Abstract
1. The sensitivity of outward Na(+)-Ca2+ exchange current to charged amphiphiles and phospholipids was tested in giant excised inside-out membrane patches from guinea-pig and rabbit myocytes. 2. Screening of membrane surface potentials with dimethonium (10 mM), spermine (200 microM) and spermidine (100 microM) was without effect, while the positively charged ionic detergents hexadecyltrimethylammonium and dodecyltrimethylammonium strongly inhibited steady-state outward exchange current (0.1-10 microM). 3. Interventions expected to increase negative surface charge included treatment of the cytoplasmic surface with phospholipase D, application of dodecylsulphate (1-10 microM), application of the short-chain phosphatidylserine derivative, dicapryl phosphatidylserine (C10PS), and inclusion of 1-3% phosphatidylserine in the hydrocarbon mixture used to coat electrodes. Each intervention strongly stimulated Na(+)-Ca2+ exchange current in a similar way to MgATP, reducing the fractional decay of outward exchange current (inactivation) during application of high cytoplasmic sodium. 4. The MgATP-stimulated exchange current was inhibited with a Ki of approximately 1 microM by pentalysine, which is known to associate with phosphatidylserine head groups. After 'deregulation' of the exchanger by chymotrypsin, pentalysine was without effect. 5. Inclusion in the pipette of 0.2 mM-pyridyldithioethylamine (an oxidizing inhibitor of aminophospholipid translocase) abolished stimulation of outward exchange current by MgATP without inhibiting basal outward exchange current or sodium pump current. 6. Application to the cytoplasmic side of 1.5 mM-diamide, which reportedly decreases membrane phospholipid asymmetry, apparently reversed the effect of MgATP. After treatment with