Abstract
1. We investigated the currents through L-type Ca2+ channels when Ca2+ (1-10 mM) was the charge carrier, as is the case physiologically. 2. Na+ was removed from both the external and internal solutions to eliminate currents through Na+ channels and Na(+)-Ca2+ exchange. 3. From a holding potential of -50 mV only L-type channels were available to open with depolarization. Macroscopic L-type currents were maximal during depolarizing pulses to +10 mV (peak current density of 4.7 +/- 0.3 nA nF-1). 4. During depolarizing steps as long as 180 ms, the decay of current through L-type channels was incomplete, in contrast to that of T-type current. 5. Unitary currents recorded with comparable ionic conditions and voltage protocols exhibited a single-channel conductance of 6.9 pS in 10 mM Ca2+. Ensemble average currents reproduced accurately the features of whole-cell L-type current, including the maintained component. 6. Convolution analysis was employed to clarify the single-channel basis of the complex current waveform of L-type channels. First openings underlie the peak, while the maintained pedestal is generated by multiple re-openings. As with T-type channels, single openings are brief and contribute little to the time course of the average current. 7. The prominent maintained component of macroscopic and ensemble average L-type current cannot be explained by simple Markov models in which current decay reflects the progressive entry of channels into an absorbing inactivated state. 8. We considered the possibility that the maintained component of current arises from the existence of multiple distinct gating patterns, one of which lacks inactivation. Individual sweeps were sorted among three patterns of gating (no openings, active-early and active-late). Patterns of activity are not randomly distributed; instead, they tend to cluster over time. 9. Most of the maintained current is attributable to the 'active-late' pattern of gating. Considered