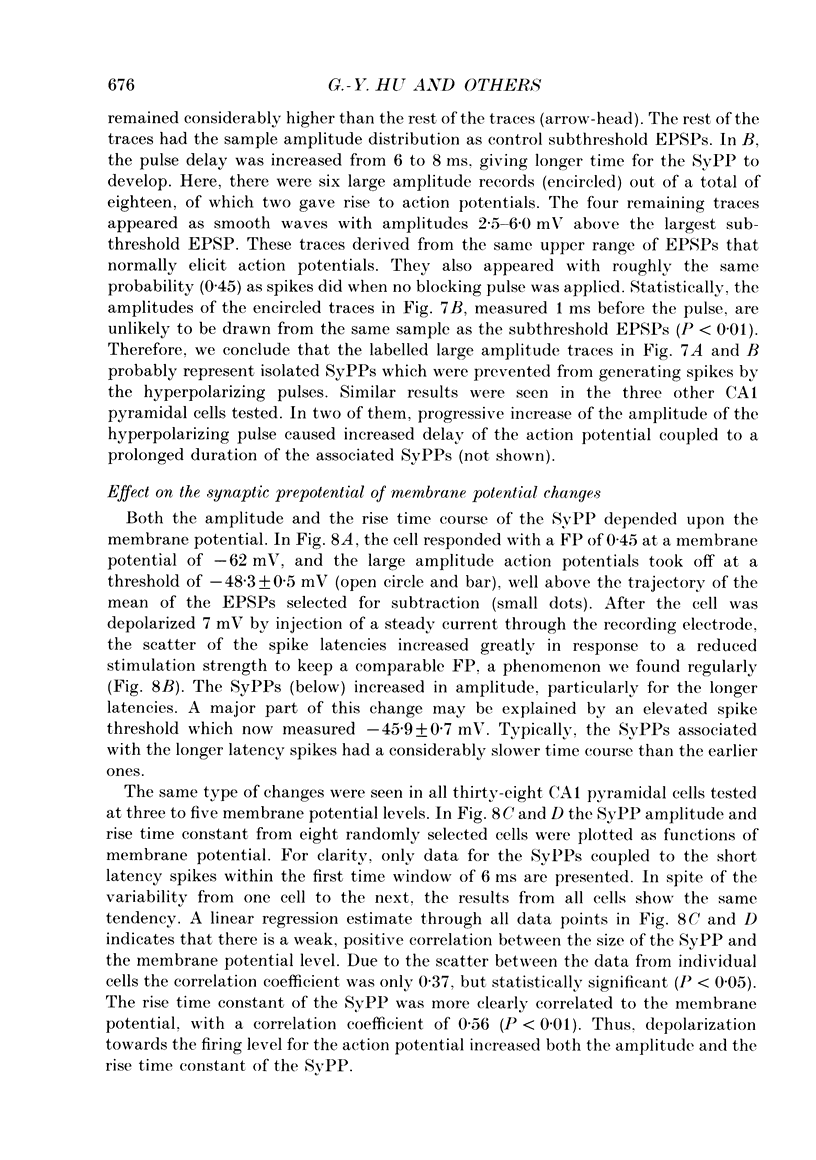
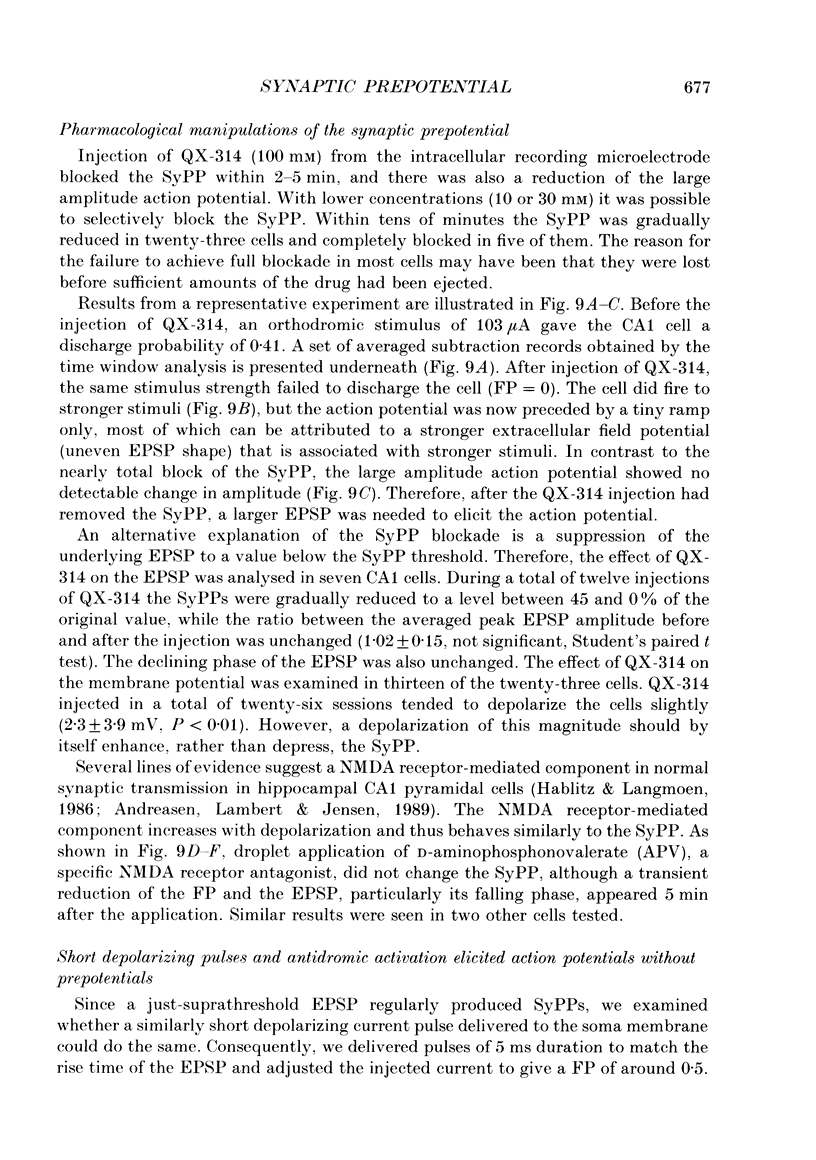
