Abstract
1. The hyperosmotic activation of the Na(+)-H+ exchanger was studied in an osteoblast-like rat cell line (RCJ 1.20). The activation was monitored by recording the intracellular pH (pHi) changes employing double excitation of the pH-sensitive fluorescent dye 2'7'-bis(carboxyethyl)-5(6)-carboxyfluorescein acetoxymethyl ester (BCECF-AM). 2. Exposure of the cells to a hyperosmotic HCO(3-)-free medium at 37 degrees C produced an initial cytosolic acidification of 0.05 pH units followed by a lag period and an alkalinization overshoot of about 0.2 pH units, without a concomitant change of the free cytosolic calcium [Ca2+]i by the use of Fura-2 calcium-sensitive probes. This response was completely inhibited by amiloride (0.33 mM) or by Na+ depletion from the external medium and insensitive to the extracellular Cl- replacement, indicating the involvement of a Na(+)-H+ exchanger in the hyperosmotic response. 3. Hyperosmotic stimuli (200 moSM sucrose) applied in the temperature range of 17-37 degrees C demonstrated a shortening of the lag period preceding alkalinization and an increased rate of proton extrusion upon temperature elevation. The biochemical reaction underlying the lag period and the proton extrusion resulted in apparent activation energies of 19 and 29 kcal mol-1, respectively, as calculated from the appropriate Arrhenius plots. 