Abstract
1. In the accompanying paper (Duchen & Biscoe, 1992) we have described graded changes in autofluorescence derived from mitochondrial NAD(P)H in type I cells of the carotid body in response to changes of PO2 over a physiologically significant range. These observations suggest that mitochondrial function in these cells is unusually sensitive to oxygen and could play a role in oxygen sensing. We have now explored further the relationships between hypoxia, mitochondrial membrane potential (delta psi m) and [Ca2+]i. 2. The fluorescence of Rhodamine 123 (Rh 123) accumulated within mitochondria is quenched by delta psi m. Mitochondrial depolarization thus increases the fluorescence signal. Blockade of electron transport (CN-, anoxia, rotenone) and uncoupling agents (e.g. carbonyl cyanide p-trifluoromethoxy-phenylhydrazone; FCCP) increased fluorescence by up to 80-120%, while fluorescence was reduced by blockade of the F0 proton channel of the mitochondrial ATP synthase complex (oligomycin). 3. delta psi m depolarized rapidly with anoxia, and was usually completely dissipated within 1-2 min. The depolarization of delta psi m with anoxia (or CN-) and repolarization on reoxygenation both followed a time course well characterized as the sum of two exponential processes. Oligomycin (0.2-2 micrograms/ml) hyperpolarized delta psi m and abolished the slower components of both the depolarization with anoxia and of the subsequent repolarization. These data (i) illustrate the role of the F1-F0 ATP synthetase in slowing the rate of dissipation of delta psi m on cessation of electron transport, (ii) confirm blockade of the ATP synthetase by oligomycin at these concentrations, and (iii) indicate significant accumulation of intramitochondrial ADP during 1-2 min of anoxia. 