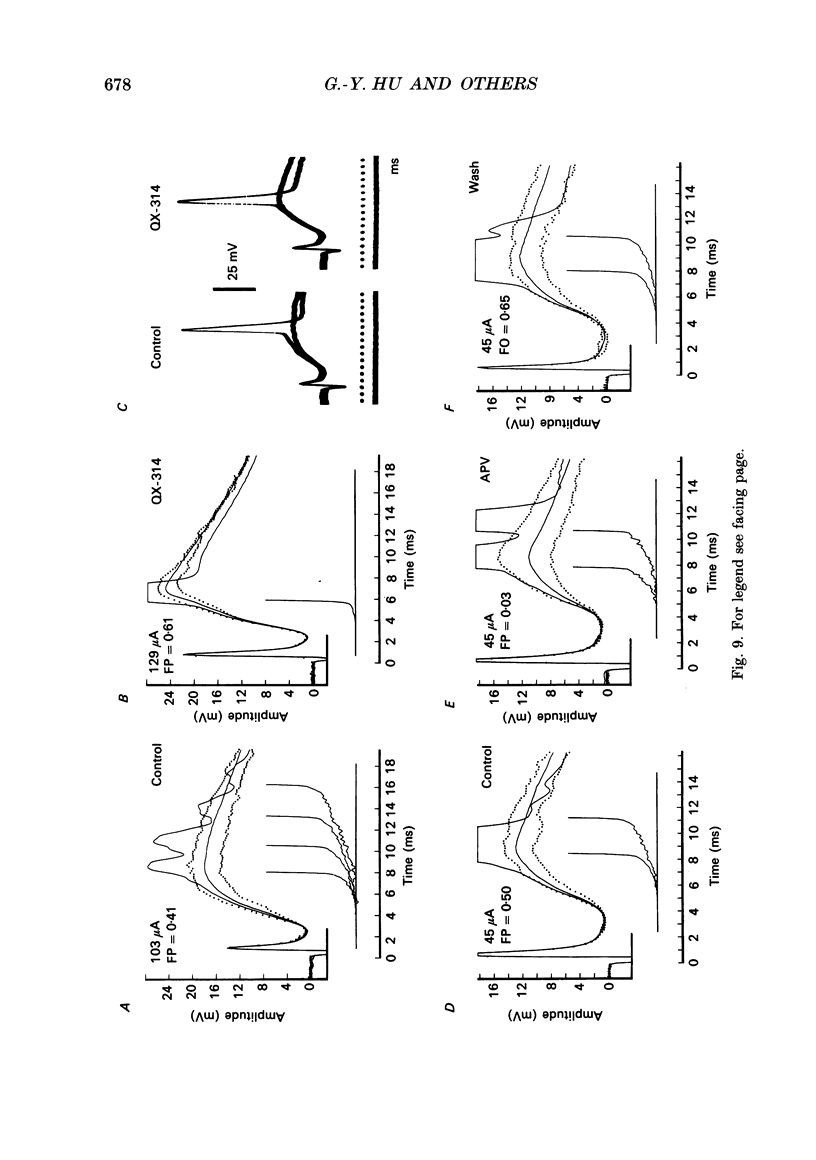
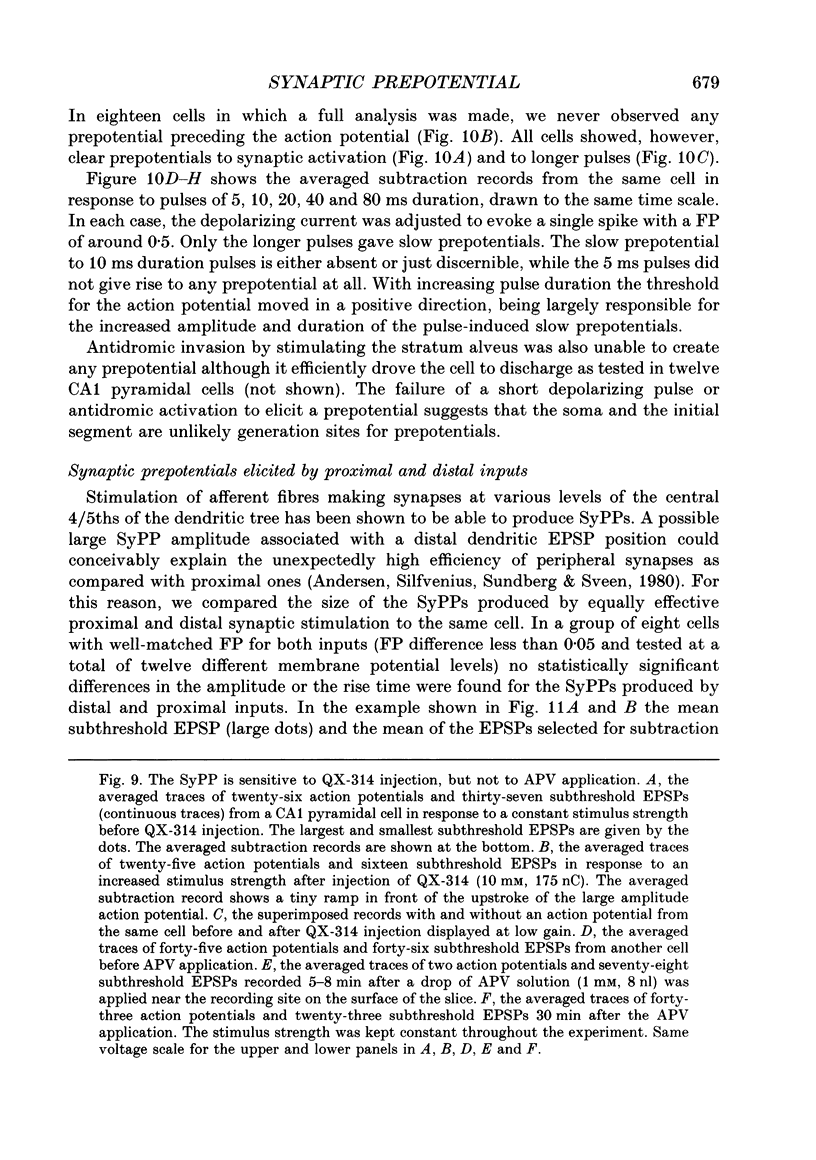
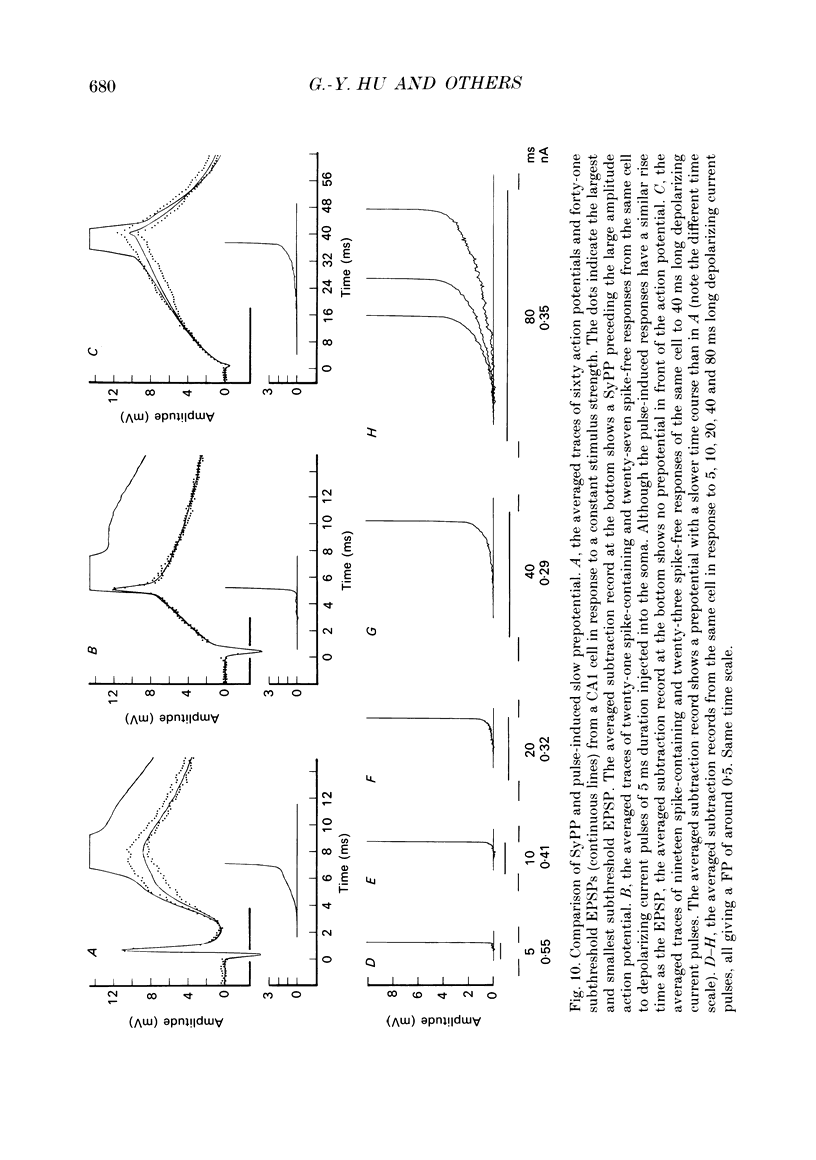