4. Stimulation of the exchanger under isosmotic conditions by 25 nM 4 beta-phorbol 12-myristate 13-acetate (PMA) and 0.1 mM vanadate resulted in an amiloride-sensitive pHi increase of about 0.08 pH units. The hyperosmotic stress was additive to the stimulatory effects of these agents, suggesting an independent hyperosmotic activation pathway. 5. The hyperosmotic activation of the Na(+)-H+ exchanger was independent of cAMP, cGMP, cytosolic Ca2+ and protein kinase C. Thus, none of the classical transduction mechanisms seem to be involved directly in the hyperosmotic activation of the antiporter. 6. The pHi response induced by the hyperosmotic stress was abolished by two calmodulin inhibitors, W-7 and chlorpromazine (50% inhibition, Ki at 28 and 20 microM, respectively), 20 microM cytochalasin B, but not by 10 microM colchicine. The results suggest the involvement of actin and calmodulin-like structural elements of the cytoskeleton in the transduction process leading to the activation of the Na(+)-H+ exchanger.
Full text
PDF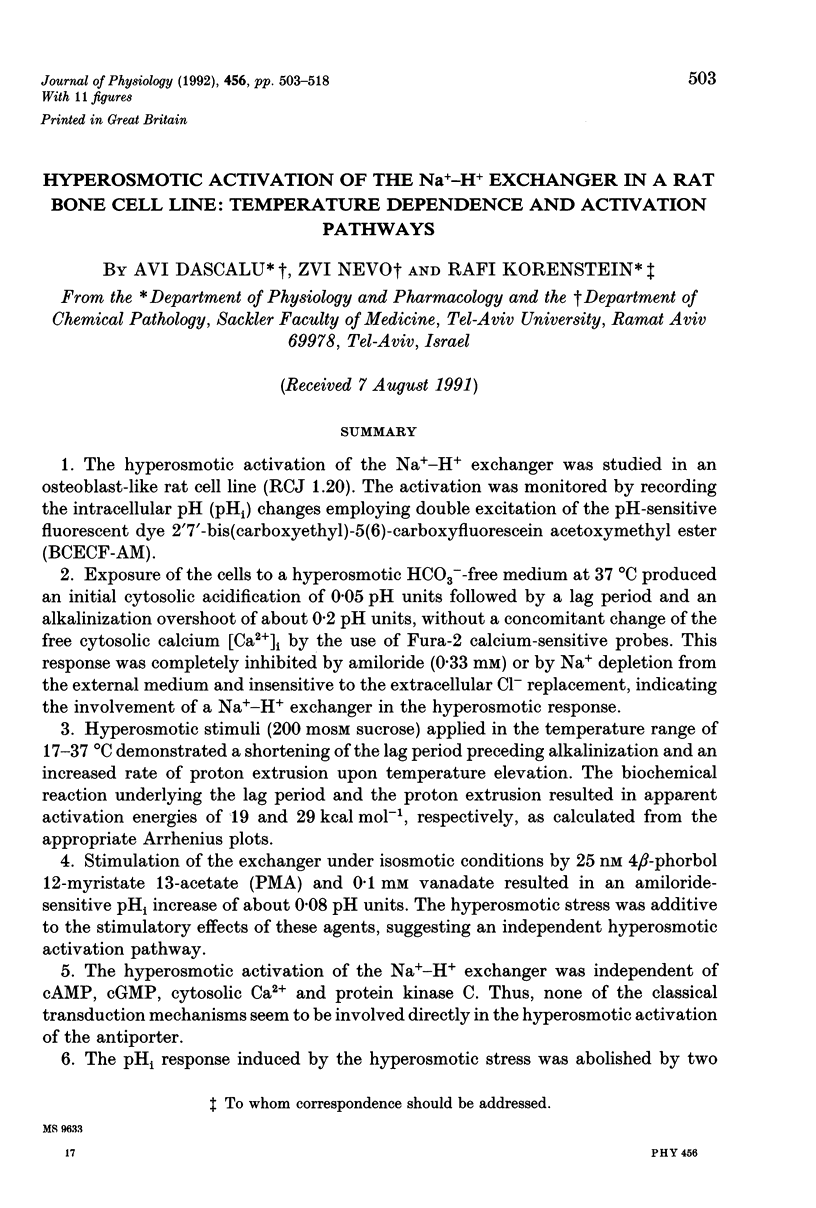
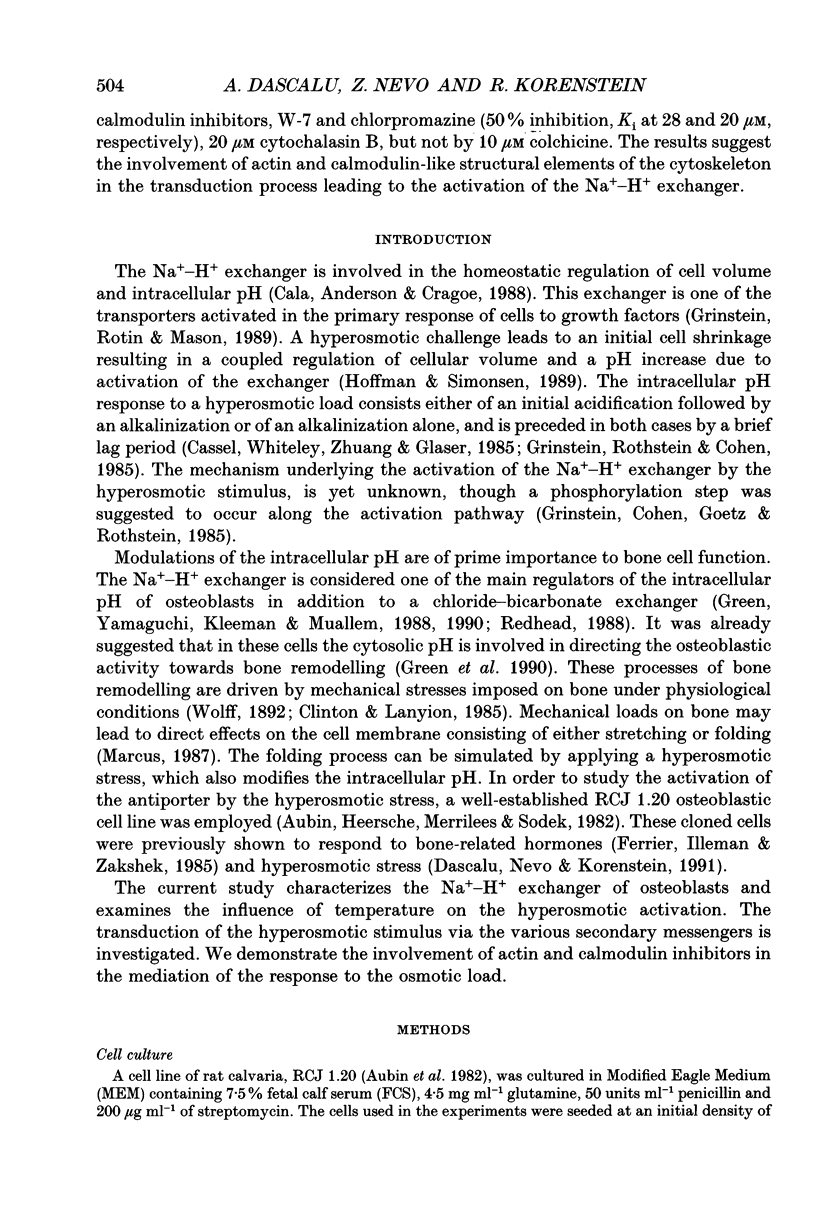
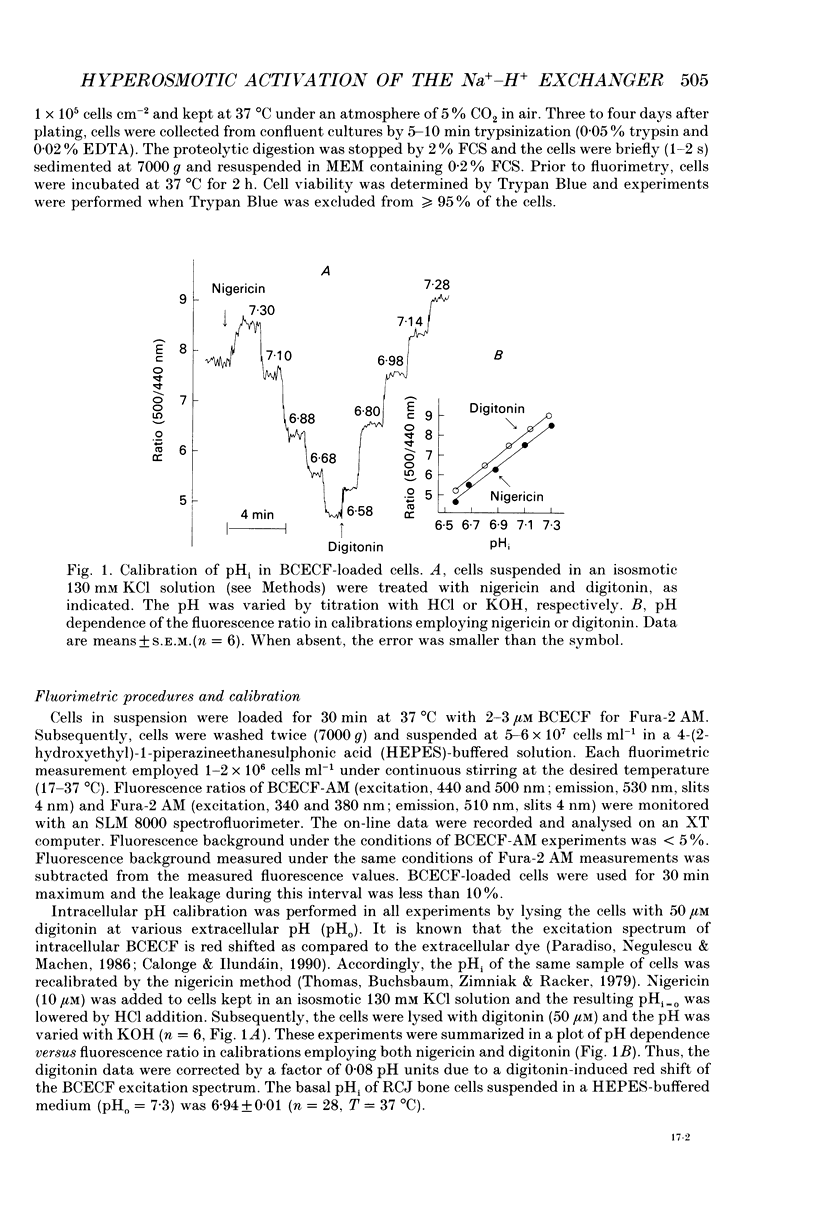
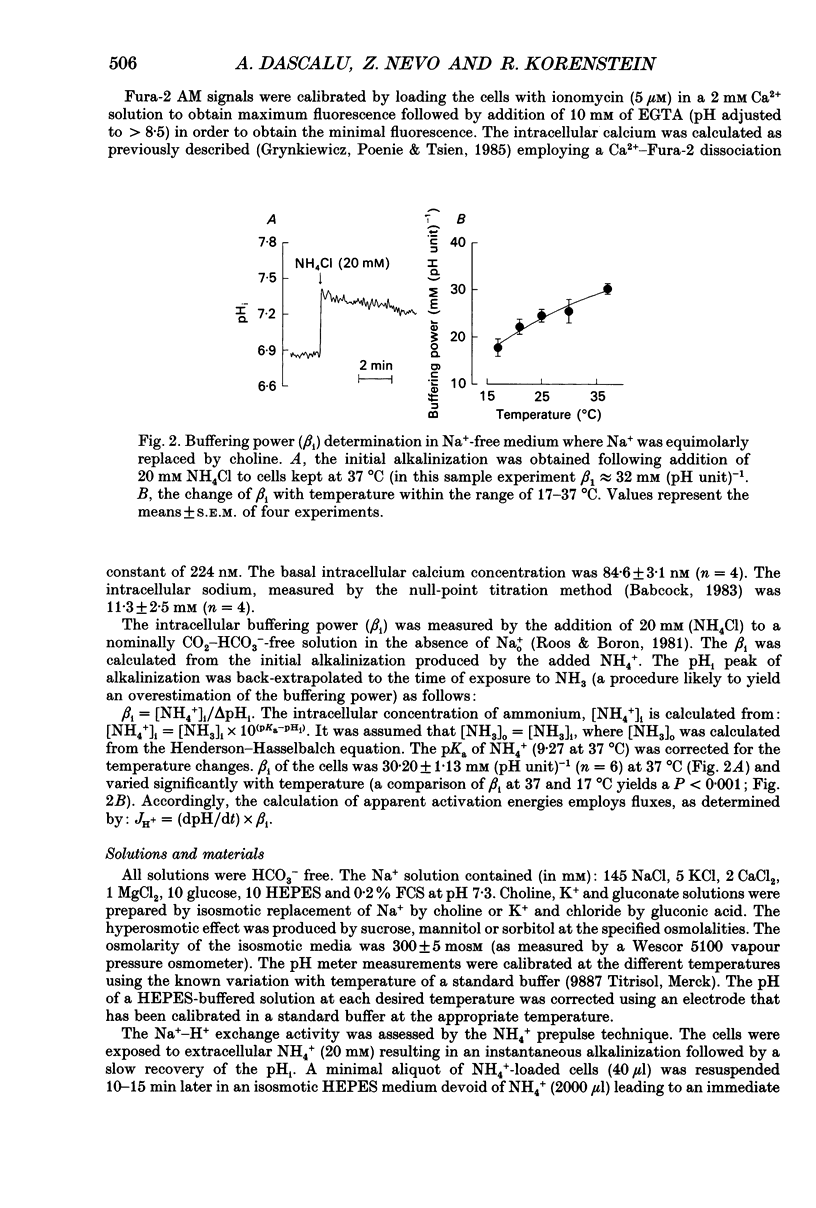
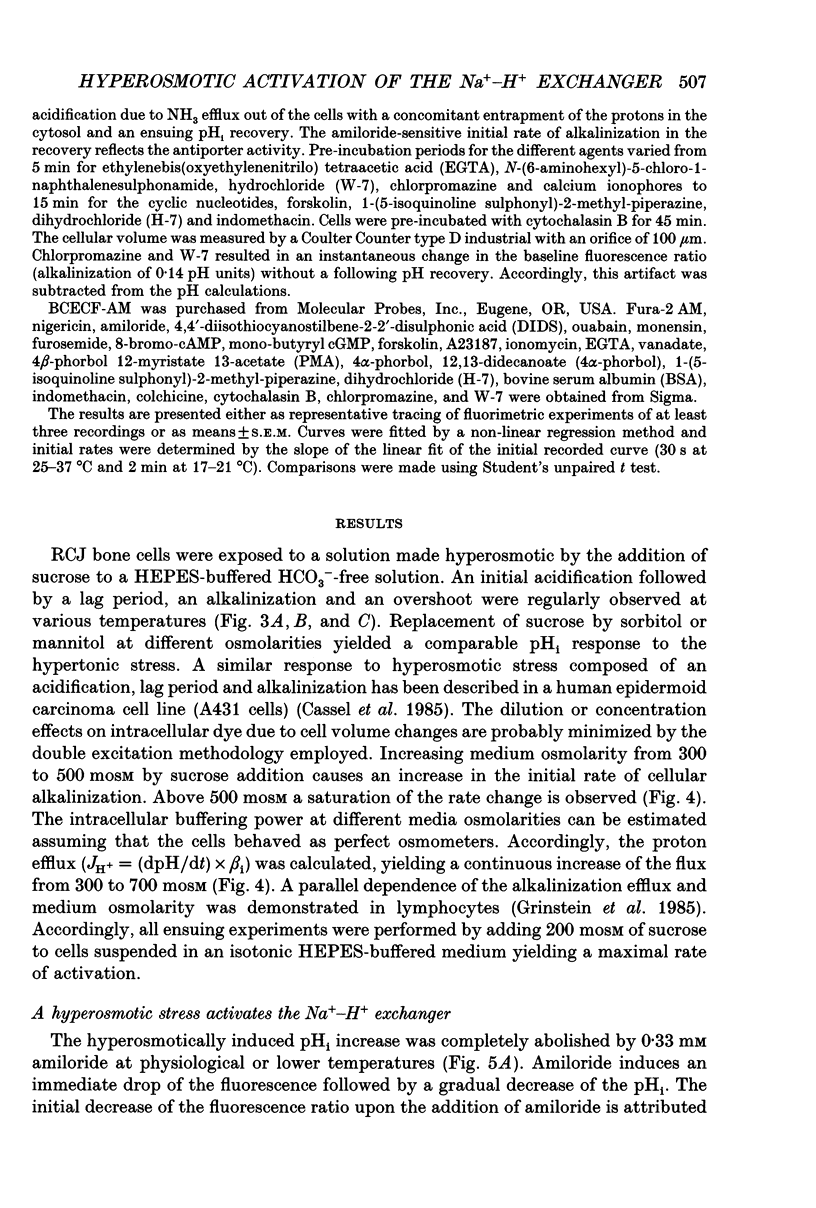
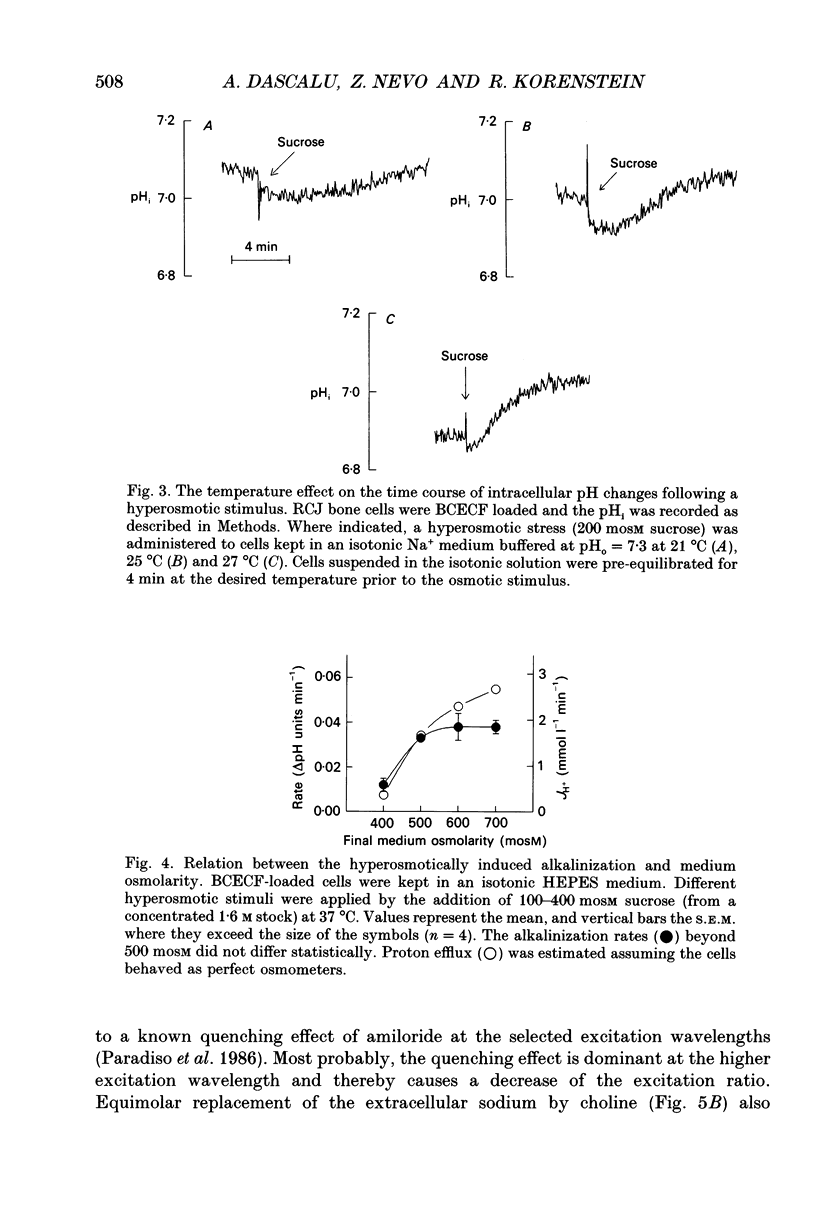
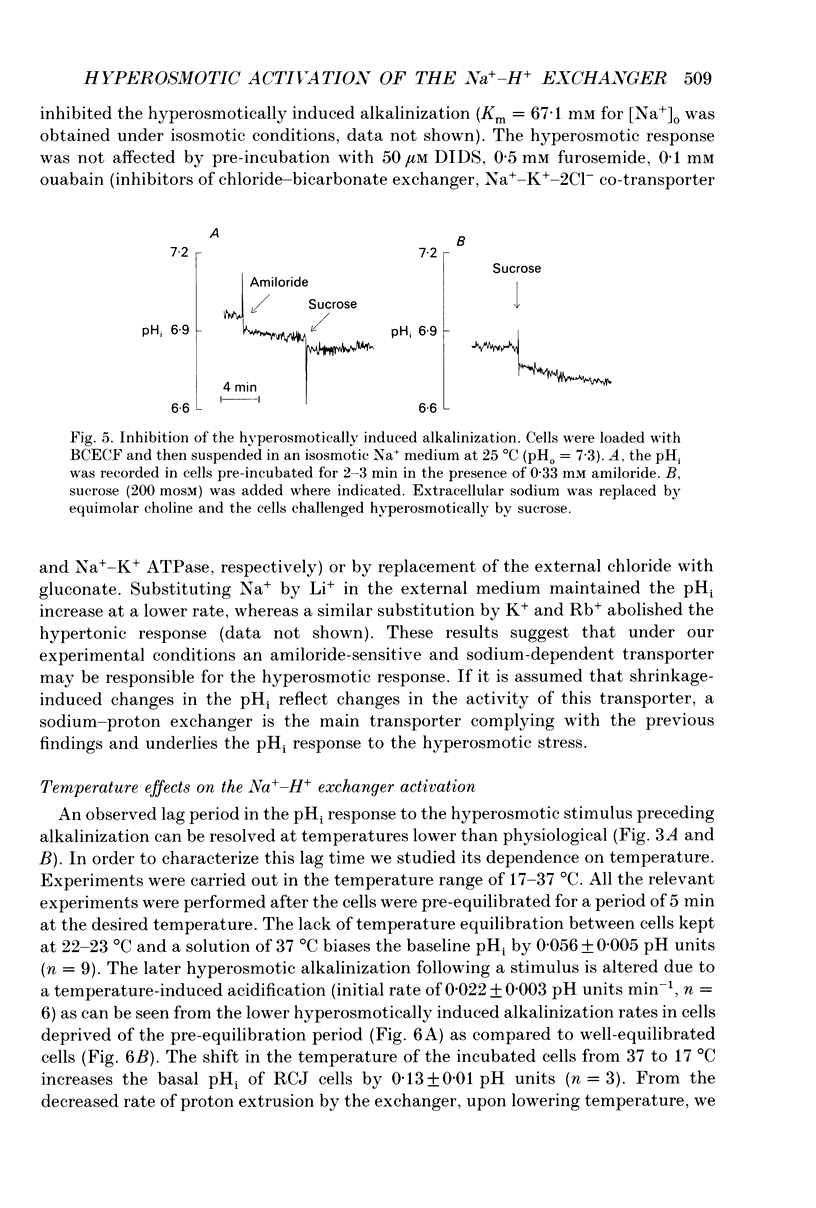
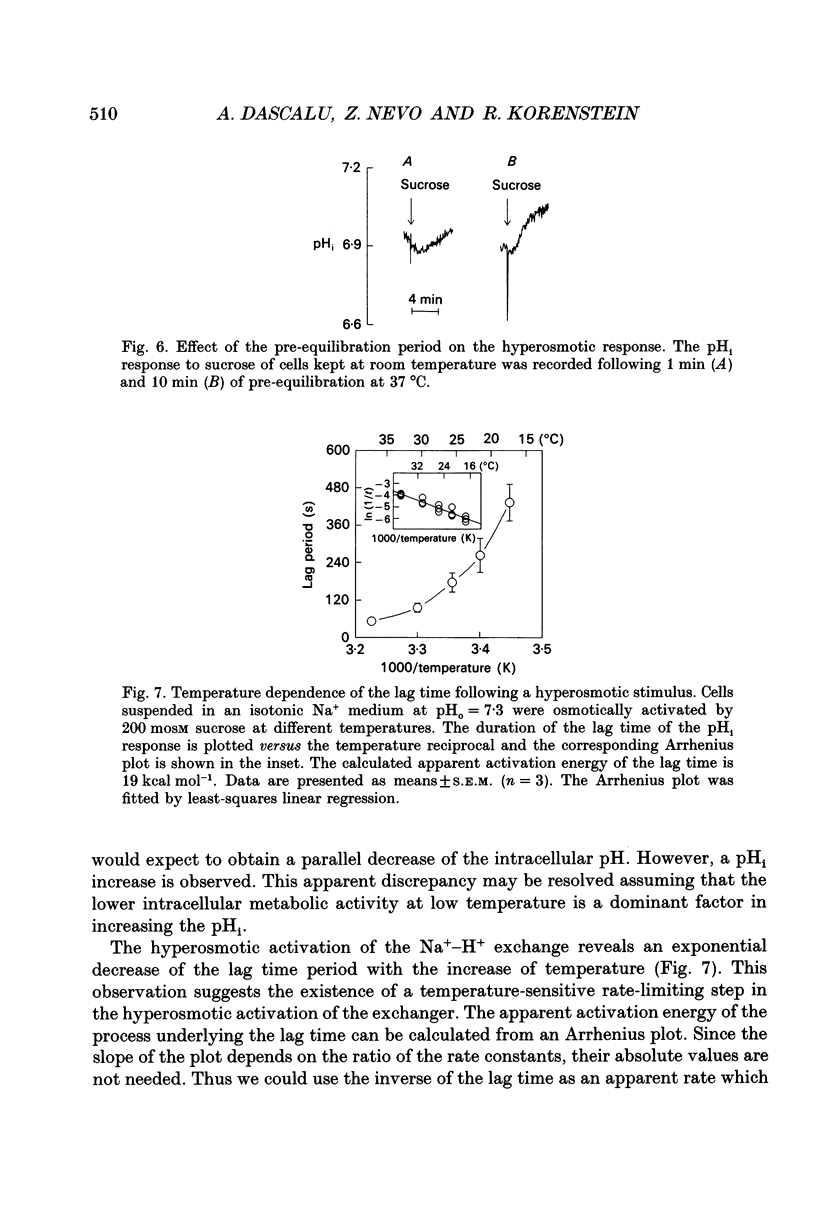
