Abstract
1. The Vaseline-gap technique was used to record calcium currents (ICa) and charge movement in single cut fibres from normal human muscle. Experiments were carried out in 2 or 10 mM-extracellular Ca2+ concentration ([Ca2+]o) and at 17 or 27 degrees C. 2. The passive electrical properties of the fibres with this technique were: membrane resistance for unit length rm = 59.4 k omega cm; longitudinal resistance per unit length ri = 4.9 M omega/cm; longitudinal resistance per unit length under the Vaseline seals re = 438 M omega/cm; specific membrane resistance Rm = 1.176 k omega cm2; input capacitance = 5.53 nF; specific membrane capacitance = 8.9 microF/cm2. 3. The maximum amplitude of ICa at 17 degrees C was: in 2 mM [Ca2+]o, -0.42 microA/microF and in 10 mM [Ca2+]o, -1.44 microA/microF. At 27 degrees C and in 10 mM [Ca2+]o, it increased to -3.04 microA/microF. The calculated temperature coefficient (Q10) for the increase in amplitude from 17 to 27 degrees C was 2.1. 4. Ca2+ permeability (PCa) was calculated using the Goldman-Katz relation; in 2 mM [Ca2+]o at 17 degrees C, PCa = 1.26 x 10(-6) cm/s; in 10 mM [Ca2+]o at 17 degrees C, PCa = 2.23 x 