Abstract
1. The effects of adenosine (50 microM) and 2-chloroadenosine (1-25 microM) were studied on Ca2+ currents in frog motor nerve endings. 2. Ca2+ currents associated with the synchronous, neurally evoked release of acetylcholine (ACh) were measured using either perineural or patch recording methods. Tetraethylammonium and/or 3,4-diaminopyridine were employed to block K+ currents. 3. Ca2+ currents were depressed by omega-conotoxin (1.5-2.5 microM), Cd2+ (100 microM-2 mM), Co2+ (500 microM-5 mM) or by a reduction of the extracellular calcium concentration. Such currents were also observed when Sr2+ was substituted for Ca2+. Both ACh release and Ca2+ currents at motor nerve endings have been reported to be insensitive to 1,4-dihydropyridine antagonists in this species. 4. Adenosine receptor agonists did not affect Ca2+ currents at concentrations that produced maximal inhibition of ACh release. 5. The effects of adenosine receptor agonists were examined on asynchronous K(+)-dependent ACh release under conditions in which the Ca2+ concentration gradient is likely to be reversed (Ca(2+)-free Ringer solution containing 1 mM EGTA). ACh release was measured by monitoring the frequency of occurrence of miniature endplate potentials (MEPPs). In Ca(2+)-free solutions containing 1 mM