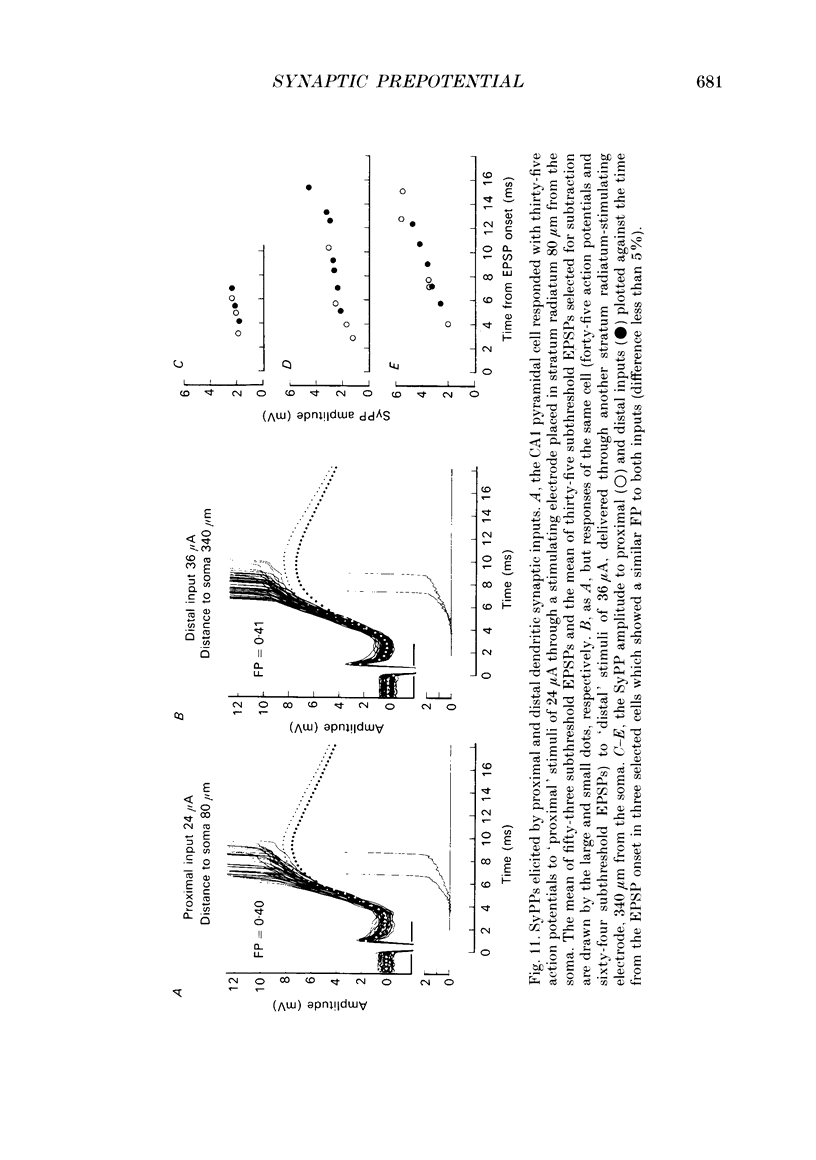
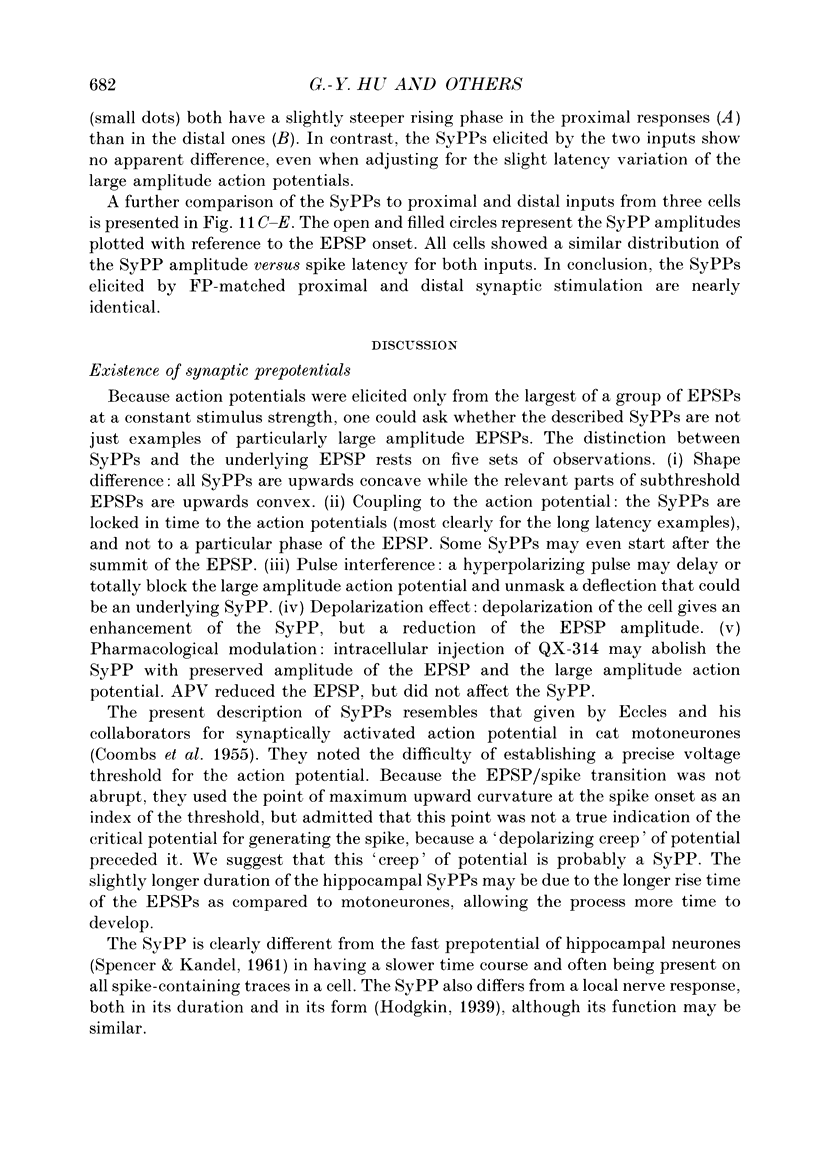
