Abstract
1. Tight-seal whole-cell recordings were made from lumbar motoneurones visually identified in thin slices of neonatal rat spinal cord. The inhibitory postsynaptic currents (IPSCs) were evoked by extracellular stimulation of a neighbouring internuncial neurone in the presence of glutamate receptor antagonists. 2. Glycinergic IPSCs were recorded in the presence of bicuculline. The IPSCs appeared in an all-or-none manner as the graded stimulus intensity exceeded a certain threshold. Their latencies showed a unimodal distribution with a mean of 0.81 ms at 37 degrees C. Thus, the observed IPSCs are suggested to be monosynaptically evoked unitary IPSCs. The mean conductance of unitary IPSCs was 2.9 +/- 1.2 nS (+/- S.D.). 3. When the external Ca2+ concentration ([Ca2+]o) was reduced, the number of failures in response to stimulation increased, thereby reducing the mean amplitude of IPSCs. The mean amplitude of IPSCs was linearly related to the [Ca2+]o (0.35-1.4 mM) with a mean slope of 3.1 +/- 0.67 on double logarithmic co-ordinates. 4. The amplitude of individual IPSCs decreased with decrease in [Ca2+]o. However, below 0.7 mM [Ca2+]o, the mean amplitude of IPSCs (excluding failures) reached a steady minimum level. The mean conductance of these IPSCs measured in 0.5 mM [Ca2+]o was 657 +/- 281 pS. 5. The minimal IPSCs had a coefficient of variation of 0.50 +/- 0.13. No clear correlation was observed between the rise time and the amplitude of minimal IPSCs evoked in individual motoneurones, indicating that the amplitude variability is not due to the different synaptic locations. 