EGTA, high K+ depolarization caused a decrease in MEPP frequency, presumably because it elicits the efflux of Ca2+ from the nerve ending via membrane Ca2+ channels in a reverse Ca2+ gradient. 6. The Ca2+ channel blocker Co2+, which blocks the exit of Ca2+ from the nerve ending, increased the frequency of MEPPs in a concentration-dependent manner in a reverse Ca2+ gradient. 7. Adenosine or 2-chloroadenosine inhibited ACh release in a reverse Ca2+ gradient. 8. The results suggest that blockade of Ca2+ entry is not responsible for the inhibitory effects of adenosine at frog motor nerve endings.
Full text
PDF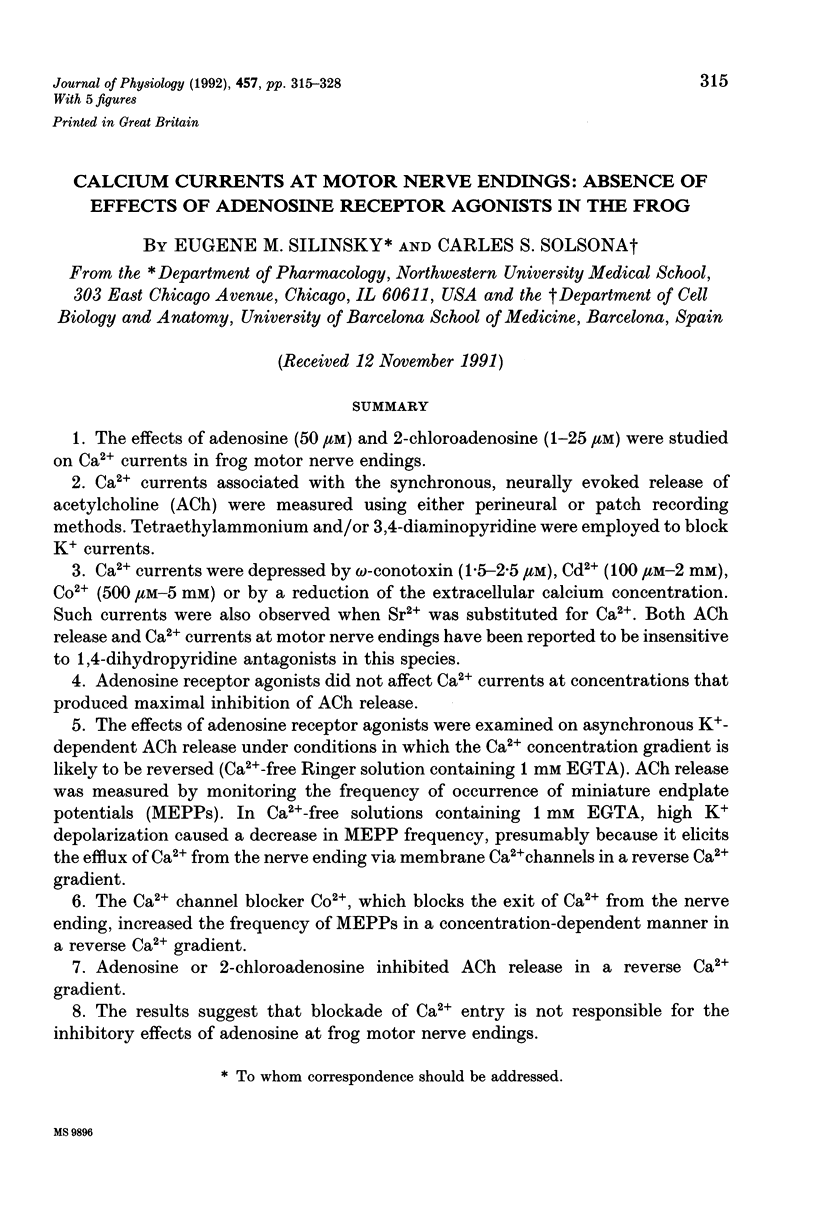
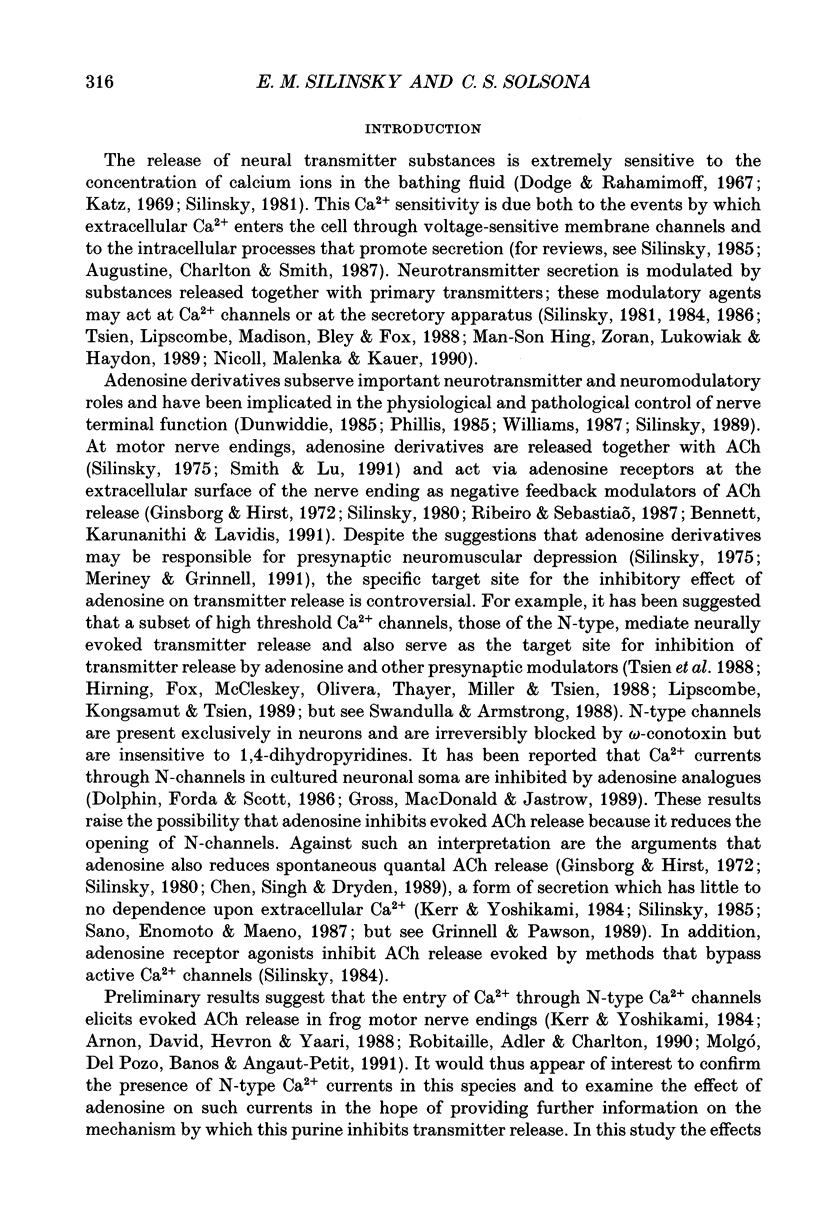
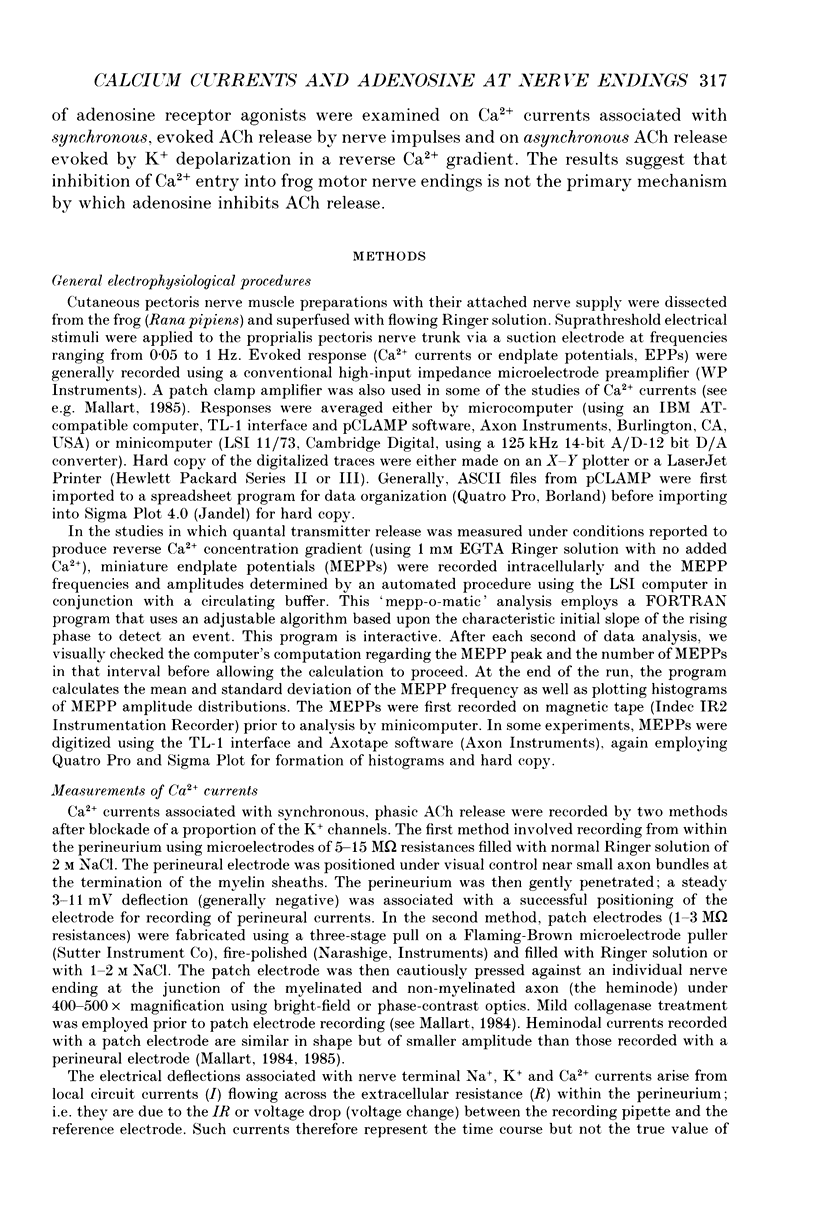
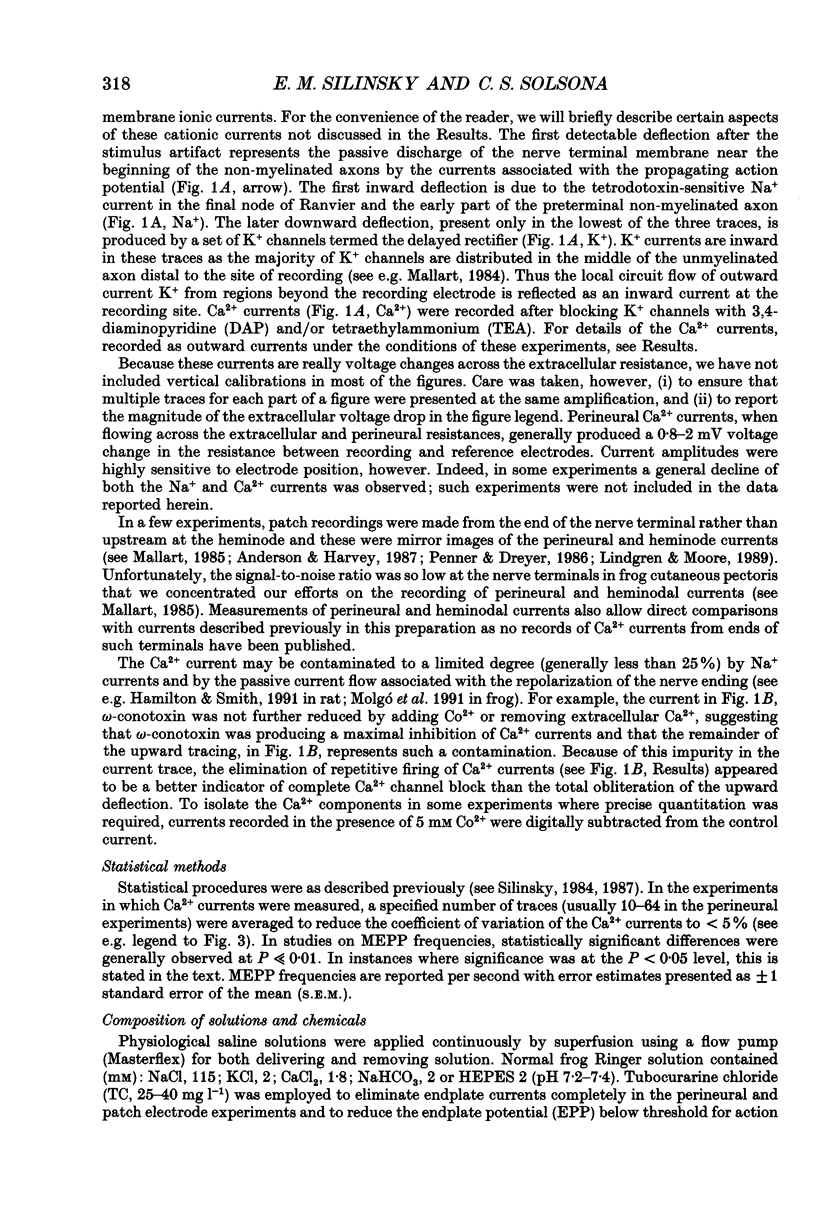
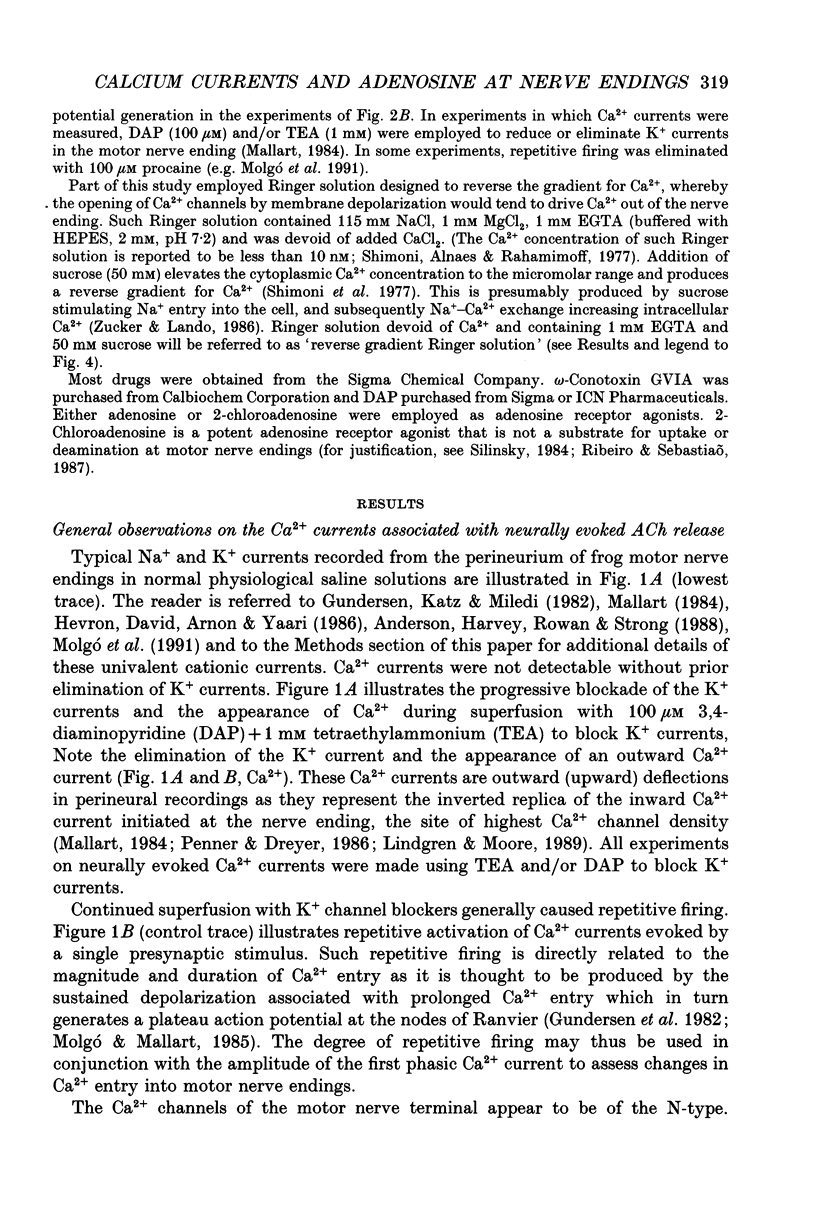
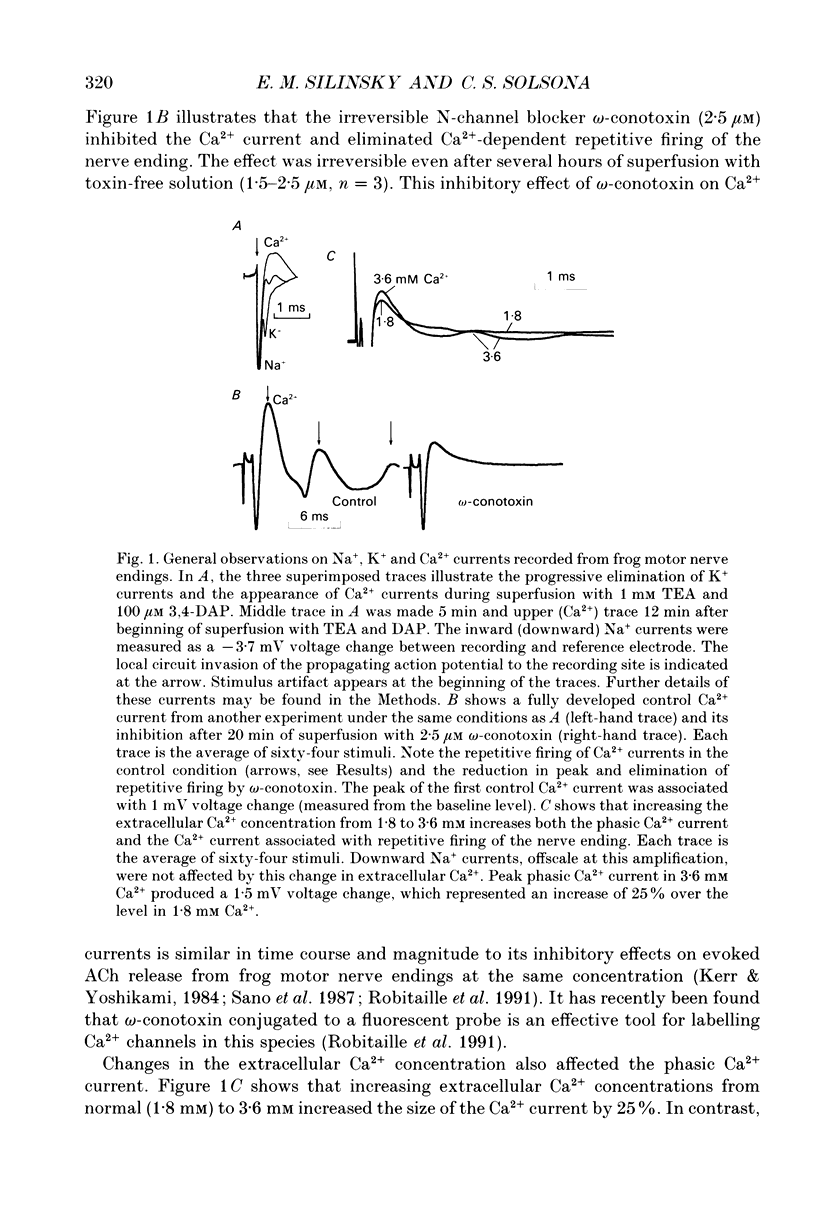
