Abstract
1. Low-frequency, rhythmic synaptic potentials and their ability to evoke and modulate membrane potential oscillations in thalamocortical (TC) cells of the cat dorsal lateral geniculate nucleus (dLGN) were investigated using intracellular recordings in a brain slice preparation. Three types of rhythmic synaptic potentials were distinguished: EPSPs, IPSPs and 'complex synaptic potentials' consisting of an IPSP followed by an EPSP. 2. The frequency of all three types of synaptic potentials was insensitive to changes in the membrane potential. At potentials positive to -50 mV, the EPSPs and the complex potentials gave rise to action potentials, while between -65 and -80 mV all three types of synaptic potential evoked low-threshold Ca2+ potentials. TC cells which displayed rhythmic synaptic potentials were either cells that showed spontaneous pacemaker oscillation or cells that were brought to oscillate by the rhythmic EPSPs or depolarizing (i.e. reversed IPSPs. 3. The low-frequency (1.9 +/- 0.2 Hz), rhythmic EPSPs were observed in 23 (out of 192) cells, were abolished by tetrodotoxin (TTX; n = 4) and by the combined application of DL-2-amino-5-phosphonovaleric acid and 6-cyano-7-nitroquinoxaline-2,3-dione (n = 3), and were insensitive to bicuculline (n = 4). Paired intracellular recordings (n = 32) demonstrated the presence of simultaneously occurring EPSPs in a pair of cells situated 75 microns apart. 