Abstract
1. Ca2+ inward currents were measured by voltage clamping cut skeletal muscle fibres of the frog (Rana esculenta) in a double-Vaseline-gap system. 2. In order to study the basis of the previously described fast gating mode induced in the Ca2+ inward current by a conditioning depolarization we quantitatively analysed the response to differing features of the conditioning prepulse. 3. The faster activation seen during the second of two depolarizations was confined to the component of the inward current which could be blocked by 5 to 10 microM nifedipine. 4. By applying depolarizing conditioning pulses of gradually increasing length the time course of the transition to the fast gating mode could be determined. 5. Both the transition to the fast gating mode (point 4) caused by a depolarization and the slow inward current activated during the same depolarization showed similar voltage-dependent kinetics. 6. The kinetic change of the test current appeared to be equal when the same fractional activation was achieved at the end of the conditioning pulse independent of its duration or amplitude. 7. Flash photolysis of nifedipine in the interval between conditioning and test pulse showed that the predepolarization causes a rate-enhancing effect even though the slow channels were blocked by nifedipine during the conditioning pulse. 8. We conclude that the transition of the calcium channel from its slow to its fast gating mode is determined by the slow voltage-dependent reaction which limits the rate of channel opening under control conditions. This reaction is apparently not prevented by the binding of nifedipine and the block of current flow through the channel.
Full text
PDF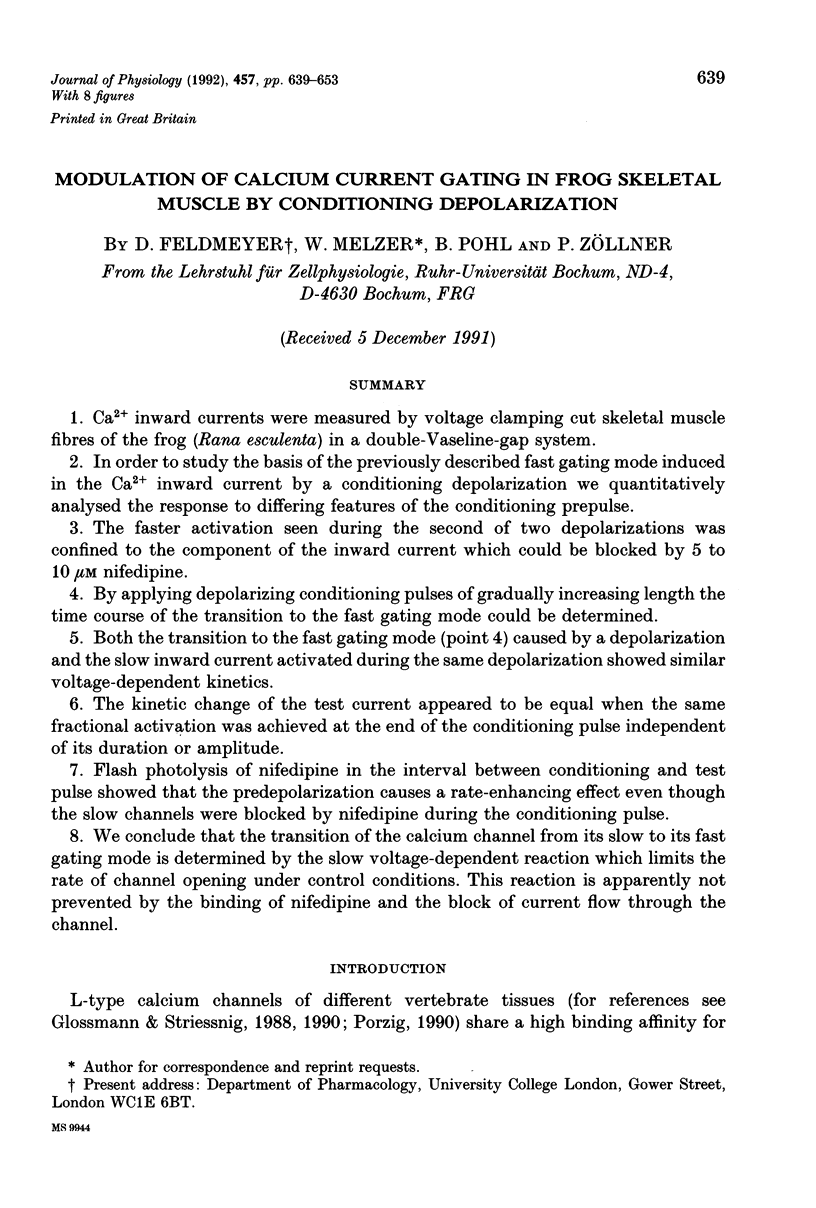
