Abstract
1. Single isolated rat cardiac myocytes were loaded with either the pentapotassium salt form or the acetoxymethyl ester (AM) form of the calcium-sensitive fluorescent probe, Indo-1. The relationship of the Indo-1 fluorescence transient, an index of the change in cytosolic calcium [Ca2+]i concentration, to the simultaneously measured cell length during the electrically stimulated twitch originating from slack length at 23 degrees C was evaluated. It was demonstrated that even if the Ca2+ dissociation rate from Indo-1 was assumed to be as slow as 10 s-1, the descending limb ('relaxation phase') of the Indo-1 fluorescence transient induced by excitation under these conditions is in equilibrium with the [Ca2+]i transient. Additionally, the extent of Indo-1 loading employed did not substantially alter the twitch characteristics. 2. A unique relationship between the fluorescence transient and cell length was observed during relaxation of contractions that varied in amplitude. This was manifest as a common trajectory in the cell length vs. [Ca2+]i phase-plane diagrams beginning at the time of cell relengthening. The common trajectory could also be demonstrated in Indo-1 AM-loaded cells. The Indo-1 fluorescence-length relation defined by this common trajectory is steeper than that described by the relation of peak contraction amplitude and peak fluorescence during the twitch contractions. 3. The trajectory of the [Ca2+]i-length relation elicited via an abrupt, rapid, brief (200 ms) pulse of caffeine directly onto the cell