4. Depolarization of delta psi m was graded with graded changes in PO2 below about 60 mmHg. The stimulus-response curves thus constructed strongly resemble those for [Ca2+]i and NAD(P)H with PO2. The change in delta psi m closely followed changes in PO2 with time. 5. The rate of rise of [Ca2+]i in response to anoxia is strongly temperature sensitive. The rate of depolarization of delta psi m with anoxia similarly increased at least two- to fivefold on warming from 22 to 36 degrees C. The change with FCCP was not significantly altered by temperature. 6. These data show that the mitochondrial membrane potential changes over a physiological range of PO2 values in type I cells. This contrasts with the behaviour in dissociated chromaffin cells and sensory neurons, in which no change was measurable until the PO2 fell close to zero.(ABSTRACT TRUNCATED AT 400 WORDS)
Full text
PDF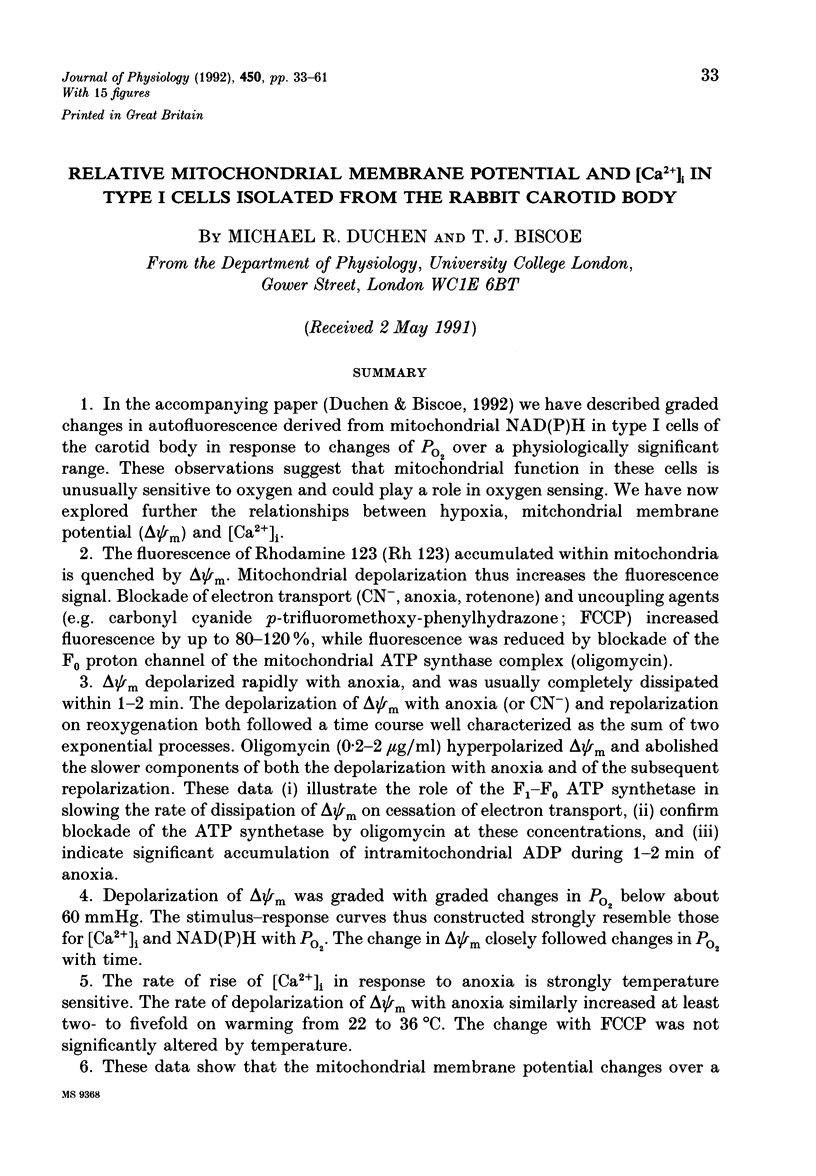
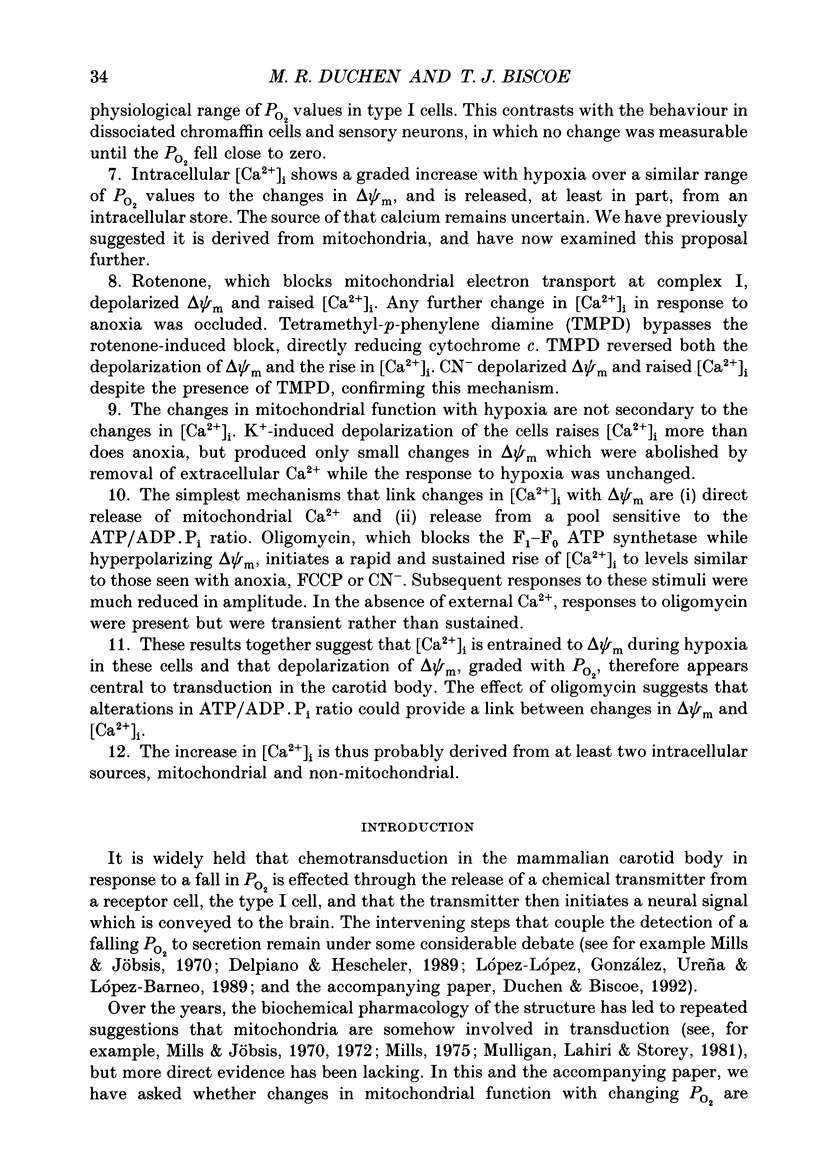
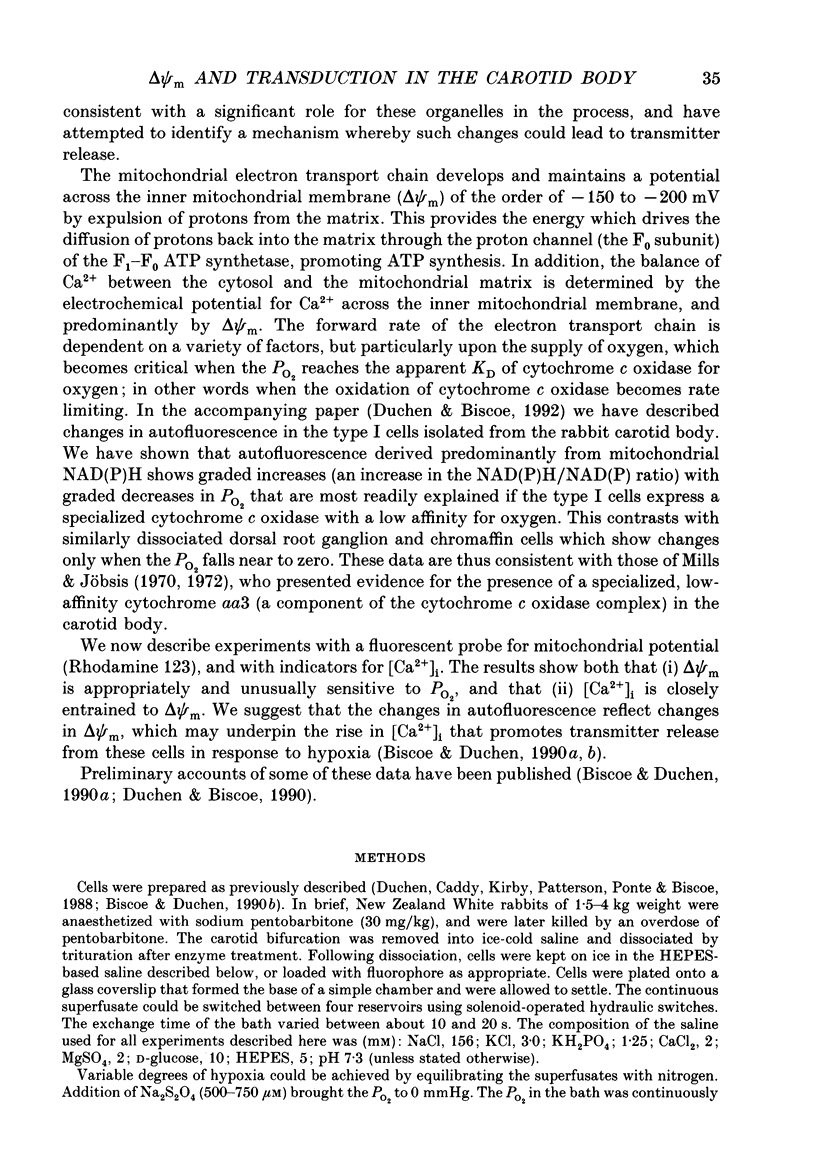
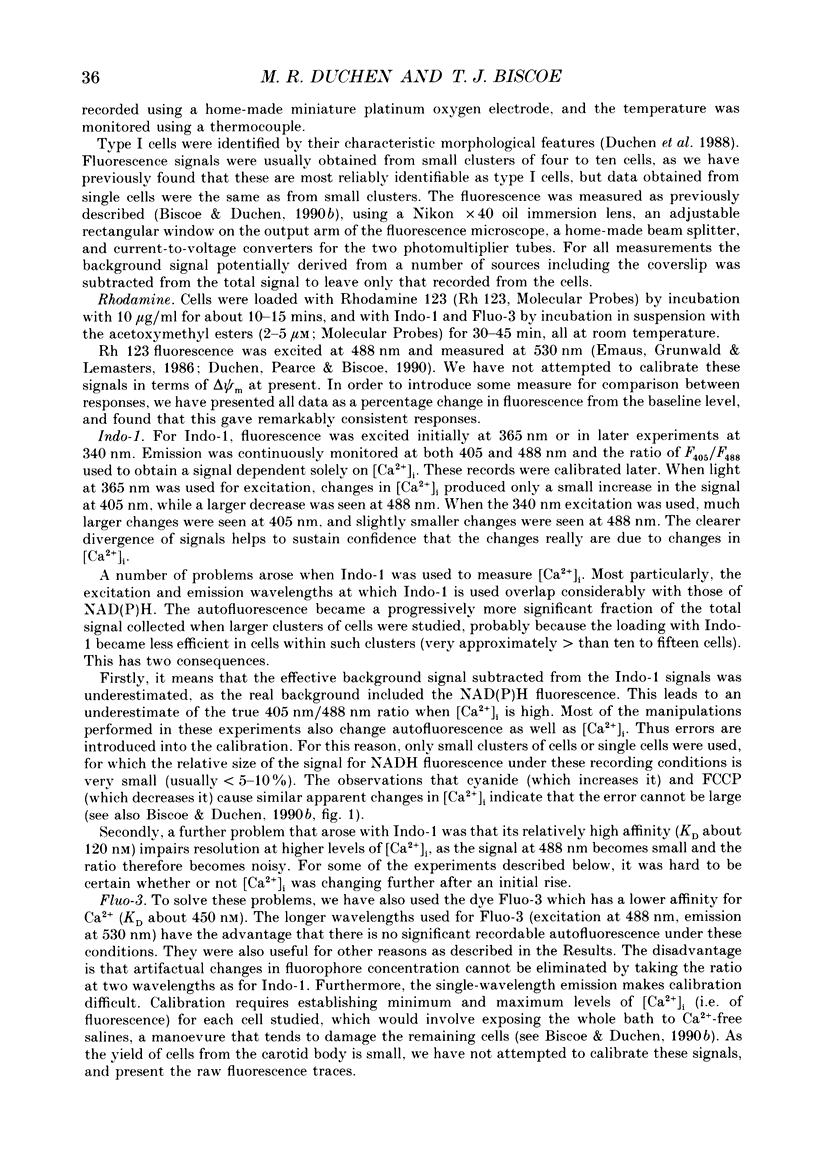
