Abstract
1. The effect of elevated muscle glycogen on anaerobic energy production, and glycogenolytic and glycolytic rates was examined in man by using the one-legged knee extension model, which enables evaluation of metabolism in a well-defined muscle group. 2. Six subjects performed very intense exercise to exhaustion (EX1) with one leg with normal glycogen (control) and one with a very high concentration (HG). With each leg, the exhaustive exercise was repeated after 1 h of recovery (EX2). Prior to and immediately after each exercise bout, a muscle biopsy was taken from m. vastus lateralis of the active leg for determination of glycogen, lactate, creatine phosphate (CP) and nucleotide concentrations. Measurements of leg blood flow and femoral arterial-venous differences for oxygen content, lactate, glucose, free fatty acids and potassium were performed before and regularly during the exhaustive exercises. 3. Muscle glycogen concentration prior to EX1 was 87.0 and 176.8 mmol (kg wet wt)-1 for the control and HG leg, respectively, and the decreases during exercise were 26.3 (control) and 25.6 (HG) mmol (kg wet wt)-1. The net glycogen utilization rate was not related to pre-exercise muscle glycogen concentration. Muscle lactate concentration at the end of EX1 was 18.8 (control) and 16.1 (HG) mmol (kg wet wt)-1, and the net lactate production (including lactate release) was 26.5 (control) and 23.6 (HG) mmol (kg wet wt)-1. Rate of lactate production was unrelated to initial muscle glycogen level. Time to exhaustion for EX1 was the same for the control leg (2.82 min) and HG leg (2.92 min). 4. Muscle glycogen concentration before EX2 was 14 mmol (kg wet wt)-1 lower than prior to EX1. During EX2 the muscle glycogen decline of 19.6 mmol (kg wet wt)-1 for the control leg was less than for the HG leg (26.2 mmol (kg wet wt)-1). The muscle lactate concentrations at the end of EX2 were about 7-8 mmol (kg wet wt)-1 lower compared to EX1, and the net