4. The low-frequency (2.2 +/- 0.3 Hz), rhythmic IPSPs were observed in 5 (out of 192) cells, were blocked by bicuculline (n = 3), and reversed in polarity at -65 mV. The low-frequency (1.3 +/- 0.3 Hz), rhythmic 'complex potentials' were observed in 5 (out of 192) cells and were abolished by TTX (n = 2). 5. Intracellular depolarizing current pulses delivered at different phases of the pacemaker oscillations revealed the existence of two different types of phase resetting. Furthermore, a current pulse of critical amplitude and duration applied at a specific phase of the cycle abolished the pacemaker oscillations. 6. These results indicate that the low-frequency, rhythmic synaptic potentials recorded in TC cells of the dLGN (i) originate from other TC cells that are in the pacemaker oscillating mode, (ii) are capable of driving other TC cells to oscillate rhythmically, or of modulating the frequency of pacemaker oscillations, and (iii) provide a means by which oscillatory activities of TC cells can be synchronized in the absence of sensory, cortical and reticular thalamic inputs.
Full text
PDF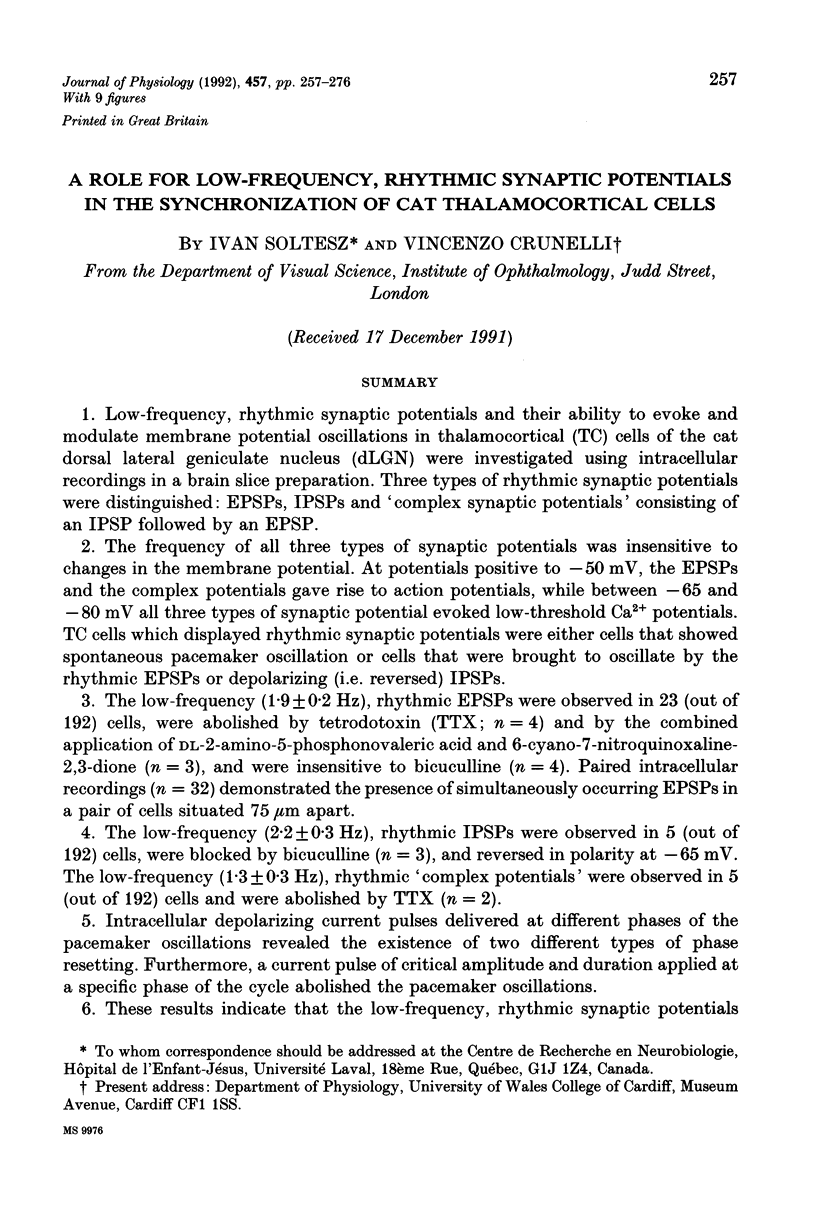
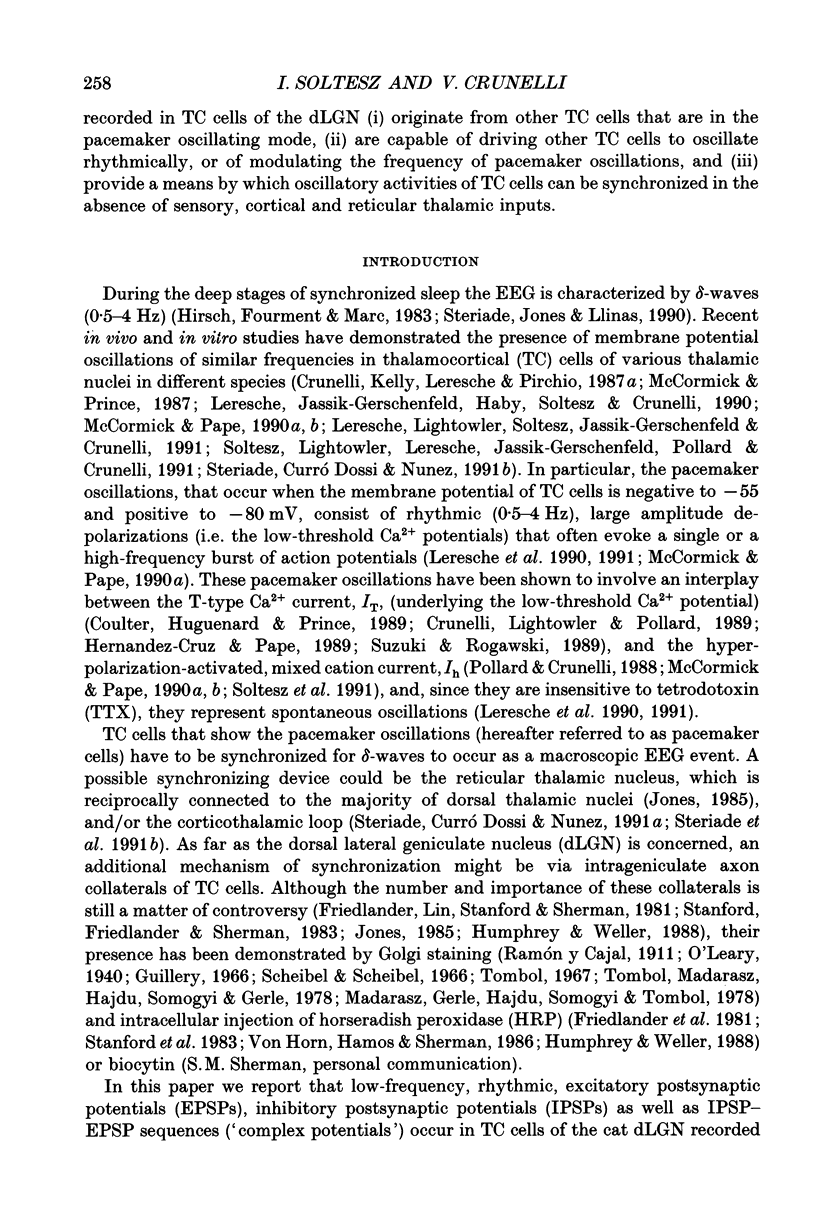
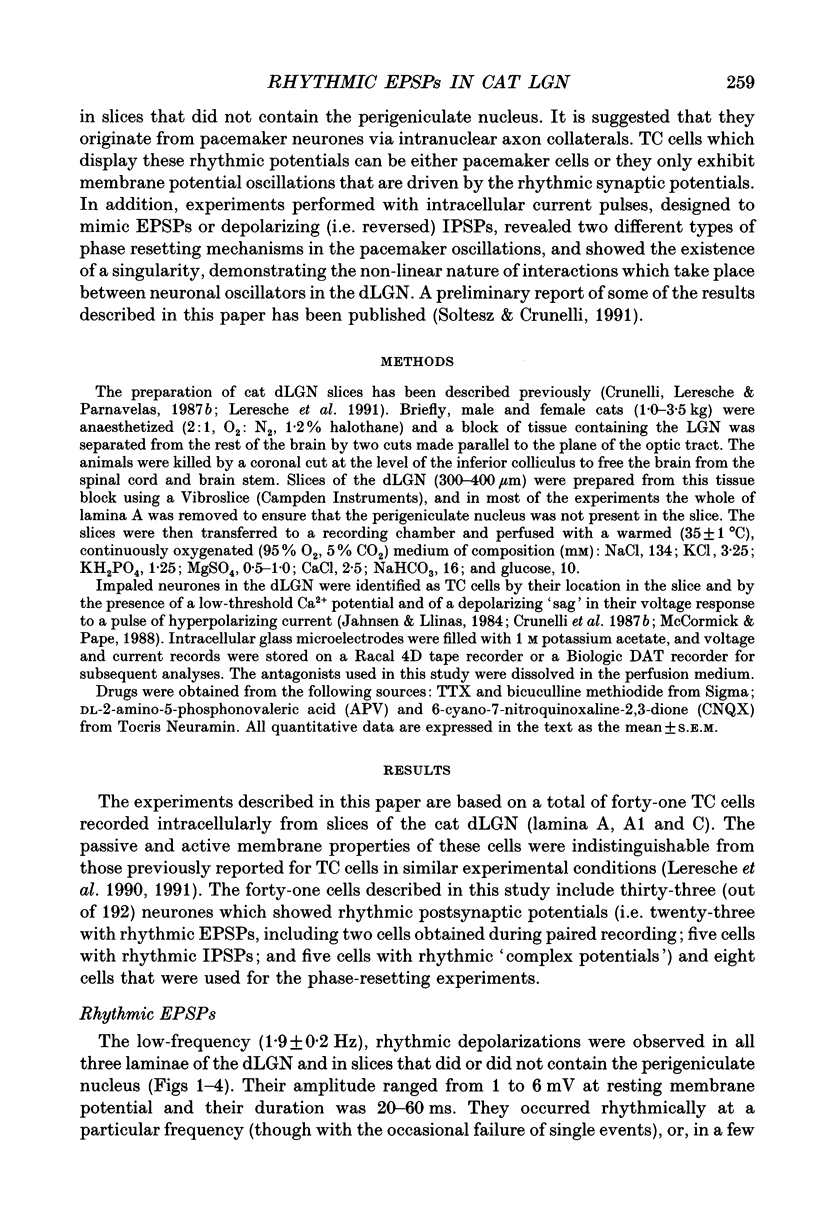
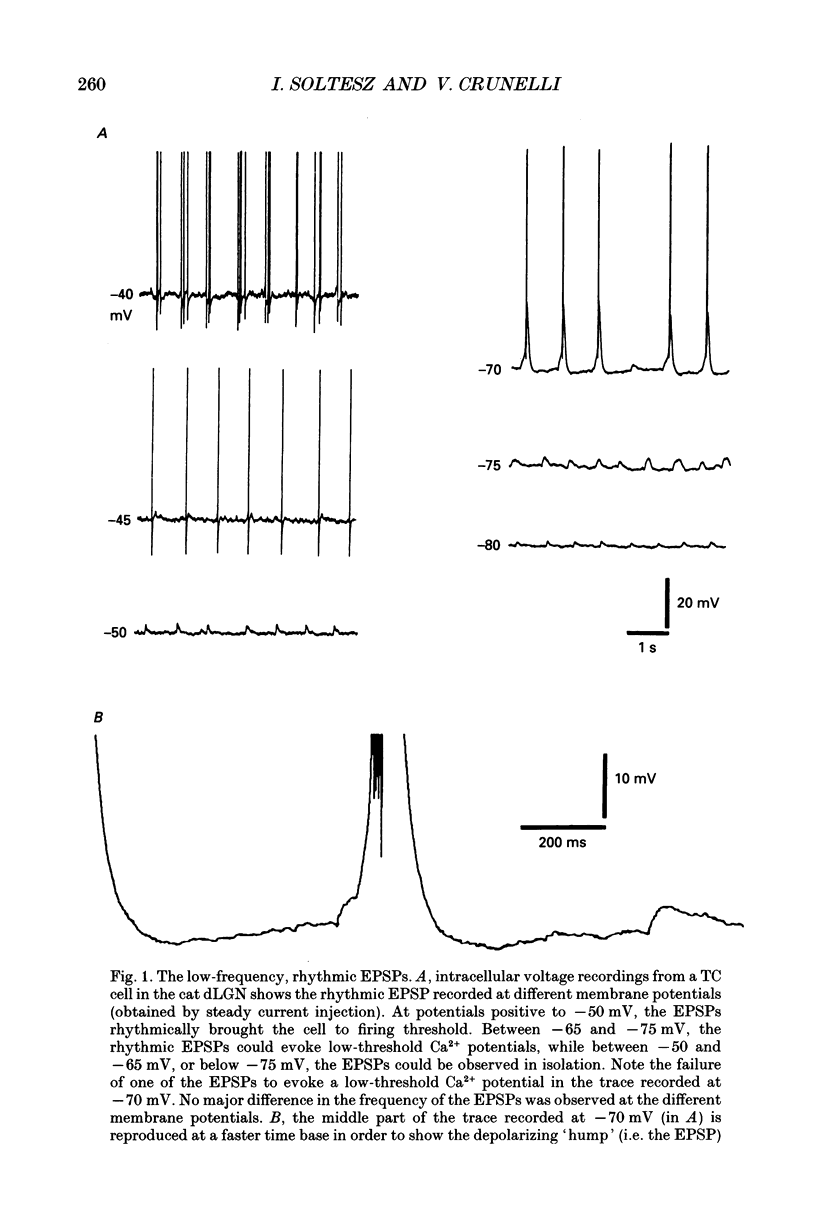
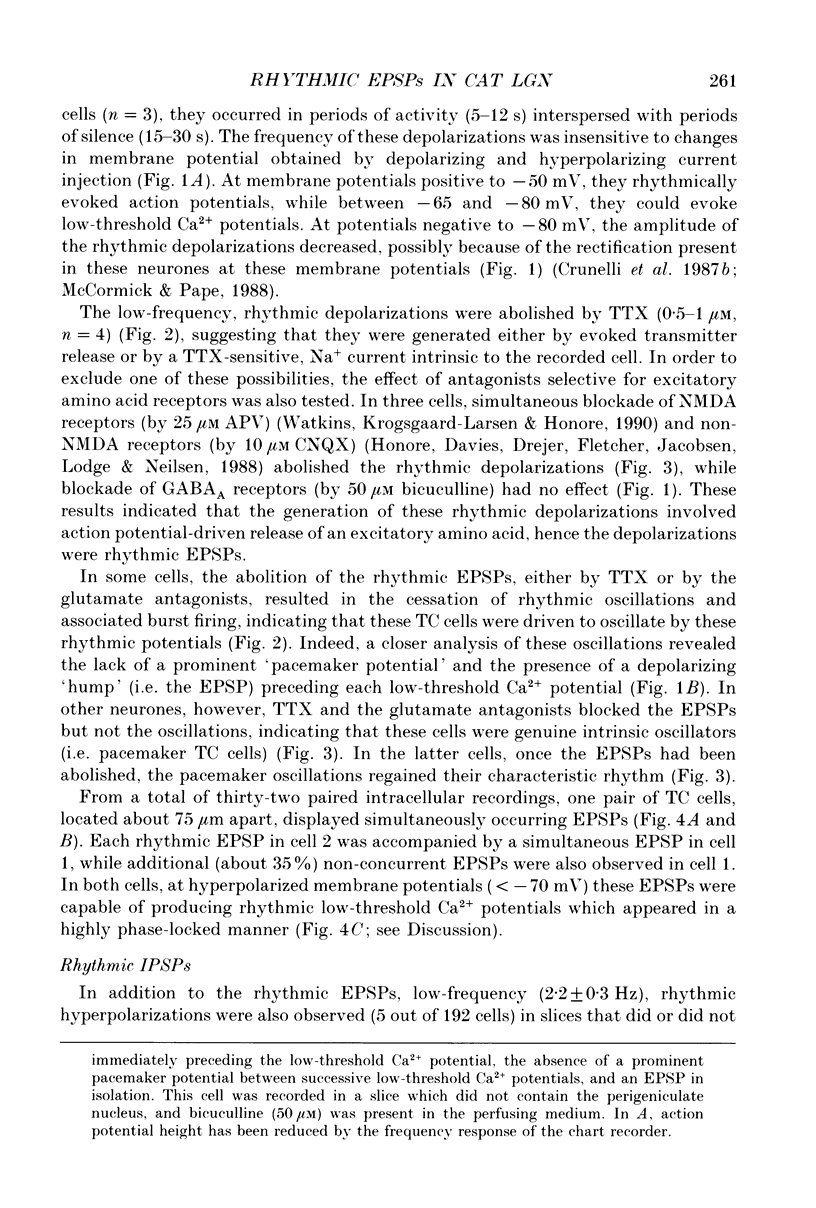
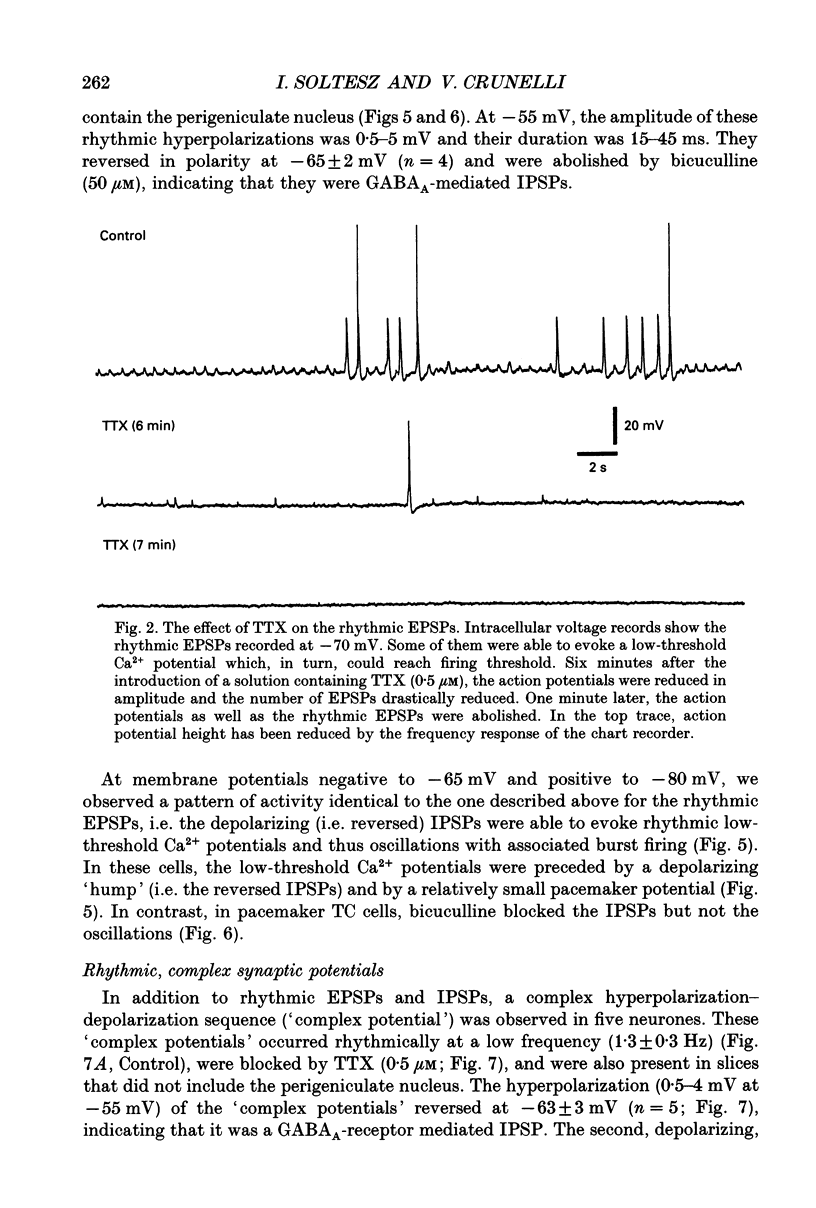
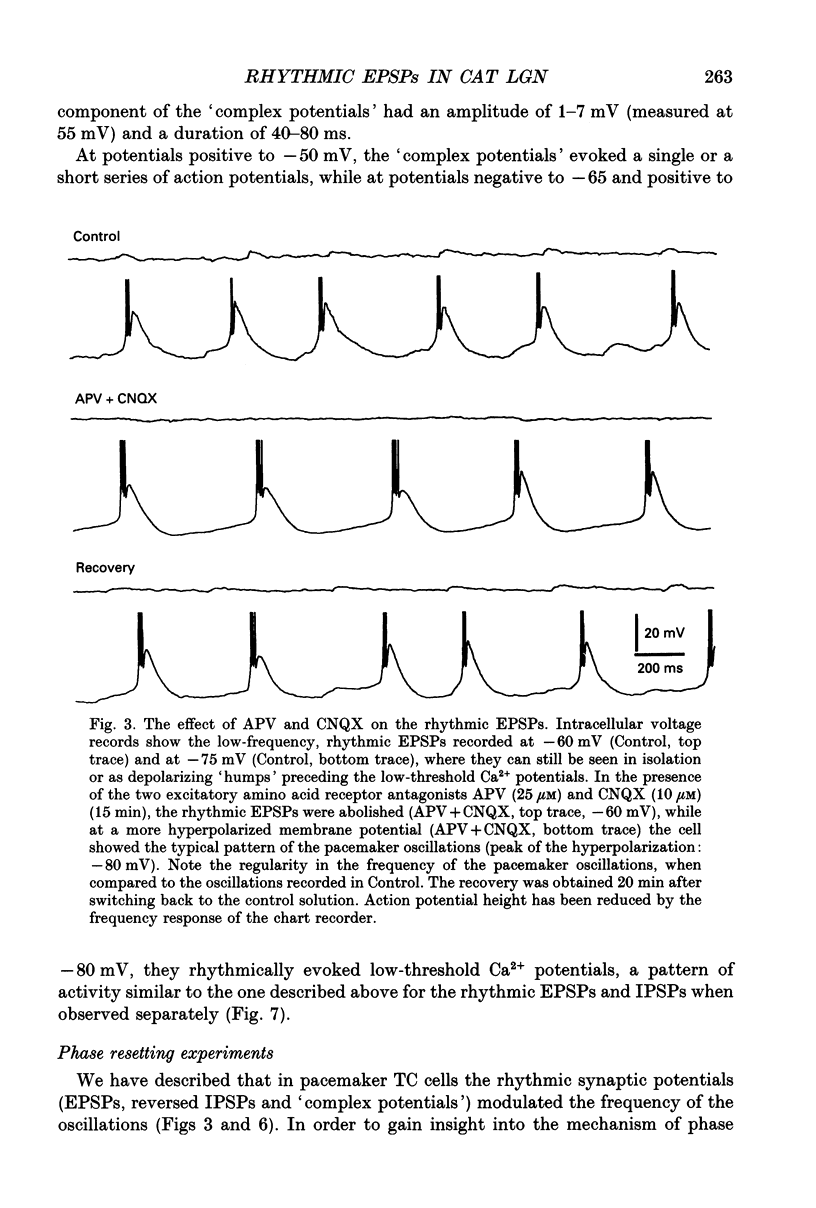
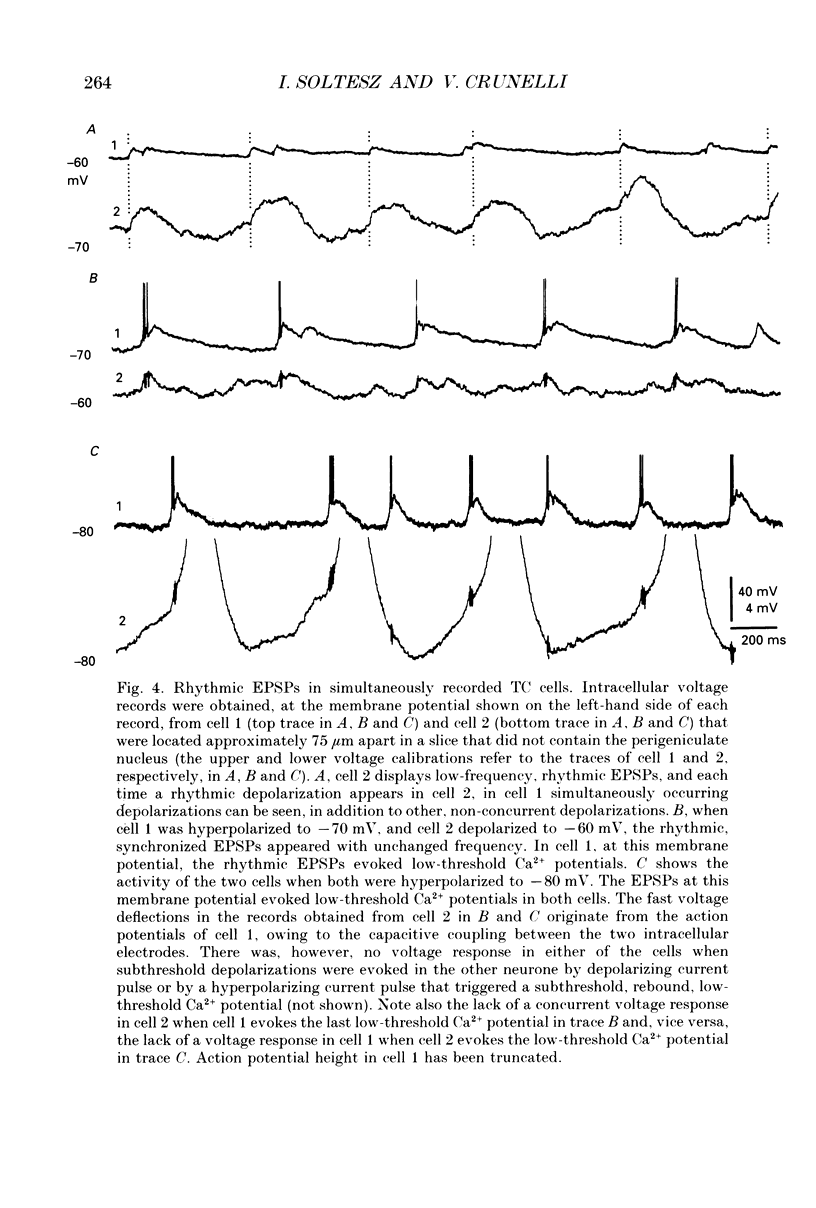
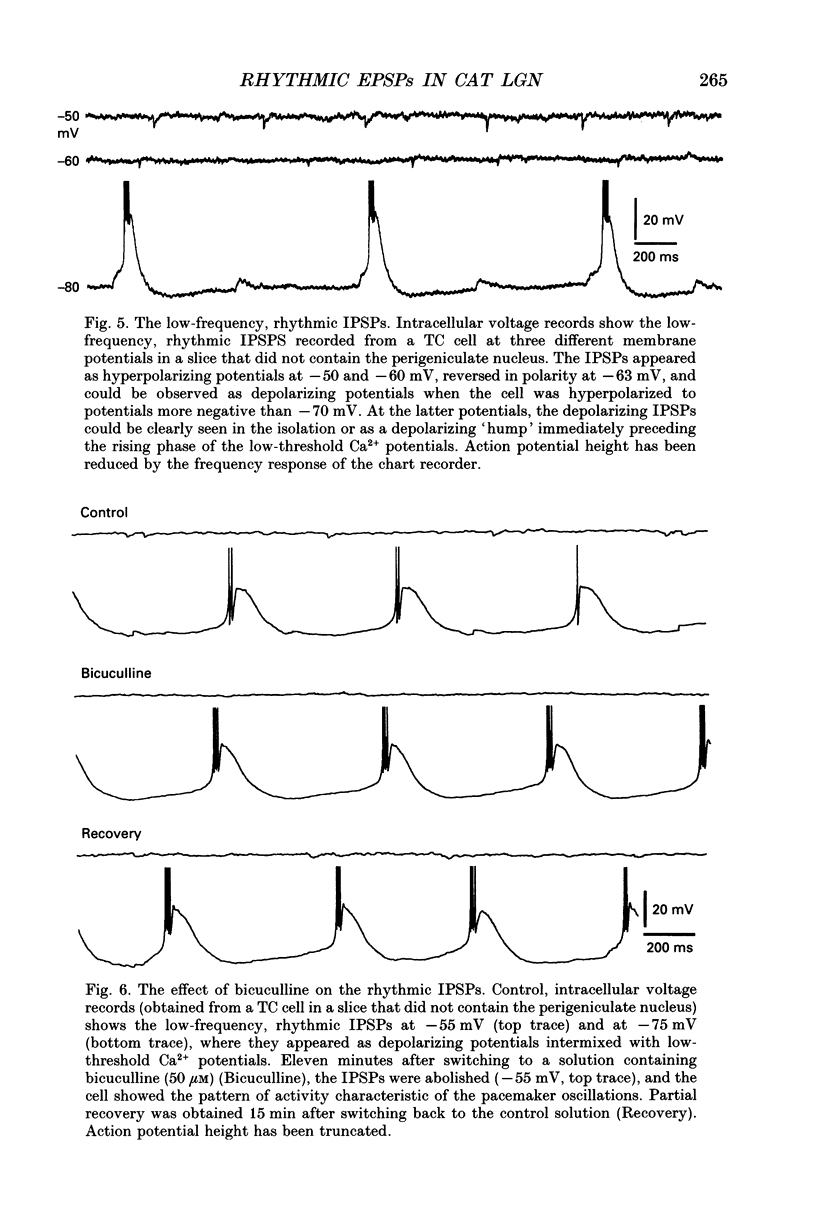
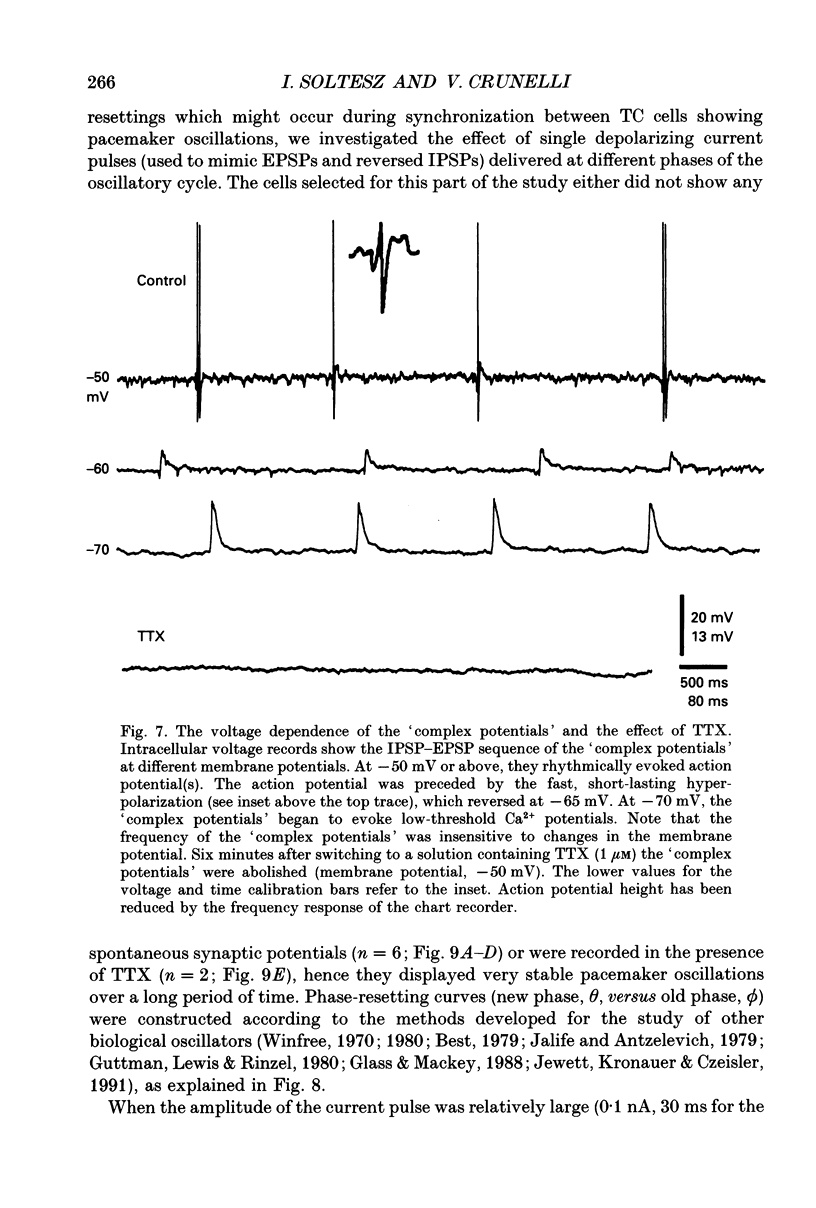
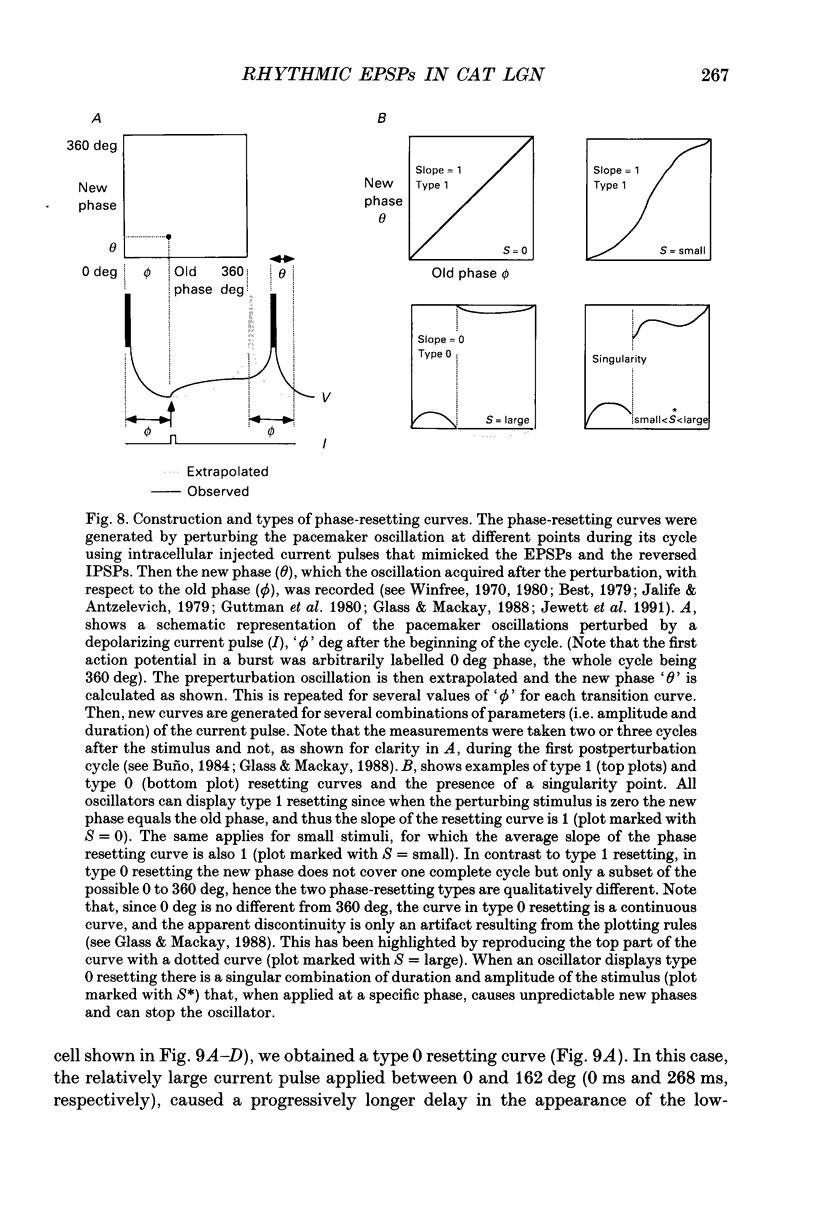