separately, this pattern can be well described by a simple Markov chain lacking an inactivated state. The 'active-early' gating pattern accounts entirely for the initial current transient, and for about one-third of the maintained component; thus, inactivation, even when present, must be reversible rather than absorbing. 10. The unitary current amplitudes and peak open probabilities measured for single L-type channels, when compared to the average macroscopic L-type current density, predict 170 functional channels per picofarad, or 28,000 L-type channels per typical ventricular myocyte.(ABSTRACT TRUNCATED AT 400 WORDS)
Full text
PDF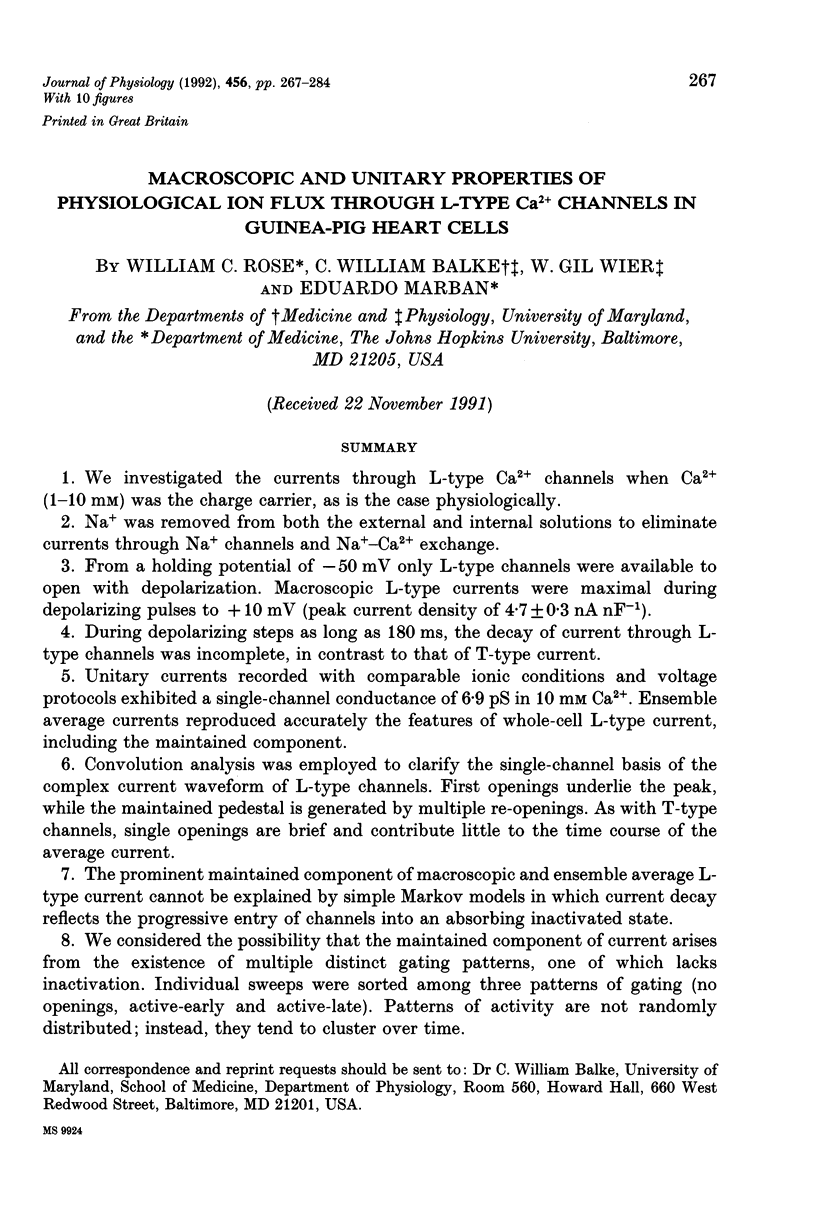
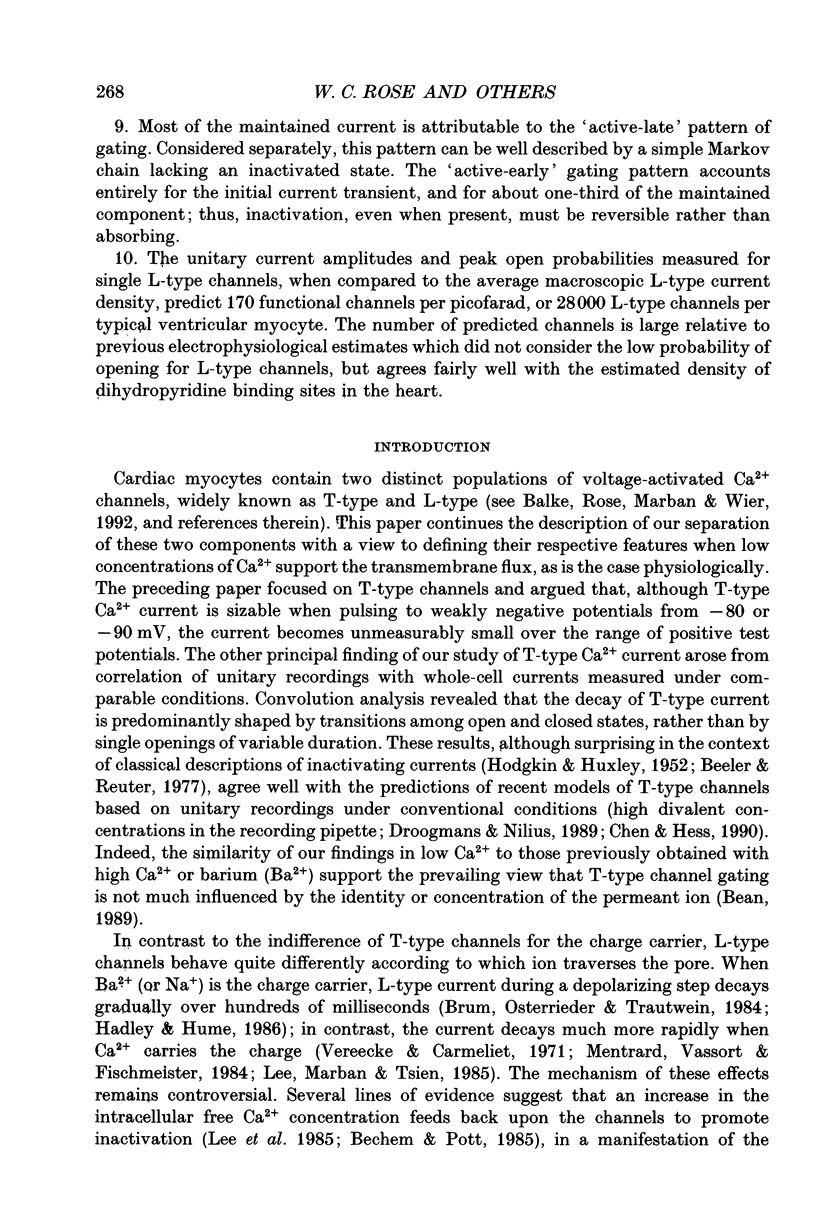
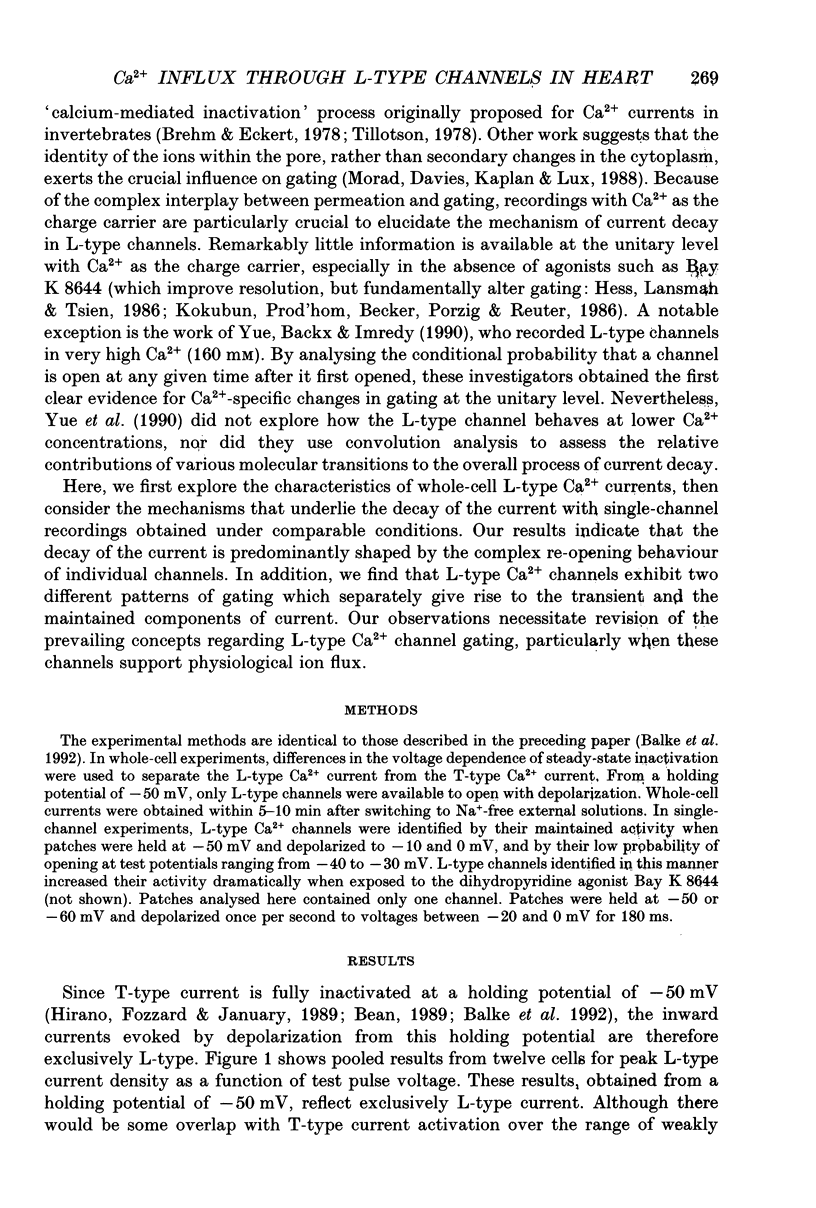
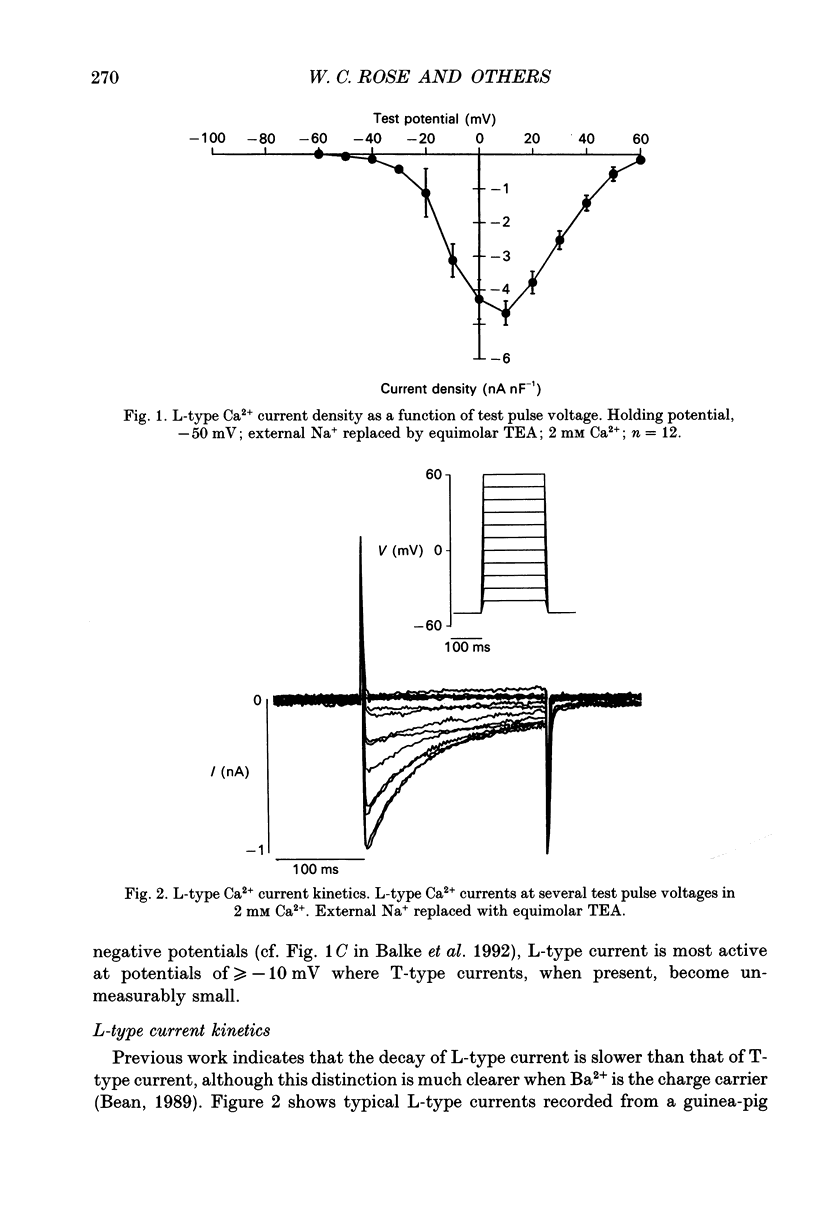
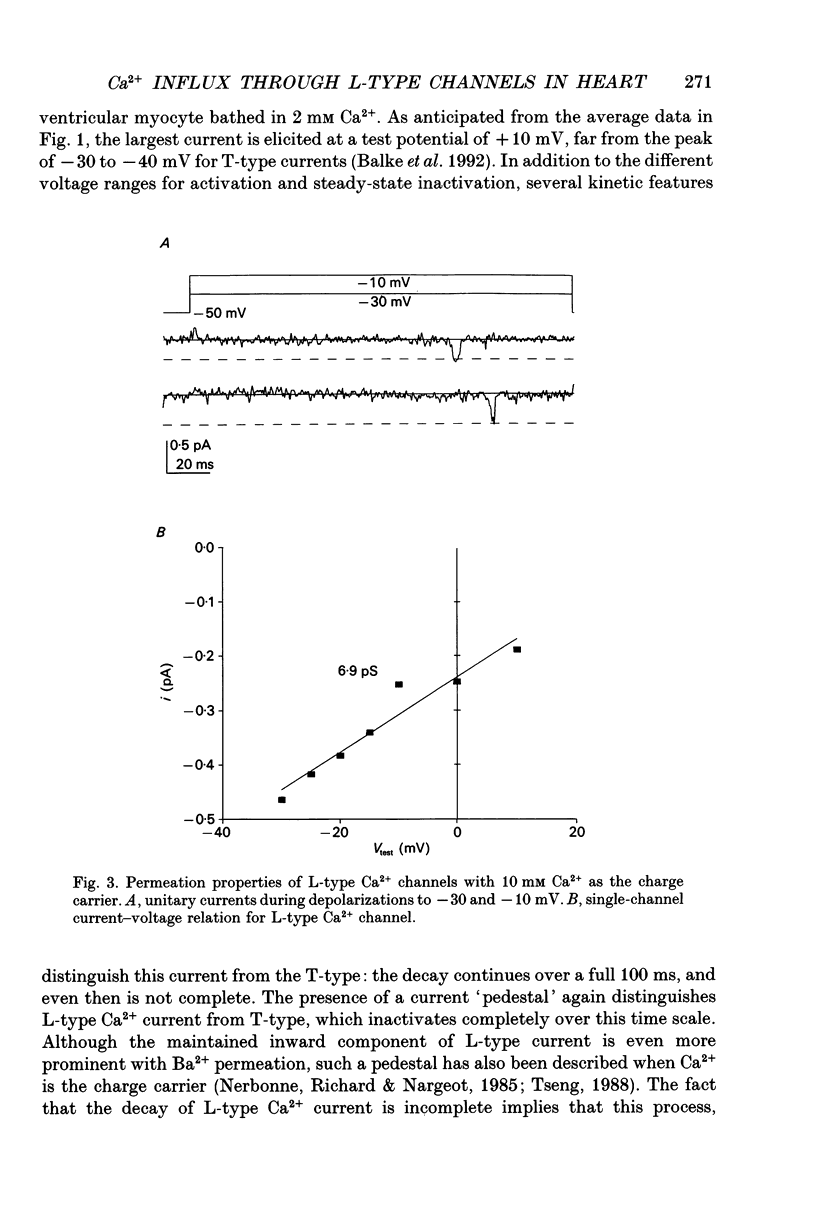
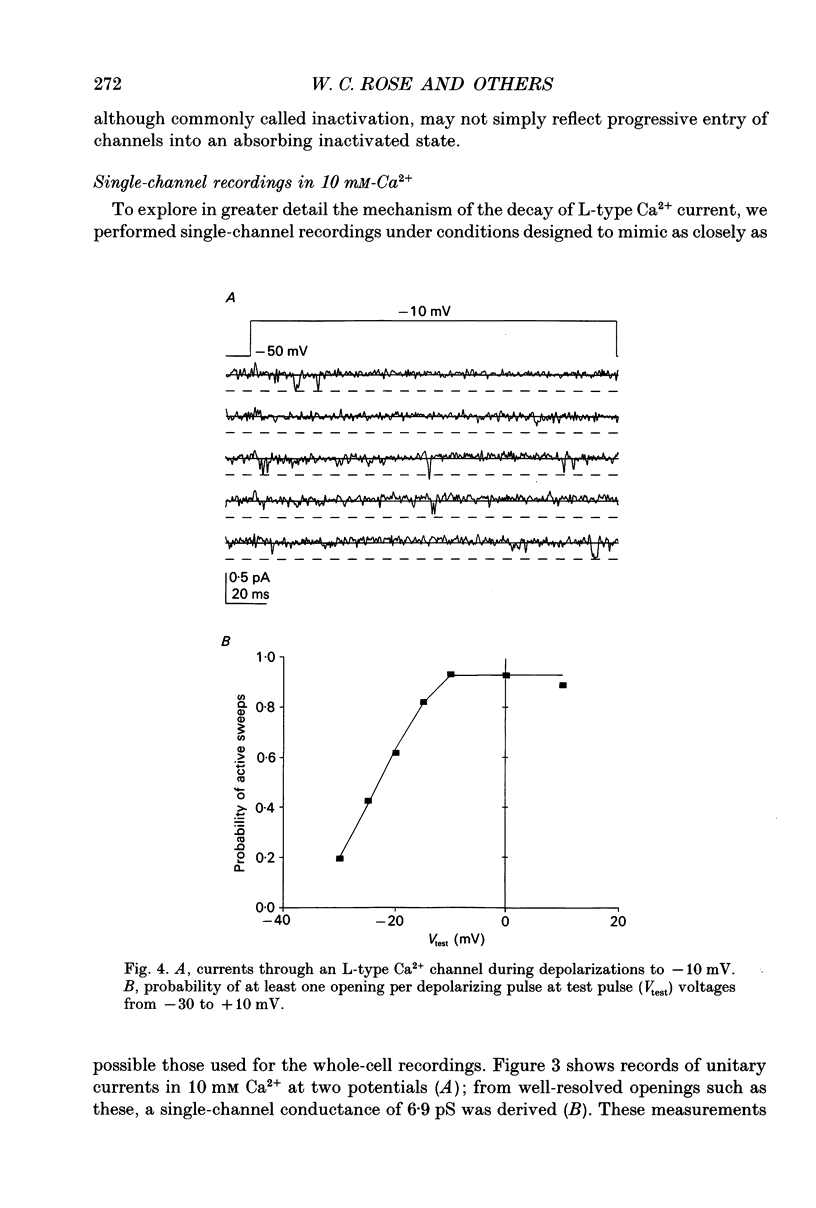
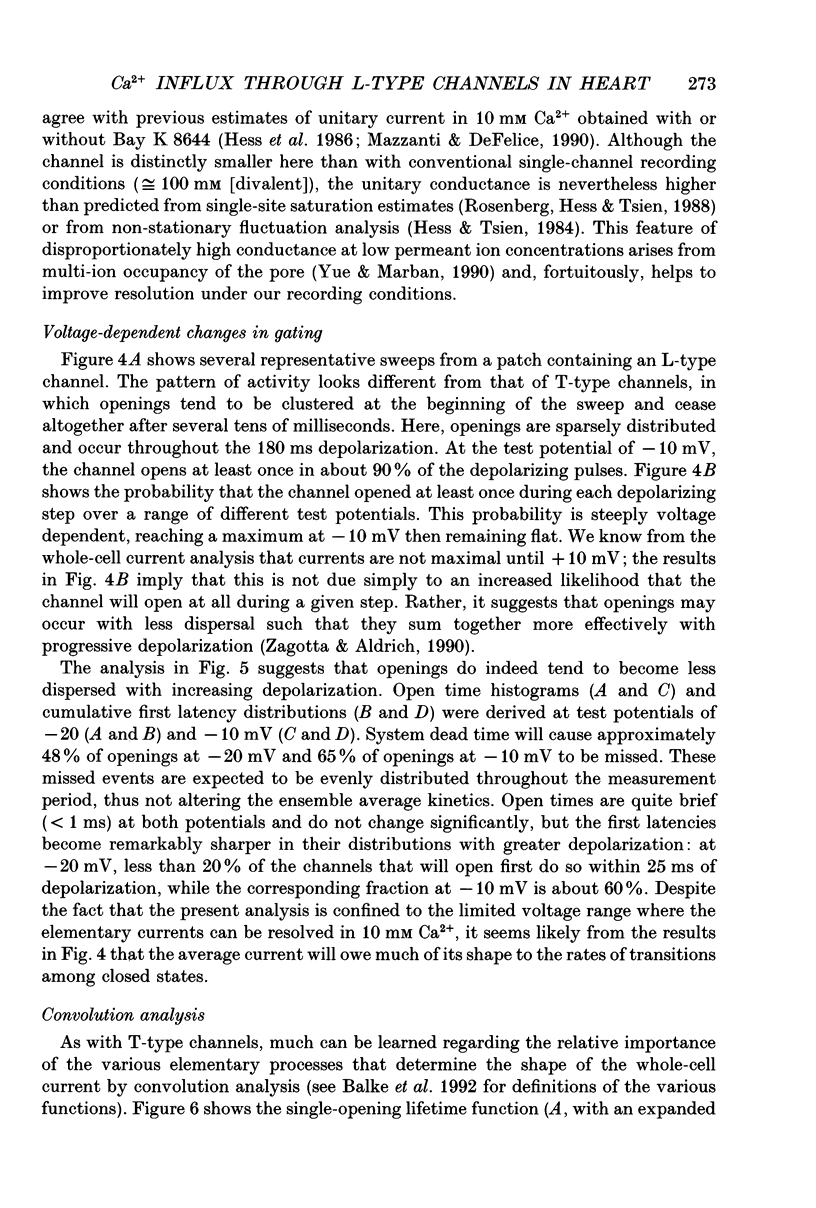
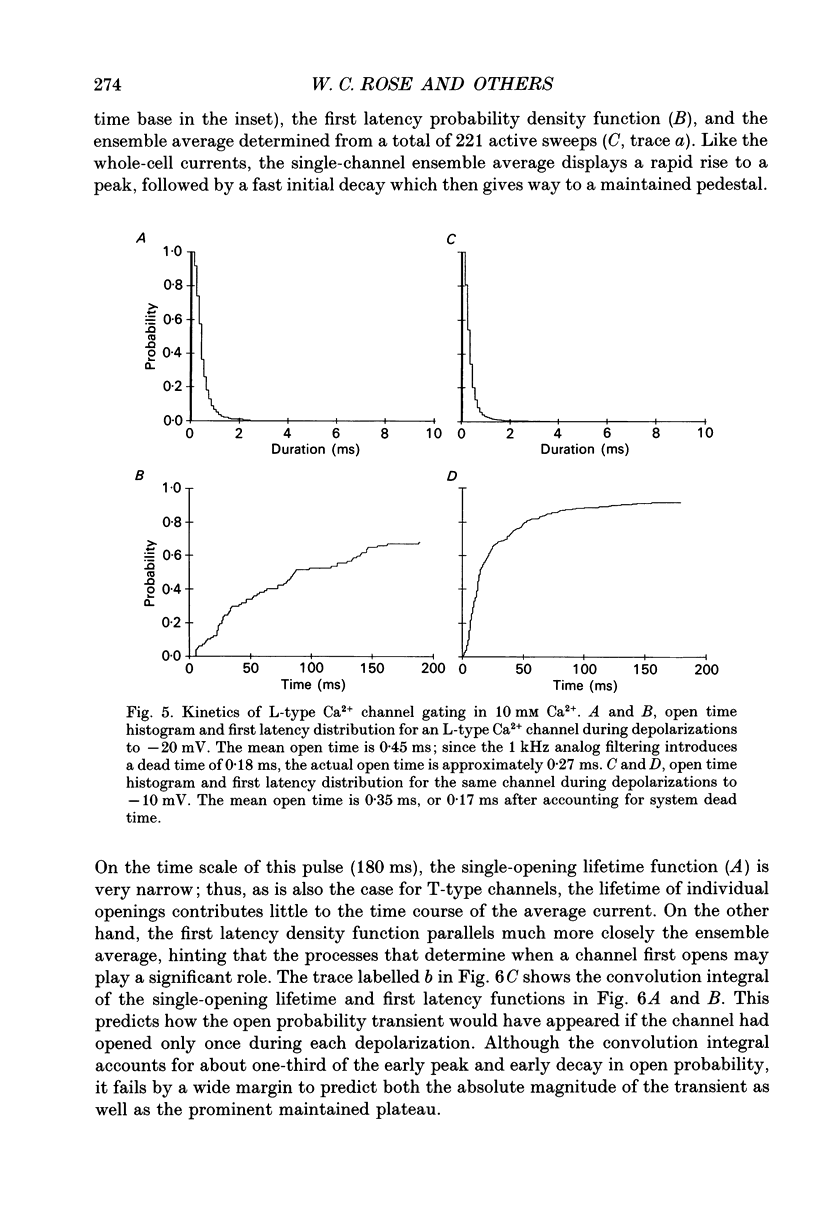
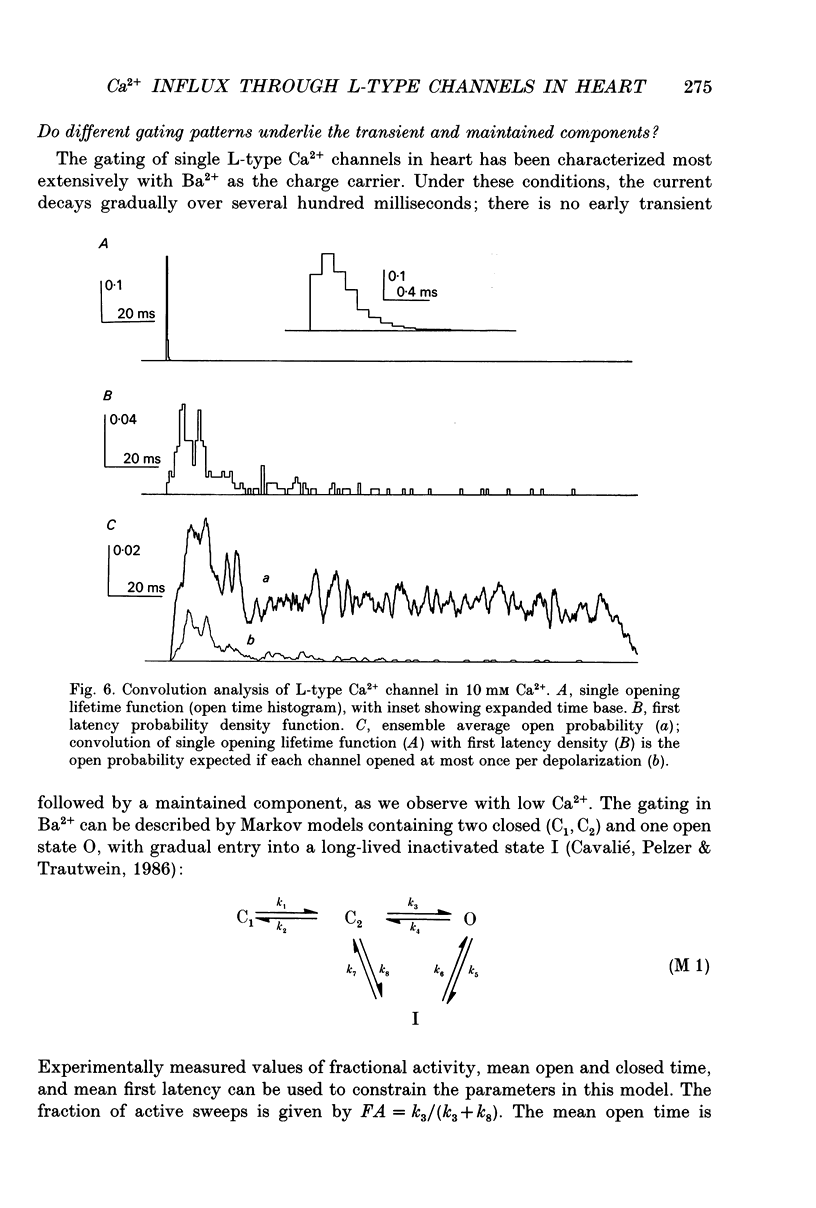