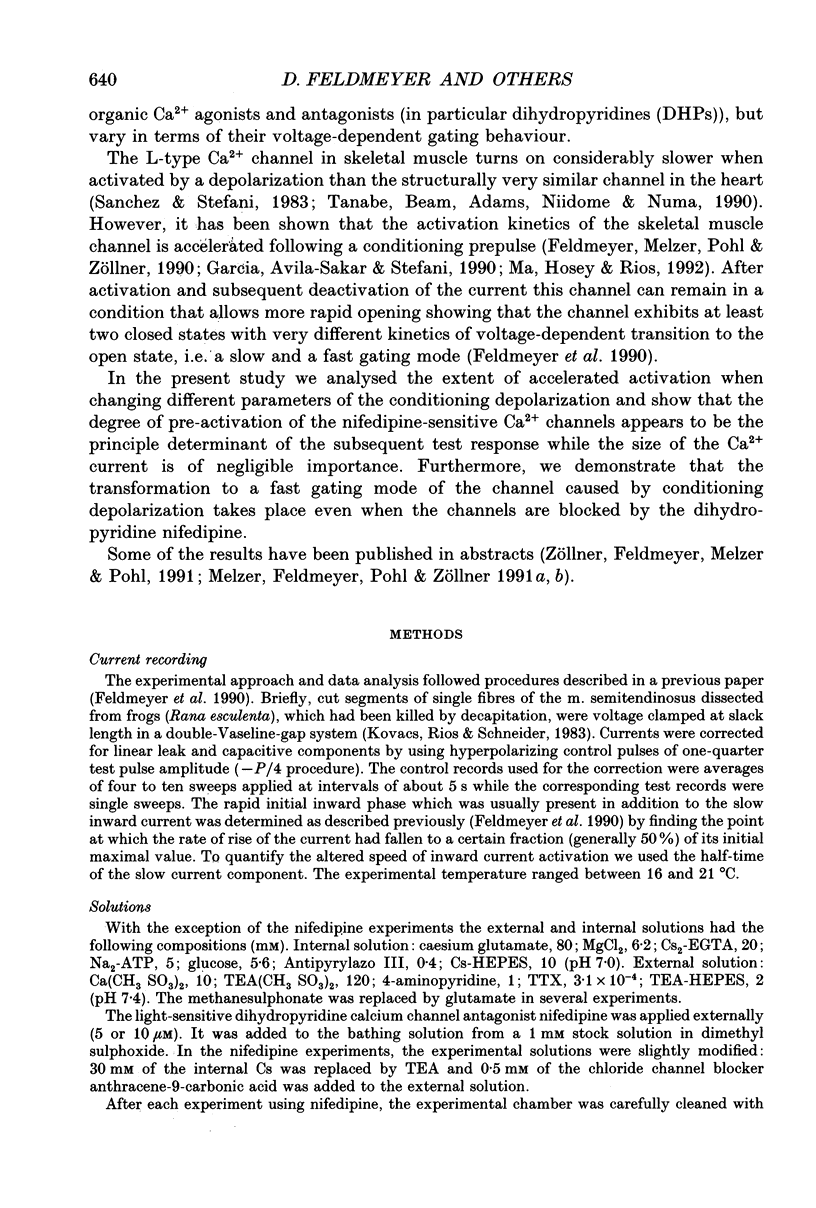
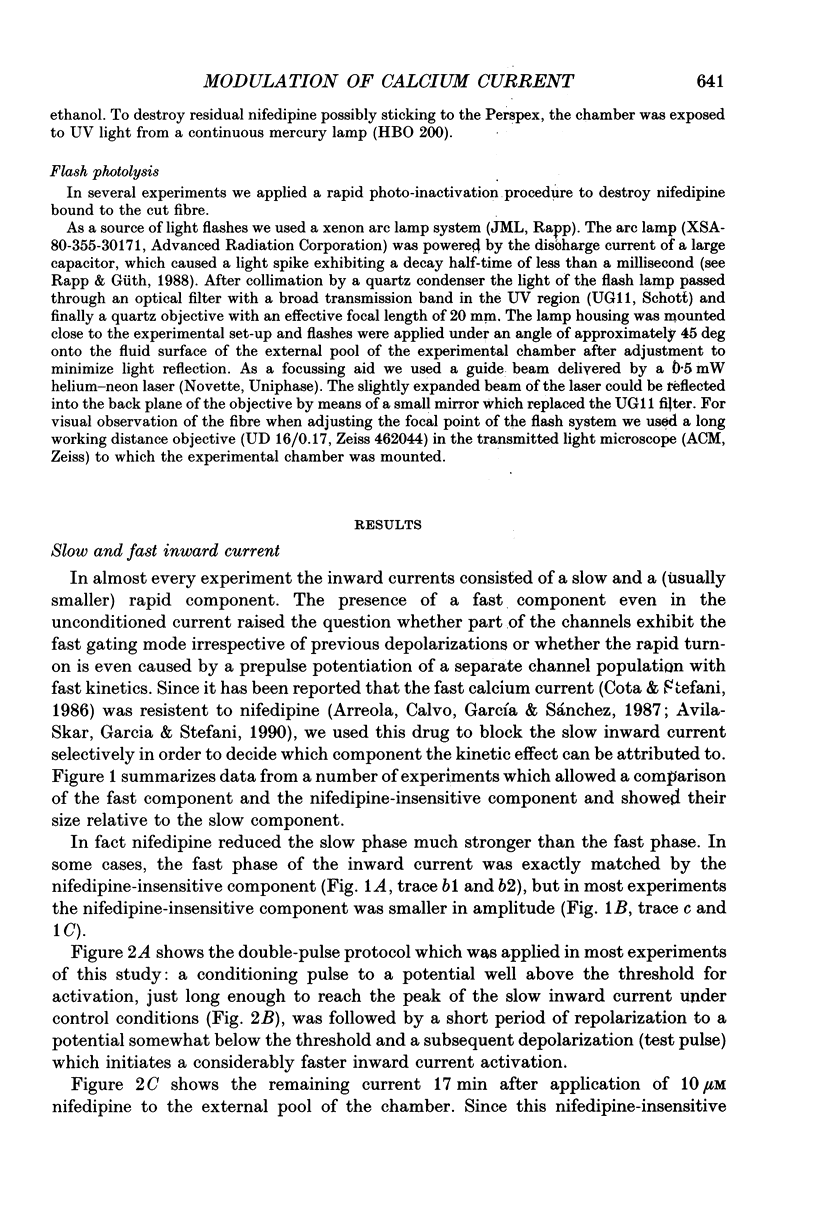
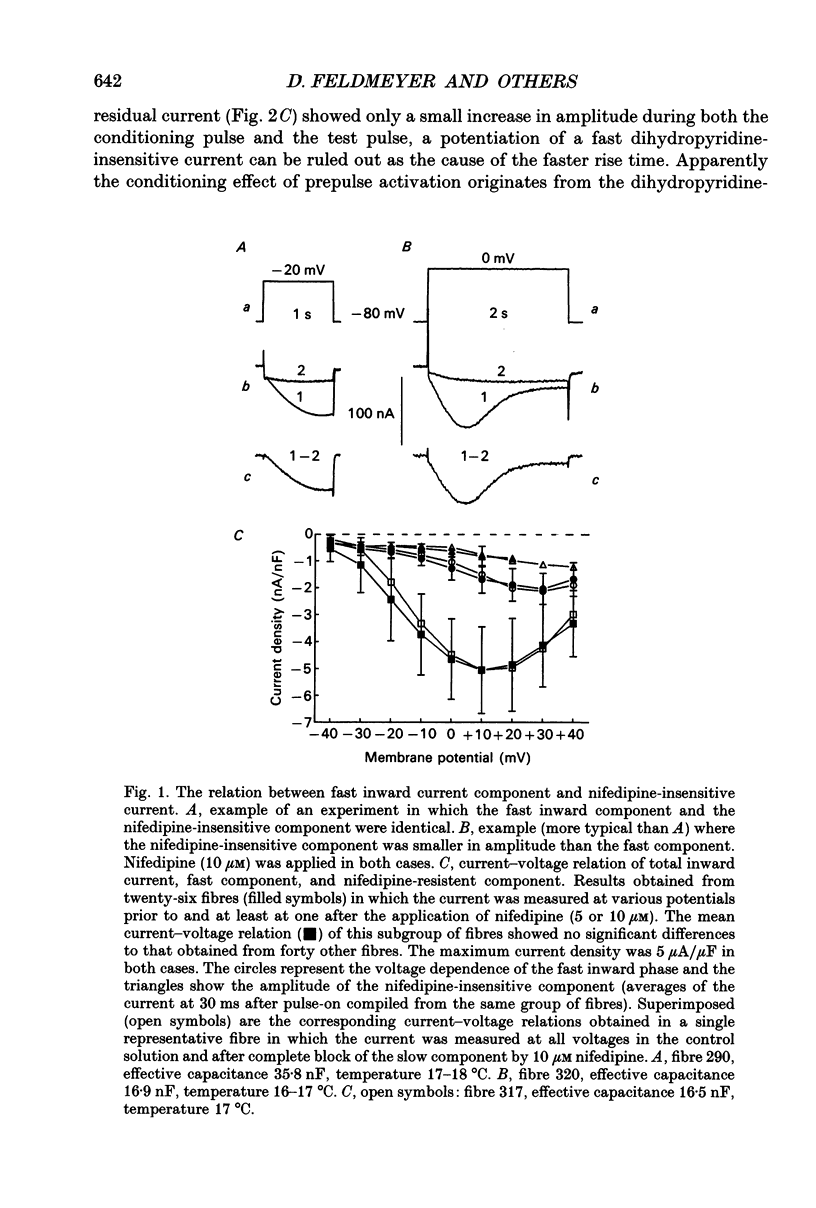
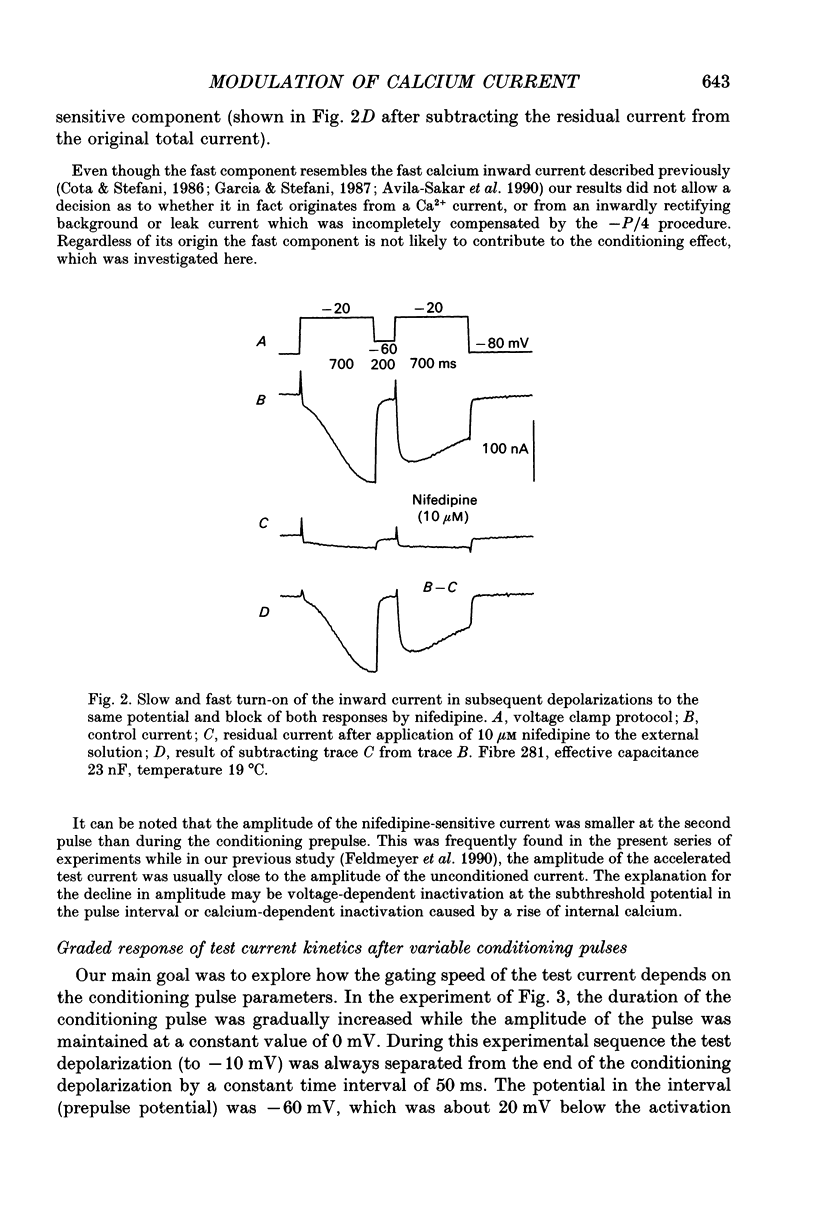
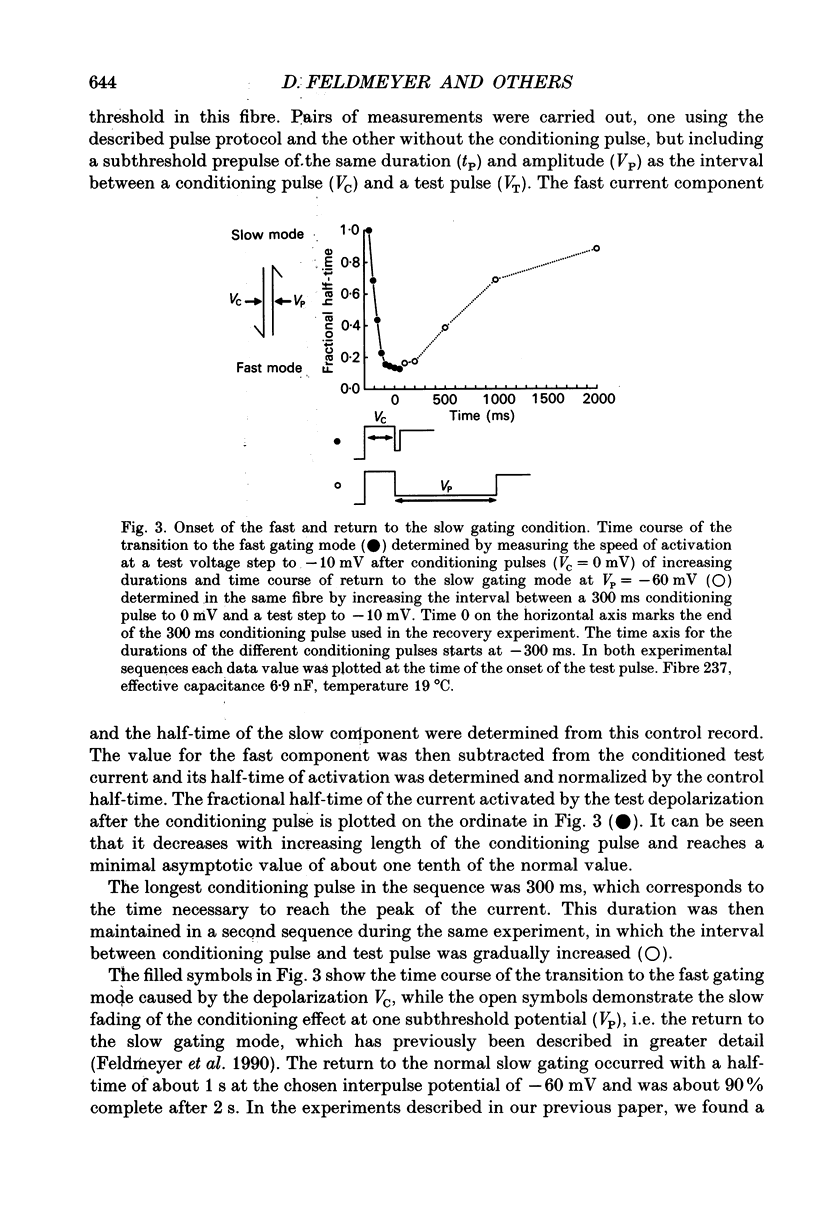
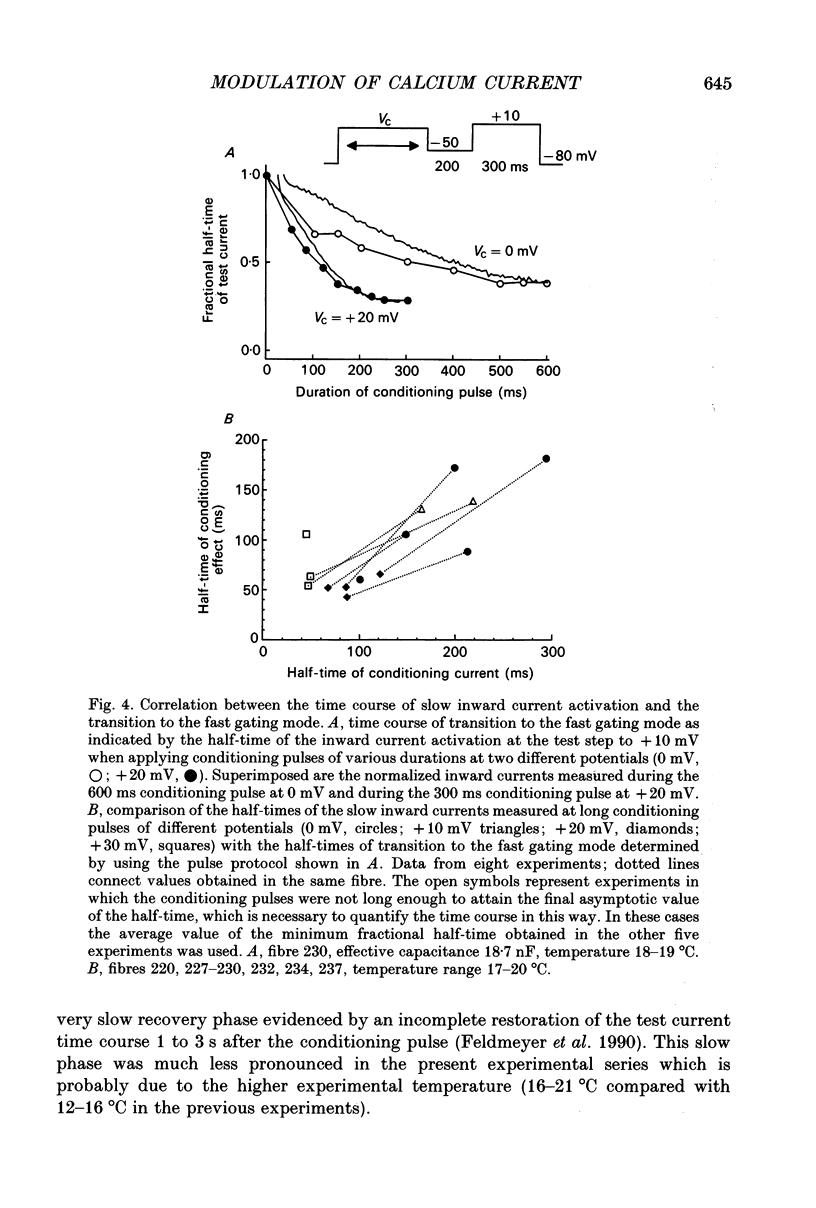
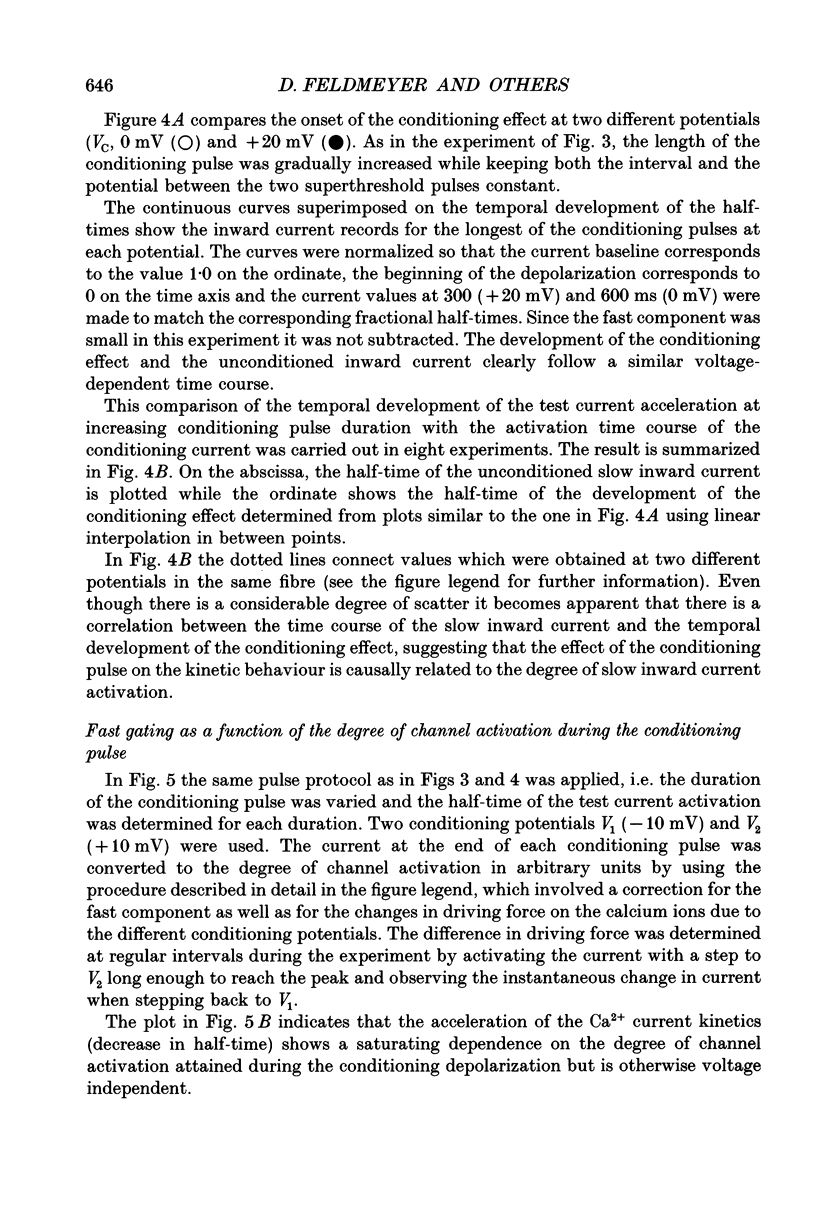
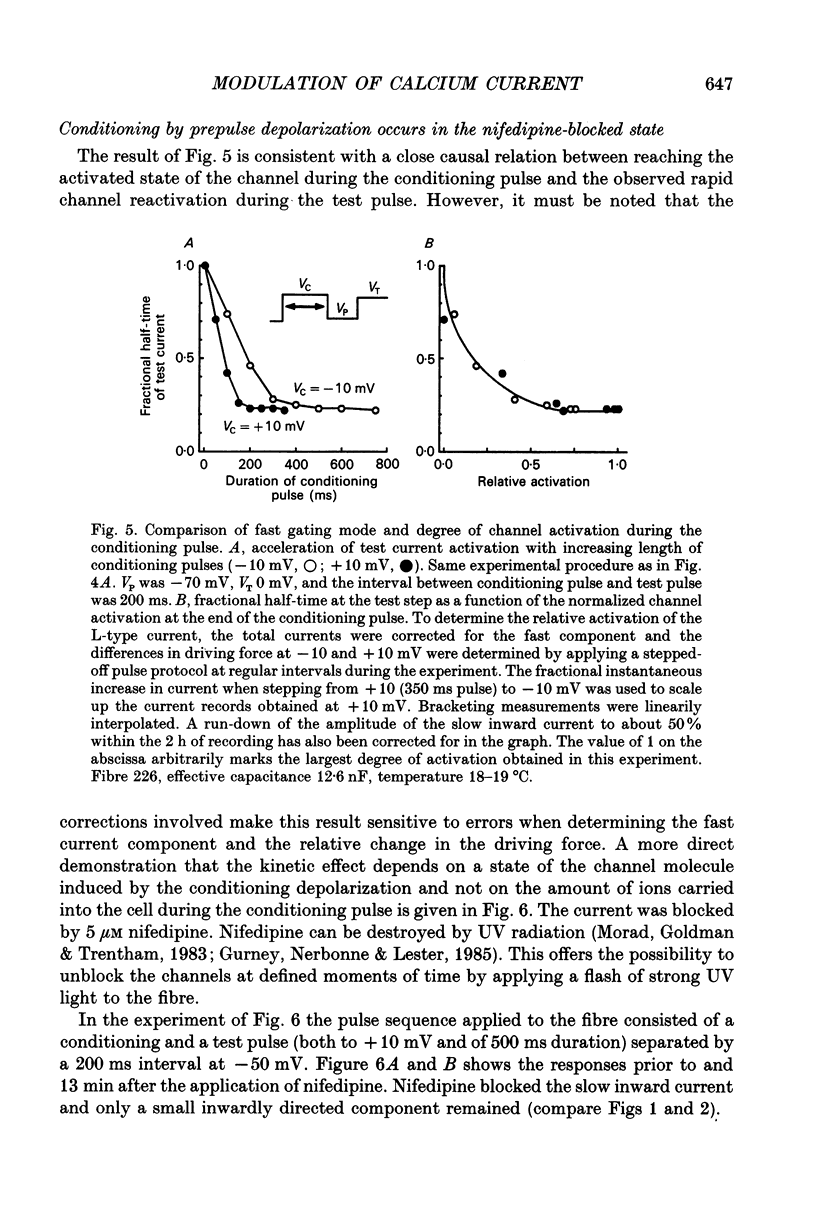