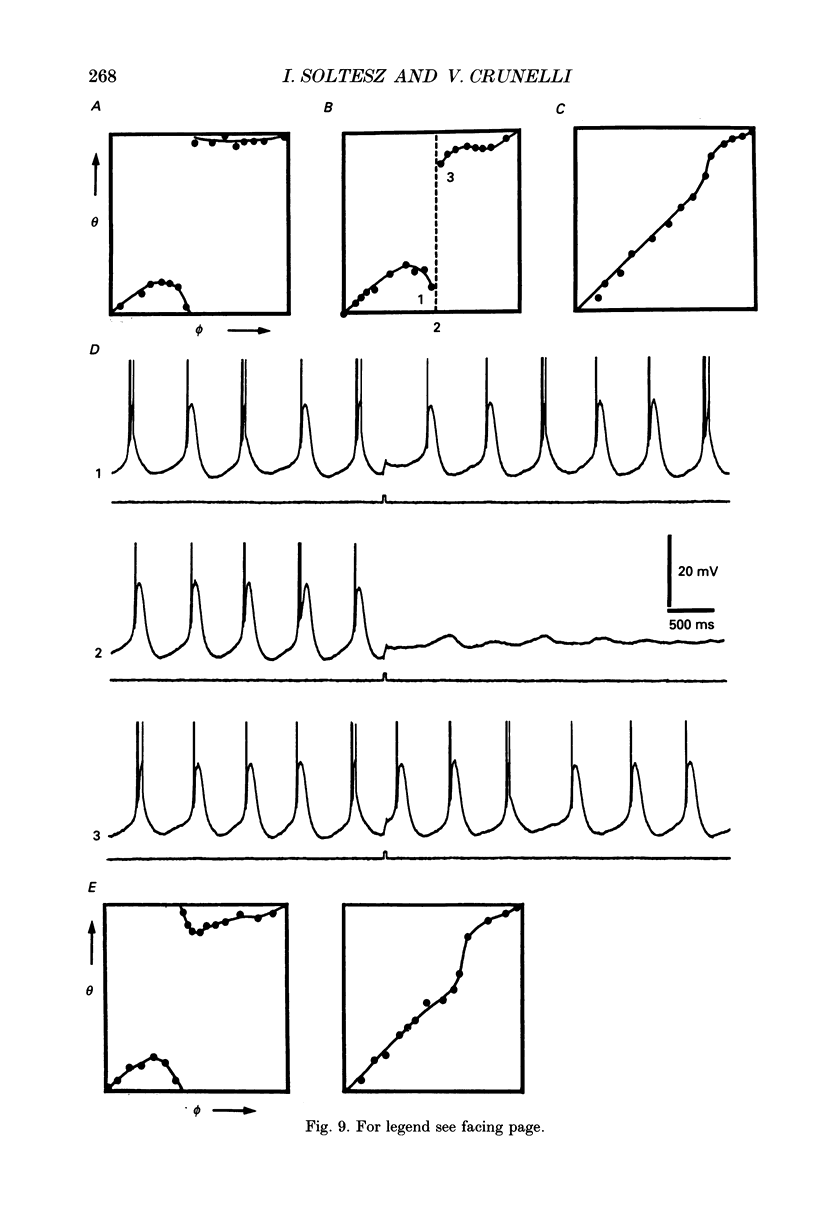
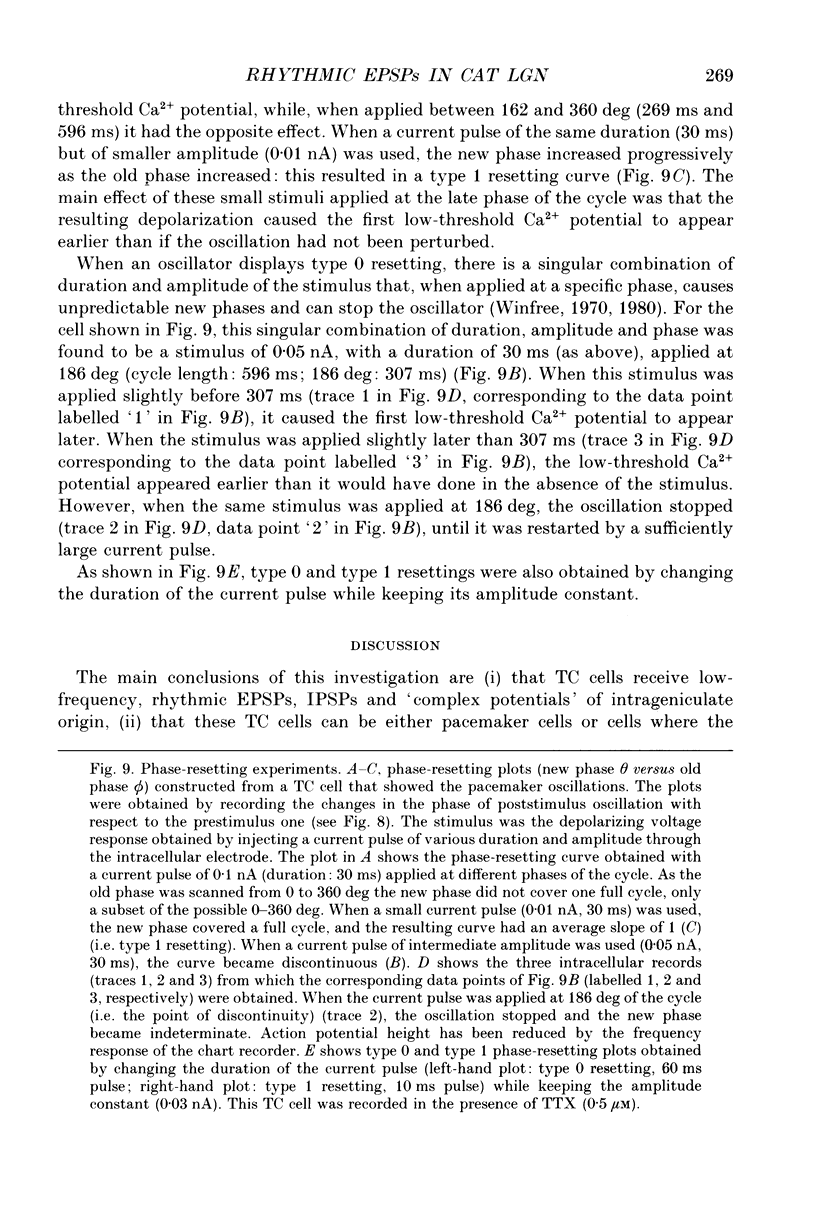
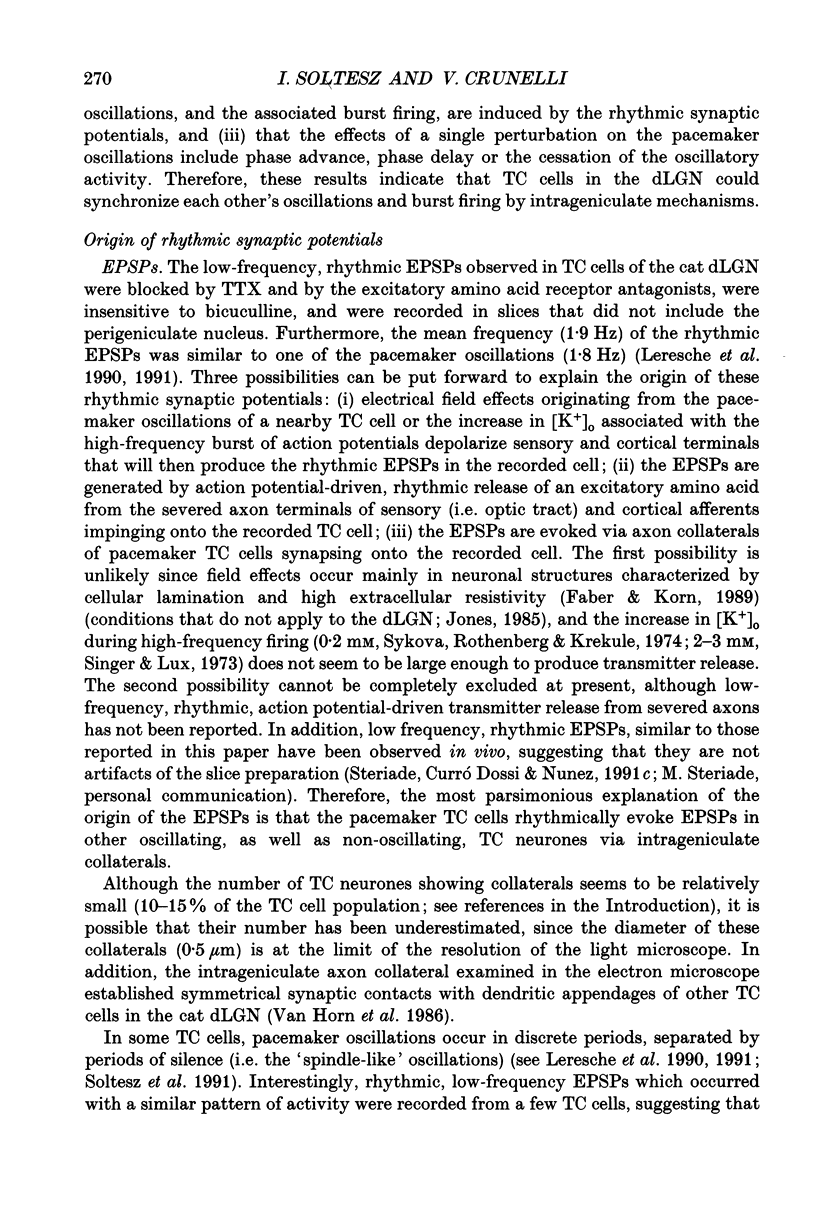
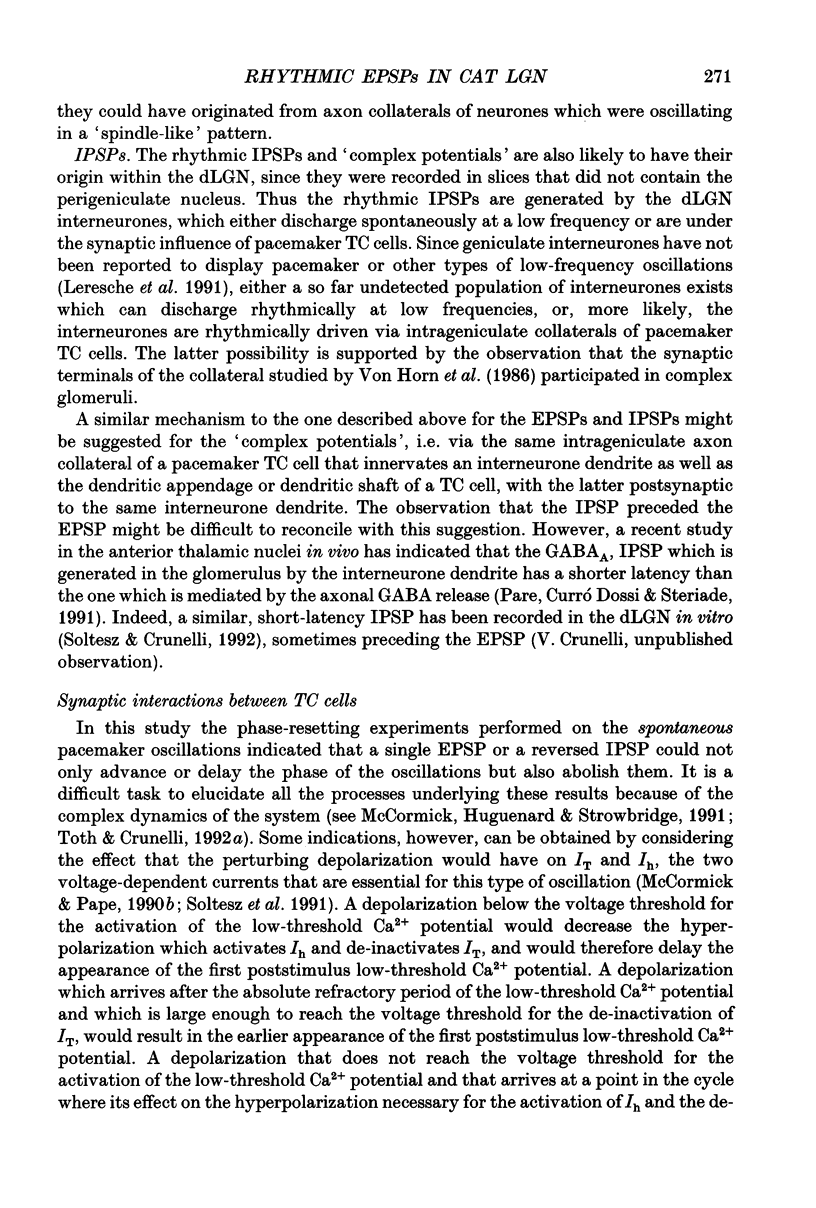
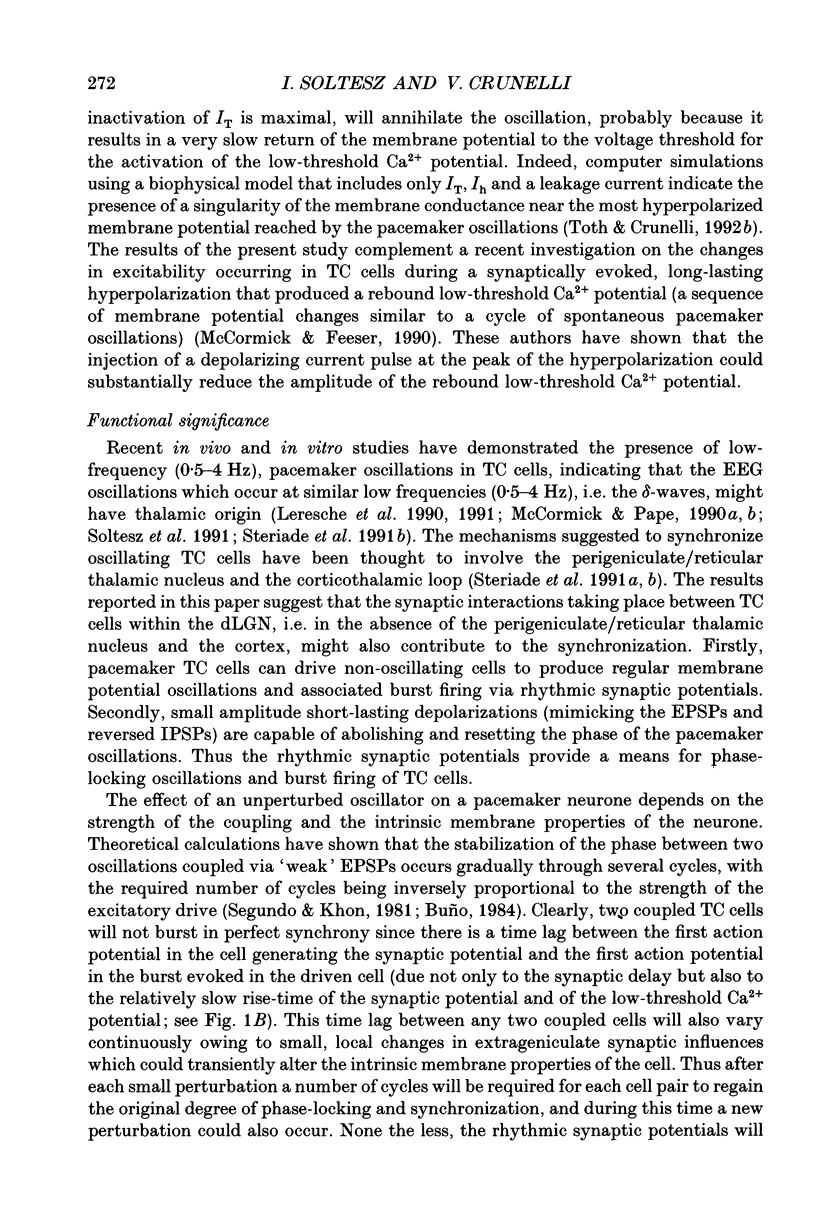
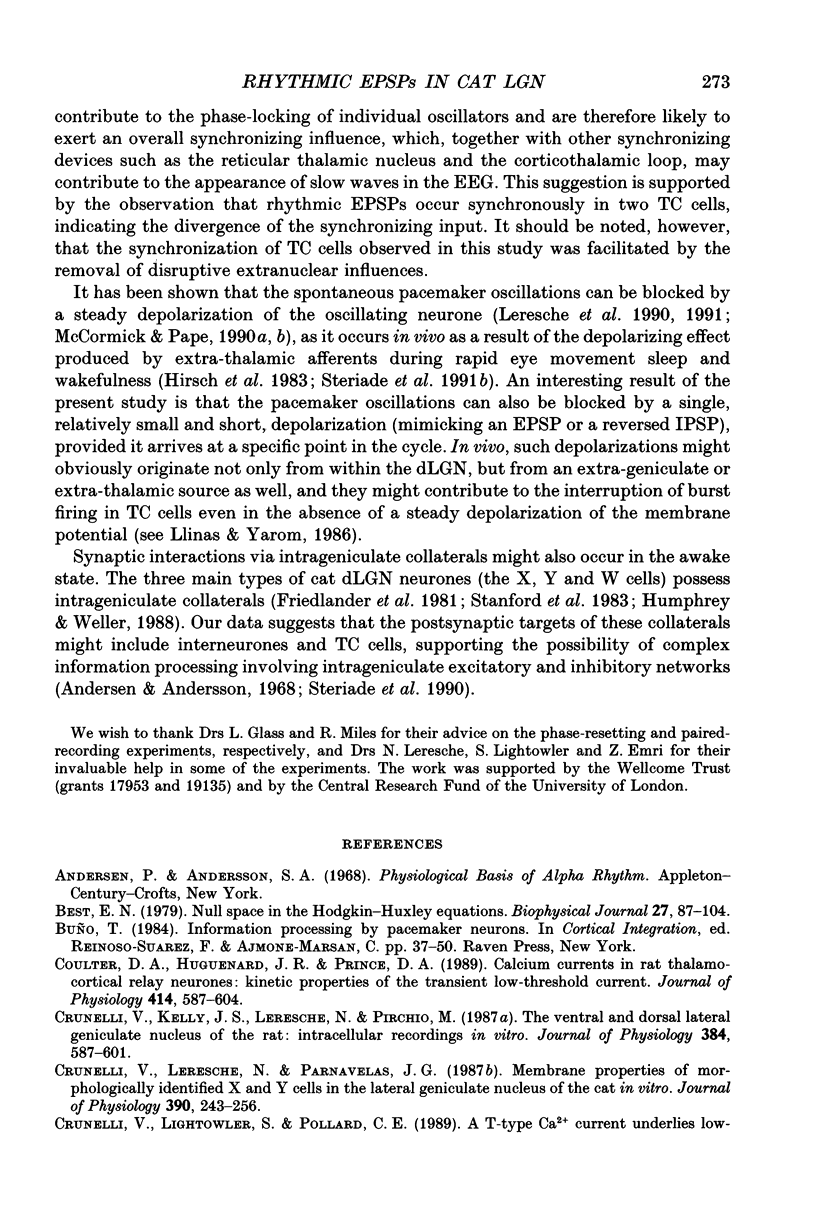
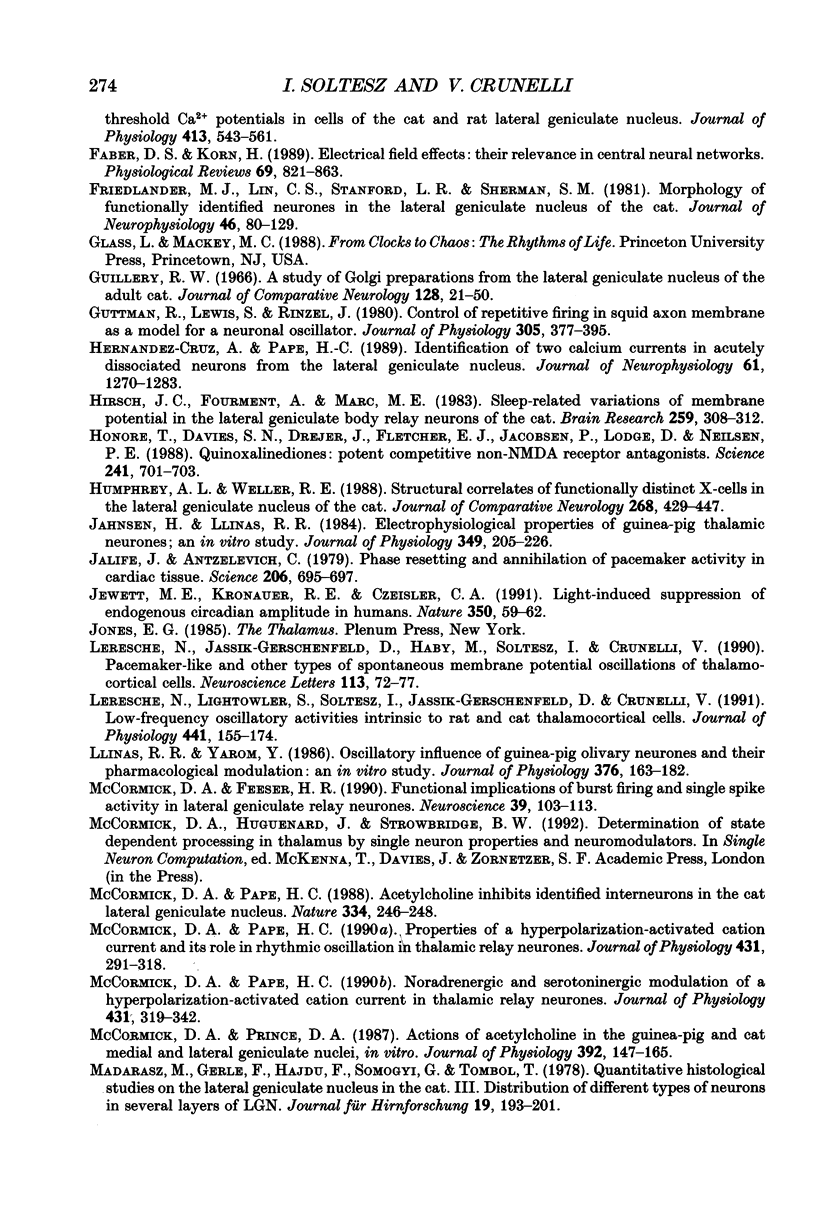
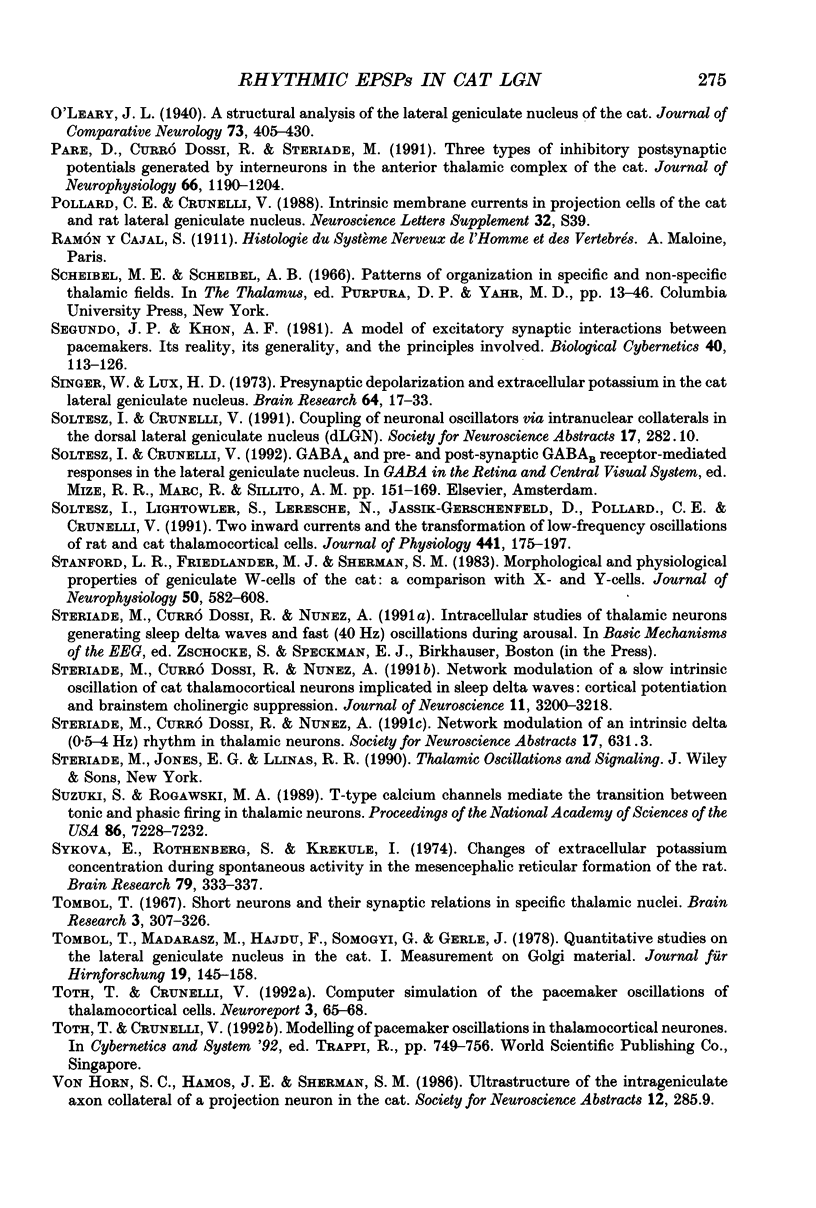
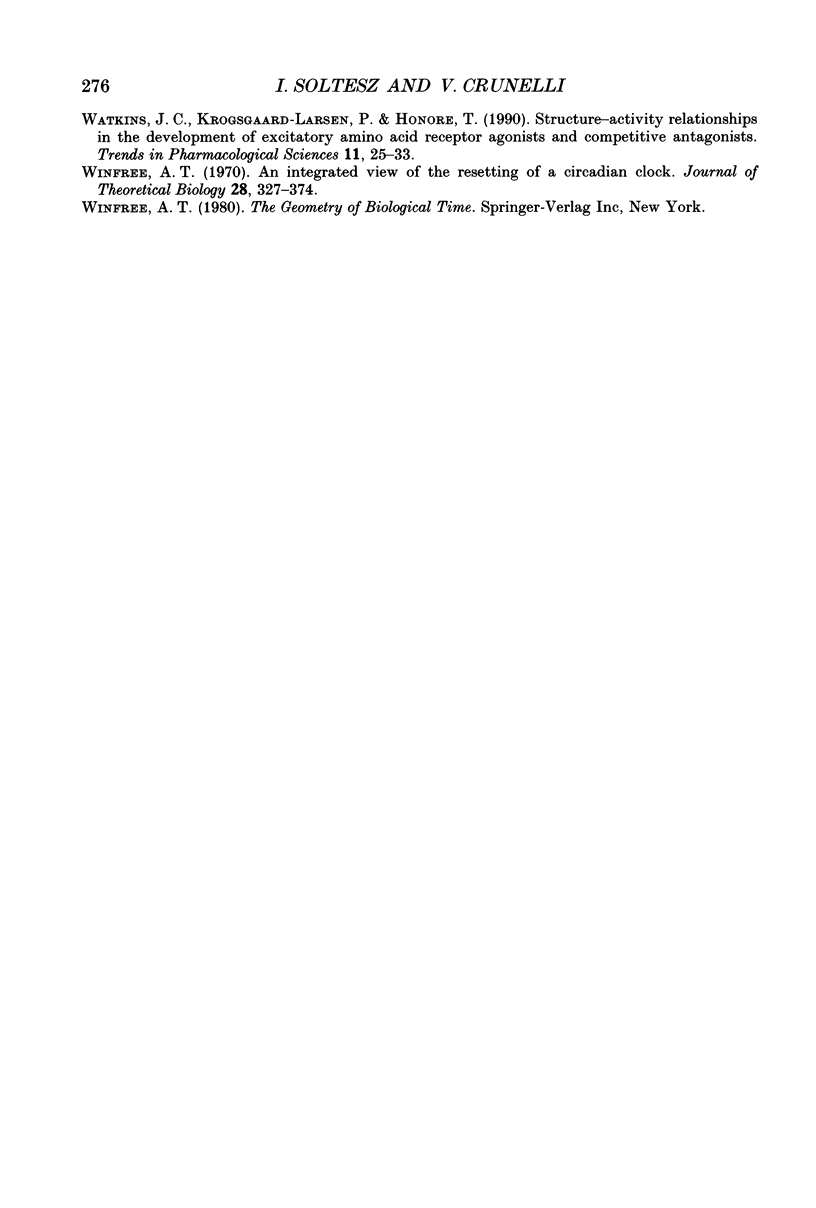
Selected References
These references are in PubMed. This may not be the complete list of references from this article.