surface or by 'tetanization' of cells in the presence of ryanodine is identical to the common [Ca2+]i-length trajectory formed by electrically stimulated contractions of different magnitudes. As the [Ca2+]i and length transients induced by caffeine application or during tetanization in the presence of ryanodine develop with a much slower time course than those elicited by electrical stimulation, the common trajectory is not fortuitous, i.e. it cannot be attributed to equivalent rate-limiting steps for the decrease of [Ca2+]i and cell relengthening. 4. The [Ca2+]i-length relation defined by the common trajectory shifts appropriately in response to perturbations that have previously been demonstrated to alter the steady-state myofilament Ca2+ sensitivity in skinned cardiac fibres. Specifically, the trajectory shifts leftward in response to an acute increase in pH or following the addition of novel myofilament calcium-sensitizing thiadiazinone derivatives; a rightward shift occurs in response to an acute reduction in pH or following the addition of butanedione monoxime.(ABSTRACT TRUNCATED AT 400 WORDS)
Full text
PDF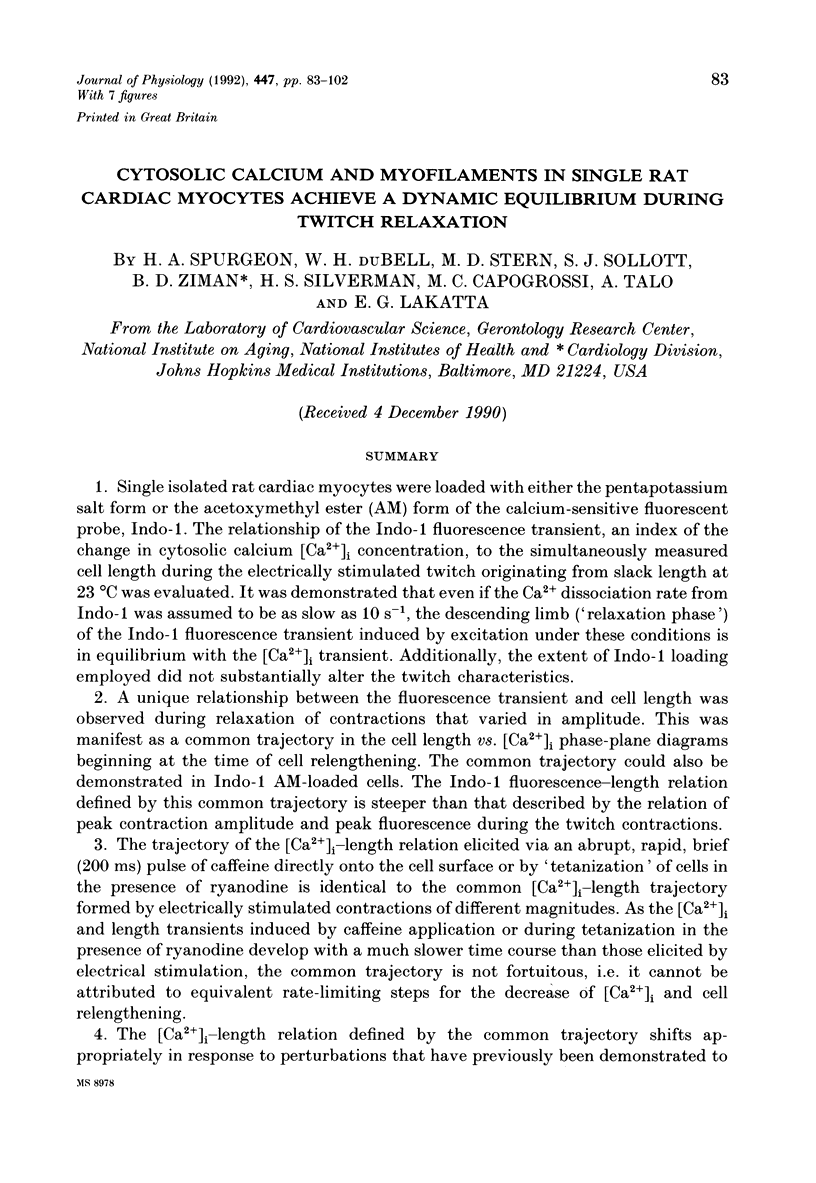
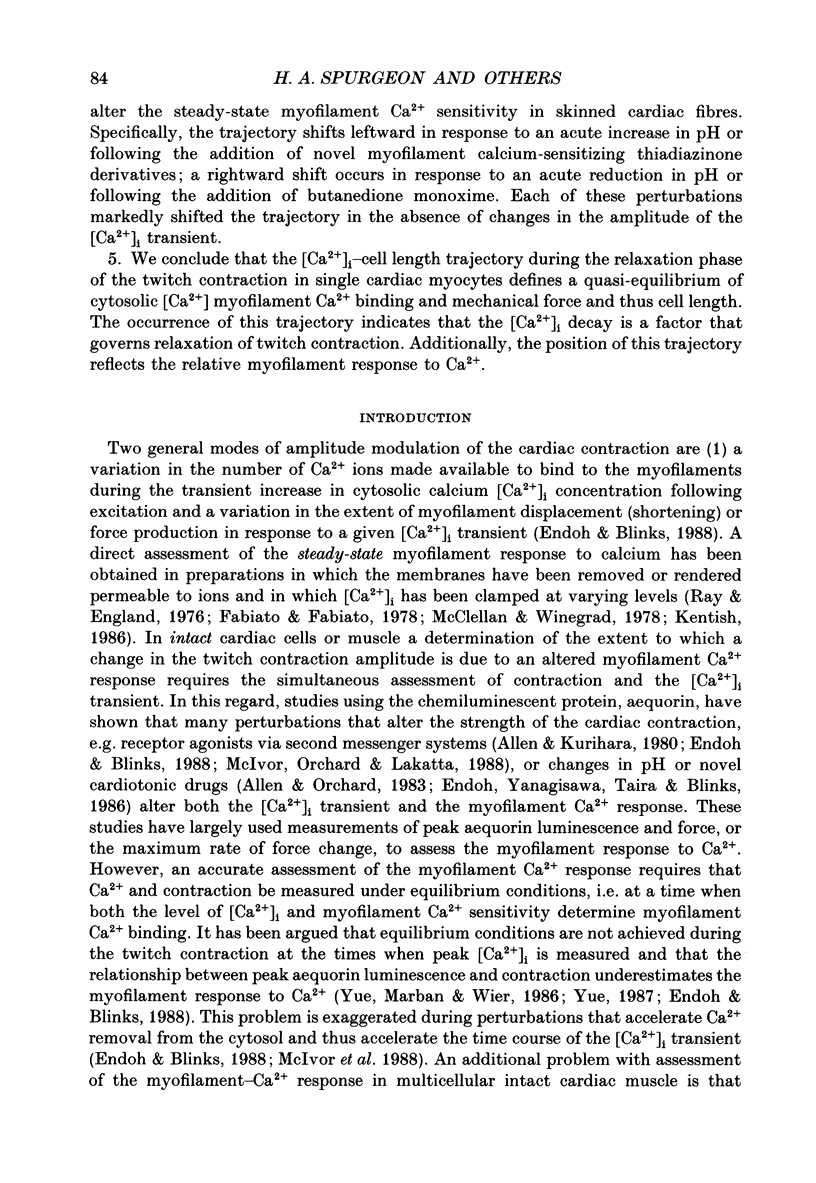
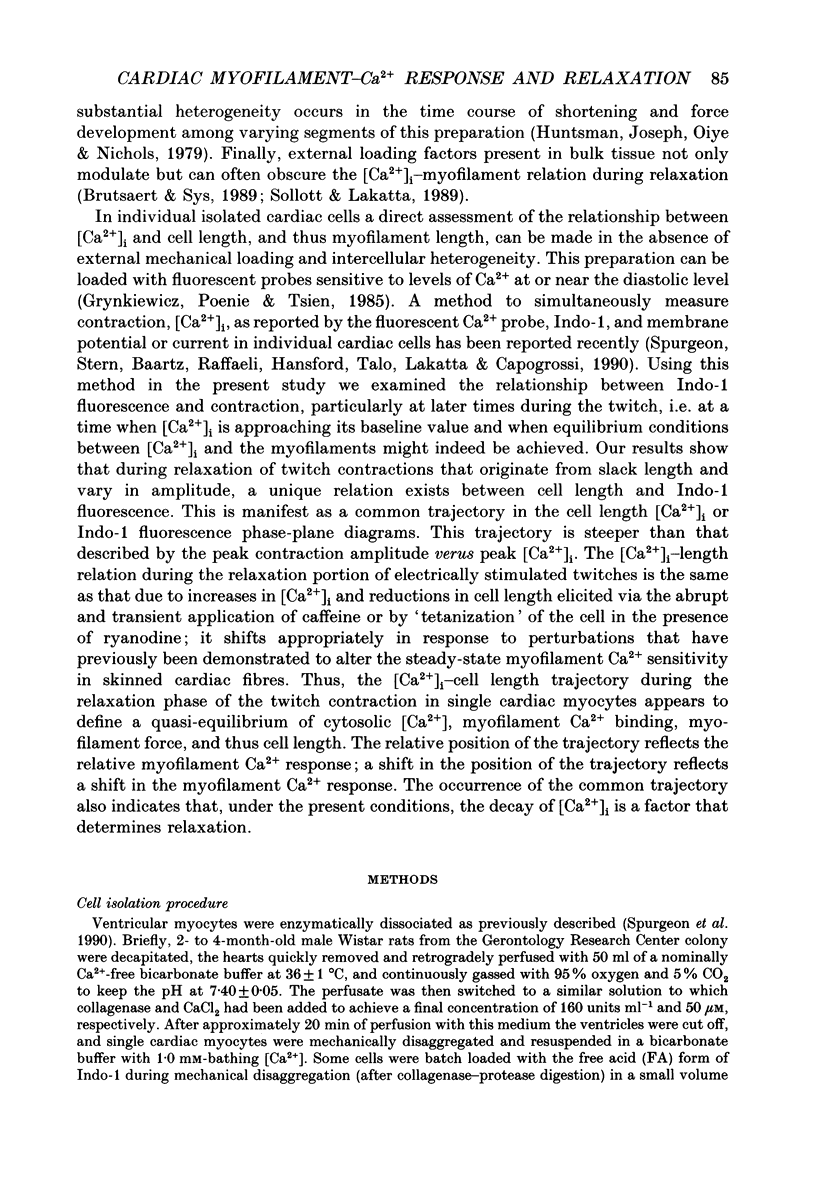
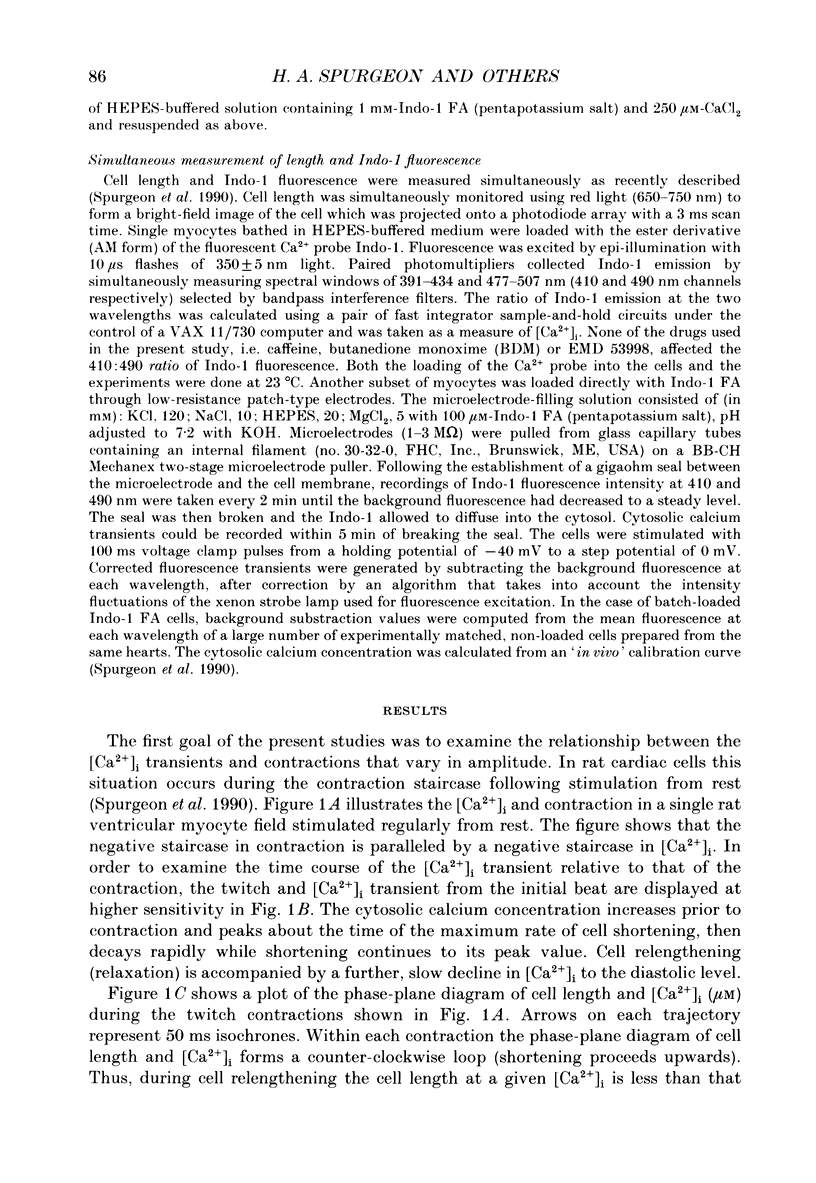
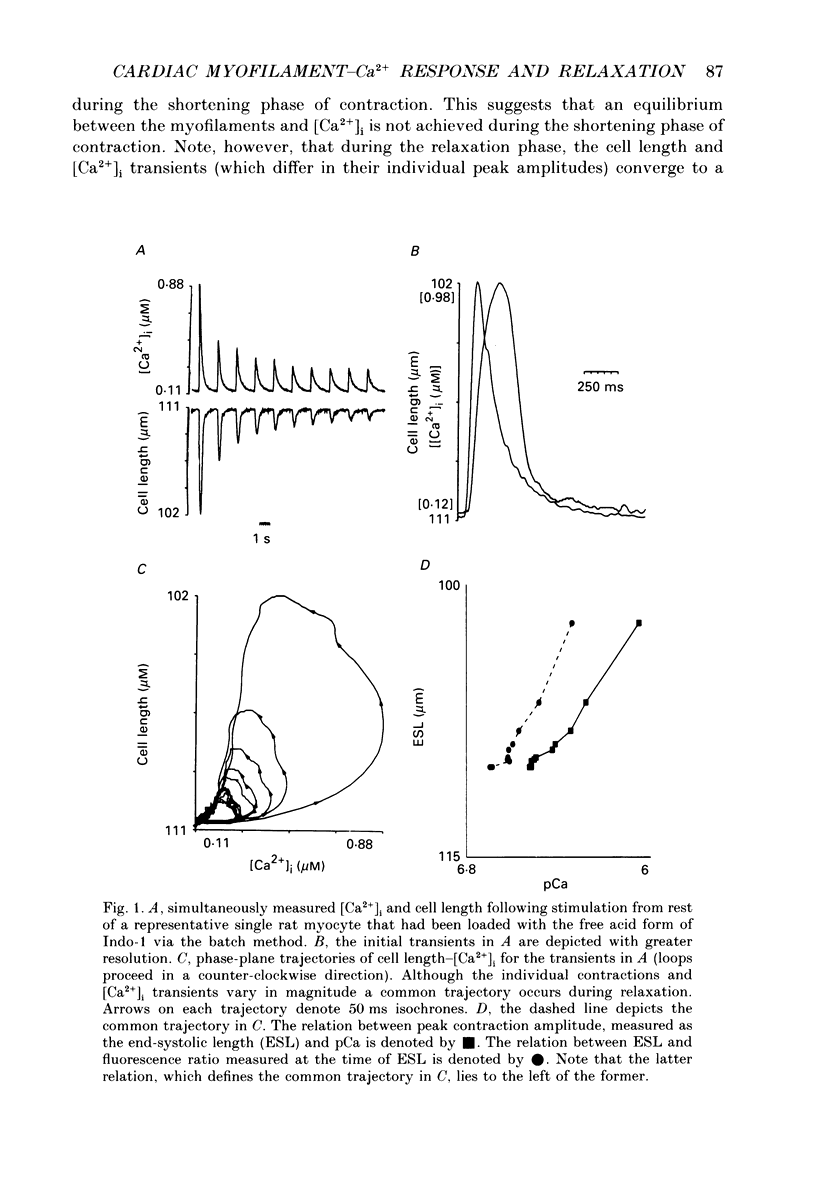
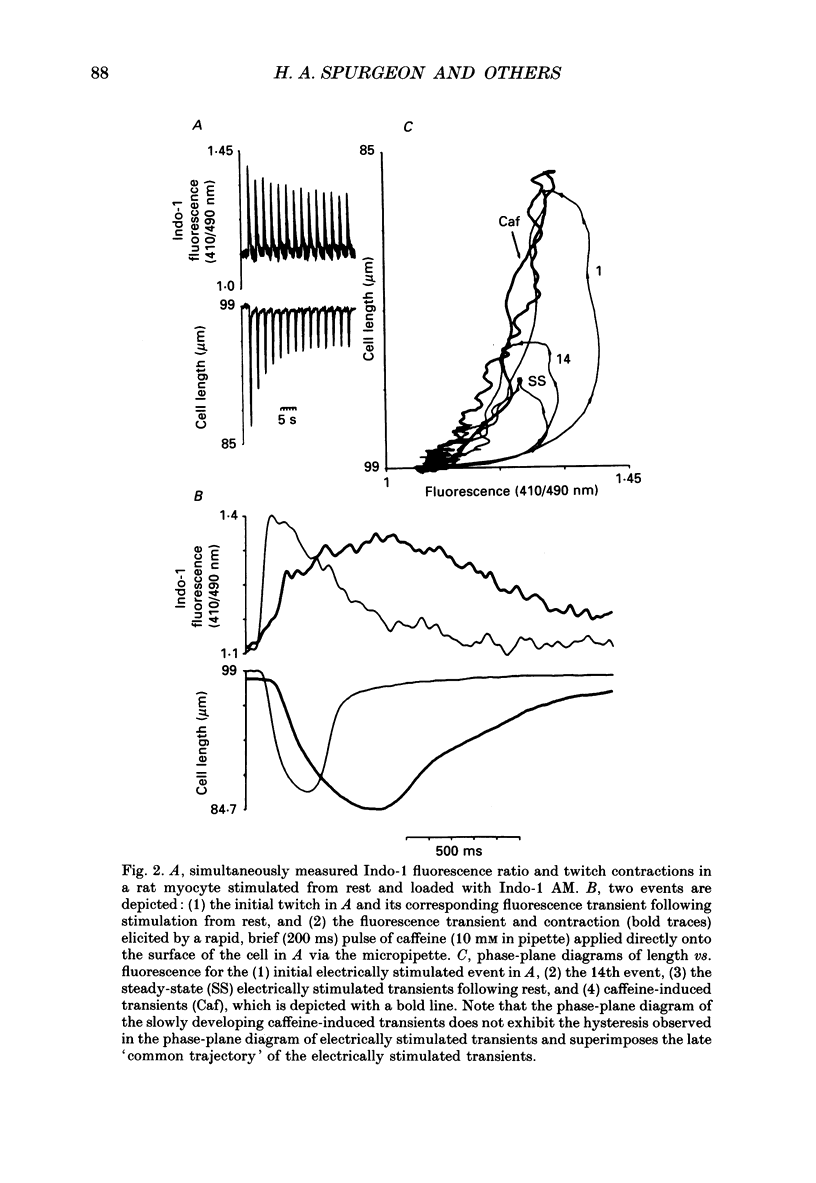
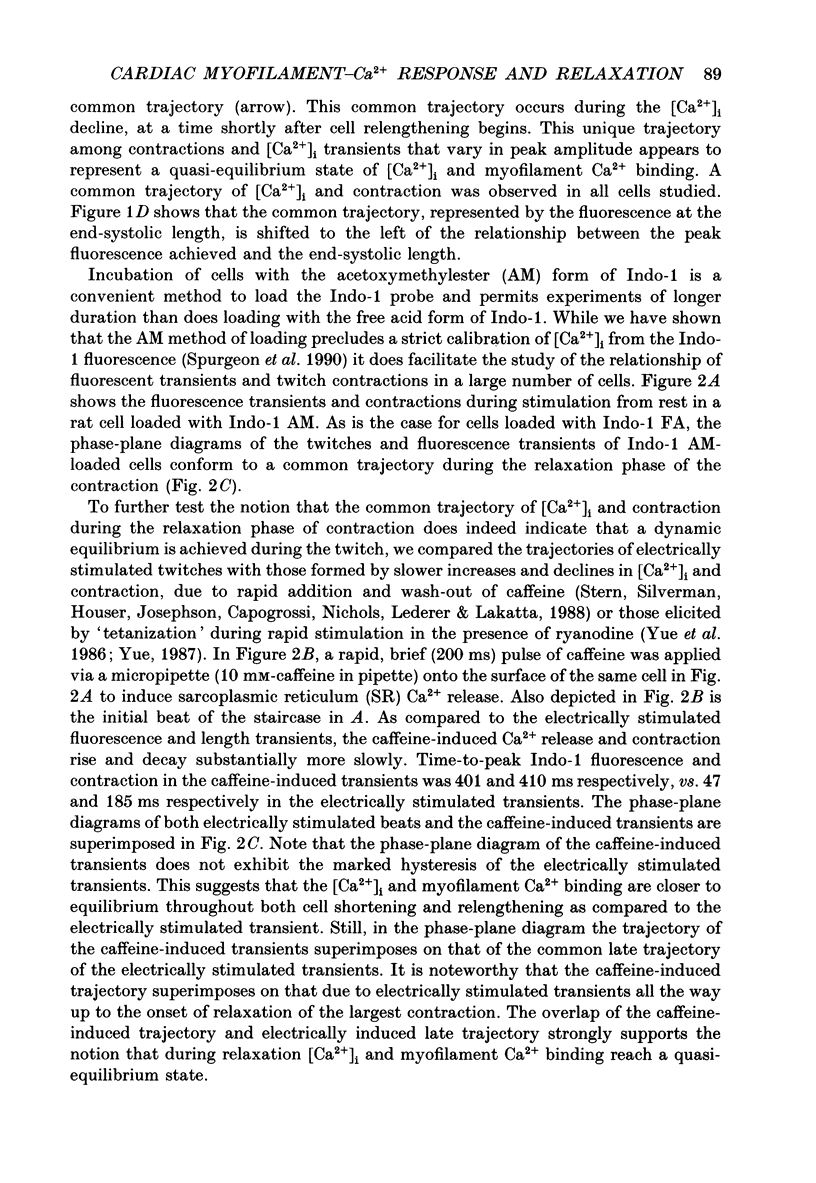
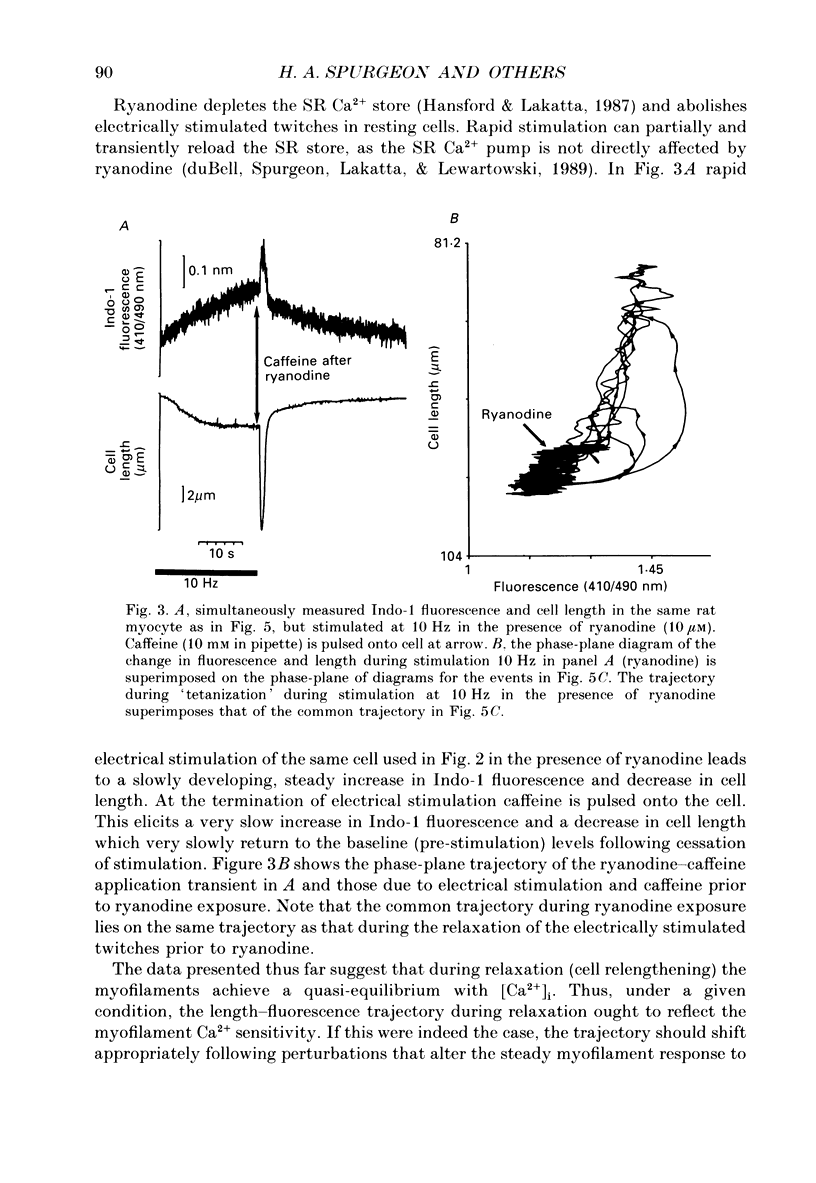
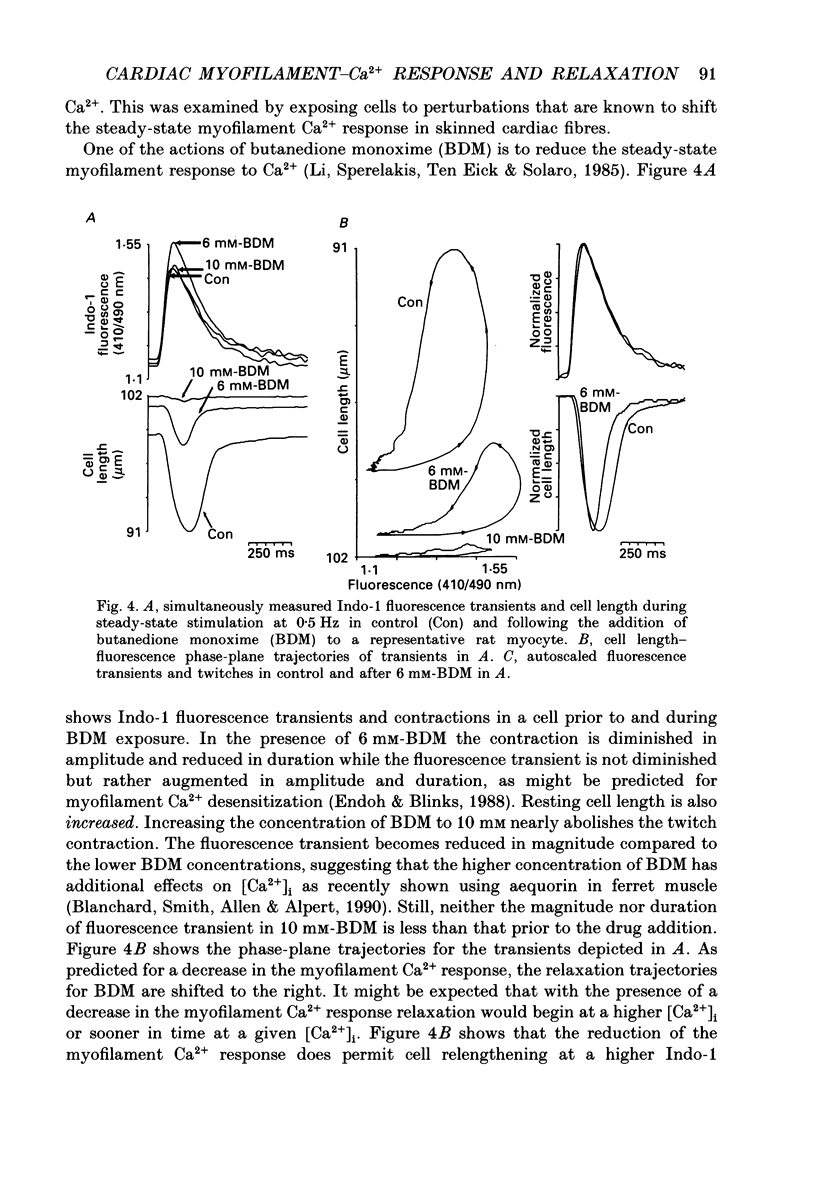
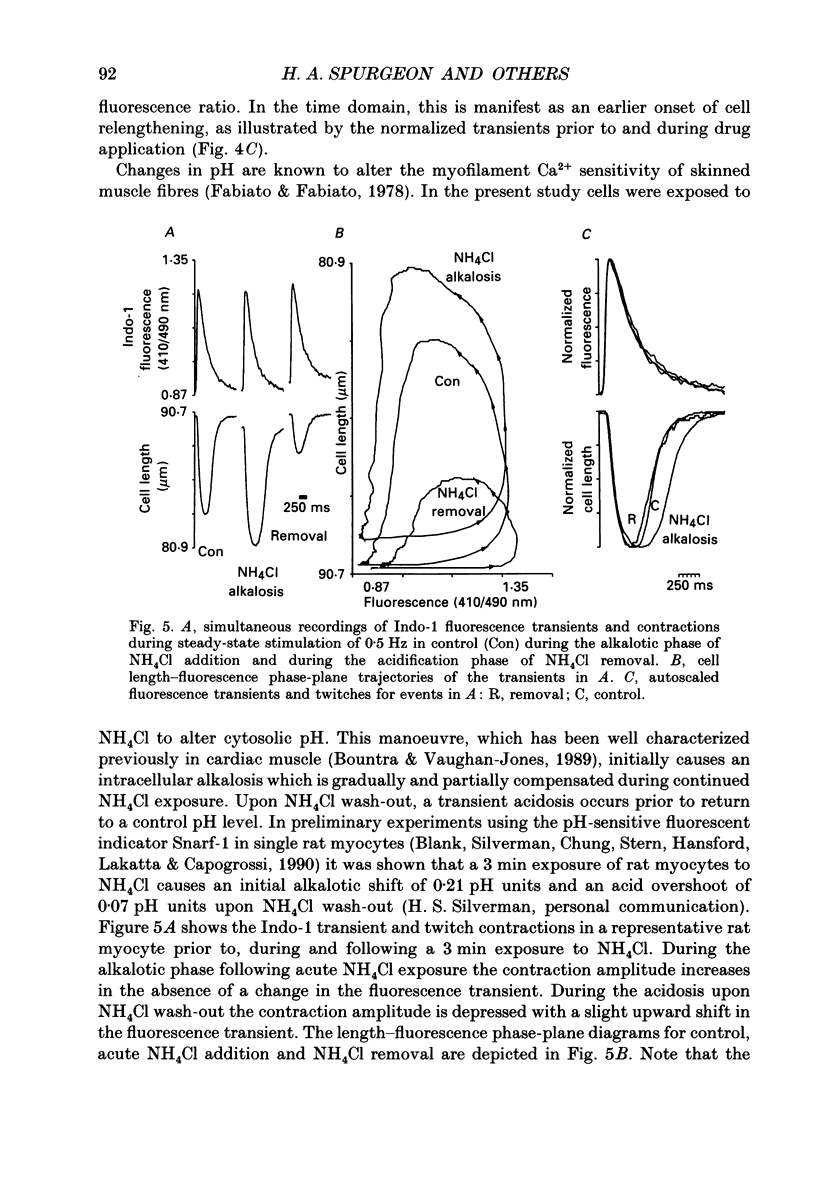