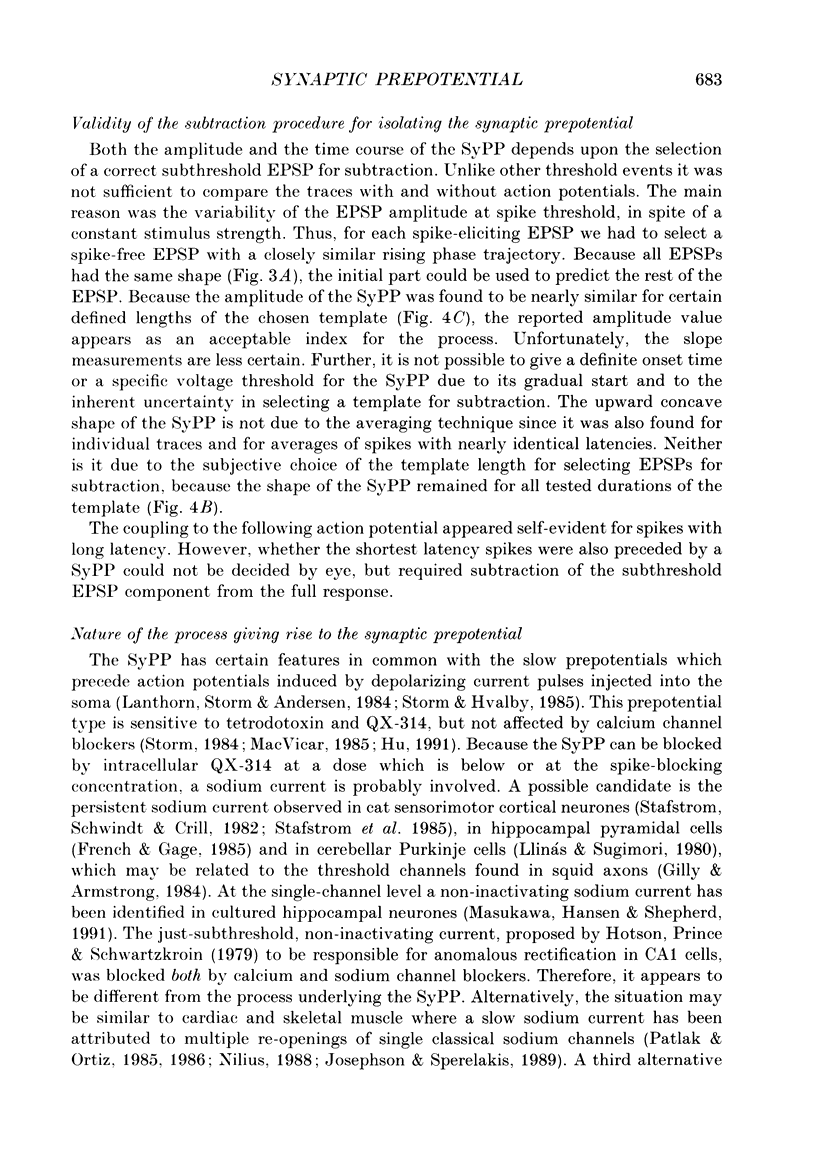
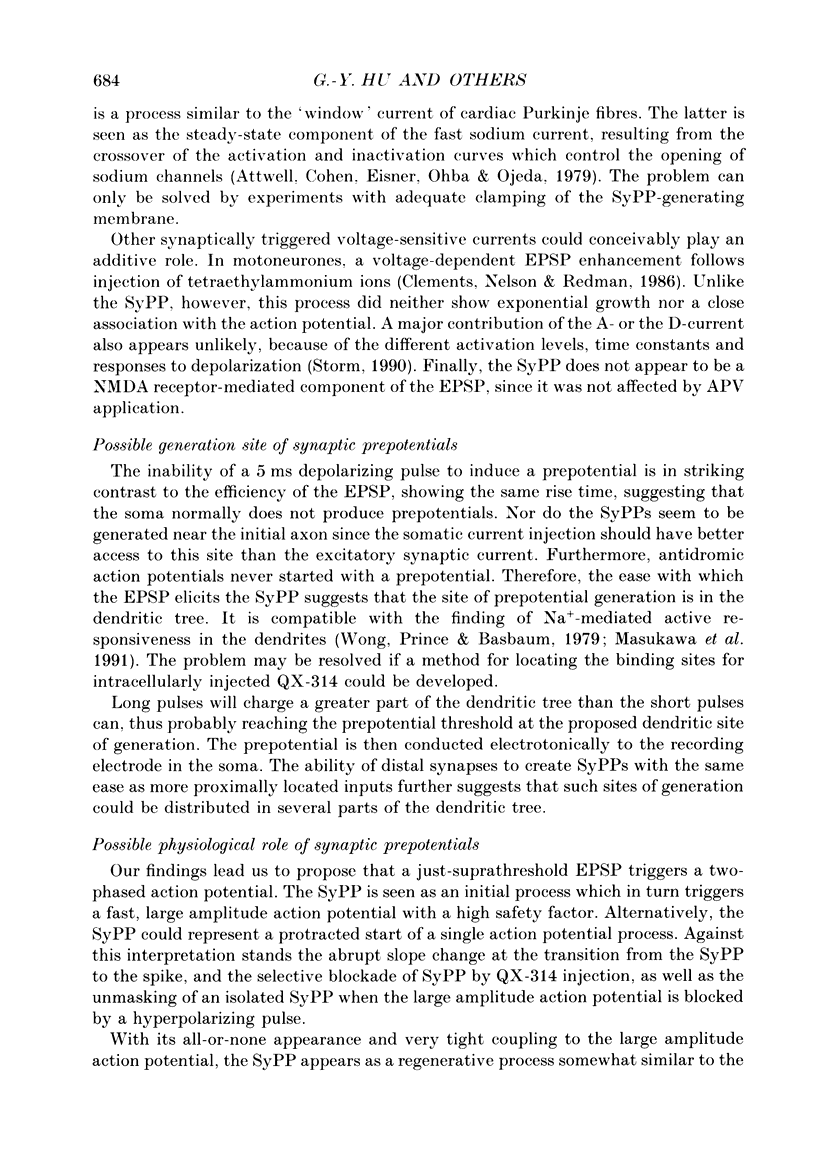
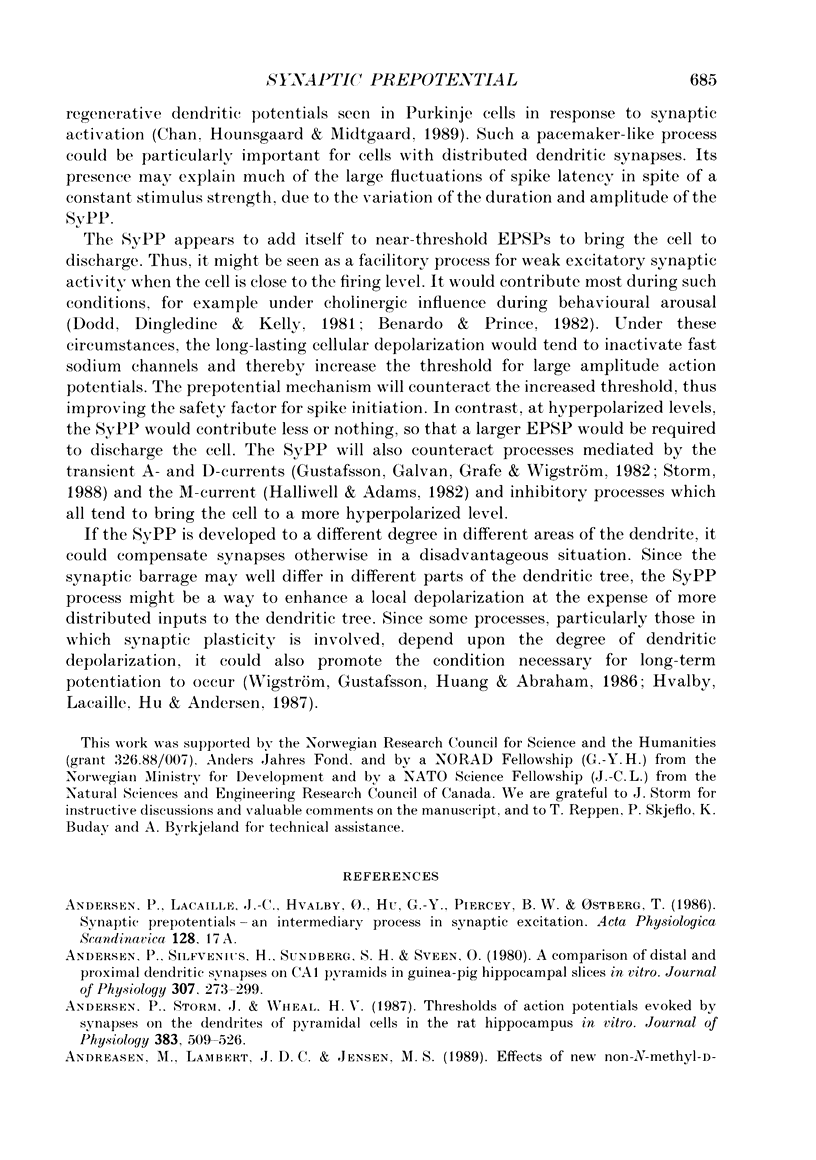