- Best E. N. Null space in the Hodgkin-Huxley Equations. A critical test. Biophys J. 1979 Jul;27(1):87–104. doi: 10.1016/S0006-3495(79)85204-2. [DOI] [PMC free article] [PubMed] [Google Scholar]
- Coulter D. A., Huguenard J. R., Prince D. A. Calcium currents in rat thalamocortical relay neurones: kinetic properties of the transient, low-threshold current. J Physiol. 1989 Jul;414:587–604. doi: 10.1113/jphysiol.1989.sp017705. [DOI] [PMC free article] [PubMed] [Google Scholar]
- Crunelli V., Kelly J. S., Leresche N., Pirchio M. The ventral and dorsal lateral geniculate nucleus of the rat: intracellular recordings in vitro. J Physiol. 1987 Mar;384:587–601. doi: 10.1113/jphysiol.1987.sp016471. [DOI] [PMC free article] [PubMed] [Google Scholar]
- Crunelli V., Leresche N., Parnavelas J. G. Membrane properties of morphologically identified X and Y cells in the lateral geniculate nucleus of the cat in vitro. J Physiol. 1987 Sep;390:243–256. doi: 10.1113/jphysiol.1987.sp016697. [DOI] [PMC free article] [PubMed] [Google Scholar]
- Crunelli V., Lightowler S., Pollard C. E. A T-type Ca2+ current underlies low-threshold Ca2+ potentials in cells of the cat and rat lateral geniculate nucleus. J Physiol. 1989 Jun;413:543–561. doi: 10.1113/jphysiol.1989.sp017668. [DOI] [PMC free article] [PubMed] [Google Scholar]
- Faber D. S., Korn H. Electrical field effects: their relevance in central neural networks. Physiol Rev. 1989 Jul;69(3):821–863. doi: 10.1152/physrev.1989.69.3.821. [DOI] [PubMed] [Google Scholar]
- Friedlander M. J., Lin C. S., Stanford L. R., Sherman S. M. Morphology of functionally identified neurons in lateral geniculate nucleus of the cat. J Neurophysiol. 1981 Jul;46(1):80–129. doi: 10.1152/jn.1981.46.1.80. [DOI] [PubMed] [Google Scholar]
- Guillery R. W. A study of Golgi preparations from the dorsal lateral geniculate nucleus of the adult cat. J Comp Neurol. 1966 Sep;128(1):21–50. doi: 10.1002/cne.901280104. [DOI] [PubMed] [Google Scholar]
- Guttman R., Lewis S., Rinzel J. Control of repetitive firing in squid axon membrane as a model for a neuroneoscillator. J Physiol. 1980 Aug;305:377–395. doi: 10.1113/jphysiol.1980.sp013370. [DOI] [PMC free article] [PubMed] [Google Scholar]
- Hernández-Cruz A., Pape H. C. Identification of two calcium currents in acutely dissociated neurons from the rat lateral geniculate nucleus. J Neurophysiol. 1989 Jun;61(6):1270–1283. doi: 10.1152/jn.1989.61.6.1270. [DOI] [PubMed] [Google Scholar]
- Hirsch J. C., Fourment A., Marc M. E. Sleep-related variations of membrane potential in the lateral geniculate body relay neurons of the cat. Brain Res. 1983 Jan 24;259(2):308–312. doi: 10.1016/0006-8993(83)91264-7. [DOI] [PubMed] [Google Scholar]
- Honoré T., Davies S. N., Drejer J., Fletcher E. J., Jacobsen P., Lodge D., Nielsen F. E. Quinoxalinediones: potent competitive non-NMDA glutamate receptor antagonists. Science. 1988 Aug 5;241(4866):701–703. doi: 10.1126/science.2899909. [DOI] [PubMed] [Google Scholar]
- Humphrey A. L., Weller R. E. Functionally distinct groups of X-cells in the lateral geniculate nucleus of the cat. J Comp Neurol. 1988 Feb 15;268(3):429–447. doi: 10.1002/cne.902680311. [DOI] [PubMed] [Google Scholar]
- Jahnsen H., Llinás R. Electrophysiological properties of guinea-pig thalamic neurones: an in vitro study. J Physiol. 1984 Apr;349:205–226. doi: 10.1113/jphysiol.1984.sp015153. [DOI] [PMC free article] [PubMed] [Google Scholar]
- Jalife J., Antzelevitch C. Phase resetting and annihilation of pacemaker activity in cardiac tissue. Science. 1979 Nov 9;206(4419):695–697. doi: 10.1126/science.493975. [DOI] [PubMed] [Google Scholar]
- Jewett M. E., Kronauer R. E., Czeisler C. A. Light-induced suppression of endogenous circadian amplitude in humans. Nature. 1991 Mar 7;350(6313):59–62. doi: 10.1038/350059a0. [DOI] [PubMed] [Google Scholar]
- Leresche N., Jassik-Gerschenfeld D., Haby M., Soltesz I., Crunelli V. Pacemaker-like and other types of spontaneous membrane potential oscillations of thalamocortical cells. Neurosci Lett. 1990 May 18;113(1):72–77. doi: 10.1016/0304-3940(90)90497-w. [DOI] [PubMed] [Google Scholar]
- Leresche N., Lightowler S., Soltesz I., Jassik-Gerschenfeld D., Crunelli V. Low-frequency oscillatory activities intrinsic to rat and cat thalamocortical cells. J Physiol. 1991 Sep;441:155–174. doi: 10.1113/jphysiol.1991.sp018744. [DOI] [PMC free article] [PubMed] [Google Scholar]
- Llinás R., Yarom Y. Oscillatory properties of guinea-pig inferior olivary neurones and their pharmacological modulation: an in vitro study. J Physiol. 1986 Jul;376:163–182. doi: 10.1113/jphysiol.1986.sp016147. [DOI] [PMC free article] [PubMed] [Google Scholar]
- Madarász M., Gerle J., Hajdu F., Somogyi G., Tömböl T. Quantitative histological studies on the lateral geniculate nucleus in the cat. III. Distribution of different types of neurons in the several layers of LGN. J Hirnforsch. 1978;19(3):193–201. [PubMed] [Google Scholar]
- McCormick D. A., Feeser H. R. Functional implications of burst firing and single spike activity in lateral geniculate relay neurons. Neuroscience. 1990;39(1):103–113. doi: 10.1016/0306-4522(90)90225-s. [DOI] [PubMed] [Google Scholar]
- McCormick D. A., Pape H. C. Acetylcholine inhibits identified interneurons in the cat lateral geniculate nucleus. Nature. 1988 Jul 21;334(6179):246–248. doi: 10.1038/334246a0. [DOI] [PubMed] [Google Scholar]
- McCormick D. A., Pape H. C. Noradrenergic and serotonergic modulation of a hyperpolarization-activated cation current in thalamic relay neurones. J Physiol. 1990 Dec;431:319–342. doi: 10.1113/jphysiol.1990.sp018332. [DOI] [PMC free article] [PubMed] [Google Scholar]
- McCormick D. A., Pape H. C. Properties of a hyperpolarization-activated cation current and its role in rhythmic oscillation in thalamic relay neurones. J Physiol. 1990 Dec;431:291–318. doi: 10.1113/jphysiol.1990.sp018331. [DOI] [PMC free article] [PubMed] [Google Scholar]
- McCormick D. A., Prince D. A. Actions of acetylcholine in the guinea-pig and cat medial and lateral geniculate nuclei, in vitro. J Physiol. 1987 Nov;392:147–165. doi: 10.1113/jphysiol.1987.sp016774. [DOI] [PMC free article] [PubMed] [Google Scholar]
- Paré D., Dossi R. C., Steriade M. Three types of inhibitory postsynaptic potentials generated by interneurons in the anterior thalamic complex of cat. J Neurophysiol. 1991 Oct;66(4):1190–1204. doi: 10.1152/jn.1991.66.4.1190. [DOI] [PubMed] [Google Scholar]
- Segundo J. P., Kohn A. F. A model of excitatory synaptic interactions between pacemakers. Its reality, its generality, and the principles involved. Biol Cybern. 1981;40(2):113–126. doi: 10.1007/BF00344290. [DOI] [PubMed] [Google Scholar]
- Singer W., Lux H. D. Presynaptic depolarization and extracellular potassium in the cat lateral geniculate nucleus. Brain Res. 1973 Dec 21;64:17–33. doi: 10.1016/0006-8993(73)90168-6. [DOI] [PubMed] [Google Scholar]
- Soltesz I., Crunelli V. GABAA and pre- and post-synaptic GABAB receptor-mediated responses in the lateral geniculate nucleus. Prog Brain Res. 1992;90:151–169. doi: 10.1016/s0079-6123(08)63613-4. [DOI] [PubMed] [Google Scholar]
- Soltesz I., Lightowler S., Leresche N., Jassik-Gerschenfeld D., Pollard C. E., Crunelli V. Two inward currents and the transformation of low-frequency oscillations of rat and cat thalamocortical cells. J Physiol. 1991 Sep;441:175–197. doi: 10.1113/jphysiol.1991.sp018745. [DOI] [PMC free article] [PubMed] [Google Scholar]
- Stanford L. R., Friedlander M. J., Sherman S. M. Morphological and physiological properties of geniculate W-cells of the cat: a comparison with X- and Y-cells. J Neurophysiol. 1983 Sep;50(3):582–608. doi: 10.1152/jn.1983.50.3.582. [DOI] [PubMed] [Google Scholar]
- Steriade M., Dossi R. C., Nuñez A. Network modulation of a slow intrinsic oscillation of cat thalamocortical neurons implicated in sleep delta waves: cortically induced synchronization and brainstem cholinergic suppression. J Neurosci. 1991 Oct;11(10):3200–3217. doi: 10.1523/JNEUROSCI.11-10-03200.1991. [DOI] [PMC free article] [PubMed] [Google Scholar]
- Suzuki S., Rogawski M. A. T-type calcium channels mediate the transition between tonic and phasic firing in thalamic neurons. Proc Natl Acad Sci U S A. 1989 Sep;86(18):7228–7232. doi: 10.1073/pnas.86.18.7228. [DOI] [PMC free article] [PubMed] [Google Scholar]
- Syková E., Rothenberg S., Krekule I. Changes of extracellular potassium concentration during spontaneous activity in the mesencephalic reticular formation of the rat. Brain Res. 1974 Oct 18;79(2):333–337. doi: 10.1016/0006-8993(74)90428-4. [DOI] [PubMed] [Google Scholar]
- Tóth T., Crunelli V. Computer simulation of the pacemaker oscillations of thalamocortical cells. Neuroreport. 1992 Jan;3(1):65–68. doi: 10.1097/00001756-199201000-00017. [DOI] [PubMed] [Google Scholar]
- Tömböl T., Madarász M., Hajdu F., Somogyi G., Gerle J. Quantitative histological studies on the lateral geniculate nucleus in the cat. I. Measurements on Golgi material. J Hirnforsch. 1978;19(2):145–158. [PubMed] [Google Scholar]
- Tömböl T. Short neurons and their synaptic relations in the specific thalamic nuclei. Brain Res. 1967 Jan 20;3(4):307–326. doi: 10.1016/0006-8993(67)90095-9. [DOI] [PubMed] [Google Scholar]
- Watkins J. C., Krogsgaard-Larsen P., Honoré T. Structure-activity relationships in the development of excitatory amino acid receptor agonists and competitive antagonists. Trends Pharmacol Sci. 1990 Jan;11(1):25–33. doi: 10.1016/0165-6147(90)90038-a. [DOI] [PubMed] [Google Scholar]
- Winfree A. T. Integrated view of resetting a circadian clock. J Theor Biol. 1970 Sep;28(3):327–374. doi: 10.1016/0022-5193(70)90075-5. [DOI] [PubMed] [Google Scholar]