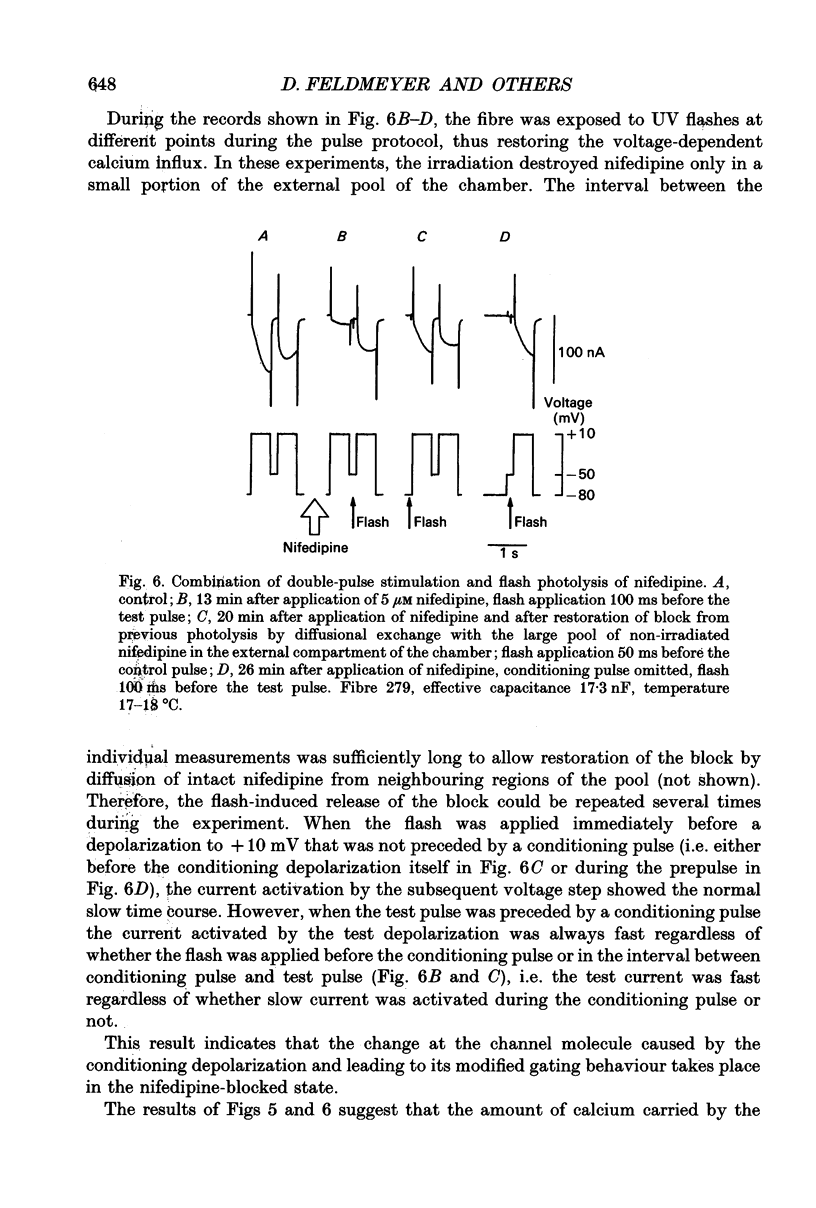
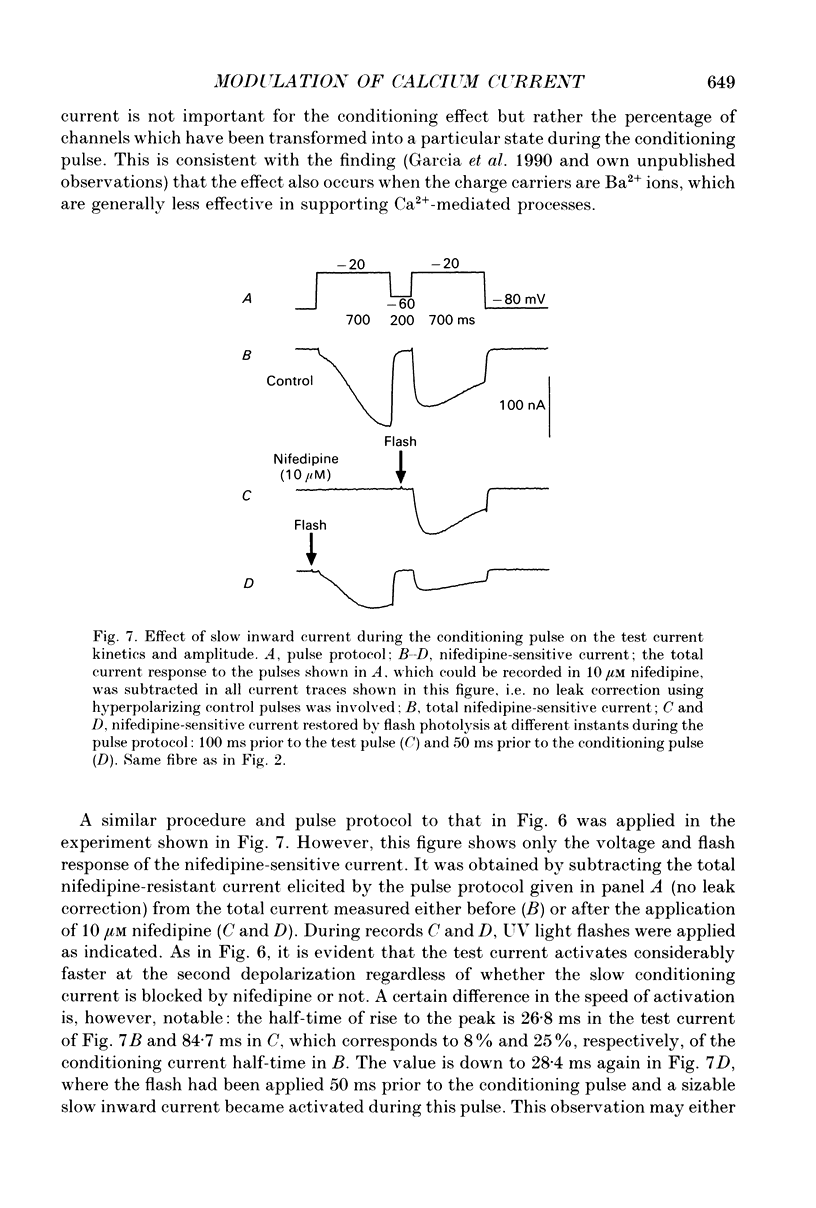
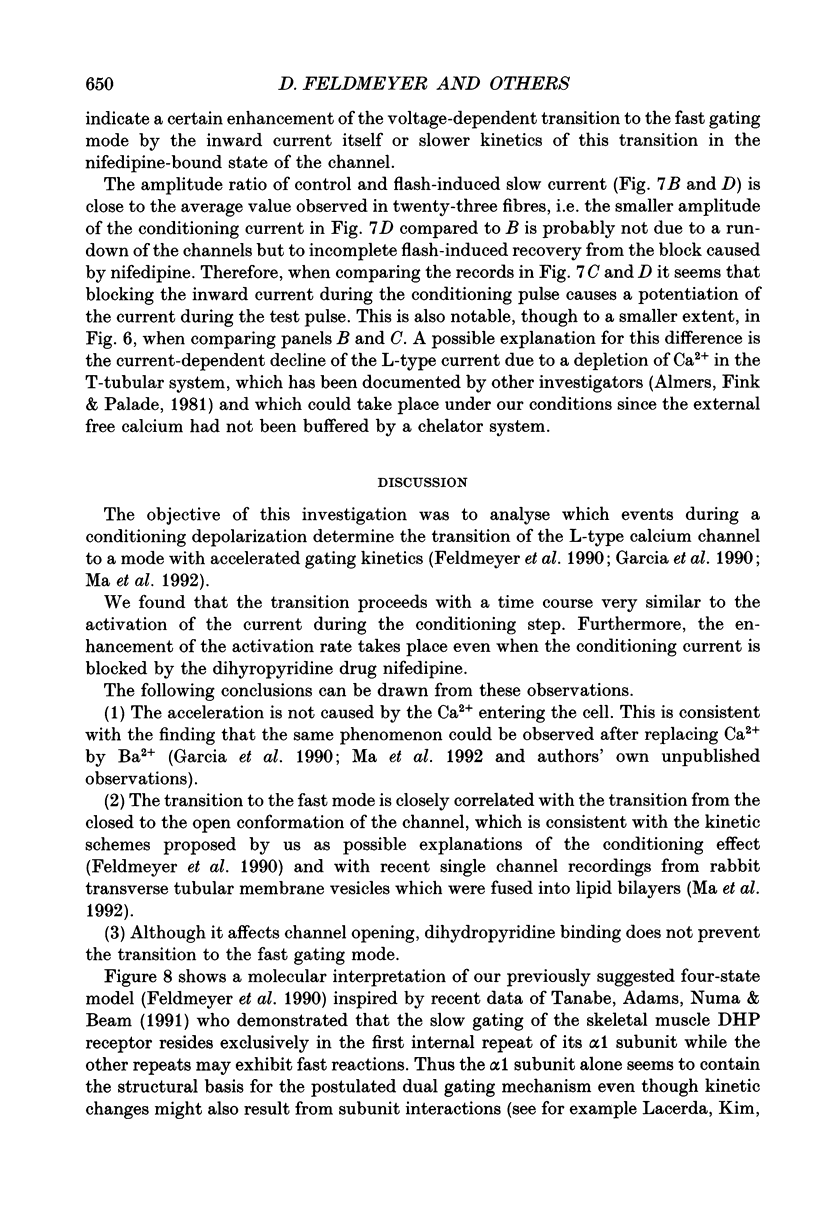
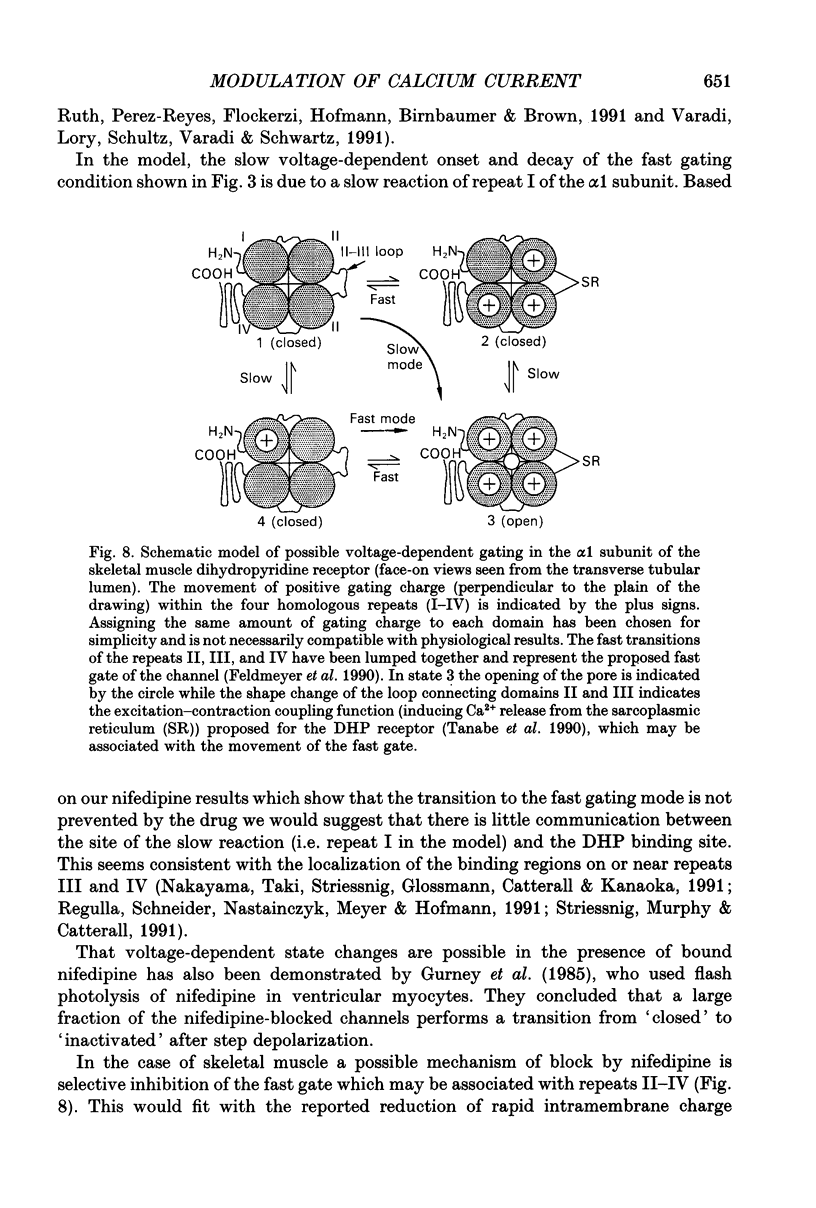
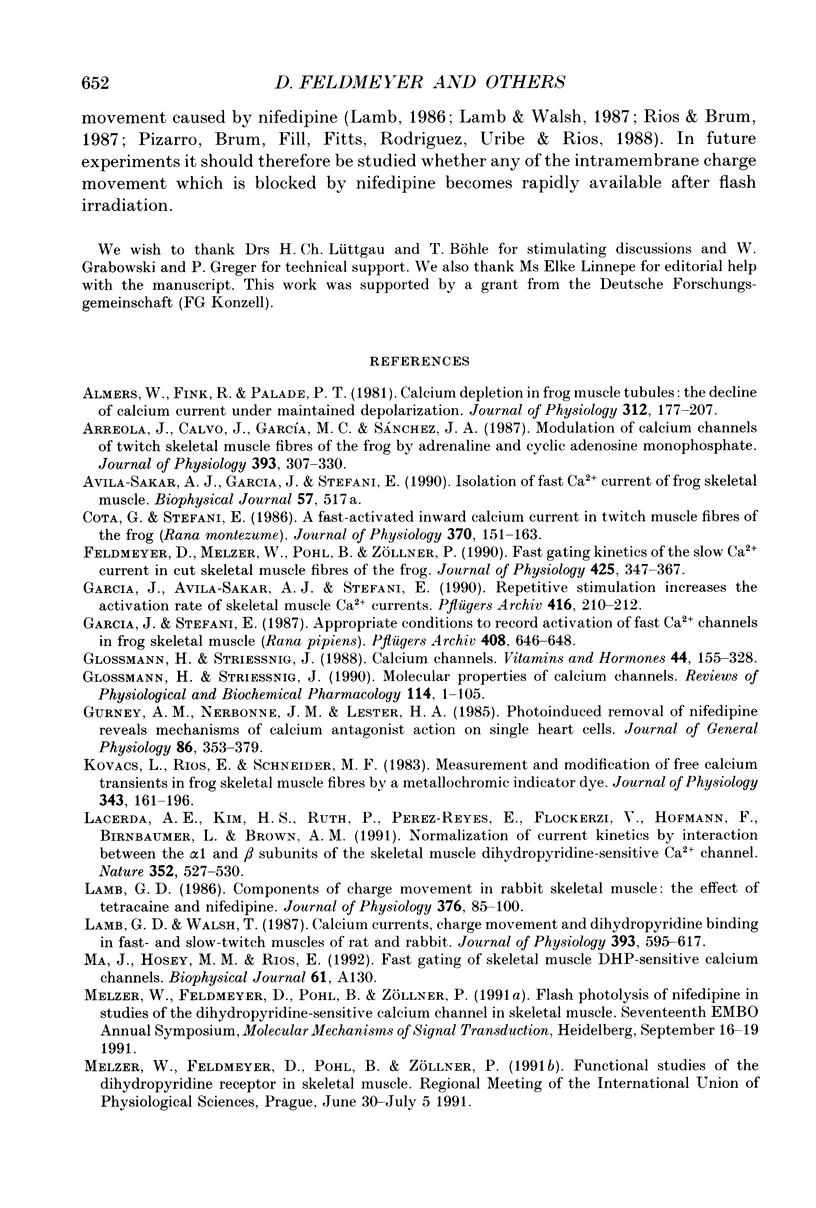
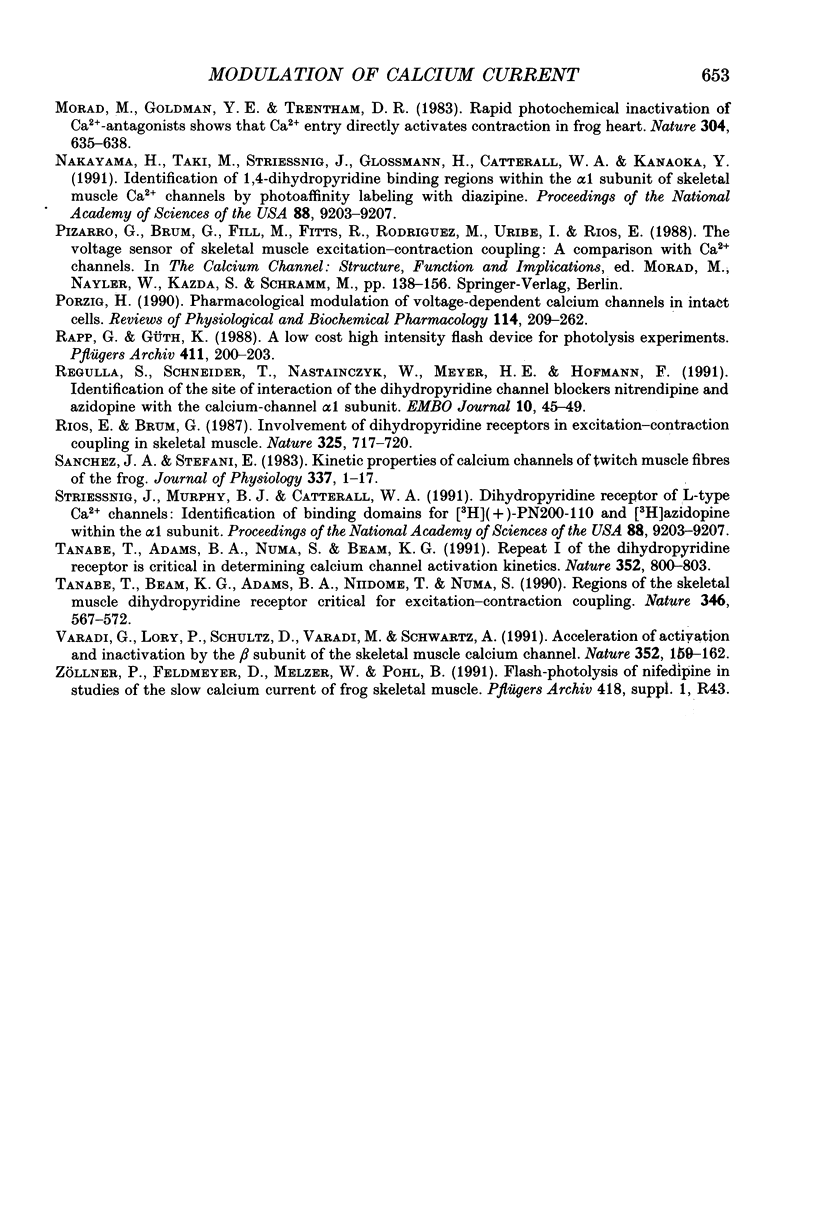
Selected References
These references are in PubMed. This may not be the complete list of references from this article.