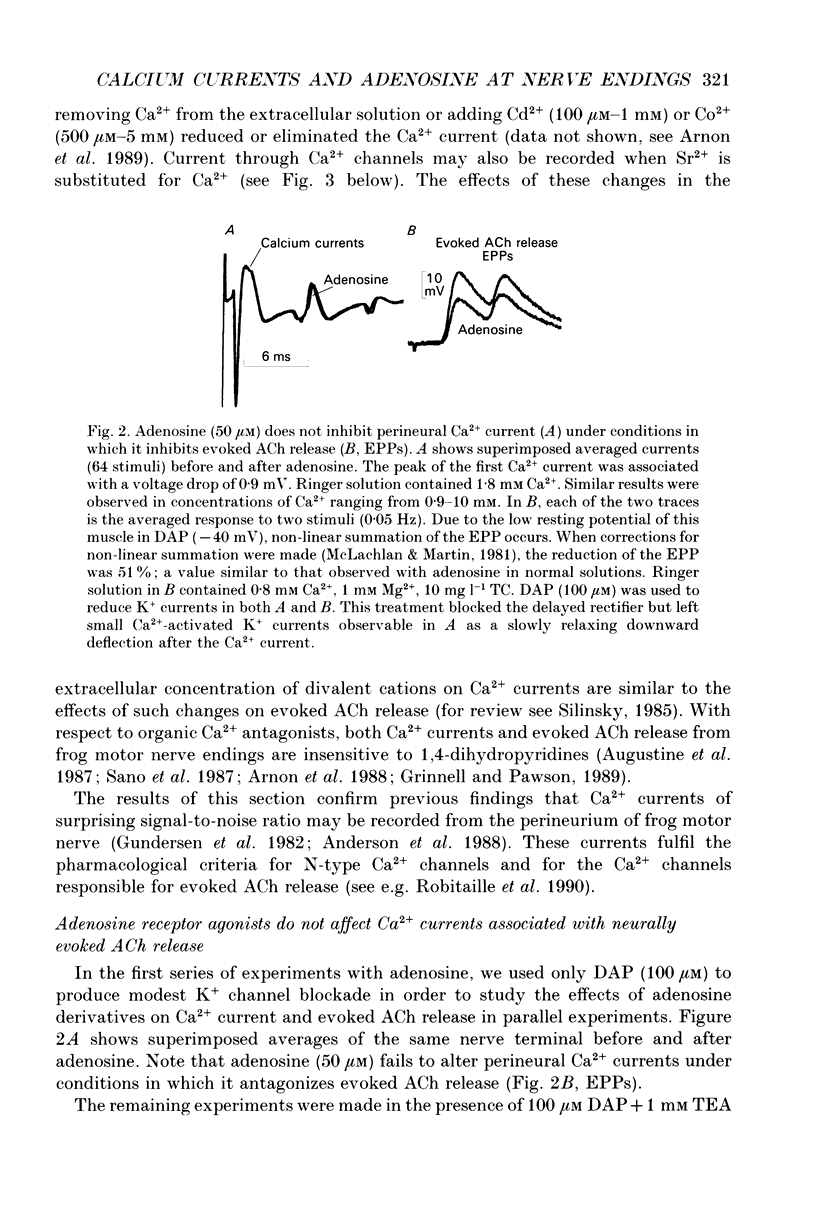
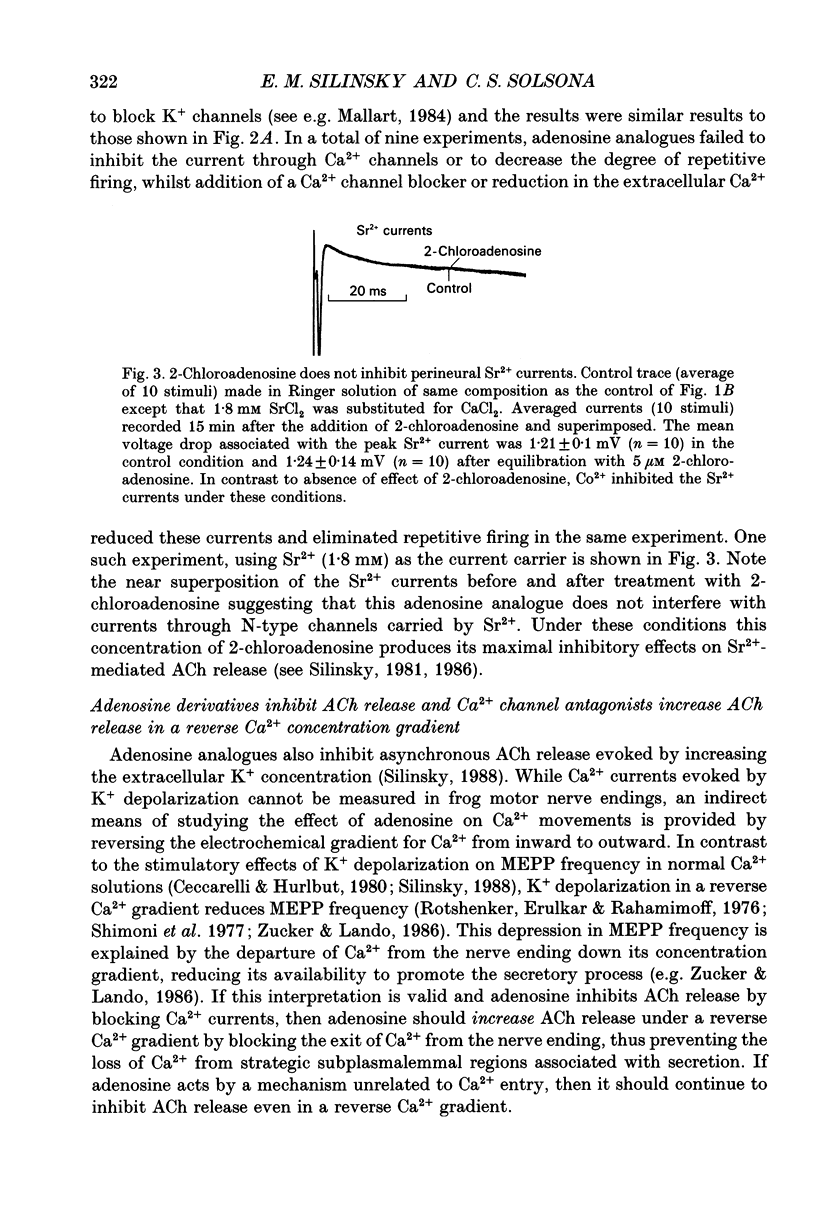
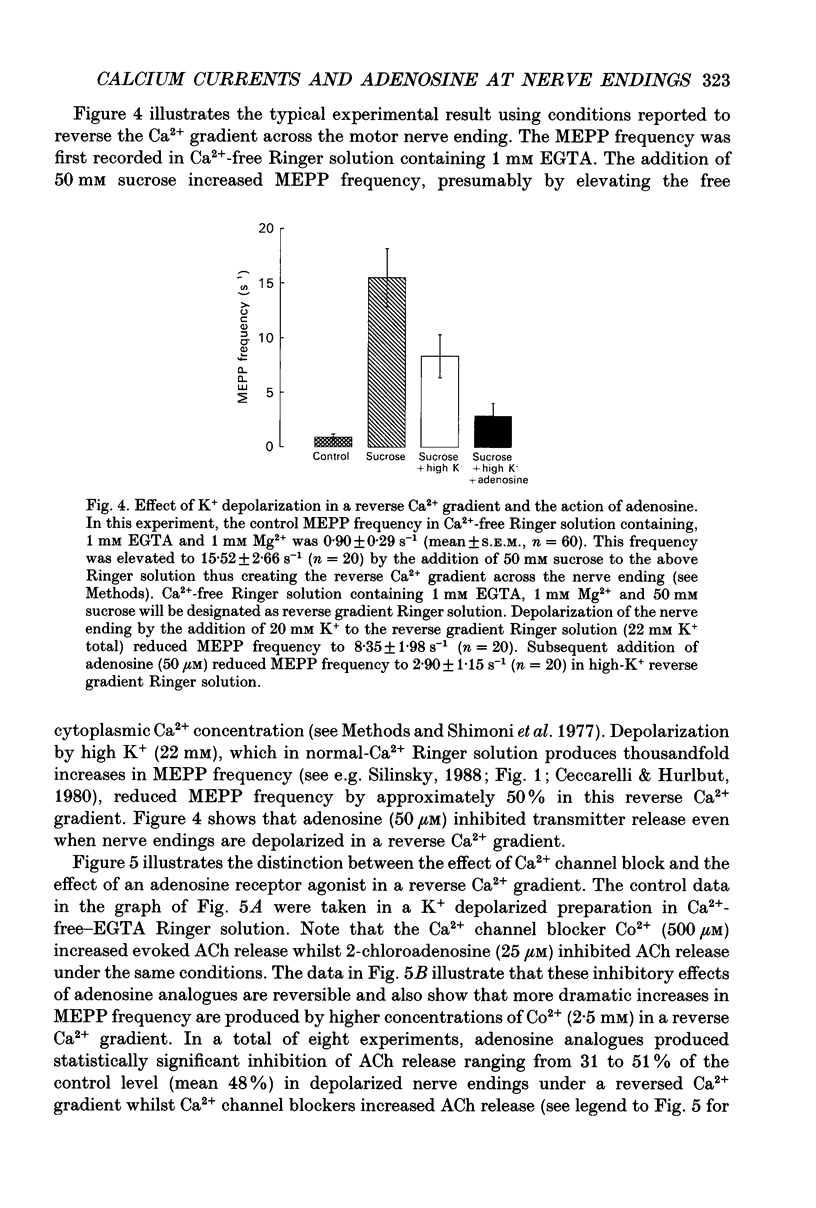
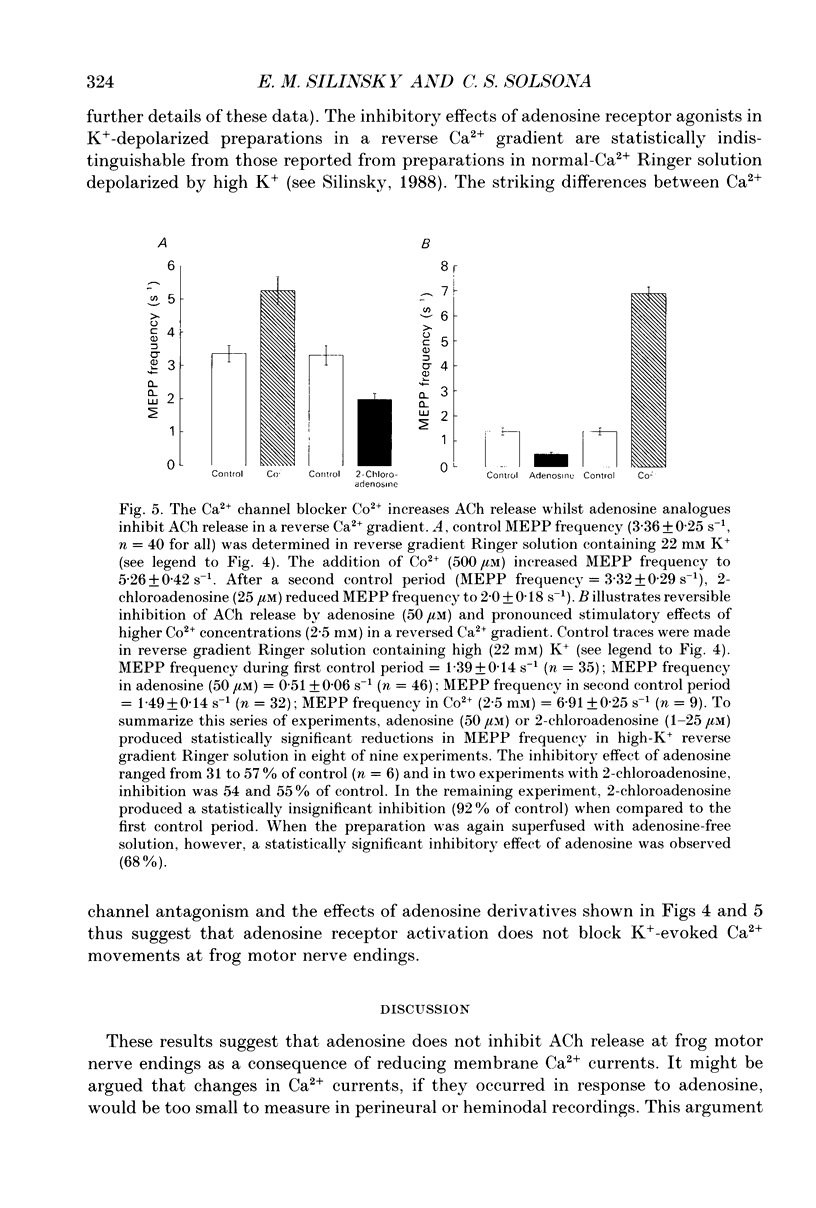
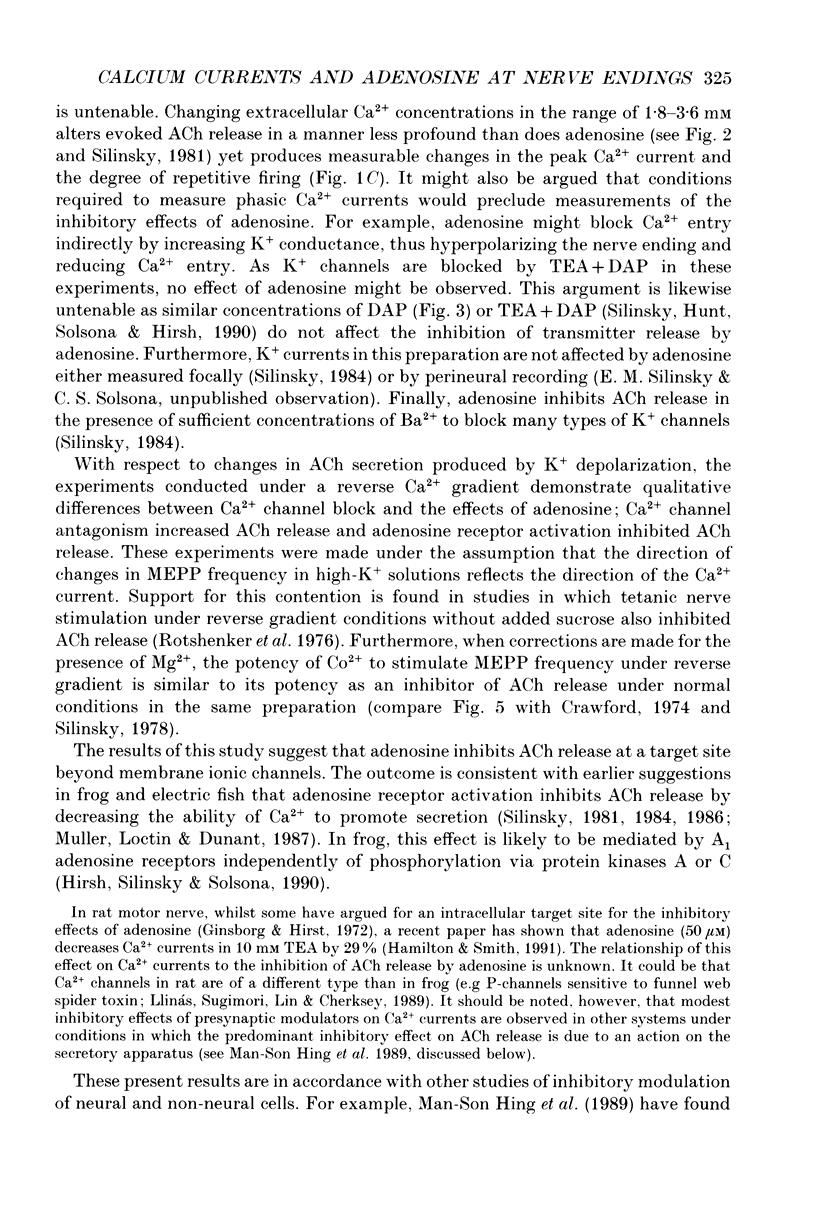
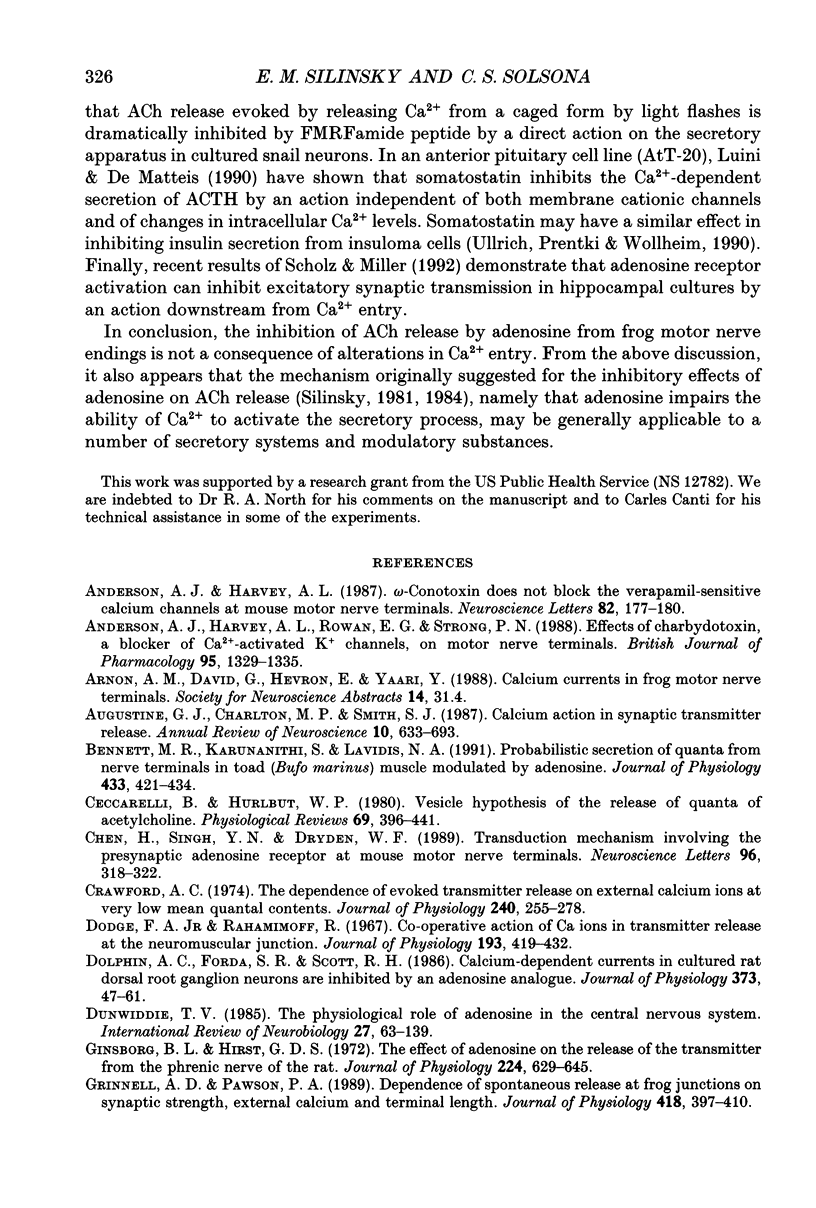
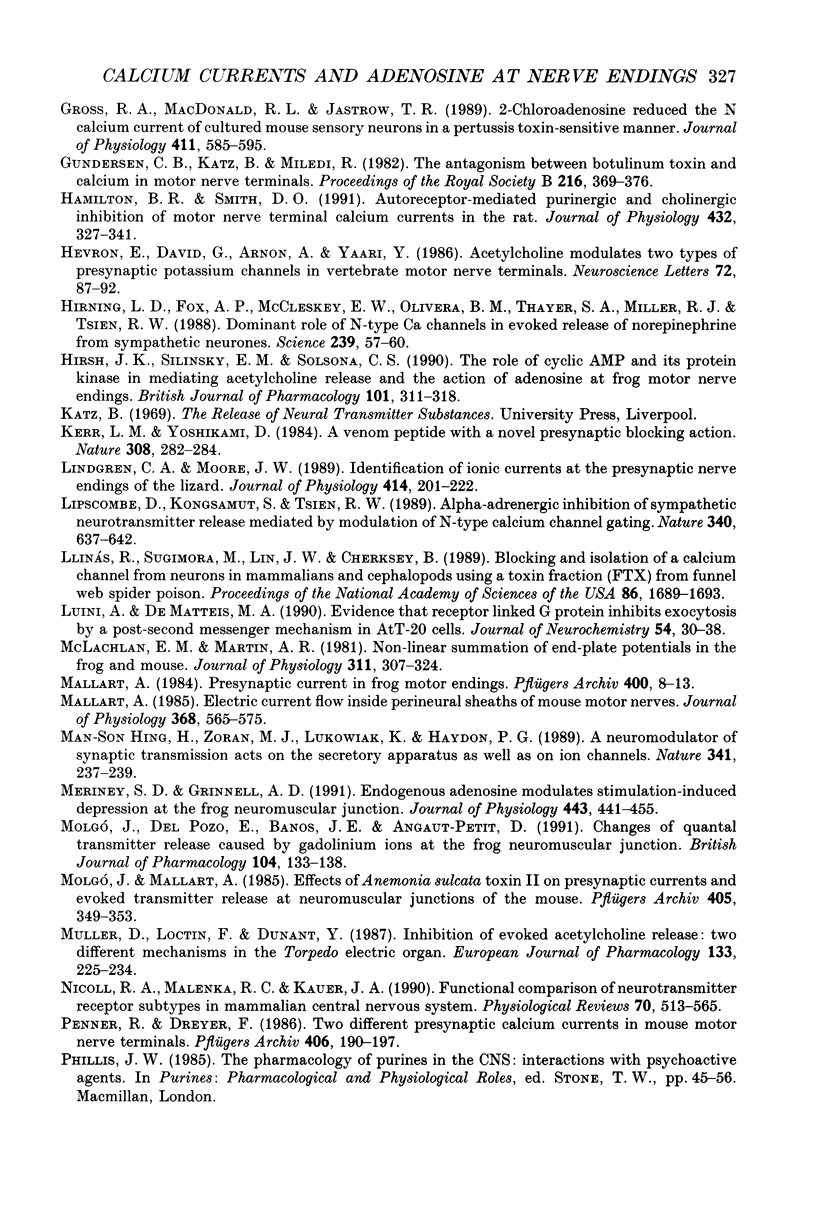
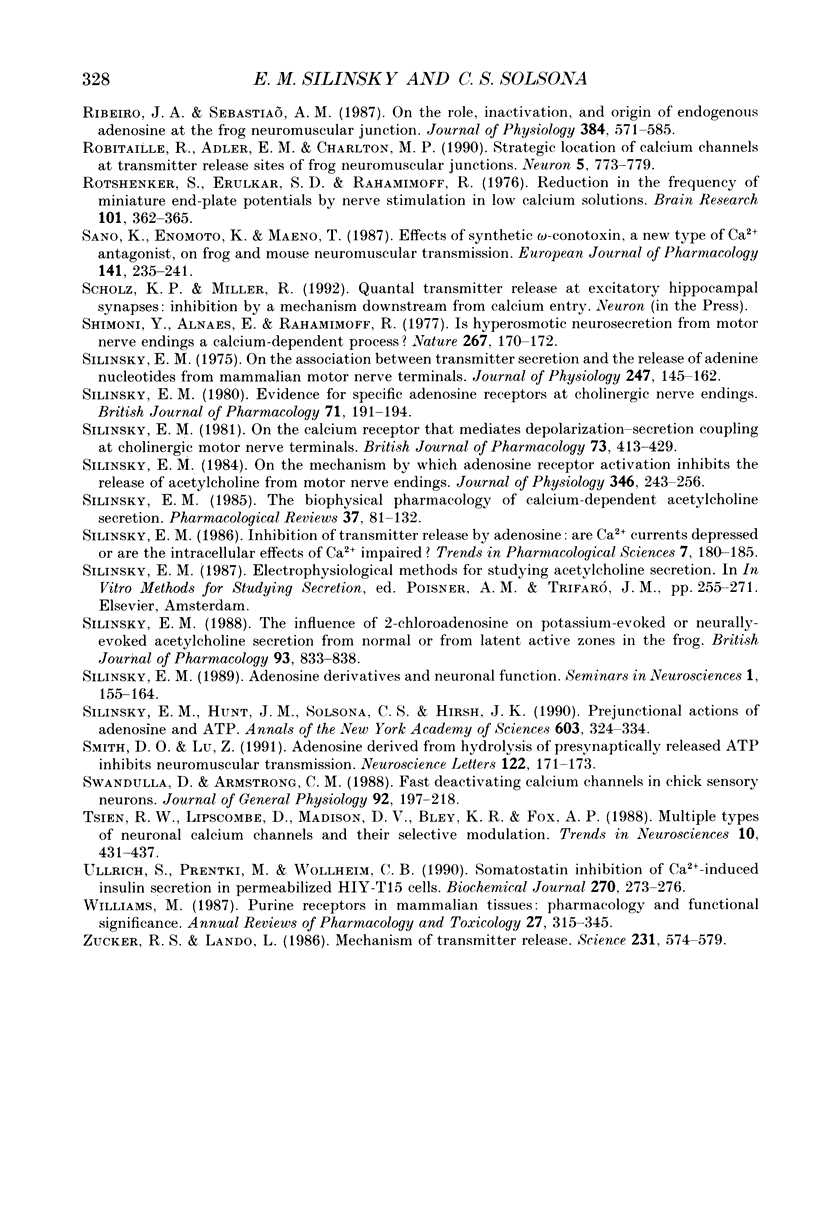
Selected References
These references are in PubMed. This may not be the complete list of references from this article.