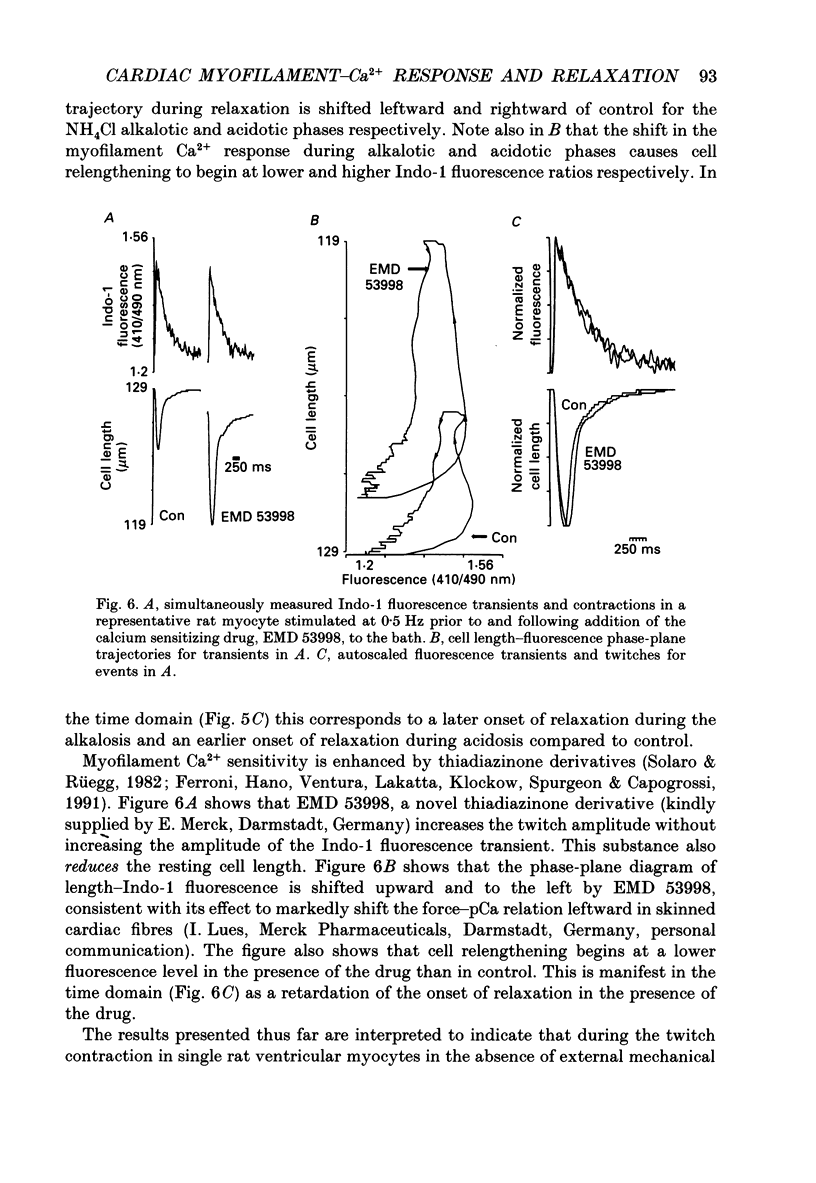
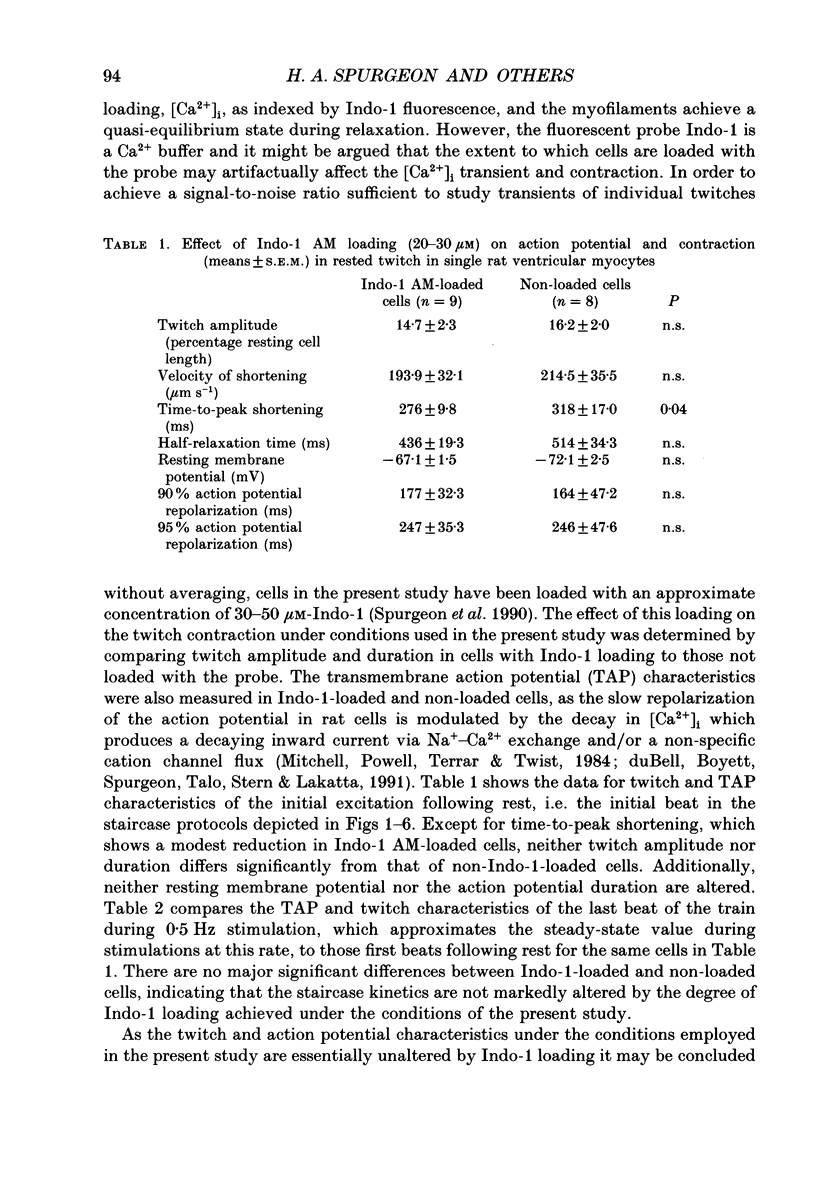
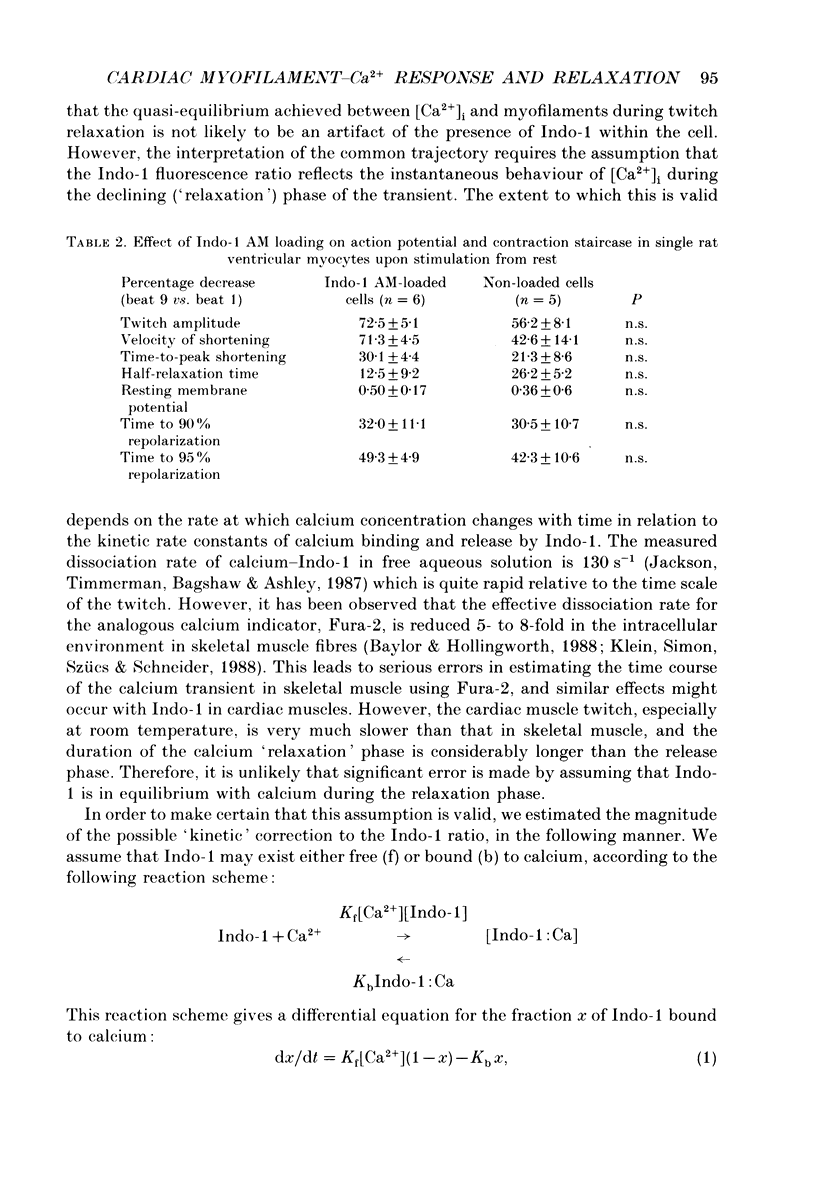
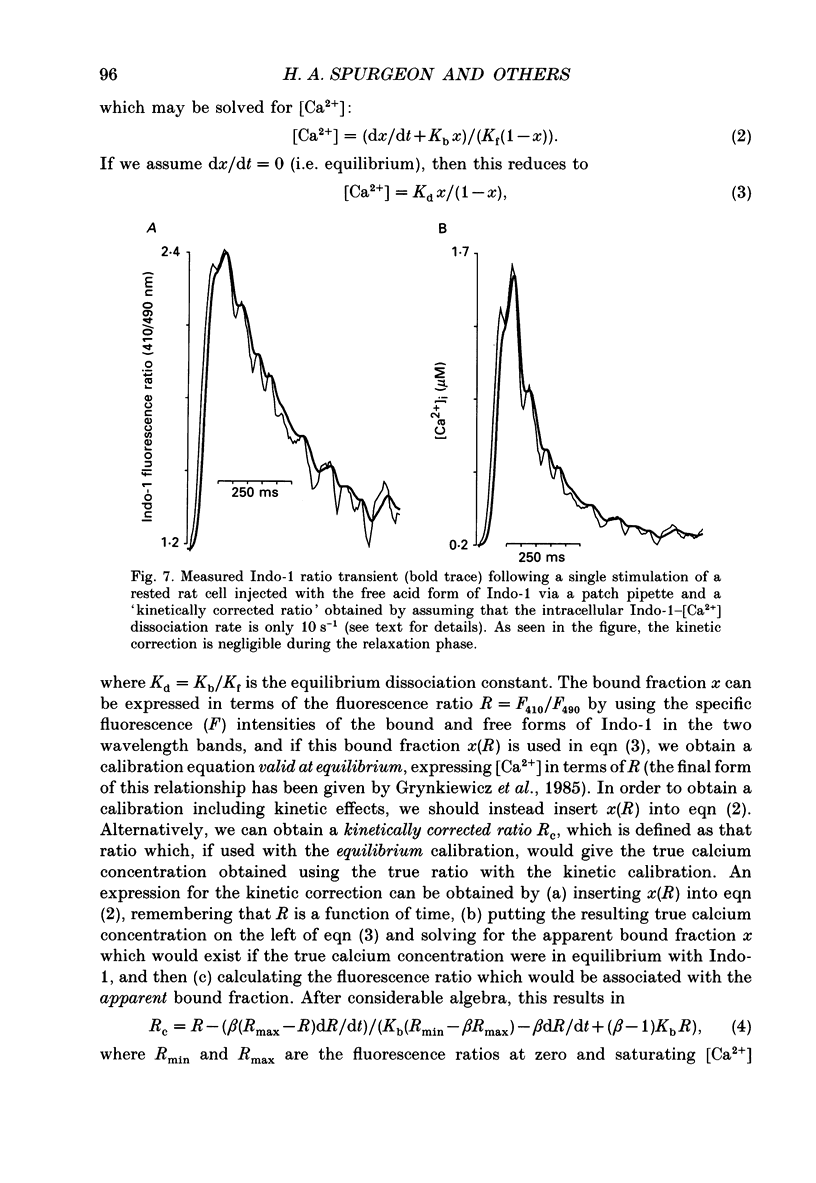
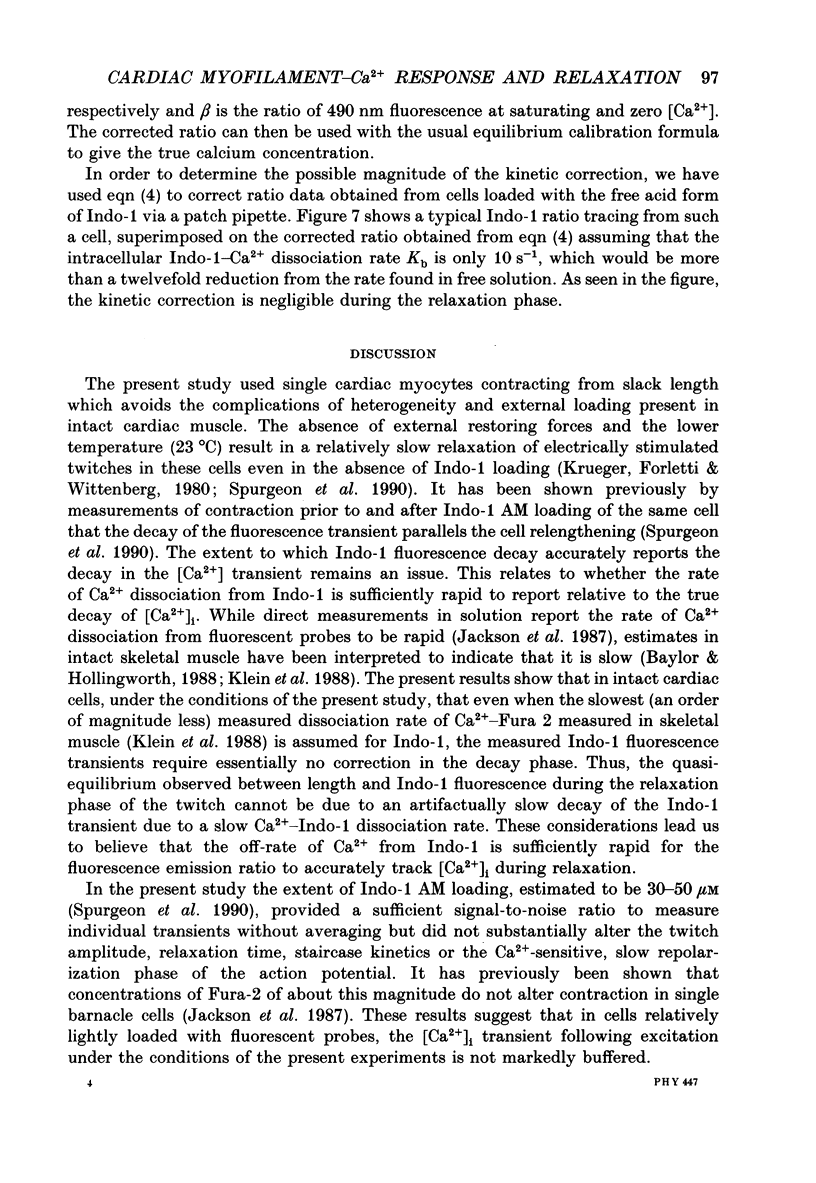
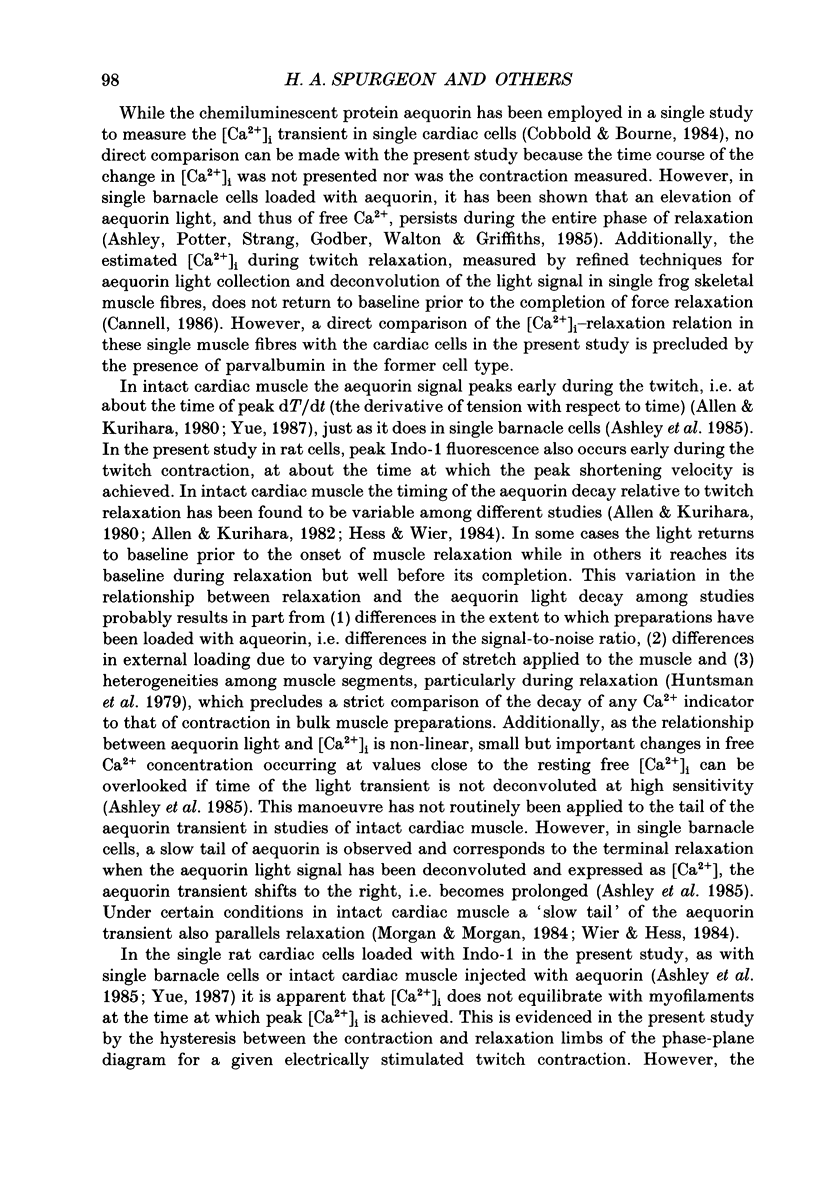
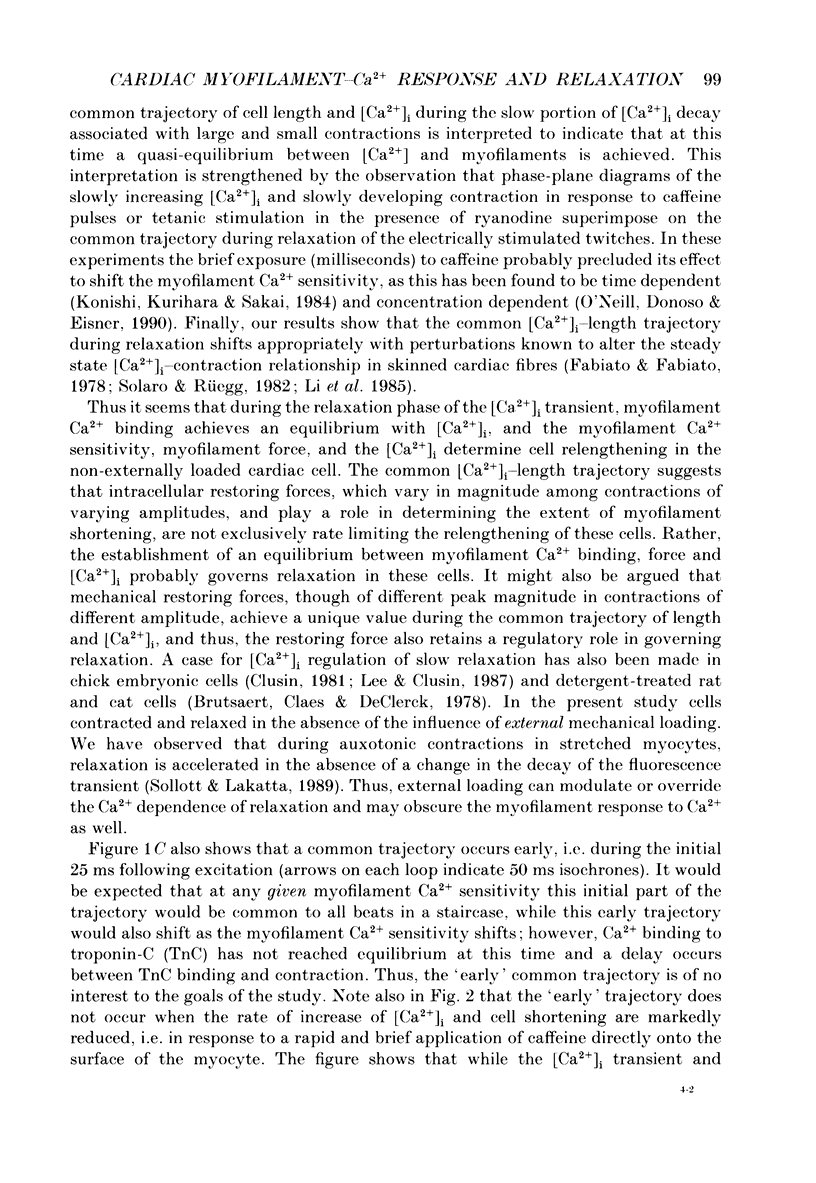
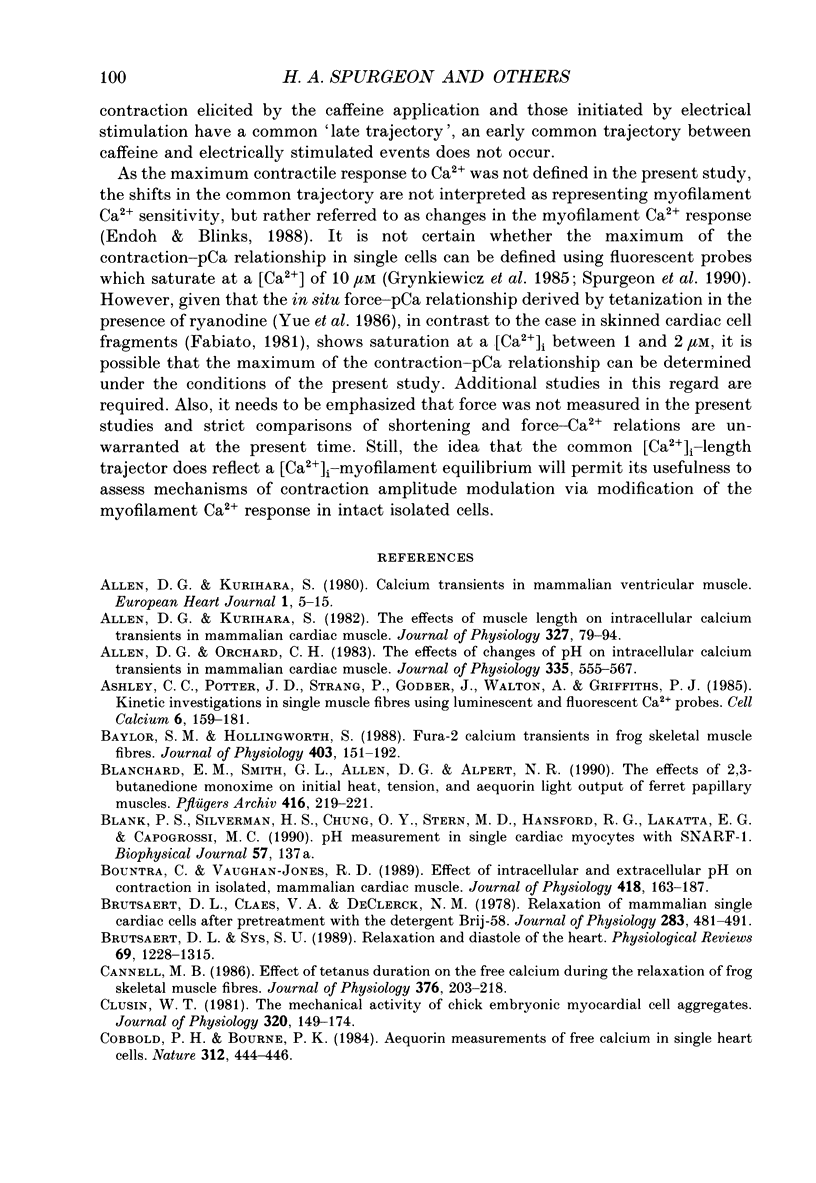
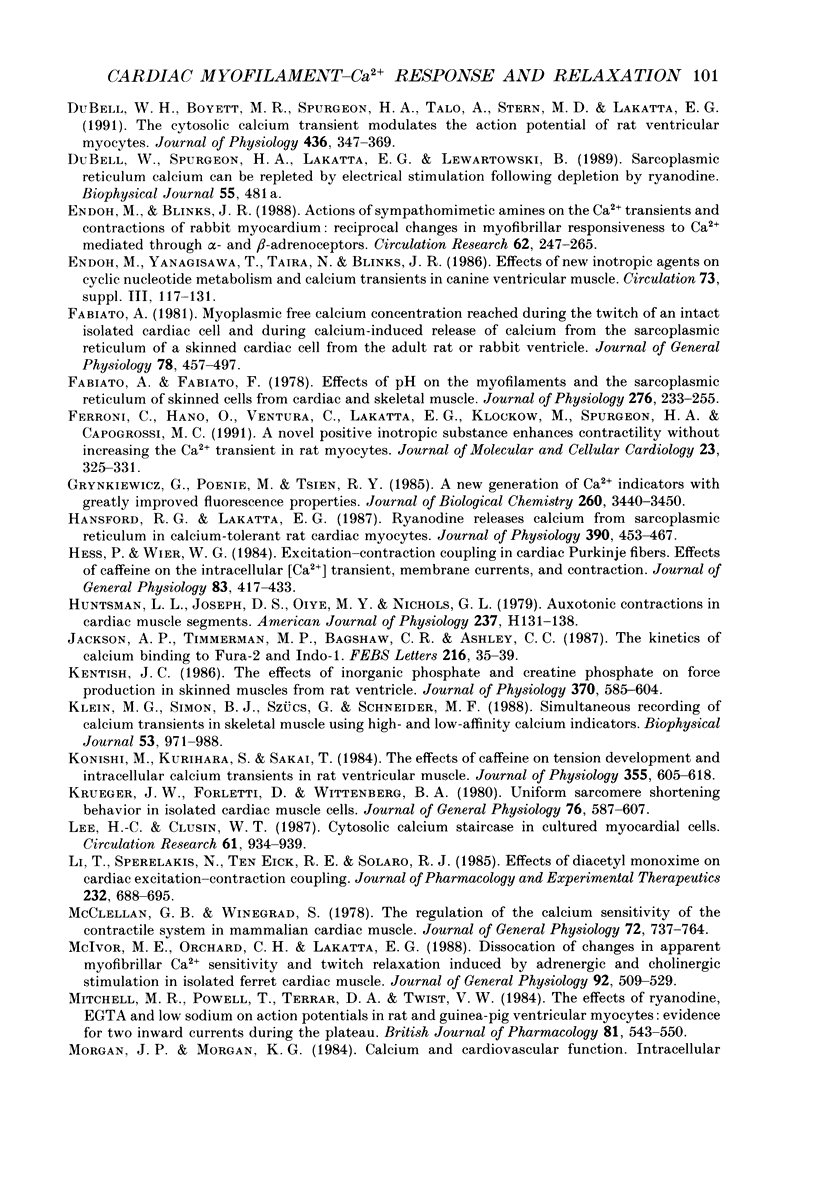
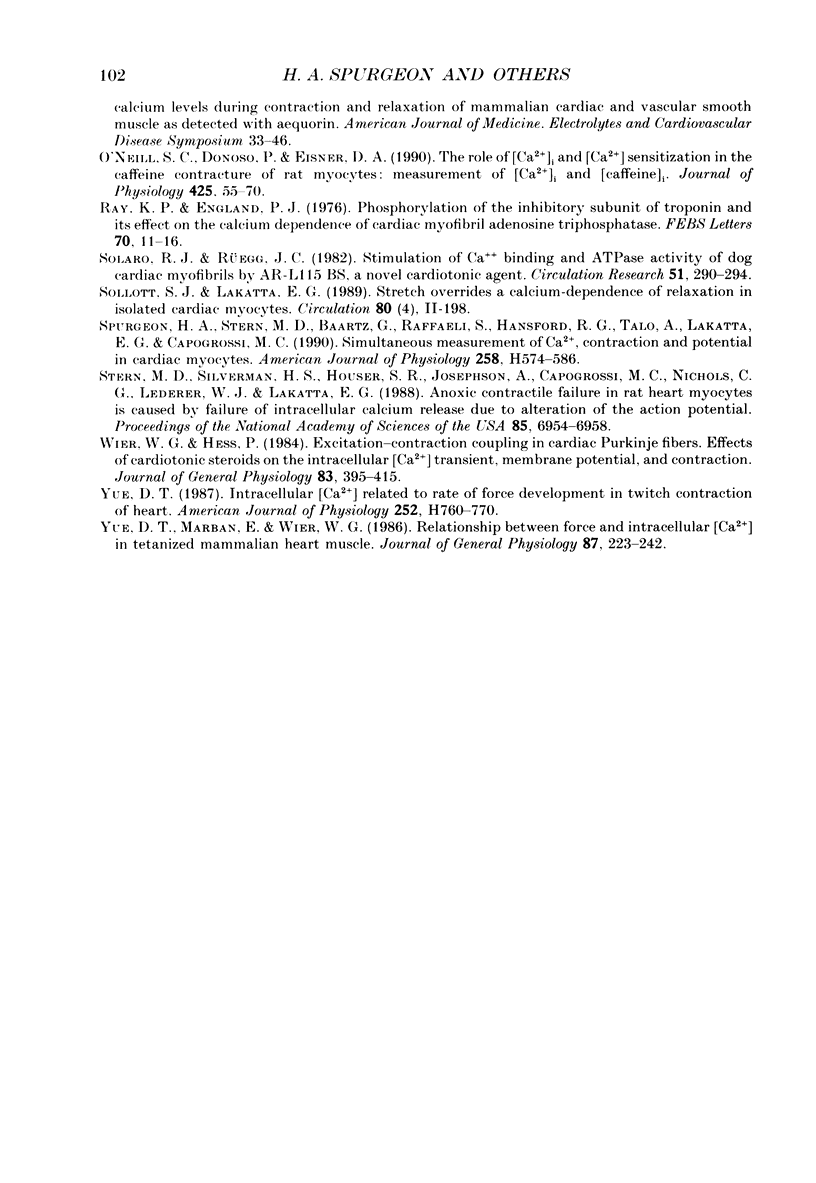
Selected References
These references are in PubMed. This may not be the complete list of references from this article.
