Abstract
1. The performance of skeletal muscle during repetitive stimulation may be limited by the development of an intracellular acidosis due to lactic acid accumulation. To study this, we have measured the intracellular pH (pHi) with the fluorescent indicator BCECF (2',7'-bis(carboxyethyl)-5(6)- carboxyfluorescein) during fatigue produced by repeated, short tetani in intact, single fibres isolated from the mouse flexor brevis muscle. 2. The pHi at rest was 7.33 +/- 0.02 (mean +/- S.E.M., n = 29, 22 degrees C). During fatiguing stimulation pHi initially went alkaline by about 0.03 units (maximum alkalinization after about ten tetani). Thereafter pHi declined slowly and at the end of fatiguing stimulation (tetanic tension reduced to 30% of the original; 0.3Po), pHi was only 0.063 +/- 0.011 units (n = 14) more acid than in control. 3. We considered three possible causes of acidosis being so small in fatigue: (i) a high oxidative capacity so that fatigue occurs without marked production of lactic acid; (ii) an effective transport of H+ or H+ equivalents out of the fibres; a high intracellular buffer power. 4. The oxidative metabolism was inhibited by 2 mM-cyanide in three fibres. After being exposed to cyanide for 5 min without stimulation, the tetanic tension was reduced to about 0.9 Po and pHi was alkaline by about 0.1 units. The fibres fatigued faster in cyanide and the pHi decline in fatigue was more than twice as large as that under control conditions. 5. Inhibition of Na(+)-H+ exchange with amiloride resulted in a slow acidification of rested fibres; resting