6. Spontaneous miniature IPSCs were recorded from motoneurones in the presence of tetrodotoxin. The miniature IPSCs had a mean conductance of 739 +/- 278 pS, being comparable to the minimal evoked IPSCs. 7. Under various internal and external Cl- concentration, the reversal potential of the IPSCs (EIPSC) approximately coincided with the Cl- equilibrium potential. A 730-fold change in the potassium concentration gradient across the membrane did not affect the EIPSC. The permeability ratio of K+ to Cl- (Pk/PCl) was less than 0.05. 8. It is concluded that the IPSCs are carried almost exclusively by Cl- and that the minimal evoked IPSCs represent the quantal response of the transmitter.
Full text
PDF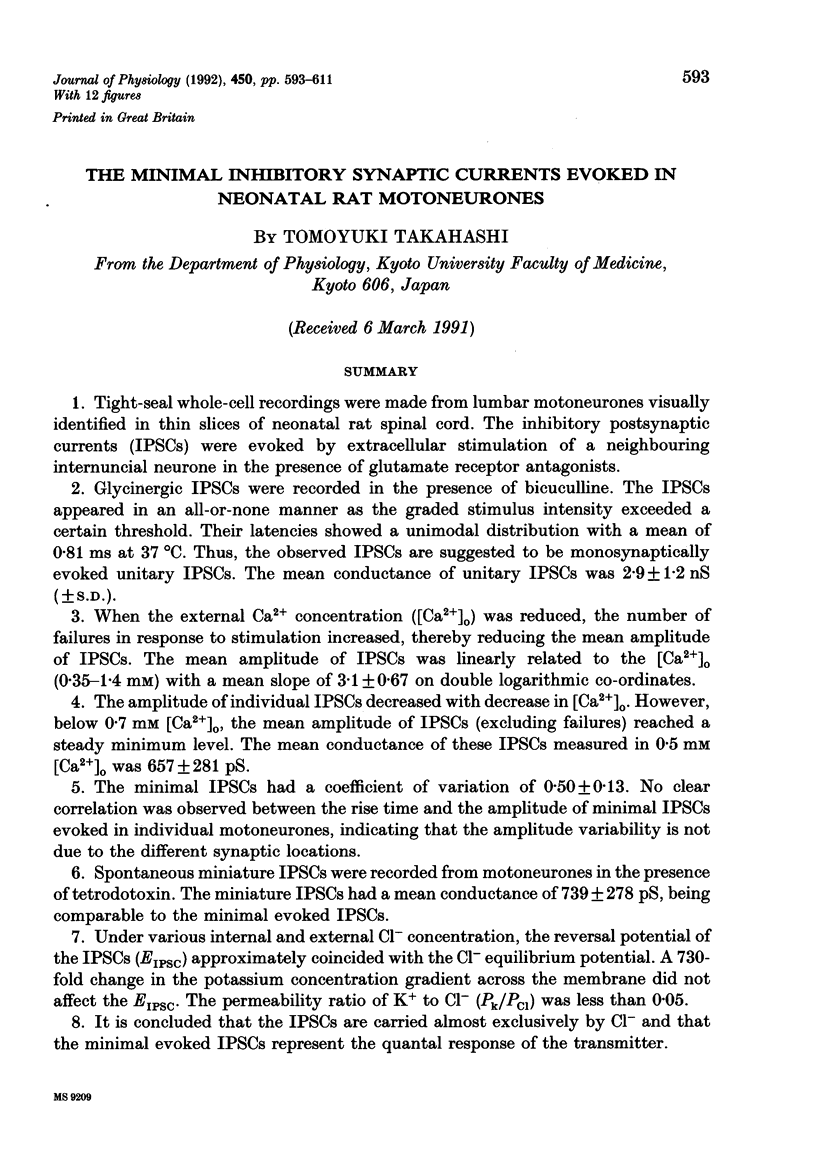
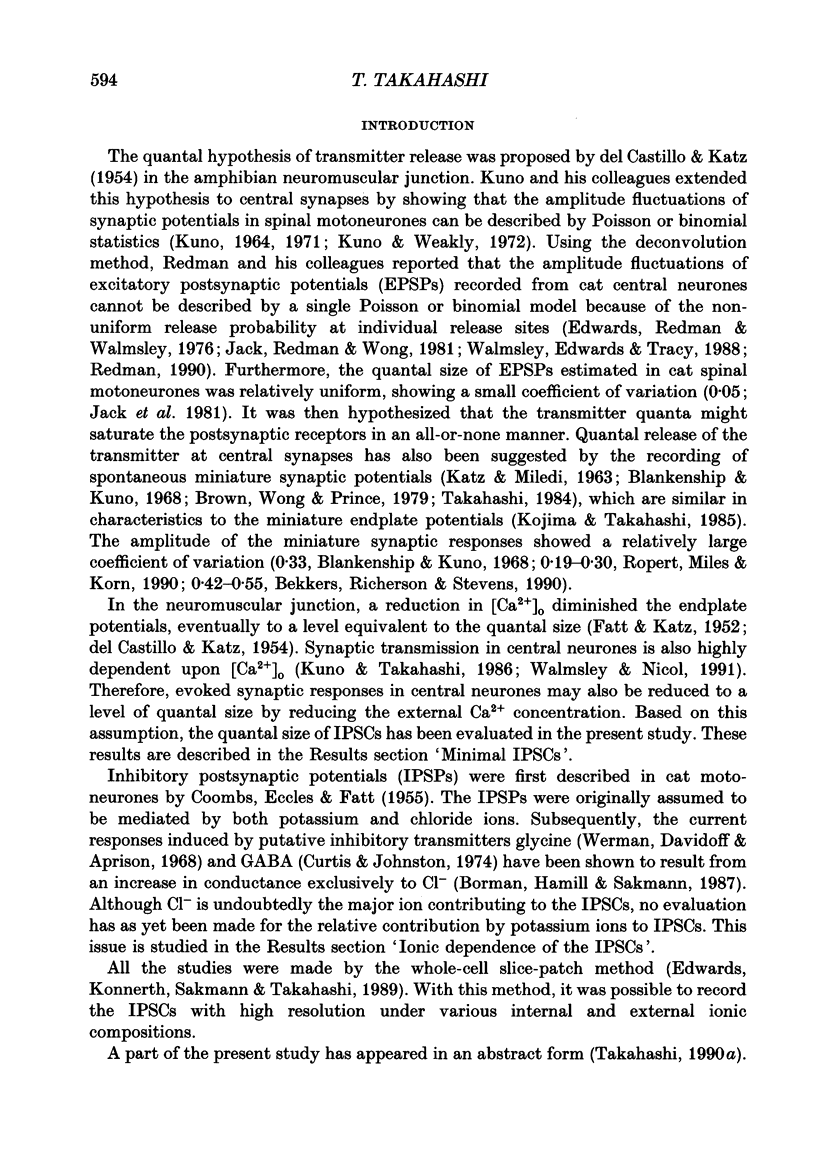
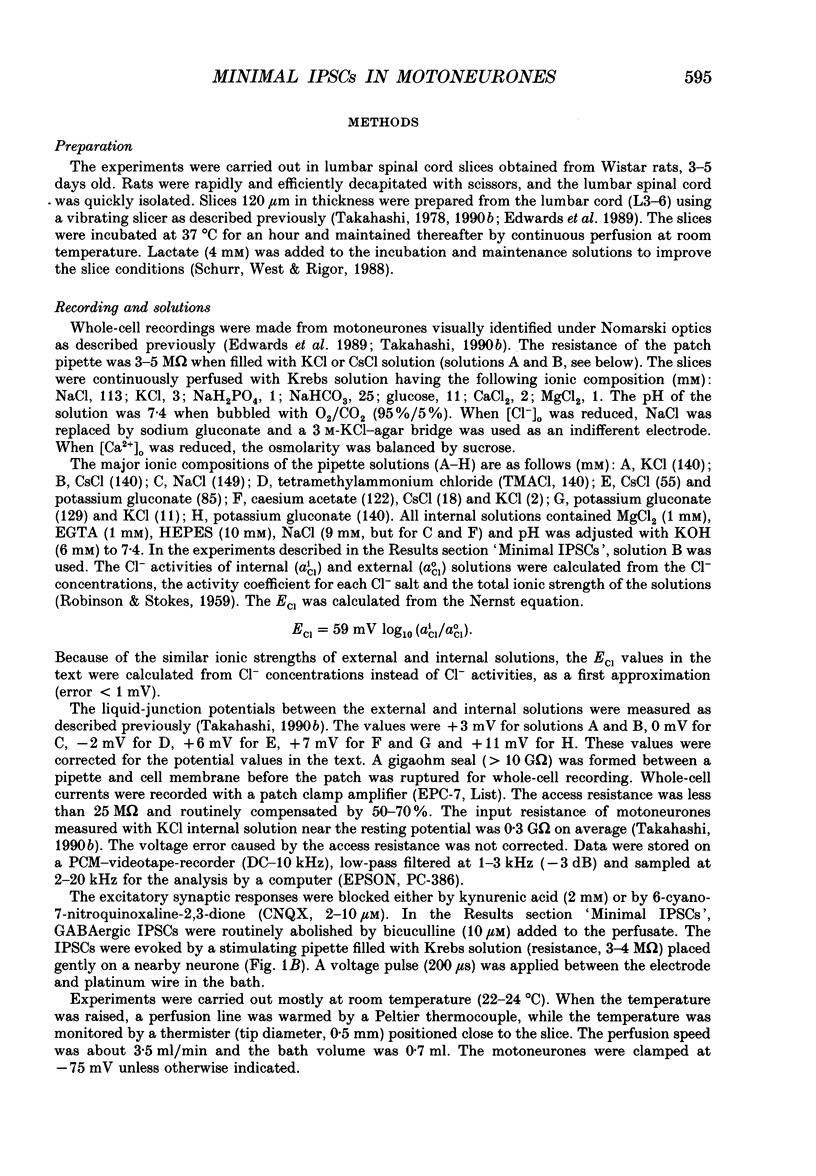
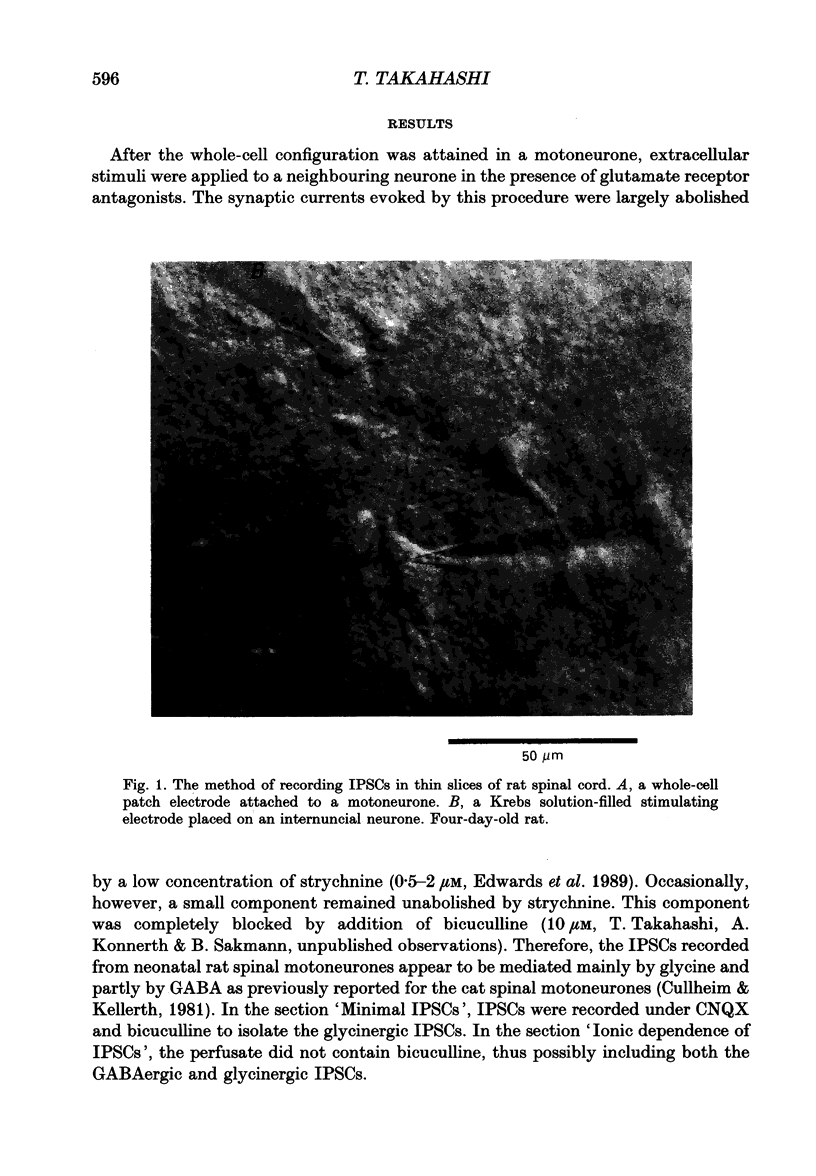
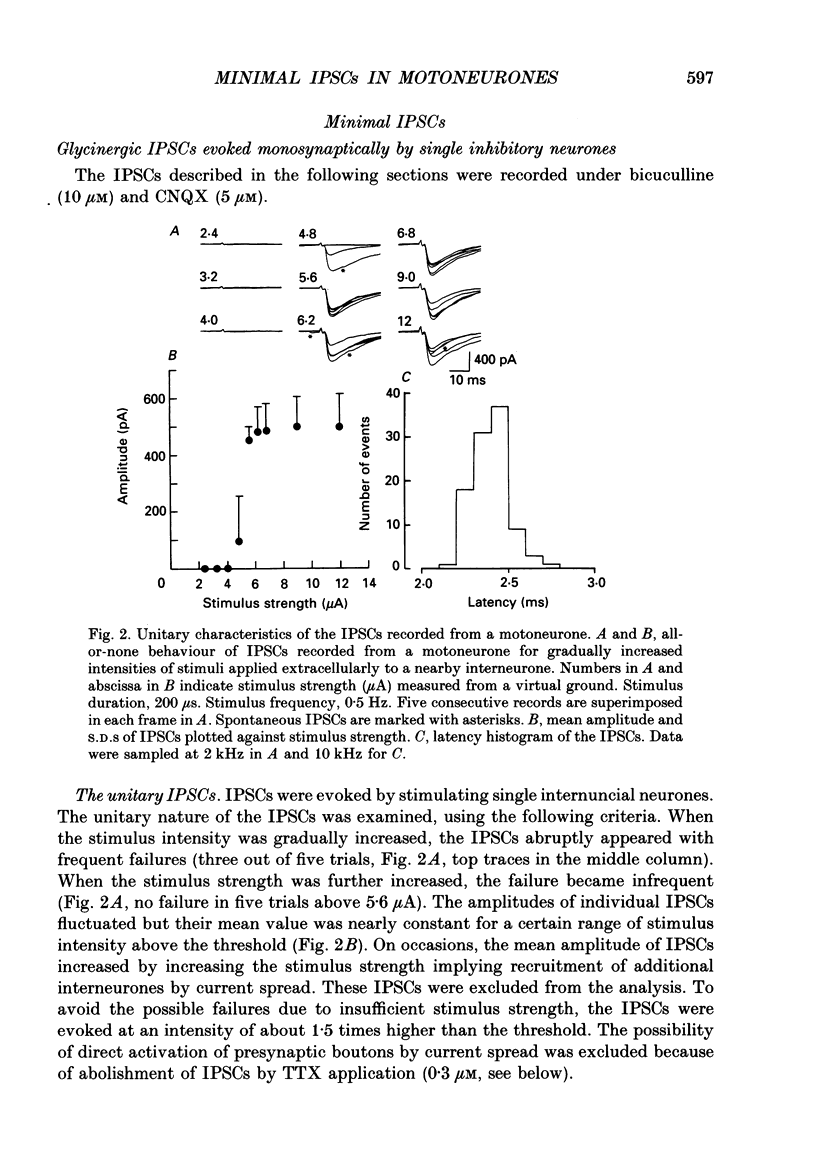
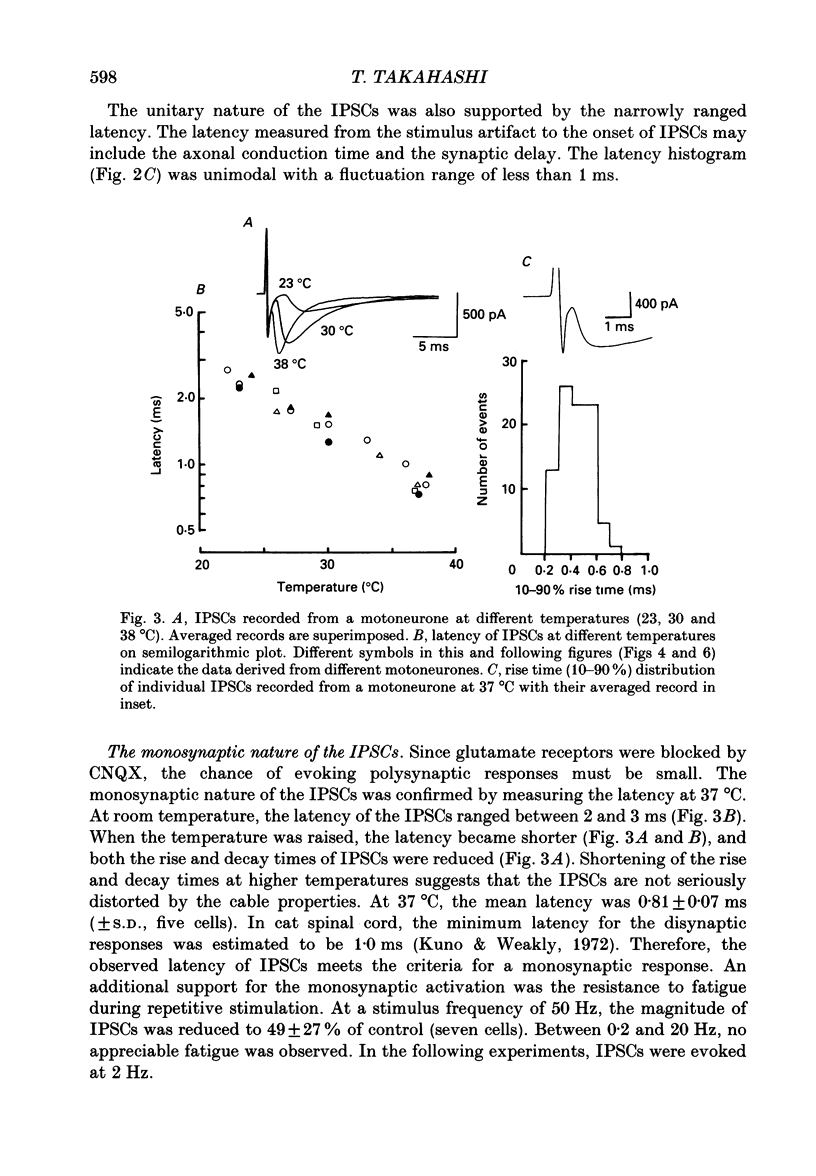
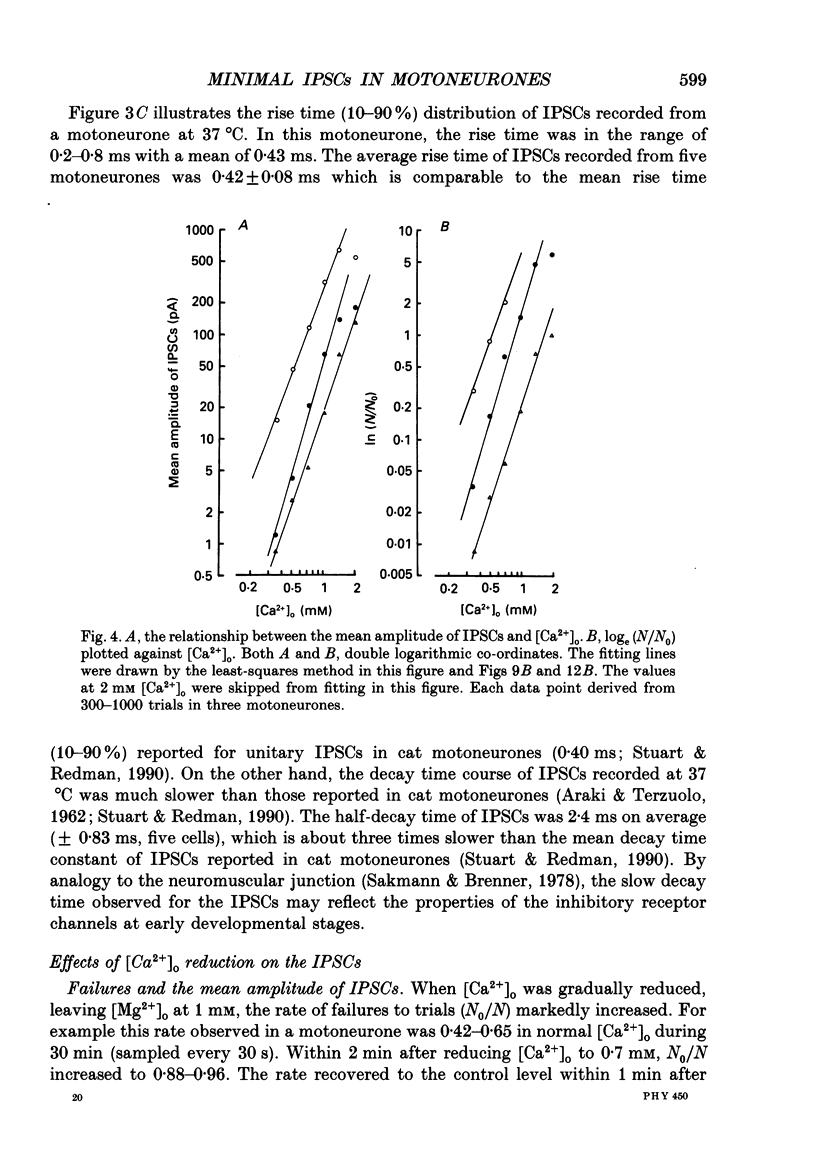
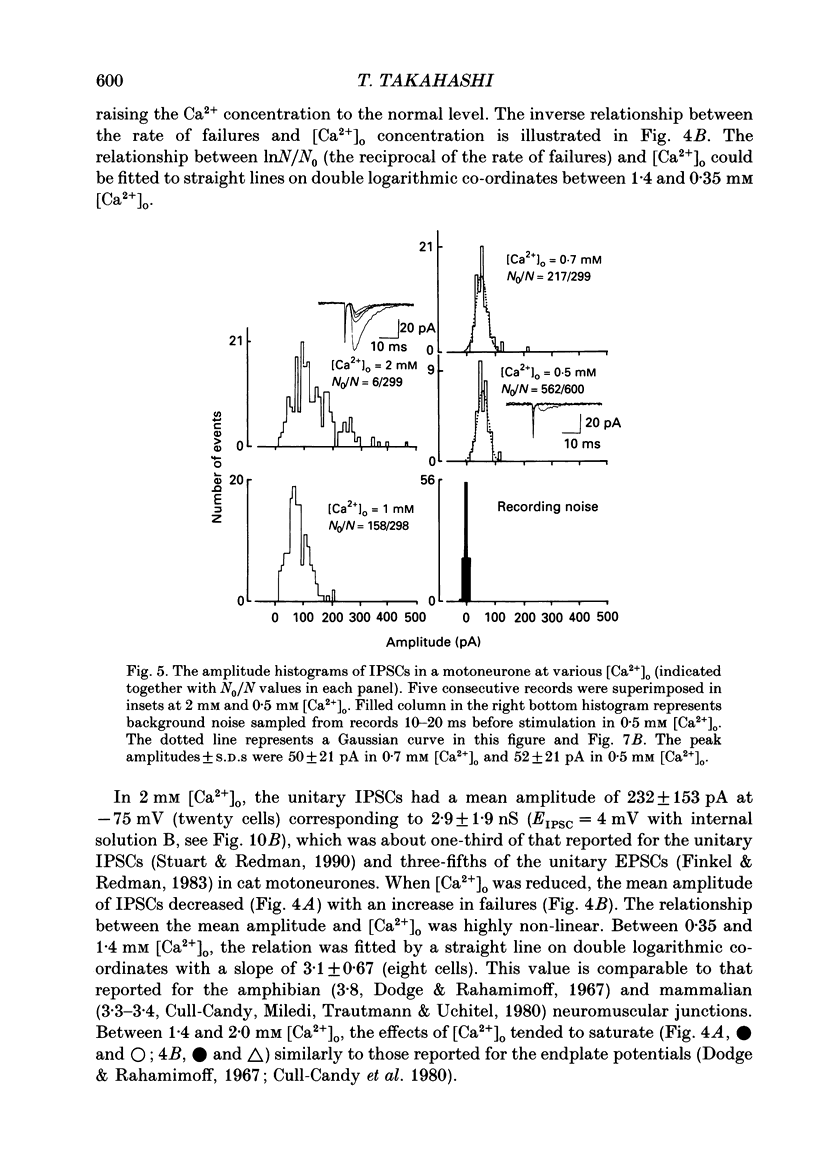
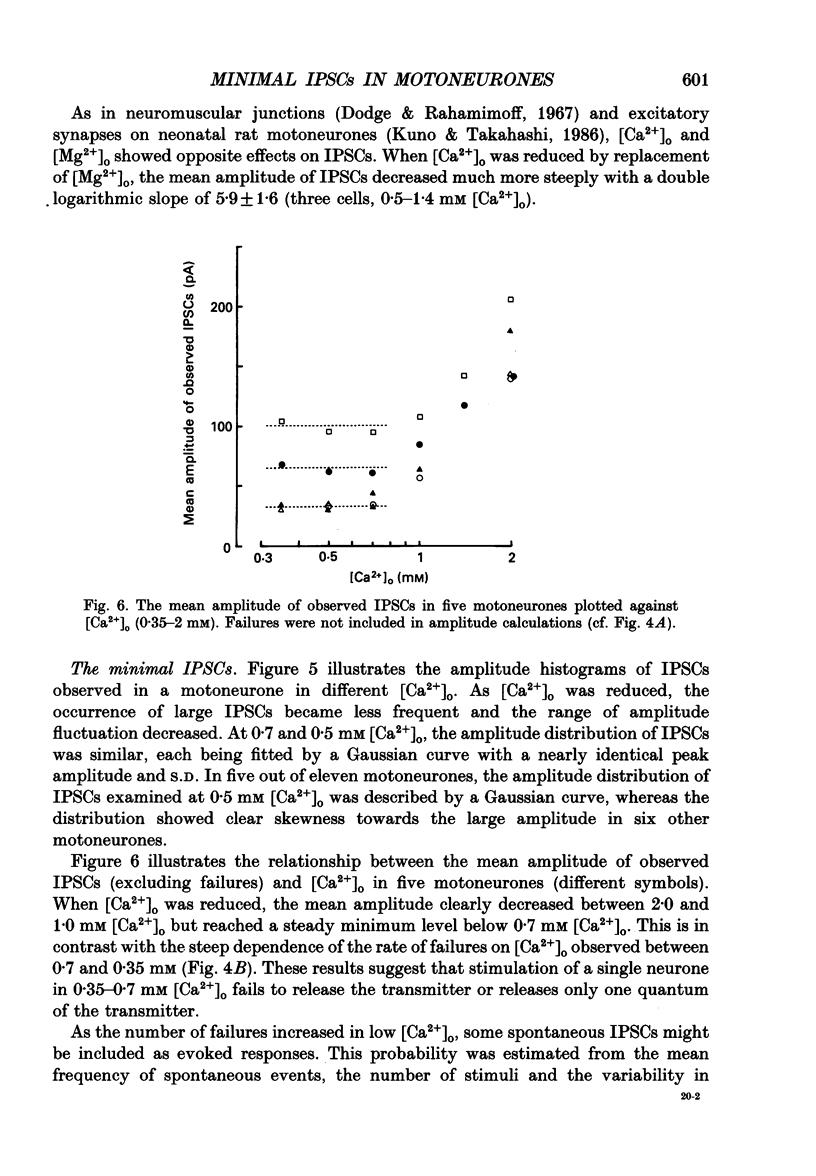