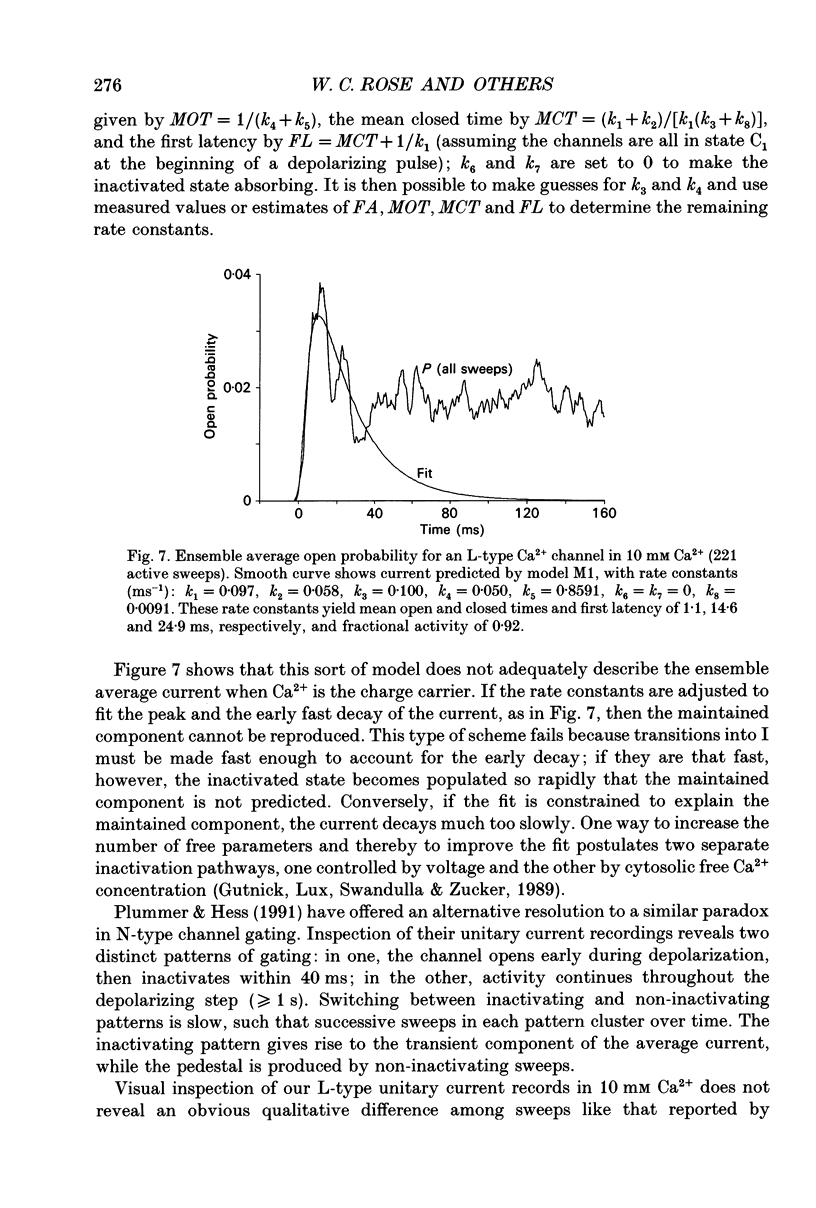
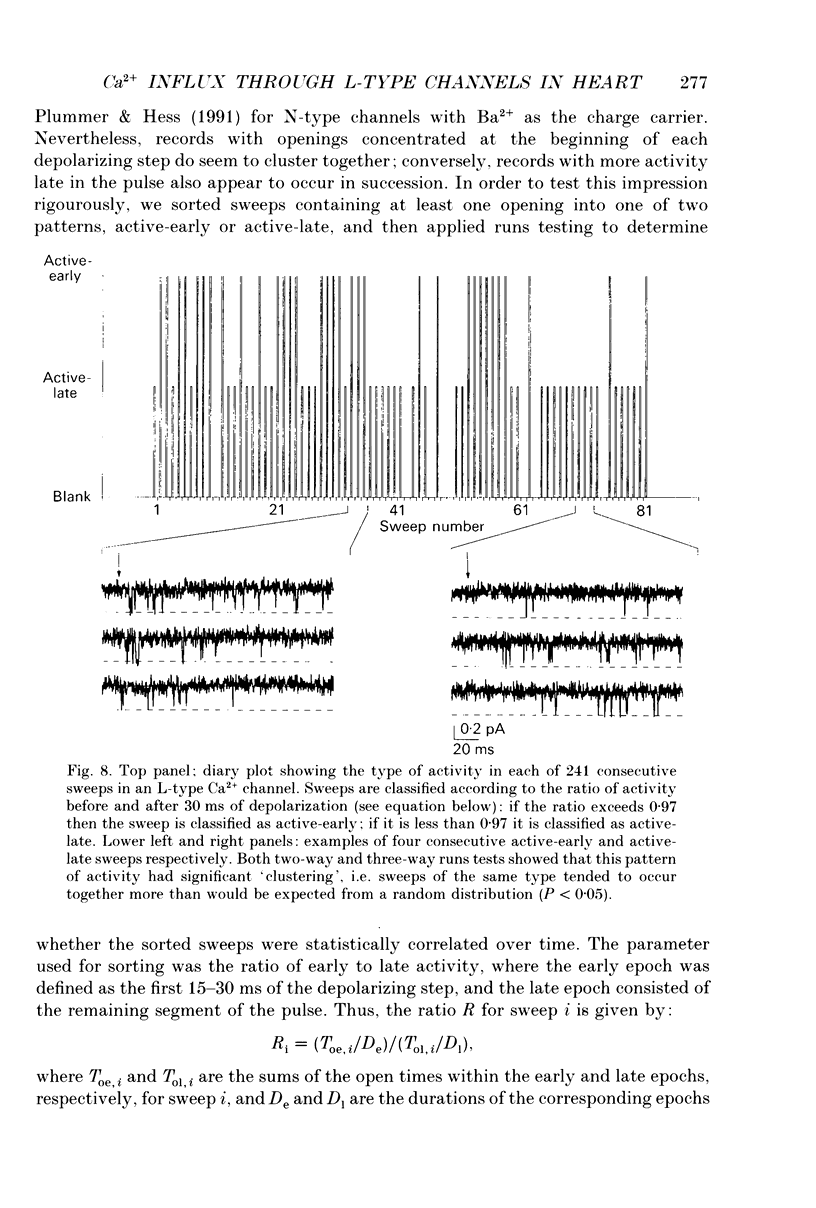
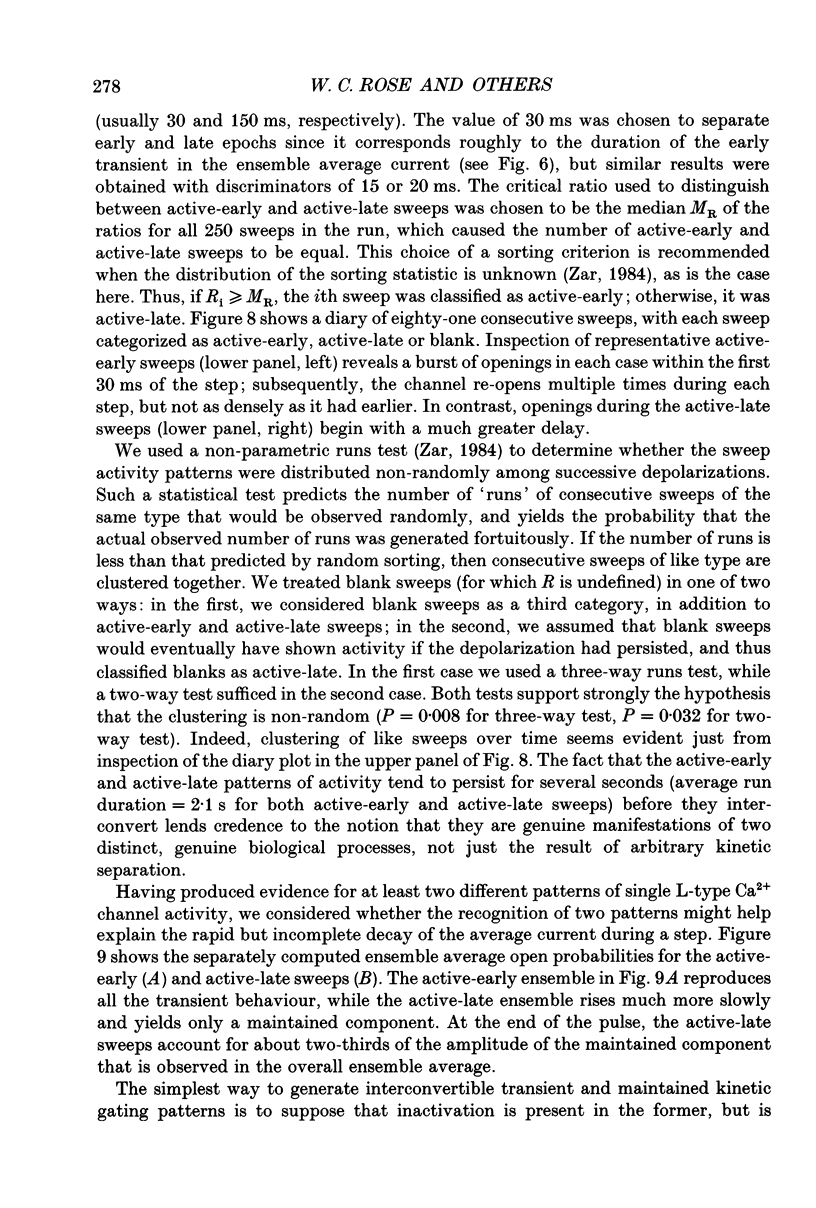
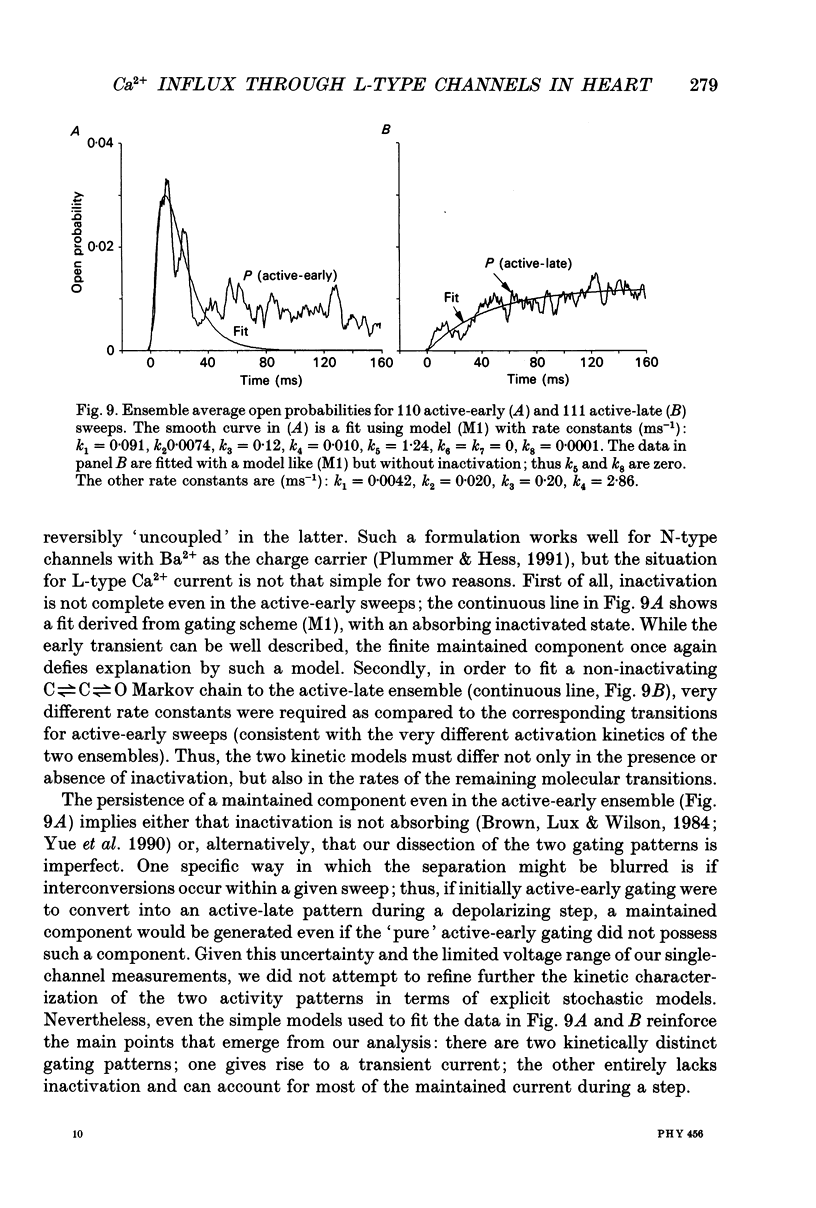
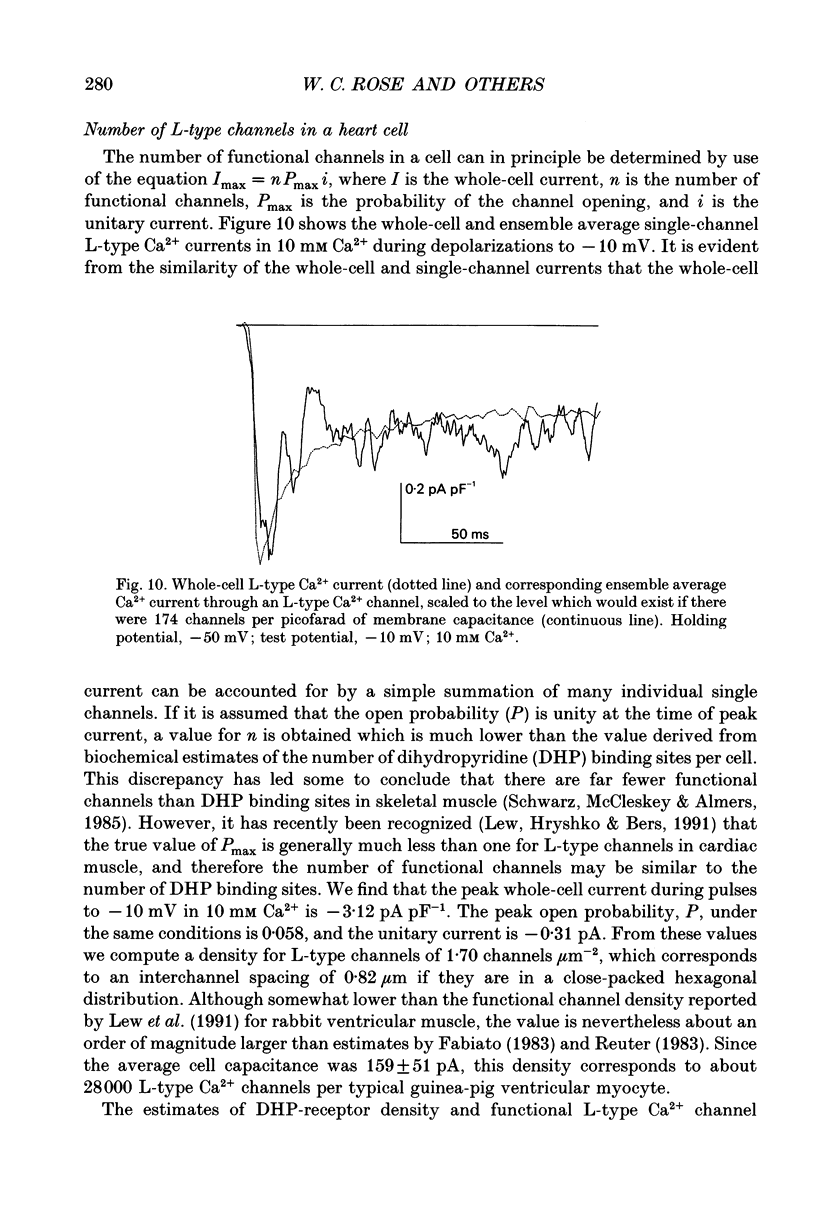
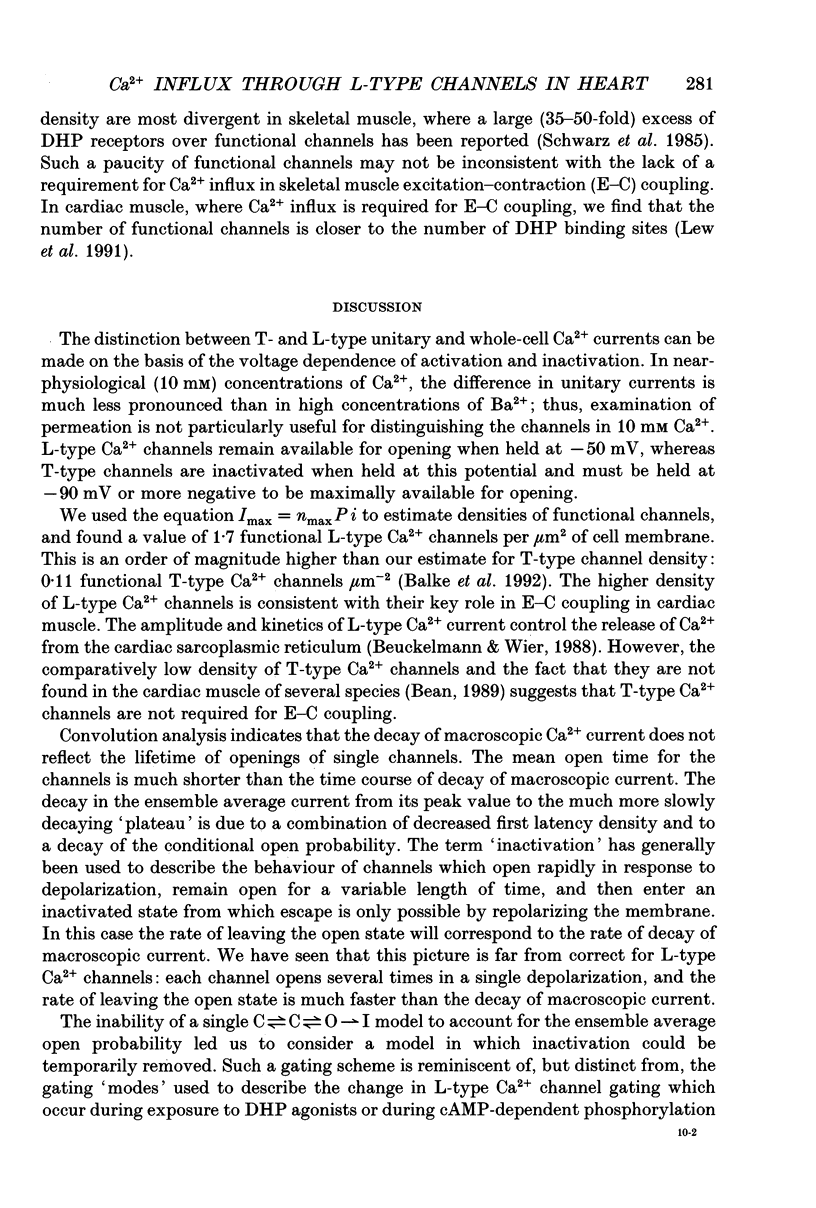
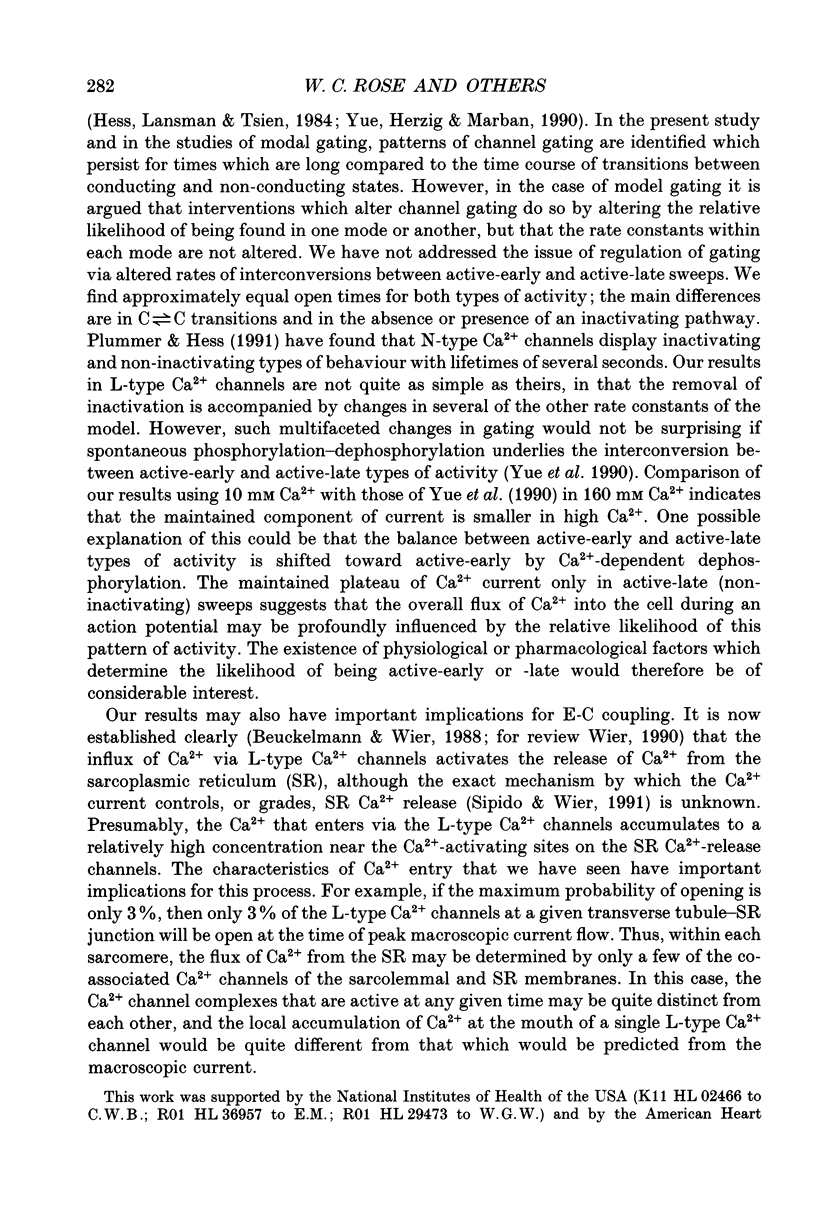
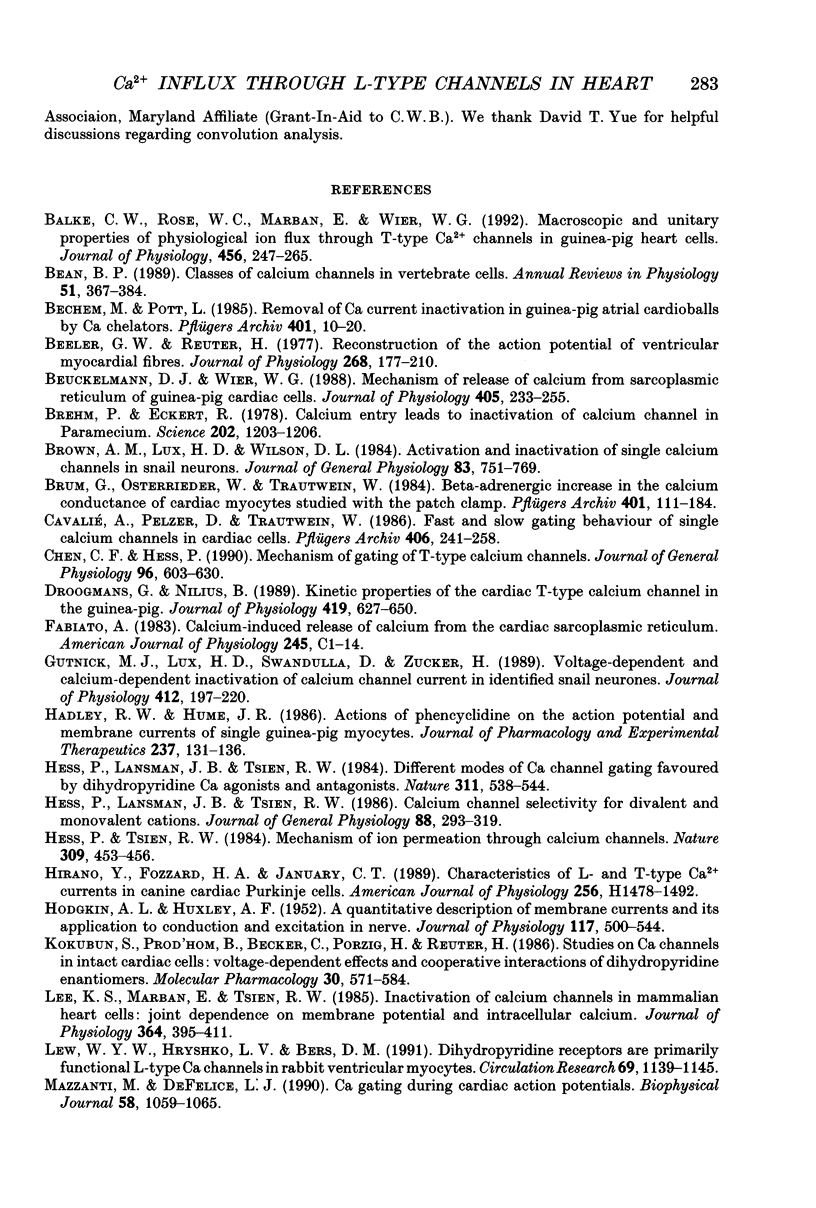
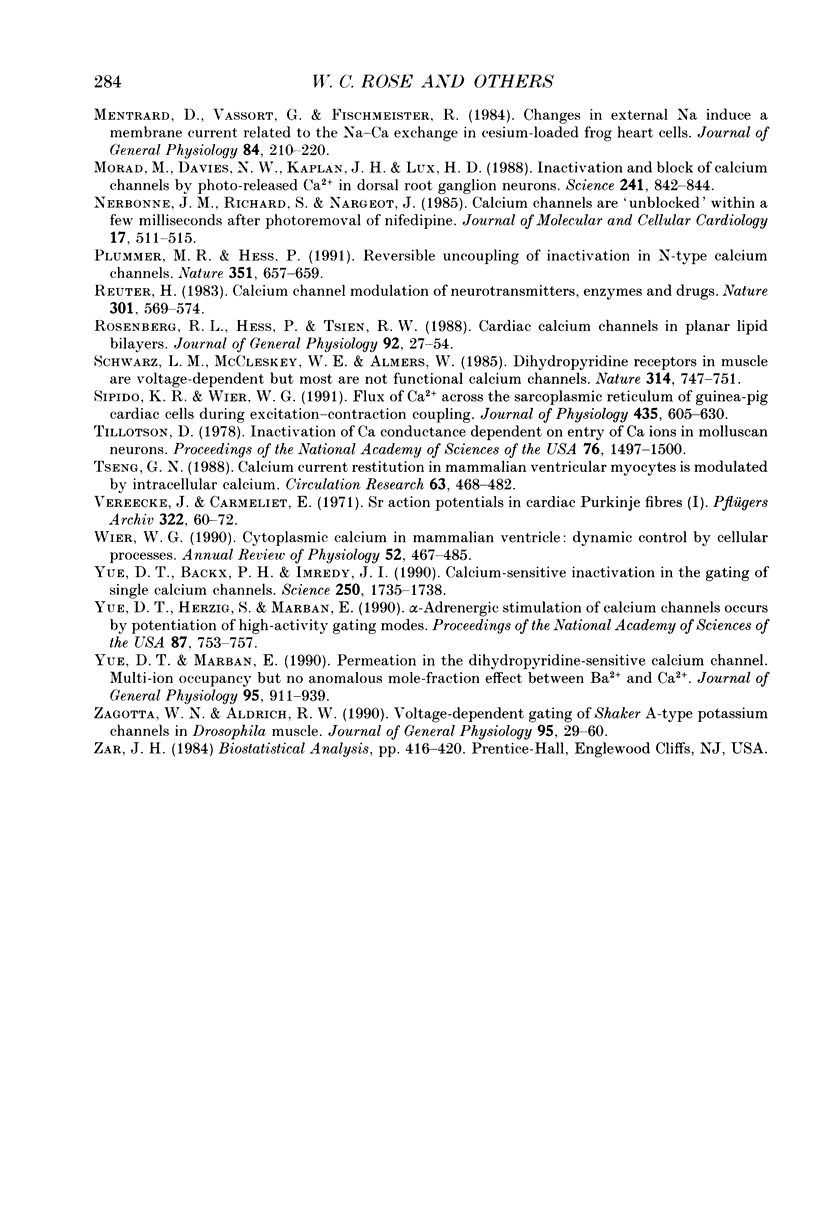
Selected References
These references are in PubMed. This may not be the complete list of references from this article.