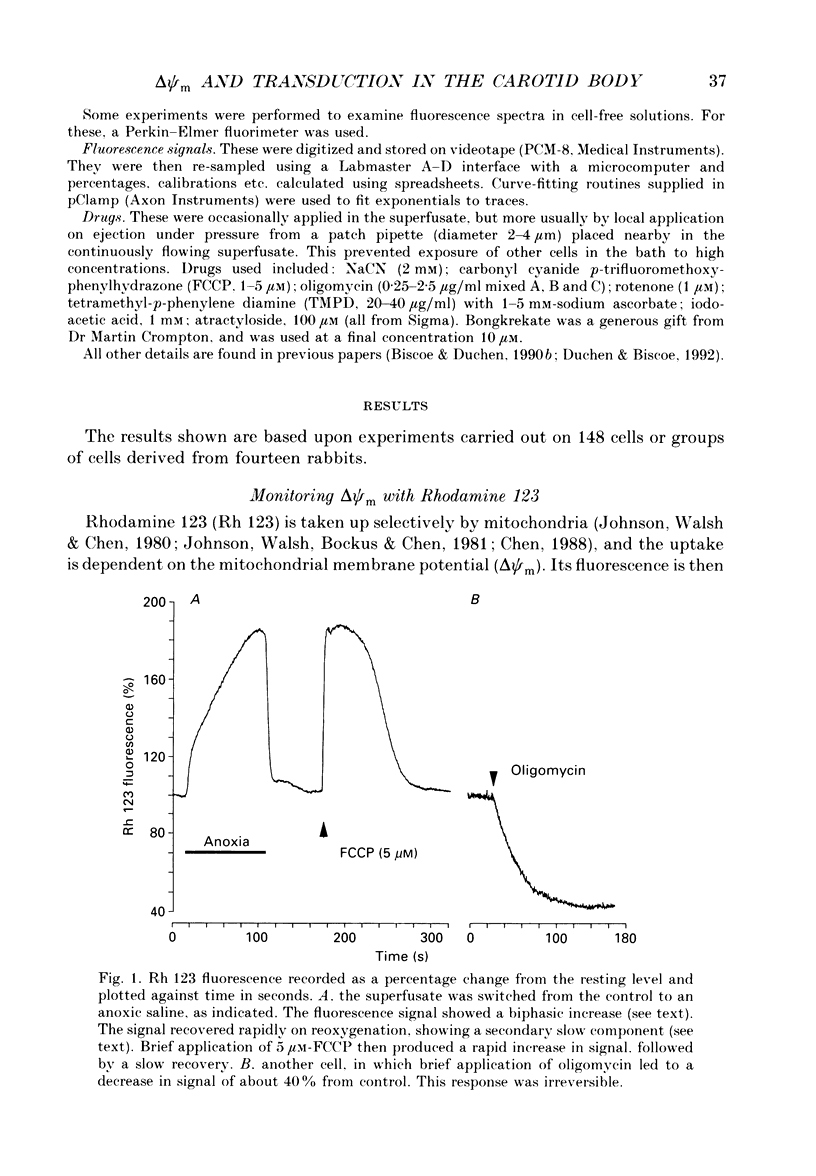
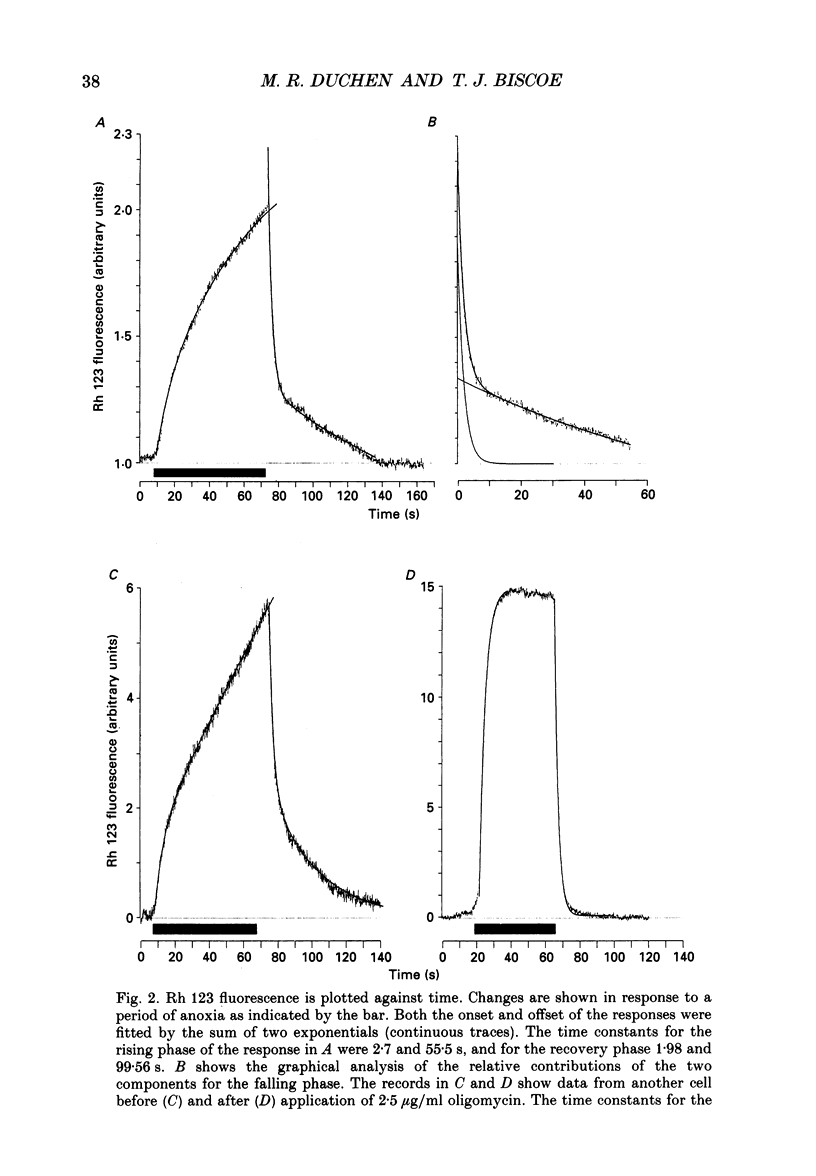
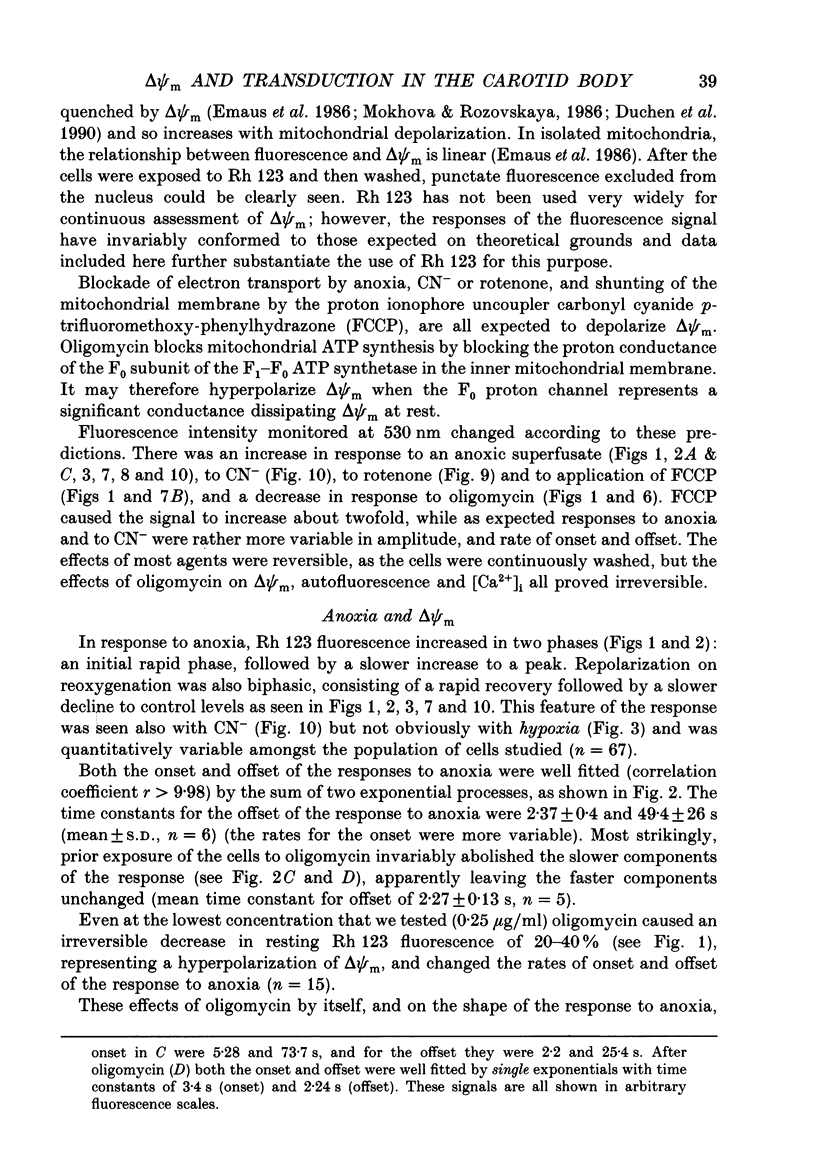
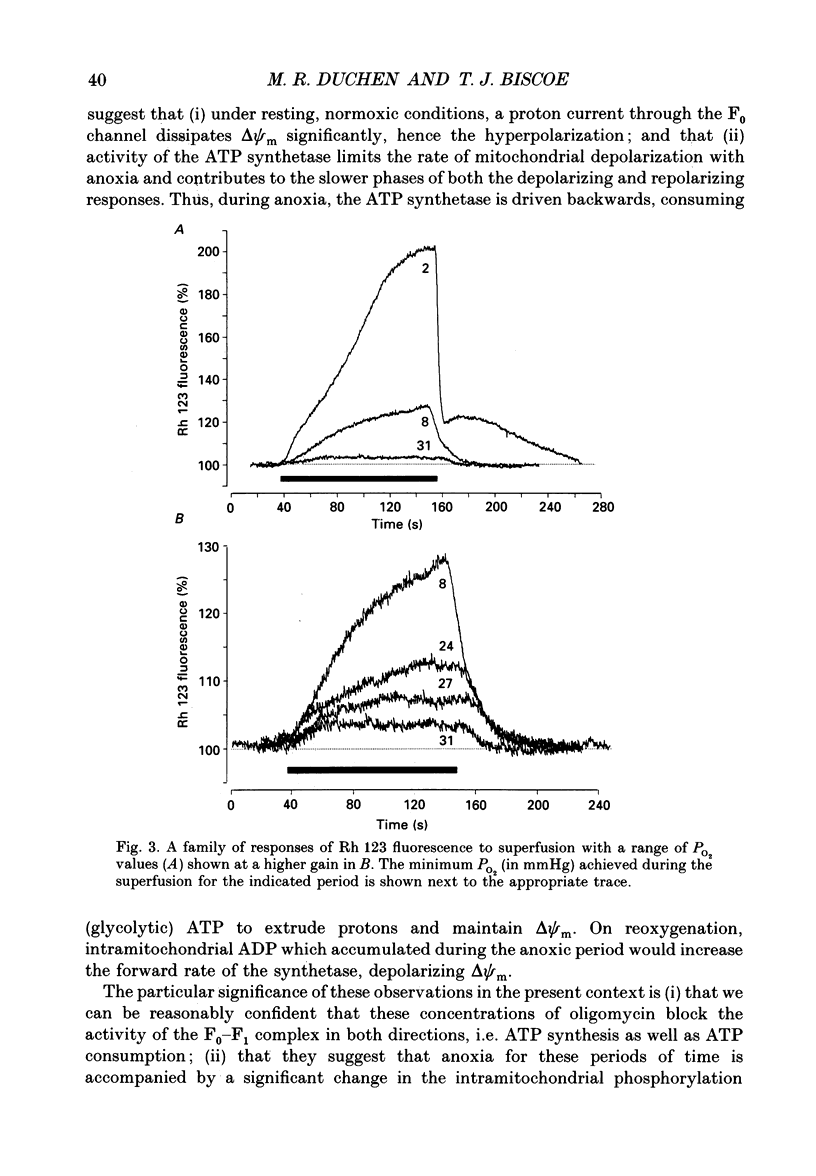
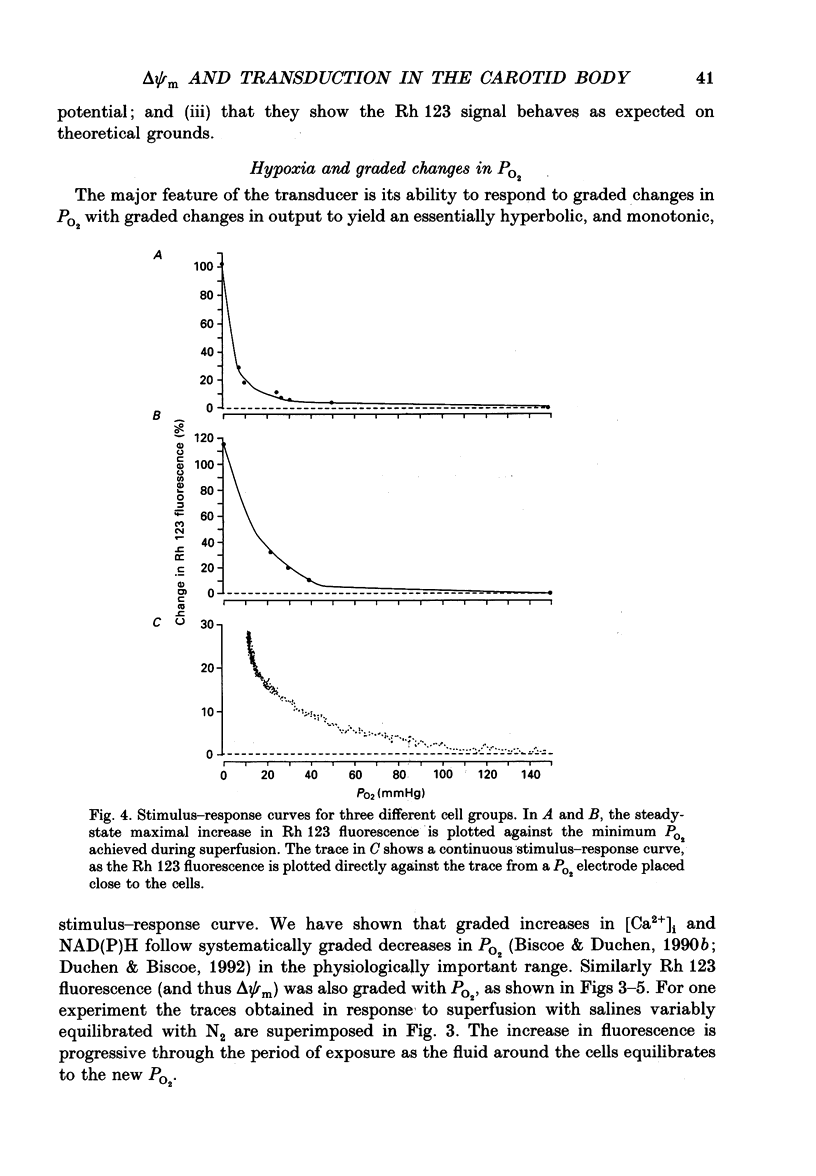
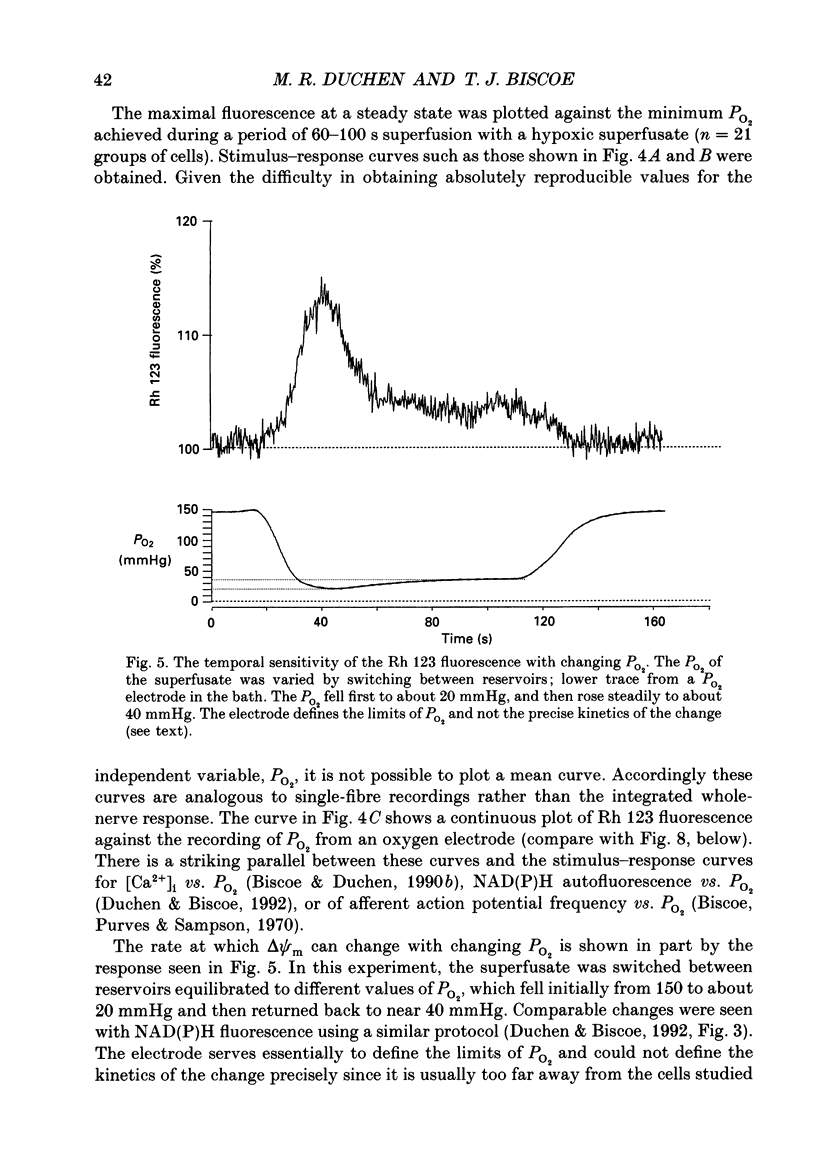
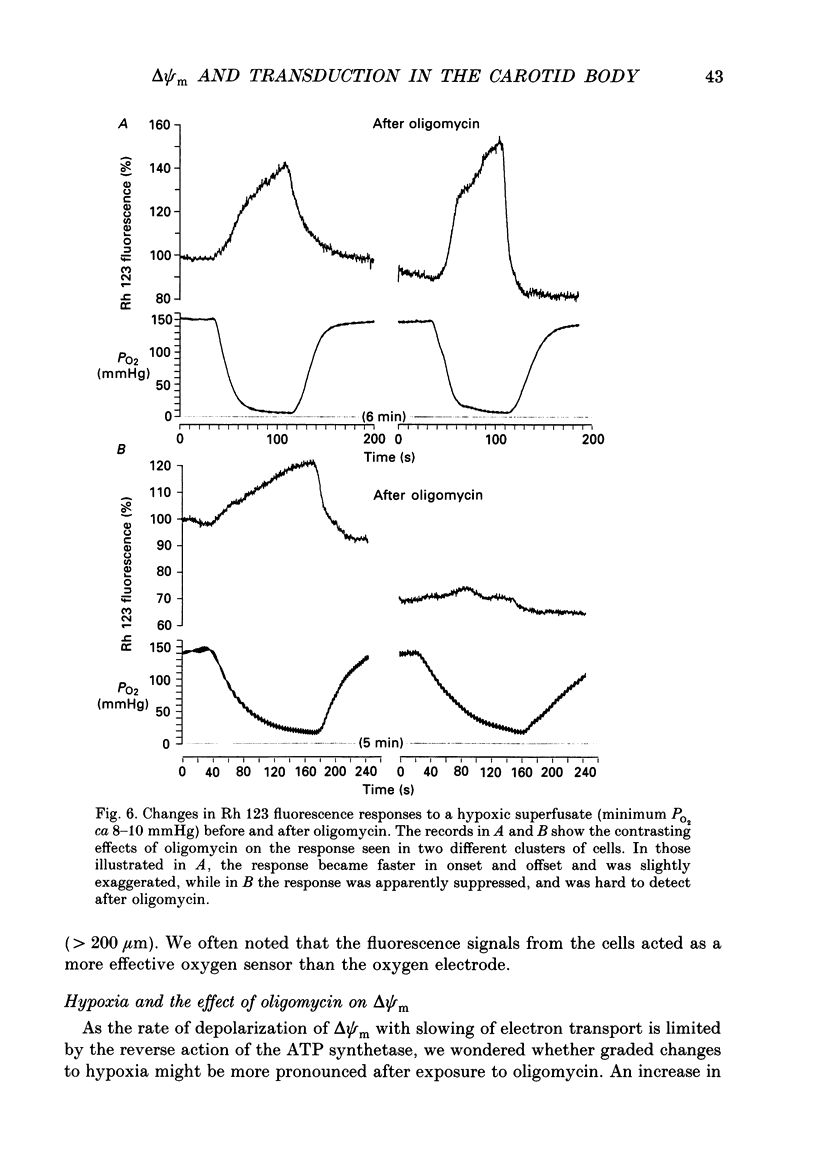
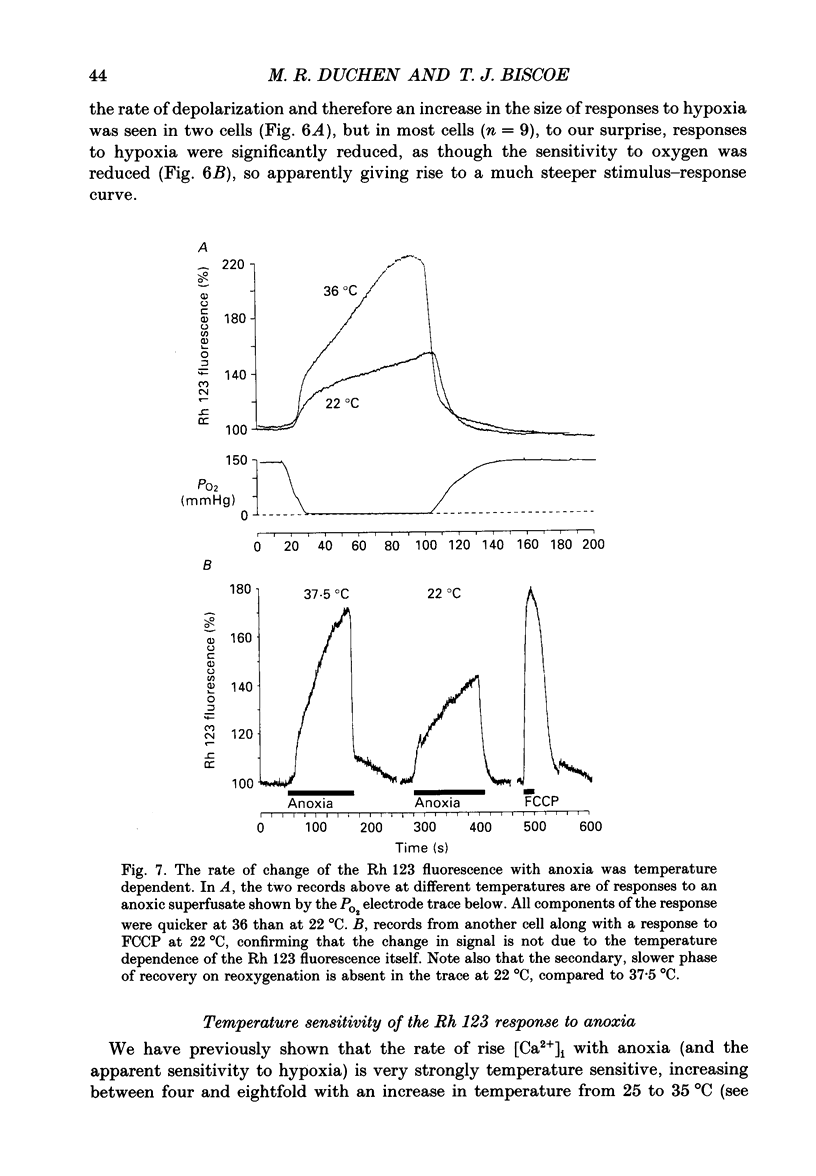
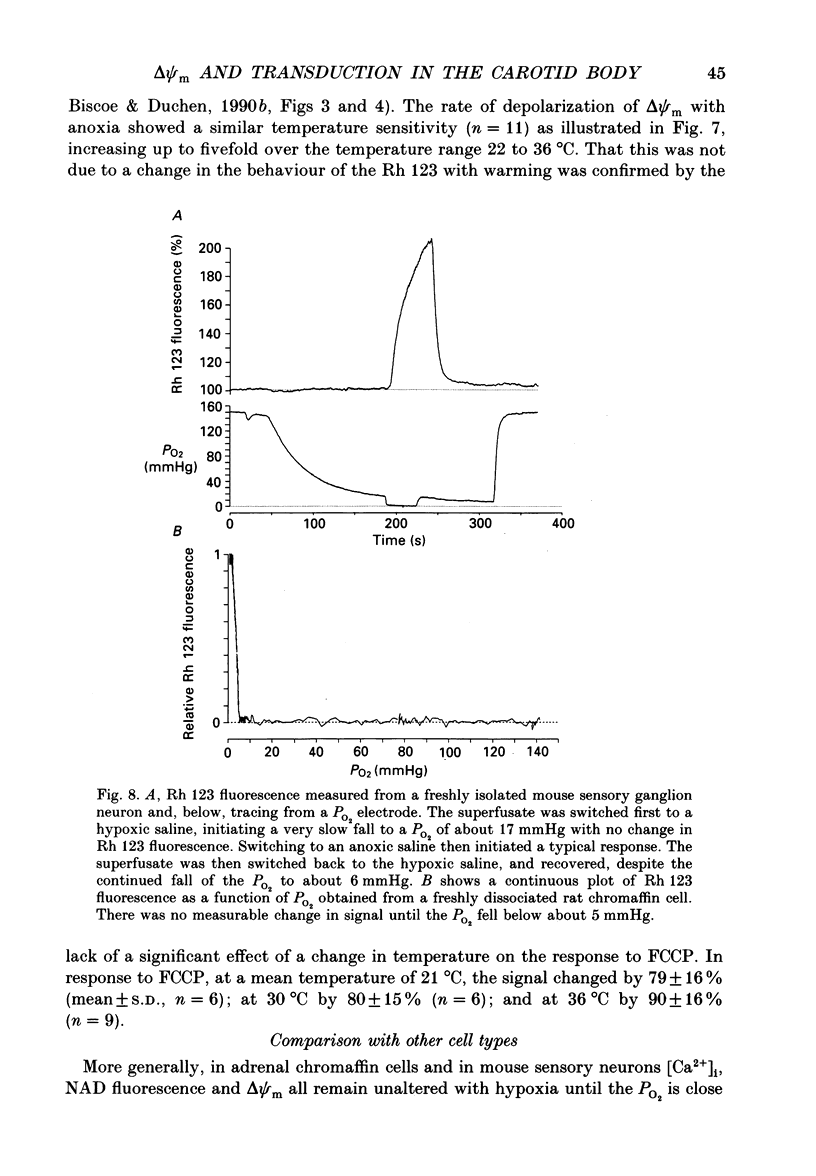
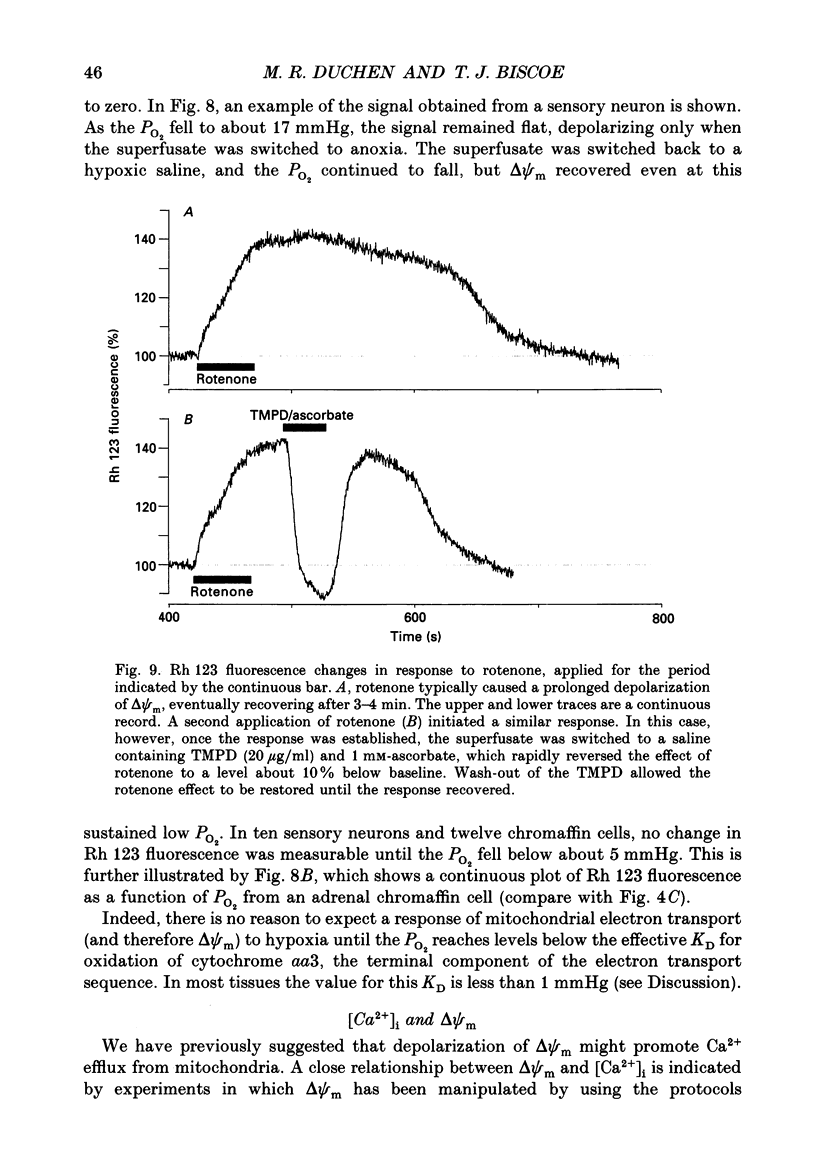
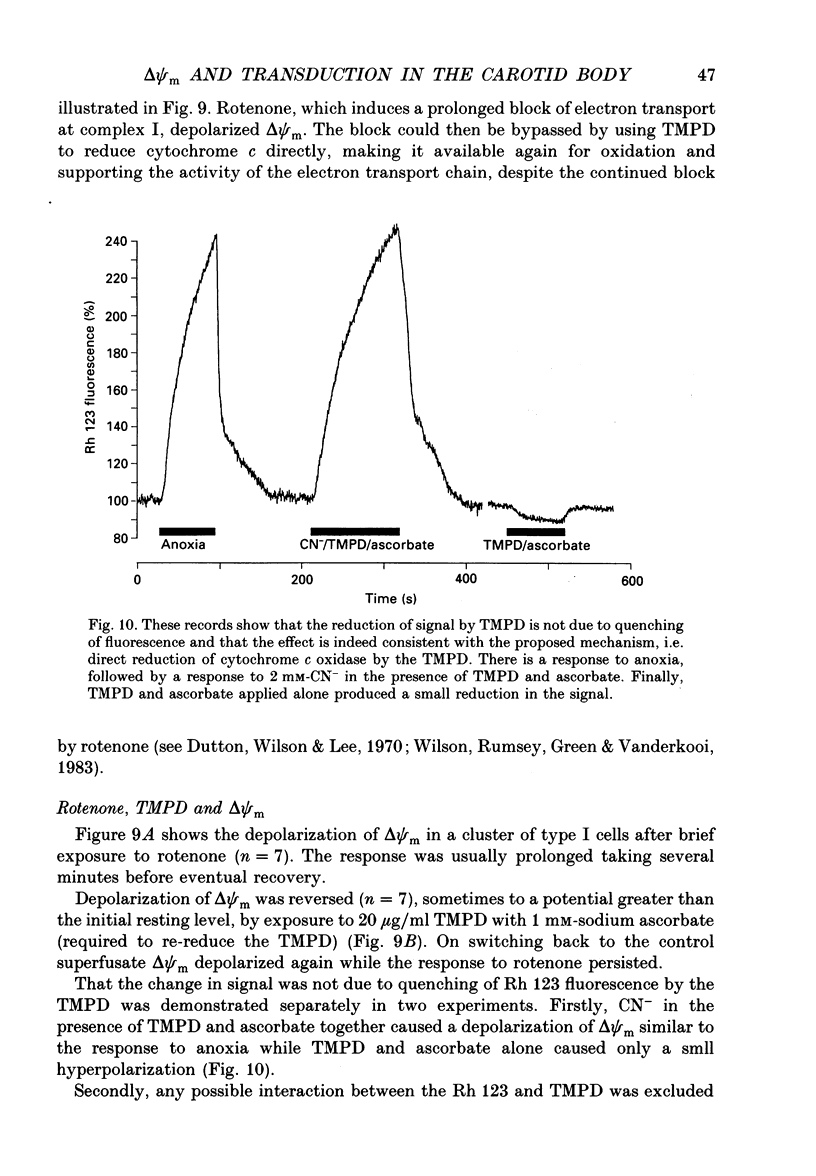
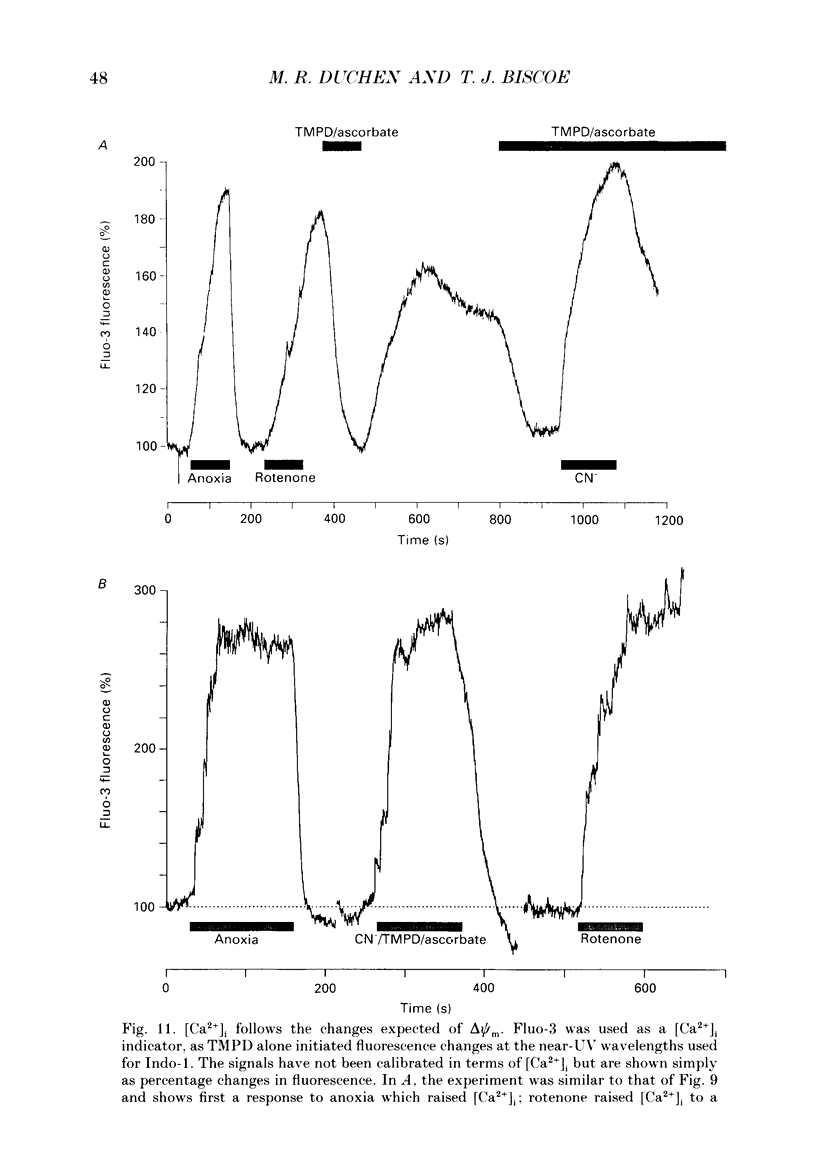
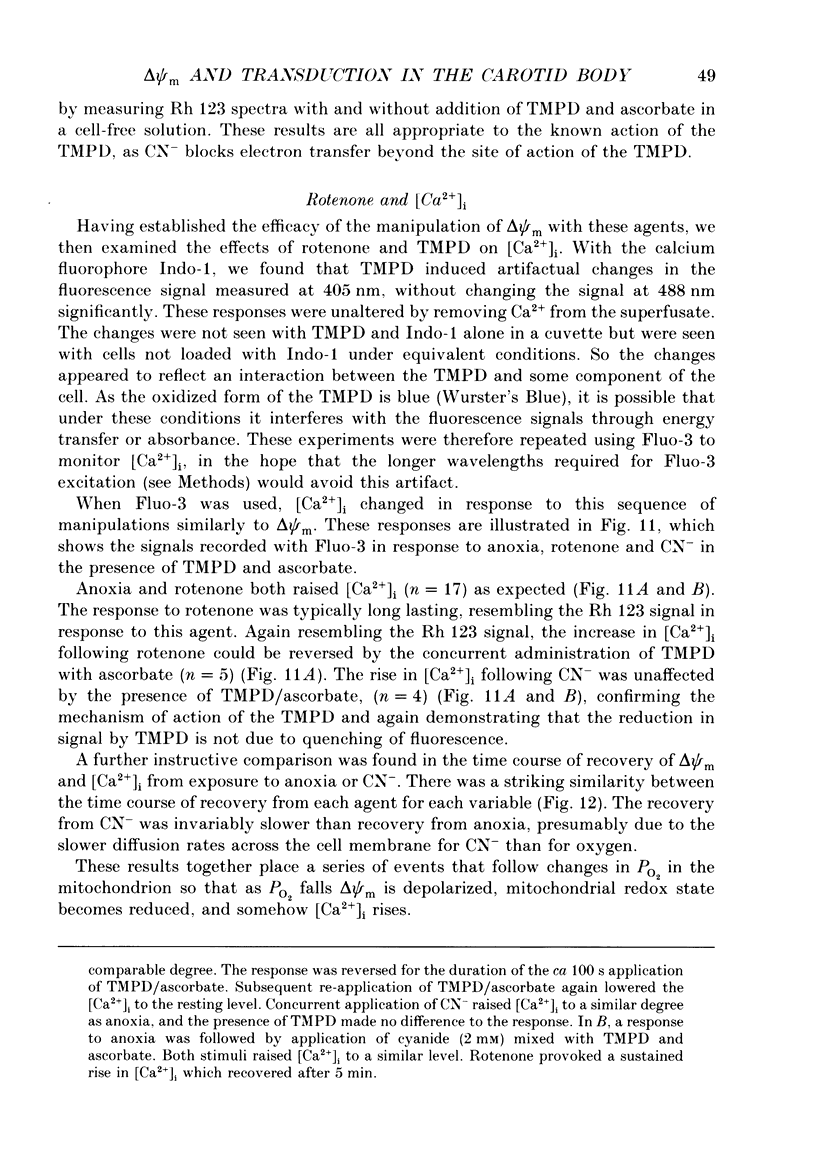
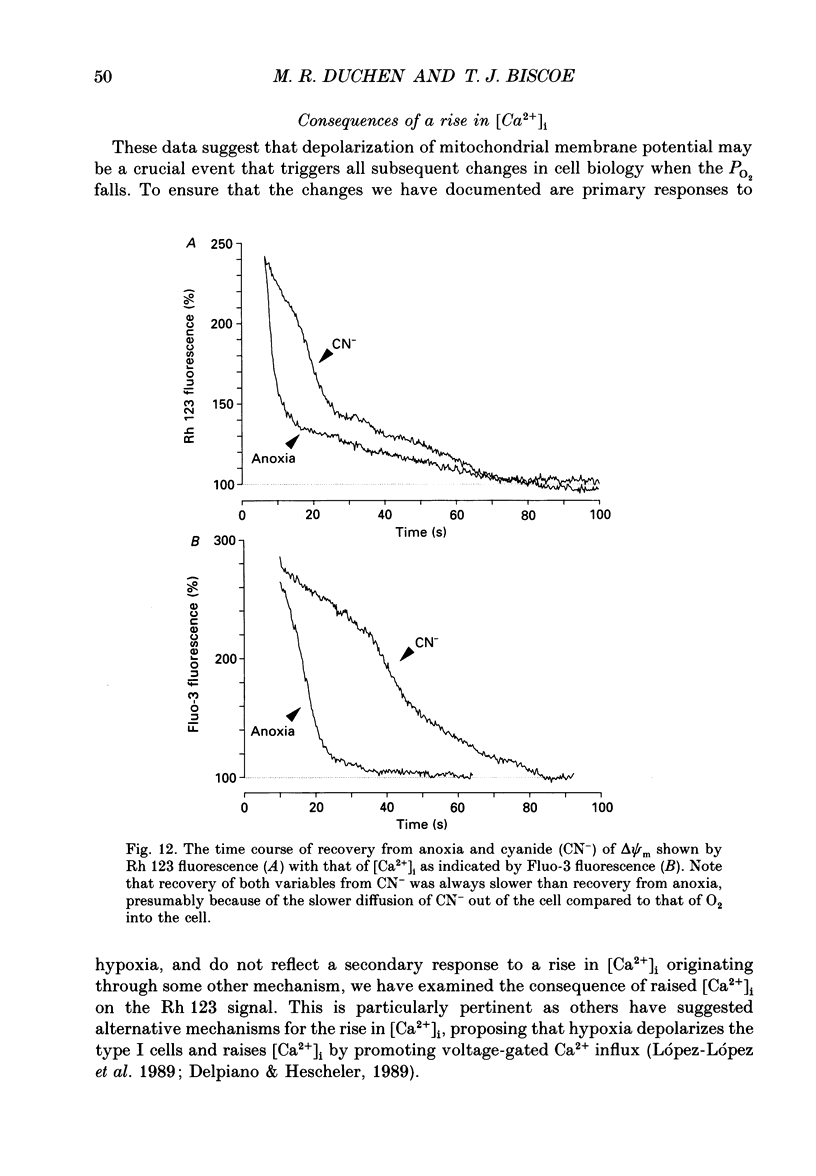