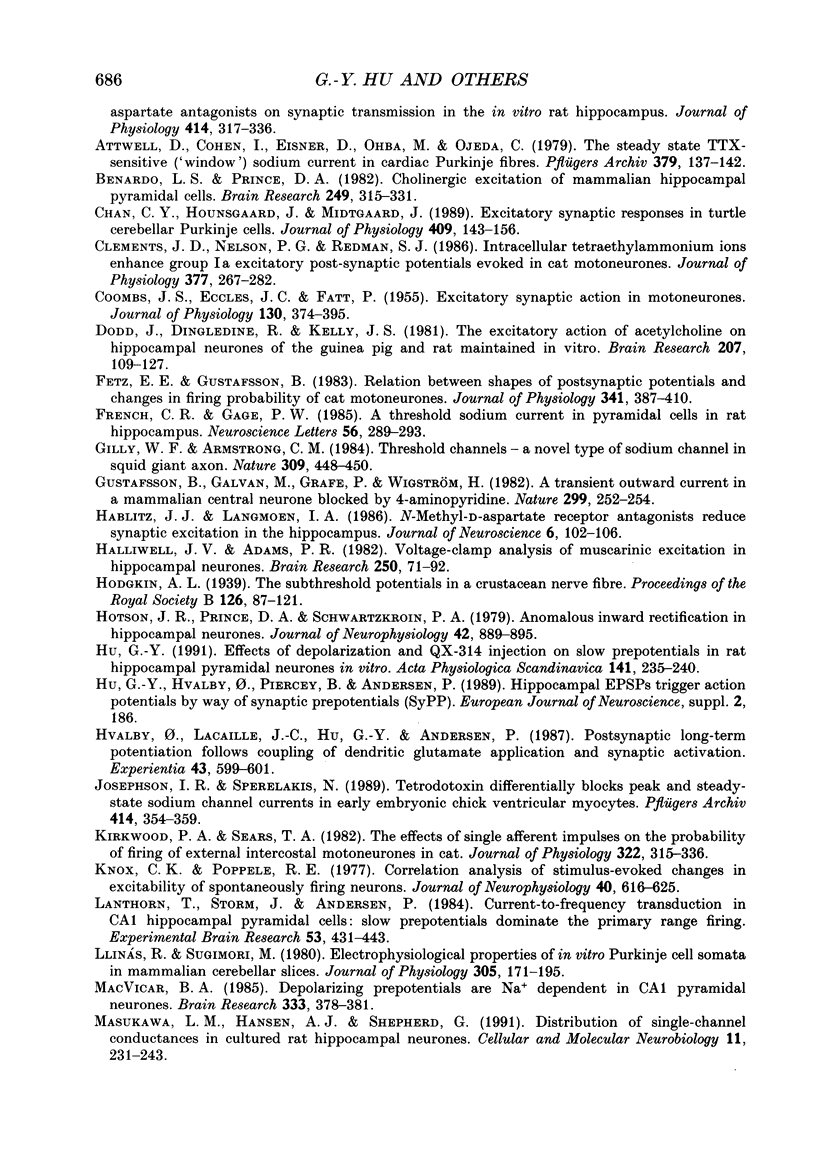
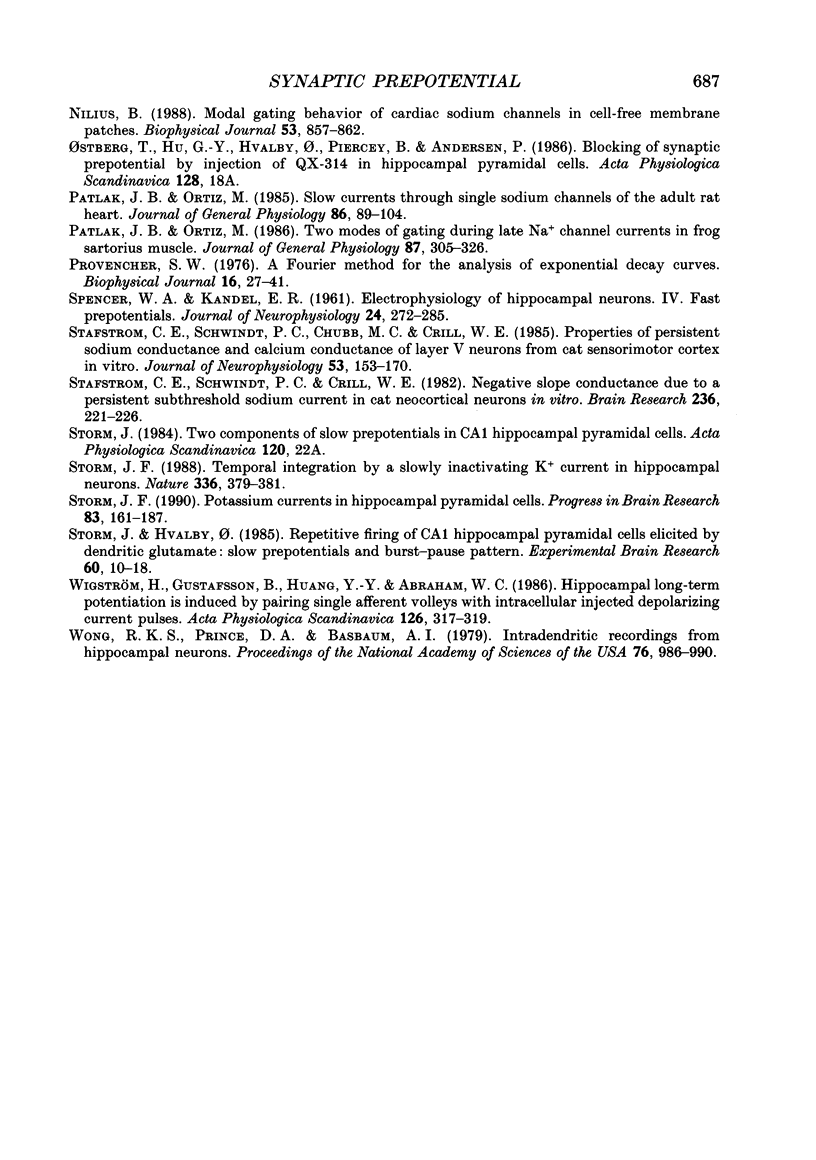
Selected References
These references are in PubMed. This may not be the complete list of references from this article.