pHi was not affected by either inhibition of HCO3(-)-Cl- exchange with DIDS (4,4'-diisothiocyanatostilbene-2,2'-disulphonic acid) or inhibition of the lactate transporter with cinnamate. 6. Fibres fatigued in cinnamate displayed a markedly larger acidification (approximately 0.4 pH units) and tension fell more rapidly than under control conditions; inhibition of Na(+)-H+ and HCO3(-)-Cl- exchange did not have any significant effect on fatigue. 7. The intracellular buffer power, assessed by exposing fibres to the weak base trimethylamine, was about 15 mM (pH unit)-1 in a HEPES-buffered solution (non-CO2 or intrinsic buffer power) and about 33 mM (pH unit)-1 in a bicarbonate-buffered solution. Somewhat higher values of the intrinsic buffer power was obtained from changes of the partial pressure of CO2 (PCO2) of the bath solution. Application of lactate or butyrate frequently gave an infinite buffer power, which indicates that powerful pH-regulating mechanisms operate in these cases.(ABSTRACT TRUNCATED AT 400 WORDS)
Full text
PDF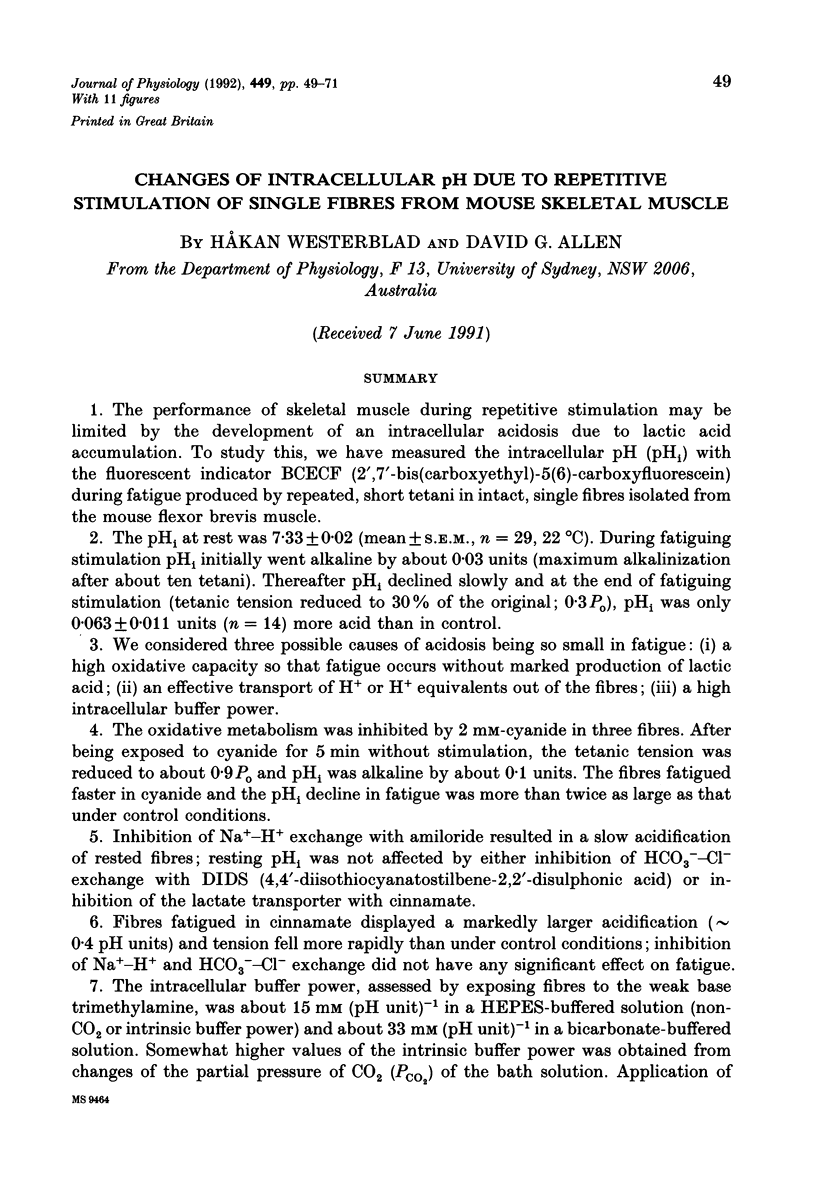
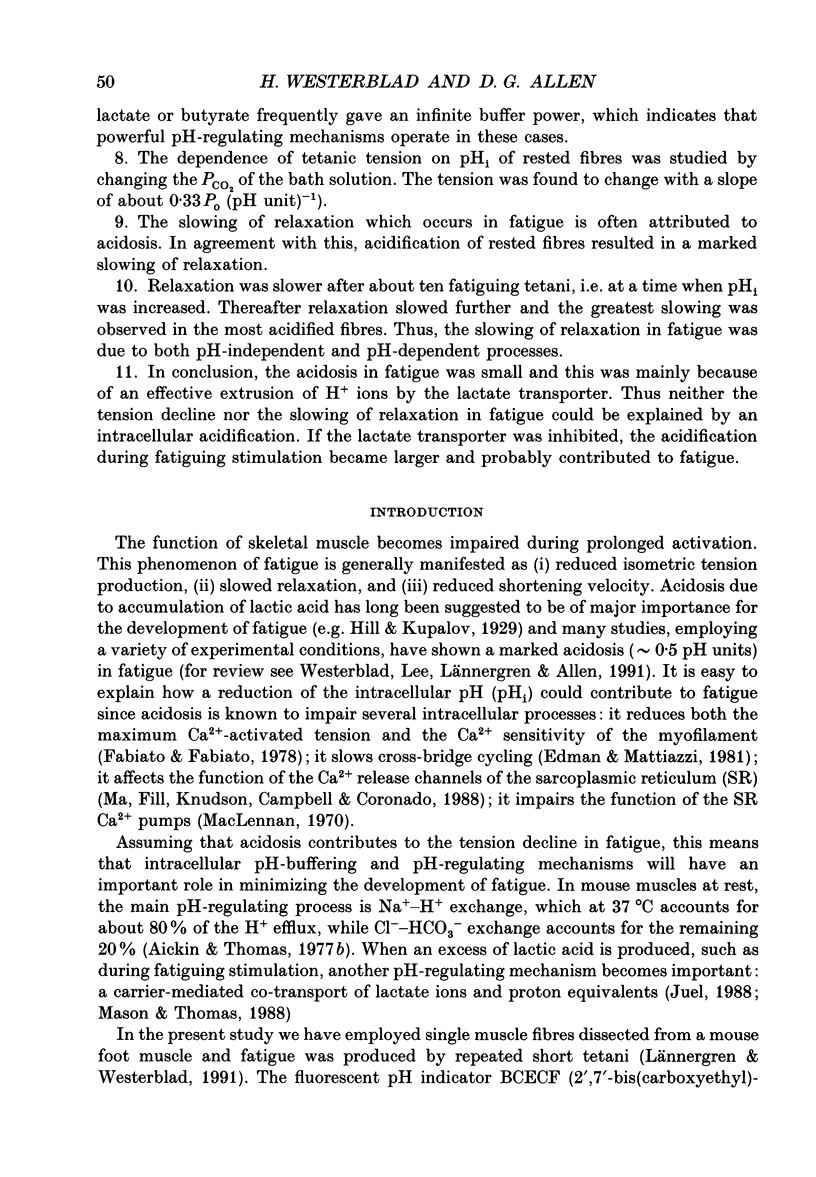
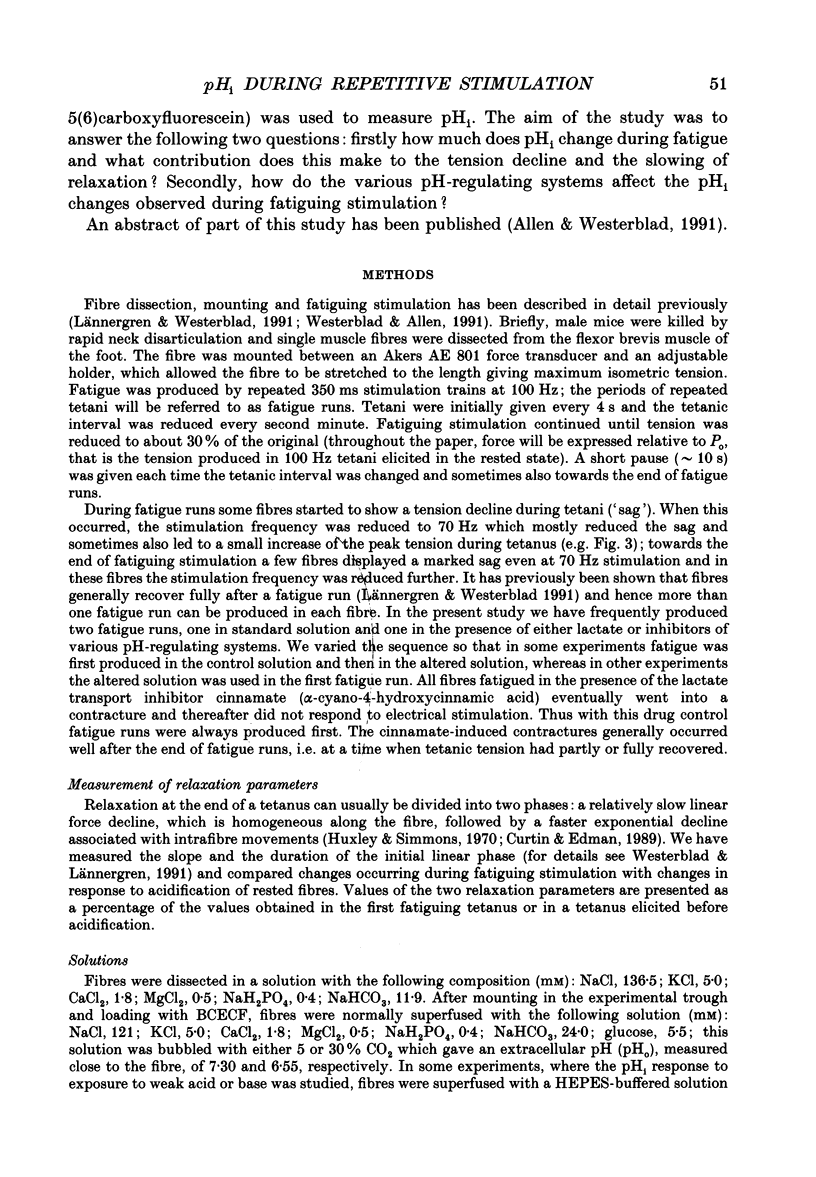
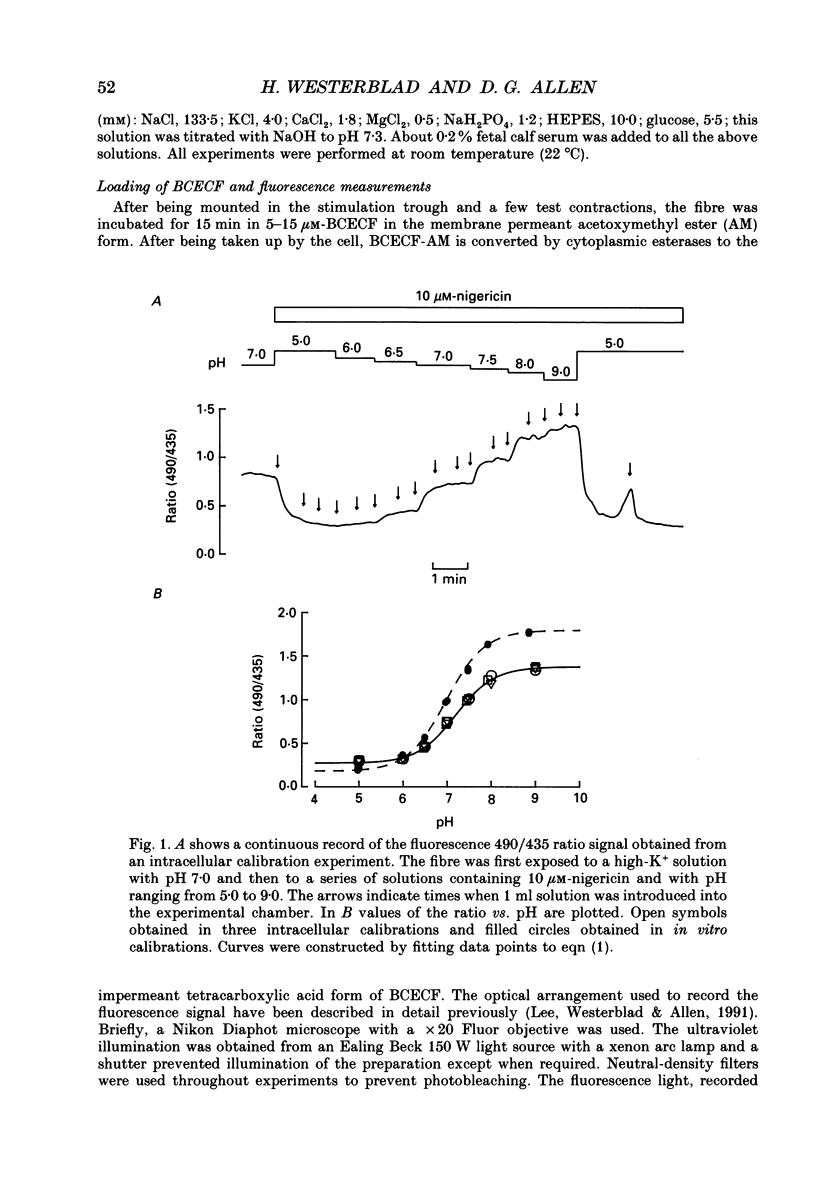
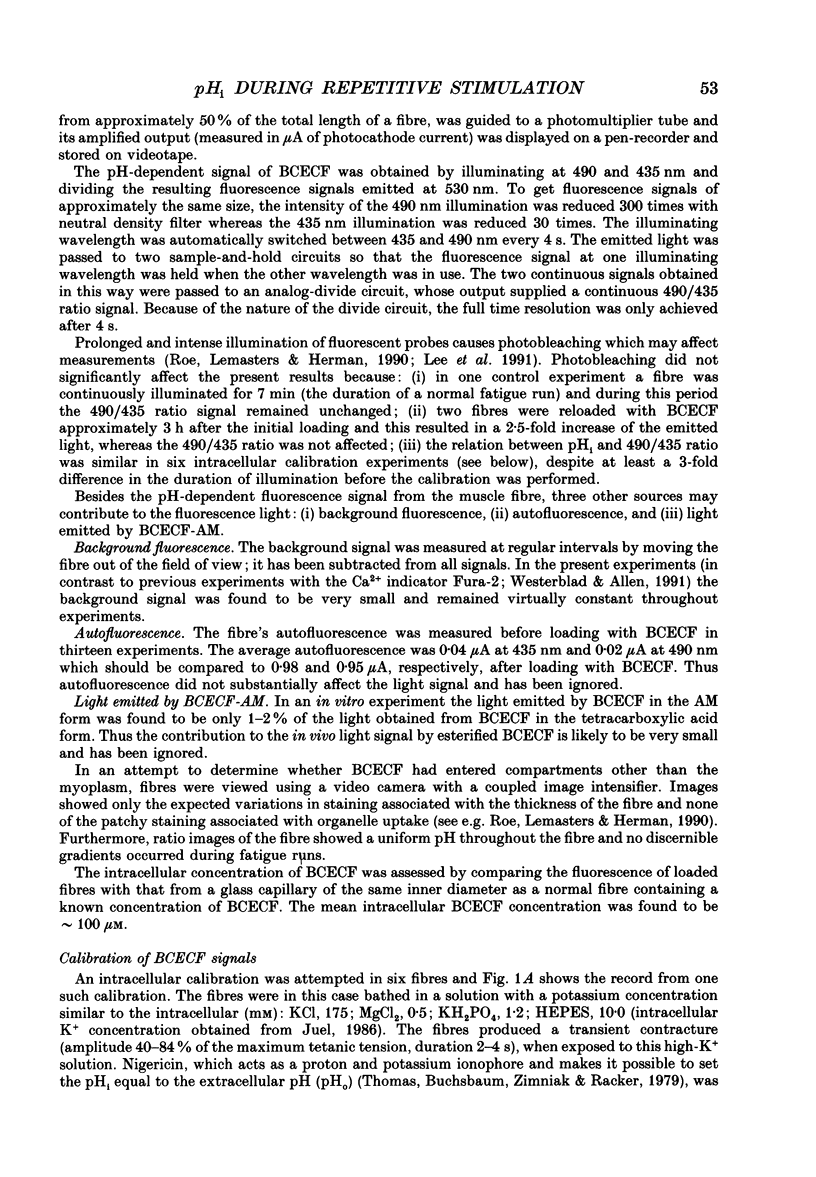
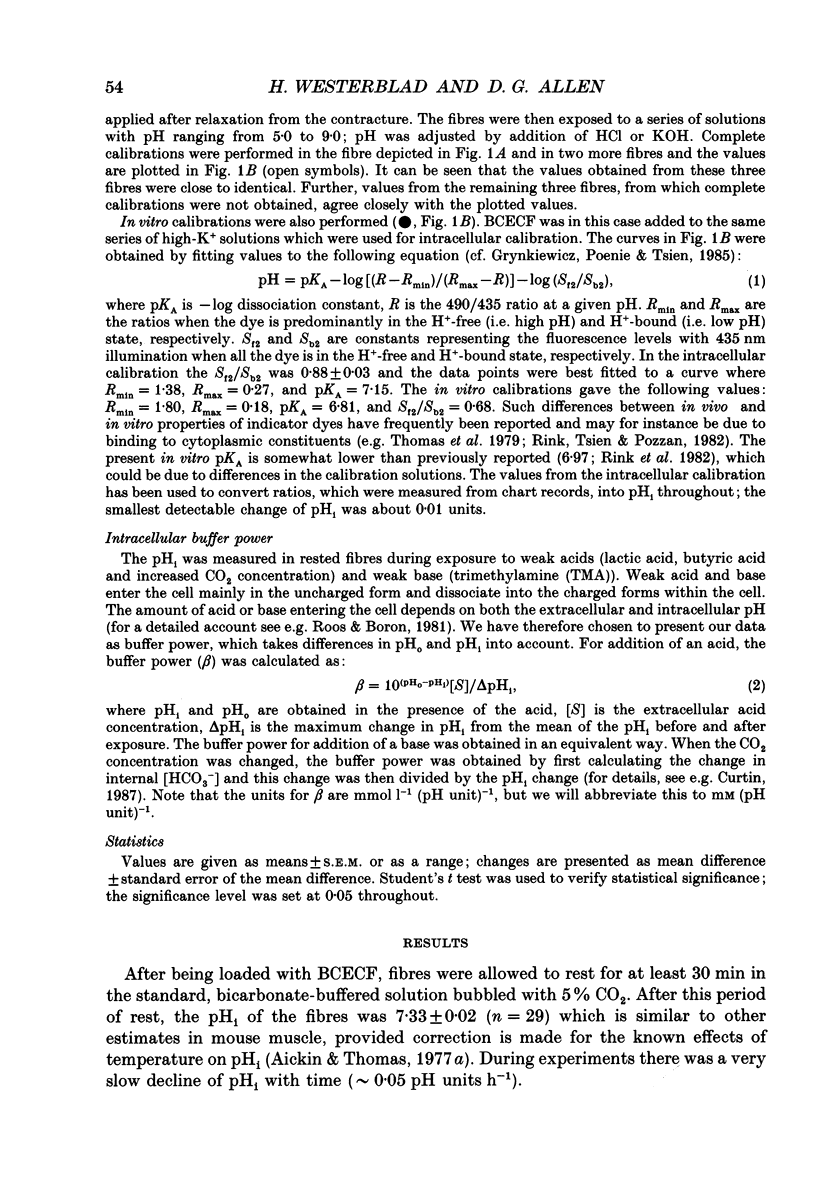
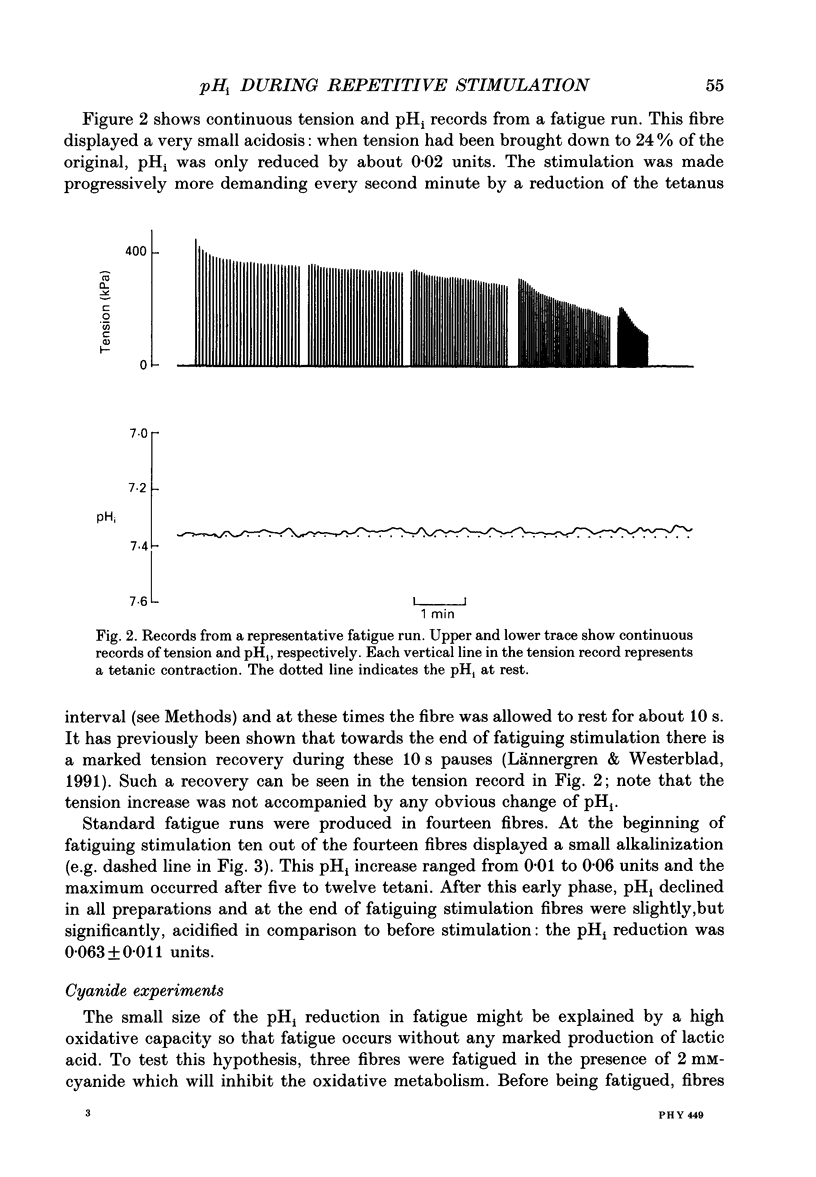
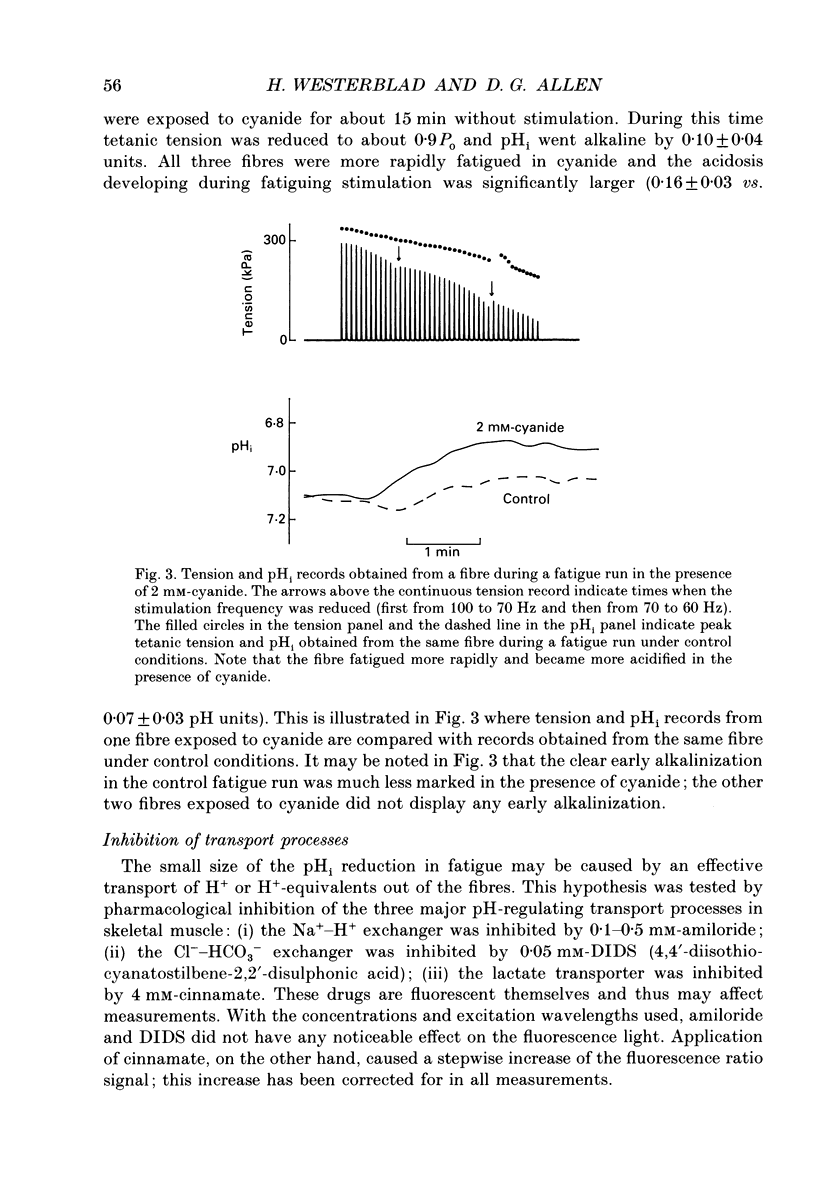
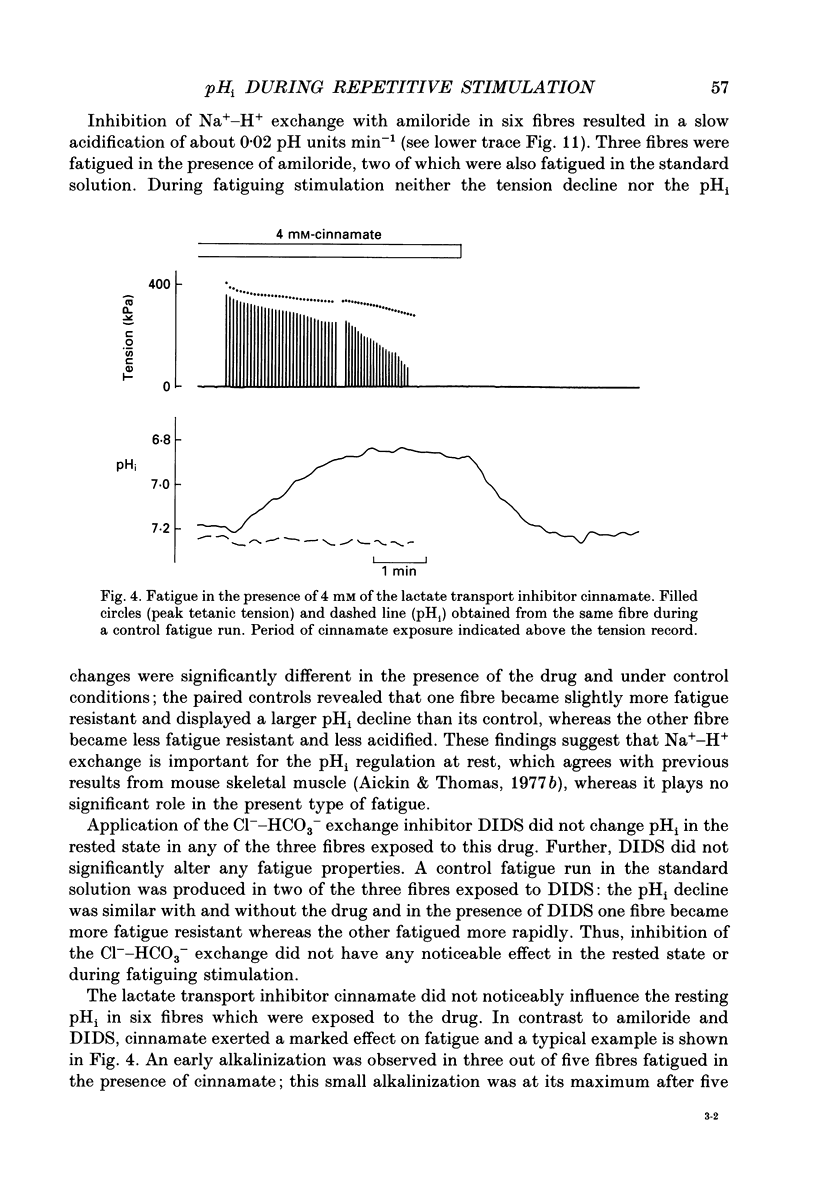
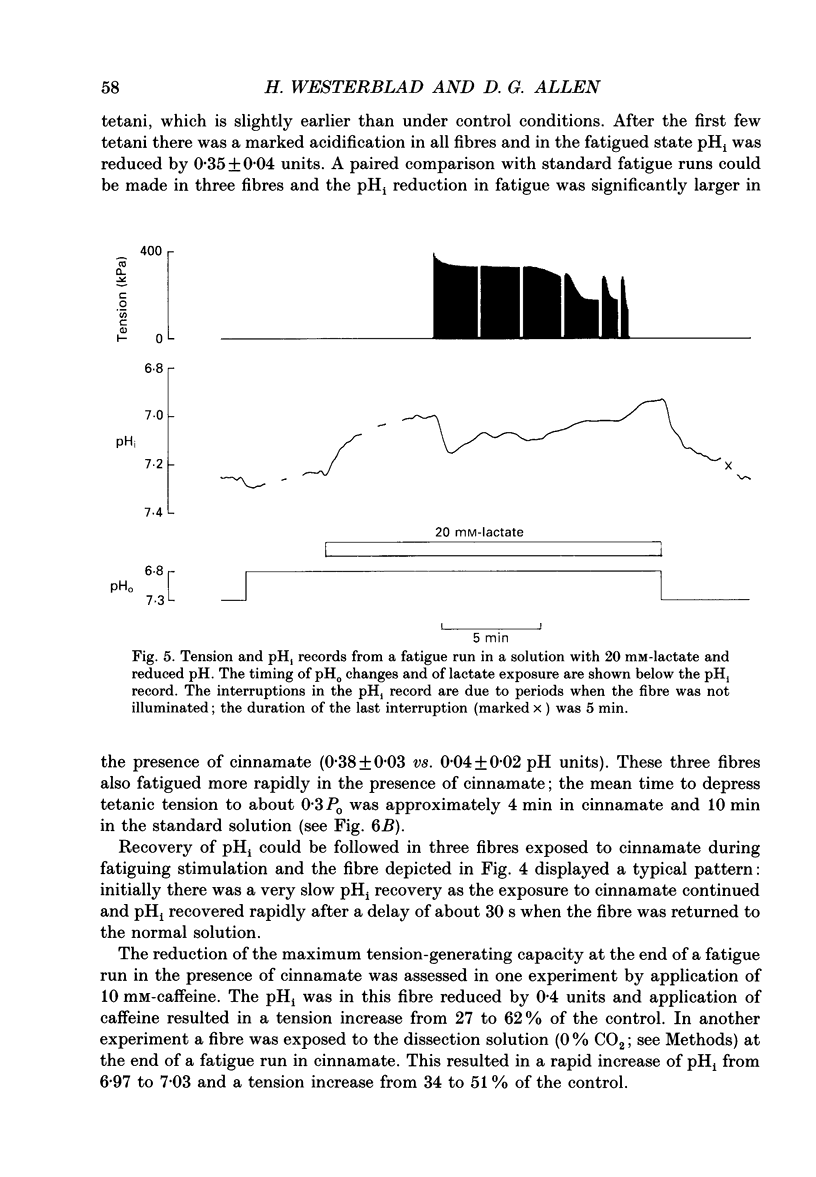
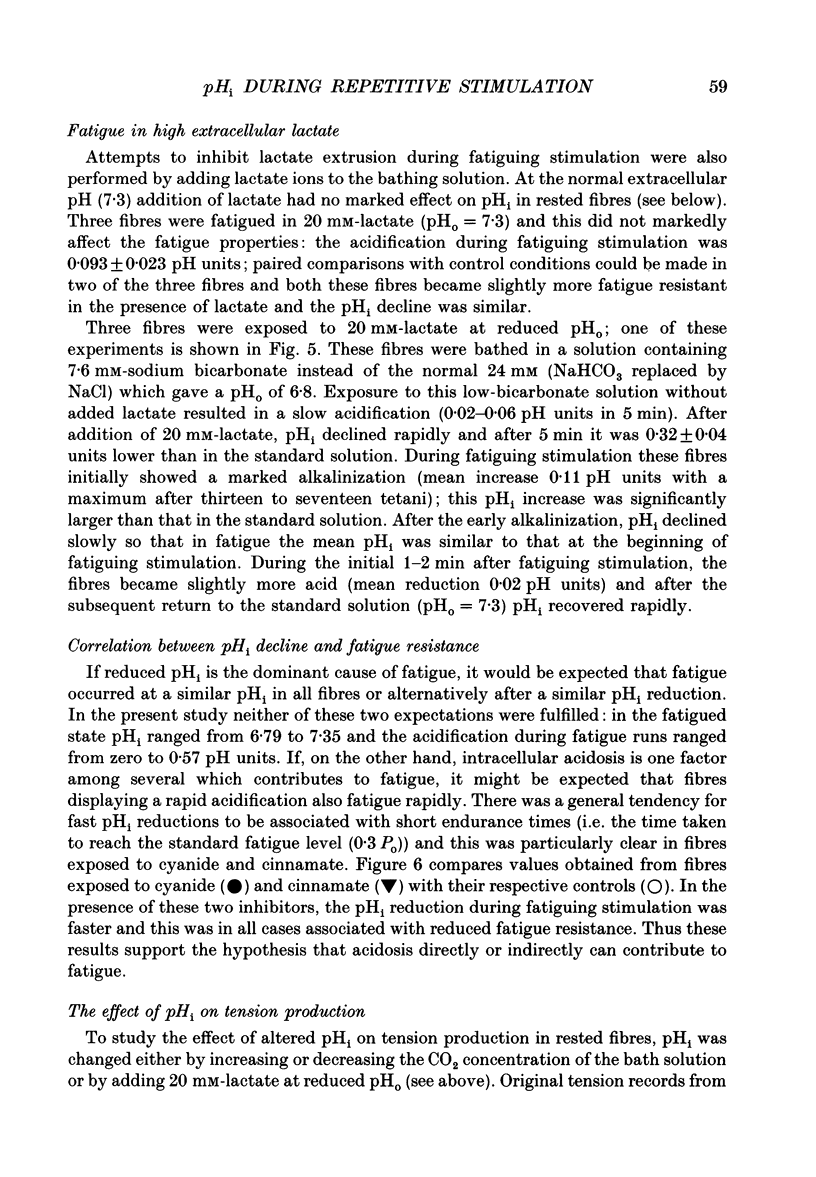
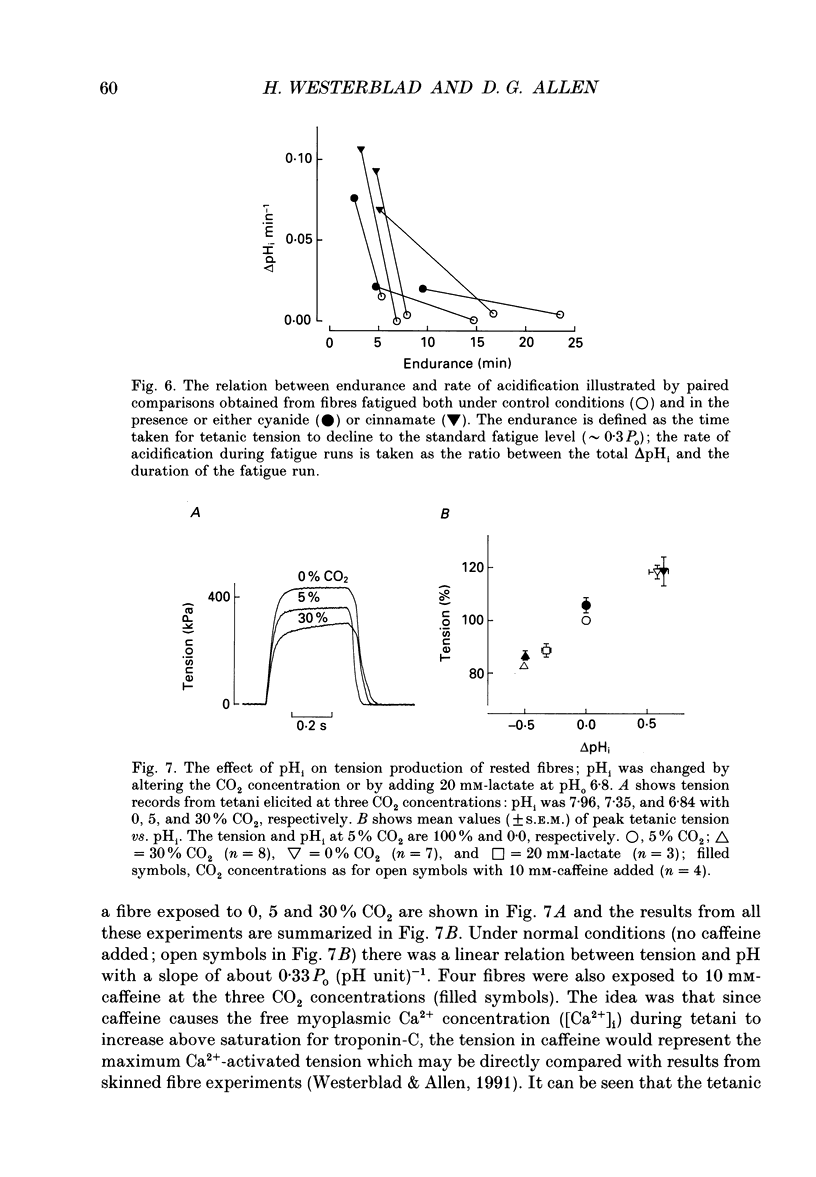
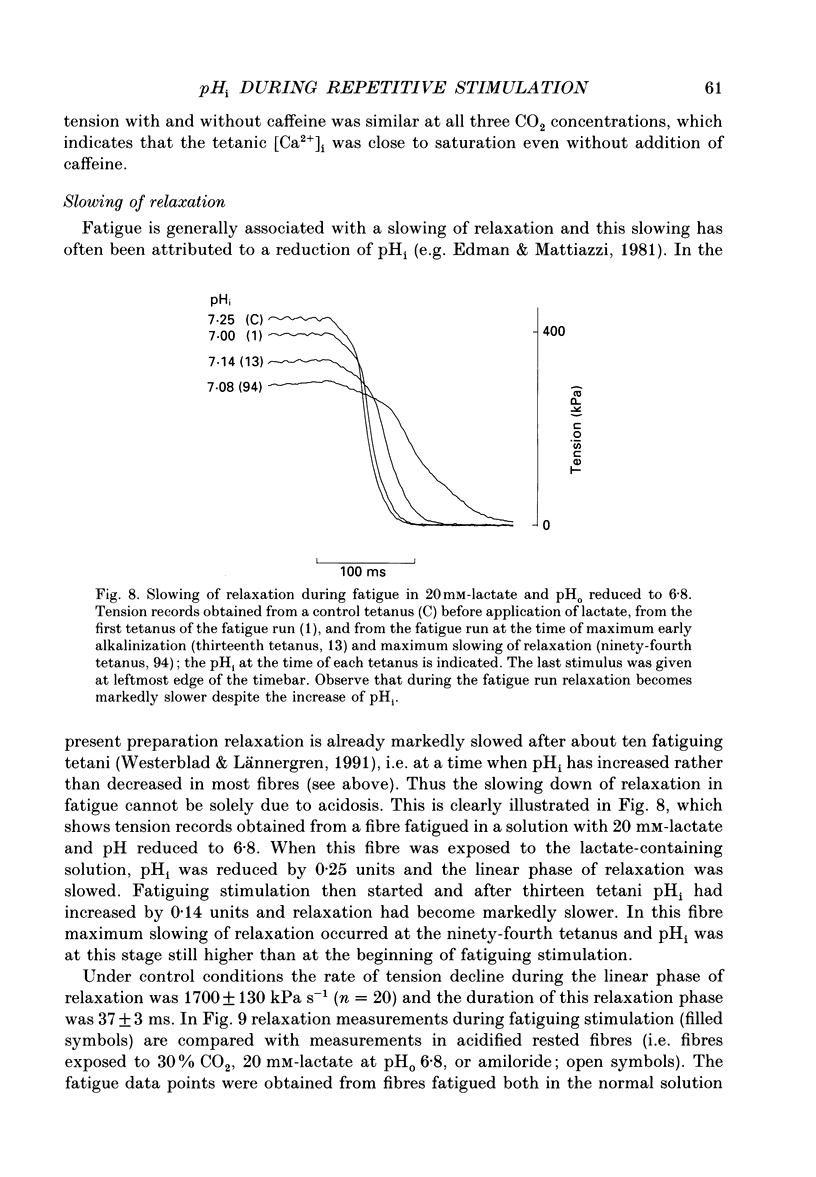