- Almers W., Fink R., Palade P. T. Calcium depletion in frog muscle tubules: the decline of calcium current under maintained depolarization. J Physiol. 1981 Mar;312:177–207. doi: 10.1113/jphysiol.1981.sp013623. [DOI] [PMC free article] [PubMed] [Google Scholar]
- Arreola J., Calvo J., García M. C., Sánchez J. A. Modulation of calcium channels of twitch skeletal muscle fibres of the frog by adrenaline and cyclic adenosine monophosphate. J Physiol. 1987 Dec;393:307–330. doi: 10.1113/jphysiol.1987.sp016825. [DOI] [PMC free article] [PubMed] [Google Scholar]
- Cota G., Stefani E. A fast-activated inward calcium current in twitch muscle fibres of the frog (Rana montezume). J Physiol. 1986 Jan;370:151–163. doi: 10.1113/jphysiol.1986.sp015927. [DOI] [PMC free article] [PubMed] [Google Scholar]
- Feldmeyer D., Melzer W., Pohl B., Zöllner P. Fast gating kinetics of the slow Ca2+ current in cut skeletal muscle fibres of the frog. J Physiol. 1990 Jun;425:347–367. doi: 10.1113/jphysiol.1990.sp018107. [DOI] [PMC free article] [PubMed] [Google Scholar]
- Garcia J., Avila-Sakar A. J., Stefani E. Repetitive stimulation increases the activation rate of skeletal muscle Ca2+ currents. Pflugers Arch. 1990 Apr;416(1-2):210–212. doi: 10.1007/BF00370245. [DOI] [PubMed] [Google Scholar]
- García J., Stefani E. Appropriate conditions to record activation of fast Ca2+ channels in frog skeletal muscle (Rana pipiens). Pflugers Arch. 1987 May;408(6):646–648. doi: 10.1007/BF00581169. [DOI] [PubMed] [Google Scholar]
- Glossmann H., Striessnig J. Calcium channels. Vitam Horm. 1988;44:155–328. doi: 10.1016/s0083-6729(08)60695-0. [DOI] [PubMed] [Google Scholar]
- Glossmann H., Striessnig J. Molecular properties of calcium channels. Rev Physiol Biochem Pharmacol. 1990;114:1–105. doi: 10.1007/BFb0031018. [DOI] [PubMed] [Google Scholar]
- Gurney A. M., Nerbonne J. M., Lester H. A. Photoinduced removal of nifedipine reveals mechanisms of calcium antagonist action on single heart cells. J Gen Physiol. 1985 Sep;86(3):353–379. doi: 10.1085/jgp.86.3.353. [DOI] [PMC free article] [PubMed] [Google Scholar]
- Kovacs L., Rios E., Schneider M. F. Measurement and modification of free calcium transients in frog skeletal muscle fibres by a metallochromic indicator dye. J Physiol. 1983 Oct;343:161–196. doi: 10.1113/jphysiol.1983.sp014887. [DOI] [PMC free article] [PubMed] [Google Scholar]
- Lacerda A. E., Kim H. S., Ruth P., Perez-Reyes E., Flockerzi V., Hofmann F., Birnbaumer L., Brown A. M. Normalization of current kinetics by interaction between the alpha 1 and beta subunits of the skeletal muscle dihydropyridine-sensitive Ca2+ channel. Nature. 1991 Aug 8;352(6335):527–530. doi: 10.1038/352527a0. [DOI] [PubMed] [Google Scholar]
- Lamb G. D. Components of charge movement in rabbit skeletal muscle: the effect of tetracaine and nifedipine. J Physiol. 1986 Jul;376:85–100. doi: 10.1113/jphysiol.1986.sp016143. [DOI] [PMC free article] [PubMed] [Google Scholar]
- Lamb G. D., Walsh T. Calcium currents, charge movement and dihydropyridine binding in fast- and slow-twitch muscles of rat and rabbit. J Physiol. 1987 Dec;393:595–617. doi: 10.1113/jphysiol.1987.sp016843. [DOI] [PMC free article] [PubMed] [Google Scholar]
- Morad M., Goldman Y. E., Trentham D. R. Rapid photochemical inactivation of Ca2+-antagonists shows that Ca2+ entry directly activates contraction in frog heart. Nature. 1983 Aug 18;304(5927):635–638. doi: 10.1038/304635a0. [DOI] [PubMed] [Google Scholar]
- Nakayama H., Taki M., Striessnig J., Glossmann H., Catterall W. A., Kanaoka Y. Identification of 1,4-dihydropyridine binding regions within the alpha 1 subunit of skeletal muscle Ca2+ channels by photoaffinity labeling with diazipine. Proc Natl Acad Sci U S A. 1991 Oct 15;88(20):9203–9207. doi: 10.1073/pnas.88.20.9203. [DOI] [PMC free article] [PubMed] [Google Scholar]
- Nakayama H., Taki M., Striessnig J., Glossmann H., Catterall W. A., Kanaoka Y. Identification of 1,4-dihydropyridine binding regions within the alpha 1 subunit of skeletal muscle Ca2+ channels by photoaffinity labeling with diazipine. Proc Natl Acad Sci U S A. 1991 Oct 15;88(20):9203–9207. doi: 10.1073/pnas.88.20.9203. [DOI] [PMC free article] [PubMed] [Google Scholar]
- Porzig H. Pharmacological modulation of voltage-dependent calcium channels in intact cells. Rev Physiol Biochem Pharmacol. 1990;114:209–262. doi: 10.1007/BFb0031020. [DOI] [PubMed] [Google Scholar]
- Rapp G., Güth K. A low cost high intensity flash device for photolysis experiments. Pflugers Arch. 1988 Feb;411(2):200–203. doi: 10.1007/BF00582315. [DOI] [PubMed] [Google Scholar]
- Regulla S., Schneider T., Nastainczyk W., Meyer H. E., Hofmann F. Identification of the site of interaction of the dihydropyridine channel blockers nitrendipine and azidopine with the calcium-channel alpha 1 subunit. EMBO J. 1991 Jan;10(1):45–49. doi: 10.1002/j.1460-2075.1991.tb07919.x. [DOI] [PMC free article] [PubMed] [Google Scholar]
- Rios E., Brum G. Involvement of dihydropyridine receptors in excitation-contraction coupling in skeletal muscle. Nature. 1987 Feb 19;325(6106):717–720. doi: 10.1038/325717a0. [DOI] [PubMed] [Google Scholar]
- Sánchez J. A., Stefani E. Kinetic properties of calcium channels of twitch muscle fibres of the frog. J Physiol. 1983 Apr;337:1–17. doi: 10.1113/jphysiol.1983.sp014607. [DOI] [PMC free article] [PubMed] [Google Scholar]
- Tanabe T., Adams B. A., Numa S., Beam K. G. Repeat I of the dihydropyridine receptor is critical in determining calcium channel activation kinetics. Nature. 1991 Aug 29;352(6338):800–803. doi: 10.1038/352800a0. [DOI] [PubMed] [Google Scholar]
- Tanabe T., Beam K. G., Adams B. A., Niidome T., Numa S. Regions of the skeletal muscle dihydropyridine receptor critical for excitation-contraction coupling. Nature. 1990 Aug 9;346(6284):567–569. doi: 10.1038/346567a0. [DOI] [PubMed] [Google Scholar]
- Varadi G., Lory P., Schultz D., Varadi M., Schwartz A. Acceleration of activation and inactivation by the beta subunit of the skeletal muscle calcium channel. Nature. 1991 Jul 11;352(6331):159–162. doi: 10.1038/352159a0. [DOI] [PubMed] [Google Scholar]