Abstract
1. Electrophysiologically identified thalamocortical neurones have been intra- and extracellularly recorded in acutely prepared cats, under different anaesthetic conditions. 2. A slow (0.5-4 Hz) membrane potential oscillation was observed in thalamocortical cells recorded in motor, sensory, associational and intralaminar thalamic nuclei. The oscillation consisted of rhythmic low-threshold spikes alternating with after-hyperpolarizations. 3. About 80% of the neurones with intact cortical connections were set into the slow oscillatory mode by bringing their membrane potential to between -68 and -90 mV. The oscillation did not depend upon the occurrence of fast action potentials and did not outlast the imposed hyperpolarization. 4. Anatomical or functional disconnection from related cortical areas resulted in a membrane potential hyperpolarization of about 9 mV and in the occurrence of spontaneous slow oscillations in virtually all recorded neurones. The intrinsic nature of the phenomenon was supported by the lack of rhythmic postsynaptic potentials as the cells were prevented from oscillating by outward current injection. 5. In contrast with other thalamic nuclei, the slow oscillation has not been observed in anterior thalamic neurones despite their having similar basic electrophysiological properties. 6. Barbiturate administration suppressed the slow oscillatory mode, an effect accompanied by a decrease in the membrane input resistance. 7. Multiunit recordings of spontaneously oscillating cells showed epochs characterized by phase-related firing. This synchronous discharge was paralleled by a clear-cut build-up of field potentials in the frequency range of electroencephalogram slow or delta waves. 8. These results demonstrate that the majority of thalamocortical neurones are endowed with electrophysiological properties allowing them to oscillate at 0.5-4 