diamide and subsequently with dithiothreitol, Na(+)-Ca2+ exchange current was again stimulated by MgATP. Diamide was without effect when secondary exchange regulation had been previously removed by chymotrypsin. 7. Potassium current carried by the surface potential-sensitive ionophore, nonactin, was stimulated by MgATP when extracellular surface charge had been neutralized. The effect was largest (40-90%) when low ionic strength cytoplasmic solutions were employed, consistent with an increase of negative membrane charge on the cytoplasmic side during MgATP application. 8. Potassium current carried by nonactin was inhibited by MgATP when cytoplasmic surface charge had been neutralized and extracellular solutions of low ionic strength were employed, consistent with a decrease of negative membrane charge on the extracellular side. 9. These results indicate that the stimulatory effect of MgATP on Na(+)-Ca2+ exchange current could involve changes of charged membrane lipids, that the effect probably involves a transmembrane, oxidation-sensitive protein, that pentalysine-sensitive sites are involved, that phosphatidylserine mimics the effect of MgATP, and that the effect extends to a simple surface potential-sensitive ionophore.(ABSTRACT TRUNCATED AT 400 WORDS)
Full text
PDF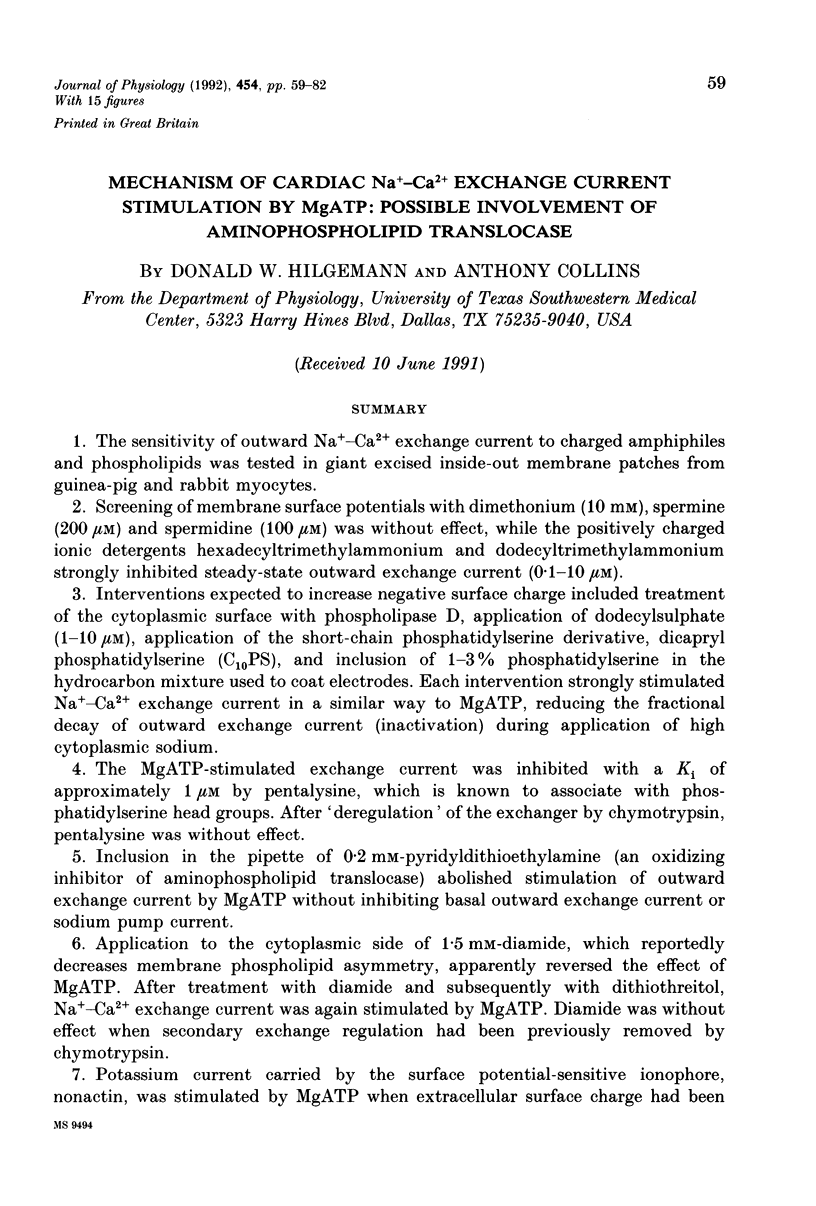
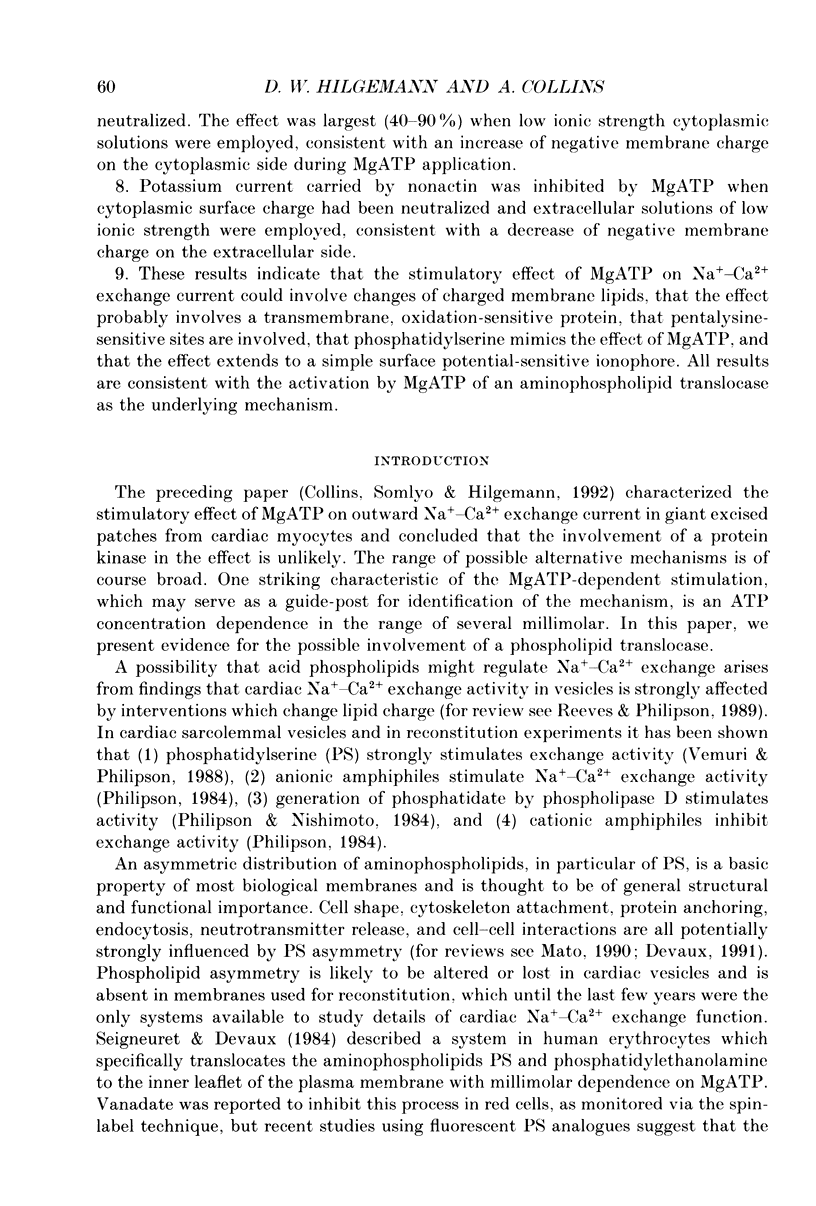
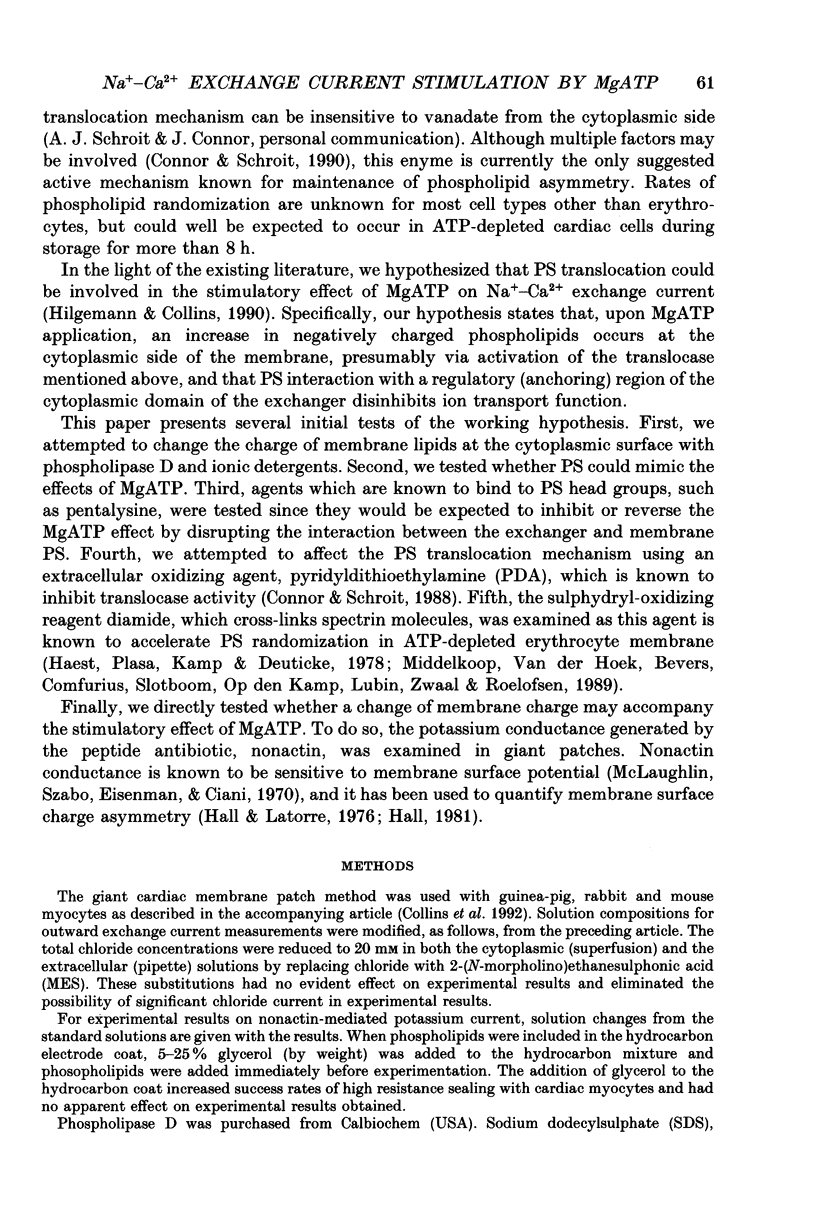
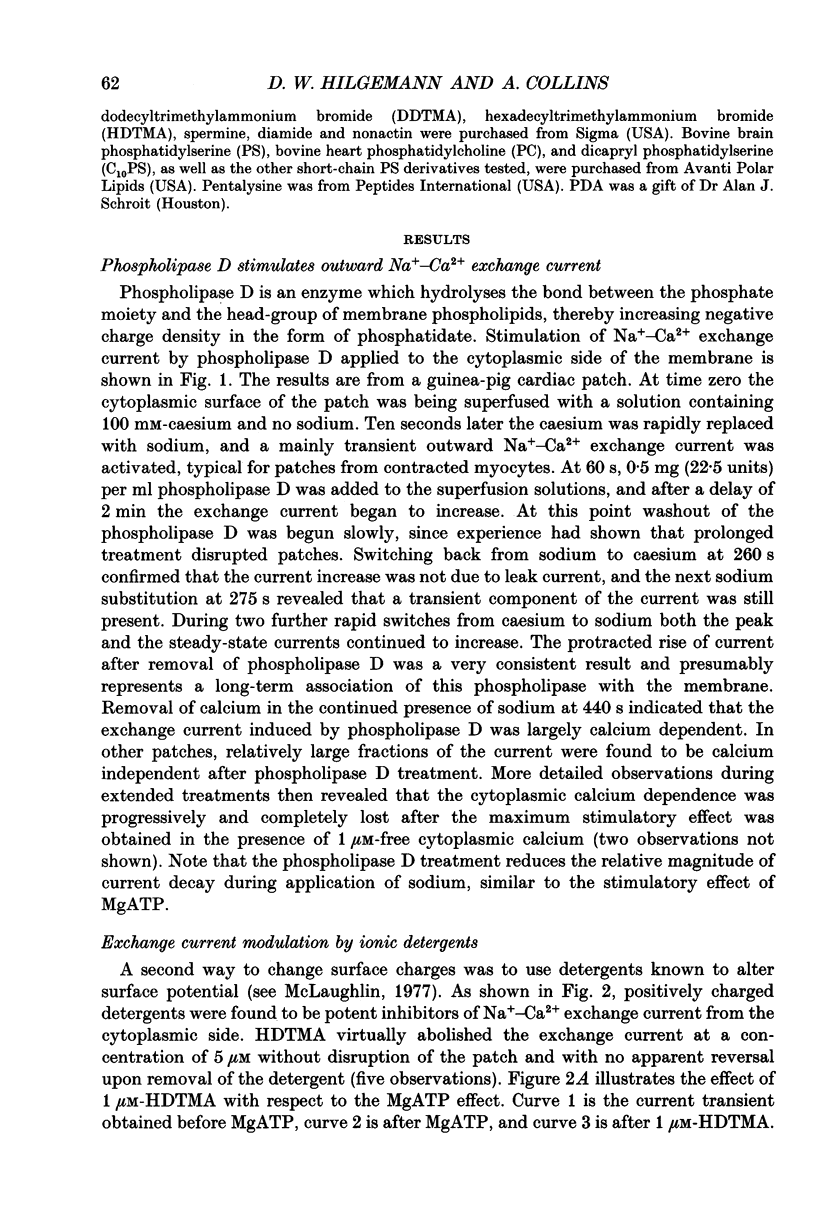
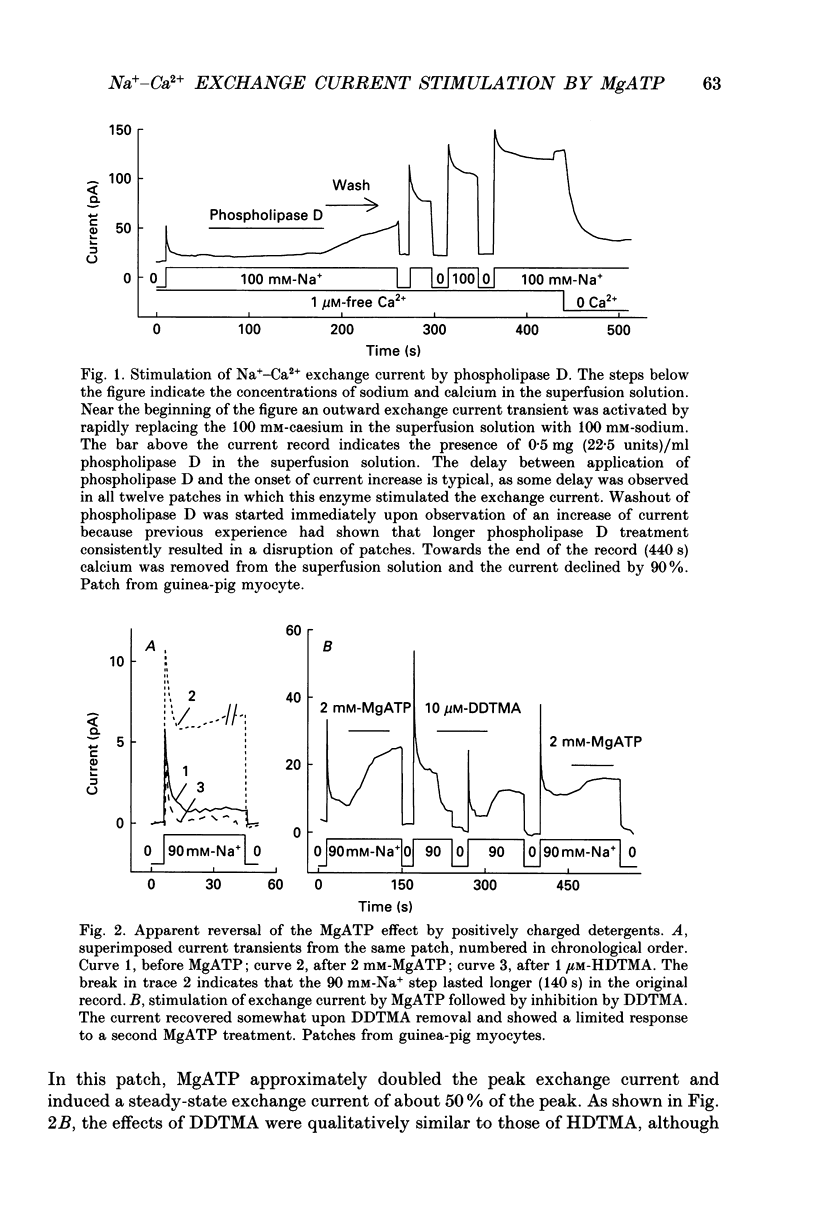
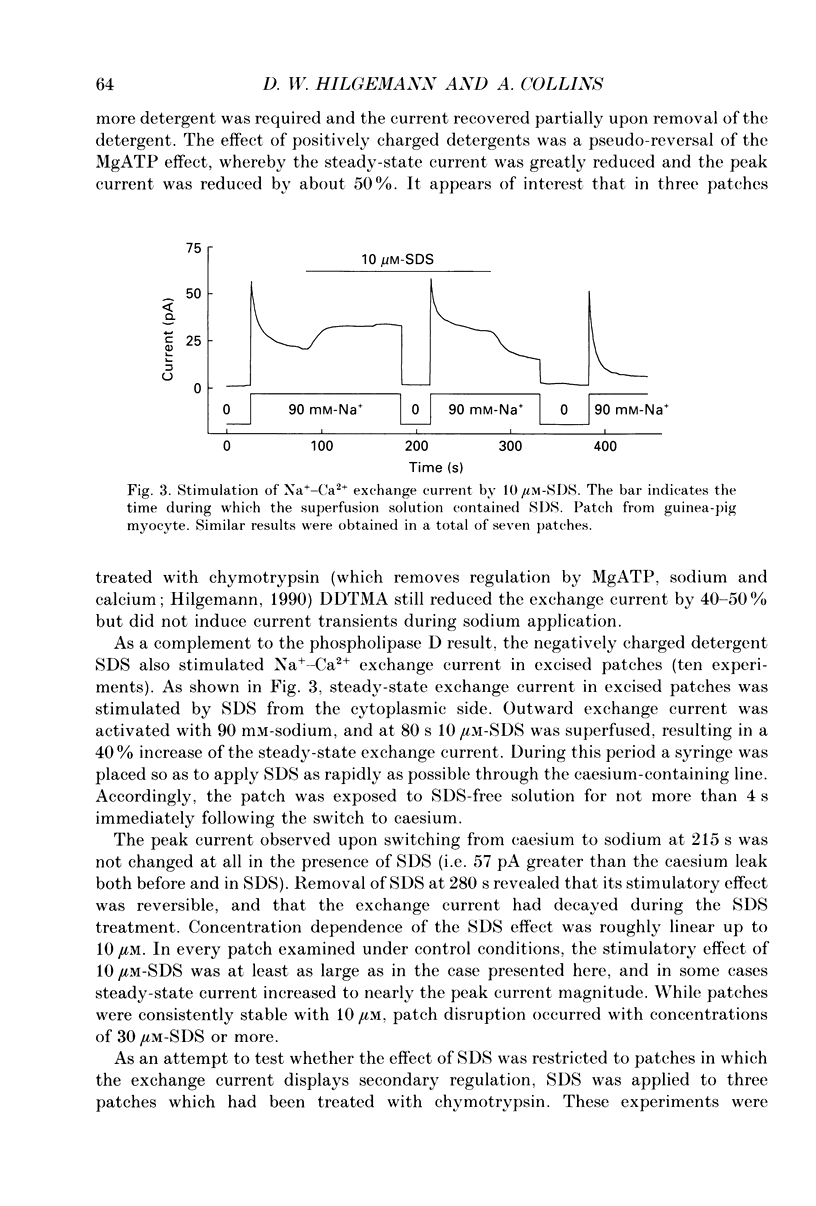
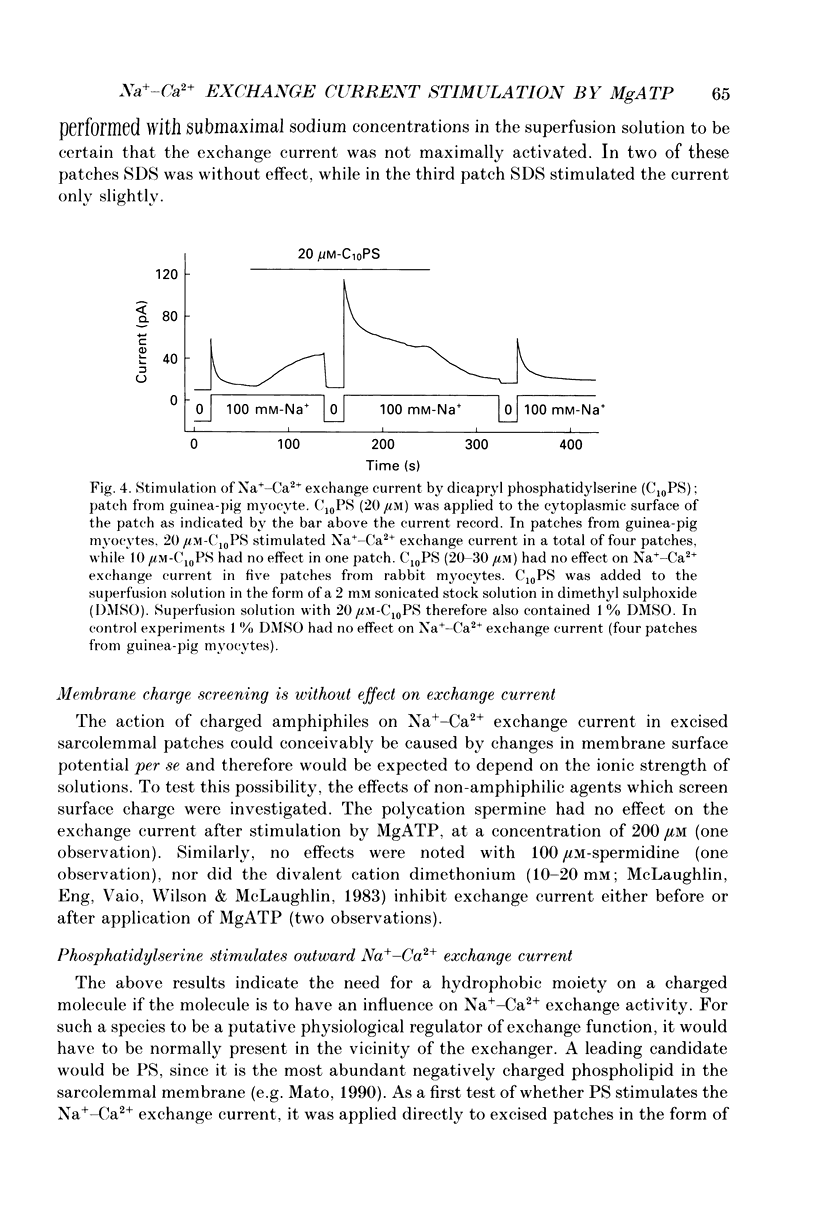
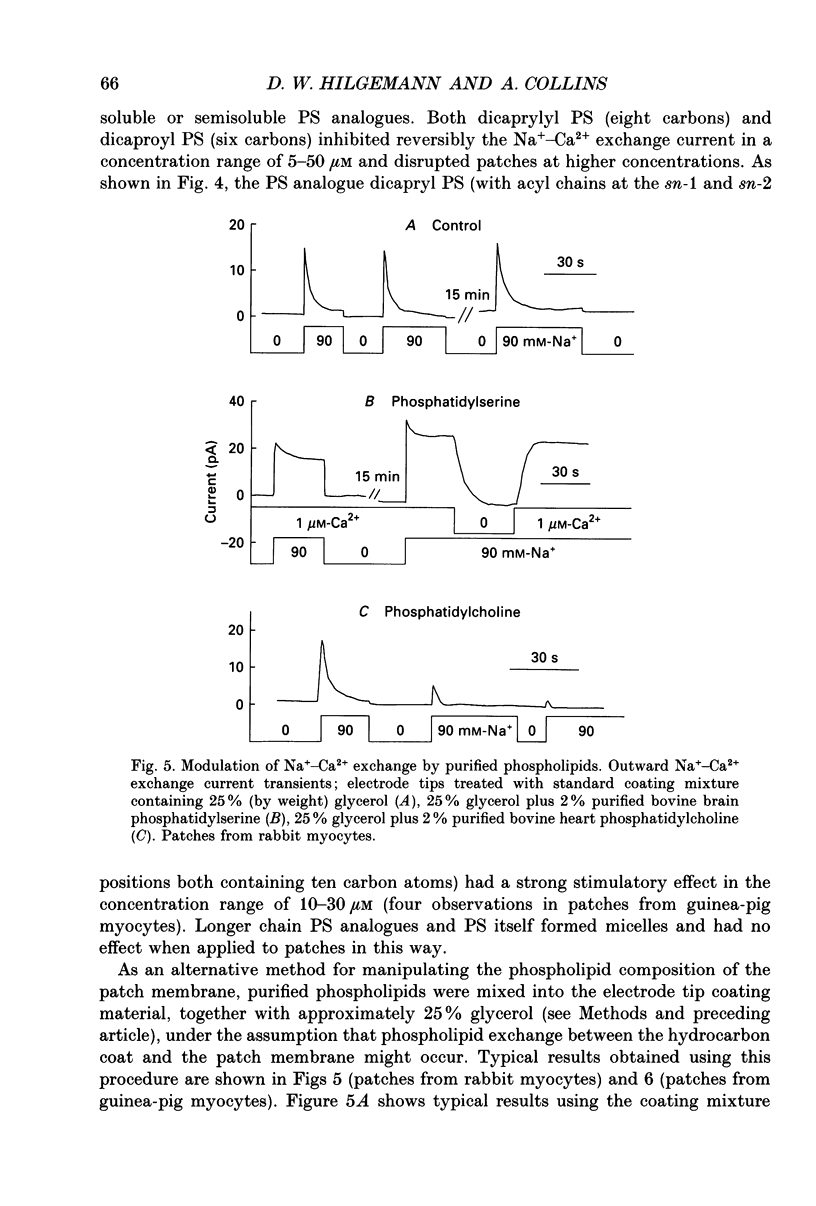
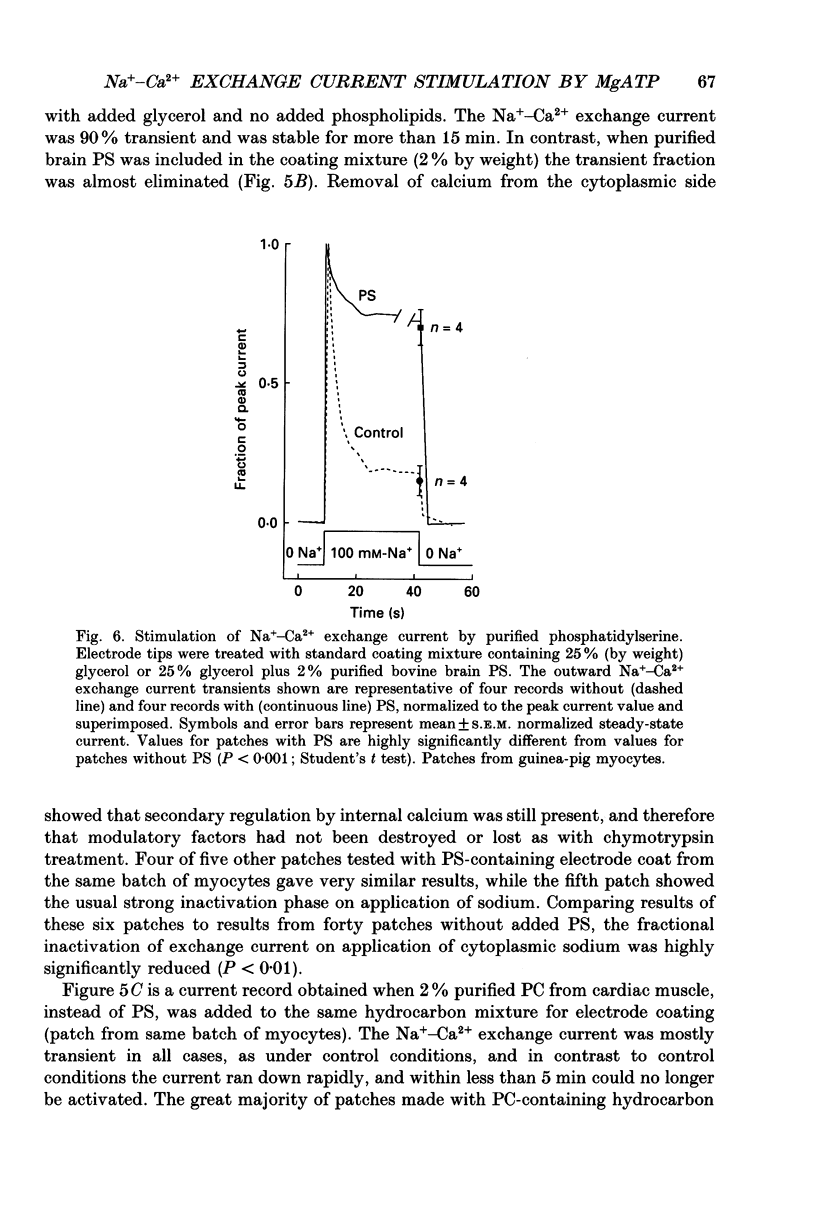
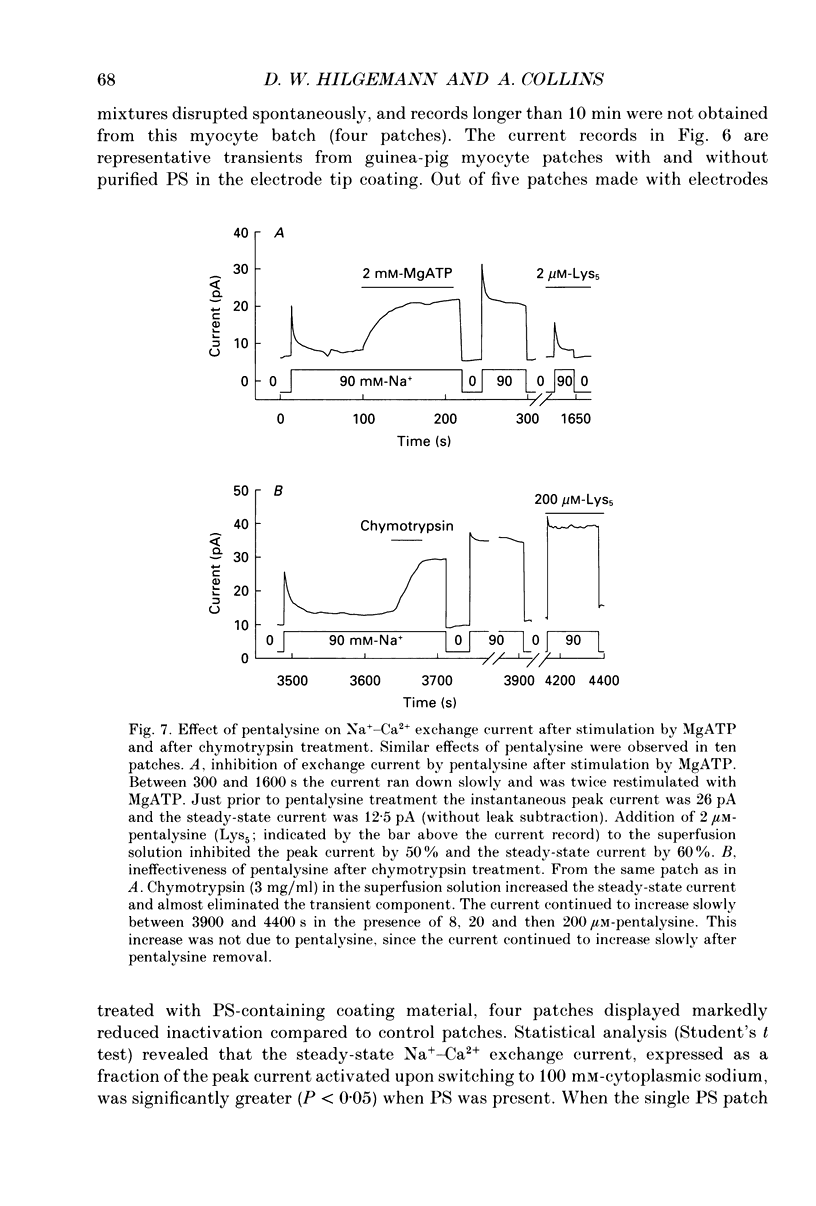
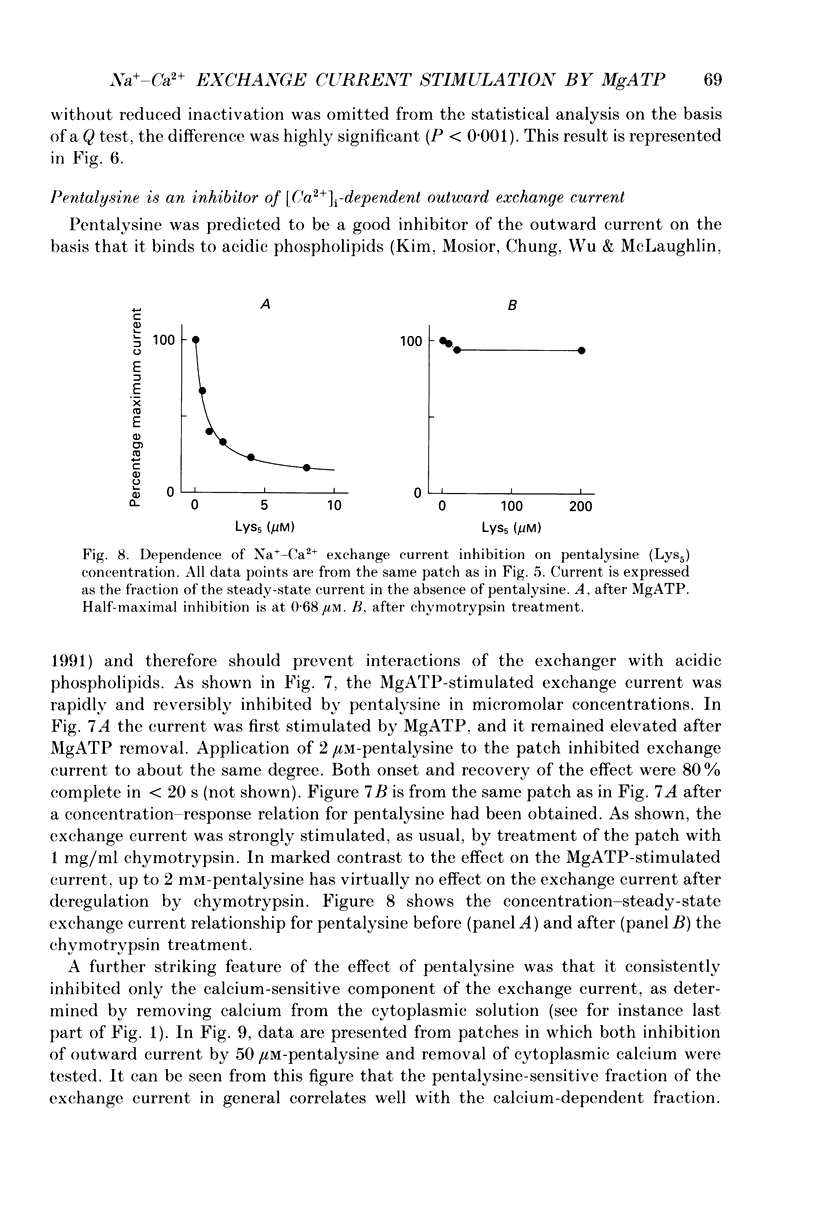
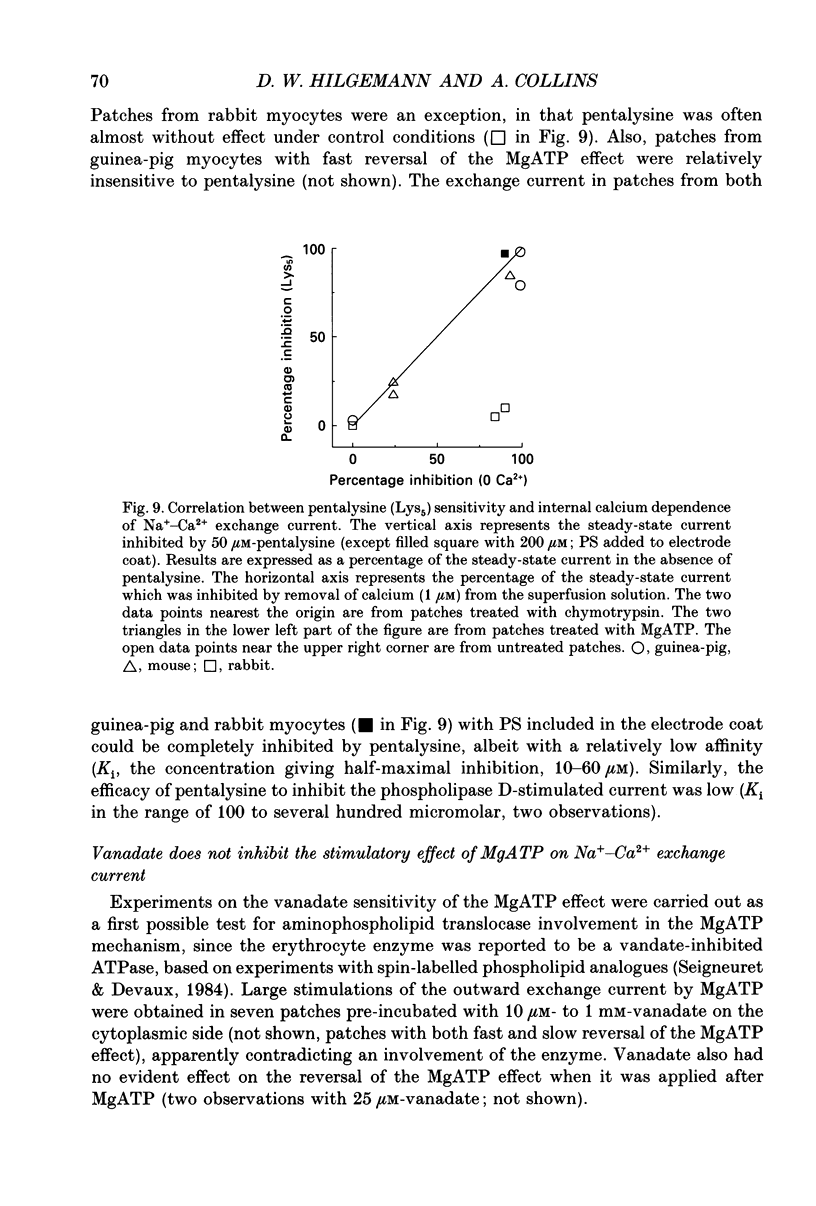
