Abstract
1. The contraction, measured optically, and the intracellular Na+ activity (aNai), measured with the Na(+)-sensitive fluorescent dye SBFI, have been recorded simultaneously in rat and guinea-pig ventricular myocytes. 2. In rat and guinea-pig ventricular myocytes at rest, aNai was 7.8 +/- 0.3 mM (n = 4) and 5.1 +/- 0.3 mM (n = 16), respectively. 3. When both rat and guinea-pig ventricular myocytes were stimulated at 1 Hz after a rest there was usually a gradual increase in twitch shortening (referred to as a 'staircase') over several minutes accompanied by an increase in aNai over a similar time course. Twitch shortening increased by 21 +/- 3% (n = 6) and 20 +/- 4% (n = 16) (of steady-state twitch shortening during 1 Hz stimulation) per millimolar rise in aNai in rat and guinea-pig ventricular myocytes, respectively. 4. When rat and guinea-pig ventricular myocytes were exposed to strophanthidin to block the Na(+)-K+ pump, there were increases in twitch shortening and aNai over similar time courses. Twitch shortening increased by 24 +/- 4% (n = 5) and 20 +/- 3% (n = 10) (of control twitch shortening) per millimolar rise in aNai in rat and guinea-pig ventricular myocytes respectively. 5. The inotropic effect of cardiac glycosides, such as strophanthidin, is widely regarded to be principally the result of the rise in aNai. The similarity of the relation between twitch shortening and aNai during the staircase and on application of strophanthidin suggests that the progressive increase in the strength of contraction