- Andersen P., Silfvenius H., Sundberg S. H., Sveen O. A comparison of distal and proximal dendritic synapses on CAi pyramids in guinea-pig hippocampal slices in vitro. J Physiol. 1980 Oct;307:273–299. doi: 10.1113/jphysiol.1980.sp013435. [DOI] [PMC free article] [PubMed] [Google Scholar]
- Anderson P., Storm J., Wheal H. V. Thresholds of action potentials evoked by synapses on the dendrites of pyramidal cells in the rat hippocampus in vitro. J Physiol. 1987 Feb;383:509–526. doi: 10.1113/jphysiol.1987.sp016425. [DOI] [PMC free article] [PubMed] [Google Scholar]
- Andreasen M., Lambert J. D., Jensen M. S. Effects of new non-N-methyl-D-aspartate antagonists on synaptic transmission in the in vitro rat hippocampus. J Physiol. 1989 Jul;414:317–336. doi: 10.1113/jphysiol.1989.sp017690. [DOI] [PMC free article] [PubMed] [Google Scholar]
- Attwell D., Cohen I., Eisner D., Ohba M., Ojeda C. The steady state TTX-sensitive ("window") sodium current in cardiac Purkinje fibres. Pflugers Arch. 1979 Mar 16;379(2):137–142. doi: 10.1007/BF00586939. [DOI] [PubMed] [Google Scholar]
- Benardo L. S., Prince D. A. Cholinergic excitation of mammalian hippocampal pyramidal cells. Brain Res. 1982 Oct 14;249(2):315–331. doi: 10.1016/0006-8993(82)90066-x. [DOI] [PubMed] [Google Scholar]
- COOMBS J. S., ECCLES J. C., FATT P. Excitatory synaptic action in motoneurones. J Physiol. 1955 Nov 28;130(2):374–395. doi: 10.1113/jphysiol.1955.sp005413. [DOI] [PMC free article] [PubMed] [Google Scholar]
- Chan C. Y., Hounsgaard J., Midtgaard J. Excitatory synaptic responses in turtle cerebellar Purkinje cells. J Physiol. 1989 Feb;409:143–156. doi: 10.1113/jphysiol.1989.sp017489. [DOI] [PMC free article] [PubMed] [Google Scholar]
- Clements J. D., Nelson P. G., Redman S. J. Intracellular tetraethylammonium ions enhance group Ia excitatory post-synaptic potentials evoked in cat motoneurones. J Physiol. 1986 Aug;377:267–282. doi: 10.1113/jphysiol.1986.sp016186. [DOI] [PMC free article] [PubMed] [Google Scholar]
- Dodd J., Dingledine R., Kelly J. S. The excitatory action of acetylcholine on hippocampal neurones of the guinea pig and rat maintained in vitro. Brain Res. 1981 Feb 23;207(1):109–127. doi: 10.1016/0006-8993(81)90682-x. [DOI] [PubMed] [Google Scholar]
- Fetz E. E., Gustafsson B. Relation between shapes of post-synaptic potentials and changes in firing probability of cat motoneurones. J Physiol. 1983 Aug;341:387–410. doi: 10.1113/jphysiol.1983.sp014812. [DOI] [PMC free article] [PubMed] [Google Scholar]
- French C. R., Gage P. W. A threshold sodium current in pyramidal cells in rat hippocampus. Neurosci Lett. 1985 May 23;56(3):289–293. doi: 10.1016/0304-3940(85)90257-5. [DOI] [PubMed] [Google Scholar]
- Gilly W. F., Armstrong C. M. Threshold channels--a novel type of sodium channel in squid giant axon. 1984 May 31-Jun 6Nature. 309(5967):448–450. doi: 10.1038/309448a0. [DOI] [PubMed] [Google Scholar]
- Gustafsson B., Galvan M., Grafe P., Wigström H. A transient outward current in a mammalian central neurone blocked by 4-aminopyridine. Nature. 1982 Sep 16;299(5880):252–254. doi: 10.1038/299252a0. [DOI] [PubMed] [Google Scholar]
- Hablitz J. J., Langmoen I. A. N-methyl-D-aspartate receptor antagonists reduce synaptic excitation in the hippocampus. J Neurosci. 1986 Jan;6(1):102–106. doi: 10.1523/JNEUROSCI.06-01-00102.1986. [DOI] [PMC free article] [PubMed] [Google Scholar]
- Halliwell J. V., Adams P. R. Voltage-clamp analysis of muscarinic excitation in hippocampal neurons. Brain Res. 1982 Oct 28;250(1):71–92. doi: 10.1016/0006-8993(82)90954-4. [DOI] [PubMed] [Google Scholar]
- Hotson J. R., Prince D. A., Schwartzkroin P. A. Anomalous inward rectification in hippocampal neurons. J Neurophysiol. 1979 May;42(3):889–895. doi: 10.1152/jn.1979.42.3.889. [DOI] [PubMed] [Google Scholar]
- Hu G. Y. Effects of depolarization and QX-314 injection on slow prepotentials in rat hippocampal pyramidal neurones in vitro. Acta Physiol Scand. 1991 Feb;141(2):235–240. doi: 10.1111/j.1748-1716.1991.tb09073.x. [DOI] [PubMed] [Google Scholar]
- Hvalby O., Lacaille J. C., Hu G. Y., Andersen P. Postsynaptic long-term potentiation follows coupling of dendritic glutamate application and synaptic activation. Experientia. 1987 Jun 15;43(6):599–601. doi: 10.1007/BF02126343. [DOI] [PubMed] [Google Scholar]