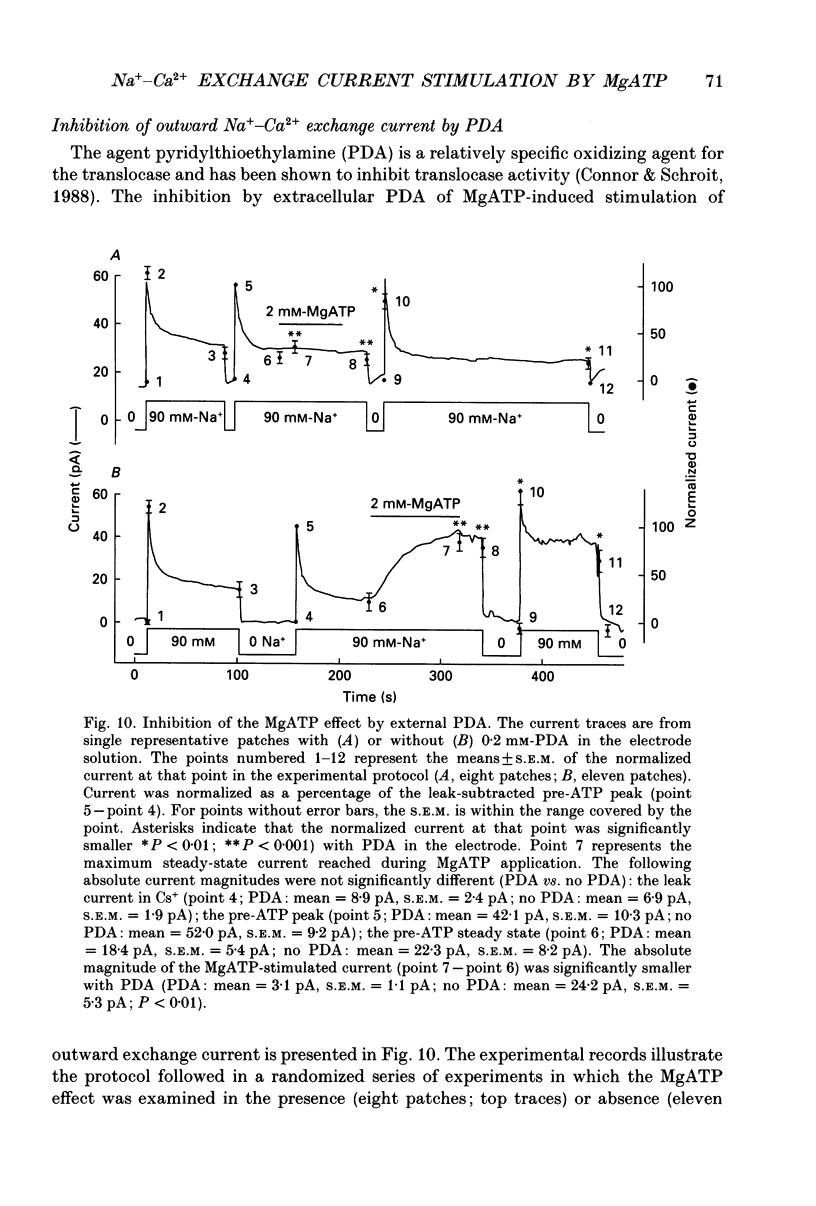
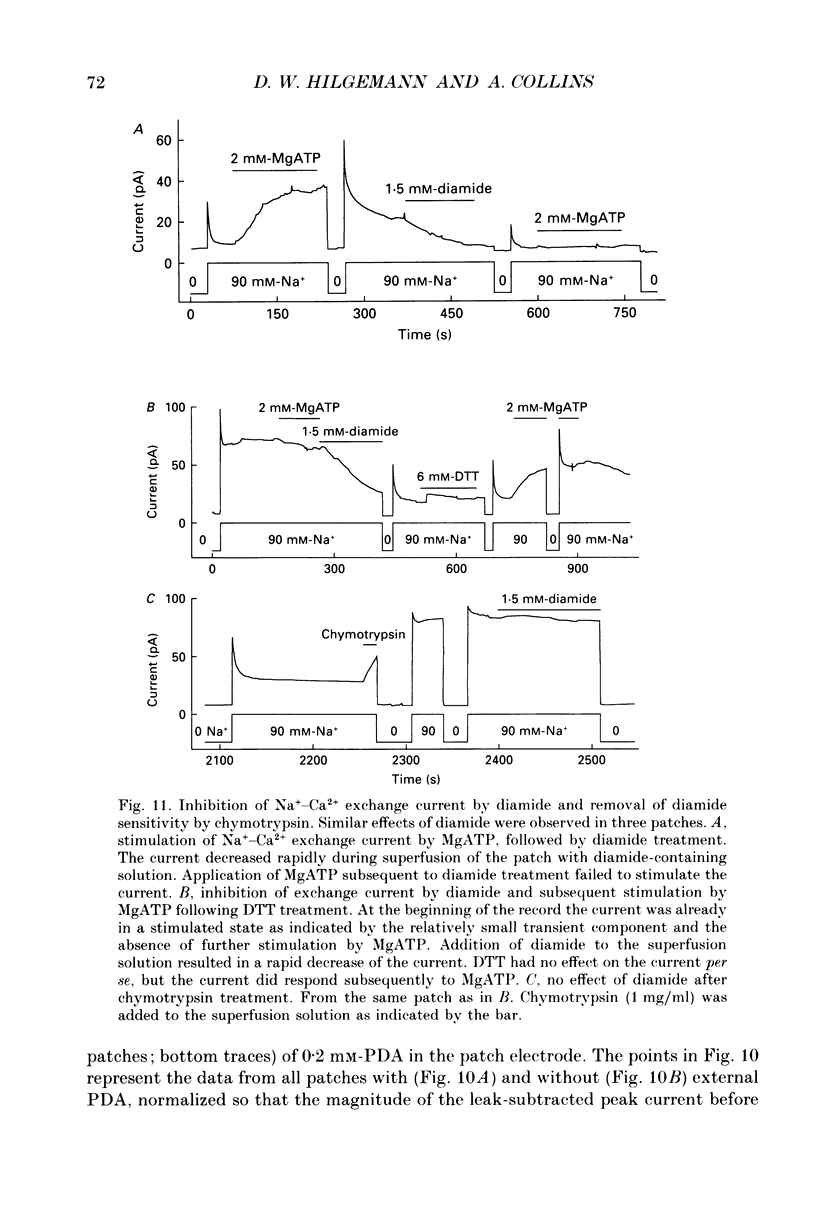
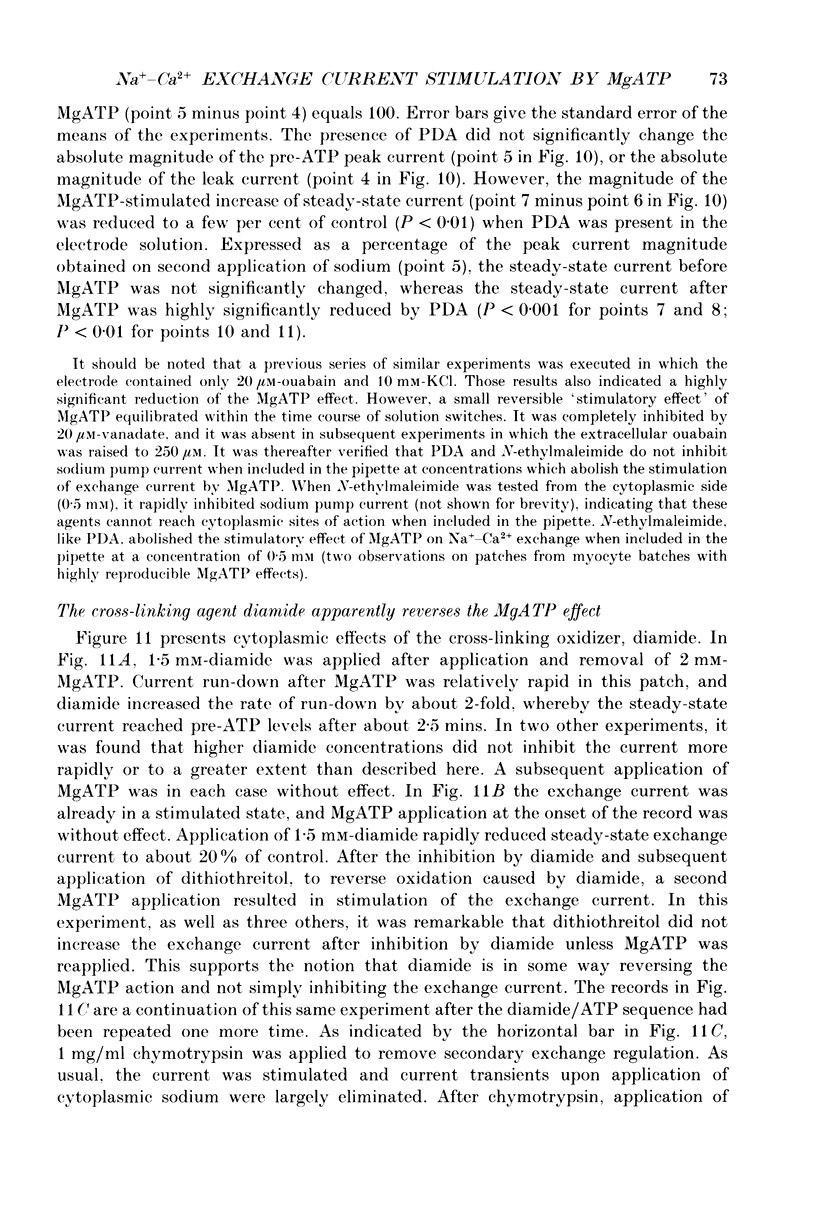
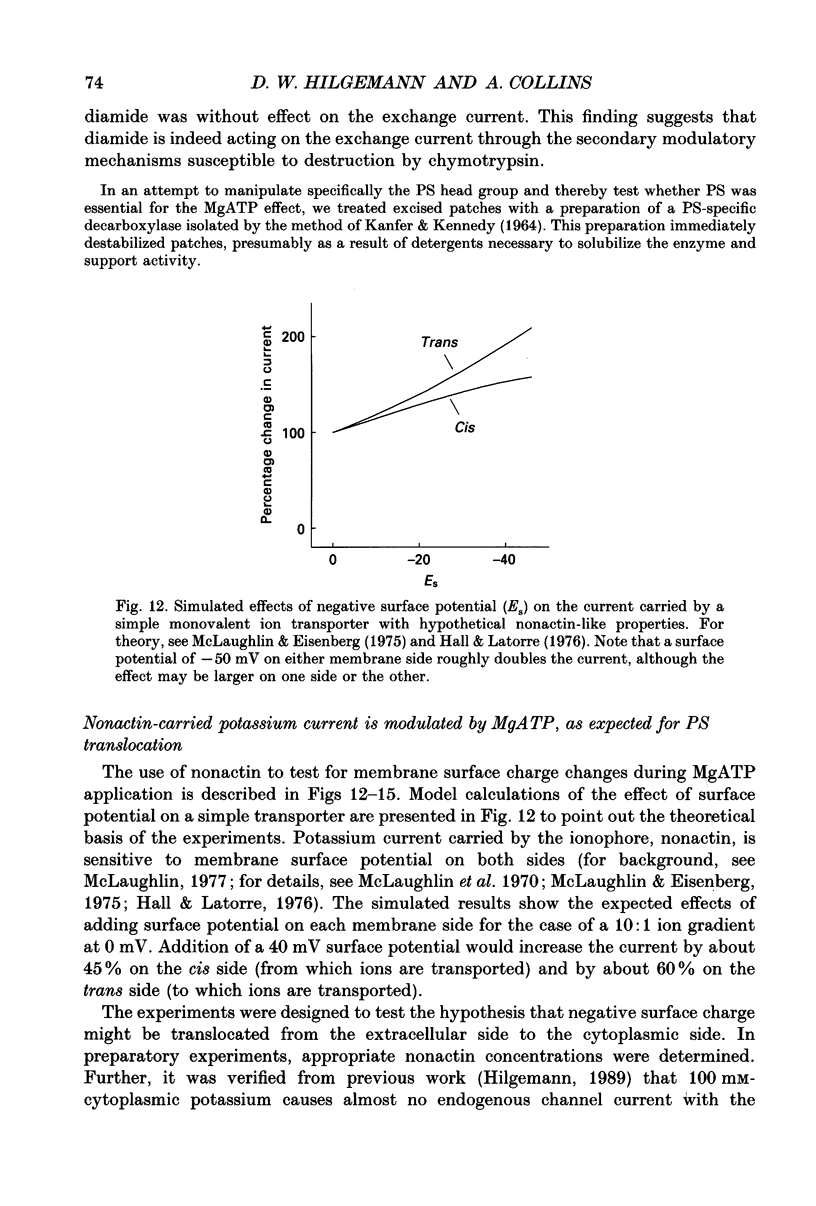
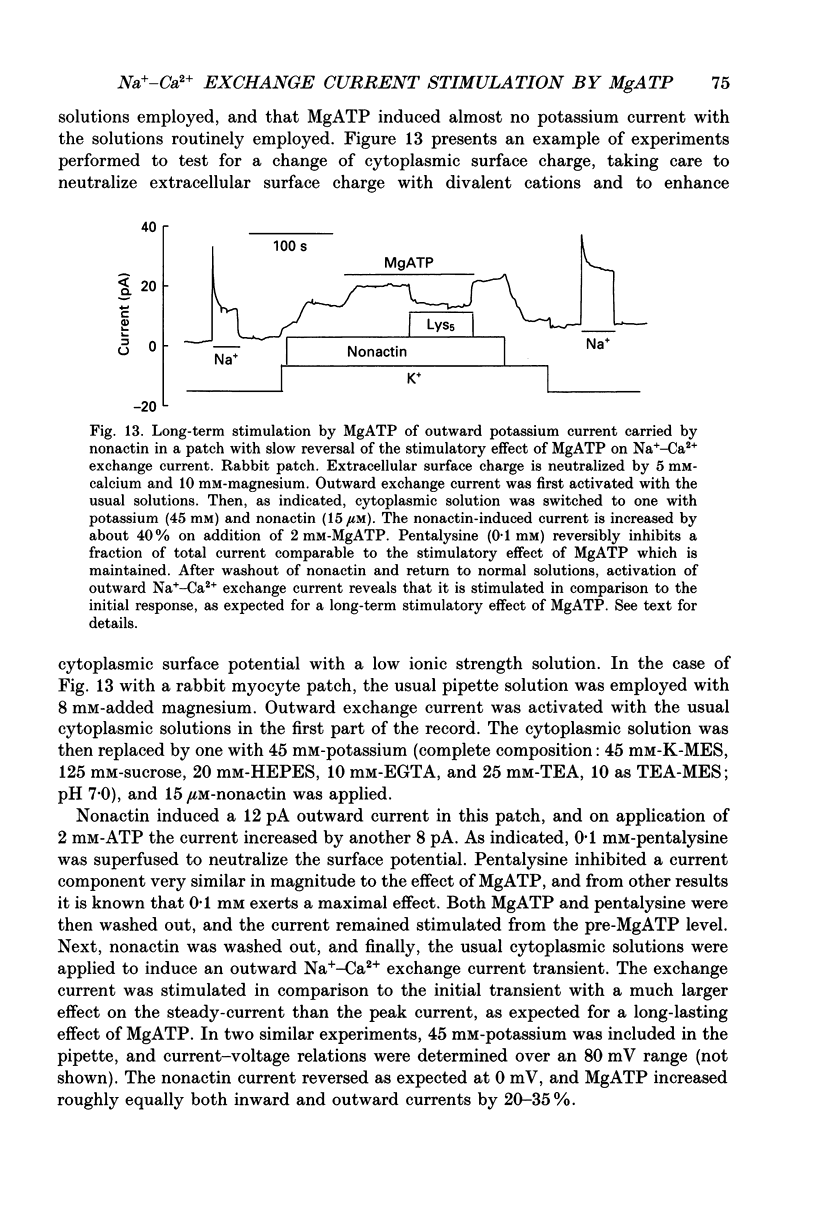
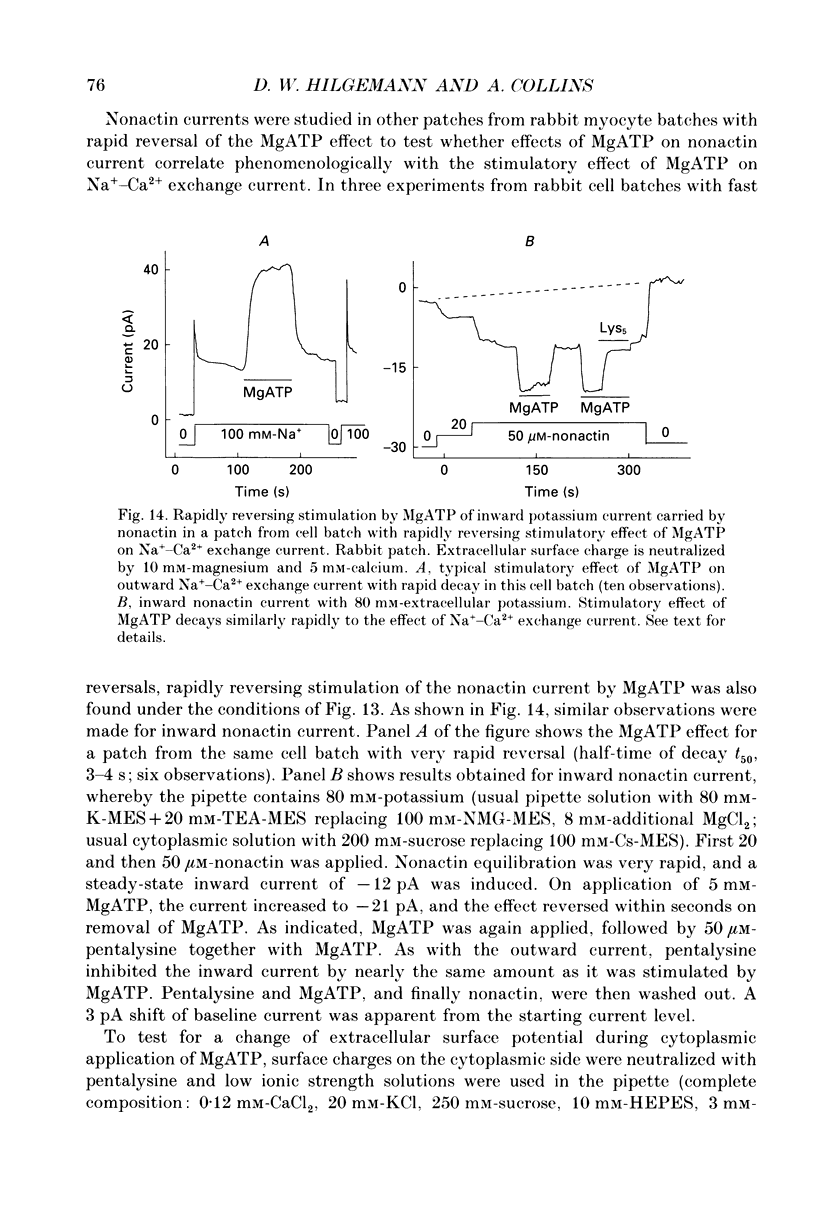
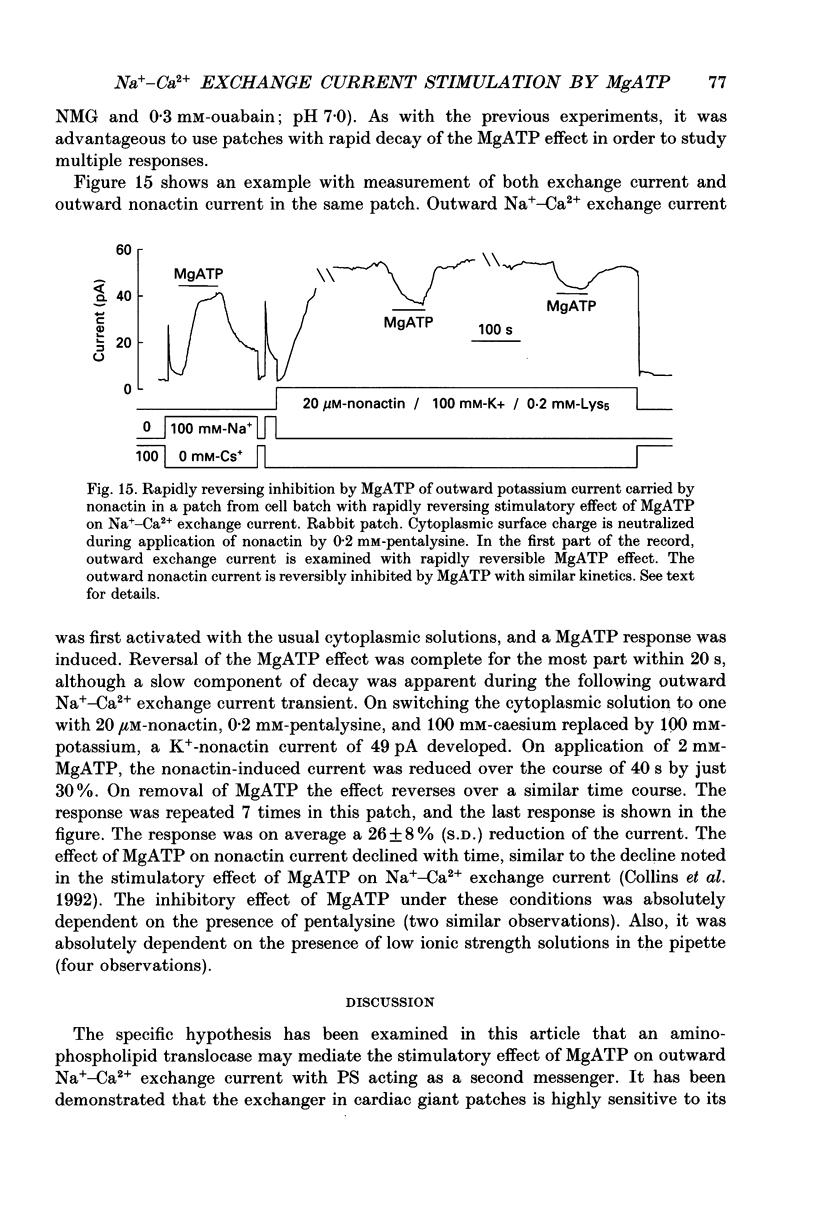
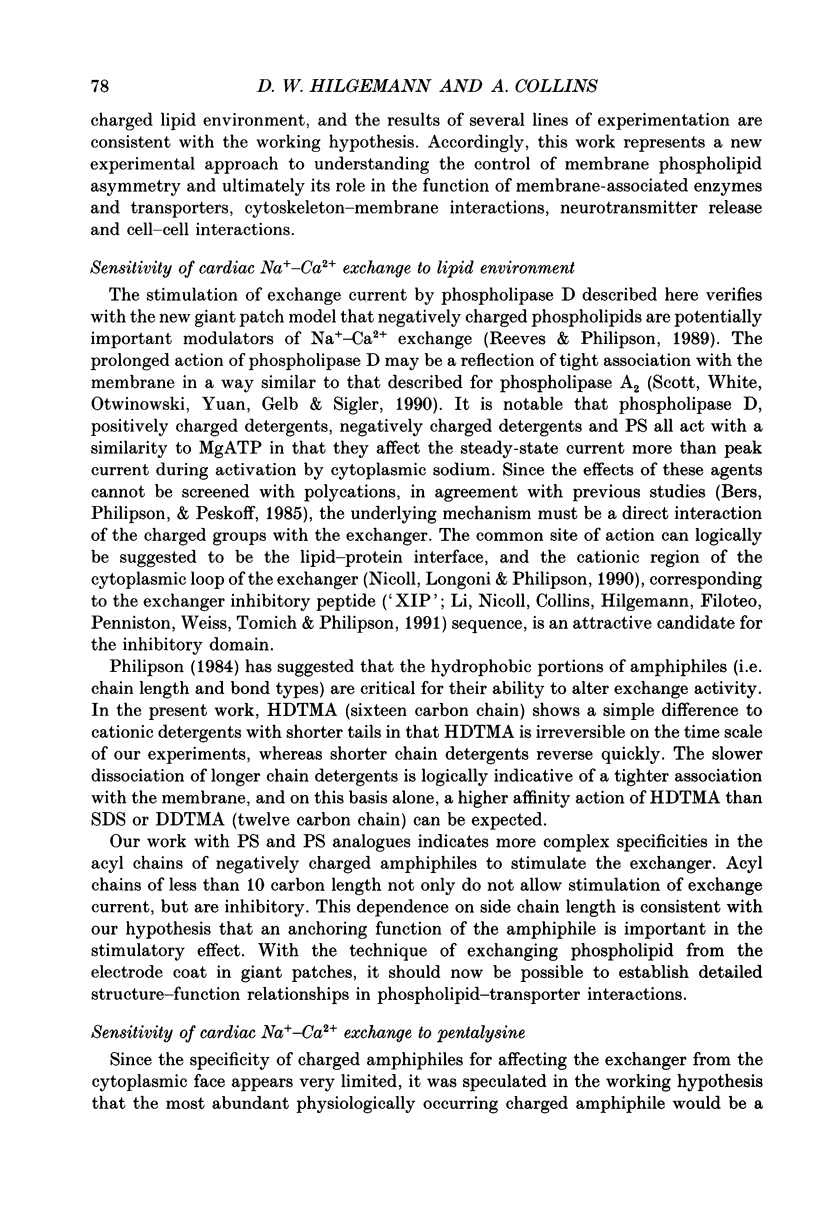
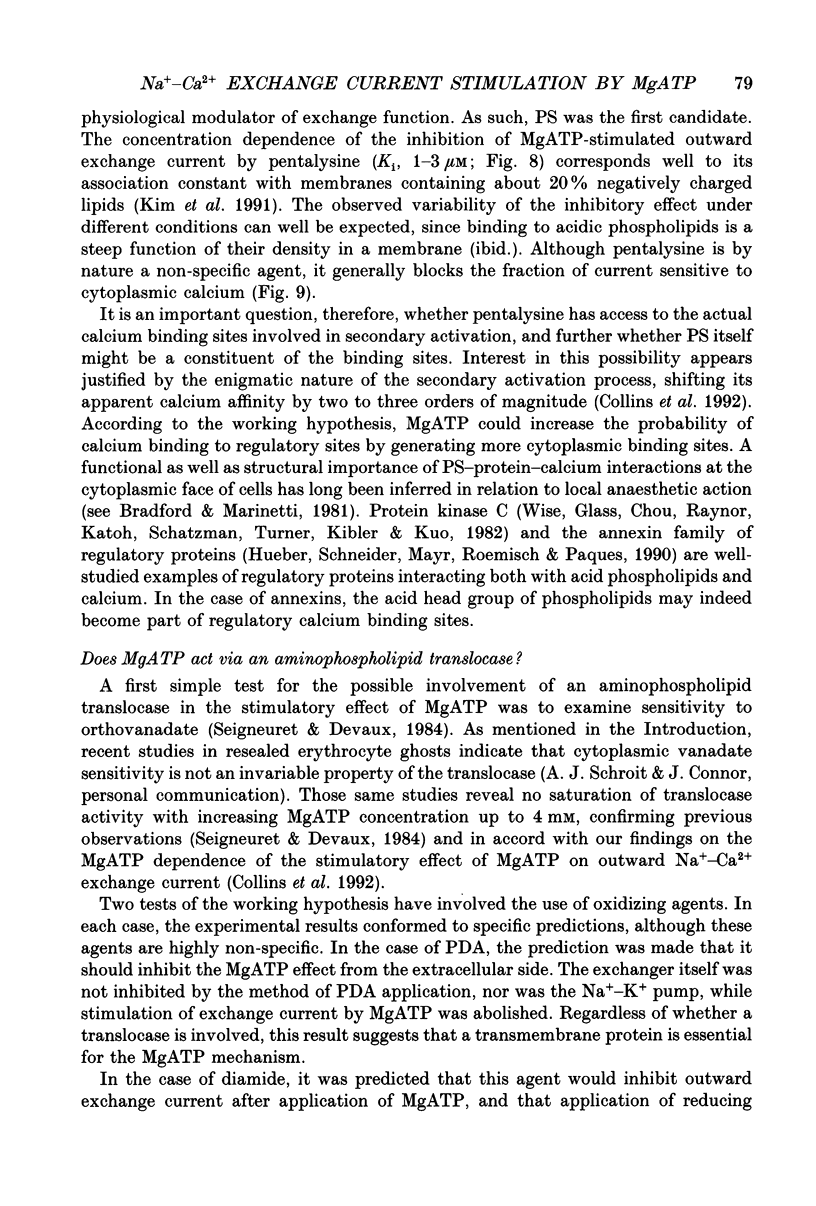
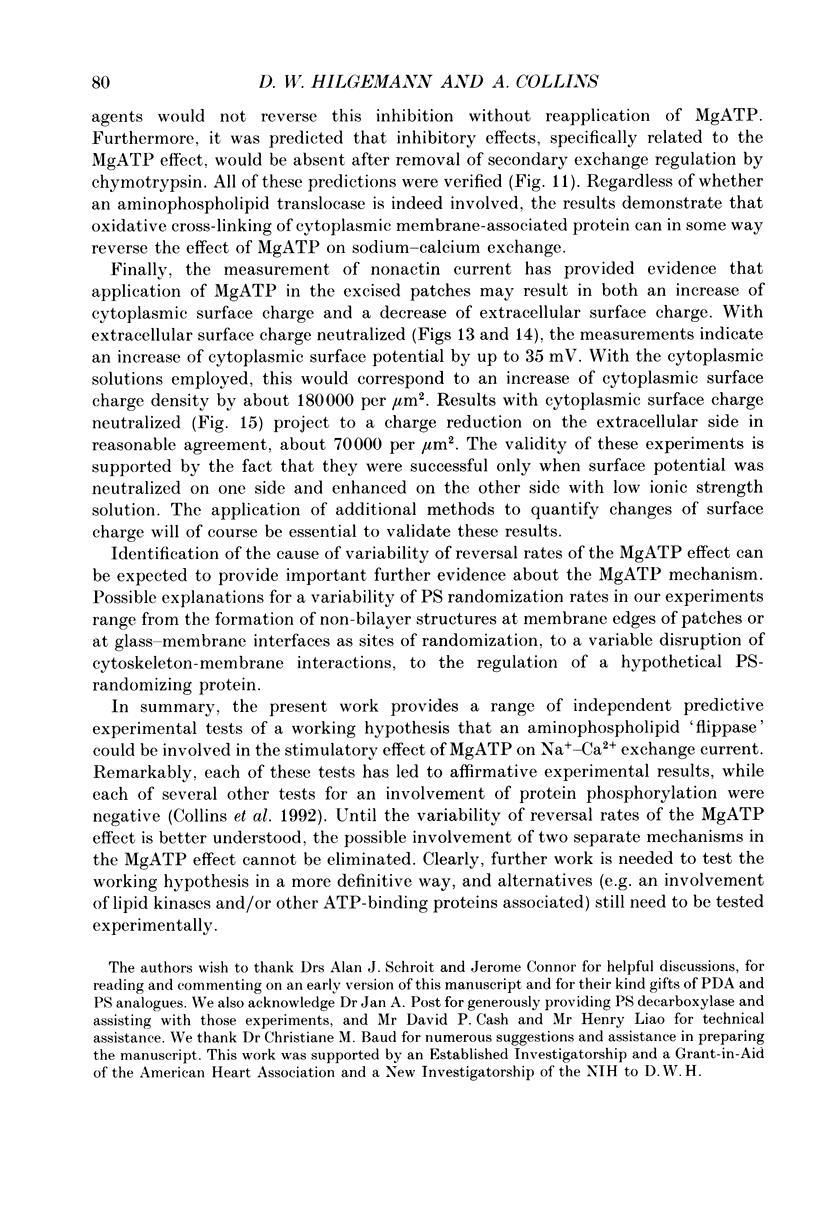
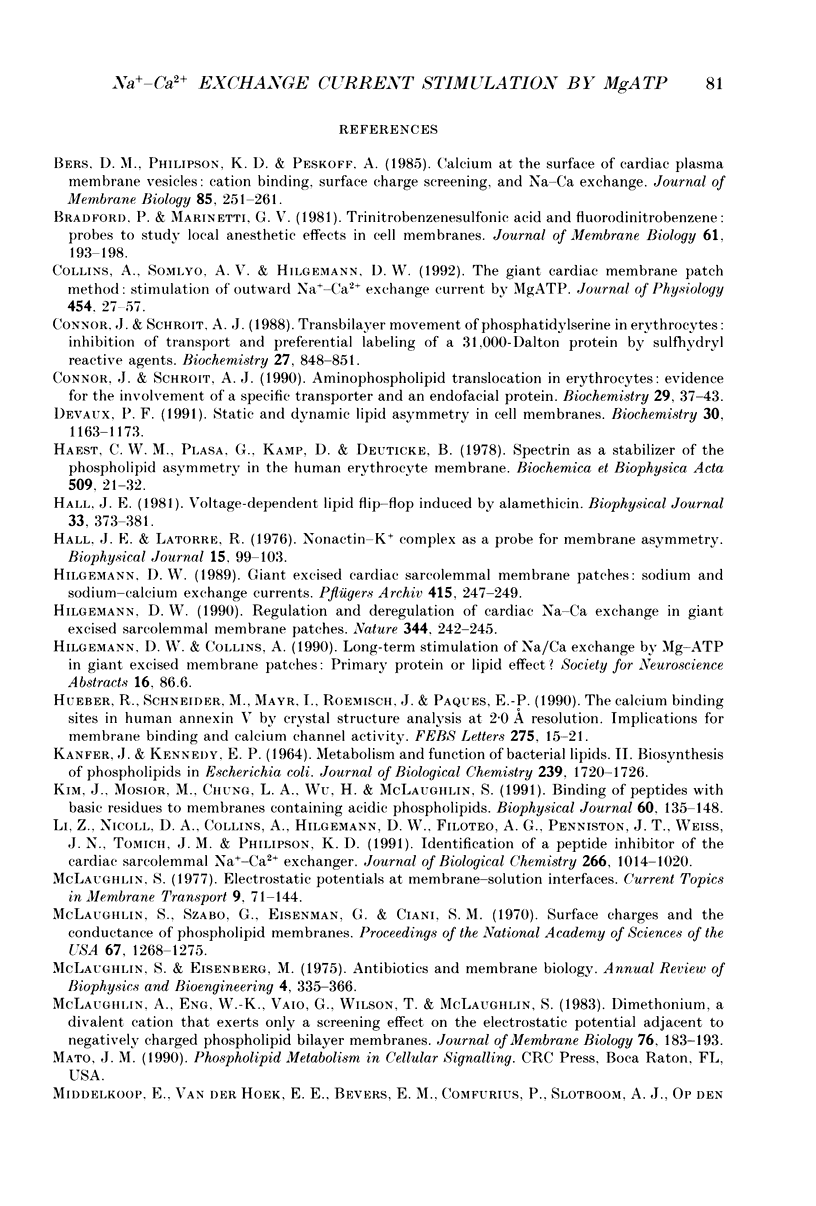
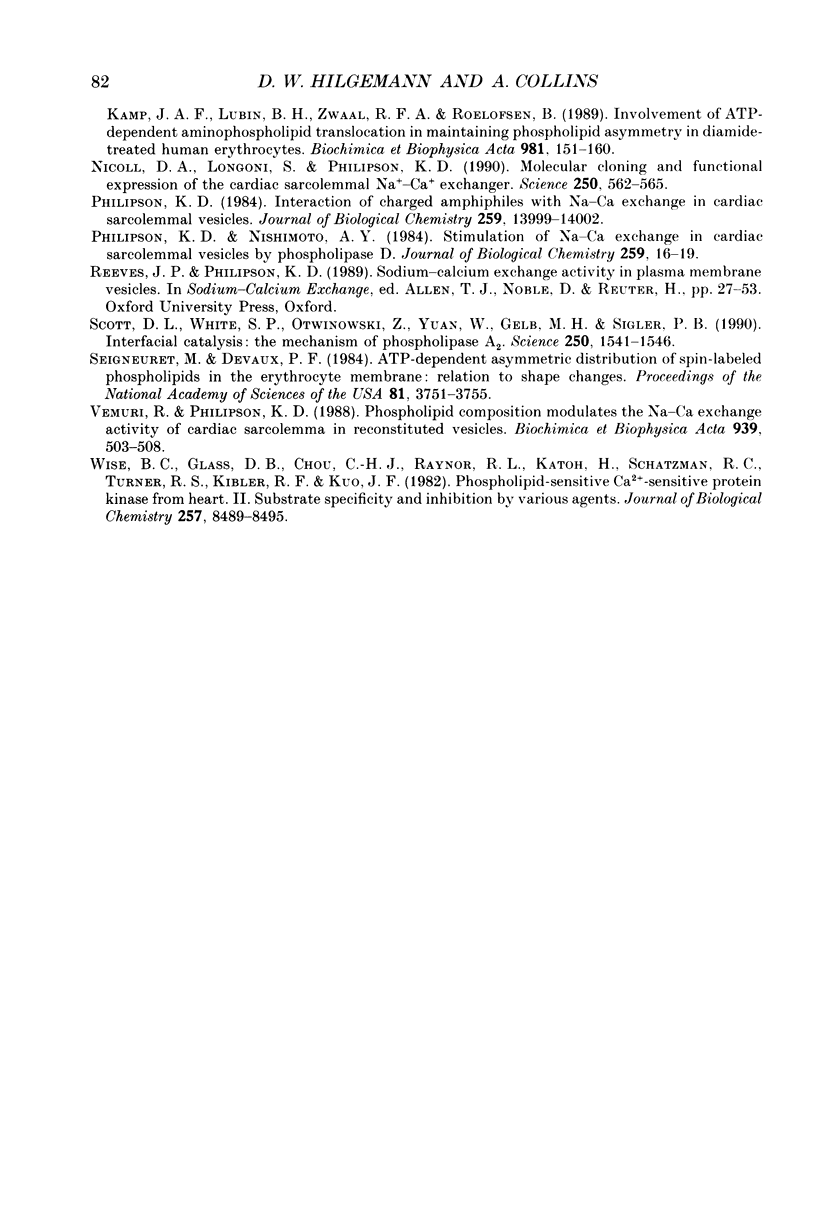
Selected References
These references are in PubMed. This may not be the complete list of references from this article.