during the staircase was also linked to the rise in aNai. 6. In guinea-pig, but not rat, ventricular myocytes there was hysteresis in the relation between twitch shortening and aNai on application and wash-off of strophanthidin. This indicates that strophanthidin has another inotropic action in guinea-pig ventricular myocytes. 7. A computer model of excitation-contraction coupling has been developed to simulate the staircase and the action of cardiac glycoside and to account for the relation between contraction and intracellular Na+.
Full text
PDF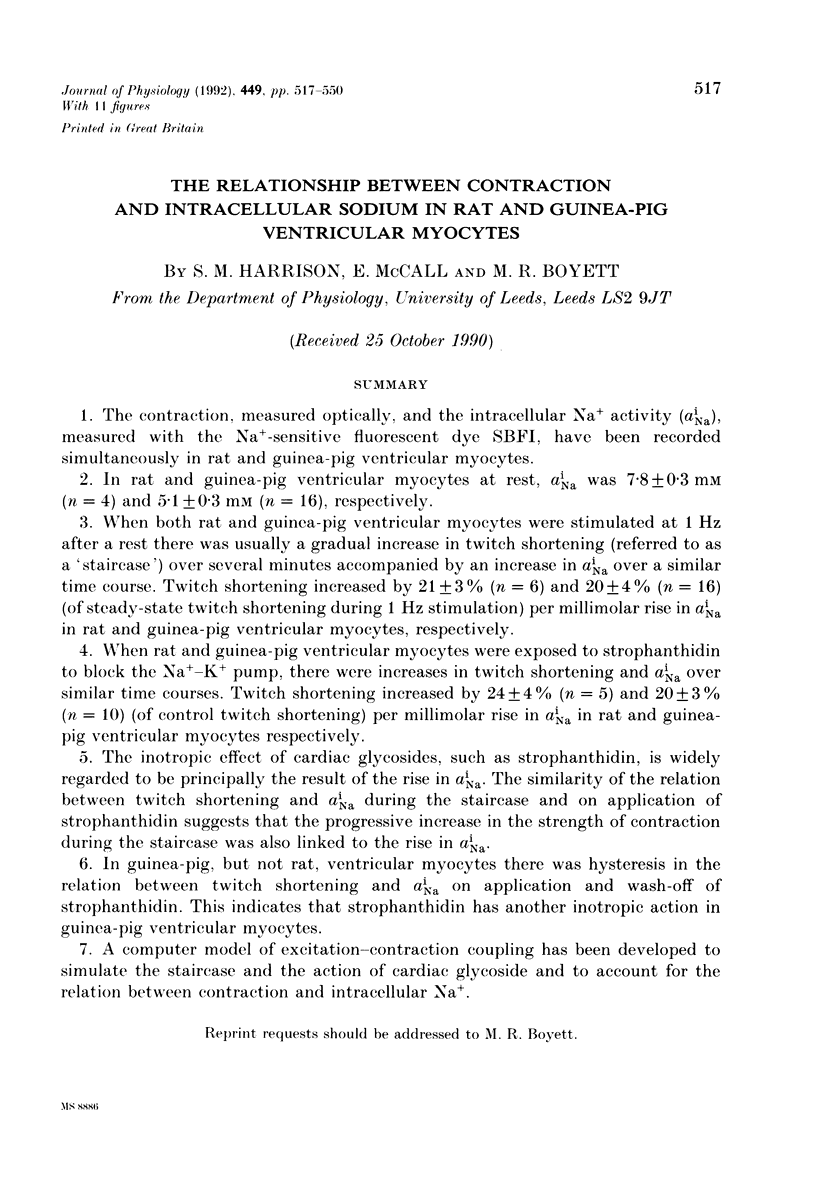
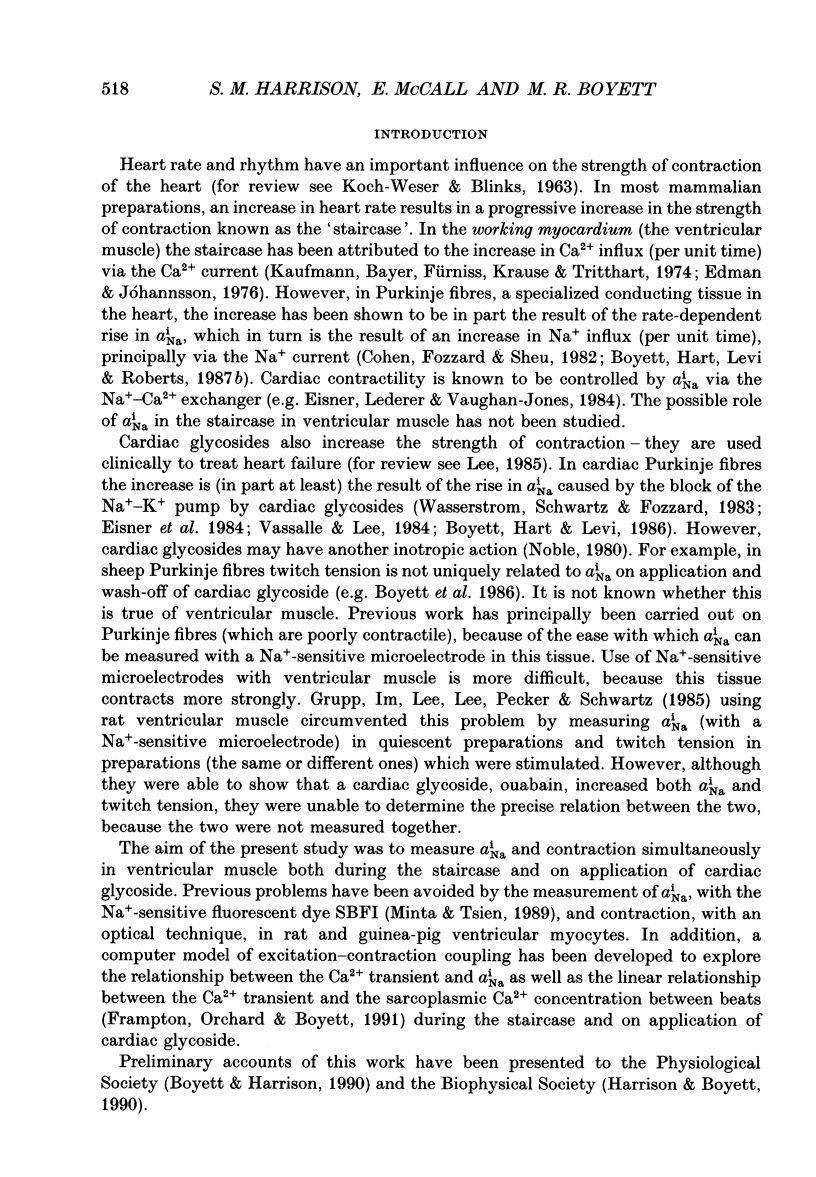
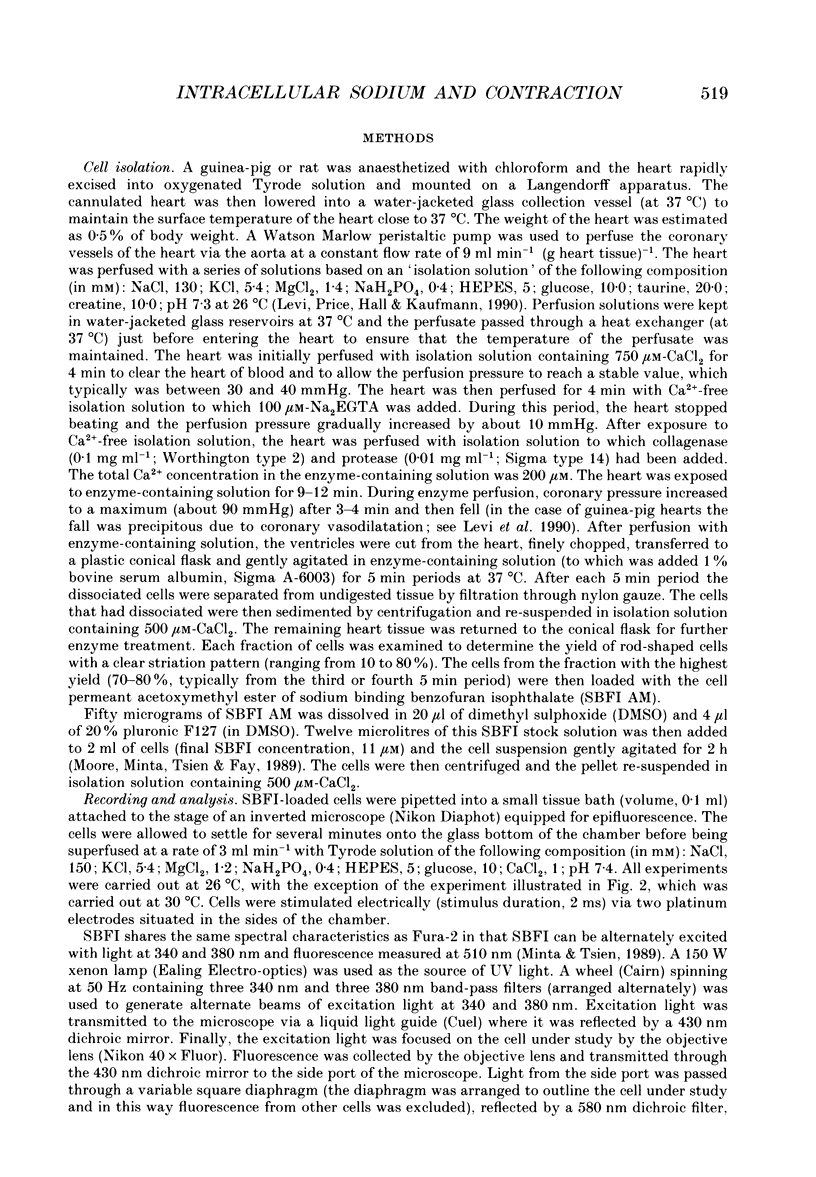
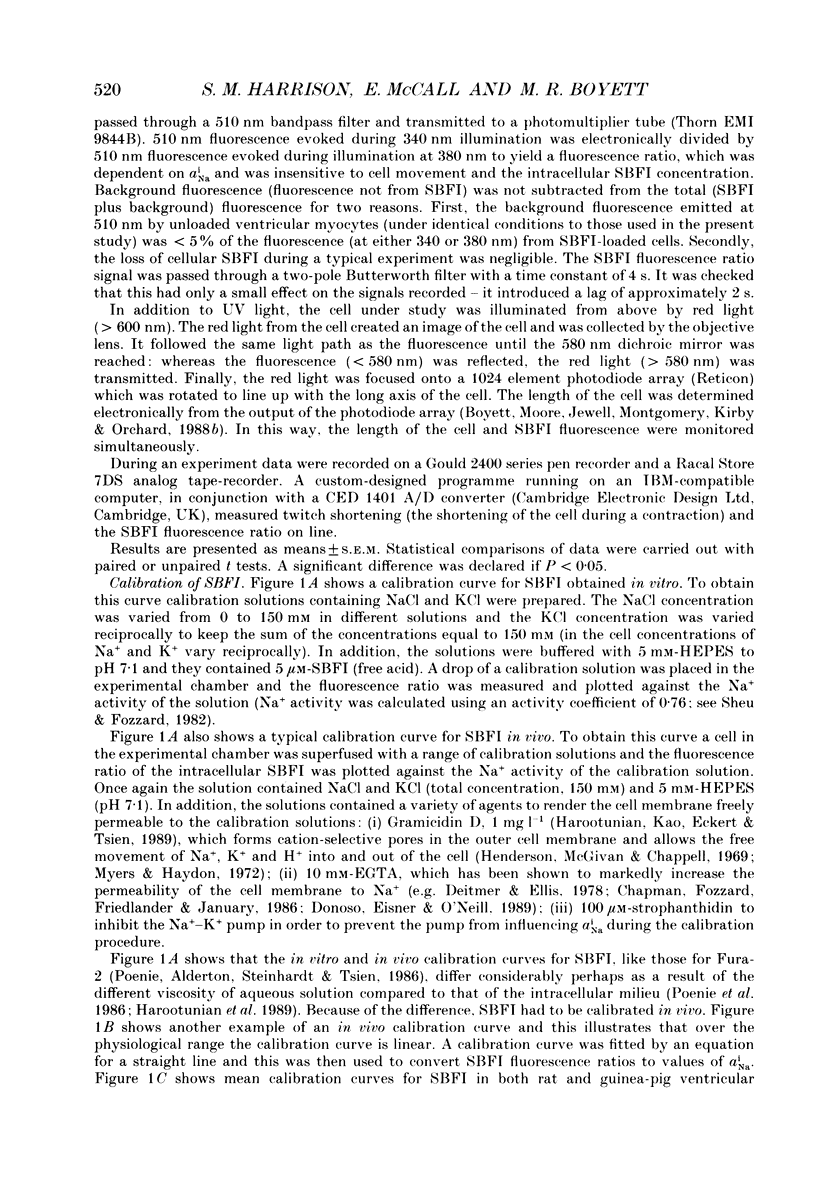
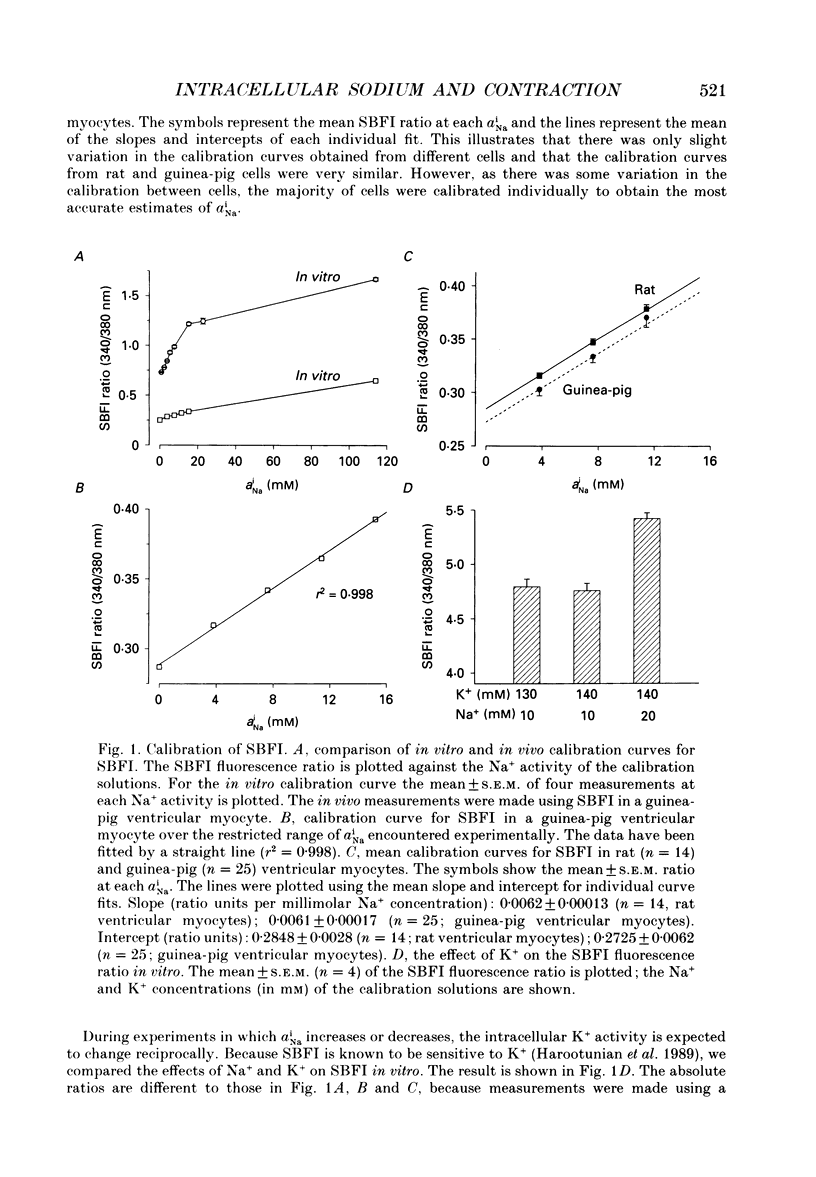
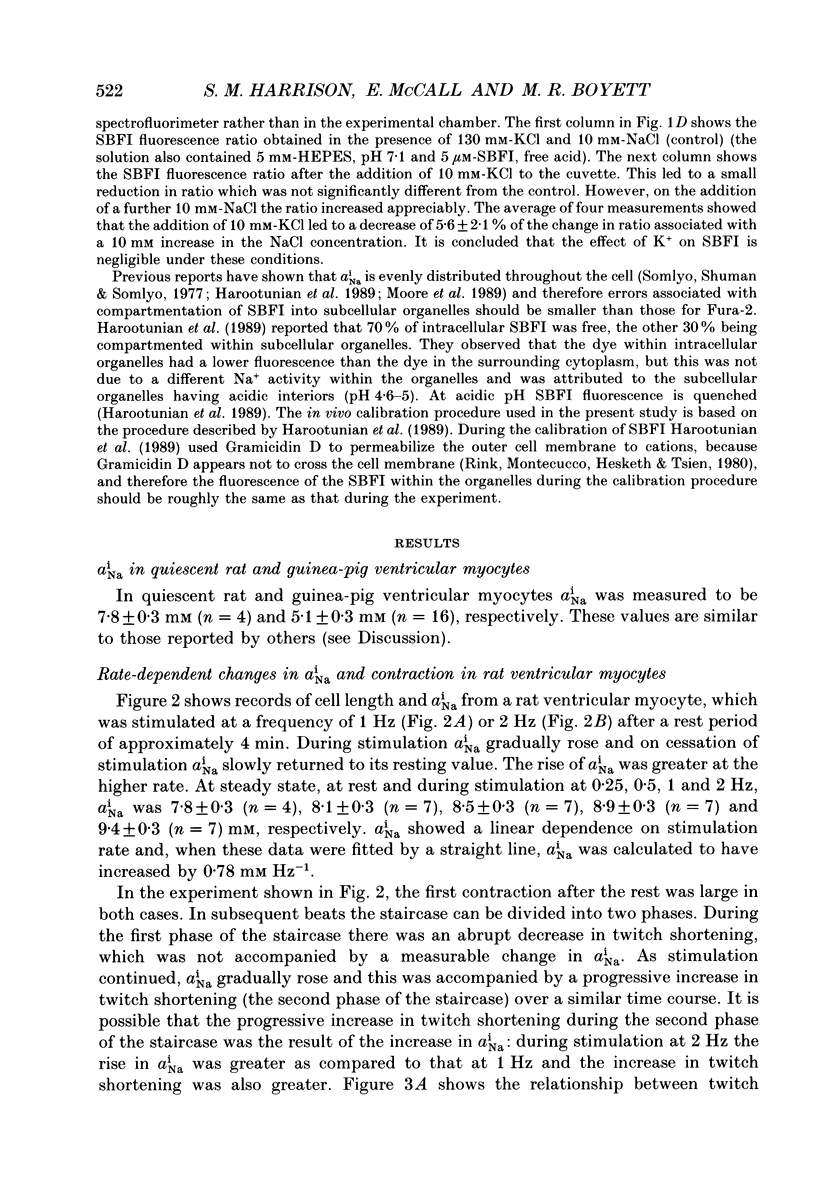
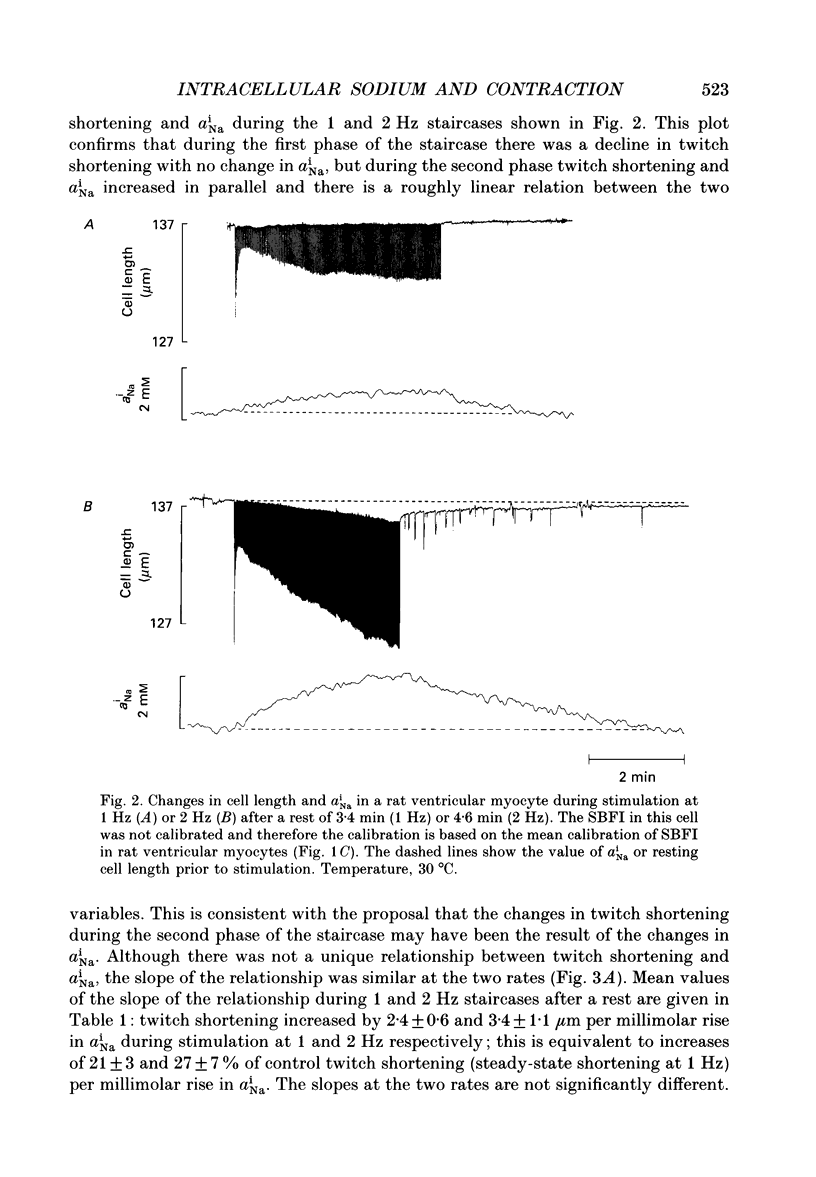
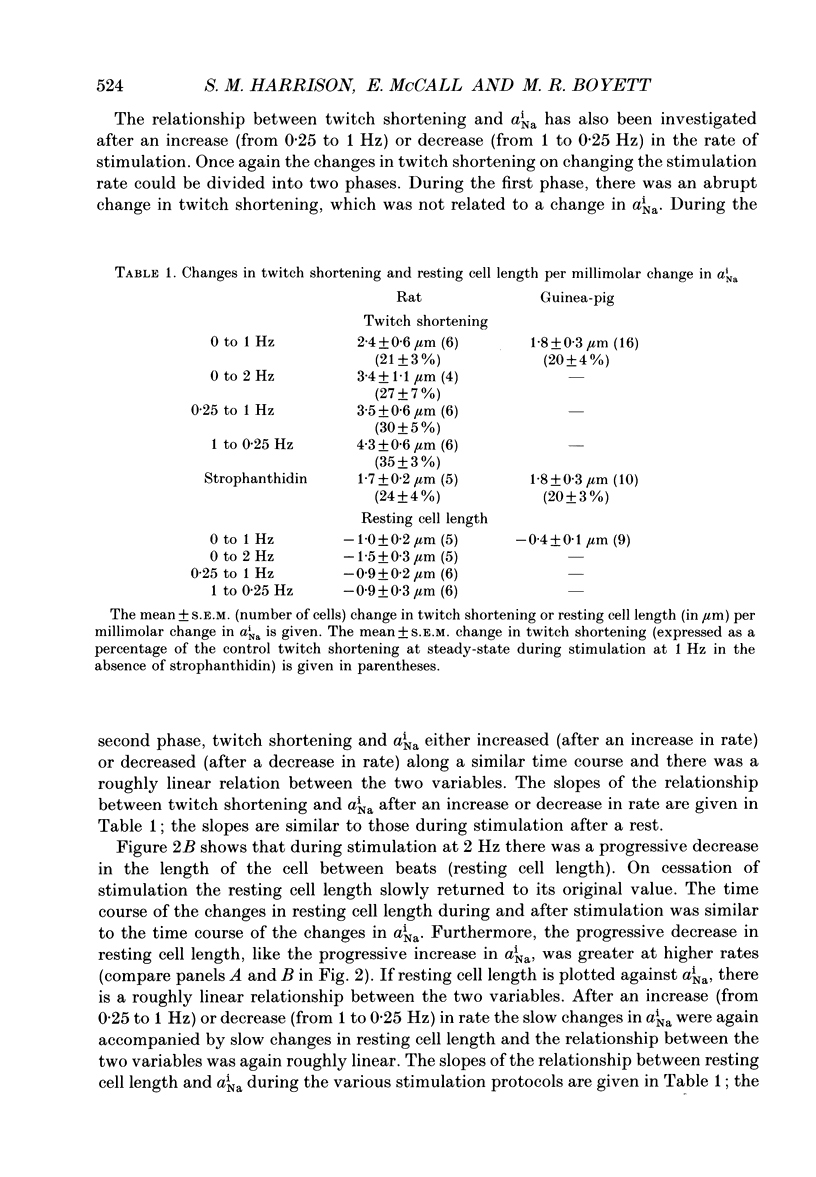
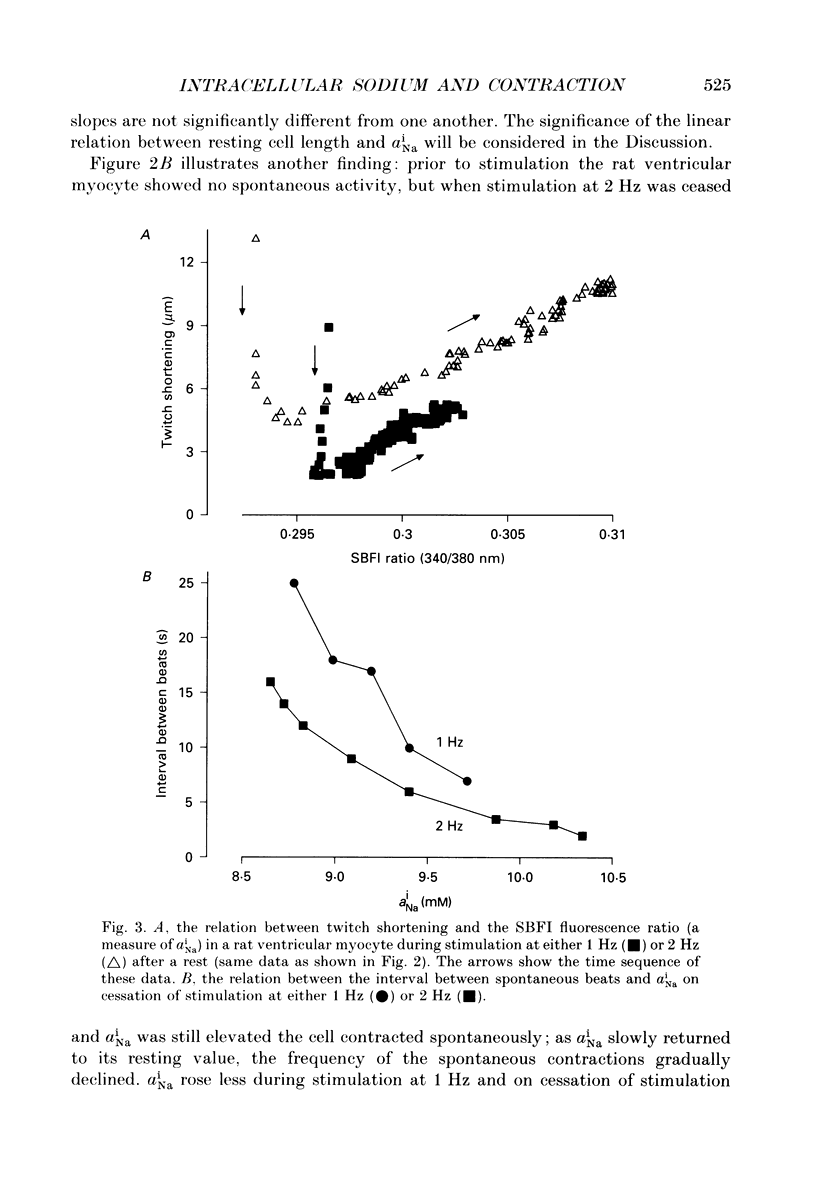
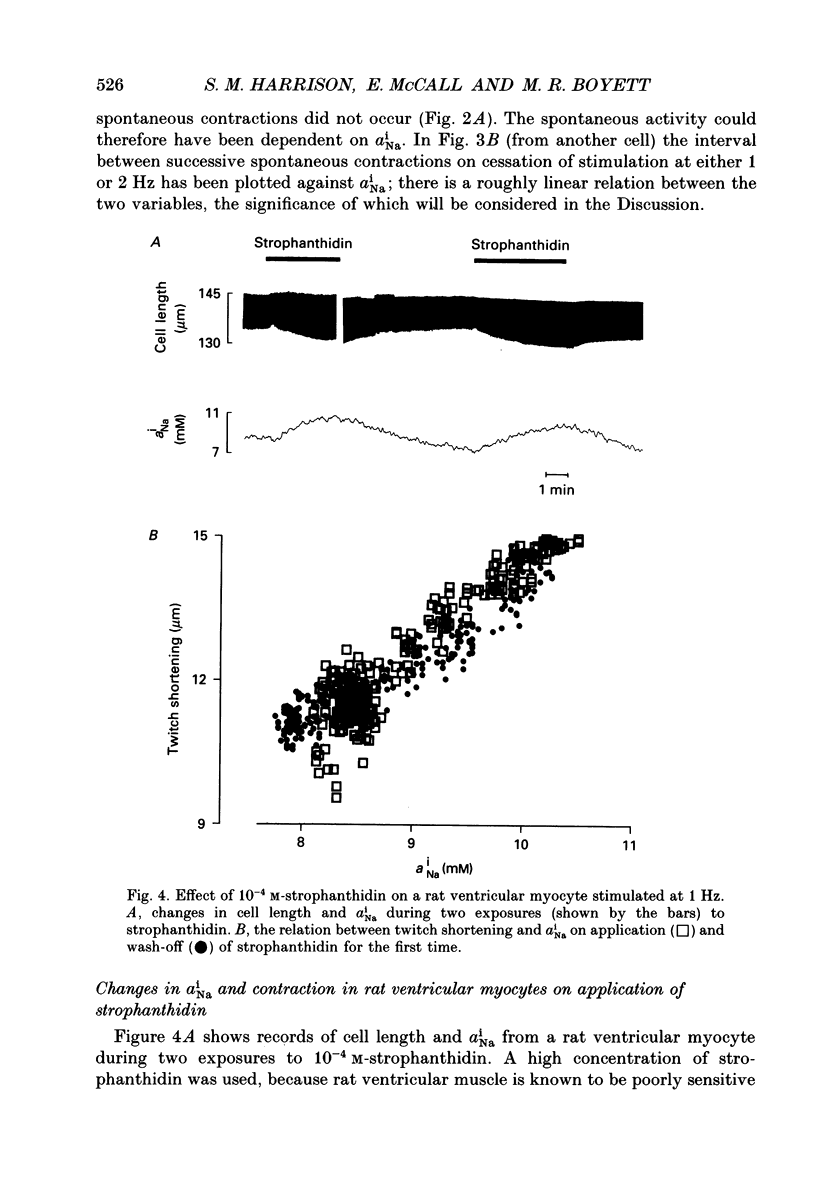
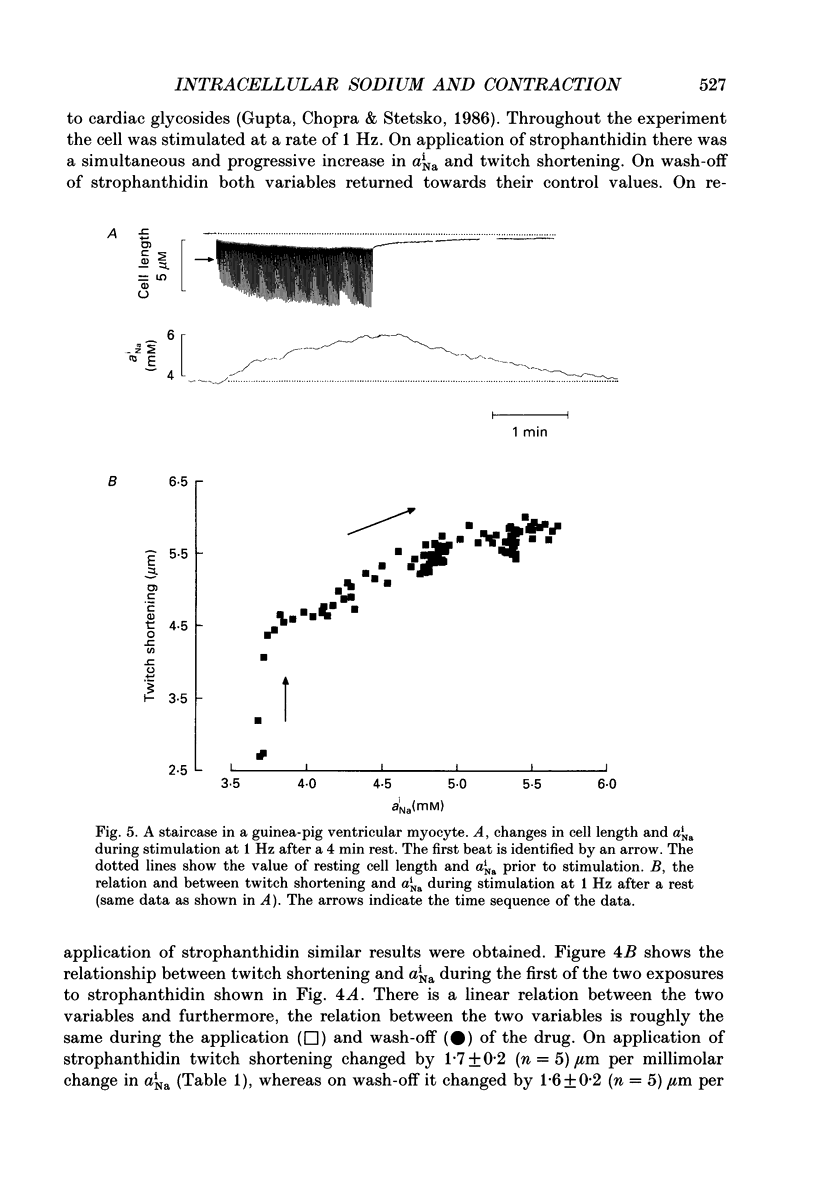
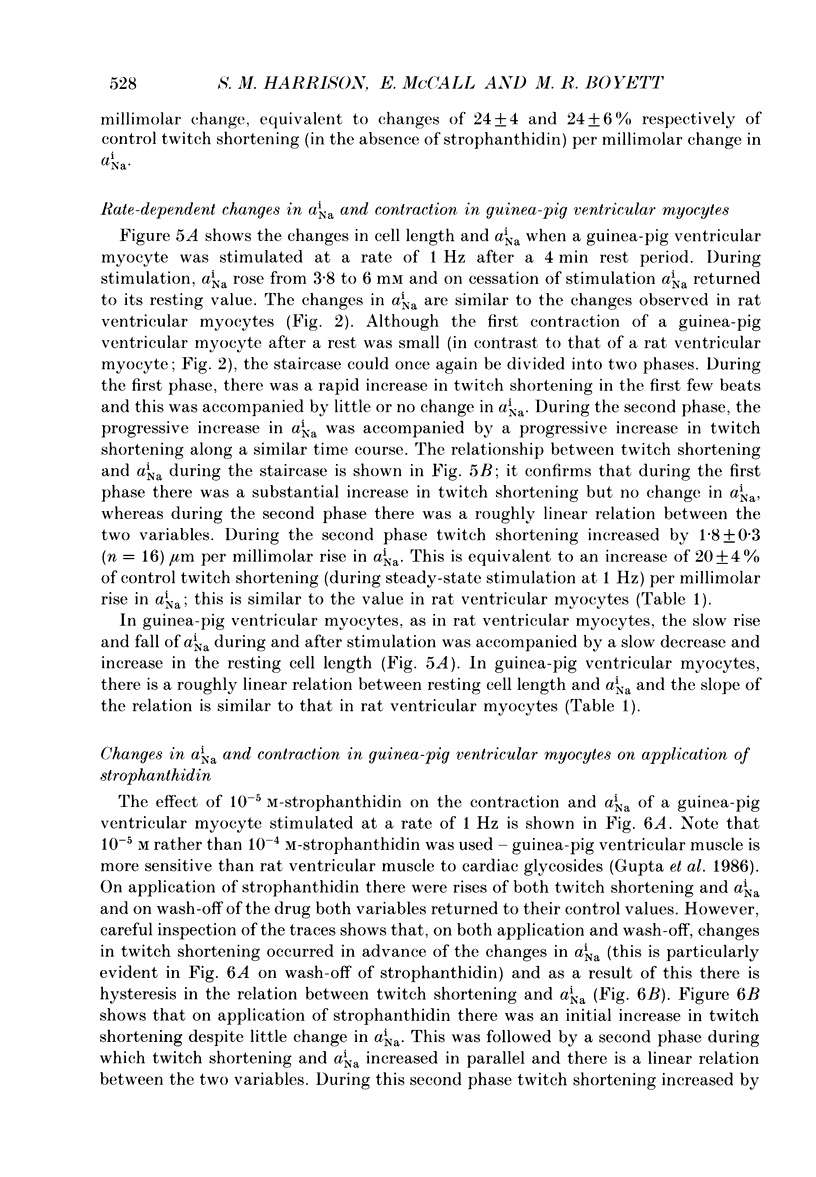