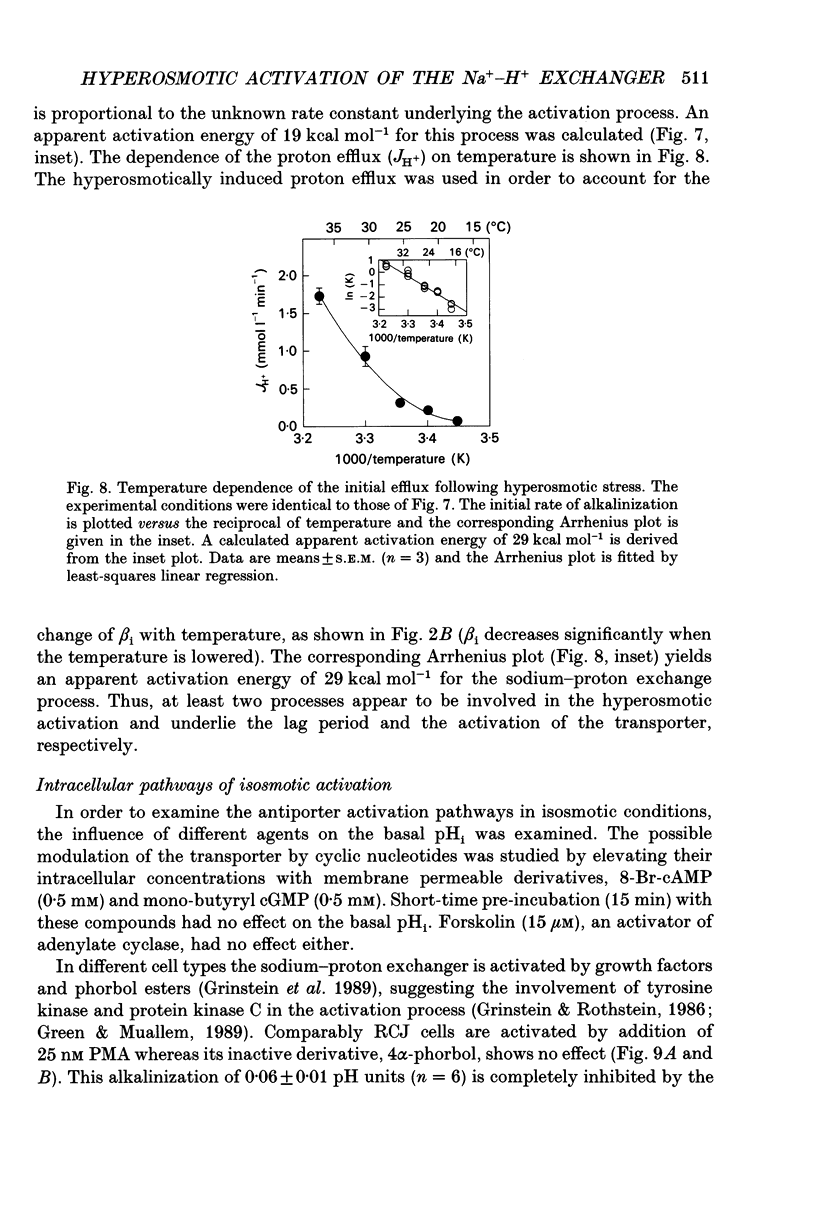
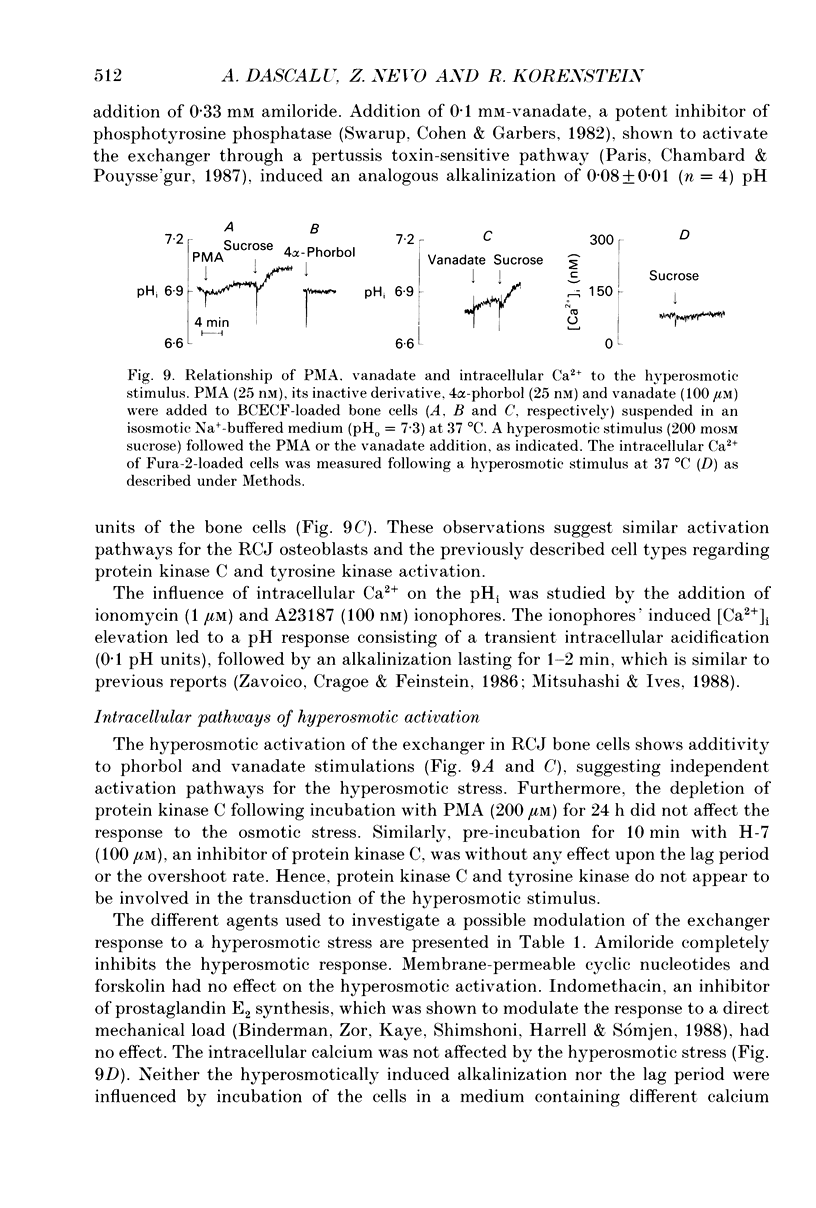
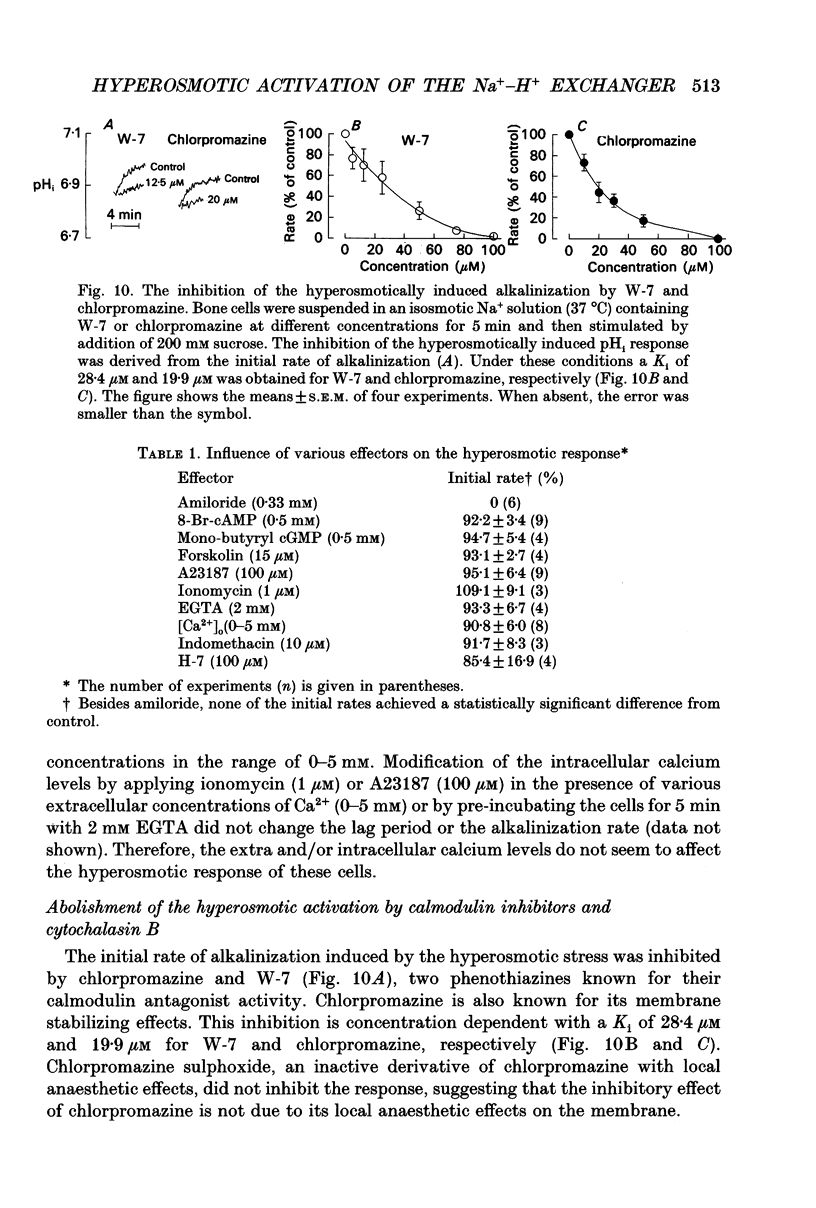
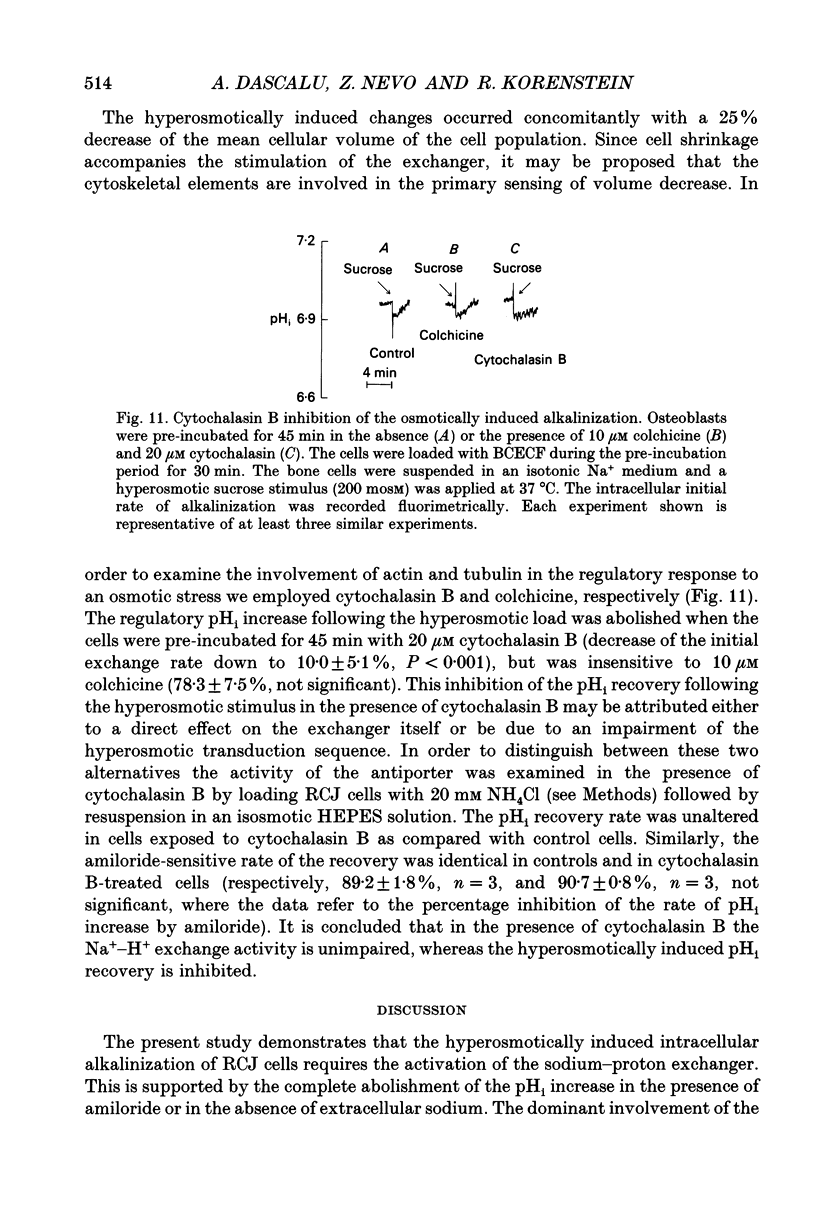
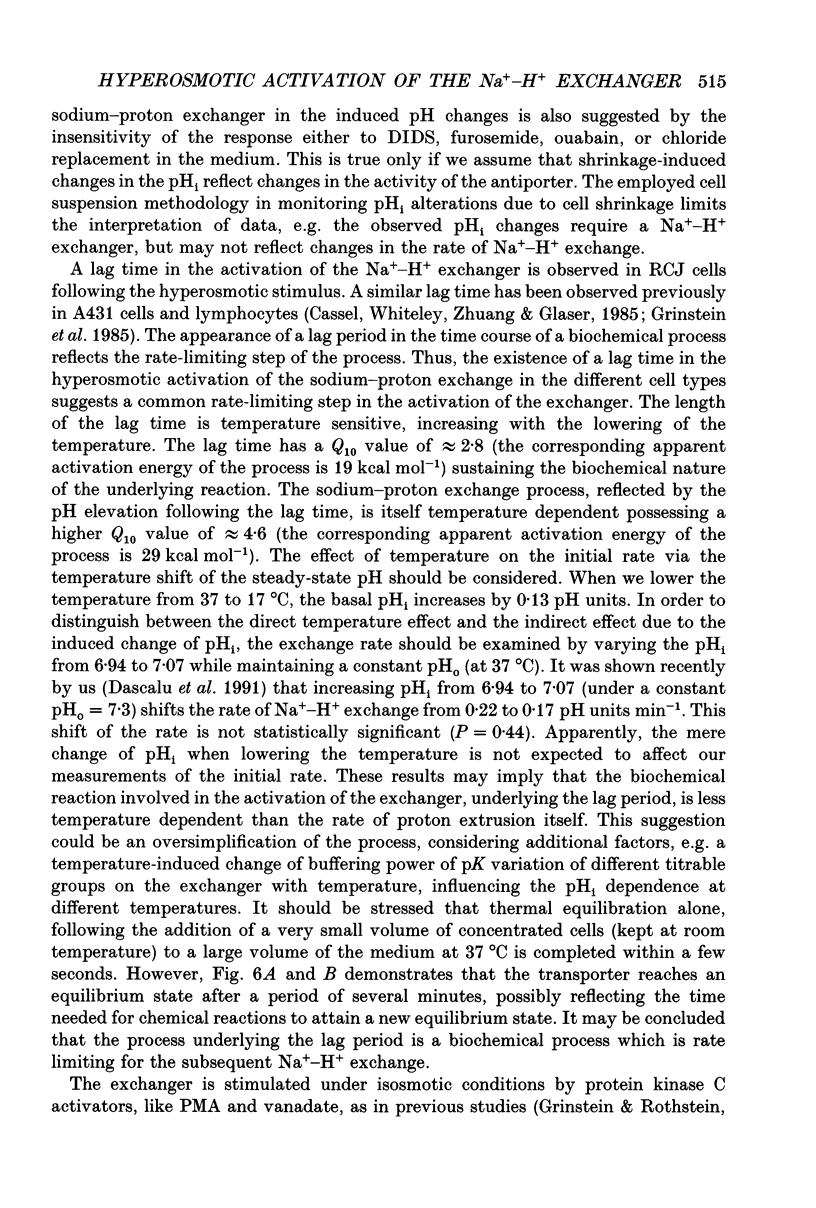
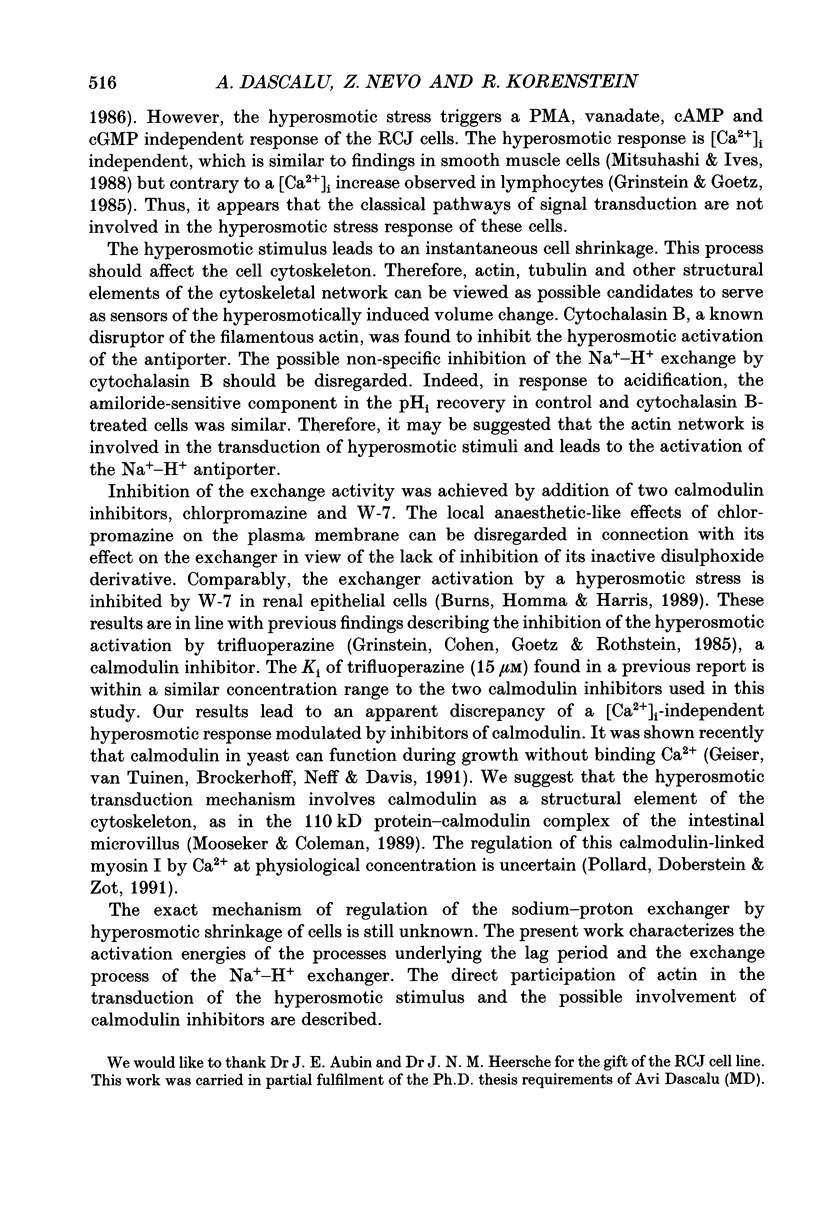
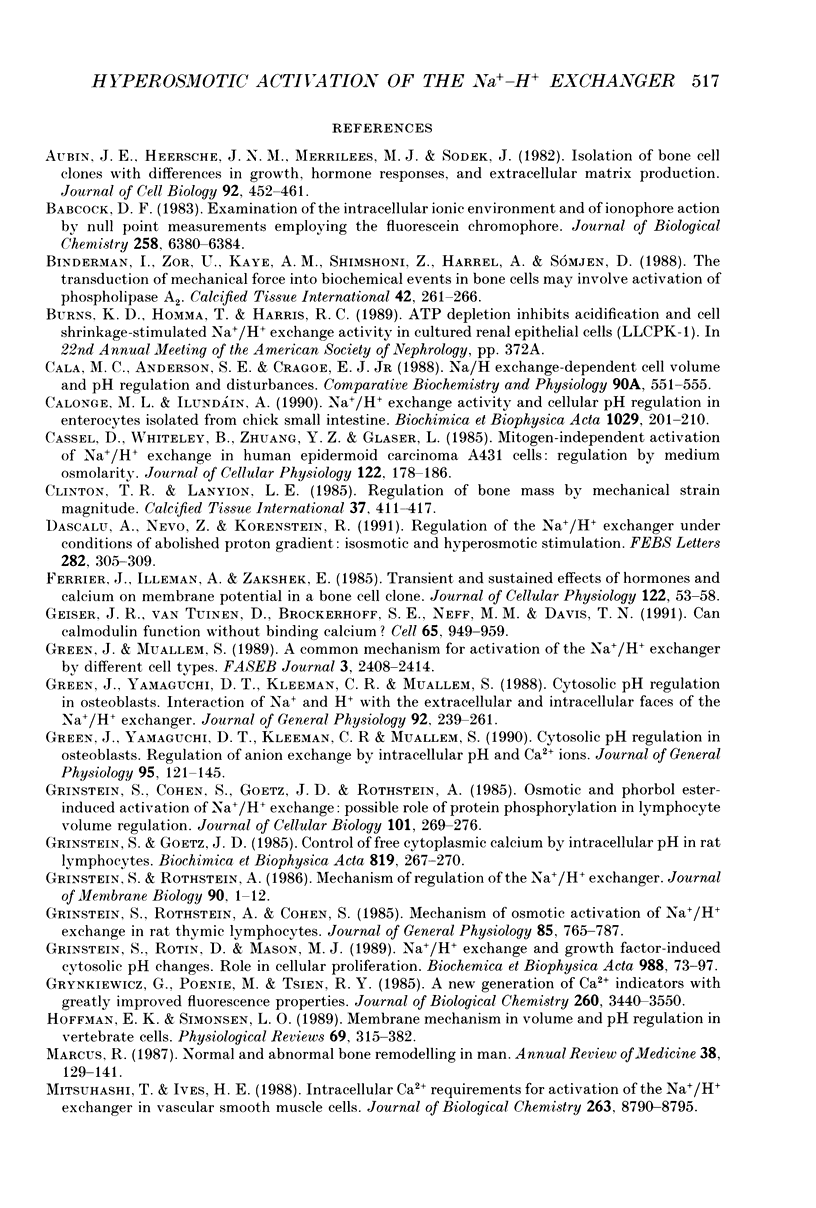
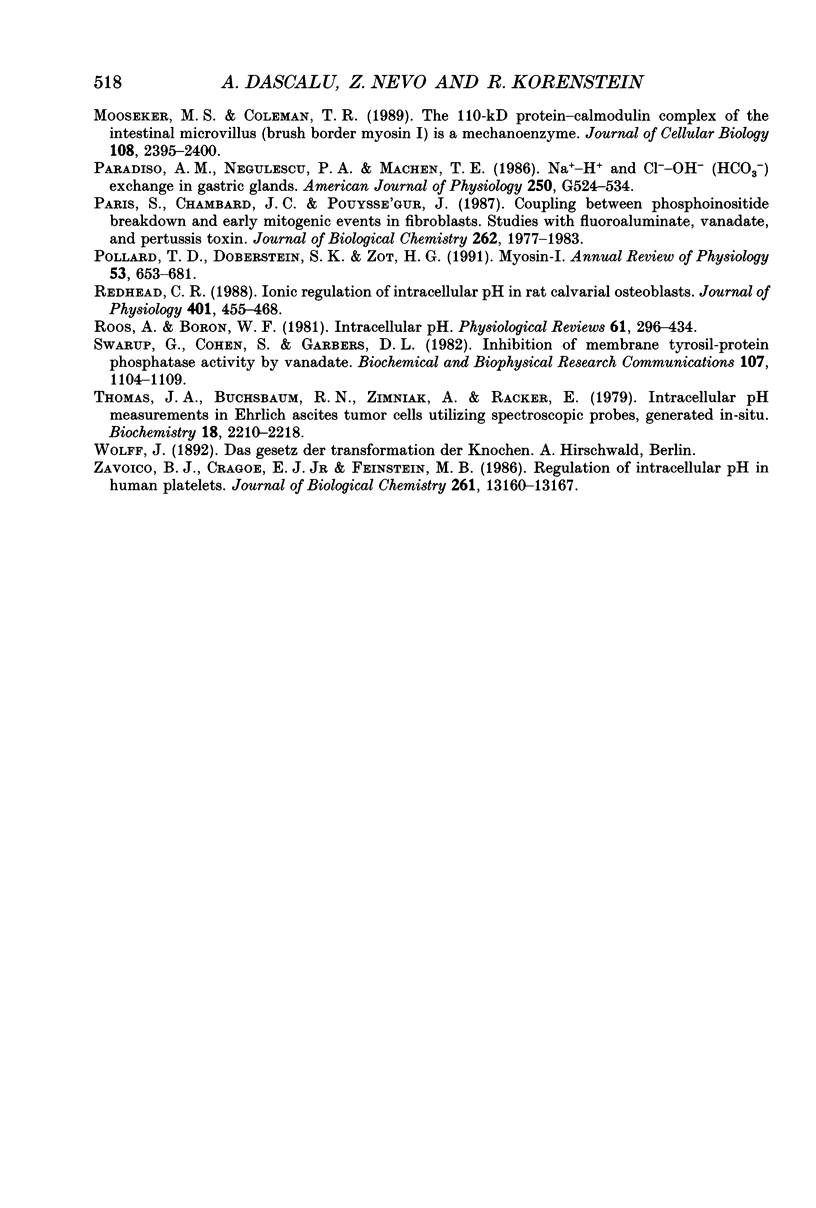
Selected References
These references are in PubMed. This may not be the complete list of references from this article.