- Bers D. M., Philipson K. D., Peskoff A. Calcium at the surface of cardiac plasma membrane vesicles: cation binding, surface charge screening, and Na-Ca exchange. J Membr Biol. 1985;85(3):251–261. doi: 10.1007/BF01871520. [DOI] [PubMed] [Google Scholar]
- Bradford P., Marinetti G. V. Trinitrobenzenesulfonic acid and fluorodinitrobenzene: probes to study local anesthetic effects in cell membranes. J Membr Biol. 1981;61(3):193–198. doi: 10.1007/BF01870523. [DOI] [PubMed] [Google Scholar]
- Collins A., Somlyo A. V., Hilgemann D. W. The giant cardiac membrane patch method: stimulation of outward Na(+)-Ca2+ exchange current by MgATP. J Physiol. 1992 Aug;454:27–57. doi: 10.1113/jphysiol.1992.sp019253. [DOI] [PMC free article] [PubMed] [Google Scholar]
- Connor J., Schroit A. J. Aminophospholipid translocation in erythrocytes: evidence for the involvement of a specific transporter and an endofacial protein. Biochemistry. 1990 Jan 9;29(1):37–43. doi: 10.1021/bi00453a005. [DOI] [PubMed] [Google Scholar]
- Connor J., Schroit A. J. Transbilayer movement of phosphatidylserine in erythrocytes: inhibition of transport and preferential labeling of a 31,000-dalton protein by sulfhydryl reactive reagents. Biochemistry. 1988 Feb 9;27(3):848–851. doi: 10.1021/bi00403a002. [DOI] [PubMed] [Google Scholar]
- Devaux P. F. Static and dynamic lipid asymmetry in cell membranes. Biochemistry. 1991 Feb 5;30(5):1163–1173. doi: 10.1021/bi00219a001. [DOI] [PubMed] [Google Scholar]
- Haest C. W., Plasa G., Kamp D., Deuticke B. Spectrin as a stabilizer of the phospholipid asymmetry in the human erythrocyte membrane. Biochim Biophys Acta. 1978 May 4;509(1):21–32. doi: 10.1016/0005-2736(78)90004-4. [DOI] [PubMed] [Google Scholar]
- Hall J. E., Latorre R. Nonactin-K+ complex as a probe for membrane asymmetry. Biophys J. 1976 Jan;16(1):99–103. doi: 10.1016/S0006-3495(76)85667-6. [DOI] [PMC free article] [PubMed] [Google Scholar]
- Hall J. E. Voltage-dependent lipid flip-flop induced by alamethicin. Biophys J. 1981 Mar;33(3):373–381. doi: 10.1016/S0006-3495(81)84901-6. [DOI] [PMC free article] [PubMed] [Google Scholar]
- Hilgemann D. W. Giant excised cardiac sarcolemmal membrane patches: sodium and sodium-calcium exchange currents. Pflugers Arch. 1989 Nov;415(2):247–249. doi: 10.1007/BF00370601. [DOI] [PubMed] [Google Scholar]
- Hilgemann D. W. Regulation and deregulation of cardiac Na(+)-Ca2+ exchange in giant excised sarcolemmal membrane patches. Nature. 1990 Mar 15;344(6263):242–245. doi: 10.1038/344242a0. [DOI] [PubMed] [Google Scholar]
- Huber R., Schneider M., Mayr I., Römisch J., Paques E. P. The calcium binding sites in human annexin V by crystal structure analysis at 2.0 A resolution. Implications for membrane binding and calcium channel activity. FEBS Lett. 1990 Nov 26;275(1-2):15–21. doi: 10.1016/0014-5793(90)81428-q. [DOI] [PubMed] [Google Scholar]
- KANFER J., KENNEDY E. P. METABOLISM AND FUNCTION OF BACTERIAL LIPIDS. II. BIOSYNTHESIS OF PHOSPHOLIPIDS IN ESCHERICHIA COLI. J Biol Chem. 1964 Jun;239:1720–1726. [PubMed] [Google Scholar]
- Kim J., Mosior M., Chung L. A., Wu H., McLaughlin S. Binding of peptides with basic residues to membranes containing acidic phospholipids. Biophys J. 1991 Jul;60(1):135–148. doi: 10.1016/S0006-3495(91)82037-9. [DOI] [PMC free article] [PubMed] [Google Scholar]
- Li Z., Nicoll D. A., Collins A., Hilgemann D. W., Filoteo A. G., Penniston J. T., Weiss J. N., Tomich J. M., Philipson K. D. Identification of a peptide inhibitor of the cardiac sarcolemmal Na(+)-Ca2+ exchanger. J Biol Chem. 1991 Jan 15;266(2):1014–1020. [PubMed] [Google Scholar]
- McLaughlin A., Eng W. K., Vaio G., Wilson T., McLaughlin S. Dimethonium, a divalent cation that exerts only a screening effect on the electrostatic potential adjacent to negatively charged phospholipid bilayer membranes. J Membr Biol. 1983;76(2):183–193. doi: 10.1007/BF02000618. [DOI] [PubMed] [Google Scholar]
- McLaughlin S. G., Szabo G., Eisenman G., Ciani S. M. Surface charge and the conductance of phospholipid membranes. Proc Natl Acad Sci U S A. 1970 Nov;67(3):1268–1275. doi: 10.1073/pnas.67.3.1268. [DOI] [PMC free article] [PubMed] [Google Scholar]
- McLaughlin S., Eisenberg M. Antibiotics and membrane biology. Annu Rev Biophys Bioeng. 1975;4(00):335–366. doi: 10.1146/annurev.bb.04.060175.002003. [DOI] [PubMed] [Google Scholar]
- Middlekoop E., Van der Hoek E. E., Bevers E. M., Comfurius P., Slotboom A. J., Op den Kamp J. A., Lubin B. H., Zwaal R. F., Roelofsen B. Involvement of ATP-dependent aminophospholipid translocation in maintaining phospholipid asymmetry in diamide-treated human erythrocytes. Biochim Biophys Acta. 1989 May 19;981(1):151–160. doi: 10.1016/0005-2736(89)90093-x. [DOI] [PubMed] [Google Scholar]
- Nicoll D. A., Longoni S., Philipson K. D. Molecular cloning and functional expression of the cardiac sarcolemmal Na(+)-Ca2+ exchanger. Science. 1990 Oct 26;250(4980):562–565. doi: 10.1126/science.1700476. [DOI] [PubMed] [Google Scholar]
- Philipson K. D. Interaction of charged amphiphiles with Na+-Ca2+ exchange in cardiac sarcolemmal vesicles. J Biol Chem. 1984 Nov 25;259(22):13999–14002. [PubMed] [Google Scholar]
- Philipson K. D., Nishimoto A. Y. Stimulation of Na+-Ca2+ exchange in cardiac sarcolemmal vesicles by phospholipase D. J Biol Chem. 1984 Jan 10;259(1):16–19. [PubMed] [Google Scholar]
- Scott D. L., White S. P., Otwinowski Z., Yuan W., Gelb M. H., Sigler P. B. Interfacial catalysis: the mechanism of phospholipase A2. Science. 1990 Dec 14;250(4987):1541–1546. doi: 10.1126/science.2274785. [DOI] [PMC free article] [PubMed] [Google Scholar]
- Seigneuret M., Devaux P. F. ATP-dependent asymmetric distribution of spin-labeled phospholipids in the erythrocyte membrane: relation to shape changes. Proc Natl Acad Sci U S A. 1984 Jun;81(12):3751–3755. doi: 10.1073/pnas.81.12.3751. [DOI] [PMC free article] [PubMed] [Google Scholar]
- Vemuri R., Philipson K. D. Protein methylation inhibits Na+-Ca2+ exchange activity in cardiac sarcolemmal vesicles. Biochim Biophys Acta. 1988 Apr 22;939(3):503–508. doi: 10.1016/0005-2736(88)90097-1. [DOI] [PubMed] [Google Scholar]
- Wise B. C., Glass D. B., Chou C. H., Raynor R. L., Katoh N., Schatzman R. C., Turner R. S., Kibler R. F., Kuo J. F. Phospholipid-sensitive Ca2+-dependent protein kinase from heart. II. Substrate specificity and inhibition by various agents. J Biol Chem. 1982 Jul 25;257(14):8489–8495. [PubMed] [Google Scholar]