Hz, if they have a membrane potential more negative than -65 mV and a high input resistance. Such a condition is physiologically achieved in the deepest stages of electroencephalogram-synchronized sleep, as a result of brain stem-thalamic as well as cortico-thalamic deafferentation. We postulate a thalamic contribution in the genesis of electroencephalogram delta waves during slow wave sleep, once independently oscillating thalamocortical cells become in phase.
Full text
PDF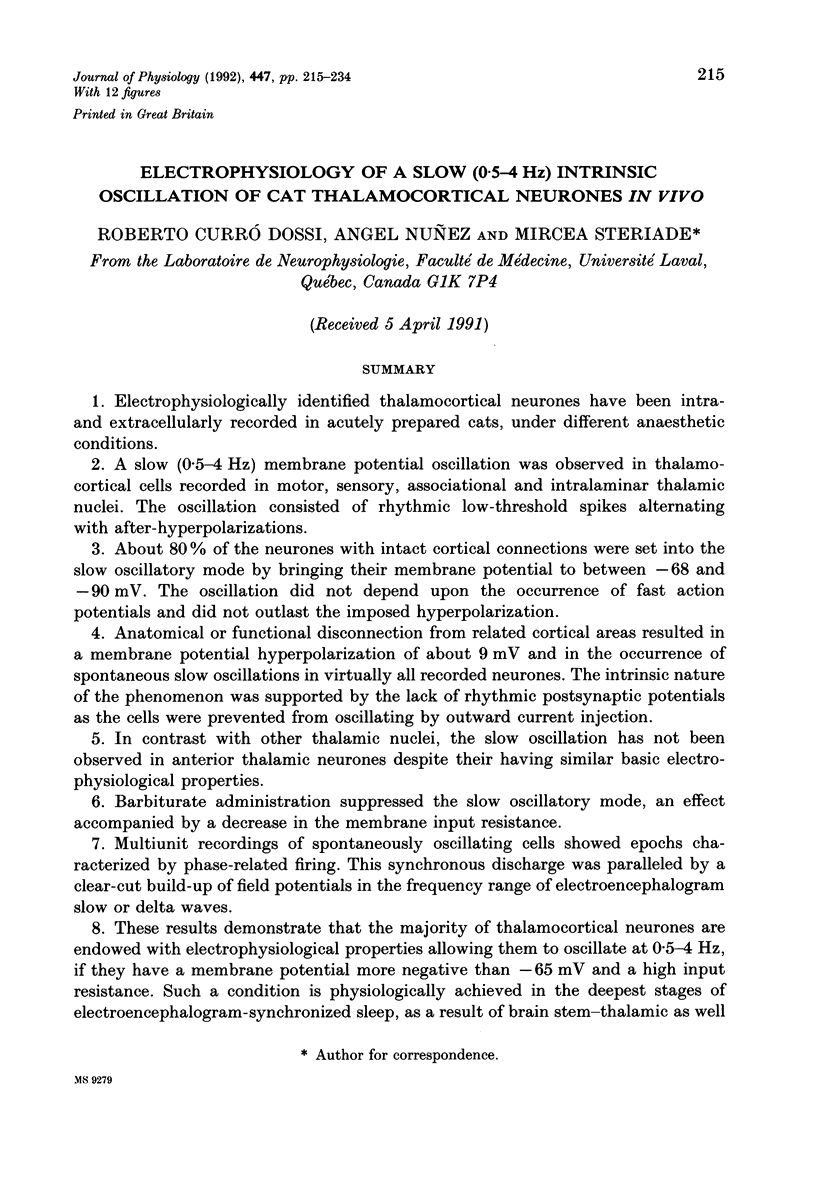
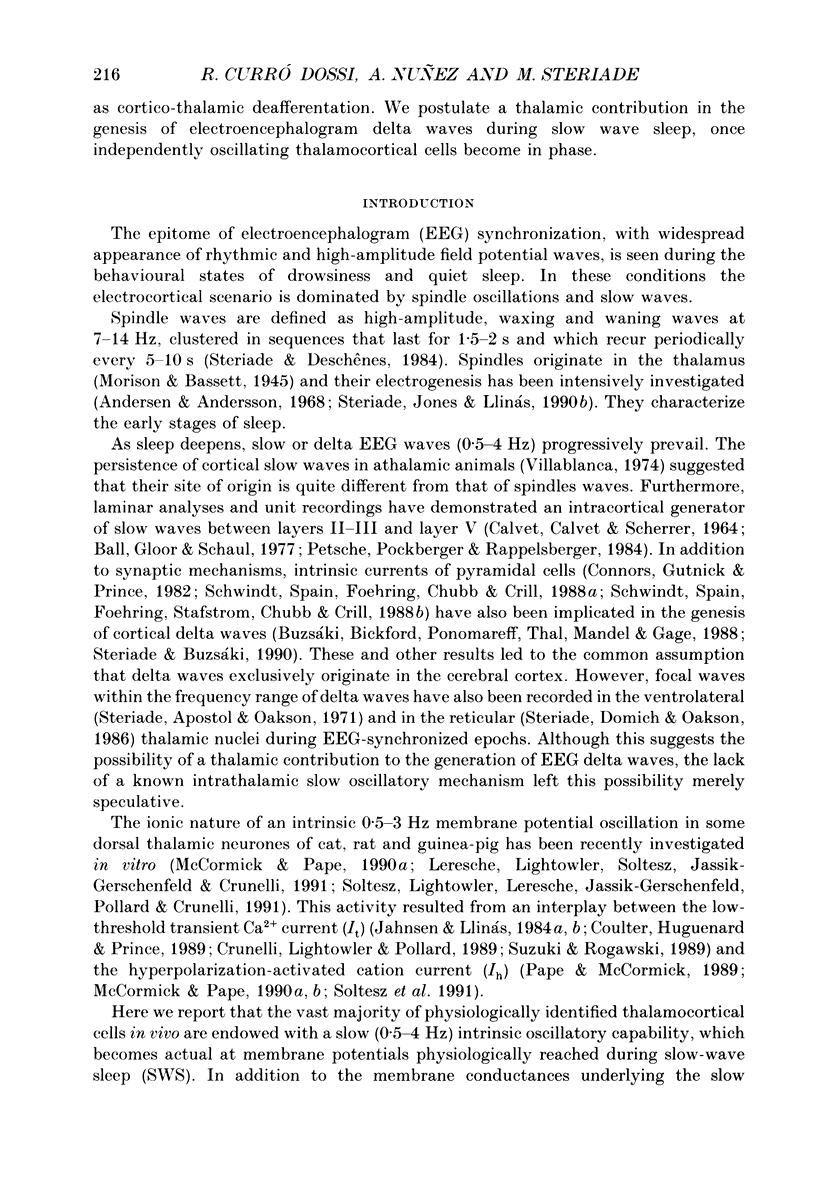
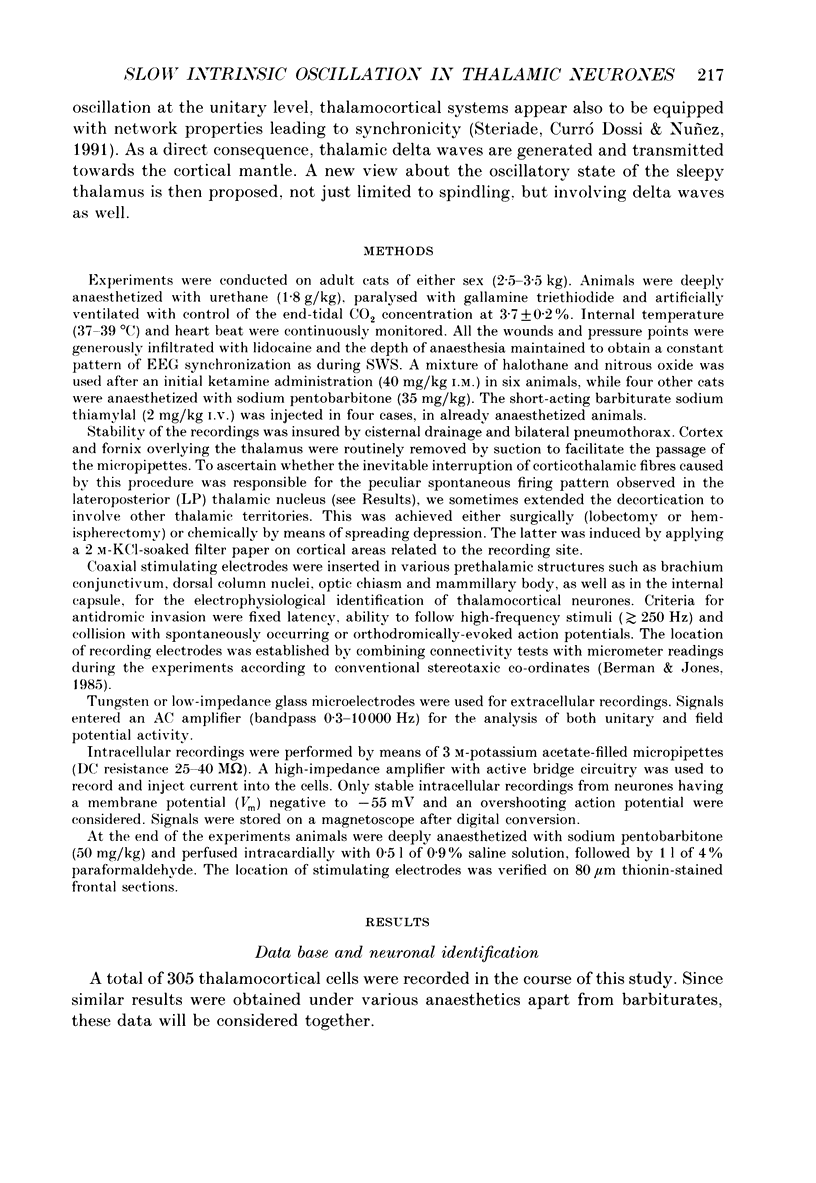
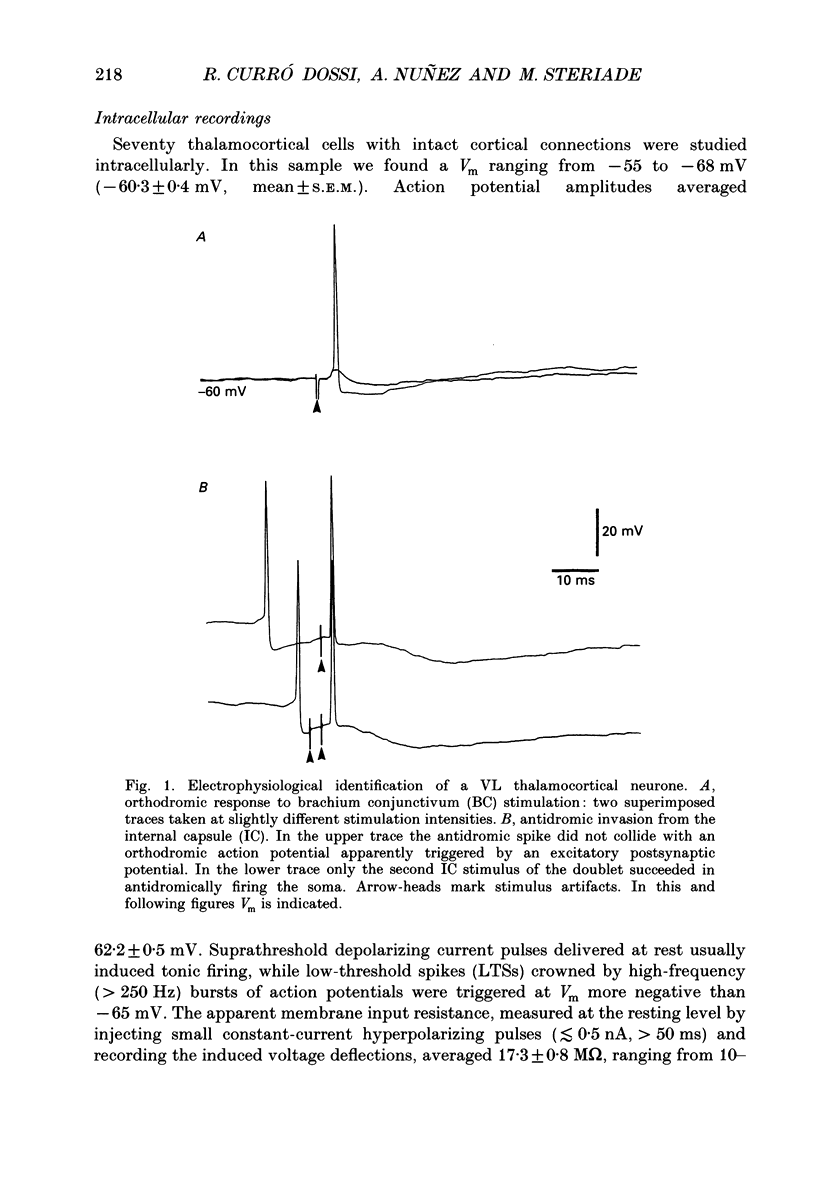
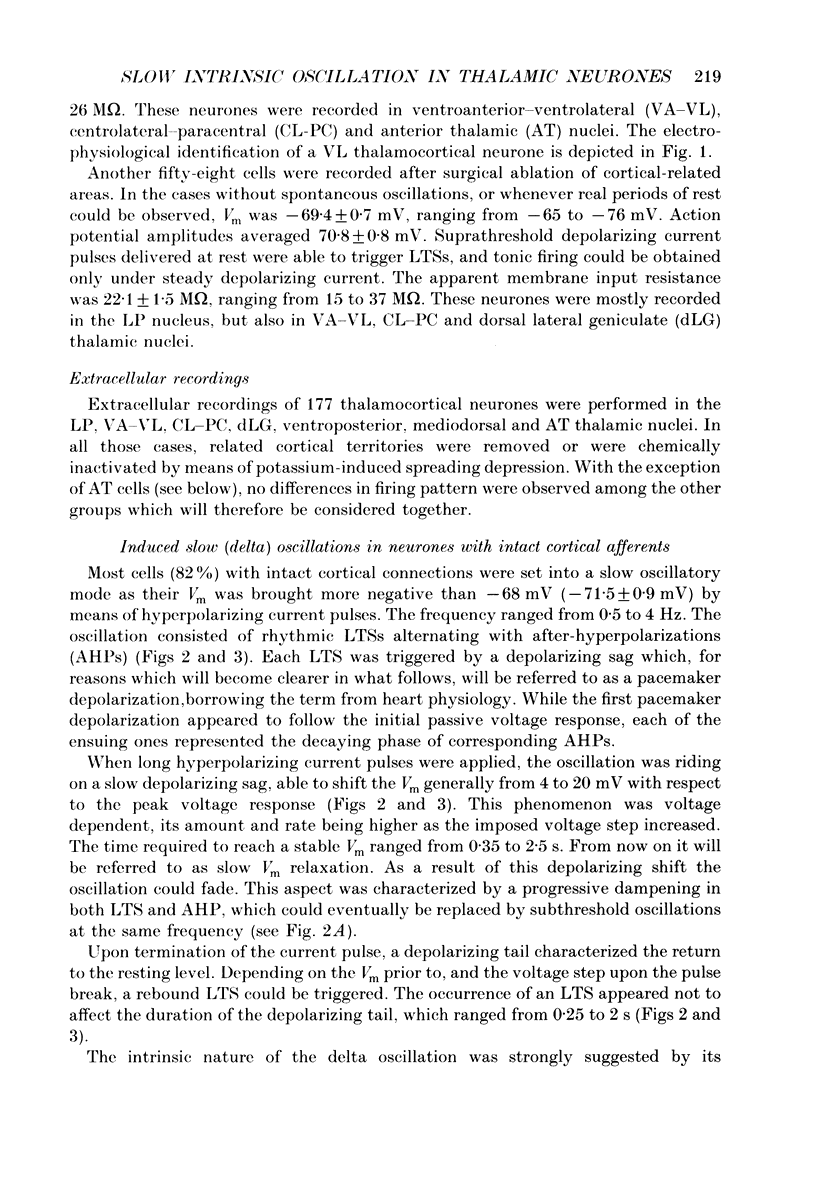
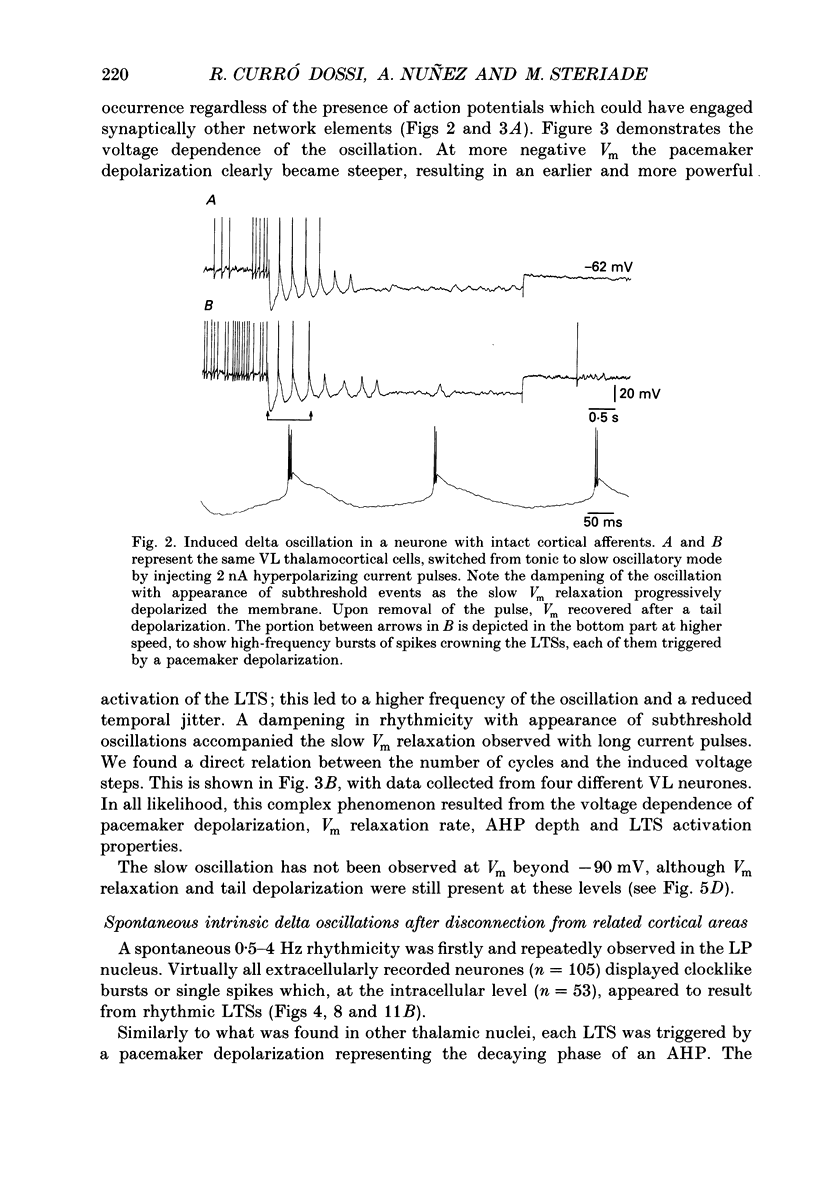
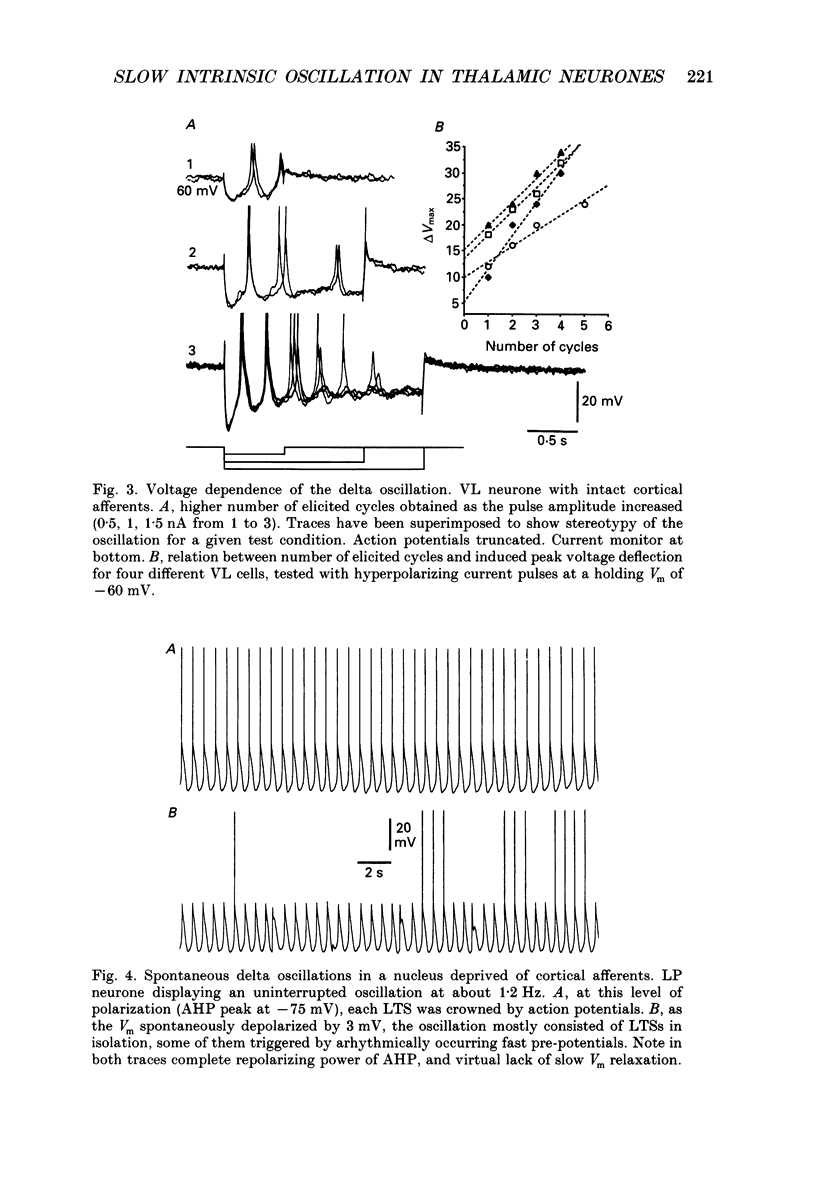
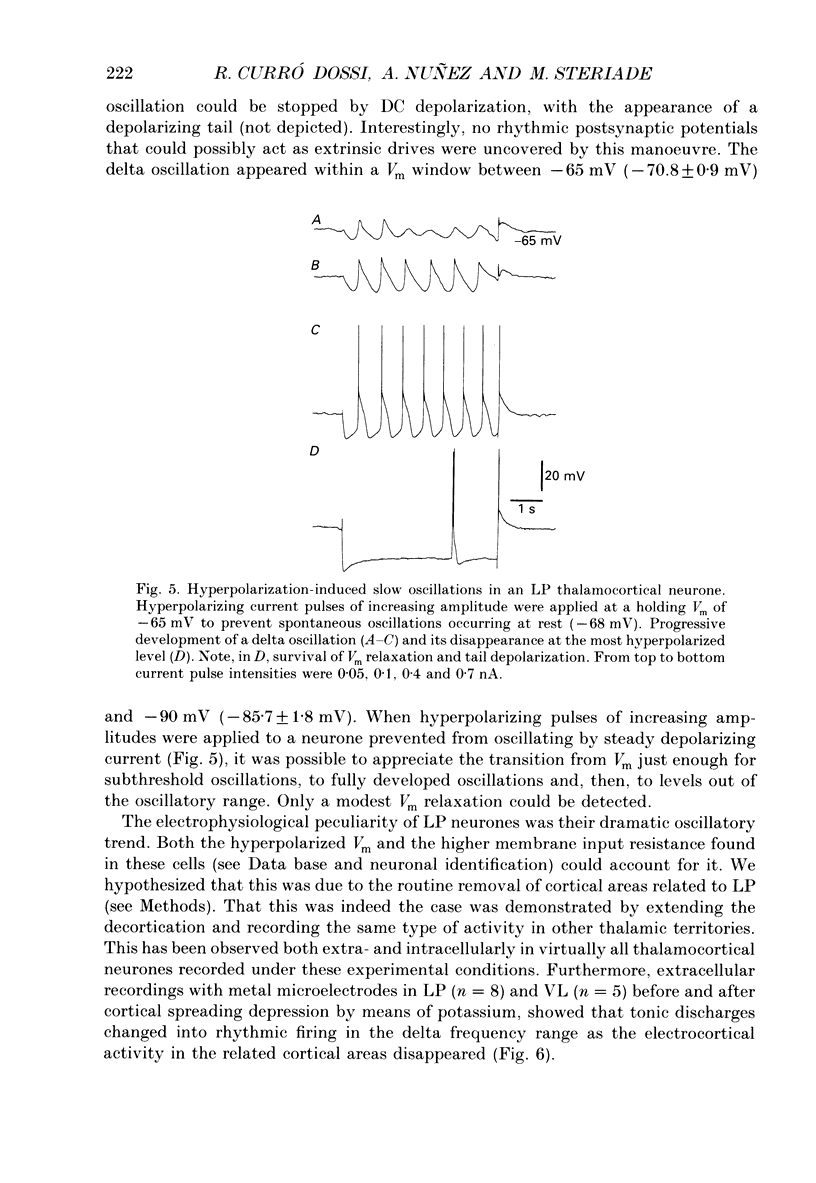
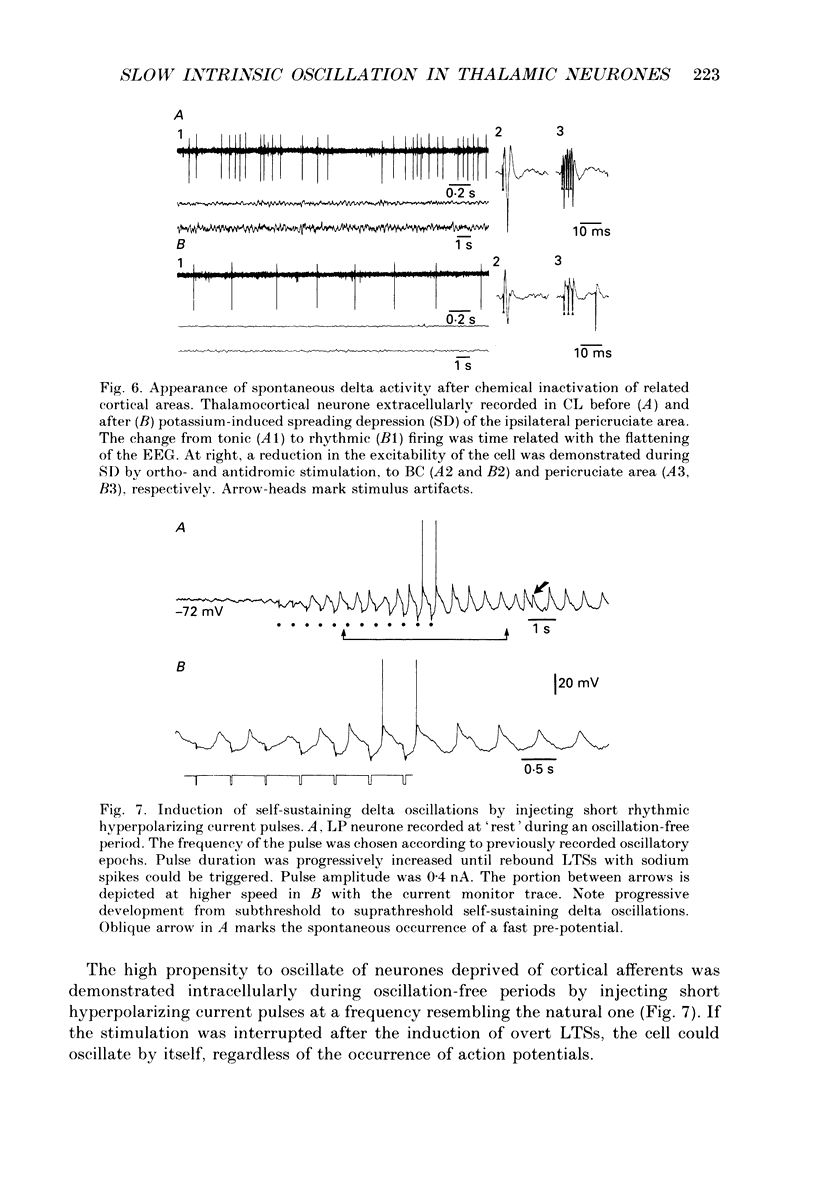
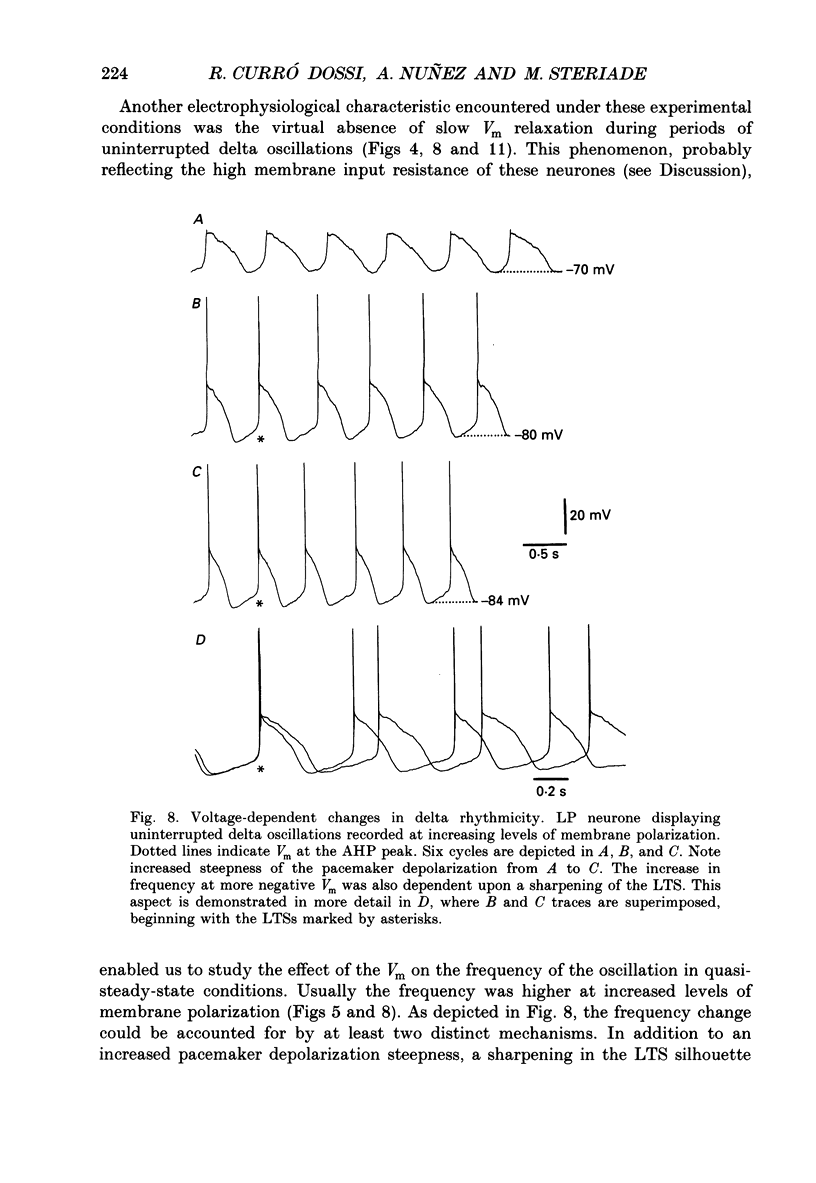
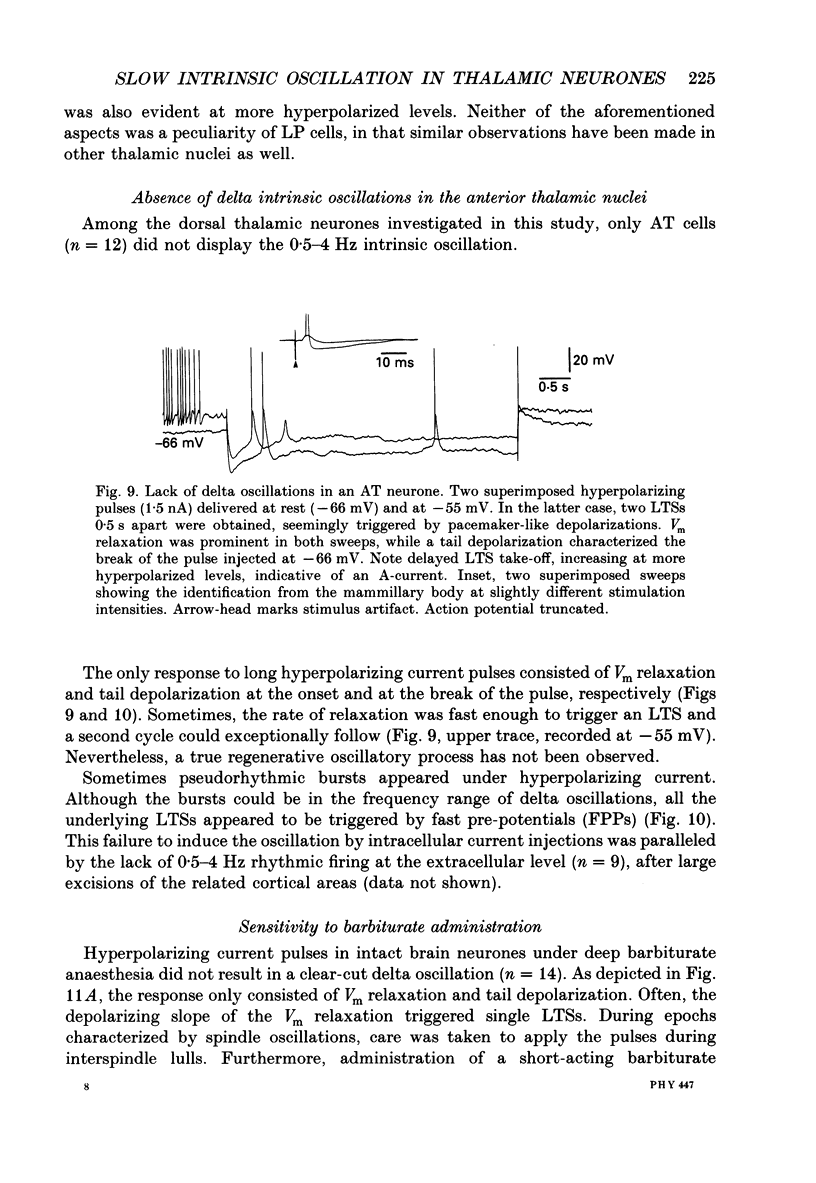