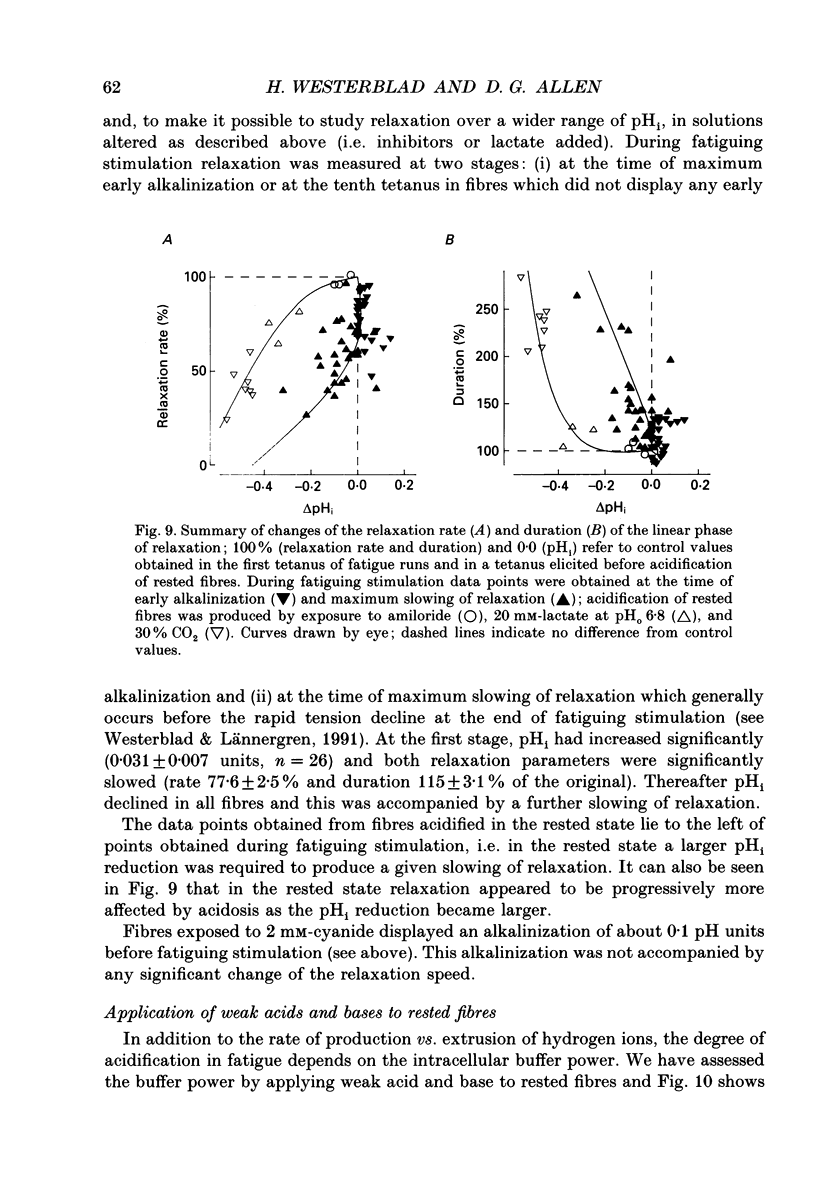
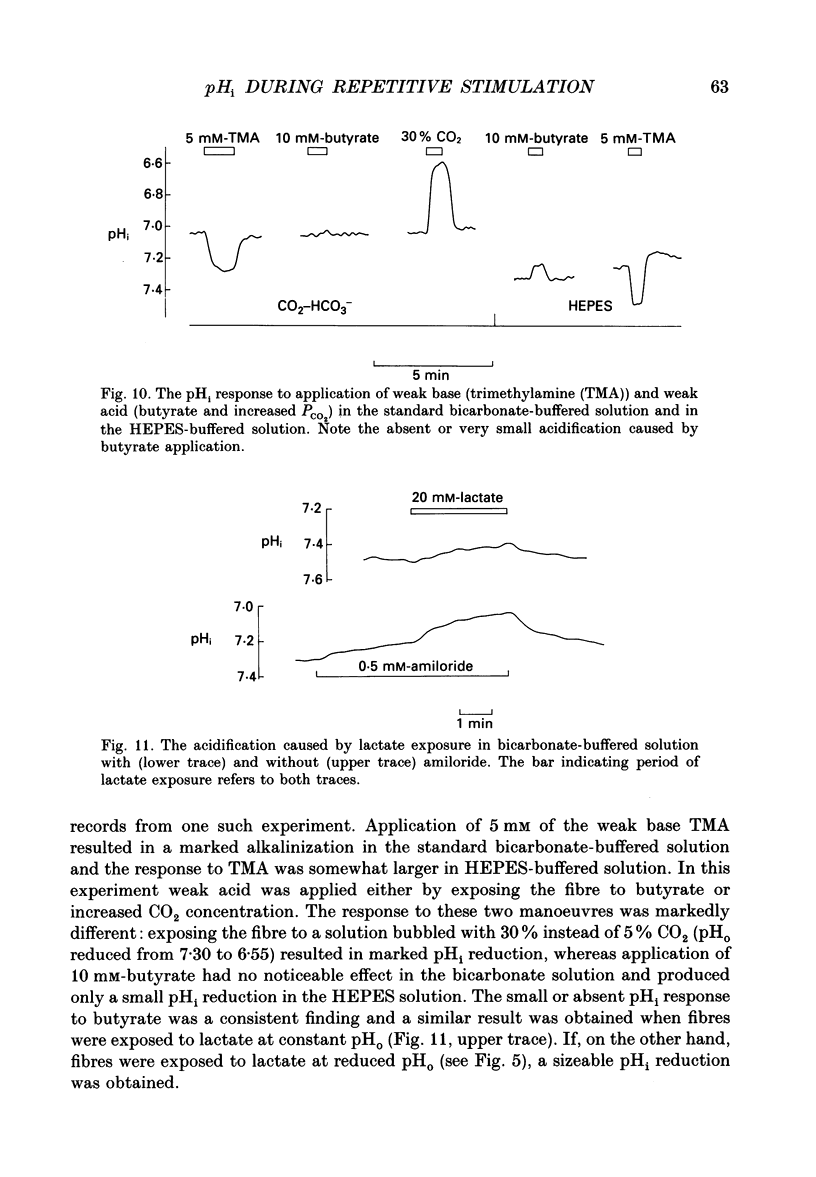
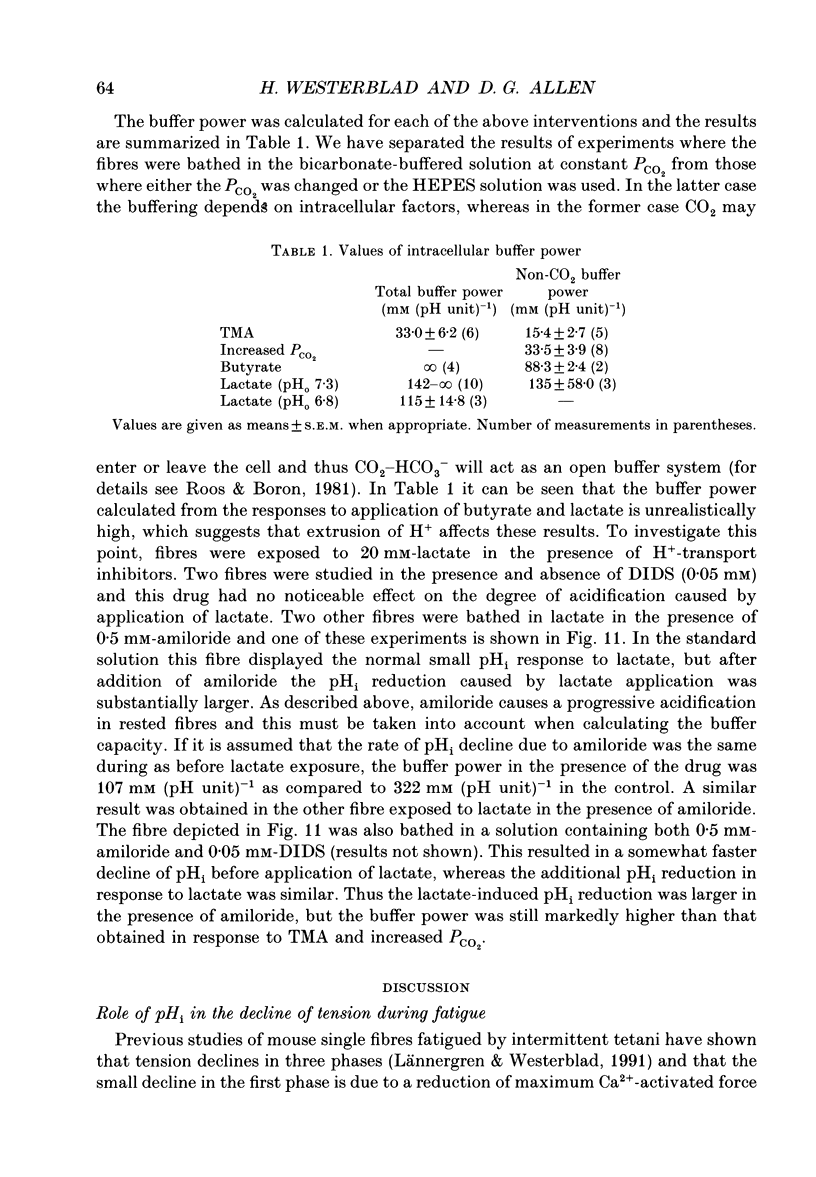
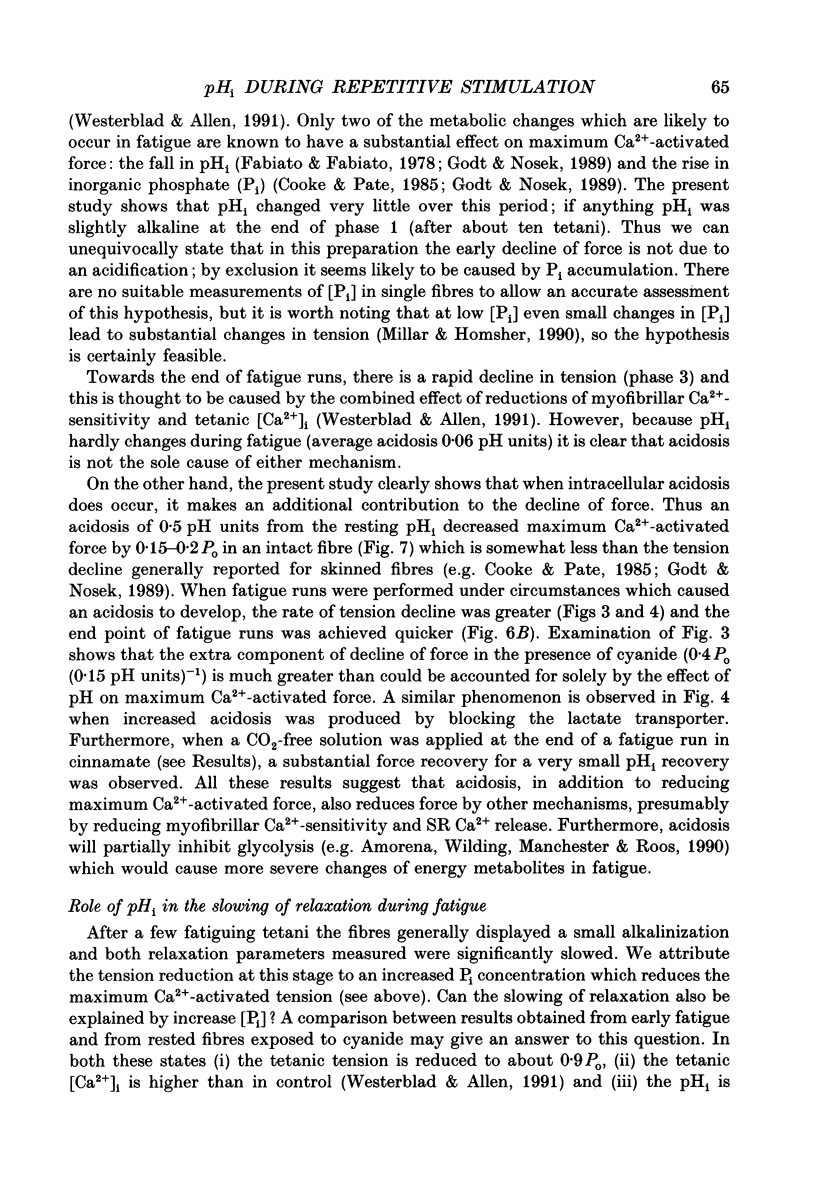
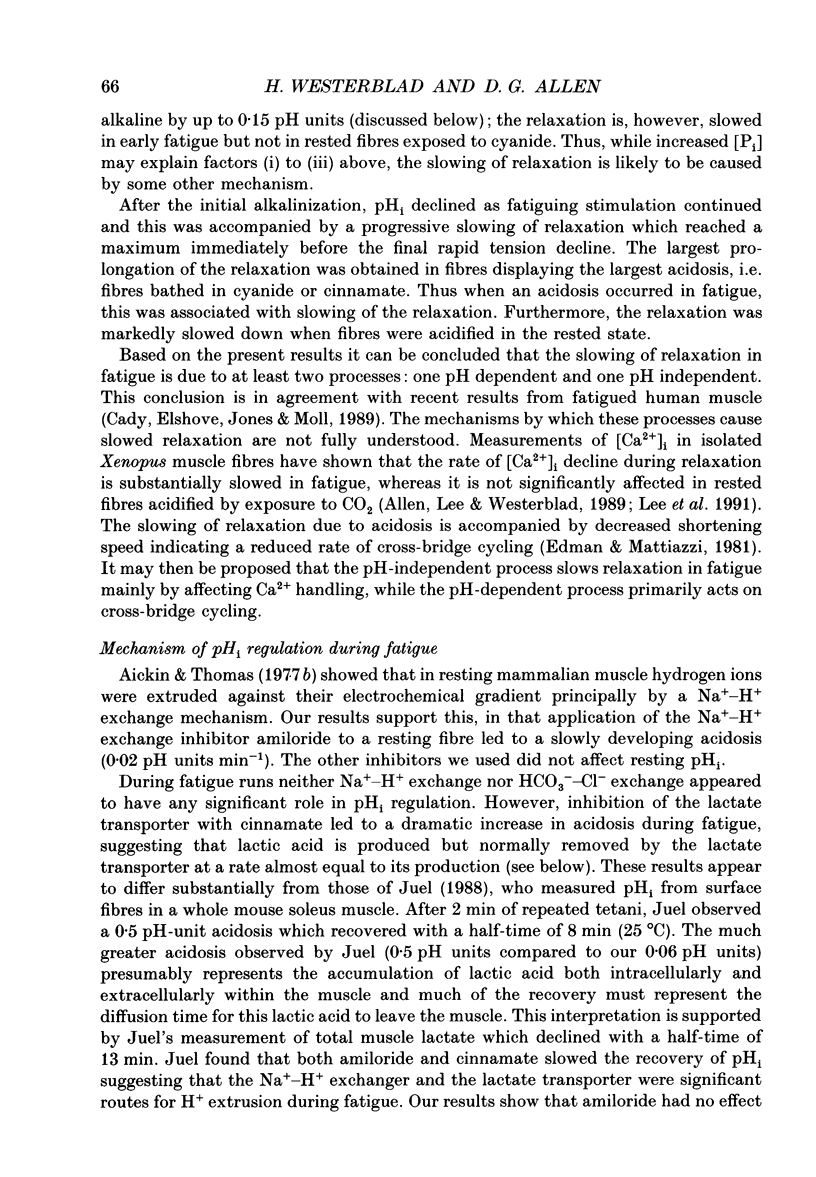
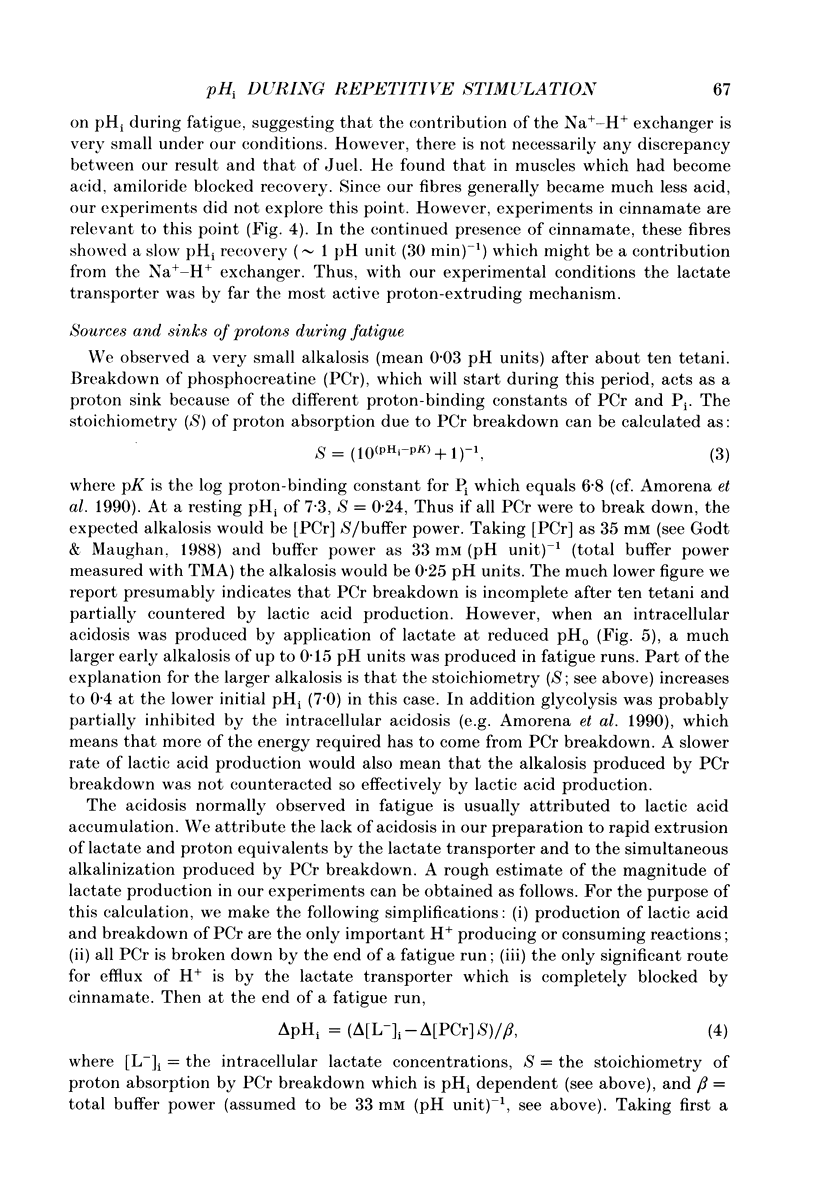
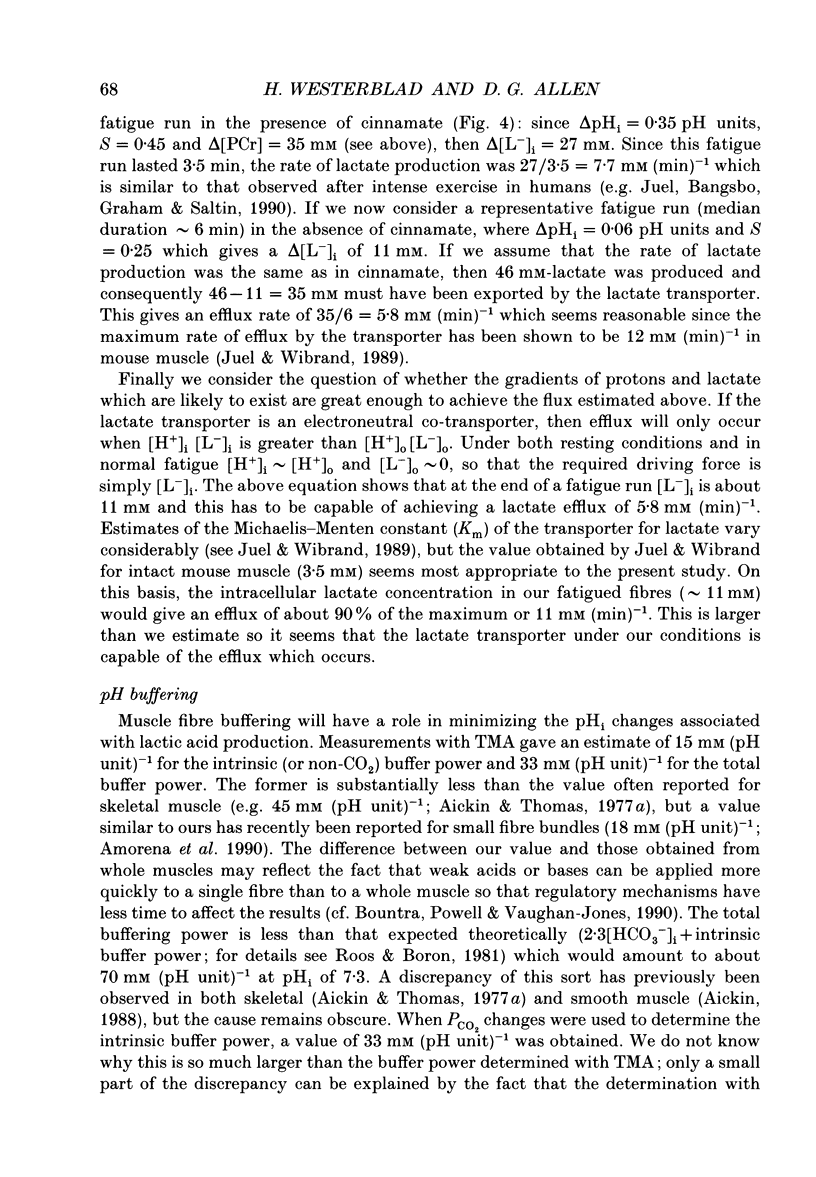
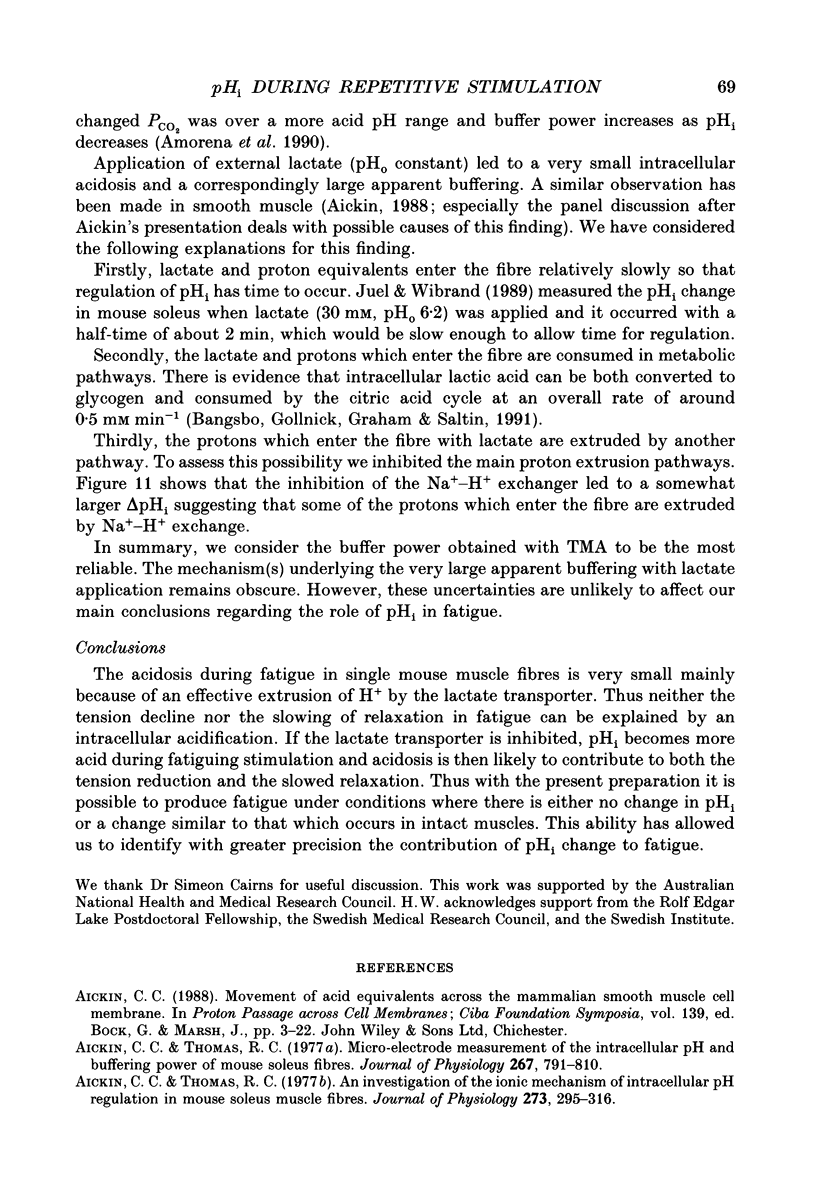
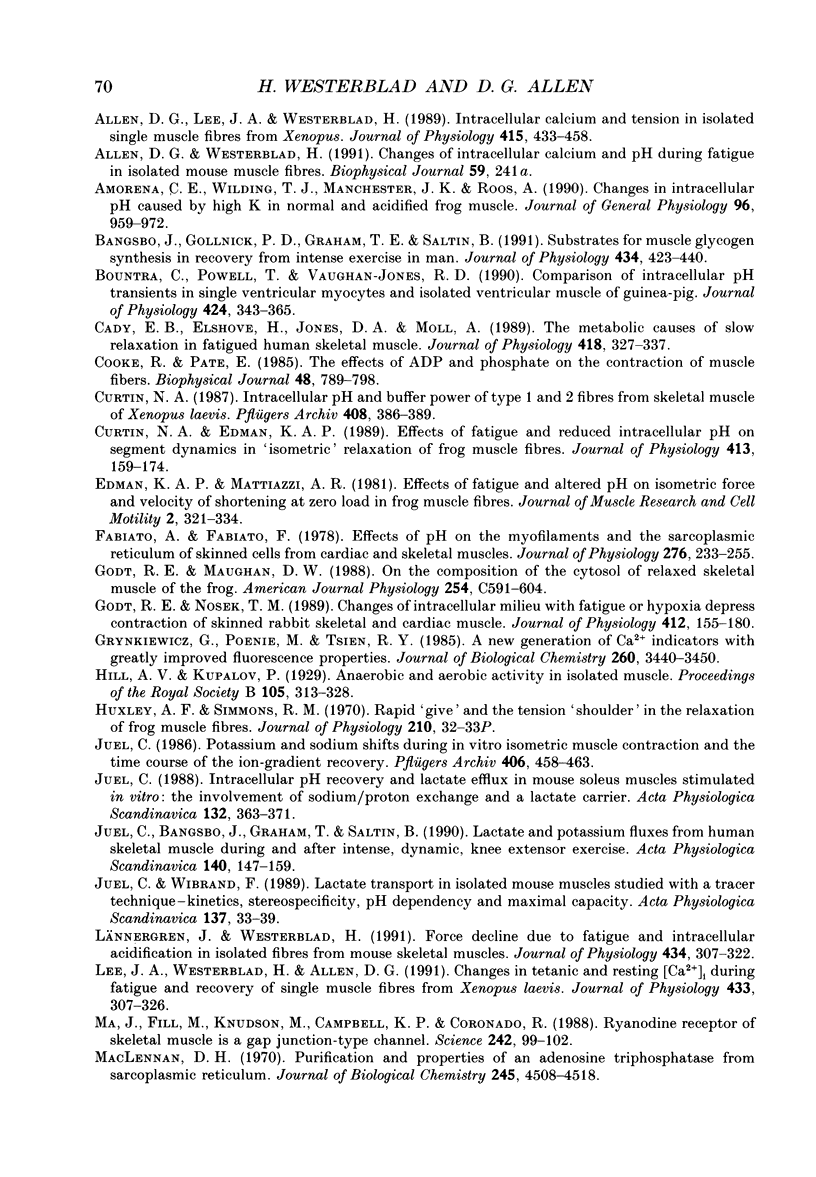
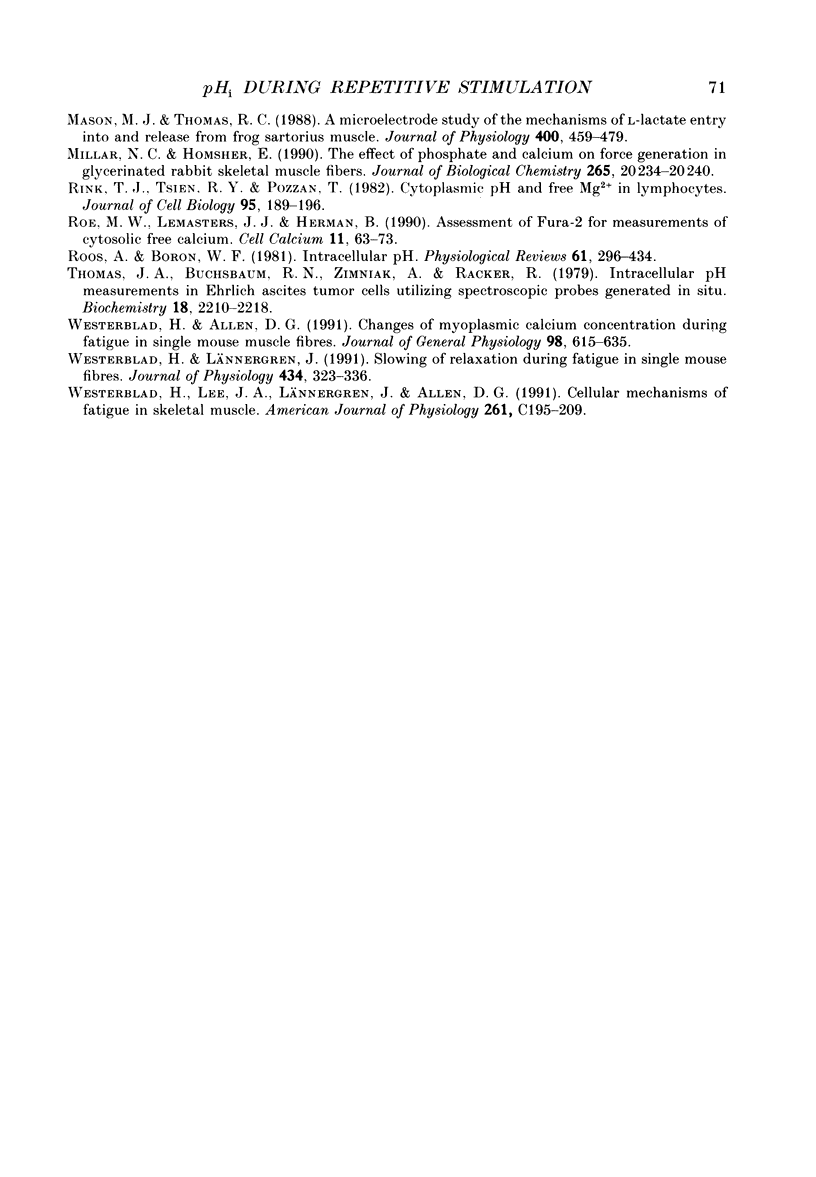
Selected References
These references are in PubMed. This may not be the complete list of references from this article.