- Josephson I. R., Sperelakis N. Tetrodotoxin differentially blocks peak and steady-state sodium channel currents in early embryonic chick ventricular myocytes. Pflugers Arch. 1989 Jul;414(3):354–359. doi: 10.1007/BF00584639. [DOI] [PubMed] [Google Scholar]
- Kirkwood P. A., Sears T. A. The effects of single afferent impulses on the probability of firing of external intercostal motoneurones in the cat. J Physiol. 1982 Jan;322:315–336. doi: 10.1113/jphysiol.1982.sp014039. [DOI] [PMC free article] [PubMed] [Google Scholar]
- Knox C. K., Poppele R. E. Correlation analysis of stimulus-evoked changes in excitability of spontaneously firing neurons. J Neurophysiol. 1977 May;40(3):616–625. doi: 10.1152/jn.1977.40.3.616. [DOI] [PubMed] [Google Scholar]
- Lanthorn T., Storm J., Andersen P. Current-to-frequency transduction in CA1 hippocampal pyramidal cells: slow prepotentials dominate the primary range firing. Exp Brain Res. 1984;53(2):431–443. doi: 10.1007/BF00238173. [DOI] [PubMed] [Google Scholar]
- Llinás R., Sugimori M. Electrophysiological properties of in vitro Purkinje cell somata in mammalian cerebellar slices. J Physiol. 1980 Aug;305:171–195. doi: 10.1113/jphysiol.1980.sp013357. [DOI] [PMC free article] [PubMed] [Google Scholar]
- Macvicar B. A. Depolarizing prepotentials are Na+ dependent in CA1 pyramidal neurons. Brain Res. 1985 May 6;333(2):378–381. doi: 10.1016/0006-8993(85)91597-5. [DOI] [PubMed] [Google Scholar]
- Masukawa L. M., Hansen A. J., Shepherd G. Distribution of single-channel conductances in cultured rat hippocampal neurons. Cell Mol Neurobiol. 1991 Apr;11(2):231–243. doi: 10.1007/BF00769036. [DOI] [PubMed] [Google Scholar]
- Nilius B. Modal gating behavior of cardiac sodium channels in cell-free membrane patches. Biophys J. 1988 Jun;53(6):857–862. doi: 10.1016/S0006-3495(88)83166-7. [DOI] [PMC free article] [PubMed] [Google Scholar]
- Patlak J. B., Ortiz M. Slow currents through single sodium channels of the adult rat heart. J Gen Physiol. 1985 Jul;86(1):89–104. doi: 10.1085/jgp.86.1.89. [DOI] [PMC free article] [PubMed] [Google Scholar]
- Patlak J. B., Ortiz M. Two modes of gating during late Na+ channel currents in frog sartorius muscle. J Gen Physiol. 1986 Feb;87(2):305–326. doi: 10.1085/jgp.87.2.305. [DOI] [PMC free article] [PubMed] [Google Scholar]
- Provencher S. W. A Fourier method for the analysis of exponential decay curves. Biophys J. 1976 Jan;16(1):27–41. doi: 10.1016/S0006-3495(76)85660-3. [DOI] [PMC free article] [PubMed] [Google Scholar]
- Stafstrom C. E., Schwindt P. C., Chubb M. C., Crill W. E. Properties of persistent sodium conductance and calcium conductance of layer V neurons from cat sensorimotor cortex in vitro. J Neurophysiol. 1985 Jan;53(1):153–170. doi: 10.1152/jn.1985.53.1.153. [DOI] [PubMed] [Google Scholar]
- Stafstrom C. E., Schwindt P. C., Crill W. E. Negative slope conductance due to a persistent subthreshold sodium current in cat neocortical neurons in vitro. Brain Res. 1982 Mar 18;236(1):221–226. doi: 10.1016/0006-8993(82)90050-6. [DOI] [PubMed] [Google Scholar]
- Storm J. F. Potassium currents in hippocampal pyramidal cells. Prog Brain Res. 1990;83:161–187. doi: 10.1016/s0079-6123(08)61248-0. [DOI] [PubMed] [Google Scholar]
- Storm J. F. Temporal integration by a slowly inactivating K+ current in hippocampal neurons. Nature. 1988 Nov 24;336(6197):379–381. doi: 10.1038/336379a0. [DOI] [PubMed] [Google Scholar]
- Storm J., Hvalby O. Repetitive firing of CA1 hippocampal pyramidal cells elicited by dendritic glutamate: slow prepotentials and burst-pause pattern. Exp Brain Res. 1985;60(1):10–18. doi: 10.1007/BF00237013. [DOI] [PubMed] [Google Scholar]
- Wigström H., Gustafsson B., Huang Y. Y., Abraham W. C. Hippocampal long-term potentiation is induced by pairing single afferent volleys with intracellularly injected depolarizing current pulses. Acta Physiol Scand. 1986 Feb;126(2):317–319. doi: 10.1111/j.1748-1716.1986.tb07822.x. [DOI] [PubMed] [Google Scholar]
- Wong R. K., Prince D. A., Basbaum A. I. Intradendritic recordings from hippocampal neurons. Proc Natl Acad Sci U S A. 1979 Feb;76(2):986–990. doi: 10.1073/pnas.76.2.986. [DOI] [PMC free article] [PubMed] [Google Scholar]