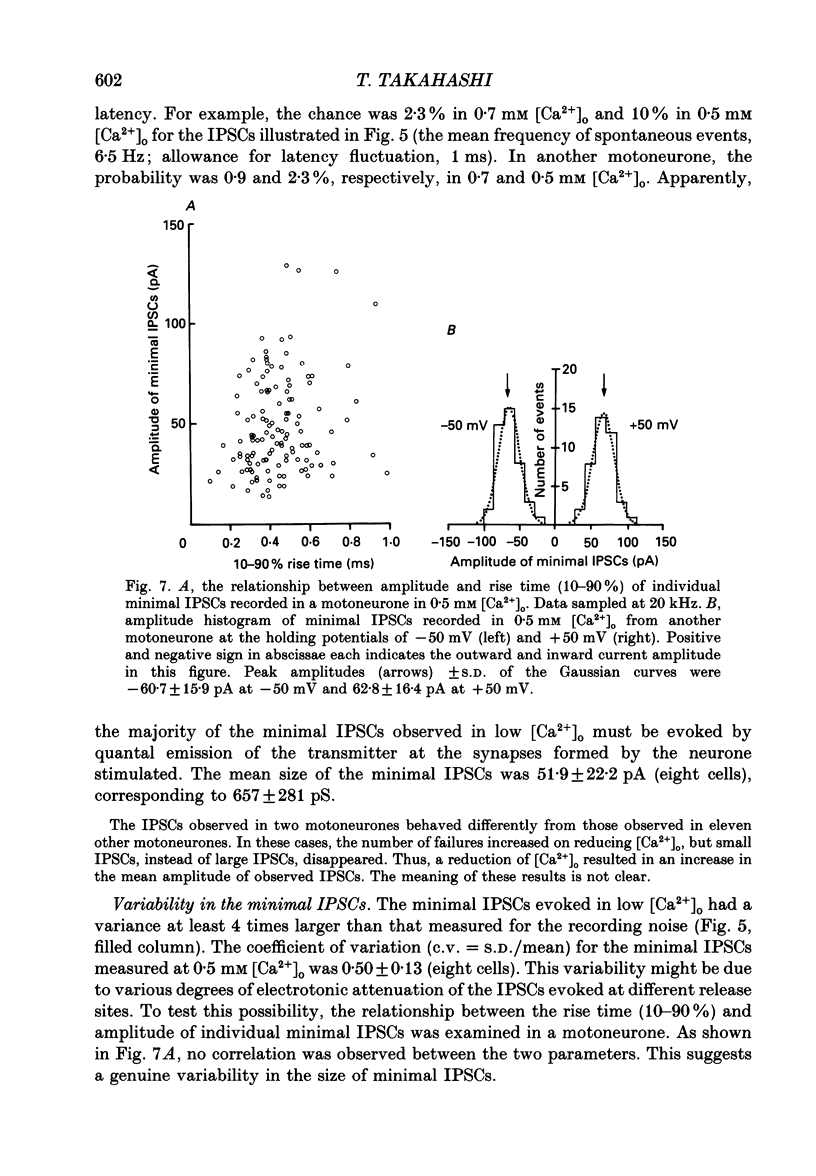
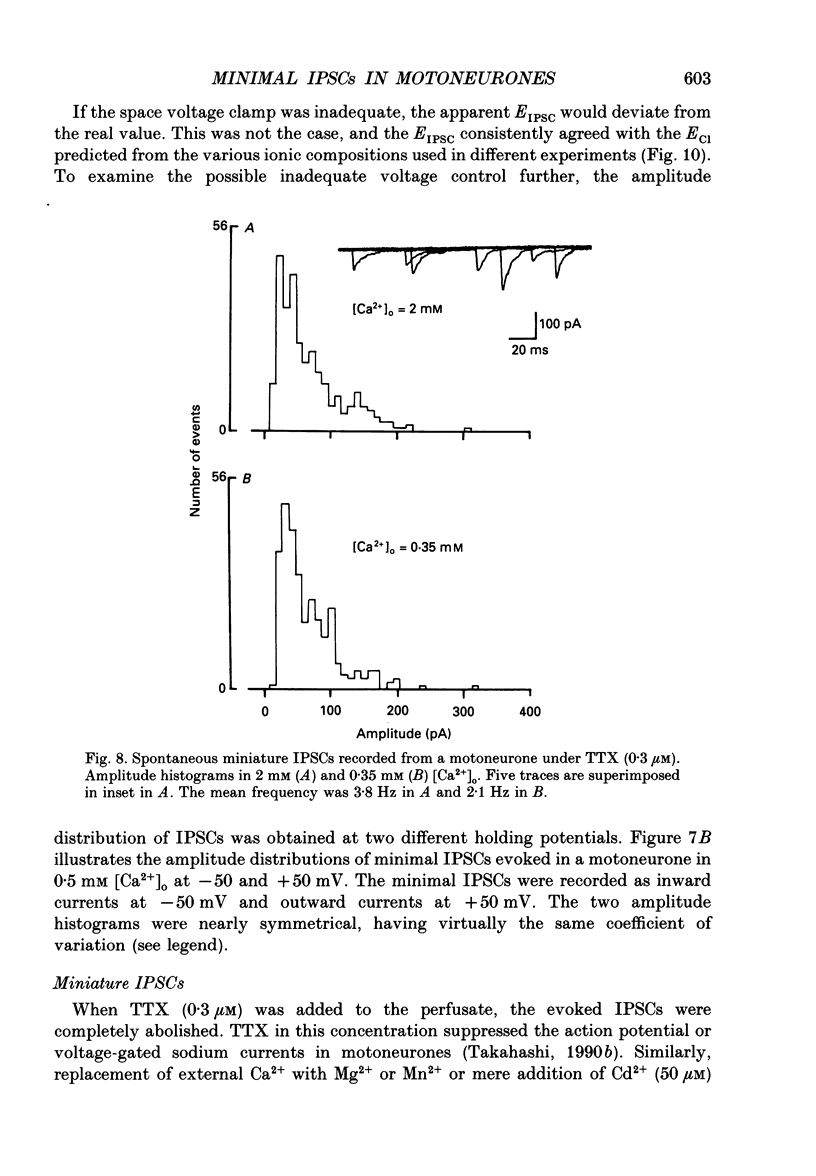
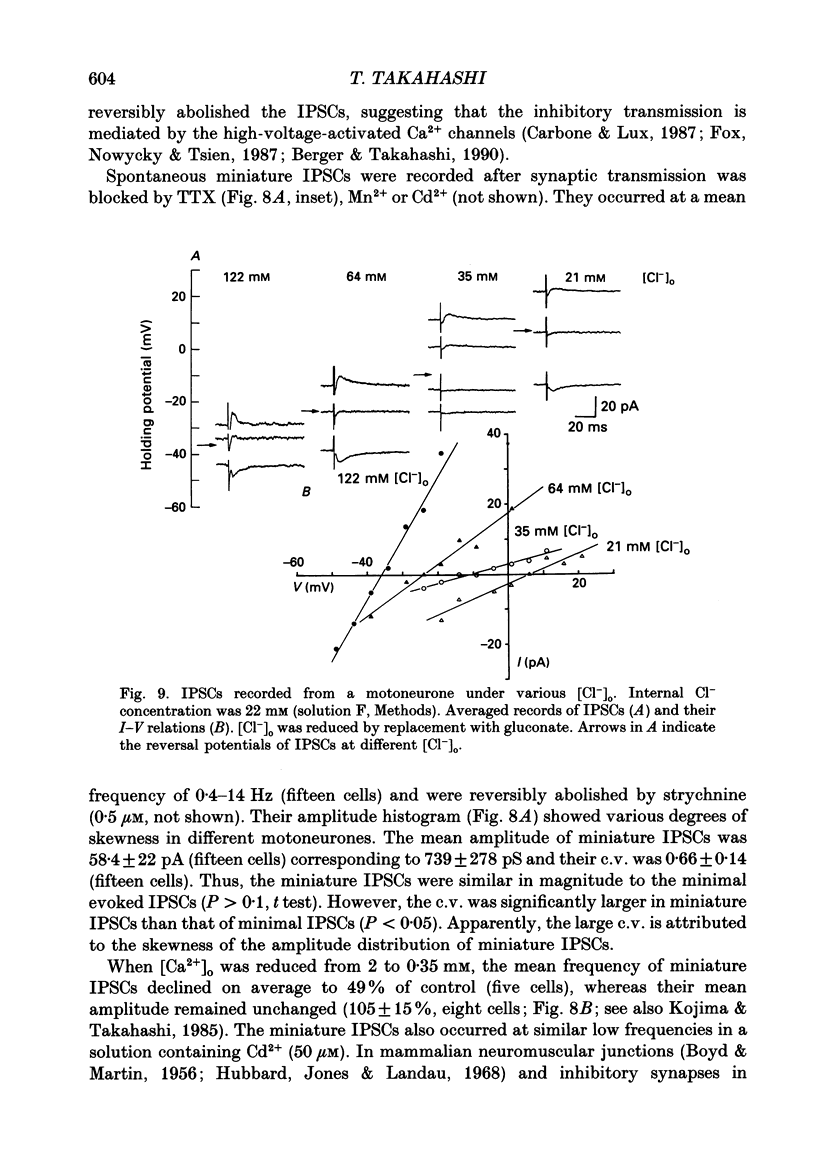
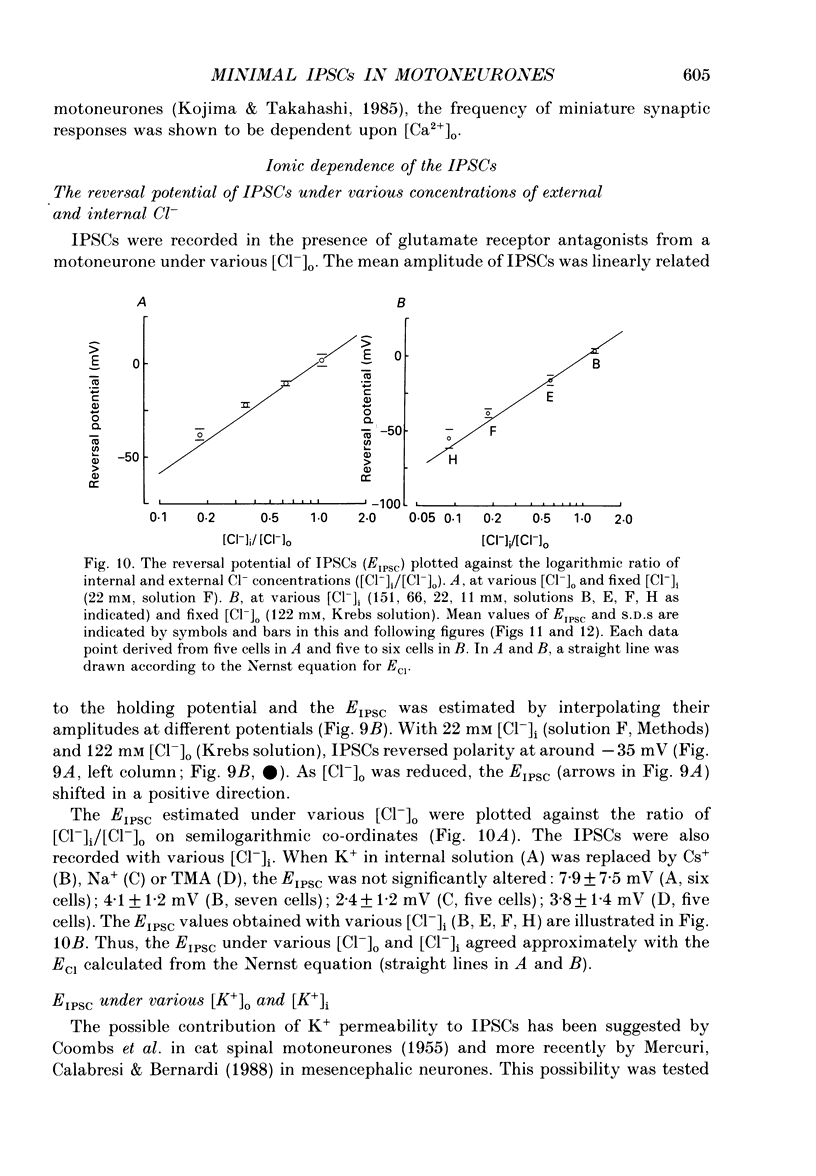
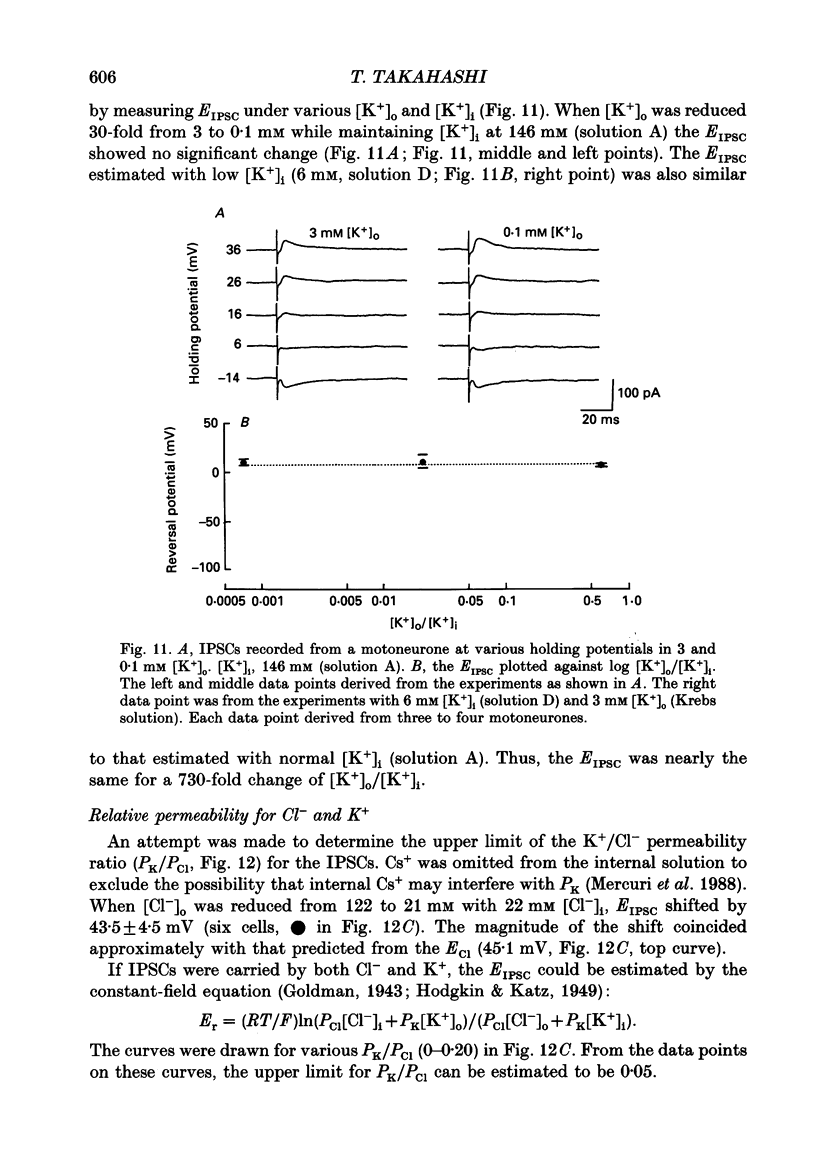
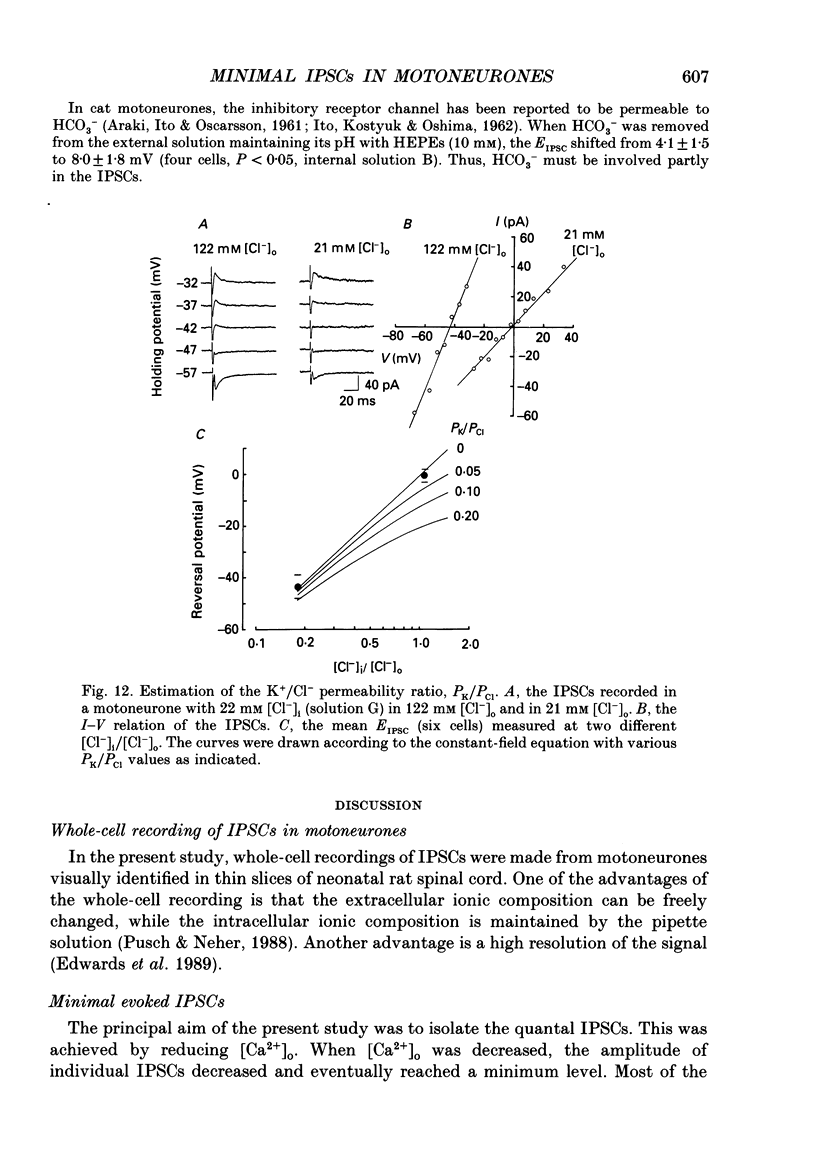
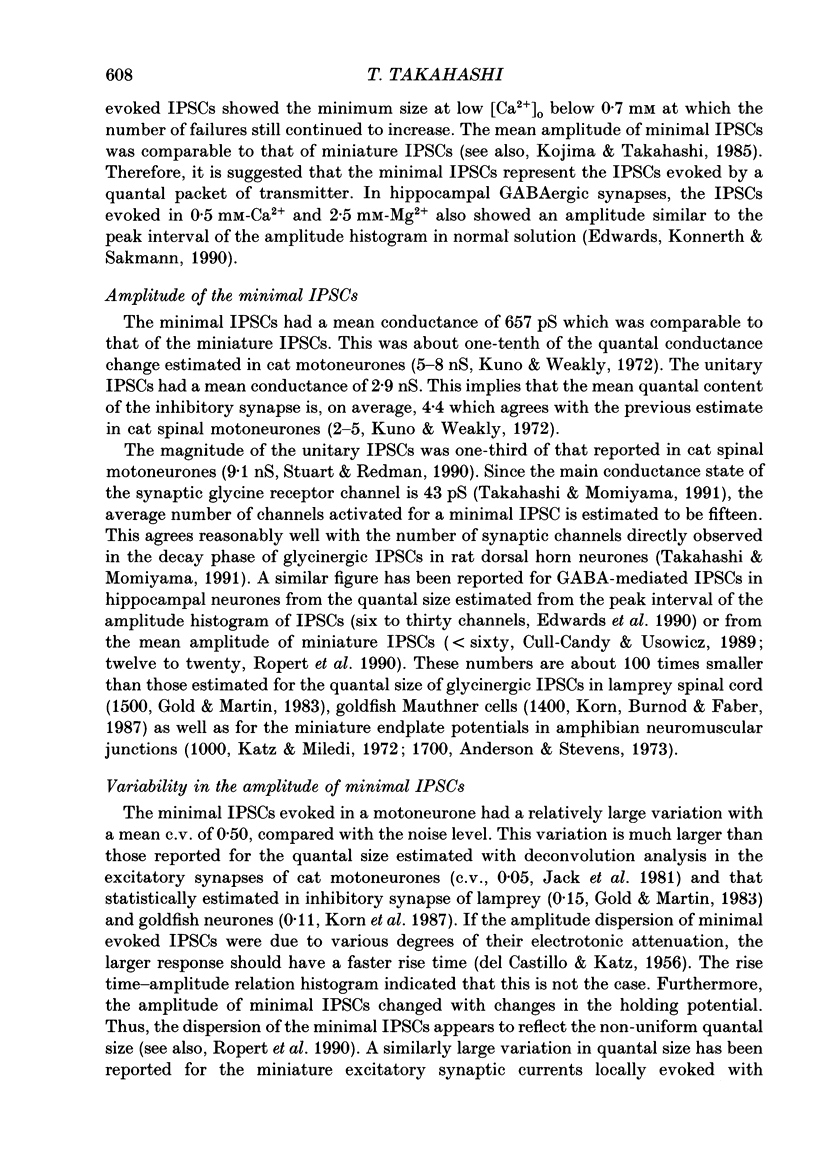
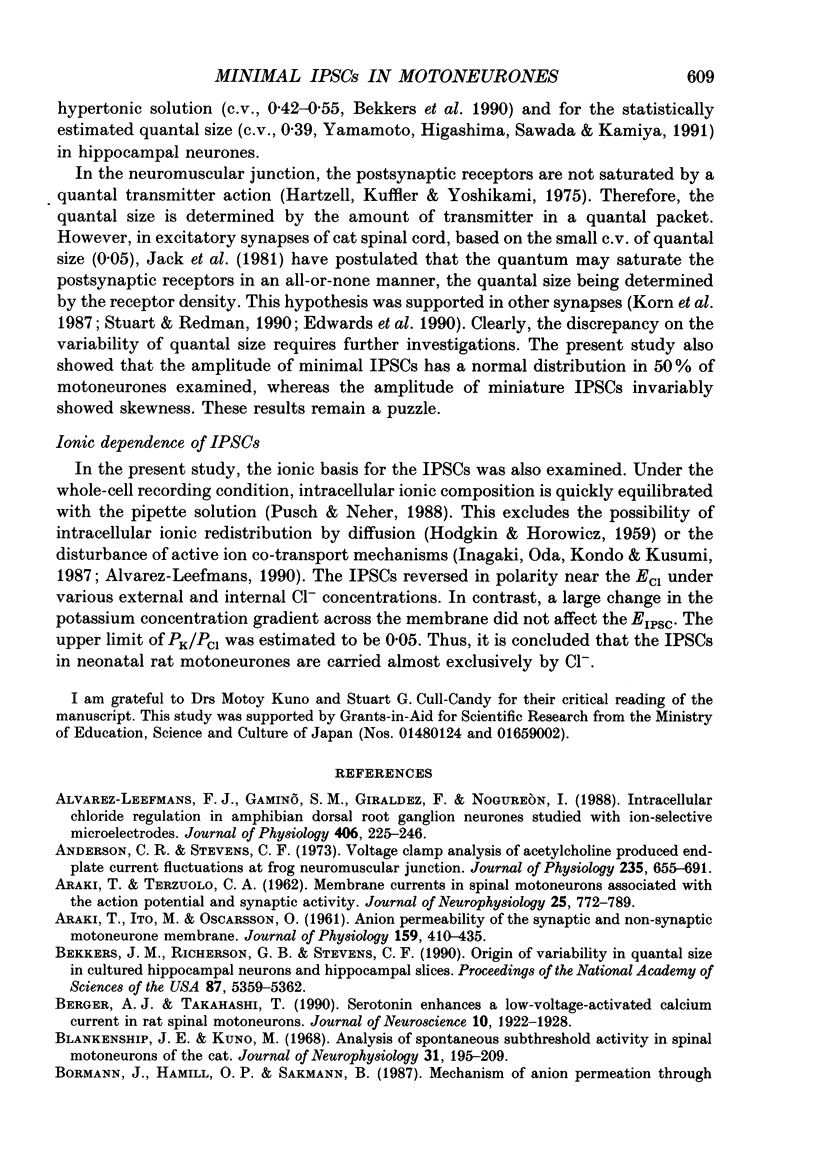
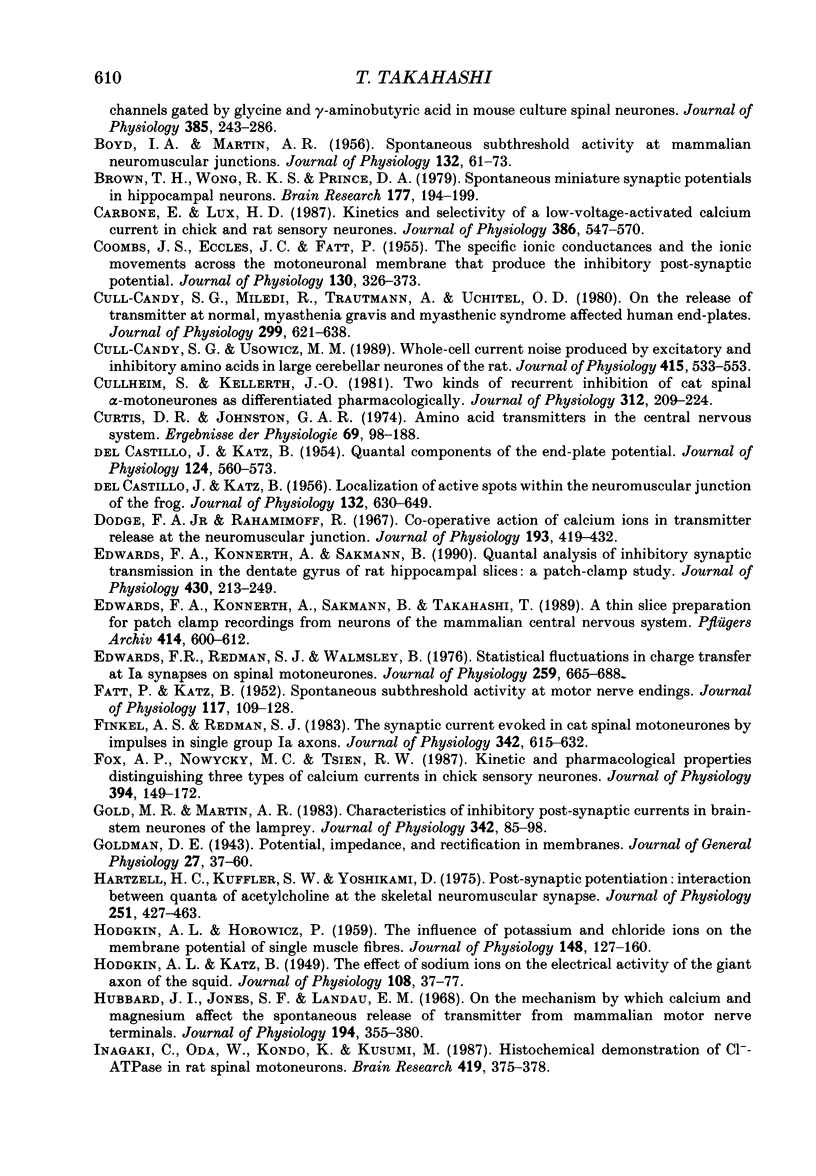
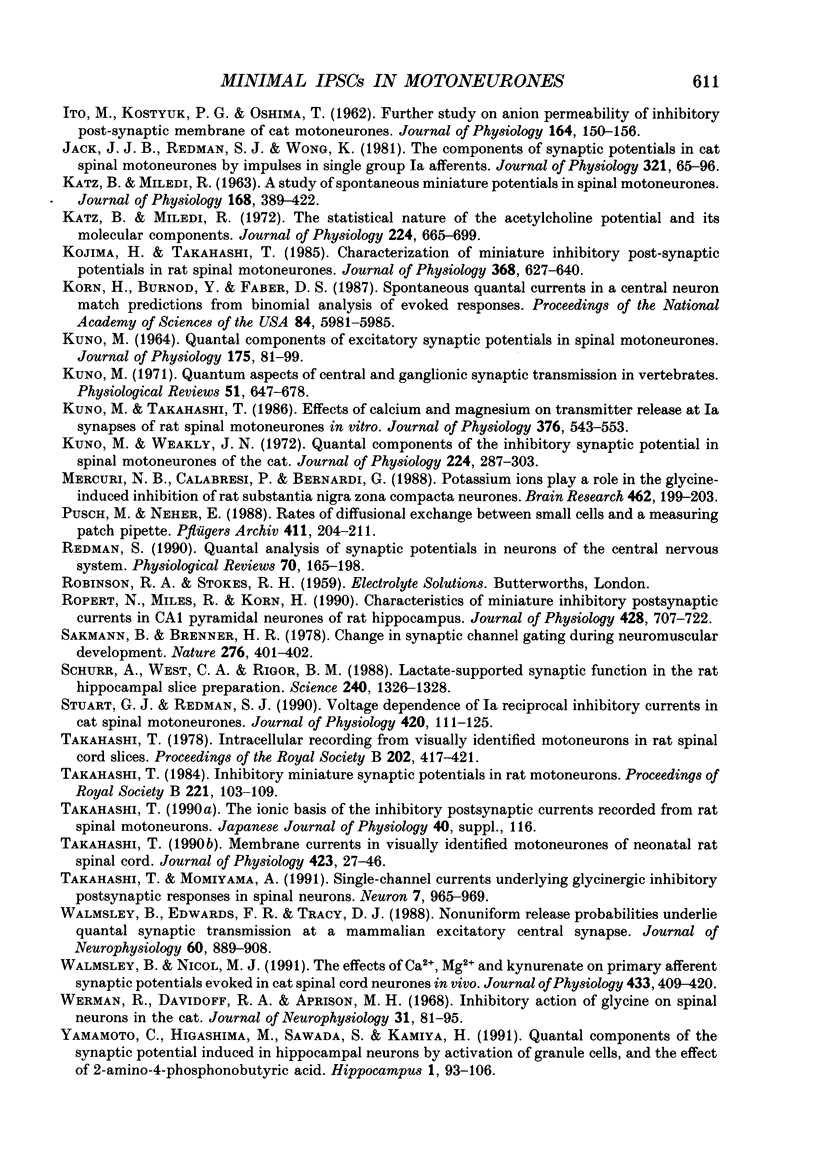
Images in this article
Selected References
These references are in PubMed. This may not be the complete list of references from this article.