10(-6) cm/s; in 10 mM [Ca2+]o at 27 degrees C, PCa = 4.03 x 10(-6) cm/s. 5. The activation curve calculated from the PCa was shifted by 10 mV to positive potentials when raising [Ca2+]o from 2 to 10 mM. Increasing the temperature did not change the curve. The mid-point potentials (Va 1/2) and steepness (k) of the activation curves were: at 17 degrees C, in 2 mM [Ca2+]o, Va 1/2 = -1.53 mV and k = 6.7 mV; in 10 mM [Ca2+]o, Va 1/2 = 9.96 mV and k = 6.8 mV; at 27 degrees C and 10 mM [Ca2+]o, Va 1/2 = 11.3 mV and k = 7.7 mV. The activation time constant in 10 mM [Ca2+]o reached a plateau at potentials positive to 10 mV, with a value of 93.8 ms at 17 degrees C and 17.4 ms at 27 degrees C. The calculated Q10 was 4.5. 6. The deactivation of the current was studied from tail currents at different membrane potentials in 10 mM [Ca2+]o.(ABSTRACT TRUNCATED AT 400 WORDS)
Full text
PDF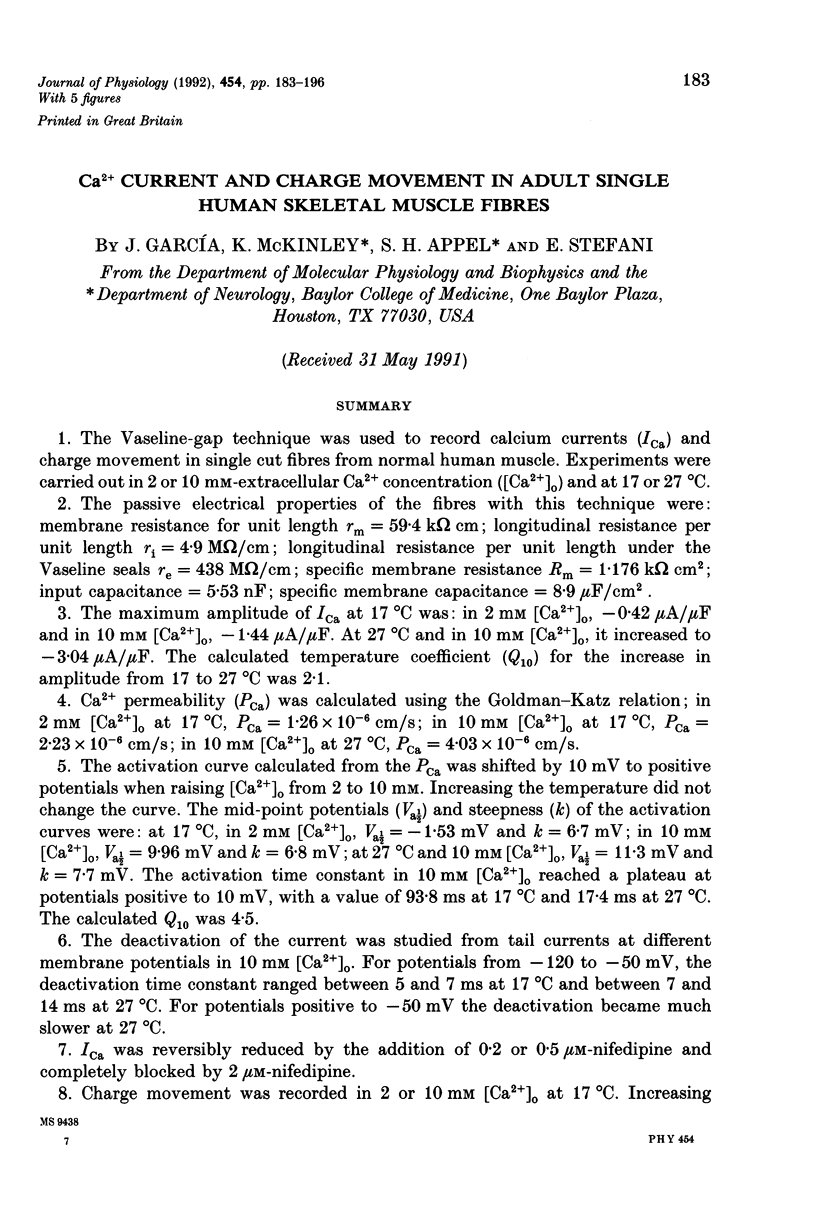
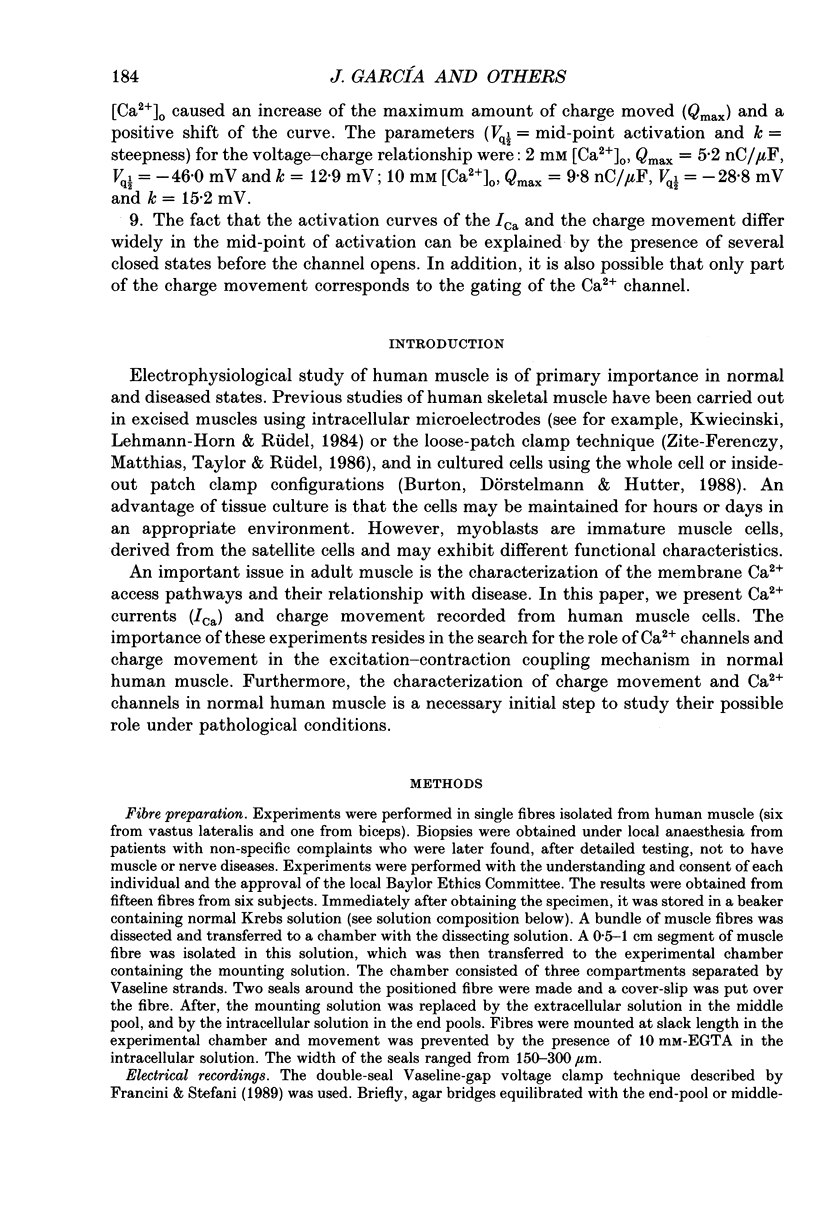
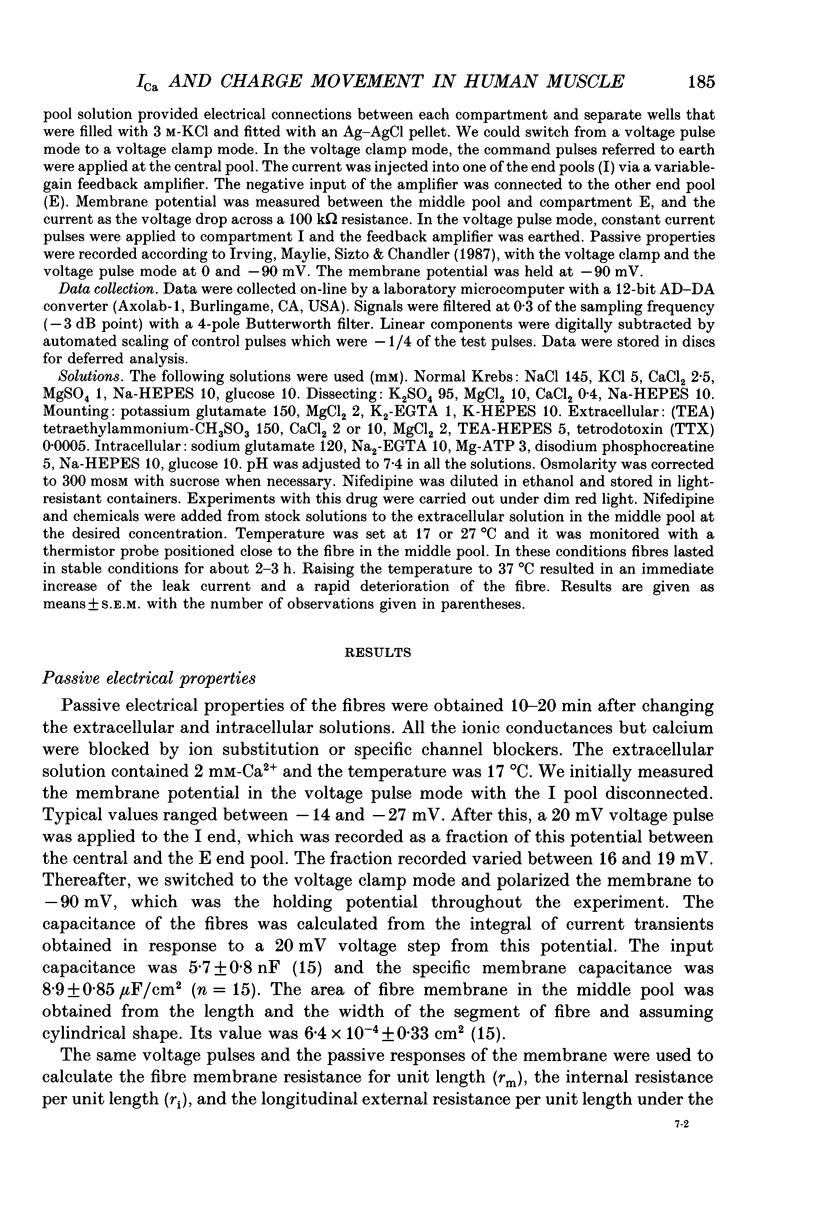
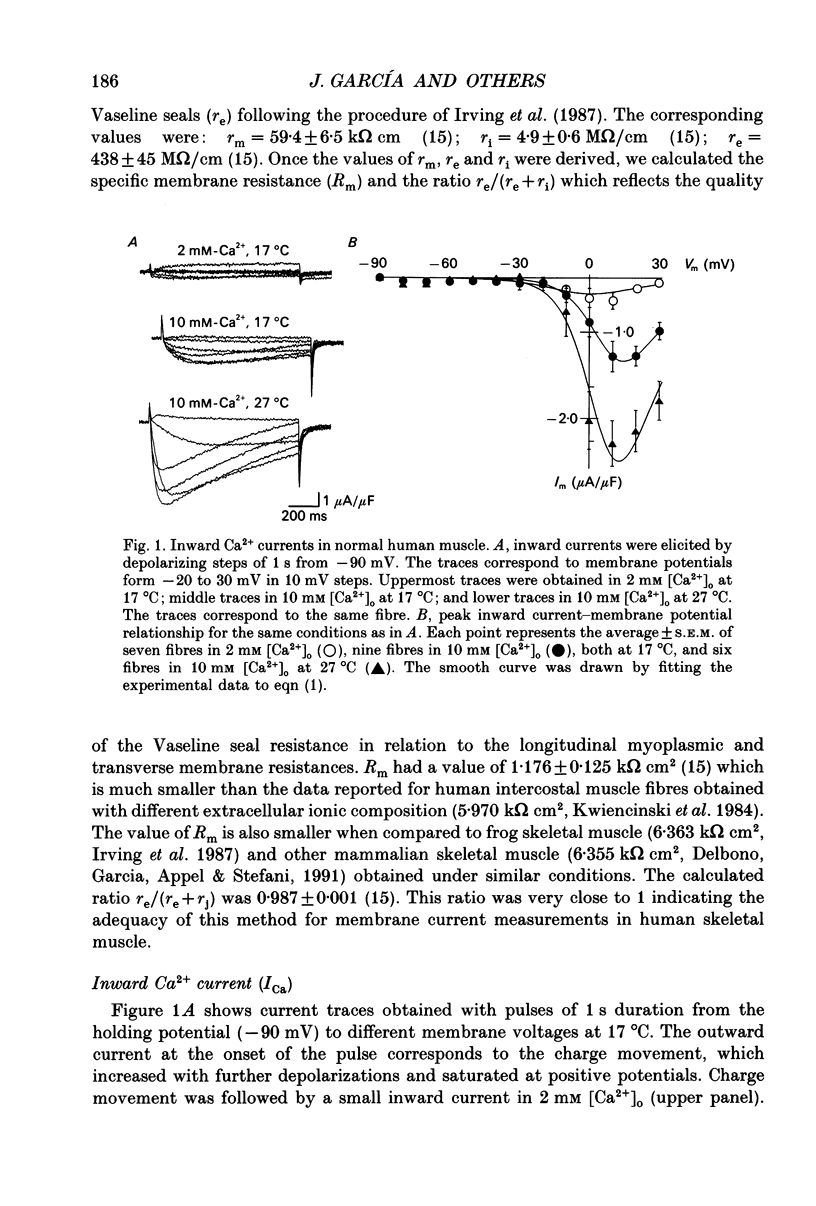
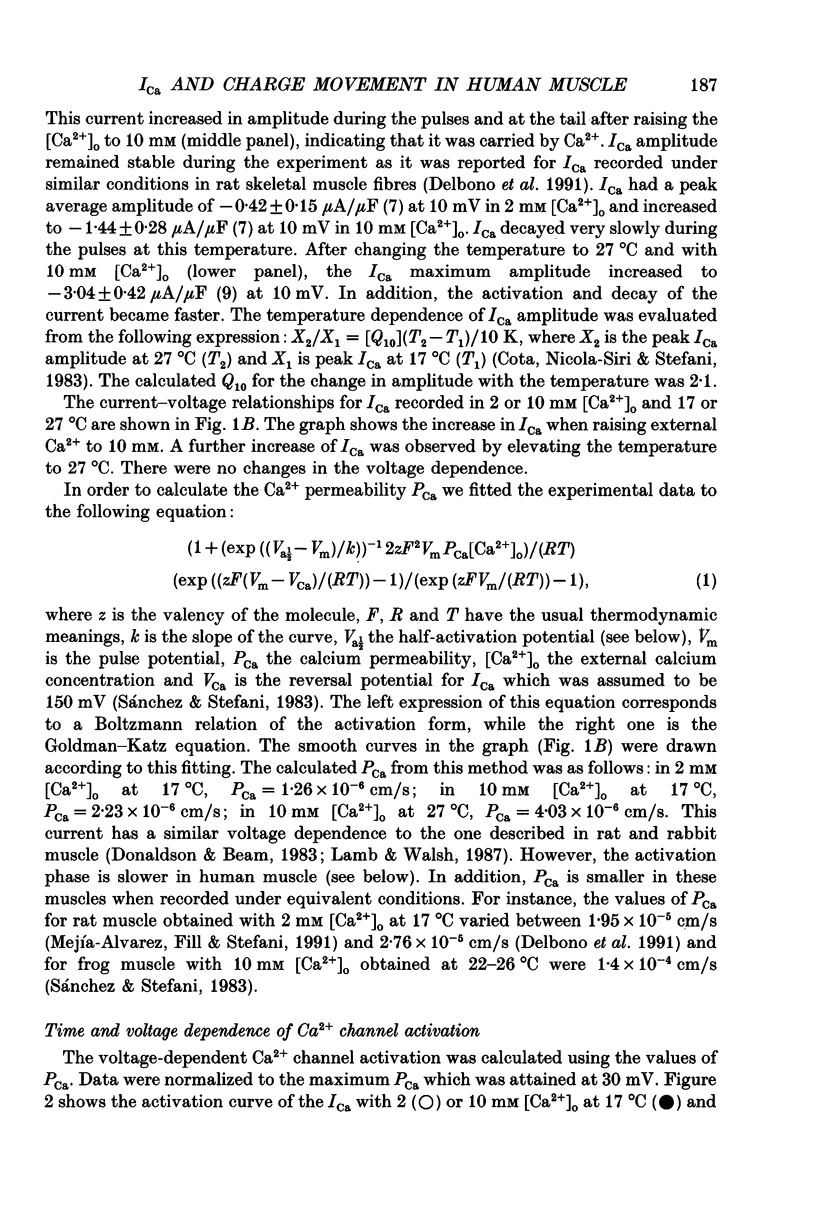
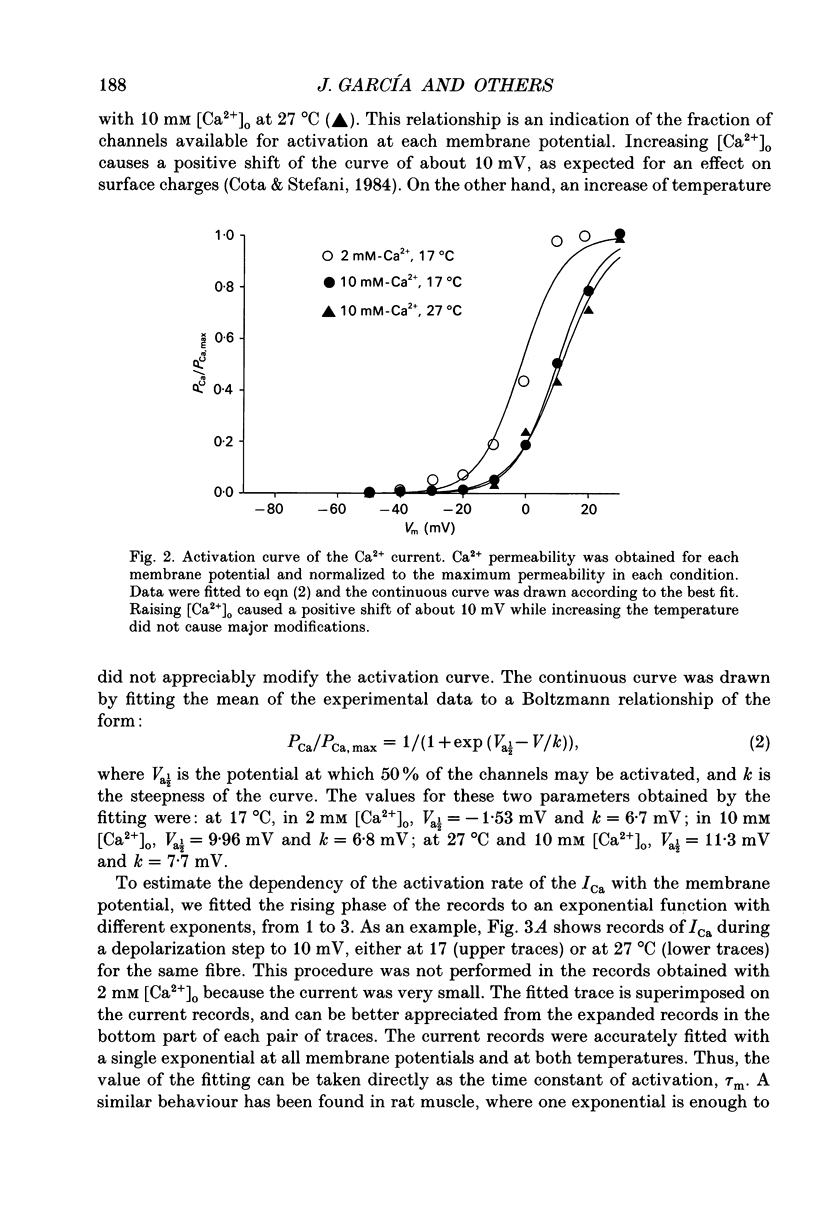
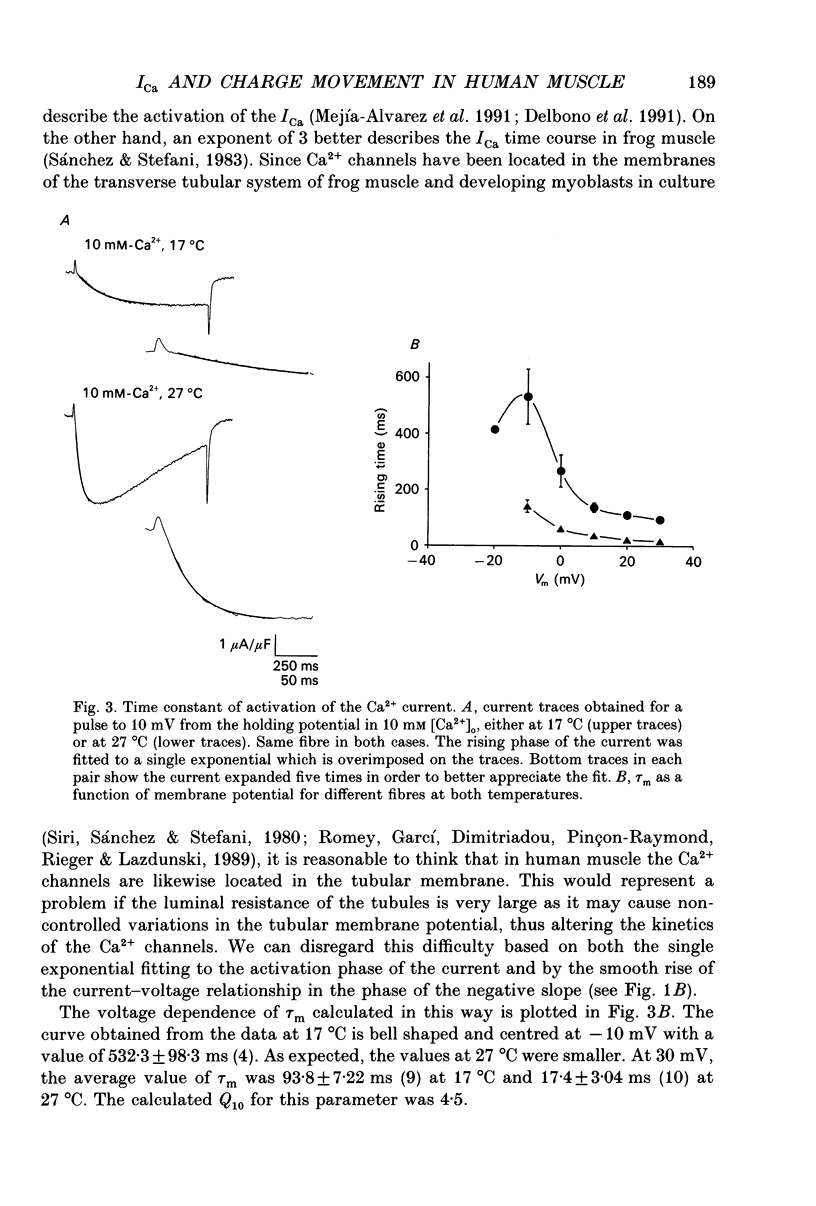