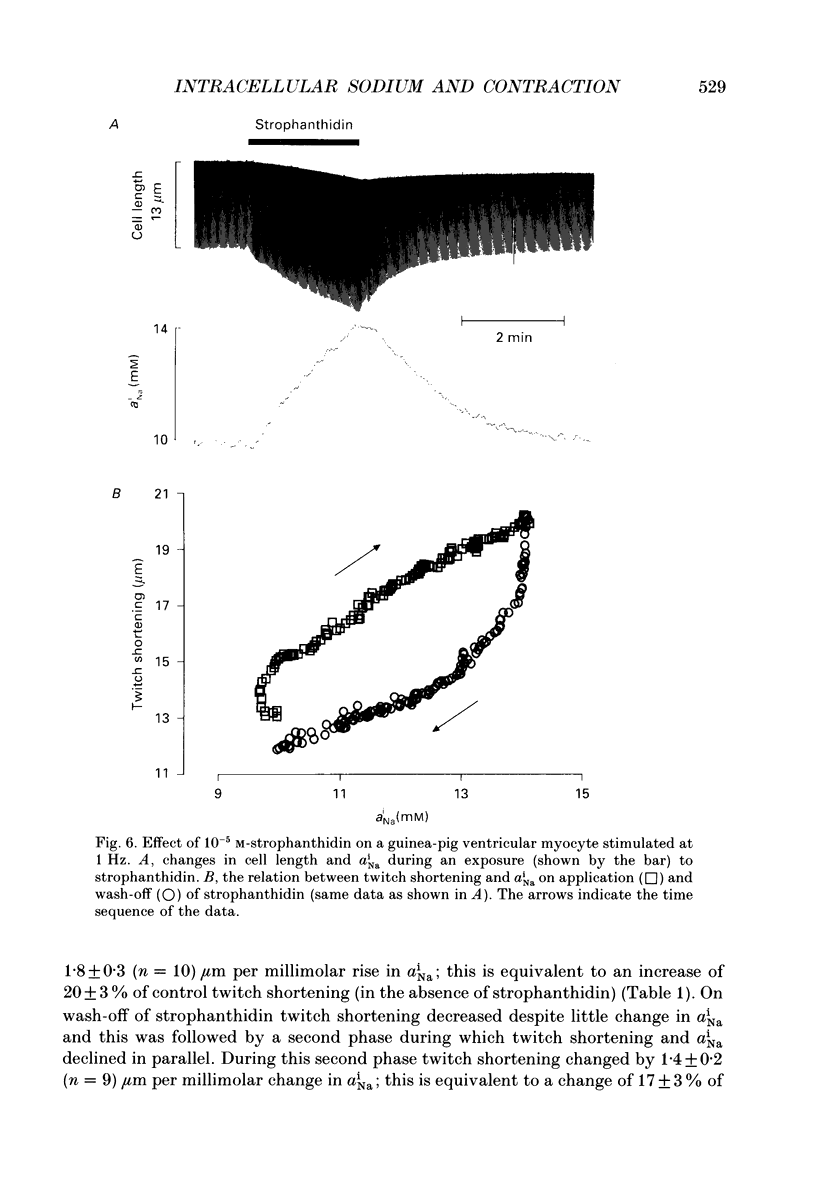
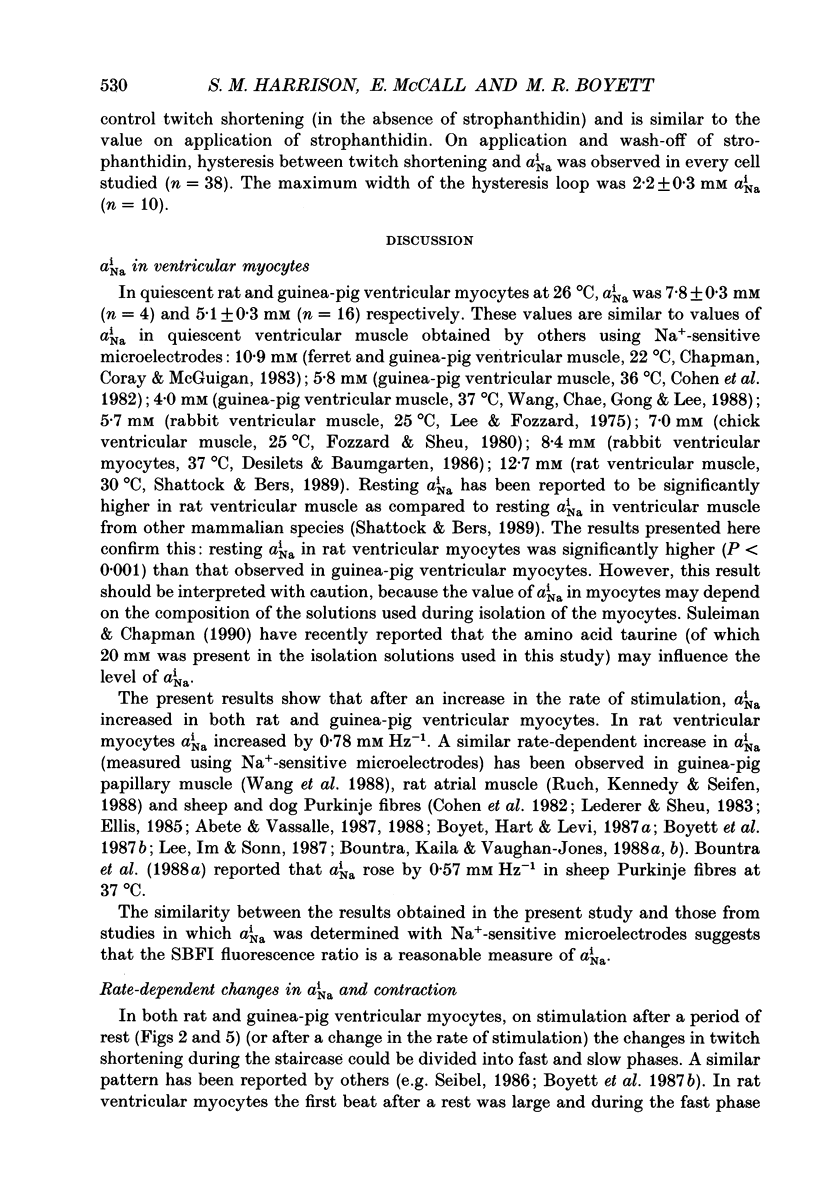
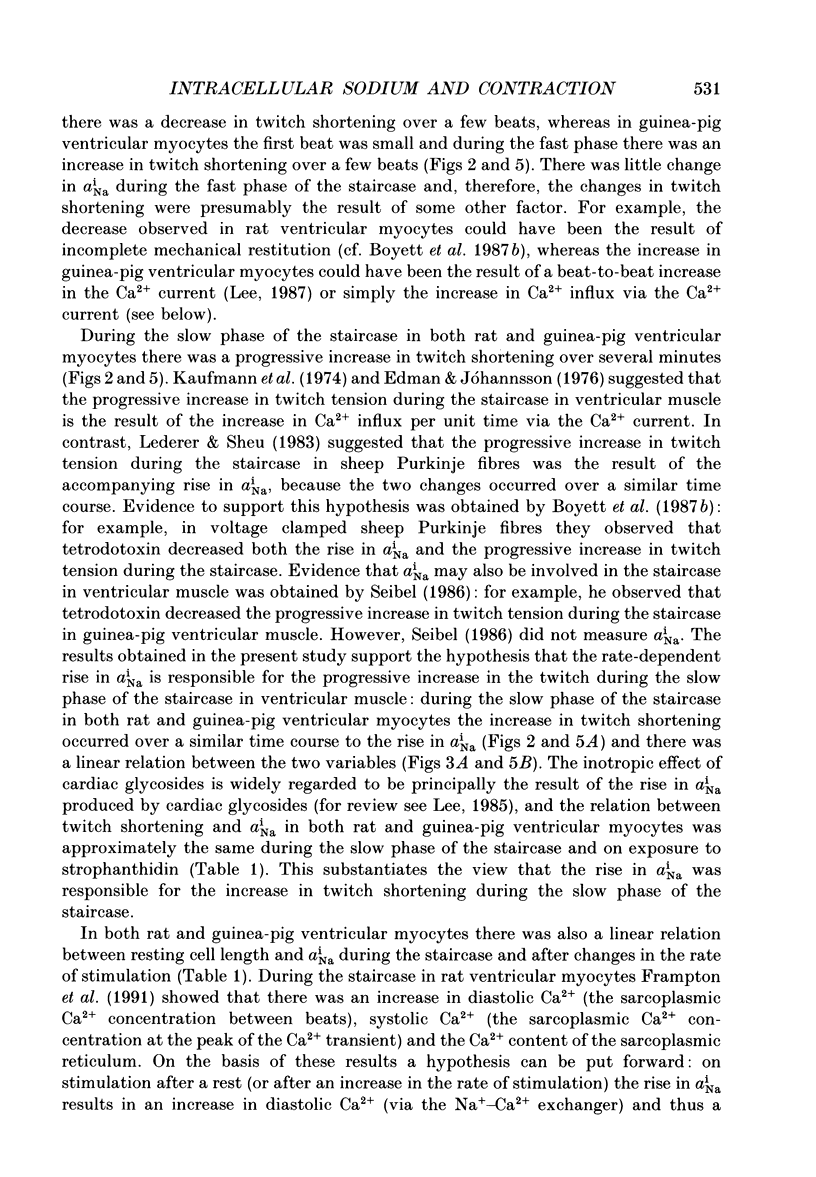
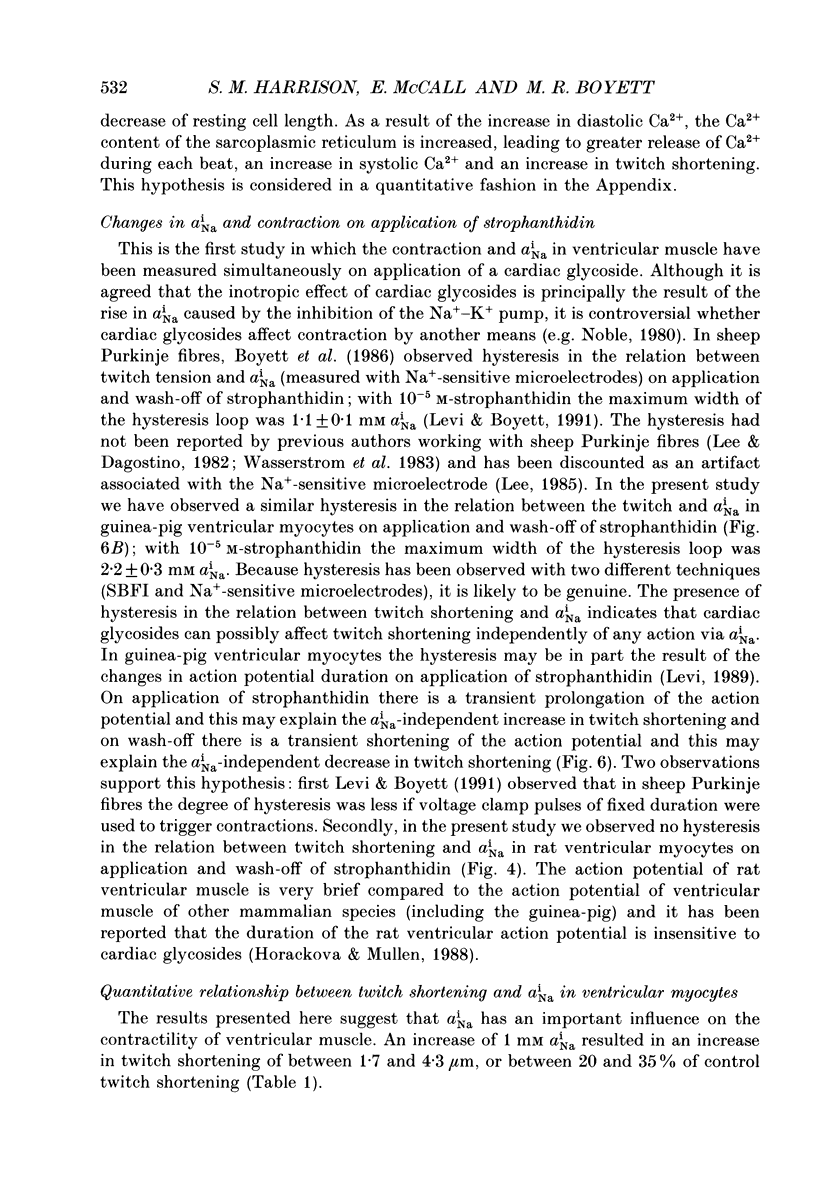
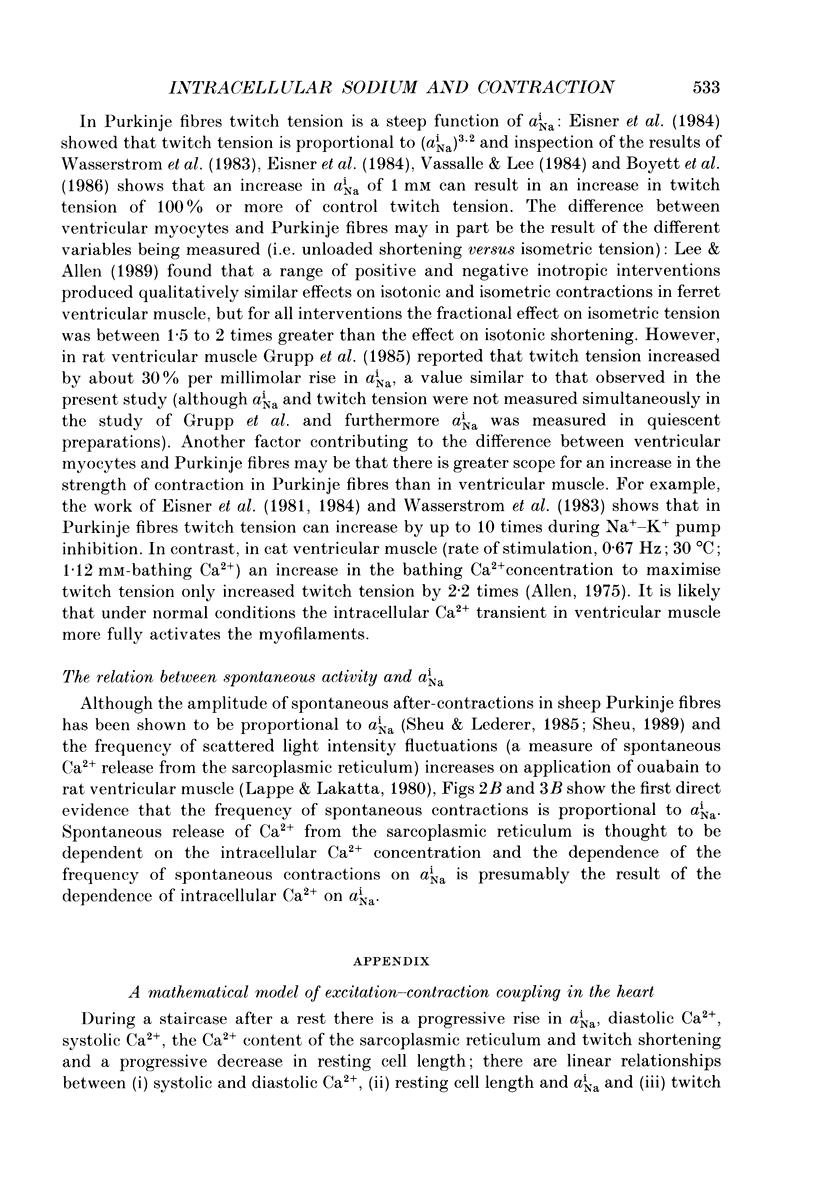
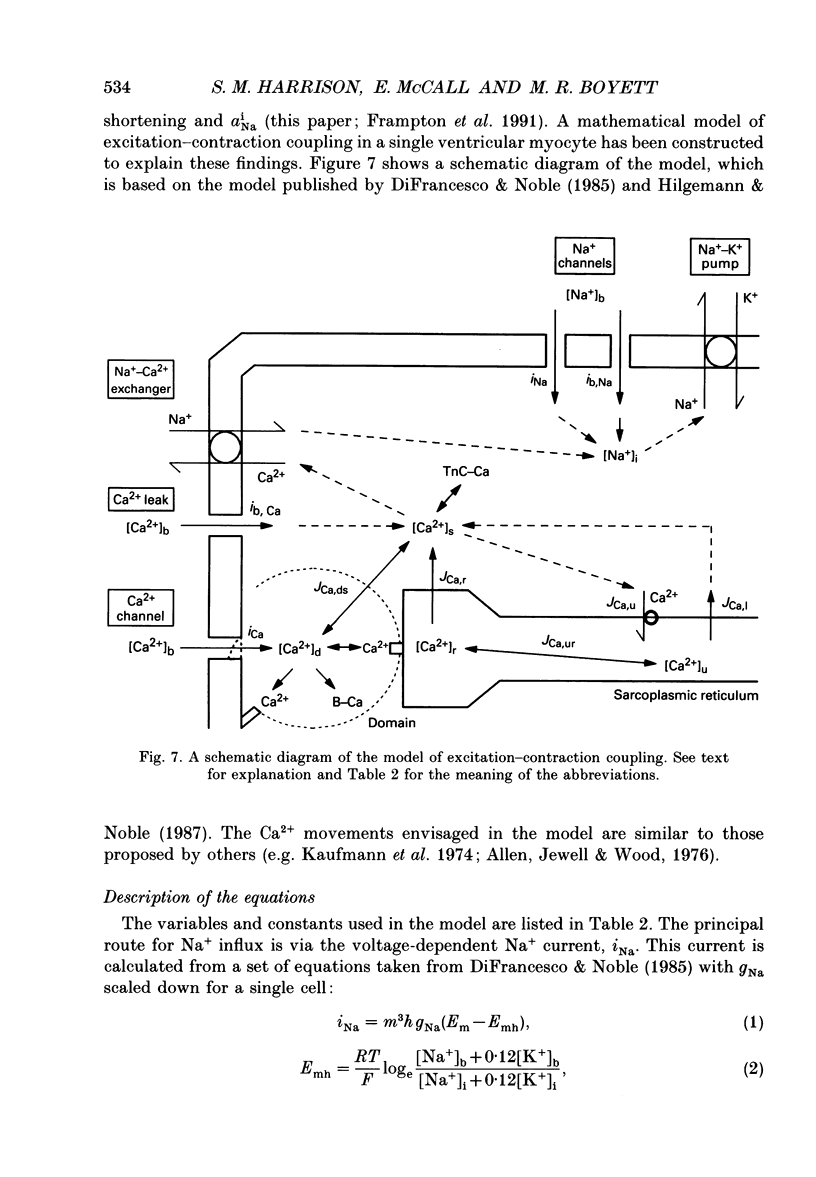
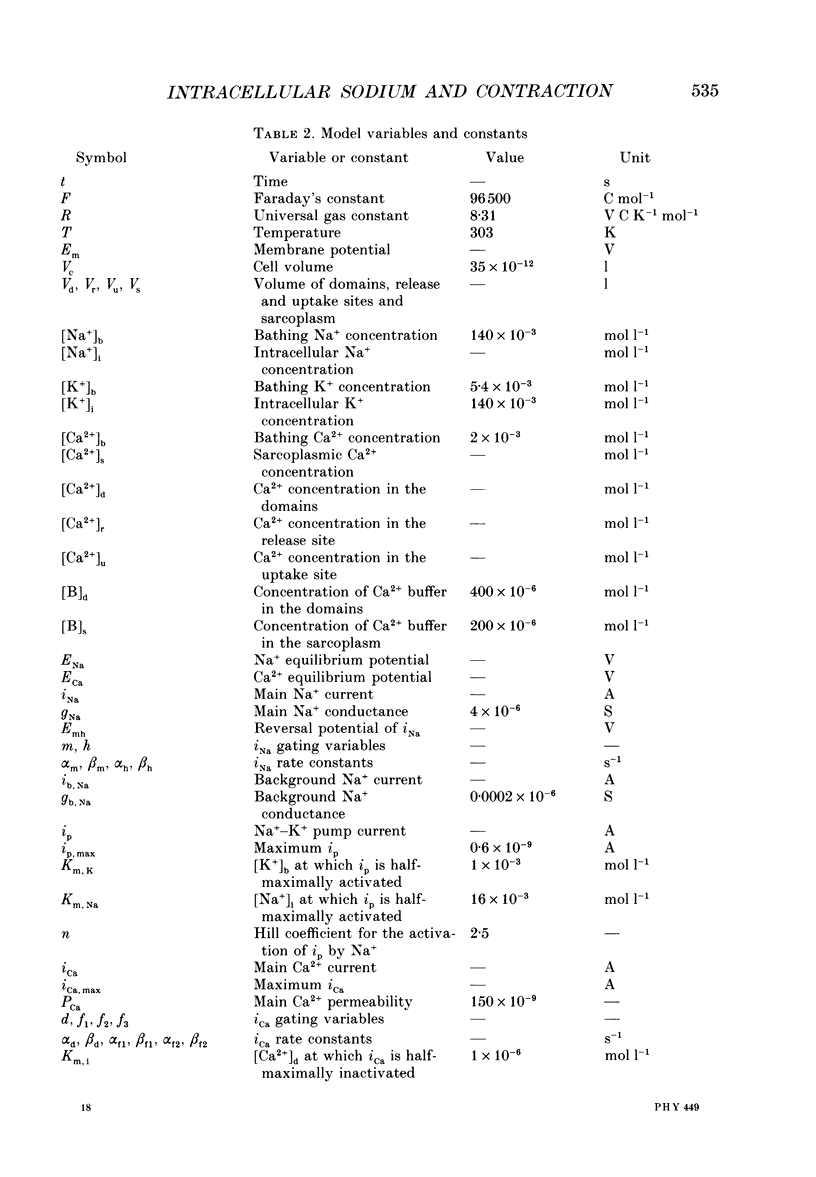
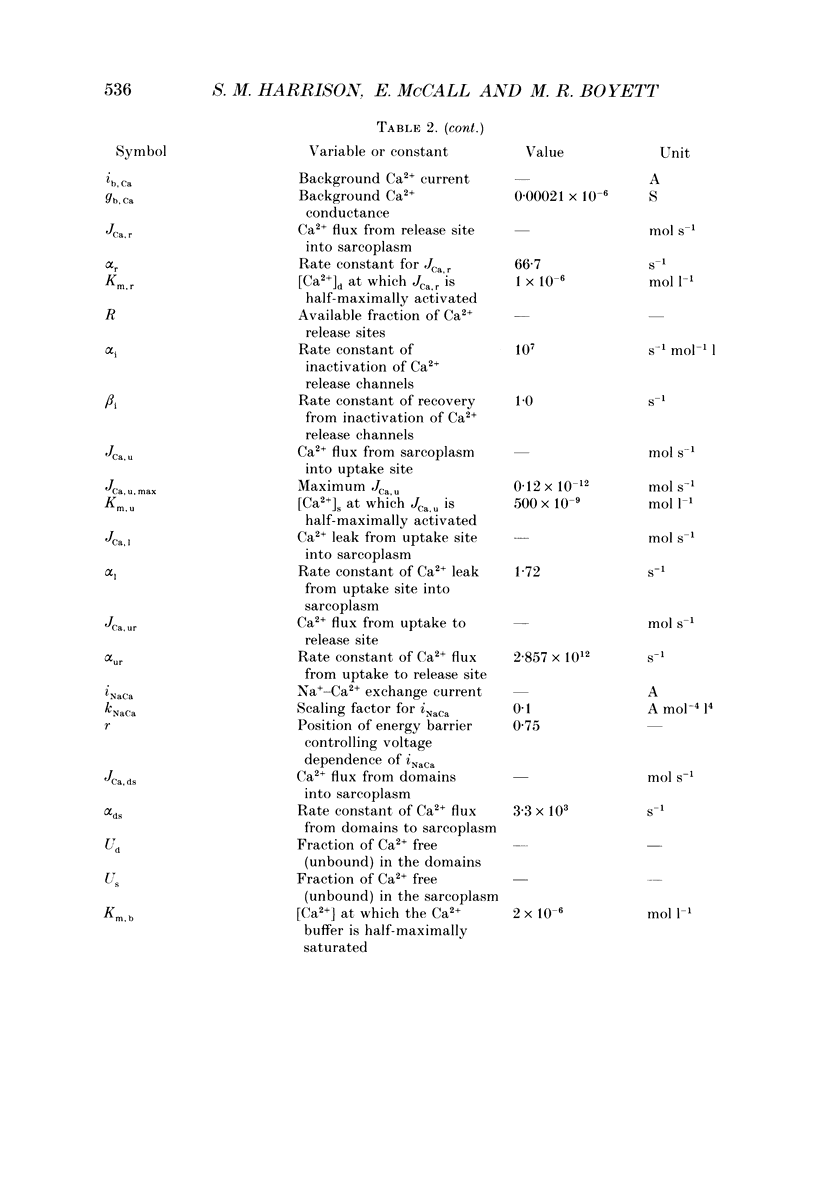
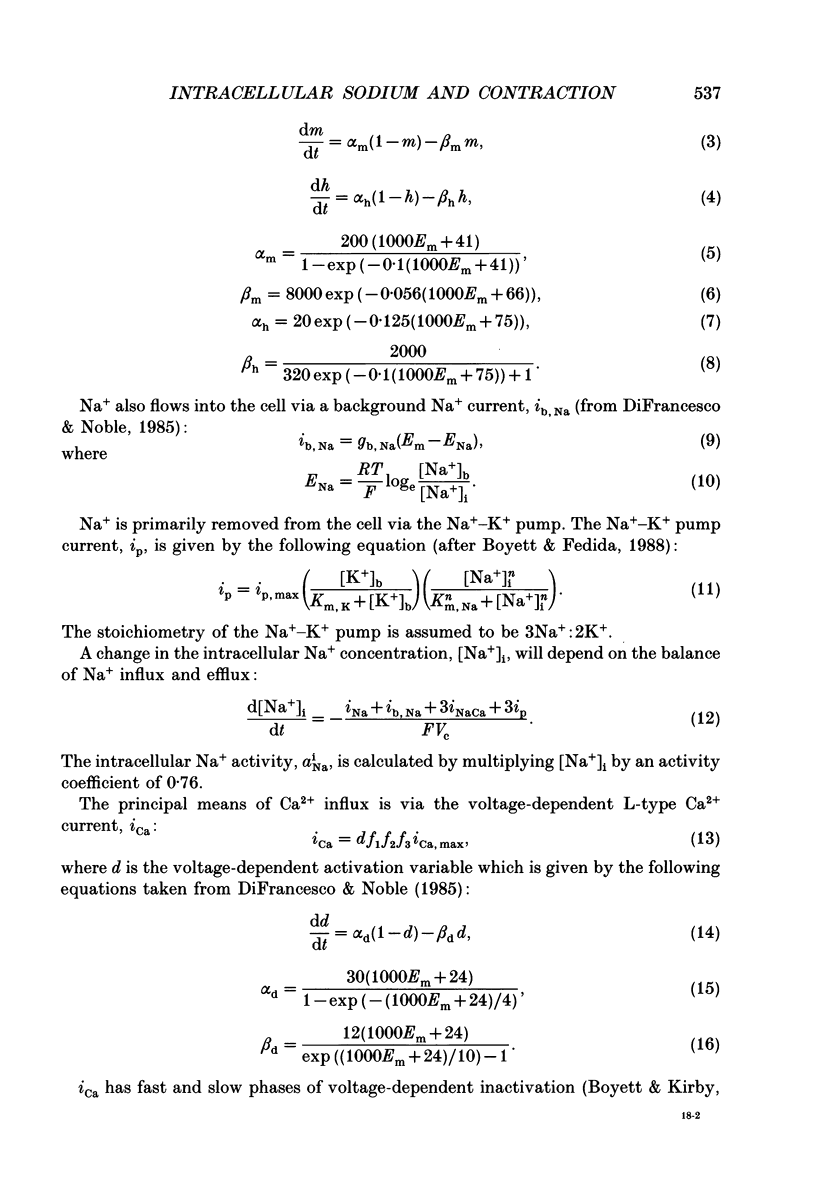
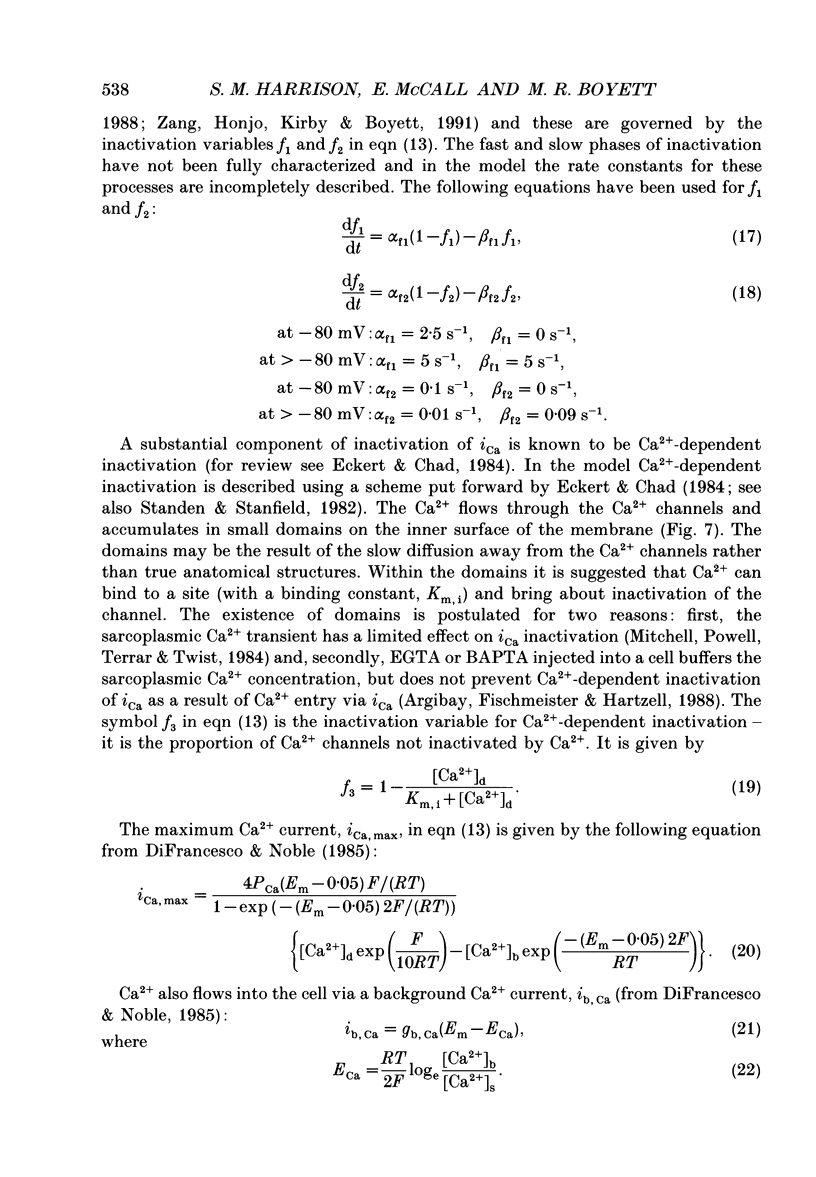
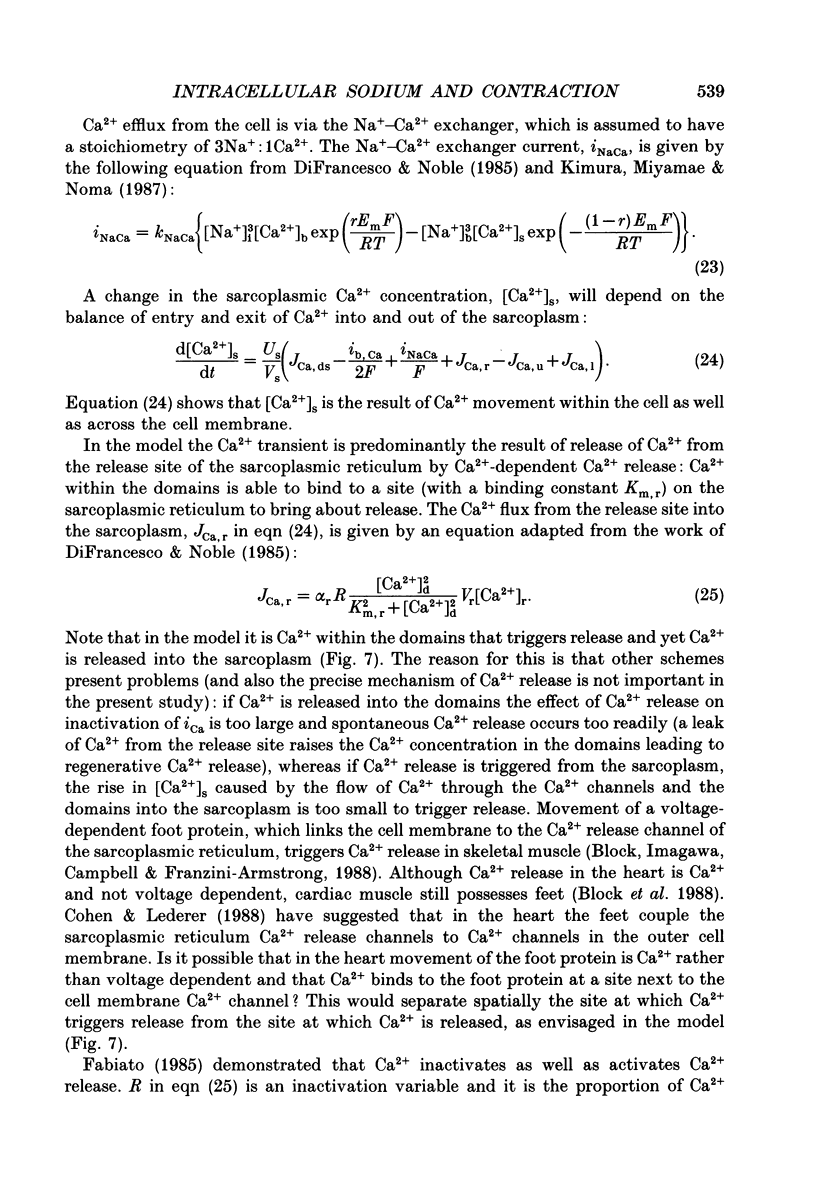
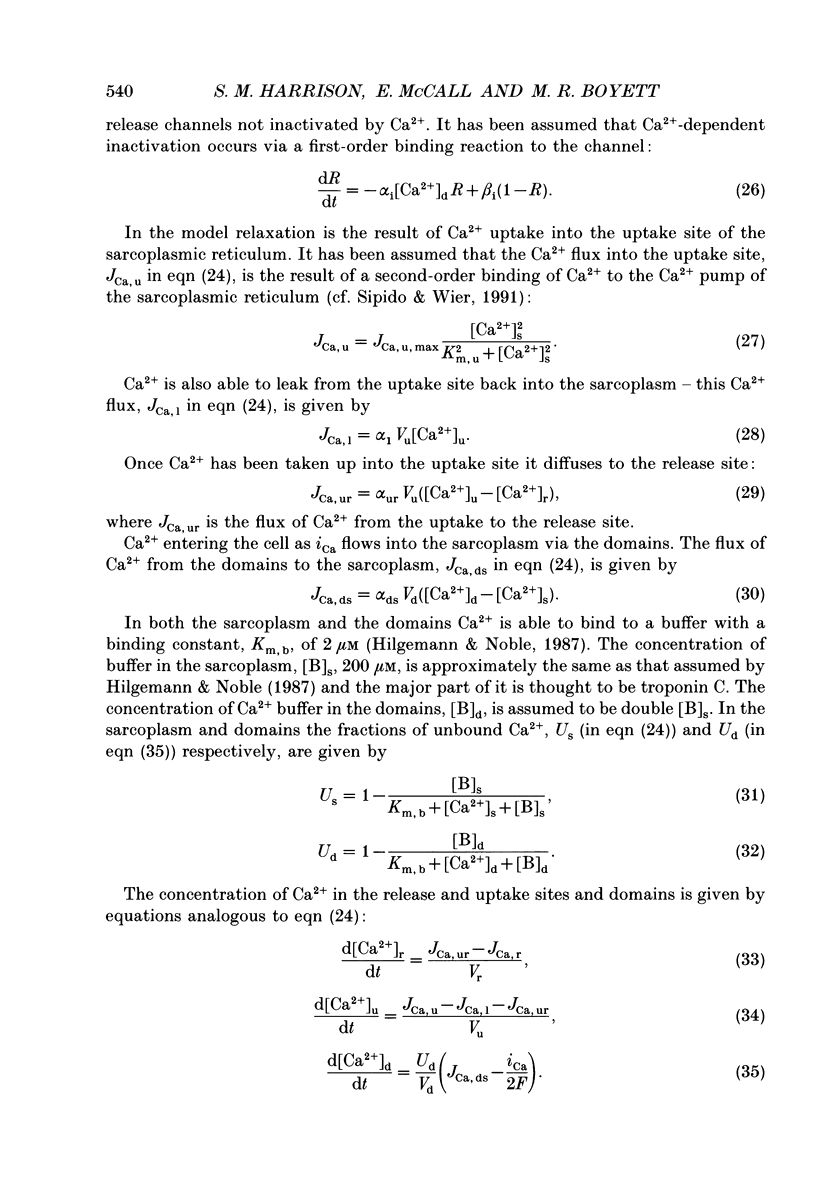