- ARAKI T., ITO M., OSCARSSON O. Anion permeability of the synaptic and non-synaptic motoneurone membrane. J Physiol. 1961 Dec;159:410–435. doi: 10.1113/jphysiol.1961.sp006818. [DOI] [PMC free article] [PubMed] [Google Scholar]
- ARAKI T., TERZUOLO C. A. Membrane currents in spinal motoneurons associated with the action potential and synaptic activity. J Neurophysiol. 1962 Nov;25:772–789. doi: 10.1152/jn.1962.25.6.772. [DOI] [PubMed] [Google Scholar]
- Alvarez-Leefmans F. J., Gamiño S. M., Giraldez F., Noguerón I. Intracellular chloride regulation in amphibian dorsal root ganglion neurones studied with ion-selective microelectrodes. J Physiol. 1988 Dec;406:225–246. doi: 10.1113/jphysiol.1988.sp017378. [DOI] [PMC free article] [PubMed] [Google Scholar]
- Anderson C. R., Stevens C. F. Voltage clamp analysis of acetylcholine produced end-plate current fluctuations at frog neuromuscular junction. J Physiol. 1973 Dec;235(3):655–691. doi: 10.1113/jphysiol.1973.sp010410. [DOI] [PMC free article] [PubMed] [Google Scholar]
- BOYD I. A., MARTIN A. R. Spontaneous subthreshold activity at mammalian neural muscular junctions. J Physiol. 1956 Apr 27;132(1):61–73. doi: 10.1113/jphysiol.1956.sp005502. [DOI] [PMC free article] [PubMed] [Google Scholar]
- Bekkers J. M., Richerson G. B., Stevens C. F. Origin of variability in quantal size in cultured hippocampal neurons and hippocampal slices. Proc Natl Acad Sci U S A. 1990 Jul;87(14):5359–5362. doi: 10.1073/pnas.87.14.5359. [DOI] [PMC free article] [PubMed] [Google Scholar]
- Berger A. J., Takahashi T. Serotonin enhances a low-voltage-activated calcium current in rat spinal motoneurons. J Neurosci. 1990 Jun;10(6):1922–1928. doi: 10.1523/JNEUROSCI.10-06-01922.1990. [DOI] [PMC free article] [PubMed] [Google Scholar]
- Blankenship J. E., Kuno M. Analysis of spontaneous subthreshold activity in spinal motoneurons of the cat. J Neurophysiol. 1968 Mar;31(2):195–209. doi: 10.1152/jn.1968.31.2.195. [DOI] [PubMed] [Google Scholar]
- Bormann J., Hamill O. P., Sakmann B. Mechanism of anion permeation through channels gated by glycine and gamma-aminobutyric acid in mouse cultured spinal neurones. J Physiol. 1987 Apr;385:243–286. doi: 10.1113/jphysiol.1987.sp016493. [DOI] [PMC free article] [PubMed] [Google Scholar]
- Brown T. H., Wong R. K., Prince D. A. Spontaneous miniature synaptic potentials in hippocampal neurons. Brain Res. 1979 Nov 9;177(1):194–199. doi: 10.1016/0006-8993(79)90931-4. [DOI] [PubMed] [Google Scholar]
- COOMBS J. S., ECCLES J. C., FATT P. The specific ionic conductances and the ionic movements across the motoneuronal membrane that produce the inhibitory post-synaptic potential. J Physiol. 1955 Nov 28;130(2):326–374. doi: 10.1113/jphysiol.1955.sp005412. [DOI] [PMC free article] [PubMed] [Google Scholar]
- Carbone E., Lux H. D. Kinetics and selectivity of a low-voltage-activated calcium current in chick and rat sensory neurones. J Physiol. 1987 May;386:547–570. doi: 10.1113/jphysiol.1987.sp016551. [DOI] [PMC free article] [PubMed] [Google Scholar]
- Cull-Candy S. G., Miledi R., Trautmann A., Uchitel O. D. On the release of transmitter at normal, myasthenia gravis and myasthenic syndrome affected human end-plates. J Physiol. 1980 Feb;299:621–638. doi: 10.1113/jphysiol.1980.sp013145. [DOI] [PMC free article] [PubMed] [Google Scholar]
- Cull-Candy S. G., Usowicz M. M. Whole-cell current noise produced by excitatory and inhibitory amino acids in large cerebellar neurones of the rat. J Physiol. 1989 Aug;415:533–553. doi: 10.1113/jphysiol.1989.sp017735. [DOI] [PMC free article] [PubMed] [Google Scholar]
- Cullheim S., Kellerth J. O. Two kinds of recurrent inhibition of cat spinal alpha-motoneurones as differentiated pharmacologically. J Physiol. 1981 Mar;312:209–224. doi: 10.1113/jphysiol.1981.sp013624. [DOI] [PMC free article] [PubMed] [Google Scholar]
- Curtis D. R., Johnston G. A. Amino acid transmitters in the mammalian central nervous system. Ergeb Physiol. 1974;69(0):97–188. doi: 10.1007/3-540-06498-2_3. [DOI] [PubMed] [Google Scholar]
- DEL CASTILLO J., KATZ B. Localization of active spots within the neuromuscular junction of the frog. J Physiol. 1956 Jun 28;132(3):630–649. doi: 10.1113/jphysiol.1956.sp005554. [DOI] [PMC free article] [PubMed] [Google Scholar]
- DEL CASTILLO J., KATZ B. Quantal components of the end-plate potential. J Physiol. 1954 Jun 28;124(3):560–573. doi: 10.1113/jphysiol.1954.sp005129. [DOI] [PMC free article] [PubMed] [Google Scholar]
- Dodge F. A., Jr, Rahamimoff R. Co-operative action a calcium ions in transmitter release at the neuromuscular junction. J Physiol. 1967 Nov;193(2):419–432. doi: 10.1113/jphysiol.1967.sp008367. [DOI] [PMC free article] [PubMed] [Google Scholar]
- Edwards F. A., Konnerth A., Sakmann B. Quantal analysis of inhibitory synaptic transmission in the dentate gyrus of rat hippocampal slices: a patch-clamp study. J Physiol. 1990 Nov;430:213–249. doi: 10.1113/jphysiol.1990.sp018289. [DOI] [PMC free article] [PubMed] [Google Scholar]
- Edwards F. A., Konnerth A., Sakmann B., Takahashi T. A thin slice preparation for patch clamp recordings from neurones of the mammalian central nervous system. Pflugers Arch. 1989 Sep;414(5):600–612. doi: 10.1007/BF00580998. [DOI] [PubMed] [Google Scholar]
- Edwards F. R., Redman S. J., Walmsley B. Statistical fluctuations in charge transfer at Ia synapses on spinal motoneurones. J Physiol. 1976 Aug;259(3):665–688. doi: 10.1113/jphysiol.1976.sp011488. [DOI] [PMC free article] [PubMed] [Google Scholar]
- FATT P., KATZ B. Spontaneous subthreshold activity at motor nerve endings. J Physiol. 1952 May;117(1):109–128. [PMC free article] [PubMed] [Google Scholar]
- Finkel A. S., Redman S. J. The synaptic current evoked in cat spinal motoneurones by impulses in single group 1a axons. J Physiol. 1983 Sep;342:615–632. doi: 10.1113/jphysiol.1983.sp014872. [DOI] [PMC free article] [PubMed] [Google Scholar]
- Fox A. P., Nowycky M. C., Tsien R. W. Kinetic and pharmacological properties distinguishing three types of calcium currents in chick sensory neurones. J Physiol. 1987 Dec;394:149–172. doi: 10.1113/jphysiol.1987.sp016864. [DOI] [PMC free article] [PubMed] [Google Scholar]
- Gold M. R., Martin A. R. Characteristics of inhibitory post-synaptic currents in brain-stem neurones of the lamprey. J Physiol. 1983 Sep;342:85–98. doi: 10.1113/jphysiol.1983.sp014841. [DOI] [PMC free article] [PubMed] [Google Scholar]
- HODGKIN A. L., HOROWICZ P. The influence of potassium and chloride ions on the membrane potential of single muscle fibres. J Physiol. 1959 Oct;148:127–160. doi: 10.1113/jphysiol.1959.sp006278. [DOI] [PMC free article] [PubMed] [Google Scholar]