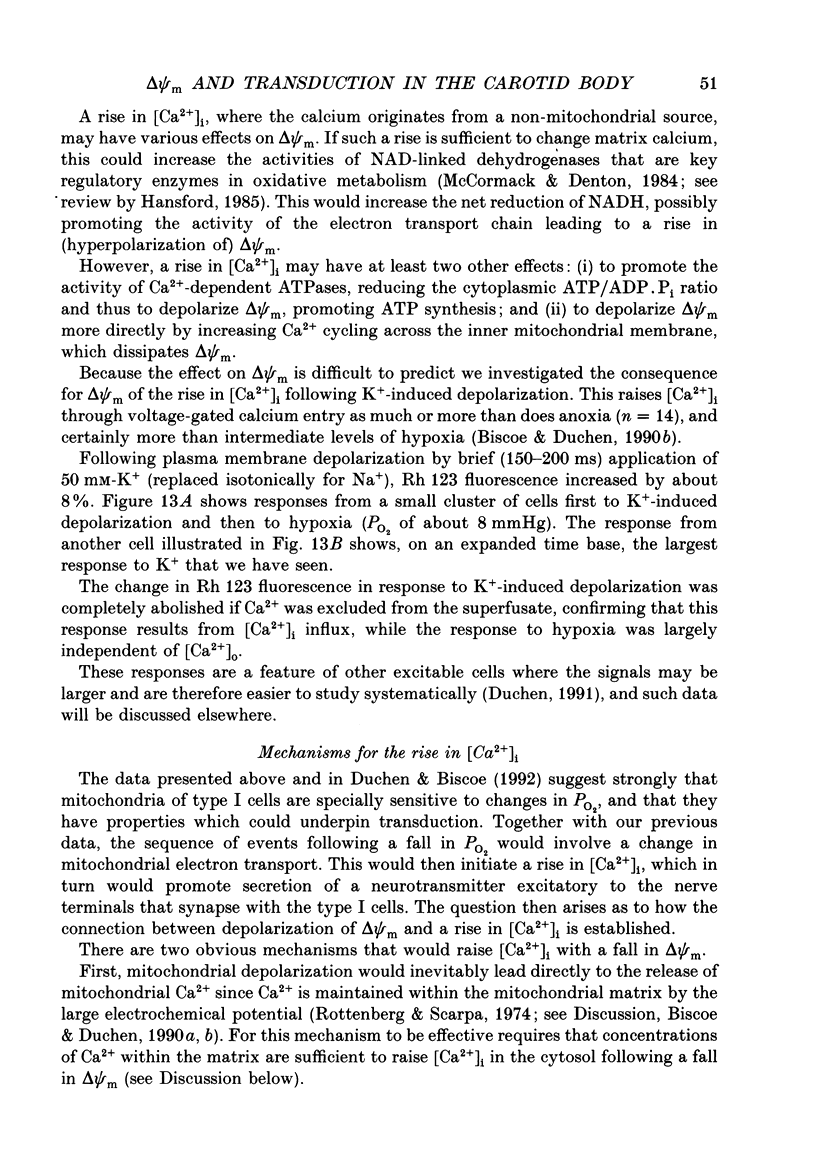
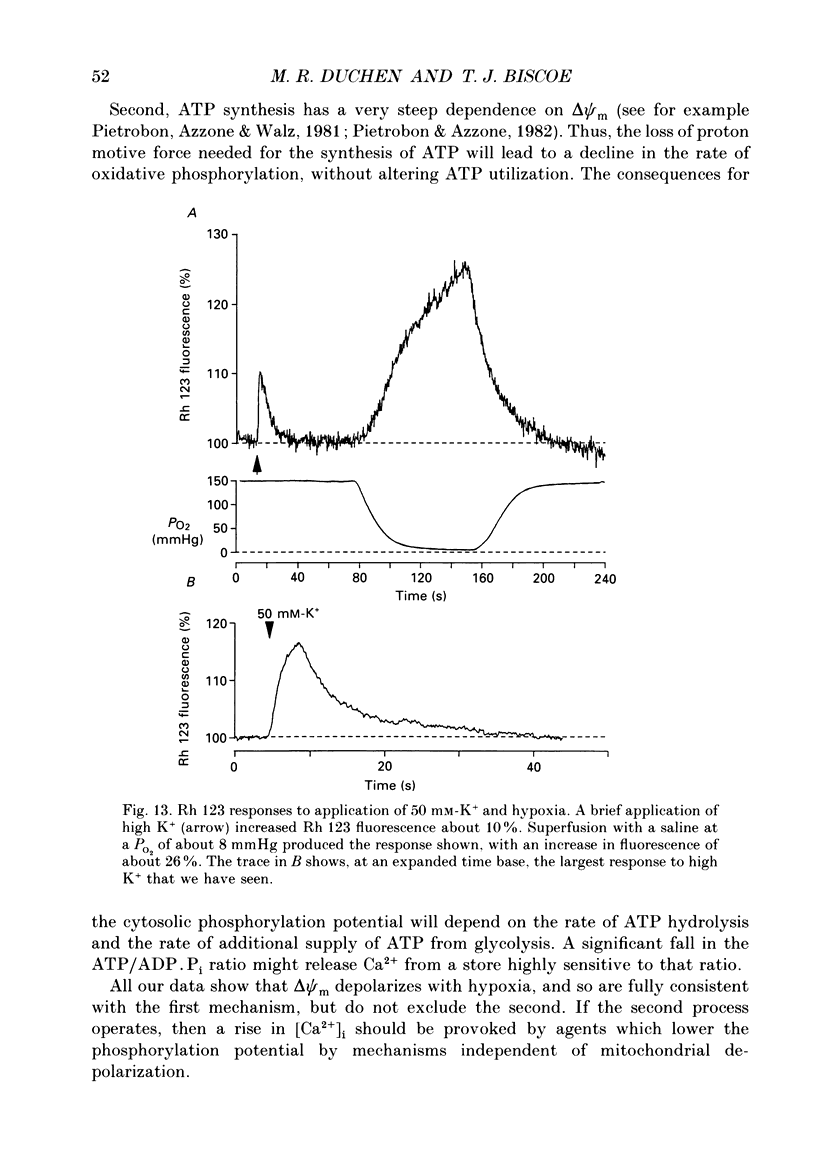
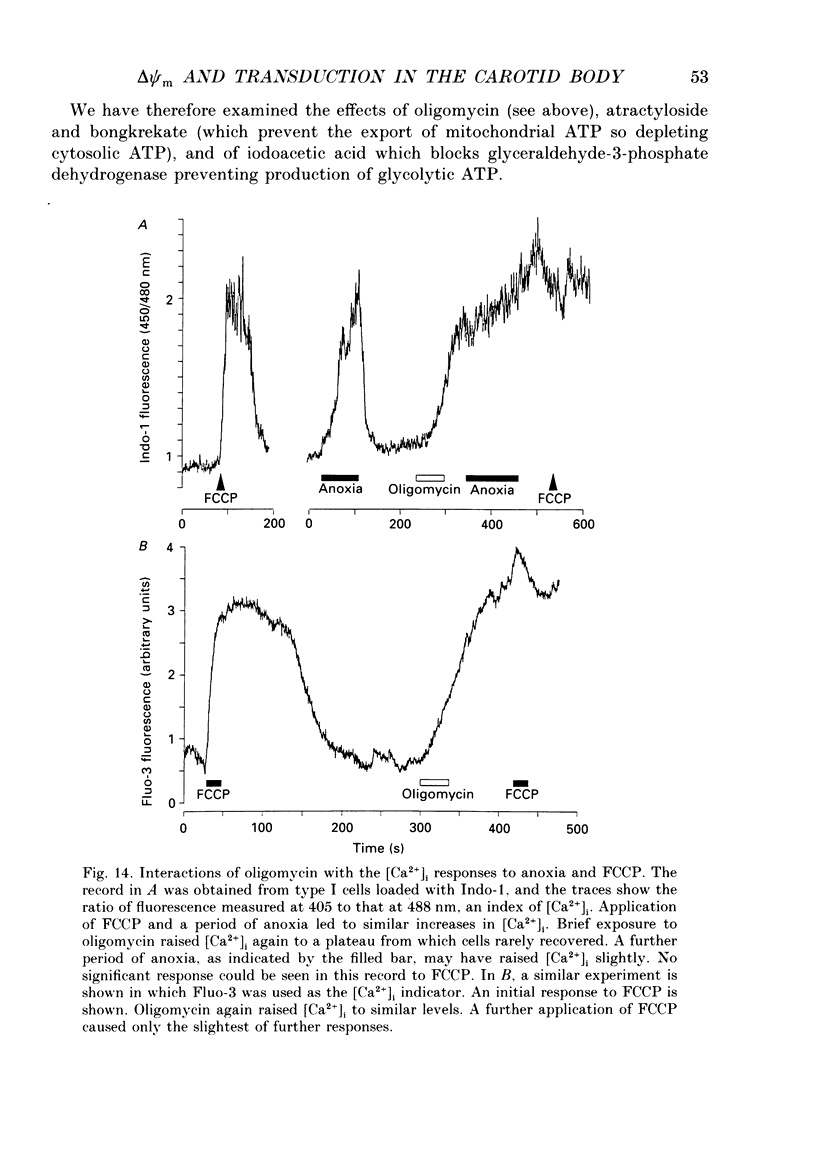
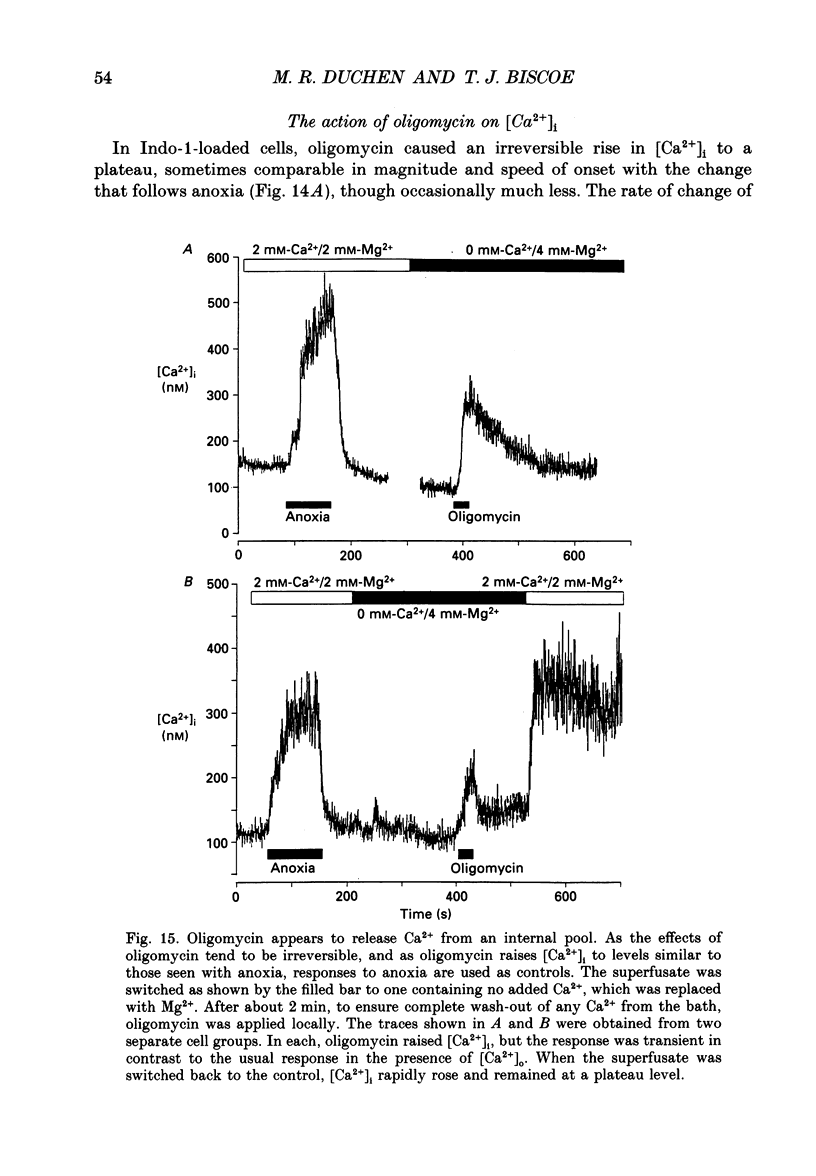
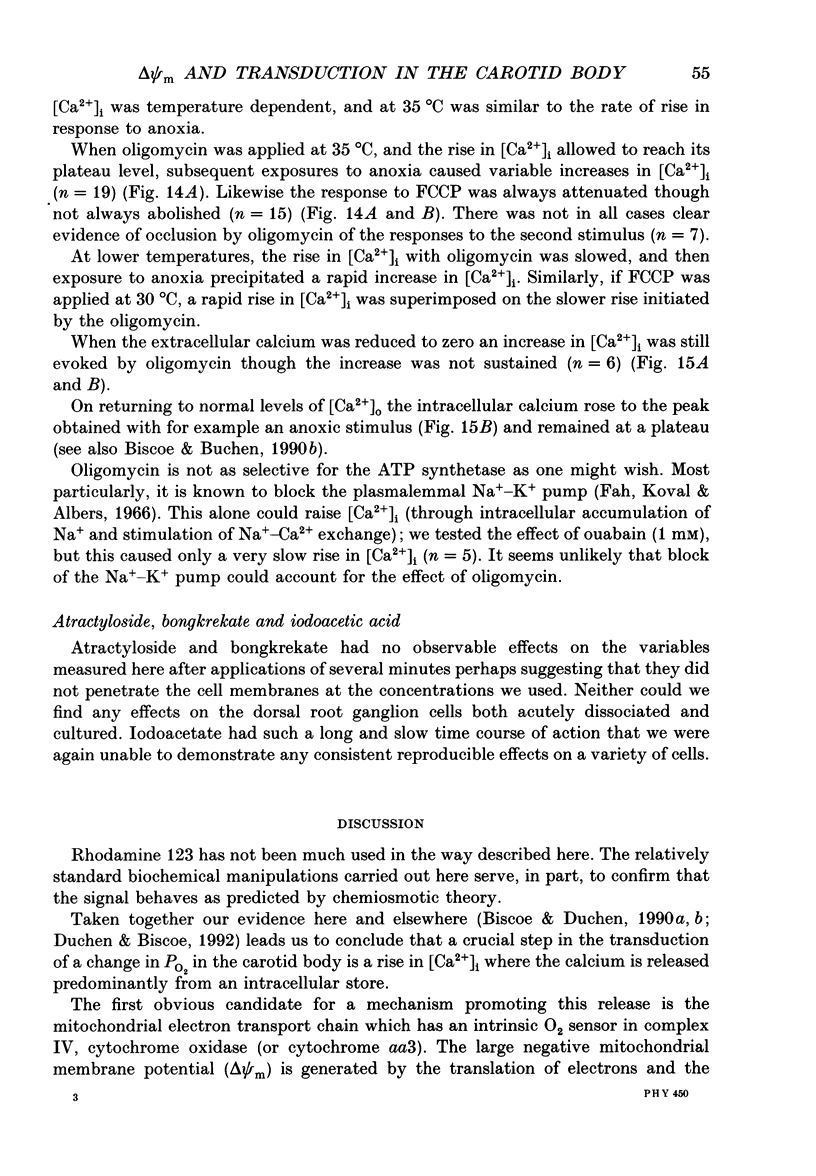
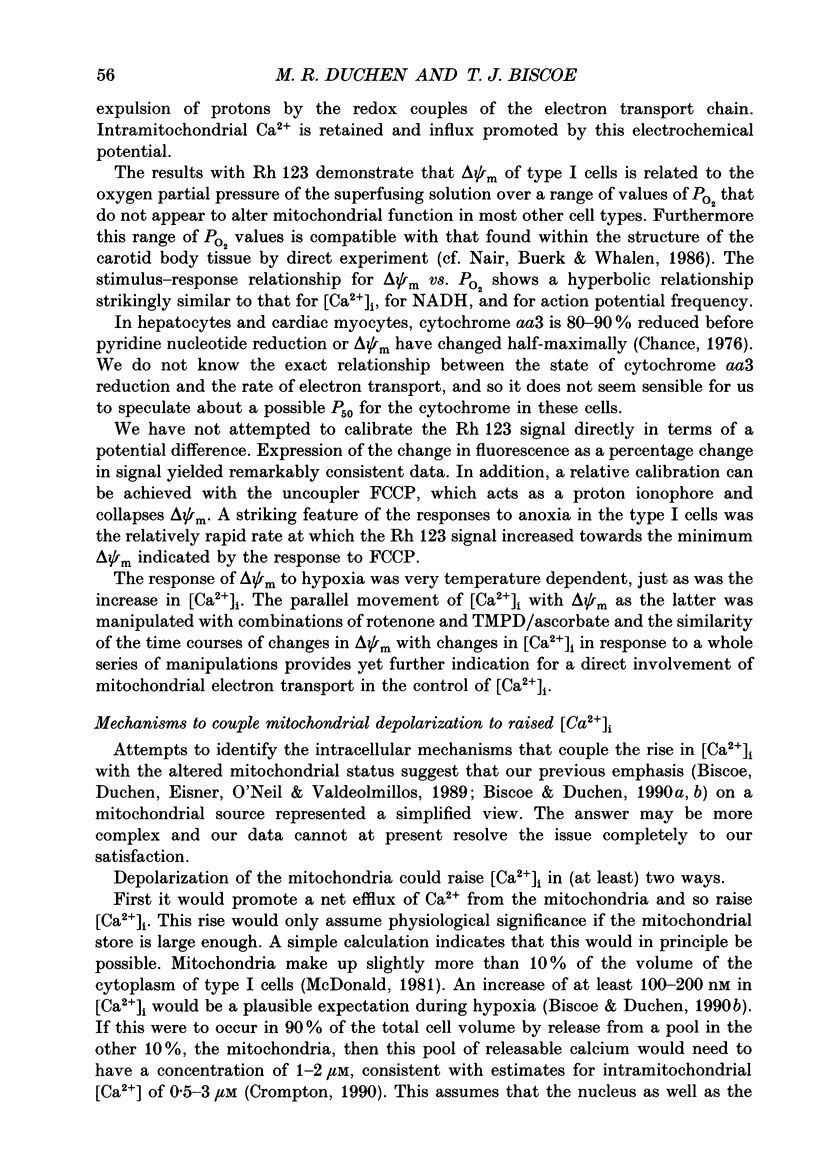
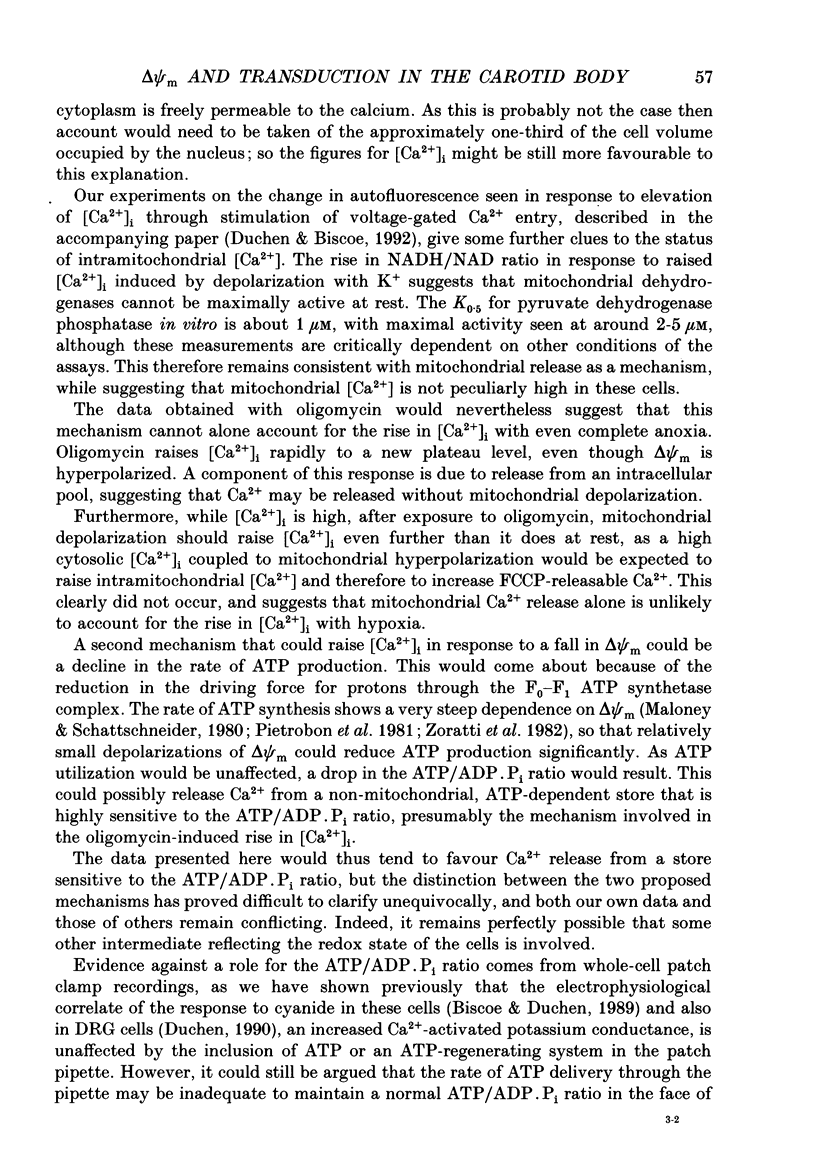
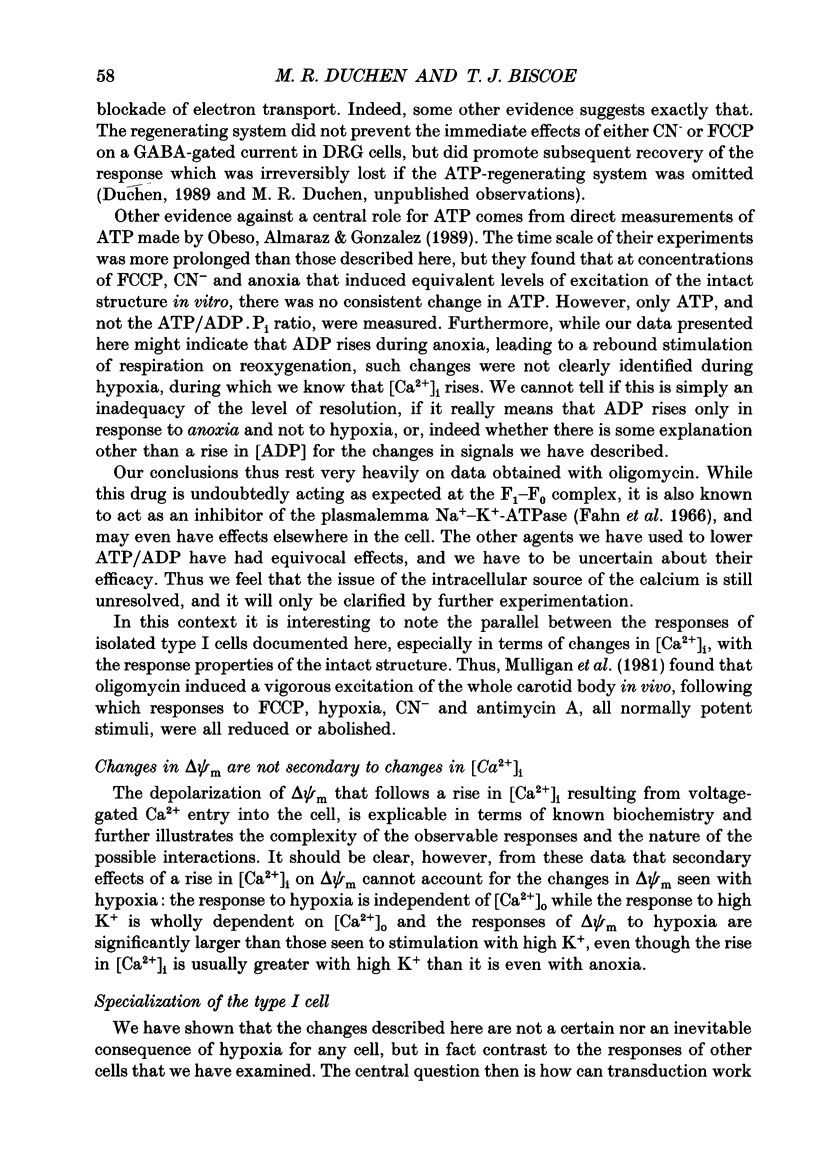
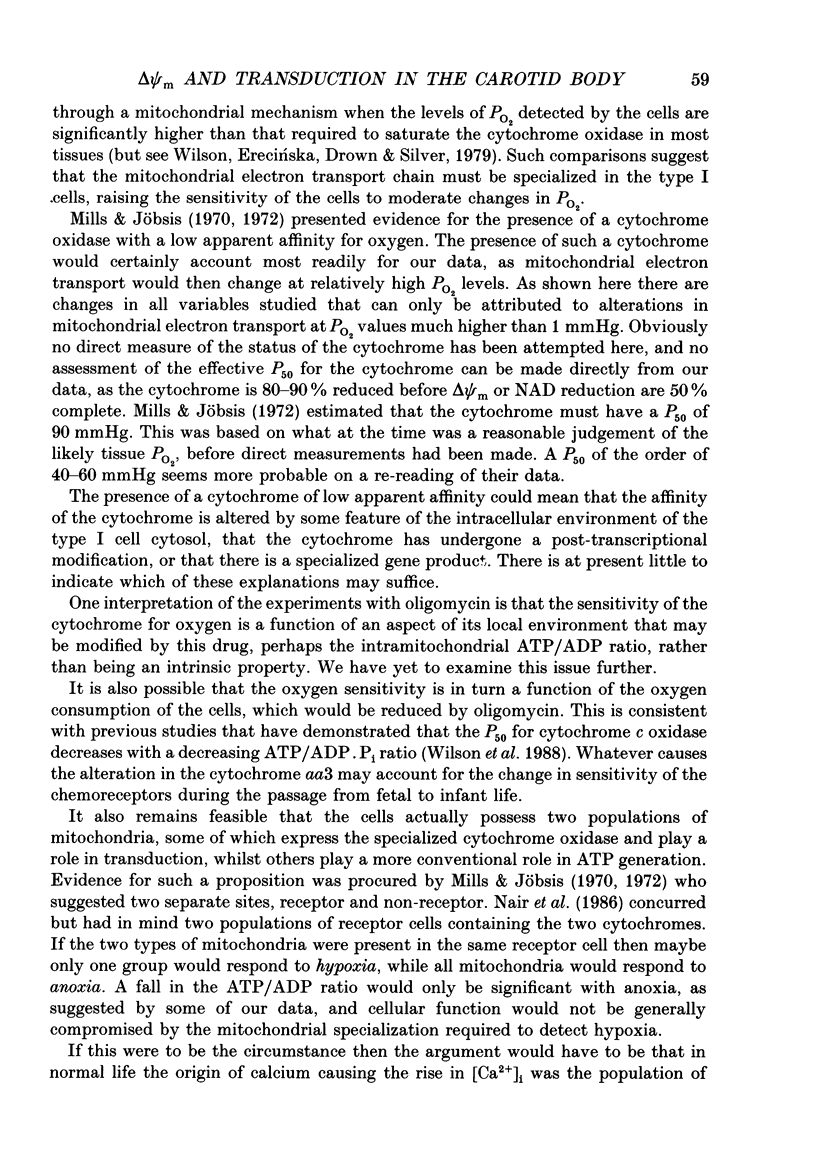
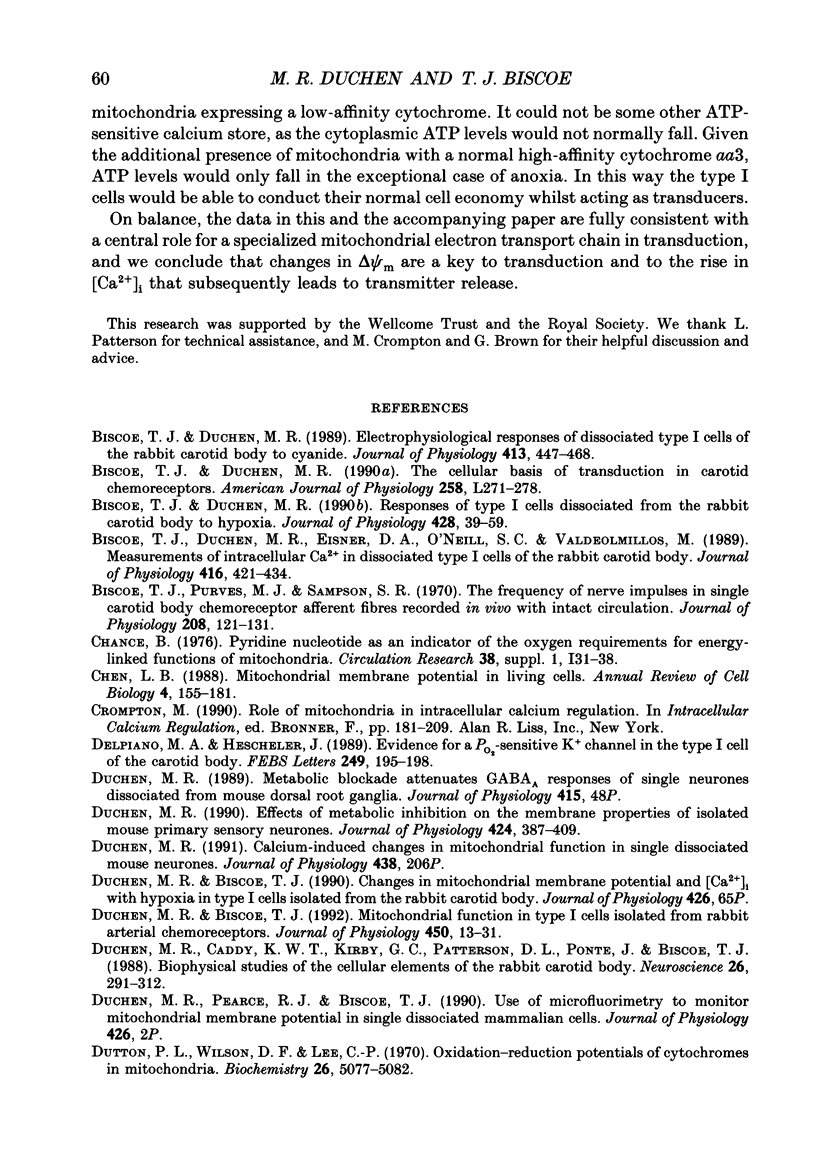
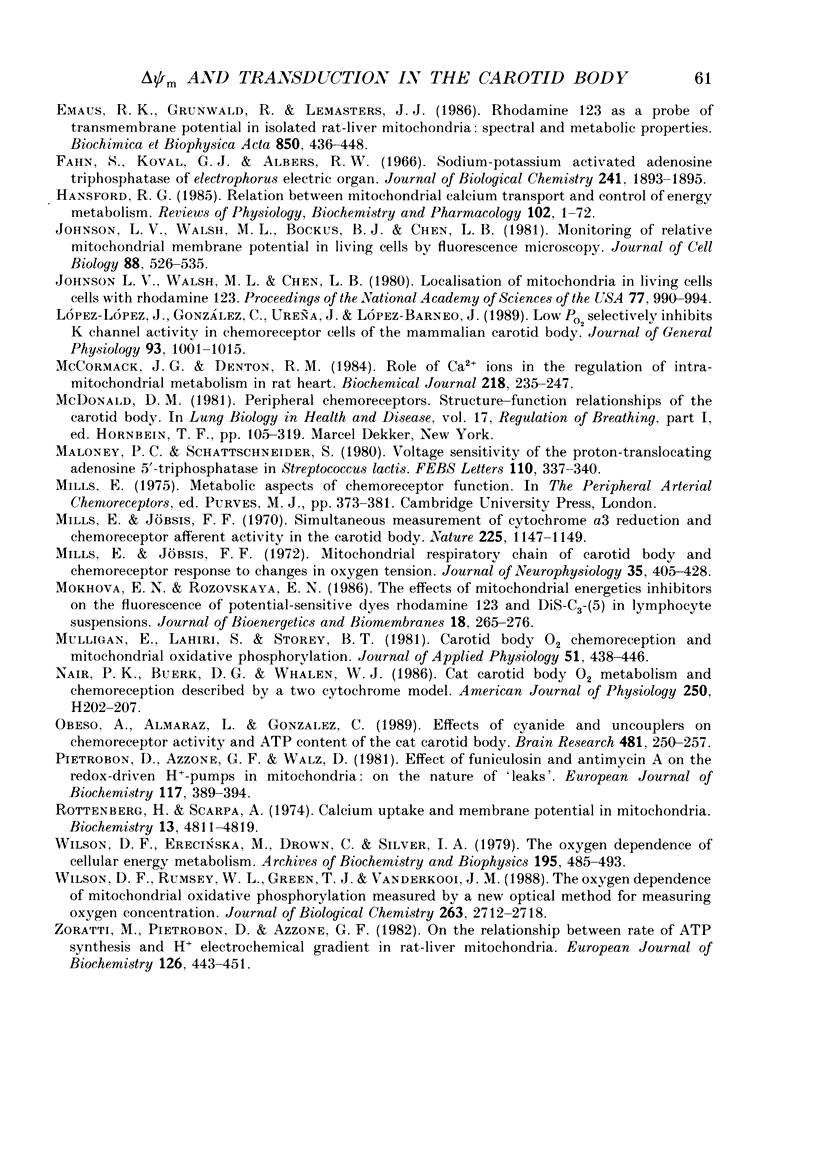
Selected References
These references are in PubMed. This may not be the complete list of references from this article.