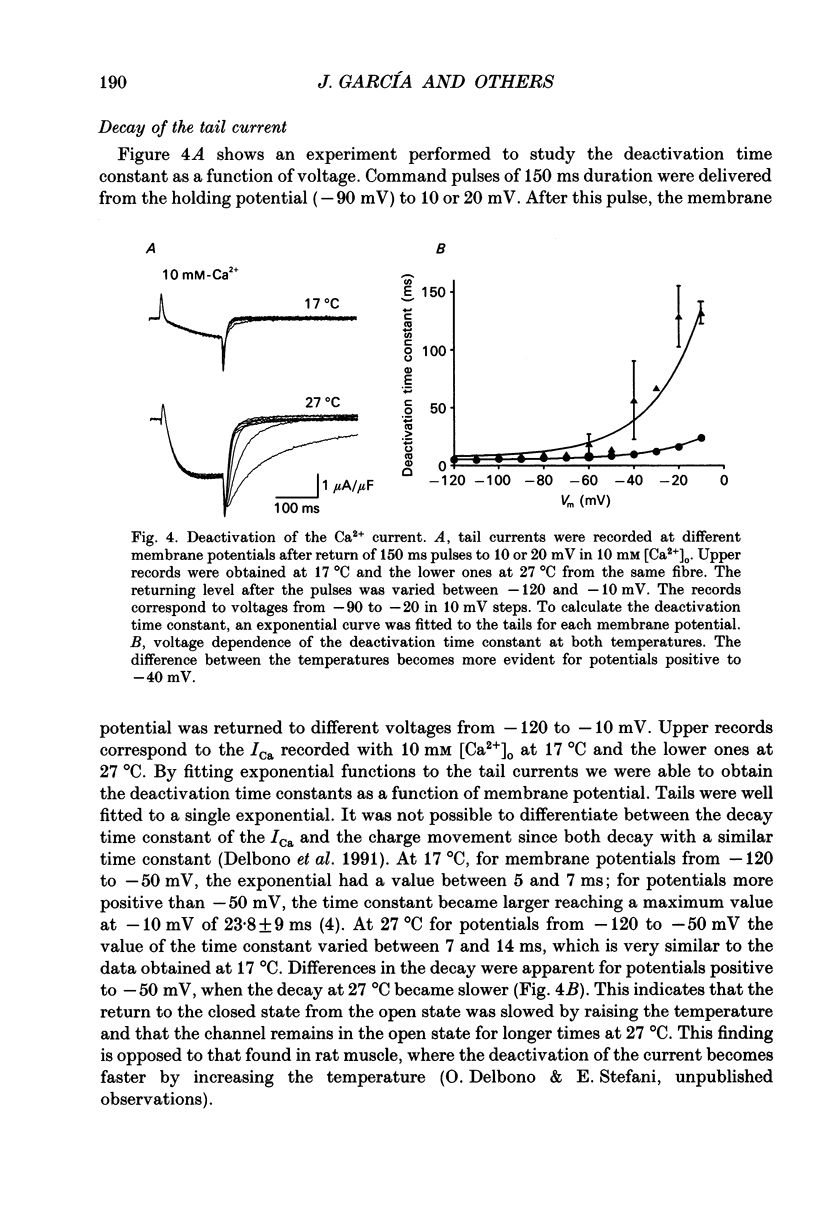
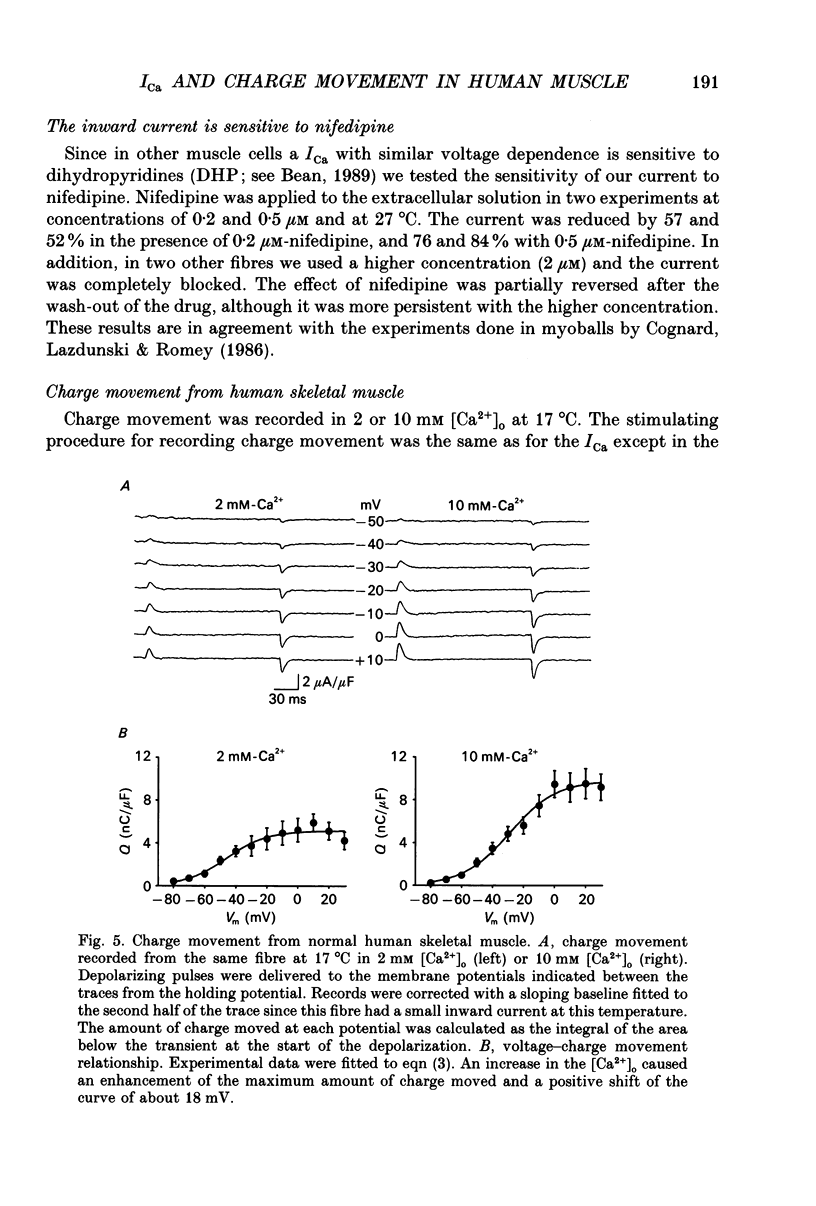
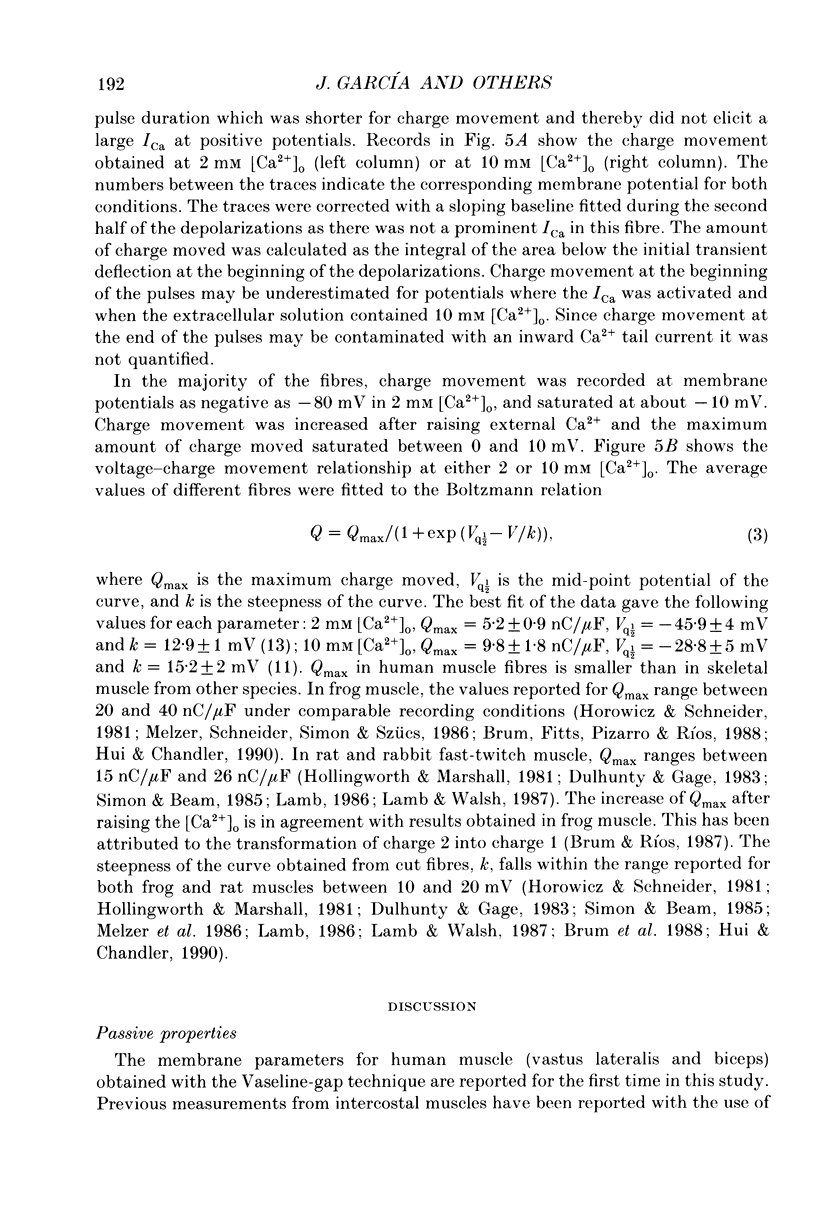
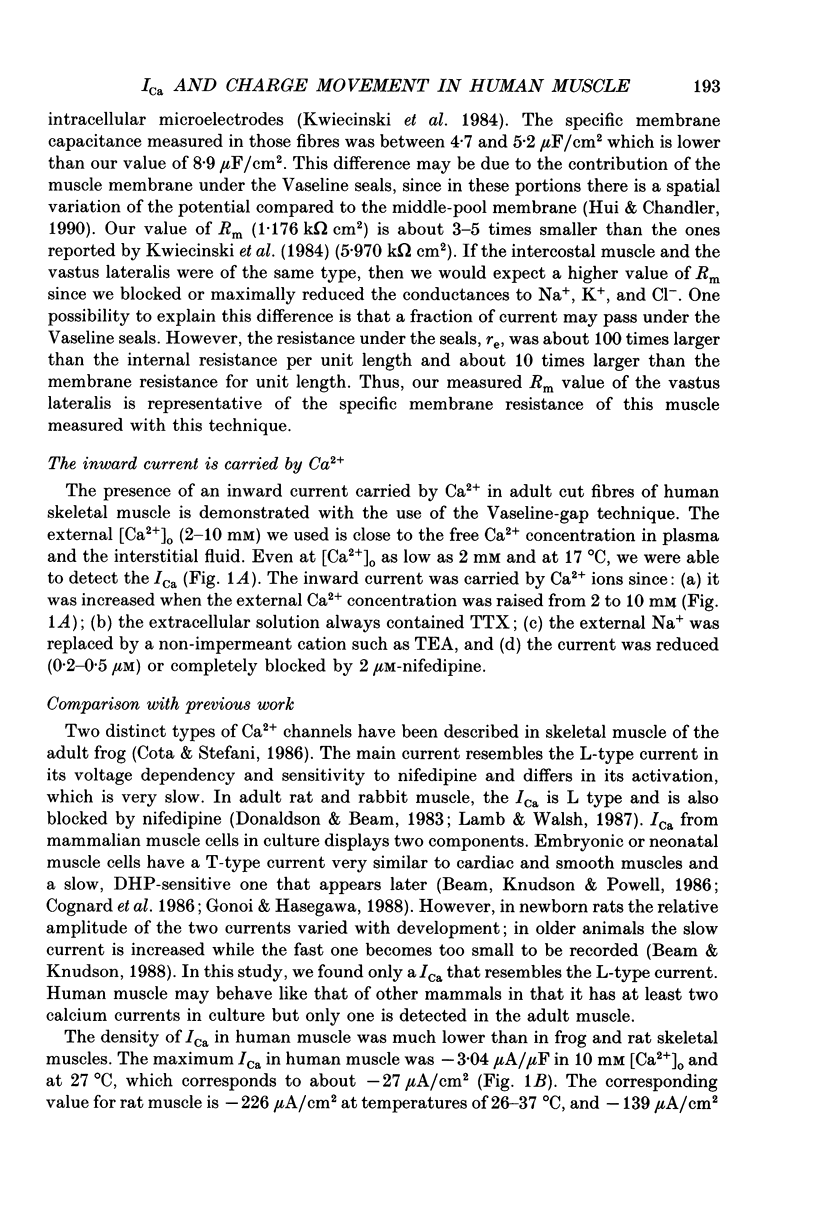
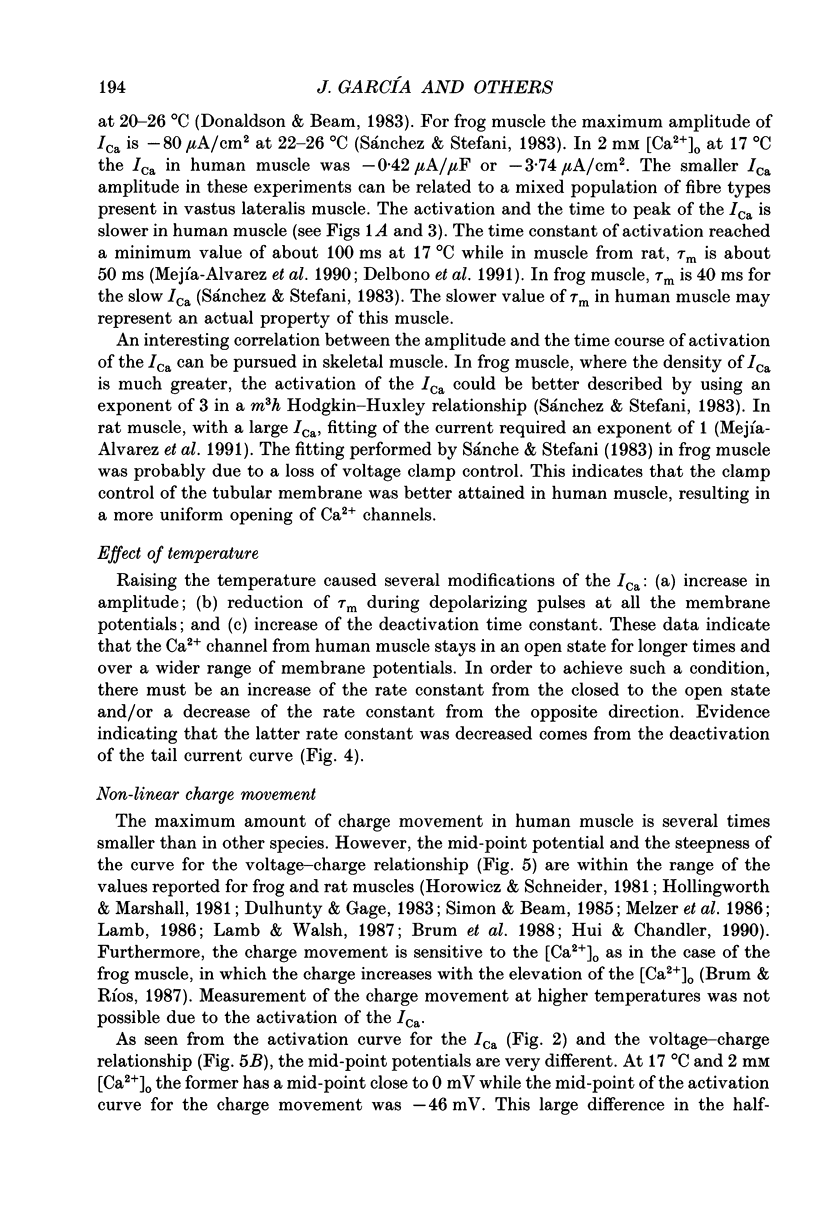
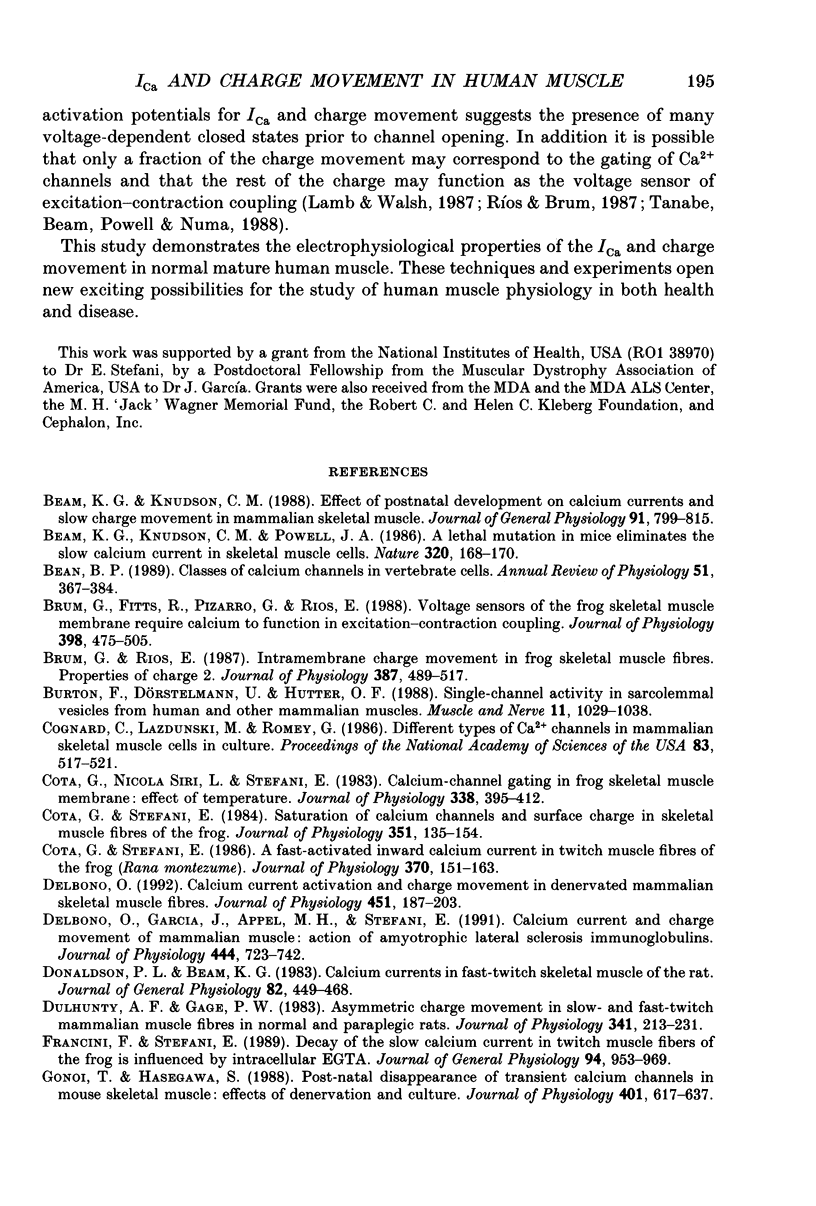
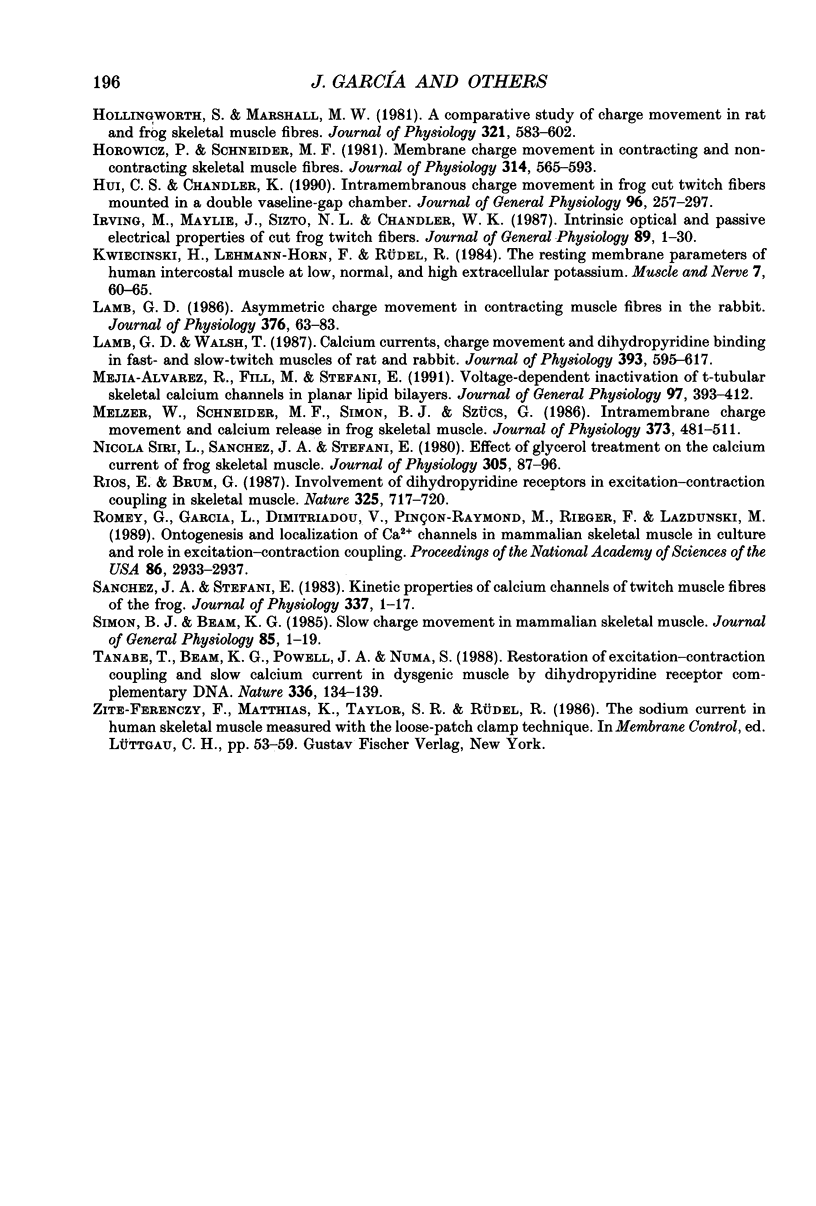
Selected References
These references are in PubMed. This may not be the complete list of references from this article.