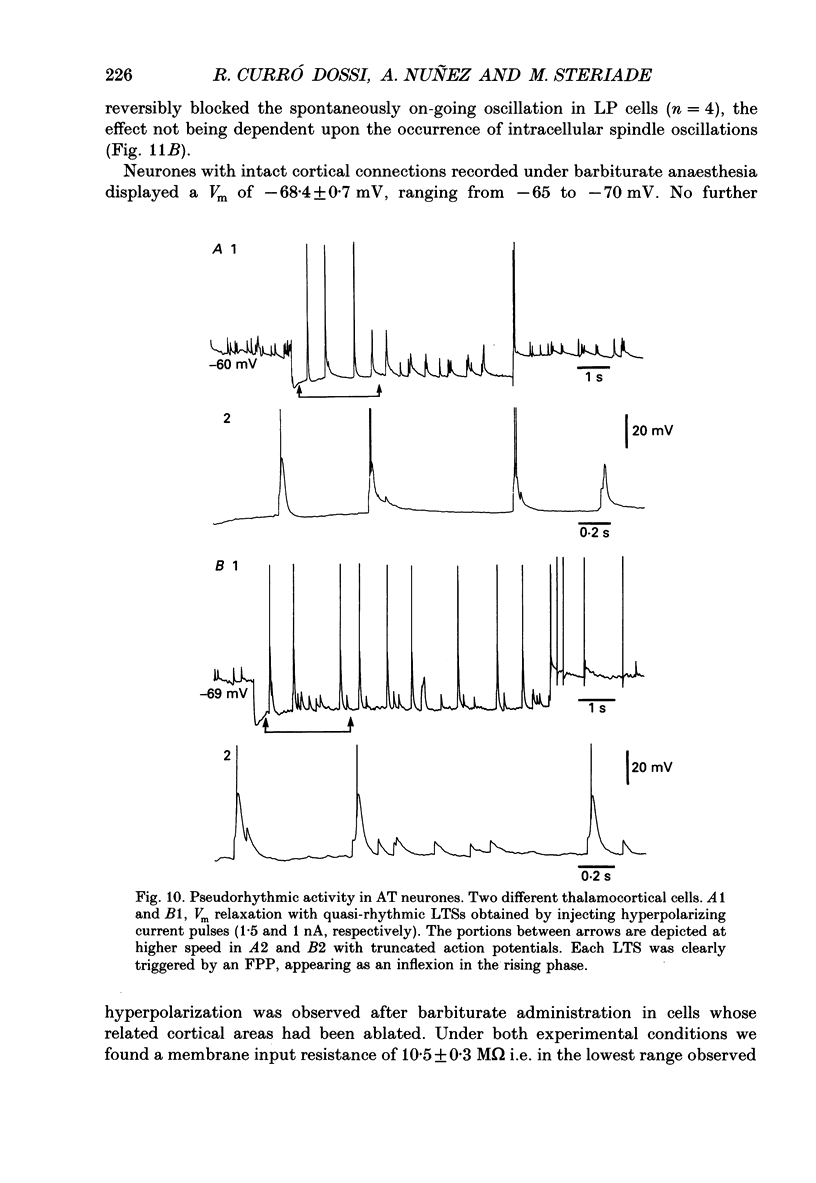
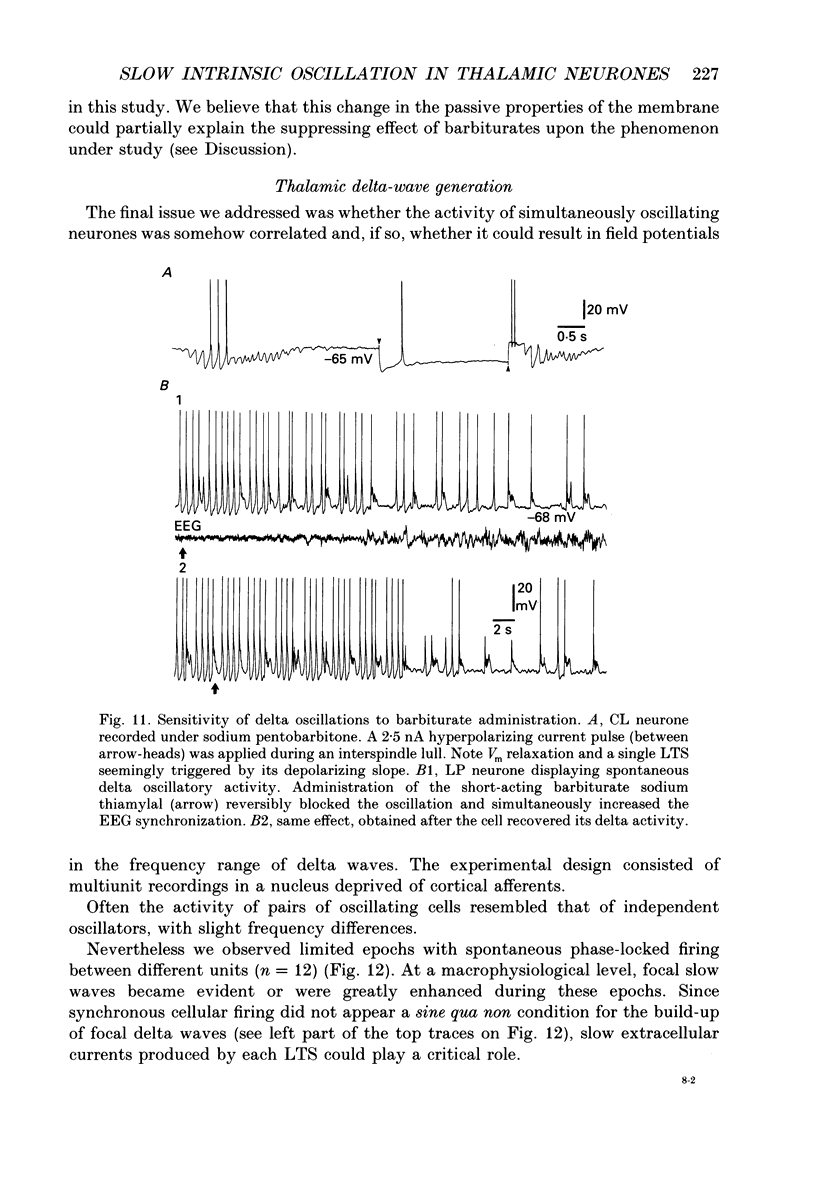
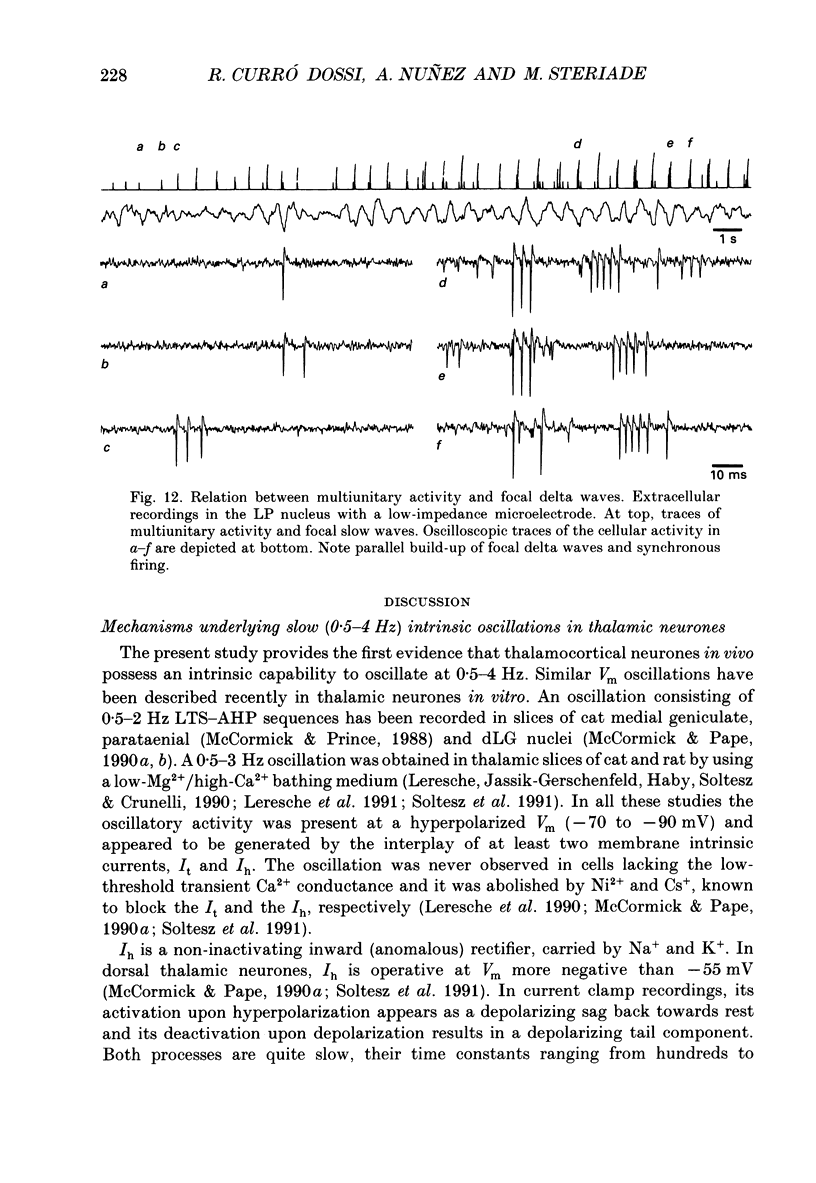
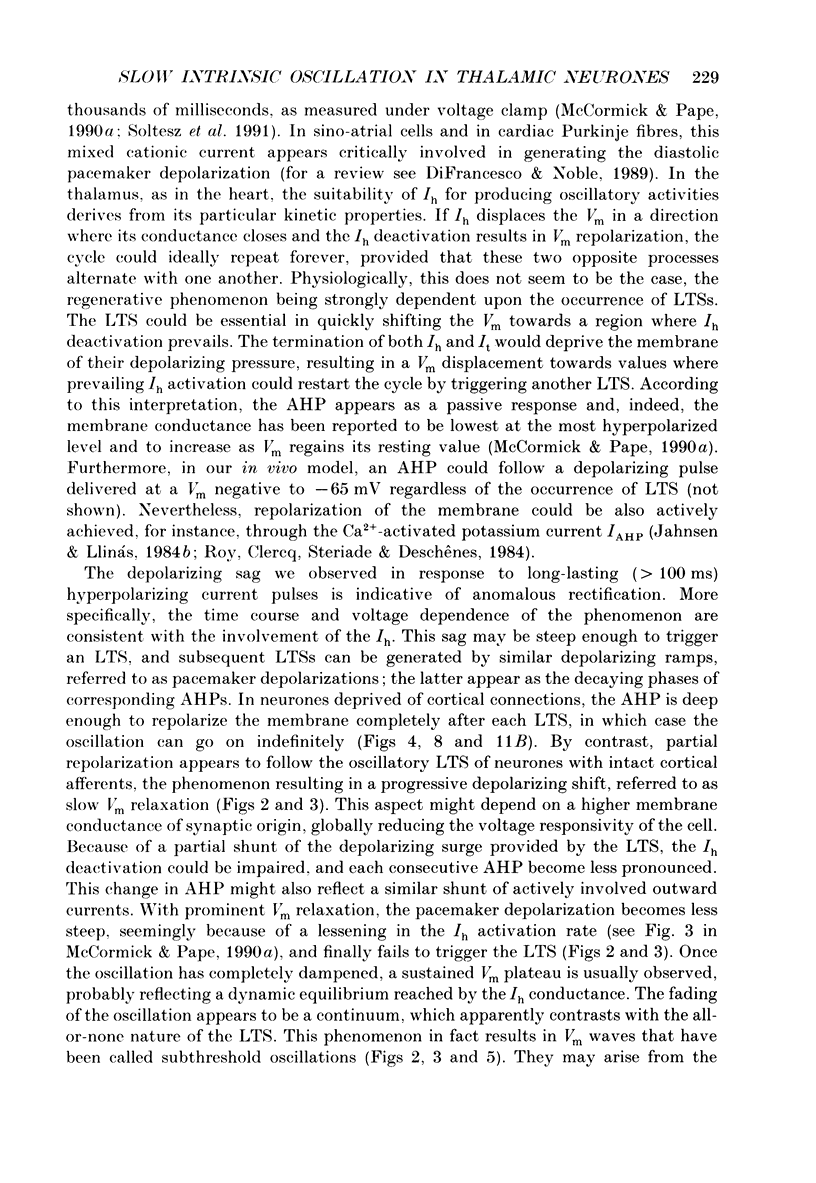
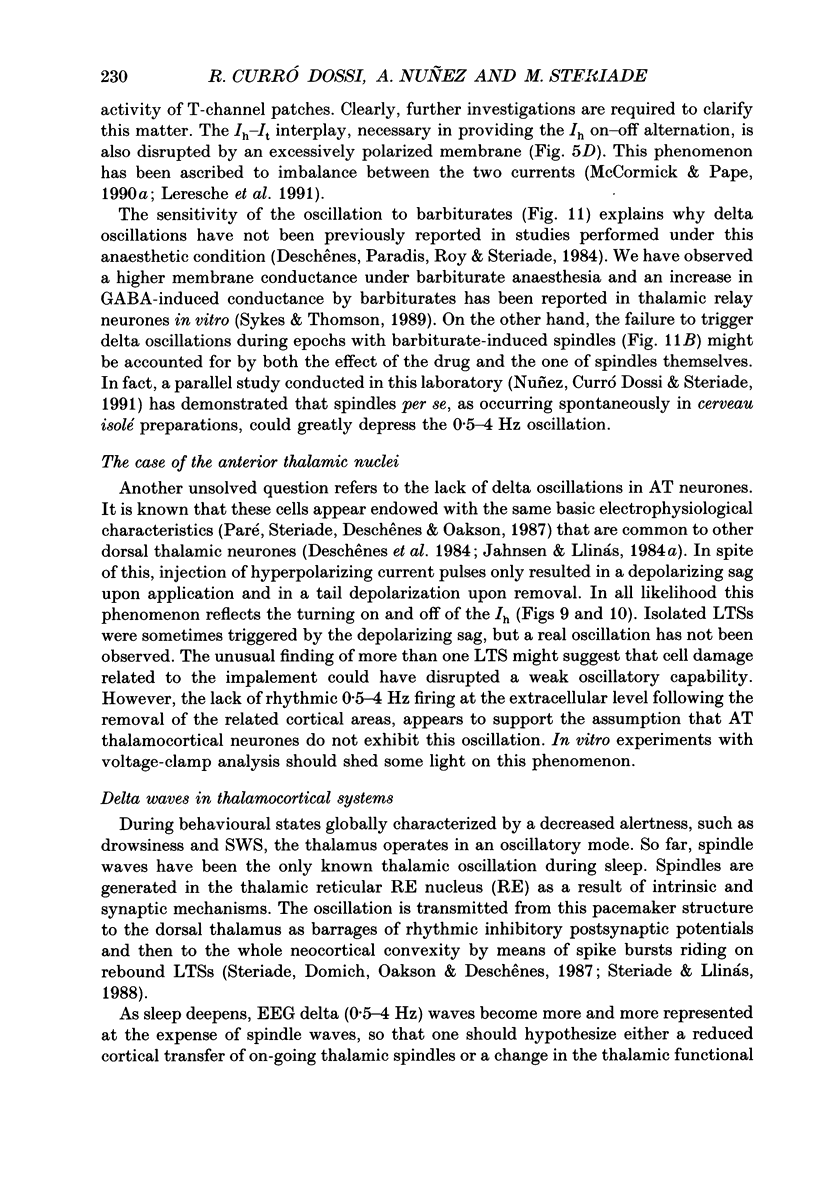
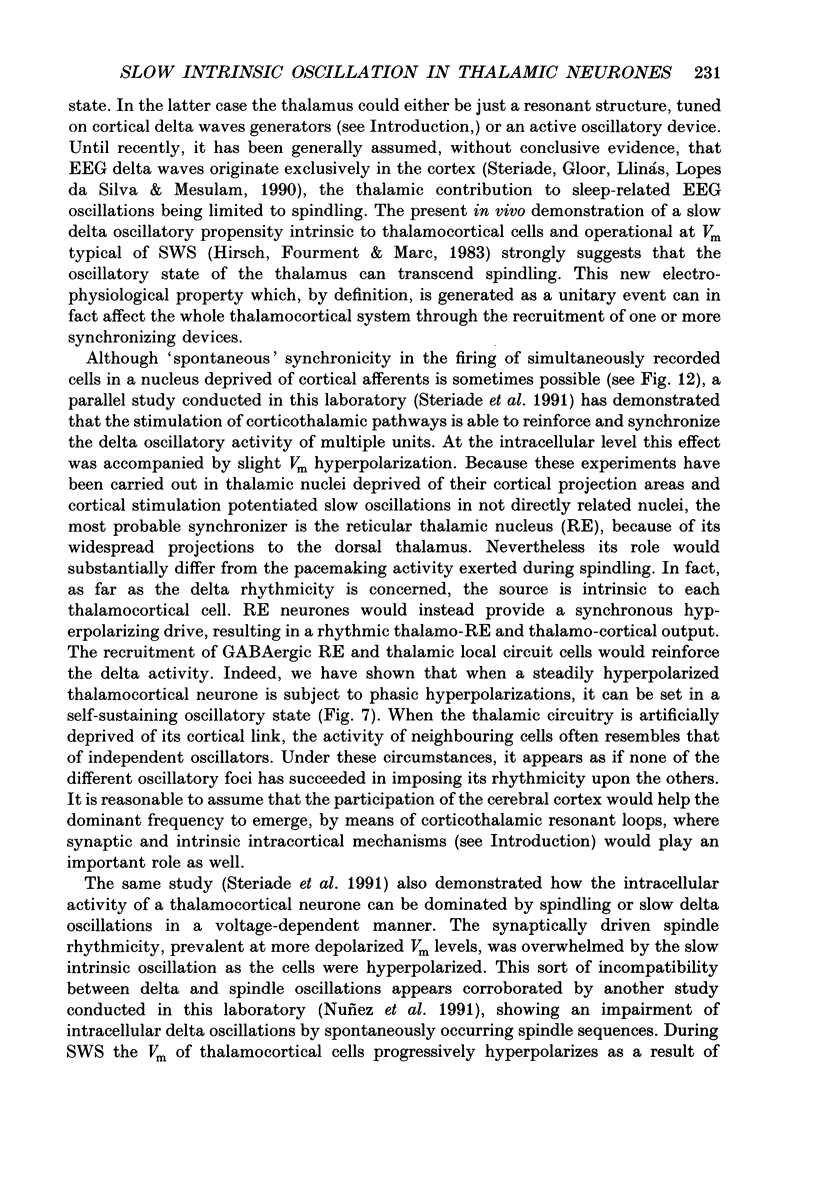
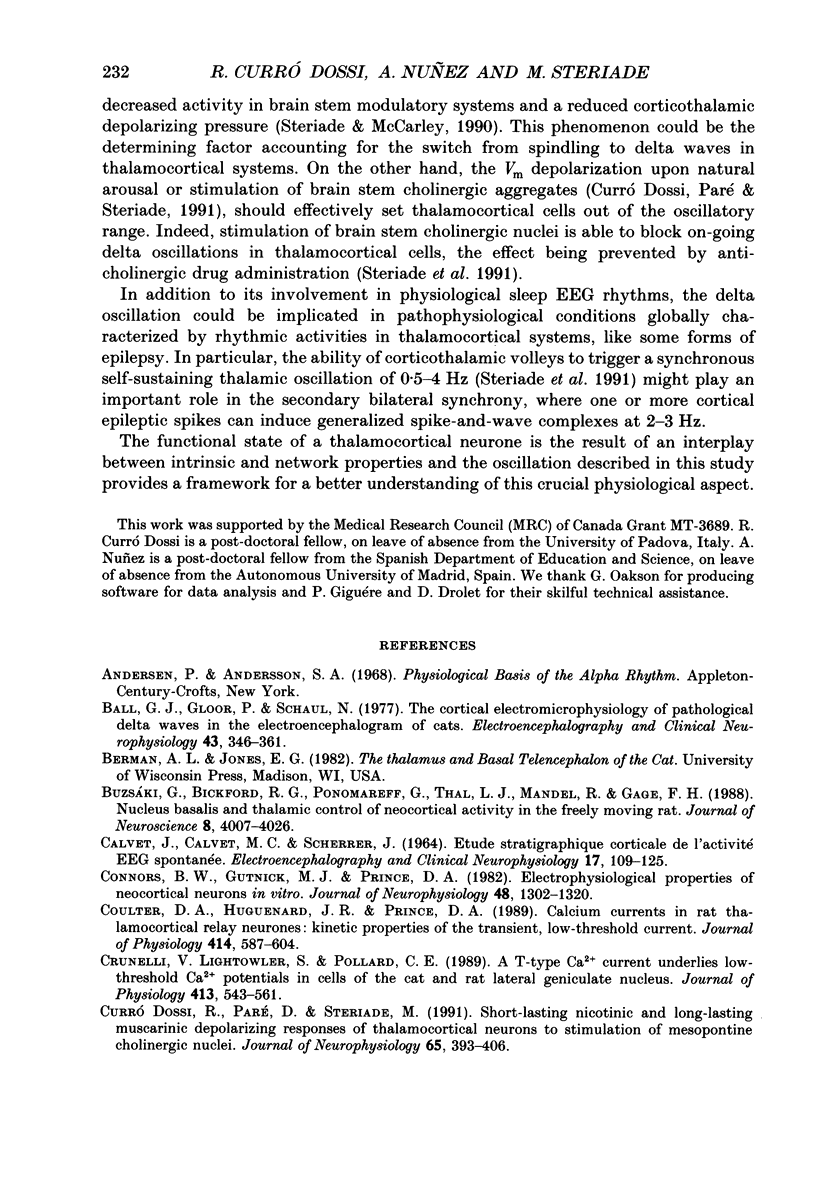
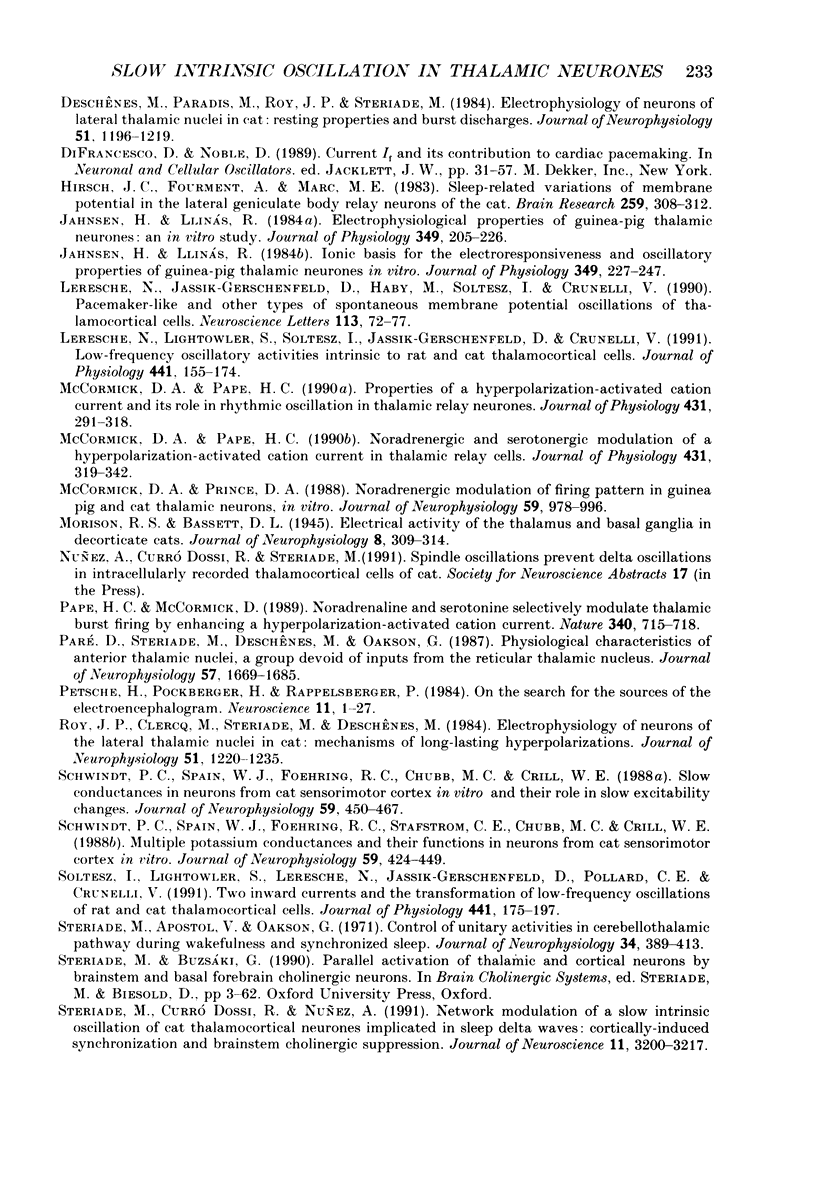
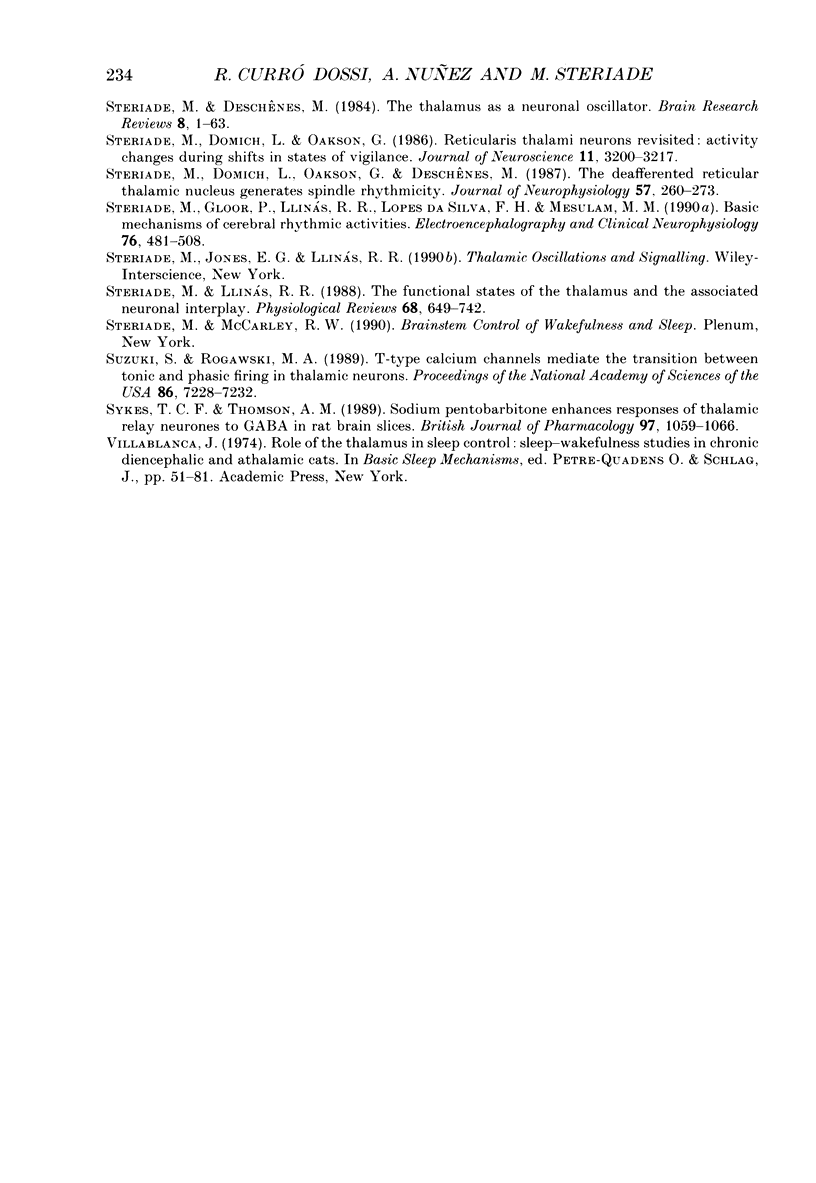
Selected References
These references are in PubMed. This may not be the complete list of references from this article.