- Biscoe T. J., Duchen M. R. Cellular basis of transduction in carotid chemoreceptors. Am J Physiol. 1990 Jun;258(6 Pt 1):L271–L278. doi: 10.1152/ajplung.1990.258.6.L271. [DOI] [PubMed] [Google Scholar]
- Biscoe T. J., Duchen M. R., Eisner D. A., O'Neill S. C., Valdeolmillos M. Measurements of intracellular Ca2+ in dissociated type I cells of the rabbit carotid body. J Physiol. 1989 Sep;416:421–434. doi: 10.1113/jphysiol.1989.sp017769. [DOI] [PMC free article] [PubMed] [Google Scholar]
- Biscoe T. J., Duchen M. R. Electrophysiological responses of dissociated type I cells of the rabbit carotid body to cyanide. J Physiol. 1989 Jun;413:447–468. doi: 10.1113/jphysiol.1989.sp017663. [DOI] [PMC free article] [PubMed] [Google Scholar]
- Biscoe T. J., Duchen M. R. Responses of type I cells dissociated from the rabbit carotid body to hypoxia. J Physiol. 1990 Sep;428:39–59. doi: 10.1113/jphysiol.1990.sp018199. [DOI] [PMC free article] [PubMed] [Google Scholar]
- Biscoe T. J., Purves M. J., Sampson S. R. The frequency of nerve impulses in single carotid body chemoreceptor afferent fibres recorded in vivo with intact circulation. J Physiol. 1970 May;208(1):121–131. doi: 10.1113/jphysiol.1970.sp009109. [DOI] [PMC free article] [PubMed] [Google Scholar]
- Delpiano M. A., Hescheler J. Evidence for a PO2-sensitive K+ channel in the type-I cell of the rabbit carotid body. FEBS Lett. 1989 Jun 5;249(2):195–198. doi: 10.1016/0014-5793(89)80623-4. [DOI] [PubMed] [Google Scholar]
- Duchen M. R., Biscoe T. J. Mitochondrial function in type I cells isolated from rabbit arterial chemoreceptors. J Physiol. 1992 May;450:13–31. doi: 10.1113/jphysiol.1992.sp019114. [DOI] [PMC free article] [PubMed] [Google Scholar]
- Duchen M. R., Caddy K. W., Kirby G. C., Patterson D. L., Ponte J., Biscoe T. J. Biophysical studies of the cellular elements of the rabbit carotid body. Neuroscience. 1988 Jul;26(1):291–311. doi: 10.1016/0306-4522(88)90146-7. [DOI] [PubMed] [Google Scholar]
- Duchen M. R. Effects of metabolic inhibition on the membrane properties of isolated mouse primary sensory neurones. J Physiol. 1990 May;424:387–409. doi: 10.1113/jphysiol.1990.sp018073. [DOI] [PMC free article] [PubMed] [Google Scholar]
- Dutton P. L., Wilson D. F., Lee C. P. Oxidation-reduction potentials of cytochromes in mitochondria. Biochemistry. 1970 Dec 22;9(26):5077–5082. doi: 10.1021/bi00828a006. [DOI] [PubMed] [Google Scholar]
- Emaus R. K., Grunwald R., Lemasters J. J. Rhodamine 123 as a probe of transmembrane potential in isolated rat-liver mitochondria: spectral and metabolic properties. Biochim Biophys Acta. 1986 Jul 23;850(3):436–448. doi: 10.1016/0005-2728(86)90112-x. [DOI] [PubMed] [Google Scholar]
- Fahn S., Hurley M. R., Koval G. J., Albers R. W. Sodium-potassium-activated adenosine triphosphatase of Electrophorus electric organ. II. Effects of N-ethylmaleimide and other sulfhydryl reagents. J Biol Chem. 1966 Apr 25;241(8):1890–1895. [PubMed] [Google Scholar]
- Hansford R. G. Relation between mitochondrial calcium transport and control of energy metabolism. Rev Physiol Biochem Pharmacol. 1985;102:1–72. doi: 10.1007/BFb0034084. [DOI] [PubMed] [Google Scholar]
- Johnson L. V., Walsh M. L., Bockus B. J., Chen L. B. Monitoring of relative mitochondrial membrane potential in living cells by fluorescence microscopy. J Cell Biol. 1981 Mar;88(3):526–535. doi: 10.1083/jcb.88.3.526. [DOI] [PMC free article] [PubMed] [Google Scholar]
- Johnson L. V., Walsh M. L., Chen L. B. Localization of mitochondria in living cells with rhodamine 123. Proc Natl Acad Sci U S A. 1980 Feb;77(2):990–994. doi: 10.1073/pnas.77.2.990. [DOI] [PMC free article] [PubMed] [Google Scholar]
- López-López J., González C., Ureña J., López-Barneo J. Low pO2 selectively inhibits K channel activity in chemoreceptor cells of the mammalian carotid body. J Gen Physiol. 1989 May;93(5):1001–1015. doi: 10.1085/jgp.93.5.1001. [DOI] [PMC free article] [PubMed] [Google Scholar]
- Maloney P. C., Schattschneider S. Voltage sensitivity of the proton-translocating adenosine 5'-triphosphatase in Streptococcus lactis. FEBS Lett. 1980 Feb 11;110(2):337–340. doi: 10.1016/0014-5793(80)80106-2. [DOI] [PubMed] [Google Scholar]
- McCormack J. G., Denton R. M. Role of Ca2+ ions in the regulation of intramitochondrial metabolism in rat heart. Evidence from studies with isolated mitochondria that adrenaline activates the pyruvate dehydrogenase and 2-oxoglutarate dehydrogenase complexes by increasing the intramitochondrial concentration of Ca2+. Biochem J. 1984 Feb 15;218(1):235–247. doi: 10.1042/bj2180235. [DOI] [PMC free article] [PubMed] [Google Scholar]
- Mills E., Jöbsis F. F. Mitochondrial respiratory chain of carotid body and chemoreceptor response to changes in oxygen tension. J Neurophysiol. 1972 Jul;35(4):405–428. doi: 10.1152/jn.1972.35.4.405. [DOI] [PubMed] [Google Scholar]
- Mills E., Jöbsis F. F. Simultaneous measurement of cytochrome a3 reduction and chemoreceptor afferent activity in the carotid body. Nature. 1970 Mar 21;225(5238):1147–1149. doi: 10.1038/2251147a0. [DOI] [PubMed] [Google Scholar]
- Mokhova E. N., Rozovskaya I. A. The effects of mitochondrial energetics inhibitors on the fluorescence of potential-sensitive dyes rhodamine 123 and diS-C3-(5) in lymphocyte suspensions. J Bioenerg Biomembr. 1986 Aug;18(4):265–276. doi: 10.1007/BF00743047. [DOI] [PubMed] [Google Scholar]
- Mulligan E., Lahiri S., Storey B. T. Carotid body O2 chemoreception and mitochondrial oxidative phosphorylation. J Appl Physiol Respir Environ Exerc Physiol. 1981 Aug;51(2):438–446. doi: 10.1152/jappl.1981.51.2.438. [DOI] [PubMed] [Google Scholar]
- Nair P. K., Buerk D. G., Whalen W. J. Cat carotid body oxygen metabolism and chemoreception described by a two-cytochrome model. Am J Physiol. 1986 Feb;250(2 Pt 2):H202–H207. doi: 10.1152/ajpheart.1986.250.2.H202. [DOI] [PubMed] [Google Scholar]
- Obeso A., Almaraz L., Gonzalez C. Effects of cyanide and uncouplers on chemoreceptor activity and ATP content of the cat carotid body. Brain Res. 1989 Mar 6;481(2):250–257. doi: 10.1016/0006-8993(89)90801-9. [DOI] [PubMed] [Google Scholar]
- Pietrobon D., Azzone G. F., Walz D. Effect of funiculosin and antimycin A on the redox-driven H+-pumps in mitochondria: on the nature of "leaks'. Eur J Biochem. 1981 Jul;117(2):389–394. doi: 10.1111/j.1432-1033.1981.tb06350.x. [DOI] [PubMed] [Google Scholar]
- Rottenberg H., Scarpa A. Calcium uptake and membrane potential in mitochondria. Biochemistry. 1974 Nov 5;13(23):4811–4817. doi: 10.1021/bi00720a020. [DOI] [PubMed] [Google Scholar]
- Wilson D. F., Erecińska M., Drown C., Silver I. A. The oxygen dependence of cellular energy metabolism. Arch Biochem Biophys. 1979 Jul;195(2):485–493. doi: 10.1016/0003-9861(79)90375-8. [DOI] [PubMed] [Google Scholar]
- Wilson D. F., Rumsey W. L., Green T. J., Vanderkooi J. M. The oxygen dependence of mitochondrial oxidative phosphorylation measured by a new optical method for measuring oxygen concentration. J Biol Chem. 1988 Feb 25;263(6):2712–2718. [PubMed] [Google Scholar]
- Zoratti M., Pietrobon D., Azzone G. F. On the relationship between rate of ATP synthesis and H+ electrochemical gradient in rat-liver mitochondria. Eur J Biochem. 1982 Sep 1;126(3):443–451. doi: 10.1111/j.1432-1033.1982.tb06800.x. [DOI] [PubMed] [Google Scholar]