- HODGKIN A. L., KATZ B. The effect of sodium ions on the electrical activity of giant axon of the squid. J Physiol. 1949 Mar 1;108(1):37–77. doi: 10.1113/jphysiol.1949.sp004310. [DOI] [PMC free article] [PubMed] [Google Scholar]
- Hartzell H. C., Kuffler S. W., Yoshikami D. Post-synaptic potentiation: interaction between quanta of acetylcholine at the skeletal neuromuscular synapse. J Physiol. 1975 Oct;251(2):427–463. doi: 10.1113/jphysiol.1975.sp011102. [DOI] [PMC free article] [PubMed] [Google Scholar]
- Hubbard J. I., Jones S. F., Landau E. M. On the mechanism by which calcium and magnesium affect the spontaneous release of transmitter from mammalian motor nerve terminals. J Physiol. 1968 Feb;194(2):355–380. doi: 10.1113/jphysiol.1968.sp008413. [DOI] [PMC free article] [PubMed] [Google Scholar]
- ITO M., KOSTYUK P. G., OSHIMA T. Further study on anion permeability of inhibitory post-synaptic membrane of cat motoneurones. J Physiol. 1962 Oct;164:150–156. doi: 10.1113/jphysiol.1962.sp007009. [DOI] [PMC free article] [PubMed] [Google Scholar]
- Inagaki C., Oda W., Kondo K., Kusumi M. Histochemical demonstration of Cl(-)-ATPase in rat spinal motoneurons. Brain Res. 1987 Sep 1;419(1-2):375–378. doi: 10.1016/0006-8993(87)90611-1. [DOI] [PubMed] [Google Scholar]
- Jack J. J., Redman S. J., Wong K. The components of synaptic potentials evoked in cat spinal motoneurones by impulses in single group Ia afferents. J Physiol. 1981 Dec;321:65–96. doi: 10.1113/jphysiol.1981.sp013972. [DOI] [PMC free article] [PubMed] [Google Scholar]
- KATZ B., MILEDI R. A STUDY OF SPONTANEOUS MINIATURE POTENTIALS IN SPINAL MOTONEURONES. J Physiol. 1963 Sep;168:389–422. doi: 10.1113/jphysiol.1963.sp007199. [DOI] [PMC free article] [PubMed] [Google Scholar]
- KUNO M. QUANTAL COMPONENTS OF EXCITATORY SYNAPTIC POTENTIALS IN SPINAL MOTONEURONES. J Physiol. 1964 Dec;175:81–99. doi: 10.1113/jphysiol.1964.sp007504. [DOI] [PMC free article] [PubMed] [Google Scholar]
- Katz B., Miledi R. The statistical nature of the acetycholine potential and its molecular components. J Physiol. 1972 Aug;224(3):665–699. doi: 10.1113/jphysiol.1972.sp009918. [DOI] [PMC free article] [PubMed] [Google Scholar]
- Kojima H., Takahashi T. Characterization of miniature inhibitory post-synaptic potentials in rat spinal motoneurones. J Physiol. 1985 Nov;368:627–640. doi: 10.1113/jphysiol.1985.sp015880. [DOI] [PMC free article] [PubMed] [Google Scholar]
- Korn H., Burnod Y., Faber D. S. Spontaneous quantal currents in a central neuron match predictions from binomial analysis of evoked responses. Proc Natl Acad Sci U S A. 1987 Aug;84(16):5981–5985. doi: 10.1073/pnas.84.16.5981. [DOI] [PMC free article] [PubMed] [Google Scholar]
- Kuno M. Quantum aspects of central and ganglionic synaptic transmission in vertebrates. Physiol Rev. 1971 Oct;51(4):647–678. doi: 10.1152/physrev.1971.51.4.647. [DOI] [PubMed] [Google Scholar]
- Kuno M., Takahashi T. Effects of calcium and magnesium on transmitter release at Ia synapses of rat spinal motoneurones in vitro. J Physiol. 1986 Jul;376:543–553. doi: 10.1113/jphysiol.1986.sp016169. [DOI] [PMC free article] [PubMed] [Google Scholar]
- Kuno M., Weakly J. N. Quantal components of the inhibitory synaptic potential in spinal mononeurones of the cat. J Physiol. 1972 Jul;224(2):287–303. doi: 10.1113/jphysiol.1972.sp009895. [DOI] [PMC free article] [PubMed] [Google Scholar]
- Mercuri N. B., Calabresi P., Bernardi G. Potassium ions play a role in the glycine-induced inhibition of rat substantia nigra zona compacta neurones. Brain Res. 1988 Oct 11;462(1):199–203. doi: 10.1016/0006-8993(88)90607-5. [DOI] [PubMed] [Google Scholar]
- Pusch M., Neher E. Rates of diffusional exchange between small cells and a measuring patch pipette. Pflugers Arch. 1988 Feb;411(2):204–211. doi: 10.1007/BF00582316. [DOI] [PubMed] [Google Scholar]
- Redman S. Quantal analysis of synaptic potentials in neurons of the central nervous system. Physiol Rev. 1990 Jan;70(1):165–198. doi: 10.1152/physrev.1990.70.1.165. [DOI] [PubMed] [Google Scholar]
- Ropert N., Miles R., Korn H. Characteristics of miniature inhibitory postsynaptic currents in CA1 pyramidal neurones of rat hippocampus. J Physiol. 1990 Sep;428:707–722. doi: 10.1113/jphysiol.1990.sp018236. [DOI] [PMC free article] [PubMed] [Google Scholar]
- Sakmann B., Brenner H. R. Change in synaptic channel gating during neuromuscular development. Nature. 1978 Nov 23;276(5686):401–402. doi: 10.1038/276401a0. [DOI] [PubMed] [Google Scholar]
- Schurr A., West C. A., Rigor B. M. Lactate-supported synaptic function in the rat hippocampal slice preparation. Science. 1988 Jun 3;240(4857):1326–1328. doi: 10.1126/science.3375817. [DOI] [PubMed] [Google Scholar]
- Stuart G. J., Redman S. J. Voltage dependence of Ia reciprocal inhibitory currents in cat spinal motoneurones. J Physiol. 1990 Jan;420:111–125. doi: 10.1113/jphysiol.1990.sp017903. [DOI] [PMC free article] [PubMed] [Google Scholar]
- Takahashi T. Inhibitory miniature synaptic potentials in rat motoneurons. Proc R Soc Lond B Biol Sci. 1984 Mar 22;221(1222):103–109. doi: 10.1098/rspb.1984.0025. [DOI] [PubMed] [Google Scholar]
- Takahashi T. Intracellular recording from visually identified motoneurons in rat spinal cord slices. Proc R Soc Lond B Biol Sci. 1978 Jul 26;202(1148):417–421. doi: 10.1098/rspb.1978.0076. [DOI] [PubMed] [Google Scholar]
- Takahashi T. Membrane currents in visually identified motoneurones of neonatal rat spinal cord. J Physiol. 1990 Apr;423:27–46. doi: 10.1113/jphysiol.1990.sp018009. [DOI] [PMC free article] [PubMed] [Google Scholar]
- Takahashi T., Momiyama A. Single-channel currents underlying glycinergic inhibitory postsynaptic responses in spinal neurons. Neuron. 1991 Dec;7(6):965–969. doi: 10.1016/0896-6273(91)90341-v. [DOI] [PubMed] [Google Scholar]
- Walmsley B., Edwards F. R., Tracey D. J. Nonuniform release probabilities underlie quantal synaptic transmission at a mammalian excitatory central synapse. J Neurophysiol. 1988 Sep;60(3):889–908. doi: 10.1152/jn.1988.60.3.889. [DOI] [PubMed] [Google Scholar]
- Walmsley B., Nicol M. J. The effects of Ca2+, Mg2+ and kynurenate on primary afferent synaptic potentials evoked in cat spinal cord neurones in vivo. J Physiol. 1991 Feb;433:409–420. doi: 10.1113/jphysiol.1991.sp018434. [DOI] [PMC free article] [PubMed] [Google Scholar]
- Werman R., Davidoff R. A., Aprison M. H. Inhibitory of glycine on spinal neurons in the cat. J Neurophysiol. 1968 Jan;31(1):81–95. doi: 10.1152/jn.1968.31.1.81. [DOI] [PubMed] [Google Scholar]
- Yamamoto C., Higashima M., Sawada S., Kamiya H. Quantal components of the synaptic potential induced in hippocampal neurons by activation of granule cells, and the effect of 2-amino-4-phosphonobutyric acid. Hippocampus. 1991 Jan;1(1):93–106. doi: 10.1002/hipo.450010109. [DOI] [PubMed] [Google Scholar]