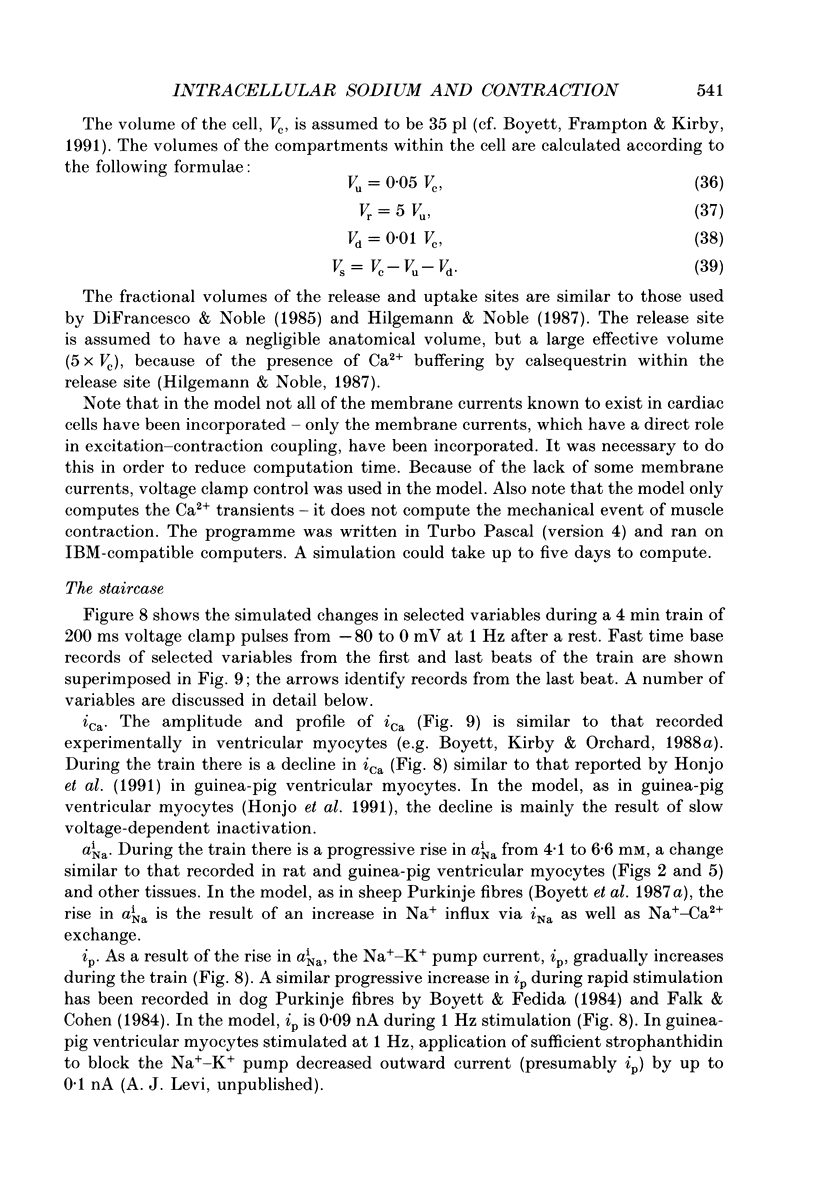
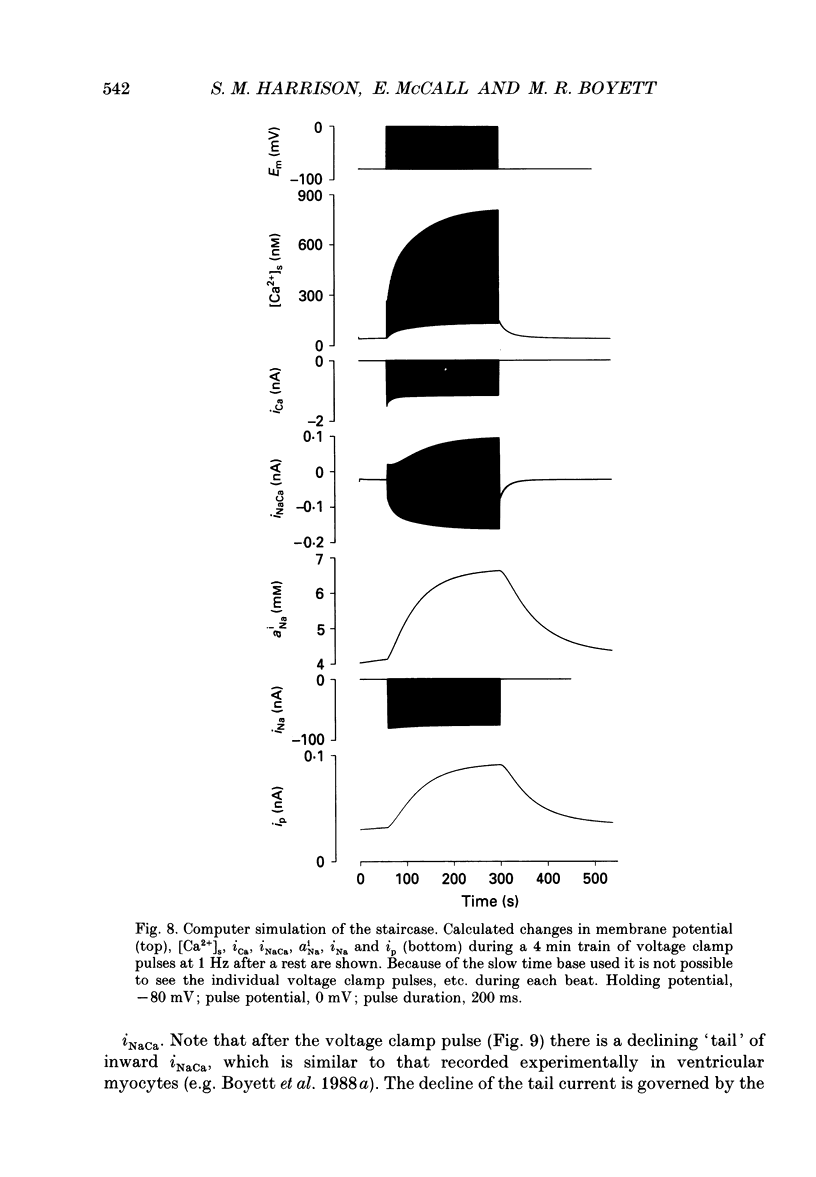
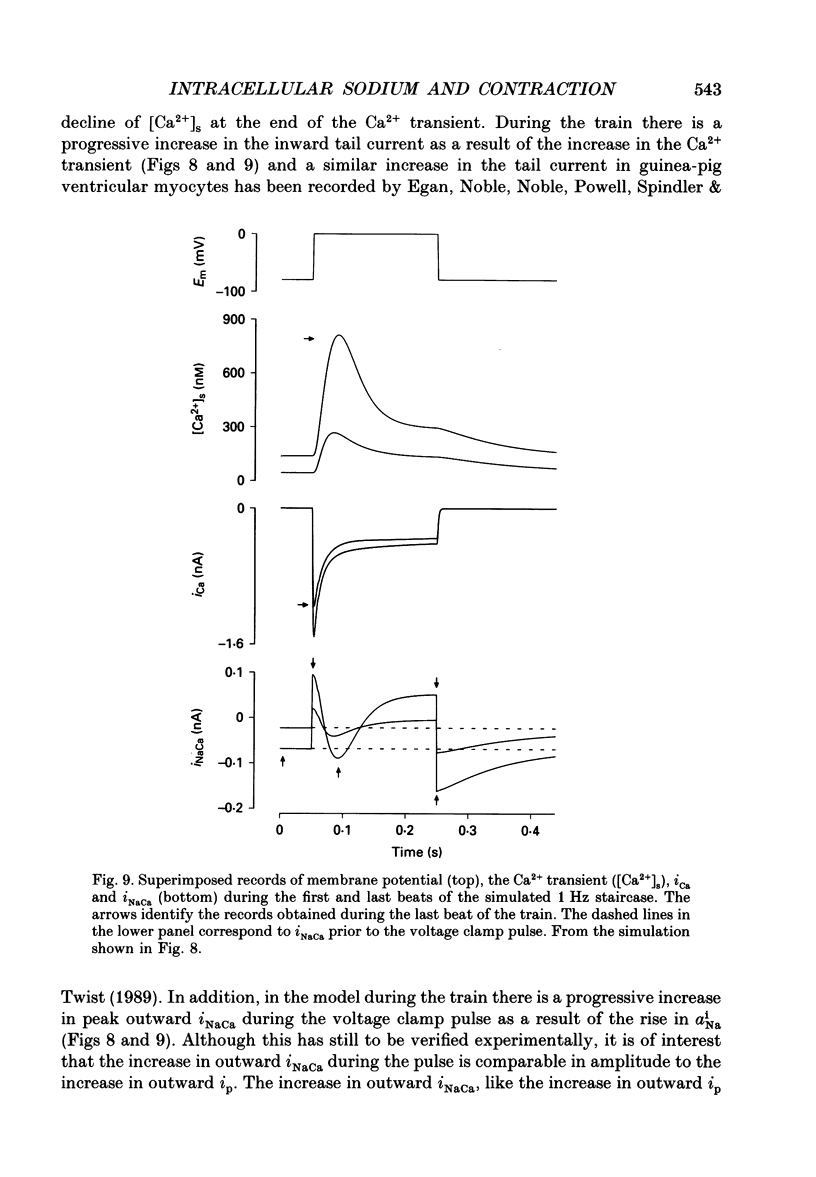
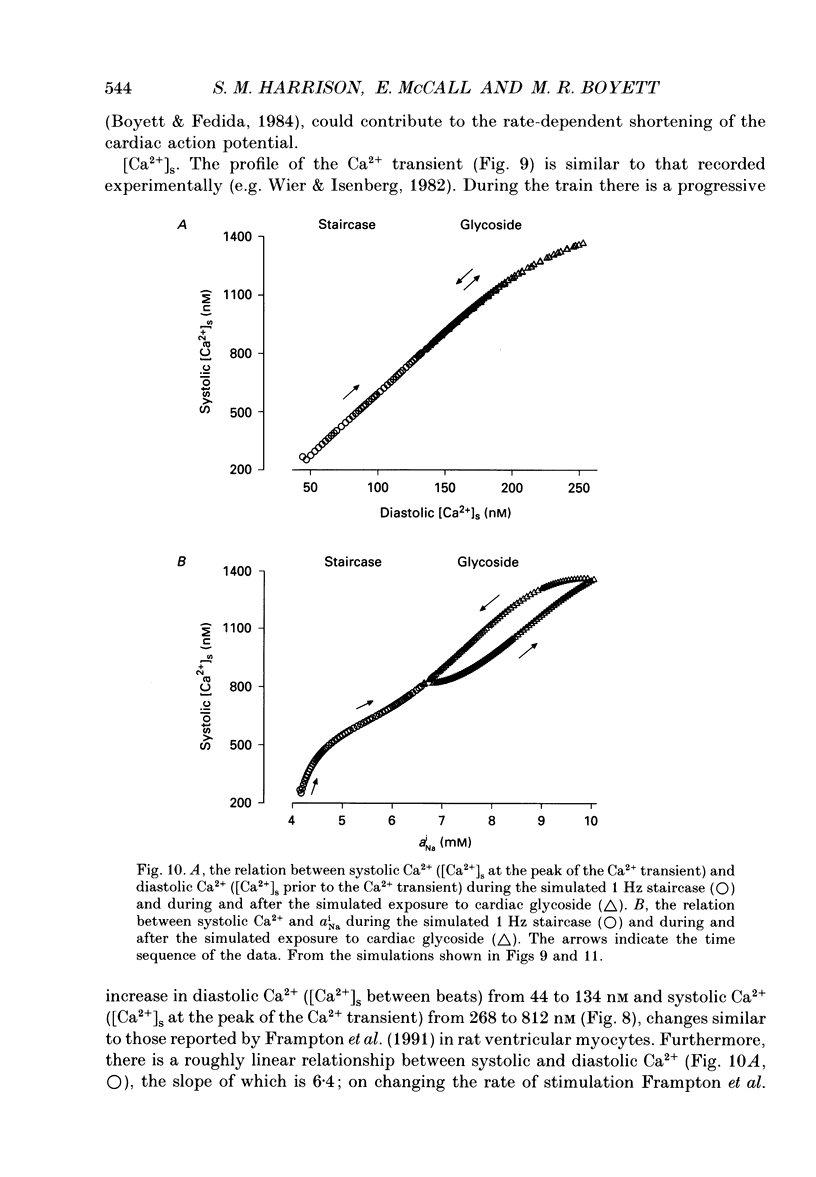
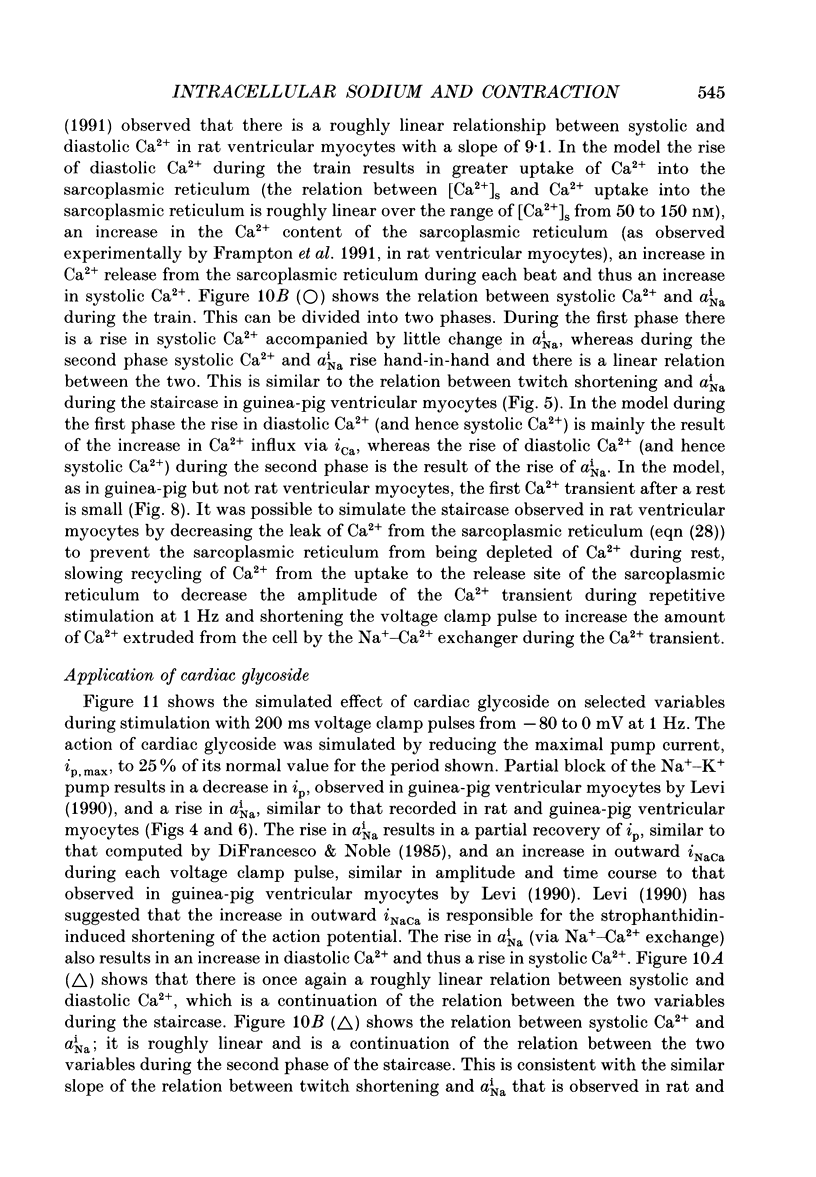
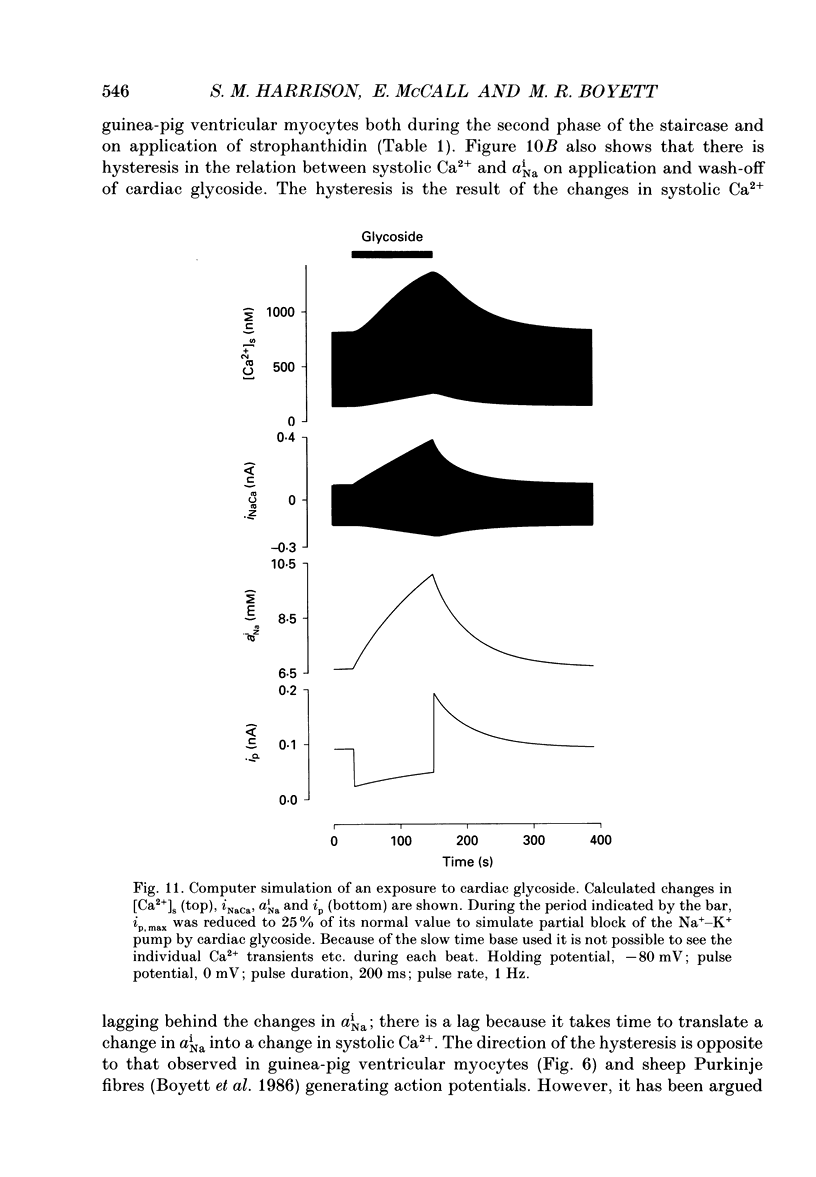
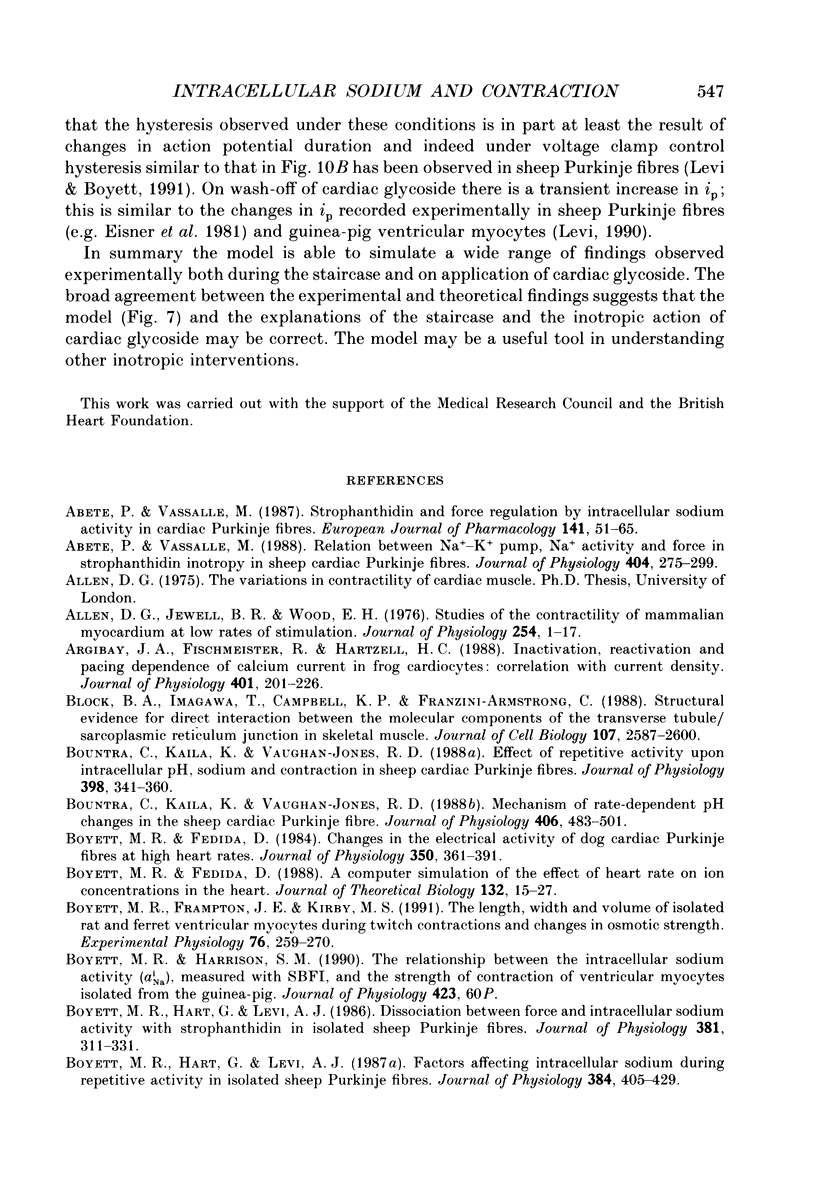
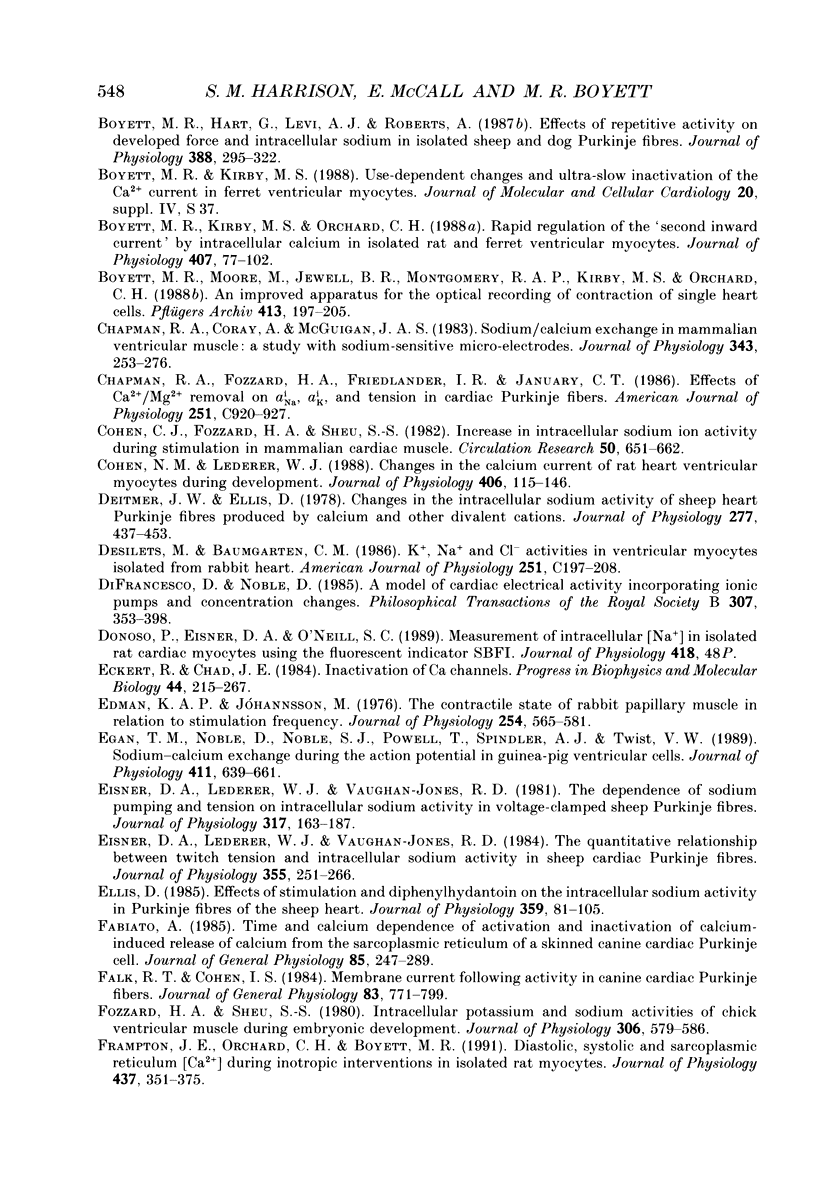
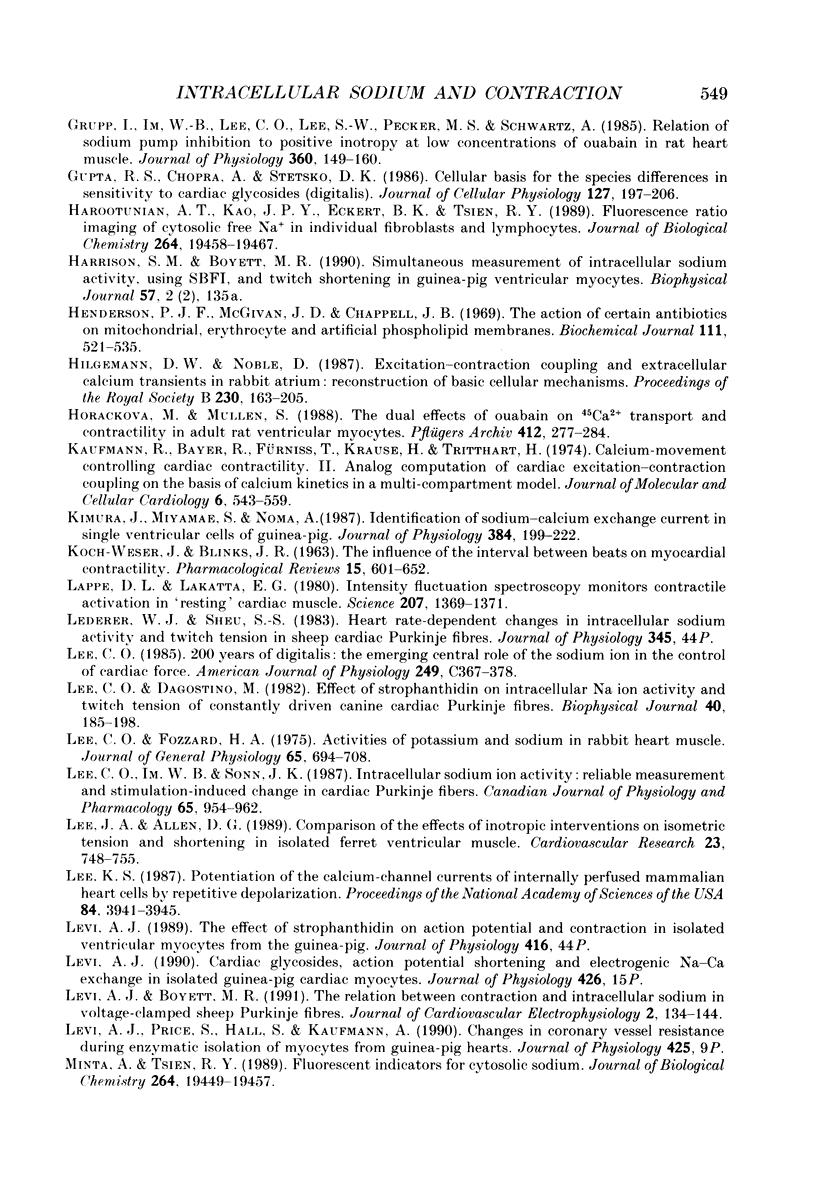
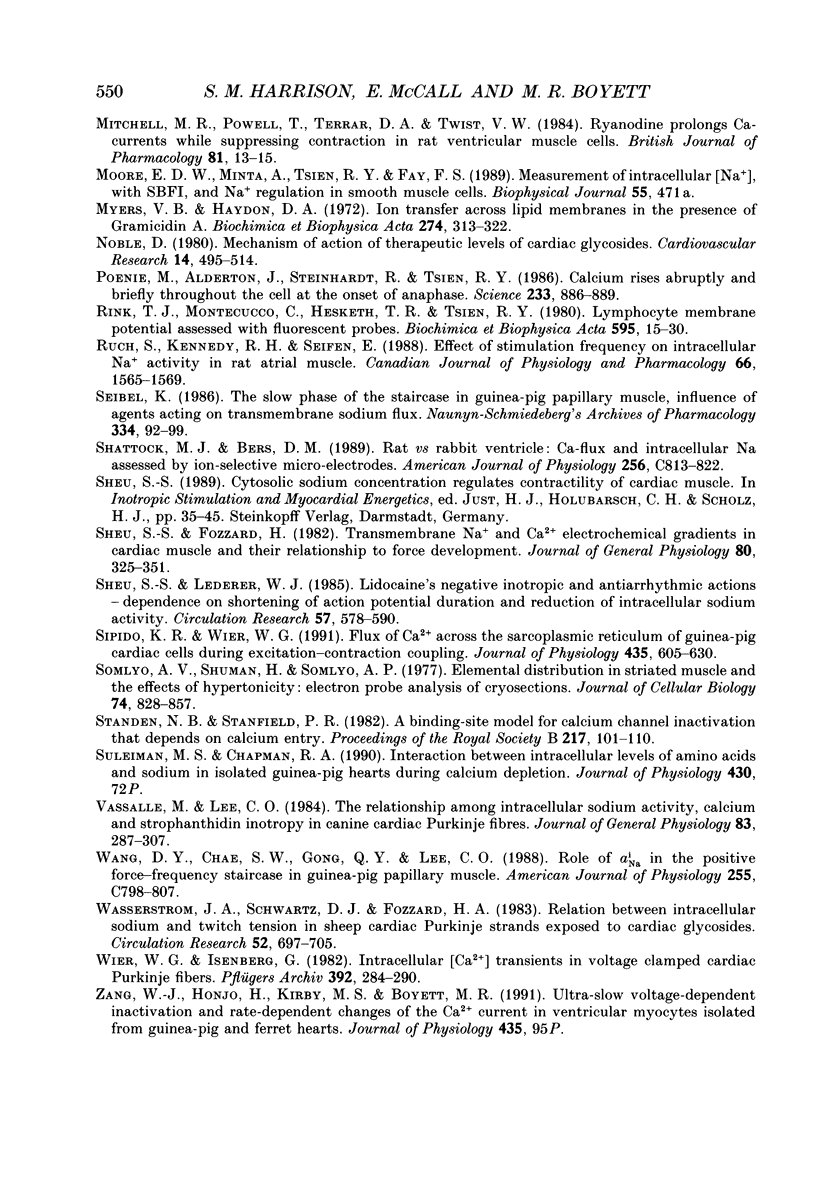
Selected References
These references are in PubMed. This may not be the complete list of references from this article.