- Beam K. G., Knudson C. M. Effect of postnatal development on calcium currents and slow charge movement in mammalian skeletal muscle. J Gen Physiol. 1988 Jun;91(6):799–815. doi: 10.1085/jgp.91.6.799. [DOI] [PMC free article] [PubMed] [Google Scholar]
- Beam K. G., Knudson C. M., Powell J. A. A lethal mutation in mice eliminates the slow calcium current in skeletal muscle cells. Nature. 1986 Mar 13;320(6058):168–170. doi: 10.1038/320168a0. [DOI] [PubMed] [Google Scholar]
- Bean B. P. Classes of calcium channels in vertebrate cells. Annu Rev Physiol. 1989;51:367–384. doi: 10.1146/annurev.ph.51.030189.002055. [DOI] [PubMed] [Google Scholar]
- Brum G., Fitts R., Pizarro G., Ríos E. Voltage sensors of the frog skeletal muscle membrane require calcium to function in excitation-contraction coupling. J Physiol. 1988 Apr;398:475–505. doi: 10.1113/jphysiol.1988.sp017053. [DOI] [PMC free article] [PubMed] [Google Scholar]
- Brum G., Rios E. Intramembrane charge movement in frog skeletal muscle fibres. Properties of charge 2. J Physiol. 1987 Jun;387:489–517. doi: 10.1113/jphysiol.1987.sp016586. [DOI] [PMC free article] [PubMed] [Google Scholar]
- Burton F., Dörstelmann U., Hutter O. F. Single-channel activity in sarcolemmal vesicles from human and other mammalian muscles. Muscle Nerve. 1988 Oct;11(10):1029–1038. doi: 10.1002/mus.880111004. [DOI] [PubMed] [Google Scholar]
- Cognard C., Lazdunski M., Romey G. Different types of Ca2+ channels in mammalian skeletal muscle cells in culture. Proc Natl Acad Sci U S A. 1986 Jan;83(2):517–521. doi: 10.1073/pnas.83.2.517. [DOI] [PMC free article] [PubMed] [Google Scholar]
- Cota G., Nicola Siri L., Stefani E. Calcium-channel gating in frog skeletal muscle membrane: effect of temperature. J Physiol. 1983 May;338:395–412. doi: 10.1113/jphysiol.1983.sp014679. [DOI] [PMC free article] [PubMed] [Google Scholar]
- Cota G., Stefani E. A fast-activated inward calcium current in twitch muscle fibres of the frog (Rana montezume). J Physiol. 1986 Jan;370:151–163. doi: 10.1113/jphysiol.1986.sp015927. [DOI] [PMC free article] [PubMed] [Google Scholar]
- Cota G., Stefani E. Saturation of calcium channels and surface charge effects in skeletal muscle fibres of the frog. J Physiol. 1984 Jun;351:135–154. doi: 10.1113/jphysiol.1984.sp015238. [DOI] [PMC free article] [PubMed] [Google Scholar]
- Delbono O. Calcium current activation and charge movement in denervated mammalian skeletal muscle fibres. J Physiol. 1992;451:187–203. doi: 10.1113/jphysiol.1992.sp019160. [DOI] [PMC free article] [PubMed] [Google Scholar]
- Delbono O., García J., Appel S. H., Stefani E. Calcium current and charge movement of mammalian muscle: action of amyotrophic lateral sclerosis immunoglobulins. J Physiol. 1991 Dec;444:723–742. doi: 10.1113/jphysiol.1991.sp018903. [DOI] [PMC free article] [PubMed] [Google Scholar]
- Donaldson P. L., Beam K. G. Calcium currents in a fast-twitch skeletal muscle of the rat. J Gen Physiol. 1983 Oct;82(4):449–468. doi: 10.1085/jgp.82.4.449. [DOI] [PMC free article] [PubMed] [Google Scholar]
- Dulhunty A. F., Gage P. W. Asymmetrical charge movement in slow- and fast-twitch mammalian muscle fibres in normal and paraplegic rats. J Physiol. 1983 Aug;341:213–231. doi: 10.1113/jphysiol.1983.sp014802. [DOI] [PMC free article] [PubMed] [Google Scholar]
- Francini F., Stefani E. Decay of the slow calcium current in twitch muscle fibers of the frog is influenced by intracellular EGTA. J Gen Physiol. 1989 Nov;94(5):953–969. doi: 10.1085/jgp.94.5.953. [DOI] [PMC free article] [PubMed] [Google Scholar]
- Gonoi T., Hasegawa S. Post-natal disappearance of transient calcium channels in mouse skeletal muscle: effects of denervation and culture. J Physiol. 1988 Jul;401:617–637. doi: 10.1113/jphysiol.1988.sp017183. [DOI] [PMC free article] [PubMed] [Google Scholar]
- Hollingworth S., Marshall M. W. A comparative study of charge movement in rat and frog skeletal muscle fibres. J Physiol. 1981 Dec;321:583–602. doi: 10.1113/jphysiol.1981.sp014004. [DOI] [PMC free article] [PubMed] [Google Scholar]
- Horowicz P., Schneider M. F. Membrane charge movement in contracting and non-contracting skeletal muscle fibres. J Physiol. 1981 May;314:565–593. doi: 10.1113/jphysiol.1981.sp013725. [DOI] [PMC free article] [PubMed] [Google Scholar]
- Hui C. S., Chandler W. K. Intramembranous charge movement in frog cut twitch fibers mounted in a double vaseline-gap chamber. J Gen Physiol. 1990 Aug;96(2):257–297. doi: 10.1085/jgp.96.2.257. [DOI] [PMC free article] [PubMed] [Google Scholar]
- Irving M., Maylie J., Sizto N. L., Chandler W. K. Intrinsic optical and passive electrical properties of cut frog twitch fibers. J Gen Physiol. 1987 Jan;89(1):1–40. doi: 10.1085/jgp.89.1.1. [DOI] [PMC free article] [PubMed] [Google Scholar]
- Kwieciński H., Lehmann-Horn F., Rüdel R. The resting membrane parameters of human intercostal muscle at low, normal, and high extracellular potassium. Muscle Nerve. 1984 Jan;7(1):60–65. doi: 10.1002/mus.880070110. [DOI] [PubMed] [Google Scholar]
- Lamb G. D. Asymmetric charge movement in contracting muscle fibres in the rabbit. J Physiol. 1986 Jul;376:63–83. doi: 10.1113/jphysiol.1986.sp016142. [DOI] [PMC free article] [PubMed] [Google Scholar]
- Lamb G. D., Walsh T. Calcium currents, charge movement and dihydropyridine binding in fast- and slow-twitch muscles of rat and rabbit. J Physiol. 1987 Dec;393:595–617. doi: 10.1113/jphysiol.1987.sp016843. [DOI] [PMC free article] [PubMed] [Google Scholar]
- Mejía-Alvarez R., Fill M., Stefani E. Voltage-dependent inactivation of T-tubular skeletal calcium channels in planar lipid bilayers. J Gen Physiol. 1991 Feb;97(2):393–412. doi: 10.1085/jgp.97.2.393. [DOI] [PMC free article] [PubMed] [Google Scholar]
- Melzer W., Schneider M. F., Simon B. J., Szucs G. Intramembrane charge movement and calcium release in frog skeletal muscle. J Physiol. 1986 Apr;373:481–511. doi: 10.1113/jphysiol.1986.sp016059. [DOI] [PMC free article] [PubMed] [Google Scholar]
- Rios E., Brum G. Involvement of dihydropyridine receptors in excitation-contraction coupling in skeletal muscle. Nature. 1987 Feb 19;325(6106):717–720. doi: 10.1038/325717a0. [DOI] [PubMed] [Google Scholar]
- Romey G., Garcia L., Dimitriadou V., Pincon-Raymond M., Rieger F., Lazdunski M. Ontogenesis and localization of Ca2+ channels in mammalian skeletal muscle in culture and role in excitation-contraction coupling. Proc Natl Acad Sci U S A. 1989 Apr;86(8):2933–2937. doi: 10.1073/pnas.86.8.2933. [DOI] [PMC free article] [PubMed] [Google Scholar]
- Simon B. J., Beam K. G. Slow charge movement in mammalian skeletal muscle. J Gen Physiol. 1985 Jan;85(1):1–19. doi: 10.1085/jgp.85.1.1. [DOI] [PMC free article] [PubMed] [Google Scholar]
- Siri L. N., Sánchez J. A., Stefani E. Effect of glycerol treatment on the calcium current of frog skeletal muscle. J Physiol. 1980 Aug;305:87–96. doi: 10.1113/jphysiol.1980.sp013351. [DOI] [PMC free article] [PubMed] [Google Scholar]
- Sánchez J. A., Stefani E. Kinetic properties of calcium channels of twitch muscle fibres of the frog. J Physiol. 1983 Apr;337:1–17. doi: 10.1113/jphysiol.1983.sp014607. [DOI] [PMC free article] [PubMed] [Google Scholar]
- Tanabe T., Beam K. G., Powell J. A., Numa S. Restoration of excitation-contraction coupling and slow calcium current in dysgenic muscle by dihydropyridine receptor complementary DNA. Nature. 1988 Nov 10;336(6195):134–139. doi: 10.1038/336134a0. [DOI] [PubMed] [Google Scholar]