- Aickin C. C. Movement of acid equivalents across the mammalian smooth muscle cell membrane. Ciba Found Symp. 1988;139:3–22. doi: 10.1002/9780470513699.ch2. [DOI] [PubMed] [Google Scholar]
- Aickin C. C., Thomas R. C. An investigation of the ionic mechanism of intracellular pH regulation in mouse soleus muscle fibres. J Physiol. 1977 Dec;273(1):295–316. doi: 10.1113/jphysiol.1977.sp012095. [DOI] [PMC free article] [PubMed] [Google Scholar]
- Allen D. G., Lee J. A., Westerblad H. Intracellular calcium and tension during fatigue in isolated single muscle fibres from Xenopus laevis. J Physiol. 1989 Aug;415:433–458. doi: 10.1113/jphysiol.1989.sp017730. [DOI] [PMC free article] [PubMed] [Google Scholar]
- Amorena C. E., Wilding T. J., Manchester J. K., Roos A. Changes in intracellular pH caused by high K in normal and acidified frog muscle. Relation to metabolic changes. J Gen Physiol. 1990 Nov;96(5):959–972. doi: 10.1085/jgp.96.5.959. [DOI] [PMC free article] [PubMed] [Google Scholar]
- Bangsbo J., Gollnick P. D., Graham T. E., Saltin B. Substrates for muscle glycogen synthesis in recovery from intense exercise in man. J Physiol. 1991 Mar;434:423–440. doi: 10.1113/jphysiol.1991.sp018478. [DOI] [PMC free article] [PubMed] [Google Scholar]
- Bountra C., Powell T., Vaughan-Jones R. D. Comparison of intracellular pH transients in single ventricular myocytes and isolated ventricular muscle of guinea-pig. J Physiol. 1990 May;424:343–365. doi: 10.1113/jphysiol.1990.sp018071. [DOI] [PMC free article] [PubMed] [Google Scholar]
- Cady E. B., Elshove H., Jones D. A., Moll A. The metabolic causes of slow relaxation in fatigued human skeletal muscle. J Physiol. 1989 Nov;418:327–337. doi: 10.1113/jphysiol.1989.sp017843. [DOI] [PMC free article] [PubMed] [Google Scholar]
- Cooke R., Pate E. The effects of ADP and phosphate on the contraction of muscle fibers. Biophys J. 1985 Nov;48(5):789–798. doi: 10.1016/S0006-3495(85)83837-6. [DOI] [PMC free article] [PubMed] [Google Scholar]
- Curtin N. A., Edman K. A. Effects of fatigue and reduced intracellular pH on segment dynamics in 'isometric' relaxation of frog muscle fibres. J Physiol. 1989 Jun;413:159–174. doi: 10.1113/jphysiol.1989.sp017647. [DOI] [PMC free article] [PubMed] [Google Scholar]
- Curtin N. A. Intracellular pH and buffer power of type 1 and 2 fibres from skeletal muscle of Xenopus laevis. Pflugers Arch. 1987 Apr;408(4):386–389. doi: 10.1007/BF00581133. [DOI] [PubMed] [Google Scholar]
- Edman K. A., Mattiazzi A. R. Effects of fatigue and altered pH on isometric force and velocity of shortening at zero load in frog muscle fibres. J Muscle Res Cell Motil. 1981 Sep;2(3):321–334. doi: 10.1007/BF00713270. [DOI] [PubMed] [Google Scholar]
- Fabiato A., Fabiato F. Effects of pH on the myofilaments and the sarcoplasmic reticulum of skinned cells from cardiace and skeletal muscles. J Physiol. 1978 Mar;276:233–255. doi: 10.1113/jphysiol.1978.sp012231. [DOI] [PMC free article] [PubMed] [Google Scholar]
- Godt R. E., Maughan D. W. On the composition of the cytosol of relaxed skeletal muscle of the frog. Am J Physiol. 1988 May;254(5 Pt 1):C591–C604. doi: 10.1152/ajpcell.1988.254.5.C591. [DOI] [PubMed] [Google Scholar]
- Godt R. E., Nosek T. M. Changes of intracellular milieu with fatigue or hypoxia depress contraction of skinned rabbit skeletal and cardiac muscle. J Physiol. 1989 May;412:155–180. doi: 10.1113/jphysiol.1989.sp017609. [DOI] [PMC free article] [PubMed] [Google Scholar]
- Grynkiewicz G., Poenie M., Tsien R. Y. A new generation of Ca2+ indicators with greatly improved fluorescence properties. J Biol Chem. 1985 Mar 25;260(6):3440–3450. [PubMed] [Google Scholar]
- Huxley A. F., Simmons R. M. Rapid 'give' and the tension 'shoulder' in the relaxation of frog muscle fibres. J Physiol. 1970 Sep;210(1):32P–33P. [PubMed] [Google Scholar]
- Juel C., Bangsbo J., Graham T., Saltin B. Lactate and potassium fluxes from human skeletal muscle during and after intense, dynamic, knee extensor exercise. Acta Physiol Scand. 1990 Oct;140(2):147–159. doi: 10.1111/j.1748-1716.1990.tb08986.x. [DOI] [PubMed] [Google Scholar]
- Juel C. Intracellular pH recovery and lactate efflux in mouse soleus muscles stimulated in vitro: the involvement of sodium/proton exchange and a lactate carrier. Acta Physiol Scand. 1988 Mar;132(3):363–371. doi: 10.1111/j.1748-1716.1988.tb08340.x. [DOI] [PubMed] [Google Scholar]
- Juel C. Potassium and sodium shifts during in vitro isometric muscle contraction, and the time course of the ion-gradient recovery. Pflugers Arch. 1986 May;406(5):458–463. doi: 10.1007/BF00583367. [DOI] [PubMed] [Google Scholar]
- Juel C., Wibrand F. Lactate transport in isolated mouse muscles studied with a tracer technique--kinetics, stereospecificity, pH dependency and maximal capacity. Acta Physiol Scand. 1989 Sep;137(1):33–39. doi: 10.1111/j.1748-1716.1989.tb08718.x. [DOI] [PubMed] [Google Scholar]
- Lee J. A., Westerblad H., Allen D. G. Changes in tetanic and resting [Ca2+]i during fatigue and recovery of single muscle fibres from Xenopus laevis. J Physiol. 1991 Feb;433:307–326. doi: 10.1113/jphysiol.1991.sp018427. [DOI] [PMC free article] [PubMed] [Google Scholar]
- Lännergren J., Westerblad H. Force decline due to fatigue and intracellular acidification in isolated fibres from mouse skeletal muscle. J Physiol. 1991 Mar;434:307–322. doi: 10.1113/jphysiol.1991.sp018471. [DOI] [PMC free article] [PubMed] [Google Scholar]
- Ma J., Fill M., Knudson C. M., Campbell K. P., Coronado R. Ryanodine receptor of skeletal muscle is a gap junction-type channel. Science. 1988 Oct 7;242(4875):99–102. doi: 10.1126/science.2459777. [DOI] [PubMed] [Google Scholar]
- MacLennan D. H. Purification and properties of an adenosine triphosphatase from sarcoplasmic reticulum. J Biol Chem. 1970 Sep 10;245(17):4508–4518. [PubMed] [Google Scholar]
- Mason M. J., Thomas R. C. A microelectrode study of the mechanisms of L-lactate entry into and release from frog sartorius muscle. J Physiol. 1988 Jun;400:459–479. doi: 10.1113/jphysiol.1988.sp017132. [DOI] [PMC free article] [PubMed] [Google Scholar]
- Millar N. C., Homsher E. The effect of phosphate and calcium on force generation in glycerinated rabbit skeletal muscle fibers. A steady-state and transient kinetic study. J Biol Chem. 1990 Nov 25;265(33):20234–20240. [PubMed] [Google Scholar]
- Rink T. J., Tsien R. Y., Pozzan T. Cytoplasmic pH and free Mg2+ in lymphocytes. J Cell Biol. 1982 Oct;95(1):189–196. doi: 10.1083/jcb.95.1.189. [DOI] [PMC free article] [PubMed] [Google Scholar]
- Roe M. W., Lemasters J. J., Herman B. Assessment of Fura-2 for measurements of cytosolic free calcium. Cell Calcium. 1990 Feb-Mar;11(2-3):63–73. doi: 10.1016/0143-4160(90)90060-8. [DOI] [PubMed] [Google Scholar]
- Roos A., Boron W. F. Intracellular pH. Physiol Rev. 1981 Apr;61(2):296–434. doi: 10.1152/physrev.1981.61.2.296. [DOI] [PubMed] [Google Scholar]
- Thomas J. A., Buchsbaum R. N., Zimniak A., Racker E. Intracellular pH measurements in Ehrlich ascites tumor cells utilizing spectroscopic probes generated in situ. Biochemistry. 1979 May 29;18(11):2210–2218. doi: 10.1021/bi00578a012. [DOI] [PubMed] [Google Scholar]
- Westerblad H., Allen D. G. Changes of myoplasmic calcium concentration during fatigue in single mouse muscle fibers. J Gen Physiol. 1991 Sep;98(3):615–635. doi: 10.1085/jgp.98.3.615. [DOI] [PMC free article] [PubMed] [Google Scholar]
- Westerblad H., Lee J. A., Lännergren J., Allen D. G. Cellular mechanisms of fatigue in skeletal muscle. Am J Physiol. 1991 Aug;261(2 Pt 1):C195–C209. doi: 10.1152/ajpcell.1991.261.2.C195. [DOI] [PubMed] [Google Scholar]
- Westerblad H., Lännergren J. Slowing of relaxation during fatigue in single mouse muscle fibres. J Physiol. 1991 Mar;434:323–336. doi: 10.1113/jphysiol.1991.sp018472. [DOI] [PMC free article] [PubMed] [Google Scholar]