- Abete P., Vassalle M. Relation between Na+-K+ pump, Na+ activity and force in strophanthidin inotropy in sheep cardiac Purkinje fibres. J Physiol. 1988 Oct;404:275–299. doi: 10.1113/jphysiol.1988.sp017290. [DOI] [PMC free article] [PubMed] [Google Scholar]
- Abete P., Vassalle M. Strophanthidin and force regulation by intracellular sodium activity in cardiac Purkinje fibers. Eur J Pharmacol. 1987 Sep 2;141(1):51–65. doi: 10.1016/0014-2999(87)90410-9. [DOI] [PubMed] [Google Scholar]
- Allen D. G., Jewell B. R., Wood E. H. Studies of the contractility of mammalian myocardium at low rates of stimulation. J Physiol. 1976 Jan;254(1):1–17. doi: 10.1113/jphysiol.1976.sp011217. [DOI] [PMC free article] [PubMed] [Google Scholar]
- Argibay J. A., Fischmeister R., Hartzell H. C. Inactivation, reactivation and pacing dependence of calcium current in frog cardiocytes: correlation with current density. J Physiol. 1988 Jul;401:201–226. doi: 10.1113/jphysiol.1988.sp017158. [DOI] [PMC free article] [PubMed] [Google Scholar]
- Block B. A., Imagawa T., Campbell K. P., Franzini-Armstrong C. Structural evidence for direct interaction between the molecular components of the transverse tubule/sarcoplasmic reticulum junction in skeletal muscle. J Cell Biol. 1988 Dec;107(6 Pt 2):2587–2600. doi: 10.1083/jcb.107.6.2587. [DOI] [PMC free article] [PubMed] [Google Scholar]
- Bountra C., Kaila K., Vaughan-Jones R. D. Effect of repetitive activity upon intracellular pH, sodium and contraction in sheep cardiac Purkinje fibres. J Physiol. 1988 Apr;398:341–360. doi: 10.1113/jphysiol.1988.sp017046. [DOI] [PMC free article] [PubMed] [Google Scholar]
- Bountra C., Kaila K., Vaughan-Jones R. D. Mechanism of rate-dependent pH changes in the sheep cardiac Purkinje fibre. J Physiol. 1988 Dec;406:483–501. doi: 10.1113/jphysiol.1988.sp017392. [DOI] [PMC free article] [PubMed] [Google Scholar]
- Boyett M. R., Fedida D. A computer simulation of the effect of heart rate on ion concentrations in the heart. J Theor Biol. 1988 May 7;132(1):15–27. doi: 10.1016/s0022-5193(88)80187-5. [DOI] [PubMed] [Google Scholar]
- Boyett M. R., Fedida D. Changes in the electrical activity of dog cardiac Purkinje fibres at high heart rates. J Physiol. 1984 May;350:361–391. doi: 10.1113/jphysiol.1984.sp015206. [DOI] [PMC free article] [PubMed] [Google Scholar]
- Boyett M. R., Frampton J. E., Kirby M. S. The length, width and volume of isolated rat and ferret ventricular myocytes during twitch contractions and changes in osmotic strength. Exp Physiol. 1991 Mar;76(2):259–270. doi: 10.1113/expphysiol.1991.sp003492. [DOI] [PubMed] [Google Scholar]
- Boyett M. R., Hart G., Levi A. J. Dissociation between force and intracellular sodium activity with strophanthidin in isolated sheep Purkinje fibres. J Physiol. 1986 Dec;381:311–331. doi: 10.1113/jphysiol.1986.sp016329. [DOI] [PMC free article] [PubMed] [Google Scholar]
- Boyett M. R., Hart G., Levi A. J. Factors affecting intracellular sodium during repetitive activity in isolated sheep Purkinje fibres. J Physiol. 1987 Mar;384:405–429. doi: 10.1113/jphysiol.1987.sp016461. [DOI] [PMC free article] [PubMed] [Google Scholar]
- Boyett M. R., Hart G., Levi A. J., Roberts A. Effects of repetitive activity on developed force and intracellular sodium in isolated sheep and dog Purkinje fibres. J Physiol. 1987 Jul;388:295–322. doi: 10.1113/jphysiol.1987.sp016616. [DOI] [PMC free article] [PubMed] [Google Scholar]
- Boyett M. R., Kirby M. S., Orchard C. H. Rapid regulation of the 'second inward current' by intracellular calcium in isolated rat and ferret ventricular myocytes. J Physiol. 1988 Dec;407:77–102. doi: 10.1113/jphysiol.1988.sp017404. [DOI] [PMC free article] [PubMed] [Google Scholar]
- Boyett M. R., Moore M., Jewell B. R., Montgomery R. A., Kirby M. S., Orchard C. H. An improved apparatus for the optical recording of contraction of single heart cells. Pflugers Arch. 1988 Dec;413(2):197–205. doi: 10.1007/BF00582531. [DOI] [PubMed] [Google Scholar]
- Chapman R. A., Coray A., McGuigan J. A. Sodium/calcium exchange in mammalian ventricular muscle: a study with sodium-sensitive micro-electrodes. J Physiol. 1983 Oct;343:253–276. doi: 10.1113/jphysiol.1983.sp014891. [DOI] [PMC free article] [PubMed] [Google Scholar]
- Chapman R. A., Fozzard H. A., Friedlander I. R., January C. T. Effects of Ca2+/Mg2+ removal on aiNa, aiK, and tension in cardiac Purkinje fibers. Am J Physiol. 1986 Dec;251(6 Pt 1):C920–C927. doi: 10.1152/ajpcell.1986.251.6.C920. [DOI] [PubMed] [Google Scholar]
- Cohen C. J., Fozzard H. A., Sheu S. S. Increase in intracellular sodium ion activity during stimulation in mammalian cardiac muscle. Circ Res. 1982 May;50(5):651–662. doi: 10.1161/01.res.50.5.651. [DOI] [PubMed] [Google Scholar]
- Cohen N. M., Lederer W. J. Changes in the calcium current of rat heart ventricular myocytes during development. J Physiol. 1988 Dec;406:115–146. doi: 10.1113/jphysiol.1988.sp017372. [DOI] [PMC free article] [PubMed] [Google Scholar]
- Deitmer J. W., Ellis D. Changes in the intracellular sodium activity of sheep heart Purkinje fibres produced by calcium and other divalent cations. J Physiol. 1978 Apr;277:437–453. doi: 10.1113/jphysiol.1978.sp012283. [DOI] [PMC free article] [PubMed] [Google Scholar]
- DiFrancesco D., Noble D. A model of cardiac electrical activity incorporating ionic pumps and concentration changes. Philos Trans R Soc Lond B Biol Sci. 1985 Jan 10;307(1133):353–398. doi: 10.1098/rstb.1985.0001. [DOI] [PubMed] [Google Scholar]
- Désilets M., Baumgarten C. M. K+, Na+, and Cl- activities in ventricular myocytes isolated from rabbit heart. Am J Physiol. 1986 Aug;251(2 Pt 1):C197–C208. doi: 10.1152/ajpcell.1986.251.2.C197. [DOI] [PubMed] [Google Scholar]
- Eckert R., Chad J. E. Inactivation of Ca channels. Prog Biophys Mol Biol. 1984;44(3):215–267. doi: 10.1016/0079-6107(84)90009-9. [DOI] [PubMed] [Google Scholar]
- Edman K. A., Jóhannsson M. The contractile state of rabbit papillary muscle in relation to stimulation frequency. J Physiol. 1976 Jan;254(3):565–581. doi: 10.1113/jphysiol.1976.sp011247. [DOI] [PMC free article] [PubMed] [Google Scholar]
- Egan T. M., Noble D., Noble S. J., Powell T., Spindler A. J., Twist V. W. Sodium-calcium exchange during the action potential in guinea-pig ventricular cells. J Physiol. 1989 Apr;411:639–661. doi: 10.1113/jphysiol.1989.sp017596. [DOI] [PMC free article] [PubMed] [Google Scholar]
- Eisner D. A., Lederer W. J., Vaughan-Jones R. D. The dependence of sodium pumping and tension on intracellular sodium activity in voltage-clamped sheep Purkinje fibres. J Physiol. 1981 Aug;317:163–187. doi: 10.1113/jphysiol.1981.sp013819. [DOI] [PMC free article] [PubMed] [Google Scholar]
- Eisner D. A., Lederer W. J., Vaughan-Jones R. D. The quantitative relationship between twitch tension and intracellular sodium activity in sheep cardiac Purkinje fibres. J Physiol. 1984 Oct;355:251–266. doi: 10.1113/jphysiol.1984.sp015417. [DOI] [PMC free article] [PubMed] [Google Scholar]
- Ellis D., MacLeod K. T. Sodium-dependent control of intracellular pH in Purkinje fibres of sheep heart. J Physiol. 1985 Feb;359:81–105. doi: 10.1113/jphysiol.1985.sp015576. [DOI] [PMC free article] [PubMed] [Google Scholar]
- Fabiato A. Time and calcium dependence of activation and inactivation of calcium-induced release of calcium from the sarcoplasmic reticulum of a skinned canine cardiac Purkinje cell. J Gen Physiol. 1985 Feb;85(2):247–289. doi: 10.1085/jgp.85.2.247. [DOI] [PMC free article] [PubMed] [Google Scholar]
- Falk R. T., Cohen I. S. Membrane current following activity in canine cardiac Purkinje fibers. J Gen Physiol. 1984 May;83(5):771–799. doi: 10.1085/jgp.83.5.771. [DOI] [PMC free article] [PubMed] [Google Scholar]
- Fozzard H. A., Sheu S. S. Intracellular potassium and sodium activities of chick ventricular muscle during embryonic development. J Physiol. 1980 Sep;306:579–586. doi: 10.1113/jphysiol.1980.sp013416. [DOI] [PMC free article] [PubMed] [Google Scholar]
- Frampton J. E., Orchard C. H., Boyett M. R. Diastolic, systolic and sarcoplasmic reticulum [Ca2+] during inotropic interventions in isolated rat myocytes. J Physiol. 1991 Jun;437:351–375. doi: 10.1113/jphysiol.1991.sp018600. [DOI] [PMC free article] [PubMed] [Google Scholar]
- Grupp I., Im W. B., Lee C. O., Lee S. W., Pecker M. S., Schwartz A. Relation of sodium pump inhibition to positive inotropy at low concentrations of ouabain in rat heart muscle. J Physiol. 1985 Mar;360:149–160. doi: 10.1113/jphysiol.1985.sp015609. [DOI] [PMC free article] [PubMed] [Google Scholar]
- Gupta R. S., Chopra A., Stetsko D. K. Cellular basis for the species differences in sensitivity to cardiac glycosides (digitalis). J Cell Physiol. 1986 May;127(2):197–206. doi: 10.1002/jcp.1041270202. [DOI] [PubMed] [Google Scholar]
- Harootunian A. T., Kao J. P., Eckert B. K., Tsien R. Y. Fluorescence ratio imaging of cytosolic free Na+ in individual fibroblasts and lymphocytes. J Biol Chem. 1989 Nov 15;264(32):19458–19467. [PubMed] [Google Scholar]
- Henderson P. J., McGivan J. D., Chappell J. B. The action of certain antibiotics on mitochondrial, erythrocyte and artificial phospholipid membranes. The role of induced proton permeability. Biochem J. 1969 Feb;111(4):521–535. doi: 10.1042/bj1110521. [DOI] [PMC free article] [PubMed] [Google Scholar]
- Hilgemann D. W., Noble D. Excitation-contraction coupling and extracellular calcium transients in rabbit atrium: reconstruction of basic cellular mechanisms. Proc R Soc Lond B Biol Sci. 1987 Mar 23;230(1259):163–205. doi: 10.1098/rspb.1987.0015. [DOI] [PubMed] [Google Scholar]
- Horackova M., Mullen S. The dual effects of ouabain on 45Ca2+ transport and contractility in adult rat ventricular myocytes. Pflugers Arch. 1988 Aug;412(3):277–284. doi: 10.1007/BF00582509. [DOI] [PubMed] [Google Scholar]
- KOCH-WESER J., BLINKS J. R. THE INFLUENCE OF THE INTERVAL BETWEEN BEATS ON MYOCARDIAL CONTRACTILITY. Pharmacol Rev. 1963 Sep;15:601–652. [PubMed] [Google Scholar]
- Kaufmann R., Bayer R., Fürniss T., Krause H., Tritthart H. Calcium-movement controlling cardiac contractility II. Analog computation of cardiac excitation-contraction coupling on the basis of calcium kinetics in a multi-compartment model. J Mol Cell Cardiol. 1974 Dec;6(6):543–559. doi: 10.1016/0022-2828(74)90035-2. [DOI] [PubMed] [Google Scholar]
- Kimura J., Miyamae S., Noma A. Identification of sodium-calcium exchange current in single ventricular cells of guinea-pig. J Physiol. 1987 Mar;384:199–222. doi: 10.1113/jphysiol.1987.sp016450. [DOI] [PMC free article] [PubMed] [Google Scholar]
- Lappé D. L., Lakatta E. G. Intensity fluctuation spectroscopy monitors contractile activation in "resting" cardiac muscle. Science. 1980 Mar 21;207(4437):1369–1371. doi: 10.1126/science.7355295. [DOI] [PubMed] [Google Scholar]
- Lee C. O. 200 years of digitalis: the emerging central role of the sodium ion in the control of cardiac force. Am J Physiol. 1985 Nov;249(5 Pt 1):C367–C378. doi: 10.1152/ajpcell.1985.249.5.C367. [DOI] [PubMed] [Google Scholar]
- Lee C. O., Dagostino M. Effect of strophanthidin on intracellular Na ion activity and twitch tension of constantly driven canine cardiac Purkinje fibers. Biophys J. 1982 Dec;40(3):185–198. doi: 10.1016/S0006-3495(82)84474-3. [DOI] [PMC free article] [PubMed] [Google Scholar]
- Lee C. O., Fozzard H. A. Activities of potassium and sodium ions in rabbit heart muscle. J Gen Physiol. 1975 Jun;65(6):695–708. doi: 10.1085/jgp.65.6.695. [DOI] [PMC free article] [PubMed] [Google Scholar]
- Lee C. O., Im W. B., Sonn J. K. Intracellular sodium ion activity: reliable measurement and stimulation-induced change in cardiac Purkinje fibers. Can J Physiol Pharmacol. 1987 May;65(5):954–962. doi: 10.1139/y87-152. [DOI] [PubMed] [Google Scholar]
- Lee J. A., Allen D. G. Comparison of the effects of inotropic interventions on isometric tension and shortening in isolated ferret ventricular muscle. Cardiovasc Res. 1989 Sep;23(9):748–755. doi: 10.1093/cvr/23.9.748. [DOI] [PubMed] [Google Scholar]
- Minta A., Tsien R. Y. Fluorescent indicators for cytosolic sodium. J Biol Chem. 1989 Nov 15;264(32):19449–19457. [PubMed] [Google Scholar]
- Mitchell M. R., Powell T., Terrar D. A., Twist V. W. Ryanodine prolongs Ca-currents while suppressing contraction in rat ventricular muscle cells. Br J Pharmacol. 1984 Jan;81(1):13–15. doi: 10.1111/j.1476-5381.1984.tb10735.x. [DOI] [PMC free article] [PubMed] [Google Scholar]
- Myers V. B., Haydon D. A. Ion transfer across lipid membranes in the presence of gramicidin A. II. The ion selectivity. Biochim Biophys Acta. 1972 Aug 9;274(2):313–322. doi: 10.1016/0005-2736(72)90179-4. [DOI] [PubMed] [Google Scholar]
- Noble D. Mechanism of action of therapeutic levels of cardiac glycosides. Cardiovasc Res. 1980 Sep;14(9):495–514. doi: 10.1093/cvr/14.9.495. [DOI] [PubMed] [Google Scholar]
- Poenie M., Alderton J., Steinhardt R., Tsien R. Calcium rises abruptly and briefly throughout the cell at the onset of anaphase. Science. 1986 Aug 22;233(4766):886–889. doi: 10.1126/science.3755550. [DOI] [PubMed] [Google Scholar]
- Rink T. J., Montecucco C., Hesketh T. R., Tsien R. Y. Lymphocyte membrane potential assessed with fluorescent probes. Biochim Biophys Acta. 1980;595(1):15–30. doi: 10.1016/0005-2736(80)90243-6. [DOI] [PubMed] [Google Scholar]
- Ruch S., Kennedy R. H., Seifen E. Effect of stimulation frequency on intracellular Na+ activity in rat atrial muscle. Can J Physiol Pharmacol. 1988 Dec;66(12):1565–1569. doi: 10.1139/y88-256. [DOI] [PubMed] [Google Scholar]
- Seibel K. The slow phase of the staircase in guinea-pig papillary muscle, influence of agents acting on transmembrane sodium flux. Naunyn Schmiedebergs Arch Pharmacol. 1986 Sep;334(1):92–99. doi: 10.1007/BF00498745. [DOI] [PubMed] [Google Scholar]
- Shattock M. J., Bers D. M. Rat vs. rabbit ventricle: Ca flux and intracellular Na assessed by ion-selective microelectrodes. Am J Physiol. 1989 Apr;256(4 Pt 1):C813–C822. doi: 10.1152/ajpcell.1989.256.4.C813. [DOI] [PubMed] [Google Scholar]
- Sheu S. S., Fozzard H. A. Transmembrane Na+ and Ca2+ electrochemical gradients in cardiac muscle and their relationship to force development. J Gen Physiol. 1982 Sep;80(3):325–351. doi: 10.1085/jgp.80.3.325. [DOI] [PMC free article] [PubMed] [Google Scholar]
- Sheu S. S., Lederer W. J. Lidocaine's negative inotropic and antiarrhythmic actions. Dependence on shortening of action potential duration and reduction of intracellular sodium activity. Circ Res. 1985 Oct;57(4):578–590. doi: 10.1161/01.res.57.4.578. [DOI] [PubMed] [Google Scholar]
- Sipido K. R., Wier W. G. Flux of Ca2+ across the sarcoplasmic reticulum of guinea-pig cardiac cells during excitation-contraction coupling. J Physiol. 1991 Apr;435:605–630. doi: 10.1113/jphysiol.1991.sp018528. [DOI] [PMC free article] [PubMed] [Google Scholar]
- Somlyo A. V., Shuman H., Somlyo A. P. Elemental distribution in striated muscle and the effects of hypertonicity. Electron probe analysis of cryo sections. J Cell Biol. 1977 Sep;74(3):828–857. doi: 10.1083/jcb.74.3.828. [DOI] [PMC free article] [PubMed] [Google Scholar]
- Standen N. B., Stanfield P. R. A binding-site model for calcium channel inactivation that depends on calcium entry. Proc R Soc Lond B Biol Sci. 1982 Dec 22;217(1206):101–110. doi: 10.1098/rspb.1982.0097. [DOI] [PubMed] [Google Scholar]
- Vassalle M., Lee C. O. The relationship among intracellular sodium activity, calcium, and strophanthidin inotropy in canine cardiac Purkinje fibers. J Gen Physiol. 1984 Feb;83(2):287–307. doi: 10.1085/jgp.83.2.287. [DOI] [PMC free article] [PubMed] [Google Scholar]
- Wang D. Y., Chae S. W., Gong Q. Y., Lee C. O. Role of aiNa in positive force-frequency staircase in guinea pig papillary muscle. Am J Physiol. 1988 Dec;255(6 Pt 1):C798–C807. doi: 10.1152/ajpcell.1988.255.6.C798. [DOI] [PubMed] [Google Scholar]
- Wasserstrom J. A., Schwartz D. J., Fozzard H. A. Relation between intracellular sodium and twitch tension in sheep cardiac Purkinje strands exposed to cardiac glycosides. Circ Res. 1983 Jun;52(6):697–705. doi: 10.1161/01.res.52.6.697. [DOI] [PubMed] [Google Scholar]
- Wier W. G., Isenberg G. Intracellular [Ca2+] transients in voltage clamped cardiac Purkinje fibers. Pflugers Arch. 1982 Jan;392(3):284–290. doi: 10.1007/BF00584312. [DOI] [PubMed] [Google Scholar]