- Anderson A. J., Harvey A. L. Omega-conotoxin does not block the verapamil-sensitive calcium channels at mouse motor nerve terminals. Neurosci Lett. 1987 Nov 23;82(2):177–180. doi: 10.1016/0304-3940(87)90125-x. [DOI] [PubMed] [Google Scholar]
- Anderson A. J., Harvey A. L., Rowan E. G., Strong P. N. Effects of charybdotoxin, a blocker of Ca2+-activated K+ channels, on motor nerve terminals. Br J Pharmacol. 1988 Dec;95(4):1329–1335. doi: 10.1111/j.1476-5381.1988.tb11772.x. [DOI] [PMC free article] [PubMed] [Google Scholar]
- Augustine G. J., Charlton M. P., Smith S. J. Calcium action in synaptic transmitter release. Annu Rev Neurosci. 1987;10:633–693. doi: 10.1146/annurev.ne.10.030187.003221. [DOI] [PubMed] [Google Scholar]
- Bennett M. R., Karunanithi S., Lavidis N. A. Probabilistic secretion of quanta from nerve terminals in toad (Bufo marinus) muscle modulated by adenosine. J Physiol. 1991 Feb;433:421–434. doi: 10.1113/jphysiol.1991.sp018435. [DOI] [PMC free article] [PubMed] [Google Scholar]
- Ceccarelli B., Hurlbut W. P. Vesicle hypothesis of the release of quanta of acetylcholine. Physiol Rev. 1980 Apr;60(2):396–441. doi: 10.1152/physrev.1980.60.2.396. [DOI] [PubMed] [Google Scholar]
- Chen H., Singh Y. N., Dryden W. F. Transduction mechanism involving the presynaptic adenosine receptor at mouse motor nerve terminals. Neurosci Lett. 1989 Jan 30;96(3):318–322. doi: 10.1016/0304-3940(89)90398-4. [DOI] [PubMed] [Google Scholar]
- Crawford A. C. The dependence of evoked transmitter release on external calcium ions at very low mean quantal contents. J Physiol. 1974 Jul;240(2):255–278. doi: 10.1113/jphysiol.1974.sp010609. [DOI] [PMC free article] [PubMed] [Google Scholar]
- Dodge F. A., Jr, Rahamimoff R. Co-operative action a calcium ions in transmitter release at the neuromuscular junction. J Physiol. 1967 Nov;193(2):419–432. doi: 10.1113/jphysiol.1967.sp008367. [DOI] [PMC free article] [PubMed] [Google Scholar]
- Dolphin A. C., Forda S. R., Scott R. H. Calcium-dependent currents in cultured rat dorsal root ganglion neurones are inhibited by an adenosine analogue. J Physiol. 1986 Apr;373:47–61. doi: 10.1113/jphysiol.1986.sp016034. [DOI] [PMC free article] [PubMed] [Google Scholar]
- Dunwiddie T. V. The physiological role of adenosine in the central nervous system. Int Rev Neurobiol. 1985;27:63–139. doi: 10.1016/s0074-7742(08)60556-5. [DOI] [PubMed] [Google Scholar]
- Ginsborg B. L., Hirst G. D. The effect of adenosine on the release of the transmitter from the phrenic nerve of the rat. J Physiol. 1972 Aug;224(3):629–645. doi: 10.1113/jphysiol.1972.sp009916. [DOI] [PMC free article] [PubMed] [Google Scholar]
- Grinnell A. D., Pawson P. A. Dependence of spontaneous release at frog junctions on synaptic strength, external calcium and terminal length. J Physiol. 1989 Nov;418:397–410. doi: 10.1113/jphysiol.1989.sp017848. [DOI] [PMC free article] [PubMed] [Google Scholar]
- Gross R. A., Macdonald R. L., Ryan-Jastrow T. 2-Chloroadenosine reduces the N calcium current of cultured mouse sensory neurones in a pertussis toxin-sensitive manner. J Physiol. 1989 Apr;411:585–595. doi: 10.1113/jphysiol.1989.sp017592. [DOI] [PMC free article] [PubMed] [Google Scholar]
- Gundersen C. B., Katz B., Miledi R. The antagonism between botulinum toxin and calcium in motor nerve terminals. Proc R Soc Lond B Biol Sci. 1982 Oct 22;216(1204):369–376. doi: 10.1098/rspb.1982.0080. [DOI] [PubMed] [Google Scholar]
- Hamilton B. R., Smith D. O. Autoreceptor-mediated purinergic and cholinergic inhibition of motor nerve terminal calcium currents in the rat. J Physiol. 1991 Jan;432:327–341. doi: 10.1113/jphysiol.1991.sp018387. [DOI] [PMC free article] [PubMed] [Google Scholar]
- Hevron E., David G., Arnon A., Yaari Y. Acetylcholine modulates two types of presynaptic potassium channels in vertebrate motor nerve terminals. Neurosci Lett. 1986 Dec 3;72(1):87–92. doi: 10.1016/0304-3940(86)90624-5. [DOI] [PubMed] [Google Scholar]
- Hirning L. D., Fox A. P., McCleskey E. W., Olivera B. M., Thayer S. A., Miller R. J., Tsien R. W. Dominant role of N-type Ca2+ channels in evoked release of norepinephrine from sympathetic neurons. Science. 1988 Jan 1;239(4835):57–61. doi: 10.1126/science.2447647. [DOI] [PubMed] [Google Scholar]
- Hirsh J. K., Silinsky E. M., Solsona C. S. The role of cyclic AMP and its protein kinase in mediating acetylcholine release and the action of adenosine at frog motor nerve endings. Br J Pharmacol. 1990 Oct;101(2):311–318. doi: 10.1111/j.1476-5381.1990.tb12707.x. [DOI] [PMC free article] [PubMed] [Google Scholar]
- Kerr L. M., Yoshikami D. A venom peptide with a novel presynaptic blocking action. Nature. 1984 Mar 15;308(5956):282–284. doi: 10.1038/308282a0. [DOI] [PubMed] [Google Scholar]
- Lindgren C. A., Moore J. W. Identification of ionic currents at presynaptic nerve endings of the lizard. J Physiol. 1989 Jul;414:201–222. doi: 10.1113/jphysiol.1989.sp017684. [DOI] [PMC free article] [PubMed] [Google Scholar]
- Lipscombe D., Kongsamut S., Tsien R. W. Alpha-adrenergic inhibition of sympathetic neurotransmitter release mediated by modulation of N-type calcium-channel gating. Nature. 1989 Aug 24;340(6235):639–642. doi: 10.1038/340639a0. [DOI] [PubMed] [Google Scholar]
- Llinás R., Sugimori M., Lin J. W., Cherksey B. Blocking and isolation of a calcium channel from neurons in mammals and cephalopods utilizing a toxin fraction (FTX) from funnel-web spider poison. Proc Natl Acad Sci U S A. 1989 Mar;86(5):1689–1693. doi: 10.1073/pnas.86.5.1689. [DOI] [PMC free article] [PubMed] [Google Scholar]
- Luini A., De Matteis M. A. Evidence that receptor-linked G protein inhibits exocytosis by a post-second-messenger mechanism in AtT-20 cells. J Neurochem. 1990 Jan;54(1):30–38. doi: 10.1111/j.1471-4159.1990.tb13279.x. [DOI] [PubMed] [Google Scholar]
- Mallart A. Electric current flow inside perineurial sheaths of mouse motor nerves. J Physiol. 1985 Nov;368:565–575. doi: 10.1113/jphysiol.1985.sp015876. [DOI] [PMC free article] [PubMed] [Google Scholar]
- Mallart A. Presynaptic currents in frog motor endings. Pflugers Arch. 1984 Jan;400(1):8–13. doi: 10.1007/BF00670529. [DOI] [PubMed] [Google Scholar]