- Ball G. J., Gloor P., Schaul N. The cortical electromicrophysiology of pathological delta waves in the electroencephalogram of cats. Electroencephalogr Clin Neurophysiol. 1977 Sep;43(3):346–361. doi: 10.1016/0013-4694(77)90258-9. [DOI] [PubMed] [Google Scholar]
- Buzsaki G., Bickford R. G., Ponomareff G., Thal L. J., Mandel R., Gage F. H. Nucleus basalis and thalamic control of neocortical activity in the freely moving rat. J Neurosci. 1988 Nov;8(11):4007–4026. doi: 10.1523/JNEUROSCI.08-11-04007.1988. [DOI] [PMC free article] [PubMed] [Google Scholar]
- CALVET J., CALVET M. C., SCHERRER J. ETUDE STRATIGRAPHIQUE CORTICALE DE L'ACTIVIT'E EEG SPONTAN'EE. Electroencephalogr Clin Neurophysiol. 1964 Aug;17:109–125. doi: 10.1016/0013-4694(64)90143-9. [DOI] [PubMed] [Google Scholar]
- Connors B. W., Gutnick M. J., Prince D. A. Electrophysiological properties of neocortical neurons in vitro. J Neurophysiol. 1982 Dec;48(6):1302–1320. doi: 10.1152/jn.1982.48.6.1302. [DOI] [PubMed] [Google Scholar]
- Coulter D. A., Huguenard J. R., Prince D. A. Calcium currents in rat thalamocortical relay neurones: kinetic properties of the transient, low-threshold current. J Physiol. 1989 Jul;414:587–604. doi: 10.1113/jphysiol.1989.sp017705. [DOI] [PMC free article] [PubMed] [Google Scholar]
- Crunelli V., Lightowler S., Pollard C. E. A T-type Ca2+ current underlies low-threshold Ca2+ potentials in cells of the cat and rat lateral geniculate nucleus. J Physiol. 1989 Jun;413:543–561. doi: 10.1113/jphysiol.1989.sp017668. [DOI] [PMC free article] [PubMed] [Google Scholar]
- Curró Dossi R., Paré D., Steriade M. Short-lasting nicotinic and long-lasting muscarinic depolarizing responses of thalamocortical neurons to stimulation of mesopontine cholinergic nuclei. J Neurophysiol. 1991 Mar;65(3):393–406. doi: 10.1152/jn.1991.65.3.393. [DOI] [PubMed] [Google Scholar]
- Deschênes M., Paradis M., Roy J. P., Steriade M. Electrophysiology of neurons of lateral thalamic nuclei in cat: resting properties and burst discharges. J Neurophysiol. 1984 Jun;51(6):1196–1219. doi: 10.1152/jn.1984.51.6.1196. [DOI] [PubMed] [Google Scholar]
- Hirsch J. C., Fourment A., Marc M. E. Sleep-related variations of membrane potential in the lateral geniculate body relay neurons of the cat. Brain Res. 1983 Jan 24;259(2):308–312. doi: 10.1016/0006-8993(83)91264-7. [DOI] [PubMed] [Google Scholar]
- Jahnsen H., Llinás R. Electrophysiological properties of guinea-pig thalamic neurones: an in vitro study. J Physiol. 1984 Apr;349:205–226. doi: 10.1113/jphysiol.1984.sp015153. [DOI] [PMC free article] [PubMed] [Google Scholar]
- Jahnsen H., Llinás R. Ionic basis for the electro-responsiveness and oscillatory properties of guinea-pig thalamic neurones in vitro. J Physiol. 1984 Apr;349:227–247. doi: 10.1113/jphysiol.1984.sp015154. [DOI] [PMC free article] [PubMed] [Google Scholar]
- Leresche N., Jassik-Gerschenfeld D., Haby M., Soltesz I., Crunelli V. Pacemaker-like and other types of spontaneous membrane potential oscillations of thalamocortical cells. Neurosci Lett. 1990 May 18;113(1):72–77. doi: 10.1016/0304-3940(90)90497-w. [DOI] [PubMed] [Google Scholar]
- Leresche N., Lightowler S., Soltesz I., Jassik-Gerschenfeld D., Crunelli V. Low-frequency oscillatory activities intrinsic to rat and cat thalamocortical cells. J Physiol. 1991 Sep;441:155–174. doi: 10.1113/jphysiol.1991.sp018744. [DOI] [PMC free article] [PubMed] [Google Scholar]
- McCormick D. A., Pape H. C. Noradrenergic and serotonergic modulation of a hyperpolarization-activated cation current in thalamic relay neurones. J Physiol. 1990 Dec;431:319–342. doi: 10.1113/jphysiol.1990.sp018332. [DOI] [PMC free article] [PubMed] [Google Scholar]
- McCormick D. A., Pape H. C. Properties of a hyperpolarization-activated cation current and its role in rhythmic oscillation in thalamic relay neurones. J Physiol. 1990 Dec;431:291–318. doi: 10.1113/jphysiol.1990.sp018331. [DOI] [PMC free article] [PubMed] [Google Scholar]
- McCormick D. A., Prince D. A. Noradrenergic modulation of firing pattern in guinea pig and cat thalamic neurons, in vitro. J Neurophysiol. 1988 Mar;59(3):978–996. doi: 10.1152/jn.1988.59.3.978. [DOI] [PubMed] [Google Scholar]
- Pape H. C., McCormick D. A. Noradrenaline and serotonin selectively modulate thalamic burst firing by enhancing a hyperpolarization-activated cation current. Nature. 1989 Aug 31;340(6236):715–718. doi: 10.1038/340715a0. [DOI] [PubMed] [Google Scholar]
- Paré D., Steriade M., Deschênes M., Oakson G. Physiological characteristics of anterior thalamic nuclei, a group devoid of inputs from reticular thalamic nucleus. J Neurophysiol. 1987 Jun;57(6):1669–1685. doi: 10.1152/jn.1987.57.6.1669. [DOI] [PubMed] [Google Scholar]
- Petsche H., Pockberger H., Rappelsberger P. On the search for the sources of the electroencephalogram. Neuroscience. 1984 Jan;11(1):1–27. doi: 10.1016/0306-4522(84)90212-4. [DOI] [PubMed] [Google Scholar]
- Roy J. P., Clercq M., Steriade M., Deschênes M. Electrophysiology of neurons of lateral thalamic nuclei in cat: mechanisms of long-lasting hyperpolarizations. J Neurophysiol. 1984 Jun;51(6):1220–1235. doi: 10.1152/jn.1984.51.6.1220. [DOI] [PubMed] [Google Scholar]
- Schwindt P. C., Spain W. J., Foehring R. C., Chubb M. C., Crill W. E. Slow conductances in neurons from cat sensorimotor cortex in vitro and their role in slow excitability changes. J Neurophysiol. 1988 Feb;59(2):450–467. doi: 10.1152/jn.1988.59.2.450. [DOI] [PubMed] [Google Scholar]
- Schwindt P. C., Spain W. J., Foehring R. C., Stafstrom C. E., Chubb M. C., Crill W. E. Multiple potassium conductances and their functions in neurons from cat sensorimotor cortex in vitro. J Neurophysiol. 1988 Feb;59(2):424–449. doi: 10.1152/jn.1988.59.2.424. [DOI] [PubMed] [Google Scholar]
- Soltesz I., Lightowler S., Leresche N., Jassik-Gerschenfeld D., Pollard C. E., Crunelli V. Two inward currents and the transformation of low-frequency oscillations of rat and cat thalamocortical cells. J Physiol. 1991 Sep;441:175–197. doi: 10.1113/jphysiol.1991.sp018745. [DOI] [PMC free article] [PubMed] [Google Scholar]
- Steriade M., Apostol V., Oakson G. Control of unitary activities in cerebellothalamic pathway during wakefulness and synchronized sleep. J Neurophysiol. 1971 May;34(3):389–413. doi: 10.1152/jn.1971.34.3.389. [DOI] [PubMed] [Google Scholar]
- Steriade M., Deschenes M. The thalamus as a neuronal oscillator. Brain Res. 1984 Nov;320(1):1–63. doi: 10.1016/0165-0173(84)90017-1. [DOI] [PubMed] [Google Scholar]
- Steriade M., Domich L., Oakson G., Deschênes M. The deafferented reticular thalamic nucleus generates spindle rhythmicity. J Neurophysiol. 1987 Jan;57(1):260–273. doi: 10.1152/jn.1987.57.1.260. [DOI] [PubMed] [Google Scholar]
- Steriade M., Dossi R. C., Nuñez A. Network modulation of a slow intrinsic oscillation of cat thalamocortical neurons implicated in sleep delta waves: cortically induced synchronization and brainstem cholinergic suppression. J Neurosci. 1991 Oct;11(10):3200–3217. doi: 10.1523/JNEUROSCI.11-10-03200.1991. [DOI] [PMC free article] [PubMed] [Google Scholar]
- Steriade M., Gloor P., Llinás R. R., Lopes de Silva F. H., Mesulam M. M. Report of IFCN Committee on Basic Mechanisms. Basic mechanisms of cerebral rhythmic activities. Electroencephalogr Clin Neurophysiol. 1990 Dec;76(6):481–508. doi: 10.1016/0013-4694(90)90001-z. [DOI] [PubMed] [Google Scholar]
- Steriade M., Llinás R. R. The functional states of the thalamus and the associated neuronal interplay. Physiol Rev. 1988 Jul;68(3):649–742. doi: 10.1152/physrev.1988.68.3.649. [DOI] [PubMed] [Google Scholar]
- Suzuki S., Rogawski M. A. T-type calcium channels mediate the transition between tonic and phasic firing in thalamic neurons. Proc Natl Acad Sci U S A. 1989 Sep;86(18):7228–7232. doi: 10.1073/pnas.86.18.7228. [DOI] [PMC free article] [PubMed] [Google Scholar]
- Sykes T. C., Thomson A. M. Sodium pentobarbitone enhances responses of thalamic relay neurones to GABA in rat brain slices. Br J Pharmacol. 1989 Aug;97(4):1059–1066. doi: 10.1111/j.1476-5381.1989.tb12562.x. [DOI] [PMC free article] [PubMed] [Google Scholar]