- Aubin J. E., Heersche J. N., Merrilees M. J., Sodek J. Isolation of bone cell clones with differences in growth, hormone responses, and extracellular matrix production. J Cell Biol. 1982 Feb;92(2):452–461. doi: 10.1083/jcb.92.2.452. [DOI] [PMC free article] [PubMed] [Google Scholar]
- Babcock D. F. Examination of the intracellular ionic environment and of ionophore action by null point measurements employing the fluorescein chromophore. J Biol Chem. 1983 May 25;258(10):6380–6389. [PubMed] [Google Scholar]
- Binderman I., Zor U., Kaye A. M., Shimshoni Z., Harell A., Sömjen D. The transduction of mechanical force into biochemical events in bone cells may involve activation of phospholipase A2. Calcif Tissue Int. 1988 Apr;42(4):261–266. doi: 10.1007/BF02553753. [DOI] [PubMed] [Google Scholar]
- Cala P. M., Anderson S. E., Cragoe E. J., Jr Na/H exchange-dependent cell volume and pH regulation and disturbances. Comp Biochem Physiol A Comp Physiol. 1988;90(4):551–555. doi: 10.1016/0300-9629(88)90666-4. [DOI] [PubMed] [Google Scholar]
- Calonge M. L., Ilundáin A. Na(+)-H+ exchange activity and cellular pH regulation in enterocytes isolated from chick small intestine. Biochim Biophys Acta. 1990 Nov 16;1029(2):201–210. doi: 10.1016/0005-2736(90)90155-h. [DOI] [PubMed] [Google Scholar]
- Cassel D., Whiteley B., Zhuang Y. X., Glaser L. Mitogen-independent activation of Na+/H+ exchange in human epidermoid carcinoma A431 cells: regulation by medium osmolarity. J Cell Physiol. 1985 Feb;122(2):178–186. doi: 10.1002/jcp.1041220203. [DOI] [PubMed] [Google Scholar]
- Dascalu A., Nevo Z., Korenstein R. Regulation of the Na+/H+ exchanger under conditions of abolished proton gradient: isosmotic and hyperosmotic stimulation. FEBS Lett. 1991 May 6;282(2):305–309. doi: 10.1016/0014-5793(91)80501-s. [DOI] [PubMed] [Google Scholar]
- Ferrier J., Illeman A., Zakshek E. Transient and sustained effects of hormones and calcium on membrane potential in a bone cell clone. J Cell Physiol. 1985 Jan;122(1):53–58. doi: 10.1002/jcp.1041220109. [DOI] [PubMed] [Google Scholar]
- Geiser J. R., van Tuinen D., Brockerhoff S. E., Neff M. M., Davis T. N. Can calmodulin function without binding calcium? Cell. 1991 Jun 14;65(6):949–959. doi: 10.1016/0092-8674(91)90547-c. [DOI] [PubMed] [Google Scholar]
- Green J., Muallem S. A common mechanism for activation of the Na+/H+ exchanger by different types of stimuli. FASEB J. 1989 Oct;3(12):2408–2414. doi: 10.1096/fasebj.3.12.2551762. [DOI] [PubMed] [Google Scholar]
- Green J., Yamaguchi D. T., Kleeman C. R., Muallem S. Cytosolic pH regulation in osteoblasts. Interaction of Na+ and H+ with the extracellular and intracellular faces of the Na+/H+ exchanger. J Gen Physiol. 1988 Aug;92(2):239–261. doi: 10.1085/jgp.92.2.239. [DOI] [PMC free article] [PubMed] [Google Scholar]
- Green J., Yamaguchi D. T., Kleeman C. R., Muallem S. Cytosolic pH regulation in osteoblasts. Regulation of anion exchange by intracellular pH and Ca2+ ions. J Gen Physiol. 1990 Jan;95(1):121–145. doi: 10.1085/jgp.95.1.121. [DOI] [PMC free article] [PubMed] [Google Scholar]
- Grinstein S., Cohen S., Goetz J. D., Rothstein A. Osmotic and phorbol ester-induced activation of Na+/H+ exchange: possible role of protein phosphorylation in lymphocyte volume regulation. J Cell Biol. 1985 Jul;101(1):269–276. doi: 10.1083/jcb.101.1.269. [DOI] [PMC free article] [PubMed] [Google Scholar]
- Grinstein S., Goetz J. D. Control of free cytoplasmic calcium by intracellular pH in rat lymphocytes. Biochim Biophys Acta. 1985 Oct 10;819(2):267–270. doi: 10.1016/0005-2736(85)90183-x. [DOI] [PubMed] [Google Scholar]
- Grinstein S., Rothstein A., Cohen S. Mechanism of osmotic activation of Na+/H+ exchange in rat thymic lymphocytes. J Gen Physiol. 1985 May;85(5):765–787. doi: 10.1085/jgp.85.5.765. [DOI] [PMC free article] [PubMed] [Google Scholar]
- Grinstein S., Rothstein A. Mechanisms of regulation of the Na+/H+ exchanger. J Membr Biol. 1986;90(1):1–12. doi: 10.1007/BF01869680. [DOI] [PubMed] [Google Scholar]
- Grinstein S., Rotin D., Mason M. J. Na+/H+ exchange and growth factor-induced cytosolic pH changes. Role in cellular proliferation. Biochim Biophys Acta. 1989 Jan 18;988(1):73–97. doi: 10.1016/0304-4157(89)90004-x. [DOI] [PubMed] [Google Scholar]
- Grynkiewicz G., Poenie M., Tsien R. Y. A new generation of Ca2+ indicators with greatly improved fluorescence properties. J Biol Chem. 1985 Mar 25;260(6):3440–3450. [PubMed] [Google Scholar]
- Hoffmann E. K., Simonsen L. O. Membrane mechanisms in volume and pH regulation in vertebrate cells. Physiol Rev. 1989 Apr;69(2):315–382. doi: 10.1152/physrev.1989.69.2.315. [DOI] [PubMed] [Google Scholar]
- Marcus R. Normal and abnormal bone remodeling in man. Annu Rev Med. 1987;38:129–141. doi: 10.1146/annurev.me.38.020187.001021. [DOI] [PubMed] [Google Scholar]
- Mitsuhashi T., Ives H. E. Intracellular Ca2+ requirement for activation of the Na+/H+ exchanger in vascular smooth muscle cells. J Biol Chem. 1988 Jun 25;263(18):8790–8795. [PubMed] [Google Scholar]
- Mooseker M. S., Coleman T. R. The 110-kD protein-calmodulin complex of the intestinal microvillus (brush border myosin I) is a mechanoenzyme. J Cell Biol. 1989 Jun;108(6):2395–2400. doi: 10.1083/jcb.108.6.2395. [DOI] [PMC free article] [PubMed] [Google Scholar]
- Paradiso A. M., Negulescu P. A., Machen T. E. Na+-H+ and Cl(-)-OH-(HCO3-) exchange in gastric glands. Am J Physiol. 1986 Apr;250(4 Pt 1):G524–G534. doi: 10.1152/ajpgi.1986.250.4.G524. [DOI] [PubMed] [Google Scholar]
- Paris S., Chambard J. C., Pouysségur J. Coupling between phosphoinositide breakdown and early mitogenic events in fibroblasts. Studies with fluoroaluminate, vanadate, and pertussis toxin. J Biol Chem. 1987 Feb 15;262(5):1977–1983. [PubMed] [Google Scholar]
- Pollard T. D., Doberstein S. K., Zot H. G. Myosin-I. Annu Rev Physiol. 1991;53:653–681. doi: 10.1146/annurev.ph.53.030191.003253. [DOI] [PubMed] [Google Scholar]
- Redhead C. R. Ionic regulation of intracellular pH in rat calvarial osteoblasts. J Physiol. 1988 Jul;401:455–468. doi: 10.1113/jphysiol.1988.sp017172. [DOI] [PMC free article] [PubMed] [Google Scholar]
- Roos A., Boron W. F. Intracellular pH. Physiol Rev. 1981 Apr;61(2):296–434. doi: 10.1152/physrev.1981.61.2.296. [DOI] [PubMed] [Google Scholar]
- Rubin C. T., Lanyon L. E. Regulation of bone mass by mechanical strain magnitude. Calcif Tissue Int. 1985 Jul;37(4):411–417. doi: 10.1007/BF02553711. [DOI] [PubMed] [Google Scholar]
- Swarup G., Cohen S., Garbers D. L. Inhibition of membrane phosphotyrosyl-protein phosphatase activity by vanadate. Biochem Biophys Res Commun. 1982 Aug;107(3):1104–1109. doi: 10.1016/0006-291x(82)90635-0. [DOI] [PubMed] [Google Scholar]
- Thomas J. A., Buchsbaum R. N., Zimniak A., Racker E. Intracellular pH measurements in Ehrlich ascites tumor cells utilizing spectroscopic probes generated in situ. Biochemistry. 1979 May 29;18(11):2210–2218. doi: 10.1021/bi00578a012. [DOI] [PubMed] [Google Scholar]
- Zavoico G. B., Cragoe E. J., Jr, Feinstein M. B. Regulation of intracellular pH in human platelets. Effects of thrombin, A23187, and ionomycin and evidence for activation of Na+/H+ exchange and its inhibition by amiloride analogs. J Biol Chem. 1986 Oct 5;261(28):13160–13167. [PubMed] [Google Scholar]