- Allen D. G., Kurihara S. Calcium transients in mammalian ventricular muscle. Eur Heart J. 1980;Suppl A:5–15. doi: 10.1093/eurheartj/1.suppl_1.5. [DOI] [PubMed] [Google Scholar]
- Allen D. G., Kurihara S. The effects of muscle length on intracellular calcium transients in mammalian cardiac muscle. J Physiol. 1982 Jun;327:79–94. doi: 10.1113/jphysiol.1982.sp014221. [DOI] [PMC free article] [PubMed] [Google Scholar]
- Allen D. G., Orchard C. H. The effects of changes of pH on intracellular calcium transients in mammalian cardiac muscle. J Physiol. 1983 Feb;335:555–567. doi: 10.1113/jphysiol.1983.sp014550. [DOI] [PMC free article] [PubMed] [Google Scholar]
- Ashley C. C., Potter J. D., Strang P., Godber J., Walton A., Griffiths P. J. Kinetic investigations in single muscle fibres using luminescent and fluorescent Ca2+ probes. Cell Calcium. 1985 Apr;6(1-2):159–181. doi: 10.1016/0143-4160(85)90042-9. [DOI] [PubMed] [Google Scholar]
- Baylor S. M., Hollingworth S. Fura-2 calcium transients in frog skeletal muscle fibres. J Physiol. 1988 Sep;403:151–192. doi: 10.1113/jphysiol.1988.sp017244. [DOI] [PMC free article] [PubMed] [Google Scholar]
- Blanchard E. M., Smith G. L., Allen D. G., Alpert N. R. The effects of 2,3-butanedione monoxime on initial heat, tension, and aequorin light output of ferret papillary muscles. Pflugers Arch. 1990 Apr;416(1-2):219–221. doi: 10.1007/BF00370248. [DOI] [PubMed] [Google Scholar]
- Bountra C., Vaughan-Jones R. D. Effect of intracellular and extracellular pH on contraction in isolated, mammalian cardiac muscle. J Physiol. 1989 Nov;418:163–187. doi: 10.1113/jphysiol.1989.sp017833. [DOI] [PMC free article] [PubMed] [Google Scholar]
- Brutsaert D. L., Claes V. A., De Clerck N. M. Relaxation of mammalian single cardiac cells after pretreatment with the detergent Brij-58. J Physiol. 1978 Oct;283:481–491. doi: 10.1113/jphysiol.1978.sp012514. [DOI] [PMC free article] [PubMed] [Google Scholar]
- Brutsaert D. L., Sys S. U. Relaxation and diastole of the heart. Physiol Rev. 1989 Oct;69(4):1228–1315. doi: 10.1152/physrev.1989.69.4.1228. [DOI] [PubMed] [Google Scholar]
- Cannell M. B. Effect of tetanus duration on the free calcium during the relaxation of frog skeletal muscle fibres. J Physiol. 1986 Jul;376:203–218. doi: 10.1113/jphysiol.1986.sp016149. [DOI] [PMC free article] [PubMed] [Google Scholar]
- Clusin W. T., Hamilton W. E., Nelson D. V. The mechanical activity of chick embryonic myocardial cell aggregates. J Physiol. 1981 Nov;320:149–174. doi: 10.1113/jphysiol.1981.sp013941. [DOI] [PMC free article] [PubMed] [Google Scholar]
- Cobbold P. H., Bourne P. K. Aequorin measurements of free calcium in single heart cells. 1984 Nov 29-Dec 5Nature. 312(5993):444–446. doi: 10.1038/312444a0. [DOI] [PubMed] [Google Scholar]
- Endoh M., Blinks J. R. Actions of sympathomimetic amines on the Ca2+ transients and contractions of rabbit myocardium: reciprocal changes in myofibrillar responsiveness to Ca2+ mediated through alpha- and beta-adrenoceptors. Circ Res. 1988 Feb;62(2):247–265. doi: 10.1161/01.res.62.2.247. [DOI] [PubMed] [Google Scholar]
- Fabiato A., Fabiato F. Effects of pH on the myofilaments and the sarcoplasmic reticulum of skinned cells from cardiace and skeletal muscles. J Physiol. 1978 Mar;276:233–255. doi: 10.1113/jphysiol.1978.sp012231. [DOI] [PMC free article] [PubMed] [Google Scholar]
- Fabiato A. Myoplasmic free calcium concentration reached during the twitch of an intact isolated cardiac cell and during calcium-induced release of calcium from the sarcoplasmic reticulum of a skinned cardiac cell from the adult rat or rabbit ventricle. J Gen Physiol. 1981 Nov;78(5):457–497. doi: 10.1085/jgp.78.5.457. [DOI] [PMC free article] [PubMed] [Google Scholar]
- Ferroni C., Hano O., Ventura C., Lakatta E. G., Klockow M., Spurgeon H., Capogrossi M. C. A novel positive inotropic substance enhances contractility without increasing the Ca2+ transient in rat myocardium. J Mol Cell Cardiol. 1991 Mar;23(3):325–331. doi: 10.1016/0022-2828(91)90068-w. [DOI] [PubMed] [Google Scholar]
- Grynkiewicz G., Poenie M., Tsien R. Y. A new generation of Ca2+ indicators with greatly improved fluorescence properties. J Biol Chem. 1985 Mar 25;260(6):3440–3450. [PubMed] [Google Scholar]
- Hansford R. G., Lakatta E. G. Ryanodine releases calcium from sarcoplasmic reticulum in calcium-tolerant rat cardiac myocytes. J Physiol. 1987 Sep;390:453–467. doi: 10.1113/jphysiol.1987.sp016711. [DOI] [PMC free article] [PubMed] [Google Scholar]
- Hess P., Wier W. G. Excitation-contraction coupling in cardiac Purkinje fibers. Effects of caffeine on the intracellular [Ca2+] transient, membrane currents, and contraction. J Gen Physiol. 1984 Mar;83(3):417–433. doi: 10.1085/jgp.83.3.417. [DOI] [PMC free article] [PubMed] [Google Scholar]
- Huntsman L. L., Joseph D. S., Oiye M. Y., Nichols G. L. Auxotonic contractions in cardiac muscle segments. Am J Physiol. 1979 Aug;237(2):H131–H138. doi: 10.1152/ajpheart.1979.237.2.H131. [DOI] [PubMed] [Google Scholar]
- Jackson A. P., Timmerman M. P., Bagshaw C. R., Ashley C. C. The kinetics of calcium binding to fura-2 and indo-1. FEBS Lett. 1987 May 25;216(1):35–39. doi: 10.1016/0014-5793(87)80752-4. [DOI] [PubMed] [Google Scholar]
- Kentish J. C. The effects of inorganic phosphate and creatine phosphate on force production in skinned muscles from rat ventricle. J Physiol. 1986 Jan;370:585–604. doi: 10.1113/jphysiol.1986.sp015952. [DOI] [PMC free article] [PubMed] [Google Scholar]
- Klein M. G., Simon B. J., Szucs G., Schneider M. F. Simultaneous recording of calcium transients in skeletal muscle using high- and low-affinity calcium indicators. Biophys J. 1988 Jun;53(6):971–988. doi: 10.1016/S0006-3495(88)83178-3. [DOI] [PMC free article] [PubMed] [Google Scholar]
- Konishi M., Kurihara S., Sakai T. The effects of caffeine on tension development and intracellular calcium transients in rat ventricular muscle. J Physiol. 1984 Oct;355:605–618. doi: 10.1113/jphysiol.1984.sp015441. [DOI] [PMC free article] [PubMed] [Google Scholar]
- Krueger J. W., Forletti D., Wittenberg B. A. Uniform sarcomere shortening behavior in isolated cardiac muscle cells. J Gen Physiol. 1980 Nov;76(5):587–607. doi: 10.1085/jgp.76.5.587. [DOI] [PMC free article] [PubMed] [Google Scholar]
- Lee H. C., Clusin W. T. Cytosolic calcium staircase in cultured myocardial cells. Circ Res. 1987 Dec;61(6):934–939. doi: 10.1161/01.res.61.6.934. [DOI] [PubMed] [Google Scholar]
- Li T., Sperelakis N., Teneick R. E., Solaro R. J. Effects of diacetyl monoxime on cardiac excitation-contraction coupling. J Pharmacol Exp Ther. 1985 Mar;232(3):688–695. [PubMed] [Google Scholar]
- McClellan G. B., Winegrad S. The regulation of the calcium sensitivity of the contractile system in mammalian cardiac muscle. J Gen Physiol. 1978 Dec;72(6):737–764. doi: 10.1085/jgp.72.6.737. [DOI] [PMC free article] [PubMed] [Google Scholar]
- McIvor M. E., Orchard C. H., Lakatta E. G. Dissociation of changes in apparent myofibrillar Ca2+ sensitivity and twitch relaxation induced by adrenergic and cholinergic stimulation in isolated ferret cardiac muscle. J Gen Physiol. 1988 Oct;92(4):509–529. doi: 10.1085/jgp.92.4.509. [DOI] [PMC free article] [PubMed] [Google Scholar]
- Mitchell M. R., Powell T., Terrar D. A., Twist V. W. The effects of ryanodine, EGTA and low-sodium on action potentials in rat and guinea-pig ventricular myocytes: evidence for two inward currents during the plateau. Br J Pharmacol. 1984 Mar;81(3):543–550. doi: 10.1111/j.1476-5381.1984.tb10107.x. [DOI] [PMC free article] [PubMed] [Google Scholar]
- O'Neill S. C., Donoso P., Eisner D. A. The role of [Ca2+]i and [Ca2+] sensitization in the caffeine contracture of rat myocytes: measurement of [Ca2+]i and [caffeine]i. J Physiol. 1990 Jun;425:55–70. doi: 10.1113/jphysiol.1990.sp018092. [DOI] [PMC free article] [PubMed] [Google Scholar]
- Ray K. P., England P. J. Phosphorylation of the inhibitory subunit of troponin and its effect on the calcium dependence of cardiac myofibril adenosine triphosphatase. FEBS Lett. 1976 Nov;70(1):11–16. doi: 10.1016/0014-5793(76)80716-8. [DOI] [PubMed] [Google Scholar]
- Solaro R. J., Rüegg J. C. Stimulation of Ca++ binding and ATPase activity of dog cardiac myofibrils by AR-L 115BS, a novel cardiotonic agent. Circ Res. 1982 Sep;51(3):290–294. doi: 10.1161/01.res.51.3.290. [DOI] [PubMed] [Google Scholar]
- Spurgeon H. A., Stern M. D., Baartz G., Raffaeli S., Hansford R. G., Talo A., Lakatta E. G., Capogrossi M. C. Simultaneous measurement of Ca2+, contraction, and potential in cardiac myocytes. Am J Physiol. 1990 Feb;258(2 Pt 2):H574–H586. doi: 10.1152/ajpheart.1990.258.2.H574. [DOI] [PubMed] [Google Scholar]
- Stern M. D., Silverman H. S., Houser S. R., Josephson R. A., Capogrossi M. C., Nichols C. G., Lederer W. J., Lakatta E. G. Anoxic contractile failure in rat heart myocytes is caused by failure of intracellular calcium release due to alteration of the action potential. Proc Natl Acad Sci U S A. 1988 Sep;85(18):6954–6958. doi: 10.1073/pnas.85.18.6954. [DOI] [PMC free article] [PubMed] [Google Scholar]
- Wier W. G., Hess P. Excitation-contraction coupling in cardiac Purkinje fibers. Effects of cardiotonic steroids on the intracellular [Ca2+] transient, membrane potential, and contraction. J Gen Physiol. 1984 Mar;83(3):395–415. doi: 10.1085/jgp.83.3.395. [DOI] [PMC free article] [PubMed] [Google Scholar]
- Yue D. T. Intracellular [Ca2+] related to rate of force development in twitch contraction of heart. Am J Physiol. 1987 Apr;252(4 Pt 2):H760–H770. doi: 10.1152/ajpheart.1987.252.4.H760. [DOI] [PubMed] [Google Scholar]
- Yue D. T., Marban E., Wier W. G. Relationship between force and intracellular [Ca2+] in tetanized mammalian heart muscle. J Gen Physiol. 1986 Feb;87(2):223–242. doi: 10.1085/jgp.87.2.223. [DOI] [PMC free article] [PubMed] [Google Scholar]
- duBell W. H., Boyett M. R., Spurgeon H. A., Talo A., Stern M. D., Lakatta E. G. The cytosolic calcium transient modulates the action potential of rat ventricular myocytes. J Physiol. 1991 May;436:347–369. doi: 10.1113/jphysiol.1991.sp018554. [DOI] [PMC free article] [PubMed] [Google Scholar]