- Man-Son-Hing H., Zoran M. J., Lukowiak K., Haydon P. G. A neuromodulator of synaptic transmission acts on the secretory apparatus as well as on ion channels. Nature. 1989 Sep 21;341(6239):237–239. doi: 10.1038/341237a0. [DOI] [PubMed] [Google Scholar]
- McLachlan E. M., Martin A. R. Non-linear summation of end-plate potentials in the frog and mouse. J Physiol. 1981 Feb;311:307–324. doi: 10.1113/jphysiol.1981.sp013586. [DOI] [PMC free article] [PubMed] [Google Scholar]
- Meriney S. D., Grinnell A. D. Endogenous adenosine modulates stimulation-induced depression at the frog neuromuscular junction. J Physiol. 1991 Nov;443:441–455. doi: 10.1113/jphysiol.1991.sp018843. [DOI] [PMC free article] [PubMed] [Google Scholar]
- Molgó J., Mallart A. Effects of Anemonia sulcata toxin II on presynaptic currents and evoked transmitter release at neuromuscular junctions of the mouse. Pflugers Arch. 1985 Dec;405(4):349–353. doi: 10.1007/BF00595687. [DOI] [PubMed] [Google Scholar]
- Molgó J., del Pozo E., Baños J. E., Angaut-Petit D. Changes of quantal transmitter release caused by gadolinium ions at the frog neuromuscular junction. Br J Pharmacol. 1991 Sep;104(1):133–138. doi: 10.1111/j.1476-5381.1991.tb12397.x. [DOI] [PMC free article] [PubMed] [Google Scholar]
- Muller D., Loctin F., Dunant Y. Inhibition of evoked acetylcholine release: two different mechanisms in the Torpedo electric organ. Eur J Pharmacol. 1987 Jan 13;133(2):225–234. doi: 10.1016/0014-2999(87)90154-3. [DOI] [PubMed] [Google Scholar]
- Nicoll R. A., Malenka R. C., Kauer J. A. Functional comparison of neurotransmitter receptor subtypes in mammalian central nervous system. Physiol Rev. 1990 Apr;70(2):513–565. doi: 10.1152/physrev.1990.70.2.513. [DOI] [PubMed] [Google Scholar]
- Penner R., Dreyer F. Two different presynaptic calcium currents in mouse motor nerve terminals. Pflugers Arch. 1986 Feb;406(2):190–197. doi: 10.1007/BF00586682. [DOI] [PubMed] [Google Scholar]
- Ribeiro J. A., Sebastião A. M. On the role, inactivation and origin of endogenous adenosine at the frog neuromuscular junction. J Physiol. 1987 Mar;384:571–585. doi: 10.1113/jphysiol.1987.sp016470. [DOI] [PMC free article] [PubMed] [Google Scholar]
- Robitaille R., Adler E. M., Charlton M. P. Strategic location of calcium channels at transmitter release sites of frog neuromuscular synapses. Neuron. 1990 Dec;5(6):773–779. doi: 10.1016/0896-6273(90)90336-e. [DOI] [PubMed] [Google Scholar]
- Rotshenker S., Erulkar S. D., Rahamimoff R. Reduction in the frequency of miniature end-plate potentials by nerve stimulation in low calcium solutions. Brain Res. 1976 Jan 16;101(2):362–365. doi: 10.1016/0006-8993(76)90277-8. [DOI] [PubMed] [Google Scholar]
- Sano K., Enomoto K., Maeno T. Effects of synthetic omega-conotoxin, a new type Ca2+ antagonist, on frog and mouse neuromuscular transmission. Eur J Pharmacol. 1987 Sep 11;141(2):235–241. doi: 10.1016/0014-2999(87)90268-8. [DOI] [PubMed] [Google Scholar]
- Shimoni Y., Alnaes E., Rahamimoff R. Is hyperosmotic neurosecretion from motor nerve endings a calcium-dependent process? Nature. 1977 May 12;267(5607):170–172. doi: 10.1038/267170a0. [DOI] [PubMed] [Google Scholar]
- Silinsky E. M. Evidence for specific adenosine receptors at cholinergic nerve endings. Br J Pharmacol. 1980;71(1):191–194. doi: 10.1111/j.1476-5381.1980.tb10925.x. [DOI] [PMC free article] [PubMed] [Google Scholar]
- Silinsky E. M., Hunt J. M., Solsona C. S., Hirsh J. K. Prejunctional adenosine and ATP receptors. Ann N Y Acad Sci. 1990;603:324–334. doi: 10.1111/j.1749-6632.1990.tb37683.x. [DOI] [PubMed] [Google Scholar]
- Silinsky E. M. On the association between transmitter secretion and the release of adenine nucleotides from mammalian motor nerve terminals. J Physiol. 1975 May;247(1):145–162. doi: 10.1113/jphysiol.1975.sp010925. [DOI] [PMC free article] [PubMed] [Google Scholar]
- Silinsky E. M. On the calcium receptor that mediates depolarization-secretion coupling at cholinergic motor nerve terminals. Br J Pharmacol. 1981 Jun;73(2):413–429. doi: 10.1111/j.1476-5381.1981.tb10438.x. [DOI] [PMC free article] [PubMed] [Google Scholar]
- Silinsky E. M. On the mechanism by which adenosine receptor activation inhibits the release of acetylcholine from motor nerve endings. J Physiol. 1984 Jan;346:243–256. doi: 10.1113/jphysiol.1984.sp015019. [DOI] [PMC free article] [PubMed] [Google Scholar]
- Silinsky E. M. The biophysical pharmacology of calcium-dependent acetylcholine secretion. Pharmacol Rev. 1985 Mar;37(1):81–132. [PubMed] [Google Scholar]
- Silinsky E. M. The influence of 2-chloroadenosine on potassium-evoked and neurally-evoked acetylcholine secretion from normal or from latent active zones in the frog. Br J Pharmacol. 1988 Apr;93(4):833–838. doi: 10.1111/j.1476-5381.1988.tb11469.x. [DOI] [PMC free article] [PubMed] [Google Scholar]
- Smith D. O., Lu Z. Adenosine derived from hydrolysis of presynaptically released ATP inhibits neuromuscular transmission in the rat. Neurosci Lett. 1991 Jan 28;122(2):171–173. doi: 10.1016/0304-3940(91)90850-s. [DOI] [PubMed] [Google Scholar]
- Swandulla D., Armstrong C. M. Fast-deactivating calcium channels in chick sensory neurons. J Gen Physiol. 1988 Aug;92(2):197–218. doi: 10.1085/jgp.92.2.197. [DOI] [PMC free article] [PubMed] [Google Scholar]
- Tsien R. W., Lipscombe D., Madison D. V., Bley K. R., Fox A. P. Multiple types of neuronal calcium channels and their selective modulation. Trends Neurosci. 1988 Oct;11(10):431–438. doi: 10.1016/0166-2236(88)90194-4. [DOI] [PubMed] [Google Scholar]
- Ullrich S., Prentki M., Wollheim C. B. Somatostatin inhibition of Ca2(+)-induced insulin secretion in permeabilized HIT-T15 cells. Biochem J. 1990 Aug 15;270(1):273–276. doi: 10.1042/bj2700273. [DOI] [PMC free article] [PubMed] [Google Scholar]
- Williams M. Purine receptors in mammalian tissues: pharmacology and functional significance. Annu Rev Pharmacol Toxicol. 1987;27:315–345. doi: 10.1146/annurev.pa.27.040187.001531. [DOI] [PubMed] [Google Scholar]
- Zucker R. S., Landò L. Mechanism of transmitter release: voltage hypothesis and calcium hypothesis. Science. 1986 Feb 7;231(4738):574–579. doi: 10.1126/science.2868525. [DOI] [PubMed] [Google Scholar]