- Balke C. W., Rose W. C., Marban E., Wier W. G. Macroscopic and unitary properties of physiological ion flux through T-type Ca2+ channels in guinea-pig heart cells. J Physiol. 1992 Oct;456:247–265. doi: 10.1113/jphysiol.1992.sp019335. [DOI] [PMC free article] [PubMed] [Google Scholar]
- Bean B. P. Classes of calcium channels in vertebrate cells. Annu Rev Physiol. 1989;51:367–384. doi: 10.1146/annurev.ph.51.030189.002055. [DOI] [PubMed] [Google Scholar]
- Bechem M., Pott L. Removal of Ca current inactivation in dialysed guinea-pig atrial cardioballs by Ca chelators. Pflugers Arch. 1985 May;404(1):10–20. doi: 10.1007/BF00581485. [DOI] [PubMed] [Google Scholar]
- Beeler G. W., Reuter H. Reconstruction of the action potential of ventricular myocardial fibres. J Physiol. 1977 Jun;268(1):177–210. doi: 10.1113/jphysiol.1977.sp011853. [DOI] [PMC free article] [PubMed] [Google Scholar]
- Beuckelmann D. J., Wier W. G. Mechanism of release of calcium from sarcoplasmic reticulum of guinea-pig cardiac cells. J Physiol. 1988 Nov;405:233–255. doi: 10.1113/jphysiol.1988.sp017331. [DOI] [PMC free article] [PubMed] [Google Scholar]
- Brehm P., Eckert R. Calcium entry leads to inactivation of calcium channel in Paramecium. Science. 1978 Dec 15;202(4373):1203–1206. doi: 10.1126/science.103199. [DOI] [PubMed] [Google Scholar]
- Brown A. M., Lux H. D., Wilson D. L. Activation and inactivation of single calcium channels in snail neurons. J Gen Physiol. 1984 May;83(5):751–769. doi: 10.1085/jgp.83.5.751. [DOI] [PMC free article] [PubMed] [Google Scholar]
- Brum G., Osterrieder W., Trautwein W. Beta-adrenergic increase in the calcium conductance of cardiac myocytes studied with the patch clamp. Pflugers Arch. 1984 Jun;401(2):111–118. doi: 10.1007/BF00583870. [DOI] [PubMed] [Google Scholar]
- Cavalié A., Pelzer D., Trautwein W. Fast and slow gating behaviour of single calcium channels in cardiac cells. Relation to activation and inactivation of calcium-channel current. Pflugers Arch. 1986 Mar;406(3):241–258. doi: 10.1007/BF00640910. [DOI] [PubMed] [Google Scholar]
- Chen C. F., Hess P. Mechanism of gating of T-type calcium channels. J Gen Physiol. 1990 Sep;96(3):603–630. doi: 10.1085/jgp.96.3.603. [DOI] [PMC free article] [PubMed] [Google Scholar]
- Droogmans G., Nilius B. Kinetic properties of the cardiac T-type calcium channel in the guinea-pig. J Physiol. 1989 Dec;419:627–650. doi: 10.1113/jphysiol.1989.sp017890. [DOI] [PMC free article] [PubMed] [Google Scholar]
- Fabiato A. Calcium-induced release of calcium from the cardiac sarcoplasmic reticulum. Am J Physiol. 1983 Jul;245(1):C1–14. doi: 10.1152/ajpcell.1983.245.1.C1. [DOI] [PubMed] [Google Scholar]
- Gutnick M. J., Lux H. D., Swandulla D., Zucker H. Voltage-dependent and calcium-dependent inactivation of calcium channel current in identified snail neurones. J Physiol. 1989 May;412:197–220. doi: 10.1113/jphysiol.1989.sp017611. [DOI] [PMC free article] [PubMed] [Google Scholar]
- HODGKIN A. L., HUXLEY A. F. A quantitative description of membrane current and its application to conduction and excitation in nerve. J Physiol. 1952 Aug;117(4):500–544. doi: 10.1113/jphysiol.1952.sp004764. [DOI] [PMC free article] [PubMed] [Google Scholar]
- Hadley R. W., Hume J. R. Actions of phencyclidine on the action potential and membrane currents of single guinea-pig myocytes. J Pharmacol Exp Ther. 1986 Apr;237(1):131–136. [PubMed] [Google Scholar]
- Hess P., Lansman J. B., Tsien R. W. Calcium channel selectivity for divalent and monovalent cations. Voltage and concentration dependence of single channel current in ventricular heart cells. J Gen Physiol. 1986 Sep;88(3):293–319. doi: 10.1085/jgp.88.3.293. [DOI] [PMC free article] [PubMed] [Google Scholar]
- Hess P., Lansman J. B., Tsien R. W. Different modes of Ca channel gating behaviour favoured by dihydropyridine Ca agonists and antagonists. Nature. 1984 Oct 11;311(5986):538–544. doi: 10.1038/311538a0. [DOI] [PubMed] [Google Scholar]
- Hess P., Tsien R. W. Mechanism of ion permeation through calcium channels. 1984 May 31-Jun 6Nature. 309(5967):453–456. doi: 10.1038/309453a0. [DOI] [PubMed] [Google Scholar]
- Hirano Y., Fozzard H. A., January C. T. Characteristics of L- and T-type Ca2+ currents in canine cardiac Purkinje cells. Am J Physiol. 1989 May;256(5 Pt 2):H1478–H1492. doi: 10.1152/ajpheart.1989.256.5.H1478. [DOI] [PubMed] [Google Scholar]
- Kokubun S., Prod'hom B., Becker C., Porzig H., Reuter H. Studies on Ca channels in intact cardiac cells: voltage-dependent effects and cooperative interactions of dihydropyridine enantiomers. Mol Pharmacol. 1986 Dec;30(6):571–584. [PubMed] [Google Scholar]
- Lee K. S., Marban E., Tsien R. W. Inactivation of calcium channels in mammalian heart cells: joint dependence on membrane potential and intracellular calcium. J Physiol. 1985 Jul;364:395–411. doi: 10.1113/jphysiol.1985.sp015752. [DOI] [PMC free article] [PubMed] [Google Scholar]
- Lew W. Y., Hryshko L. V., Bers D. M. Dihydropyridine receptors are primarily functional L-type calcium channels in rabbit ventricular myocytes. Circ Res. 1991 Oct;69(4):1139–1145. doi: 10.1161/01.res.69.4.1139. [DOI] [PubMed] [Google Scholar]
- Mazzanti M., DeFelice L. J. Ca channel gating during cardiac action potentials. Biophys J. 1990 Oct;58(4):1059–1065. doi: 10.1016/S0006-3495(90)82448-6. [DOI] [PMC free article] [PubMed] [Google Scholar]
- Mentrard D., Vassort G., Fischmeister R. Changes in external Na induce a membrane current related to the Na-Ca exchange in cesium-loaded frog heart cells. J Gen Physiol. 1984 Aug;84(2):201–220. doi: 10.1085/jgp.84.2.201. [DOI] [PMC free article] [PubMed] [Google Scholar]
- Morad M., Davies N. W., Kaplan J. H., Lux H. D. Inactivation and block of calcium channels by photo-released Ca2+ in dorsal root ganglion neurons. Science. 1988 Aug 12;241(4867):842–844. doi: 10.1126/science.2457253. [DOI] [PubMed] [Google Scholar]
- Nerbonne J. M., Richard S., Nargeot J. Calcium channels are 'unblocked' within a few milliseconds after photoremoval of nifedipine. J Mol Cell Cardiol. 1985 May;17(5):511–515. doi: 10.1016/s0022-2828(85)80056-0. [DOI] [PubMed] [Google Scholar]
- Plummer M. R., Hess P. Reversible uncoupling of inactivation in N-type calcium channels. Nature. 1991 Jun 20;351(6328):657–659. doi: 10.1038/351657a0. [DOI] [PubMed] [Google Scholar]
- Reuter H. Calcium channel modulation by neurotransmitters, enzymes and drugs. Nature. 1983 Feb 17;301(5901):569–574. doi: 10.1038/301569a0. [DOI] [PubMed] [Google Scholar]
- Rosenberg R. L., Hess P., Tsien R. W. Cardiac calcium channels in planar lipid bilayers. L-type channels and calcium-permeable channels open at negative membrane potentials. J Gen Physiol. 1988 Jul;92(1):27–54. doi: 10.1085/jgp.92.1.27. [DOI] [PMC free article] [PubMed] [Google Scholar]
- Schwartz L. M., McCleskey E. W., Almers W. Dihydropyridine receptors in muscle are voltage-dependent but most are not functional calcium channels. 1985 Apr 25-May 1Nature. 314(6013):747–751. doi: 10.1038/314747a0. [DOI] [PubMed] [Google Scholar]
- Sipido K. R., Wier W. G. Flux of Ca2+ across the sarcoplasmic reticulum of guinea-pig cardiac cells during excitation-contraction coupling. J Physiol. 1991 Apr;435:605–630. doi: 10.1113/jphysiol.1991.sp018528. [DOI] [PMC free article] [PubMed] [Google Scholar]
- Tillotson D. Inactivation of Ca conductance dependent on entry of Ca ions in molluscan neurons. Proc Natl Acad Sci U S A. 1979 Mar;76(3):1497–1500. doi: 10.1073/pnas.76.3.1497. [DOI] [PMC free article] [PubMed] [Google Scholar]
- Tseng G. N. Calcium current restitution in mammalian ventricular myocytes is modulated by intracellular calcium. Circ Res. 1988 Aug;63(2):468–482. doi: 10.1161/01.res.63.2.468. [DOI] [PubMed] [Google Scholar]
- Vereecke J., Carmeliet E. Sr action potentials in cardiac Purkyne fibres. I. Evidence for a regenerative increase in Sr conductance. Pflugers Arch. 1971;322(1):60–72. doi: 10.1007/BF00586665. [DOI] [PubMed] [Google Scholar]
- Wier W. G. Cytoplasmic [Ca2+] in mammalian ventricle: dynamic control by cellular processes. Annu Rev Physiol. 1990;52:467–485. doi: 10.1146/annurev.ph.52.030190.002343. [DOI] [PubMed] [Google Scholar]
- Yue D. T., Backx P. H., Imredy J. P. Calcium-sensitive inactivation in the gating of single calcium channels. Science. 1990 Dec 21;250(4988):1735–1738. doi: 10.1126/science.2176745. [DOI] [PubMed] [Google Scholar]
- Yue D. T., Herzig S., Marban E. Beta-adrenergic stimulation of calcium channels occurs by potentiation of high-activity gating modes. Proc Natl Acad Sci U S A. 1990 Jan;87(2):753–757. doi: 10.1073/pnas.87.2.753. [DOI] [PMC free article] [PubMed] [Google Scholar]
- Yue D. T., Marban E. Permeation in the dihydropyridine-sensitive calcium channel. Multi-ion occupancy but no anomalous mole-fraction effect between Ba2+ and Ca2+. J Gen Physiol. 1990 May;95(5):911–939. doi: 10.1085/jgp.95.5.911. [DOI] [PMC free article] [PubMed] [Google Scholar]
- Zagotta W. N., Aldrich R. W. Voltage-dependent gating of Shaker A-type potassium channels in Drosophila muscle. J Gen Physiol. 1990 Jan;95(1):29–60. doi: 10.1085/jgp.95.1.29. [DOI] [PMC free article] [PubMed] [Google Scholar]