lactate production was reduced by 40%. The exercise time during EX2 was 0.35 min shorter for the control leg, while no difference was observed for the HG leg. 5. Total reduction in ATP and CP was similar during the four exercise bouts, while a higher accumulation of inosine monophosphate (IMP) occurred during EX2 for the control leg (0.72 mmol (kg wet wt)-1) compared to the HG leg (0.20 mmol (kg wet wt)-1).(ABSTRACT TRUNCATED AT 400 WORDS)
Full text
PDF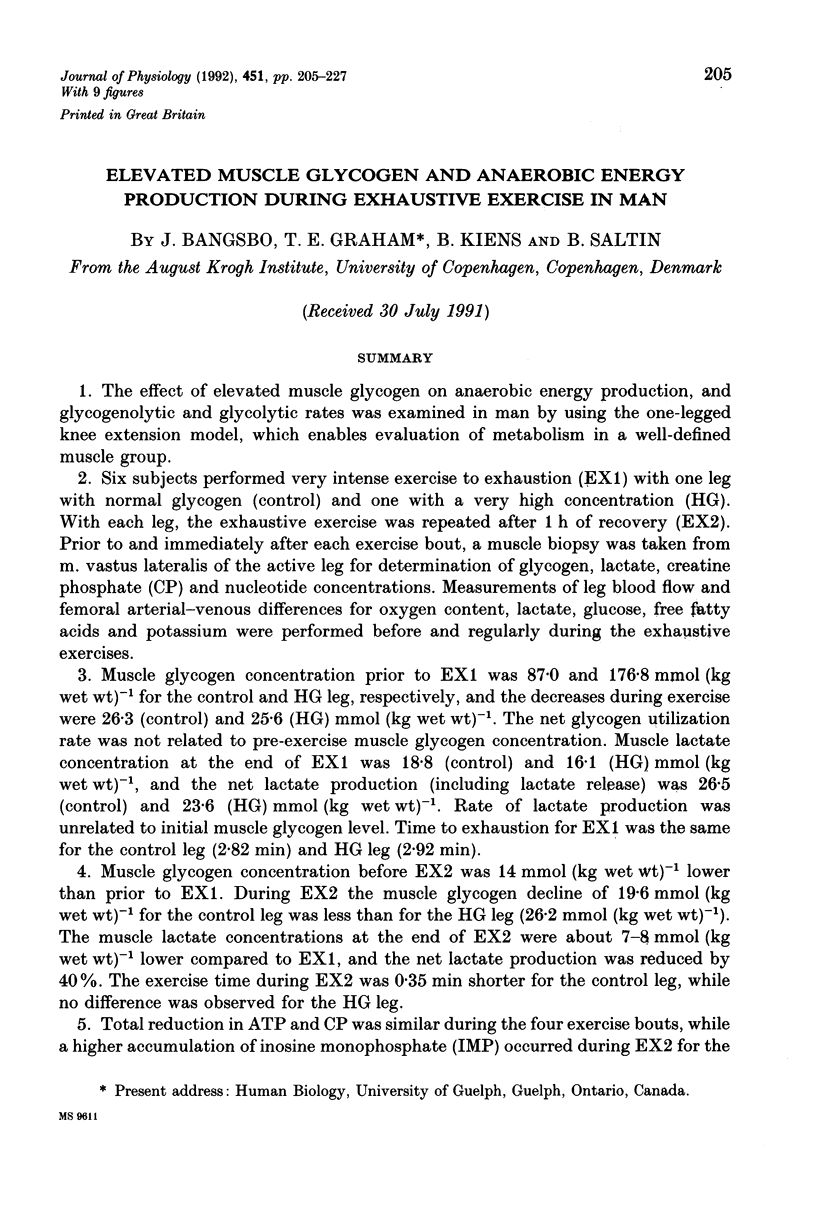
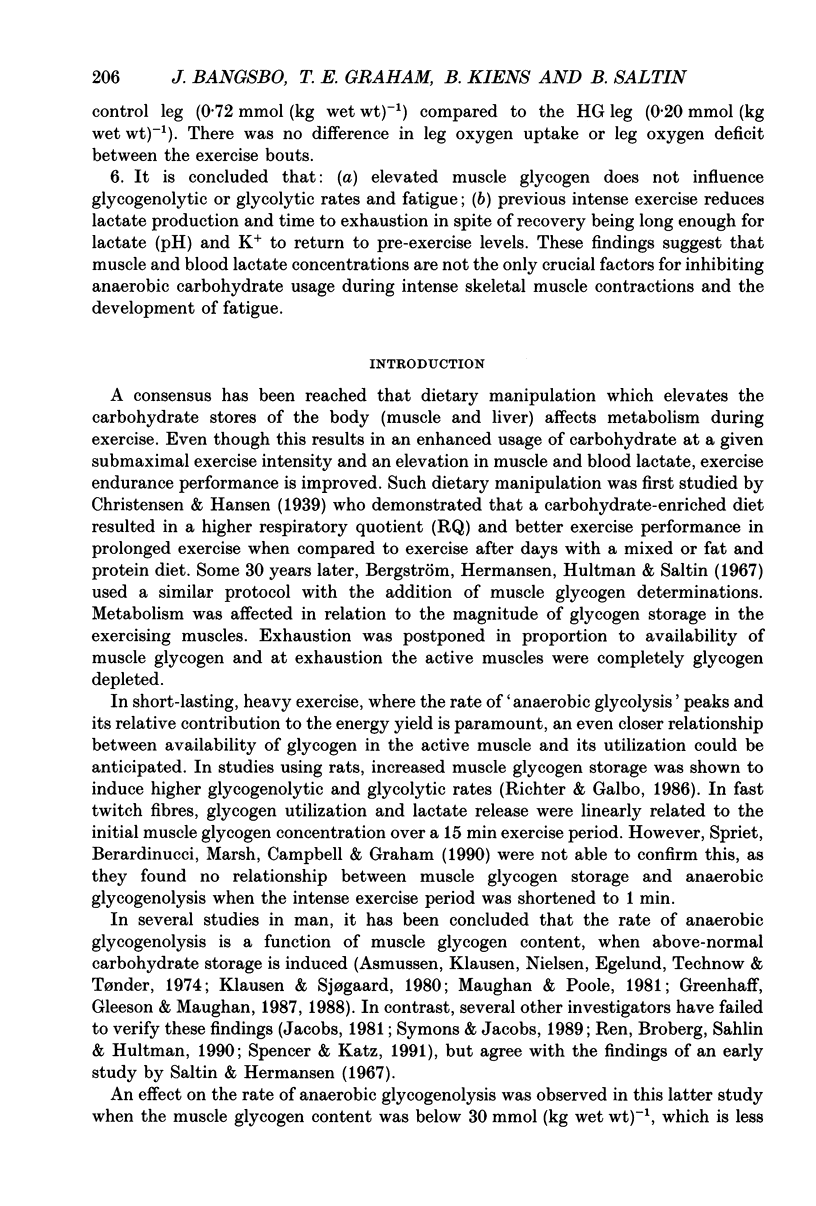
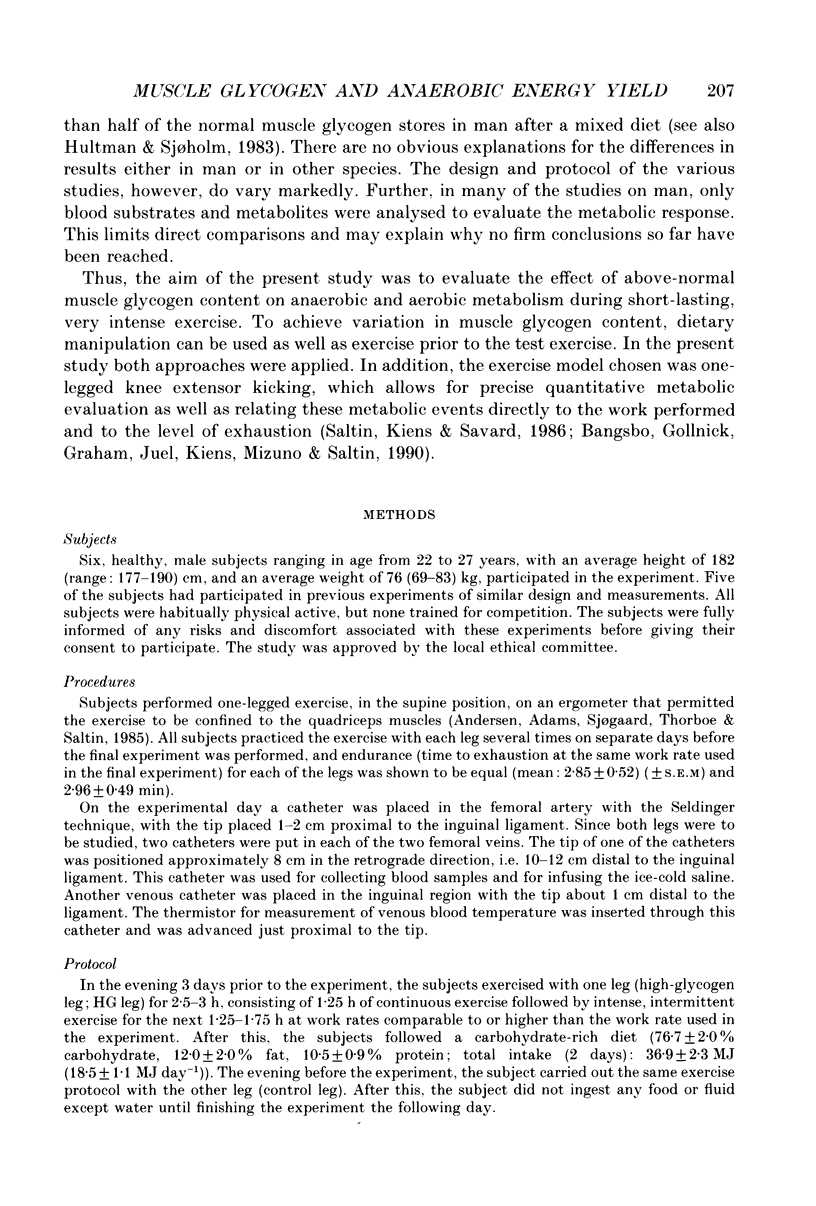
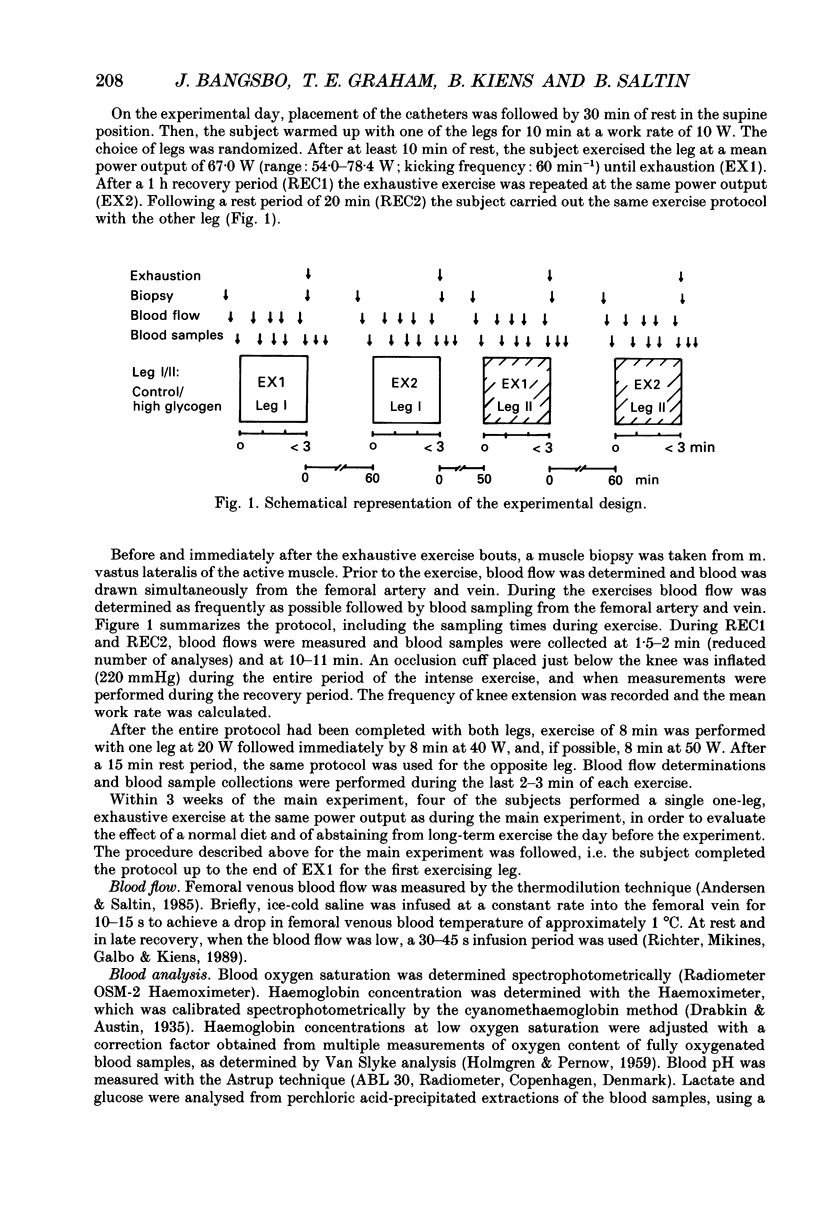
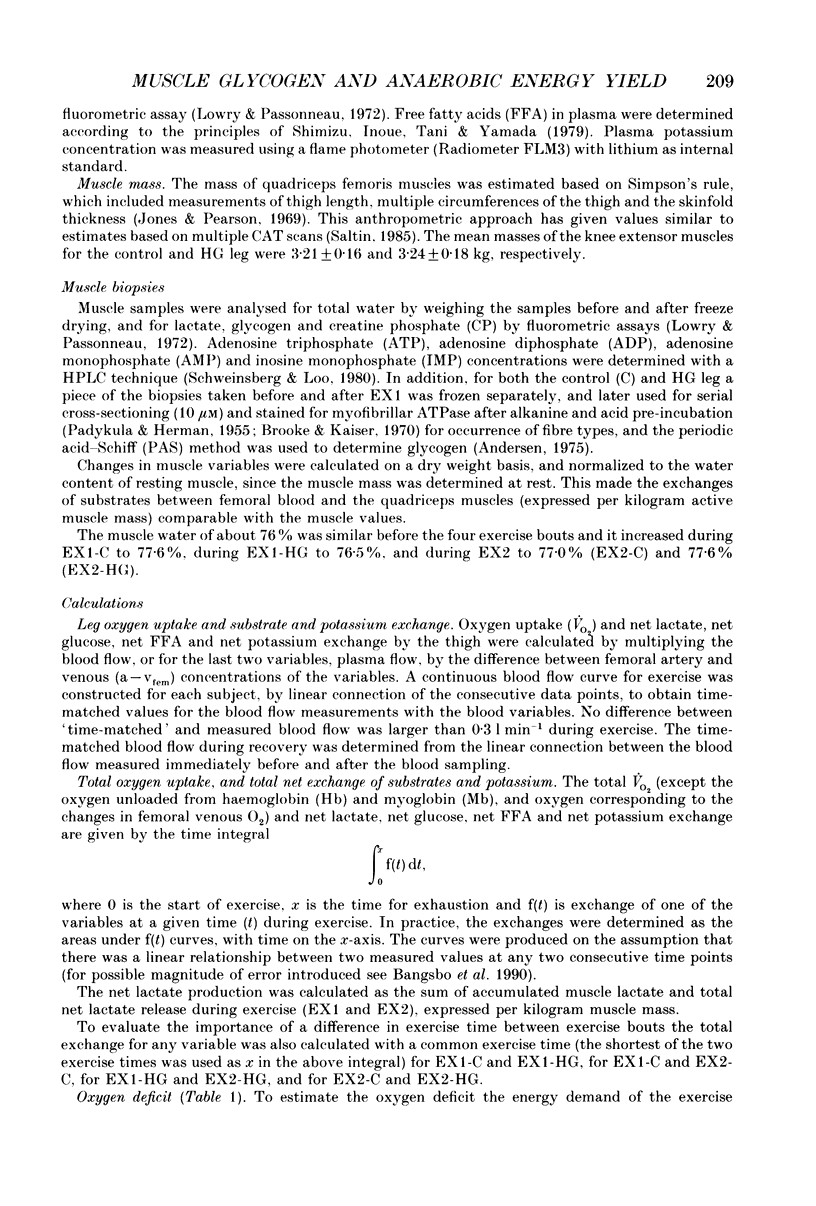
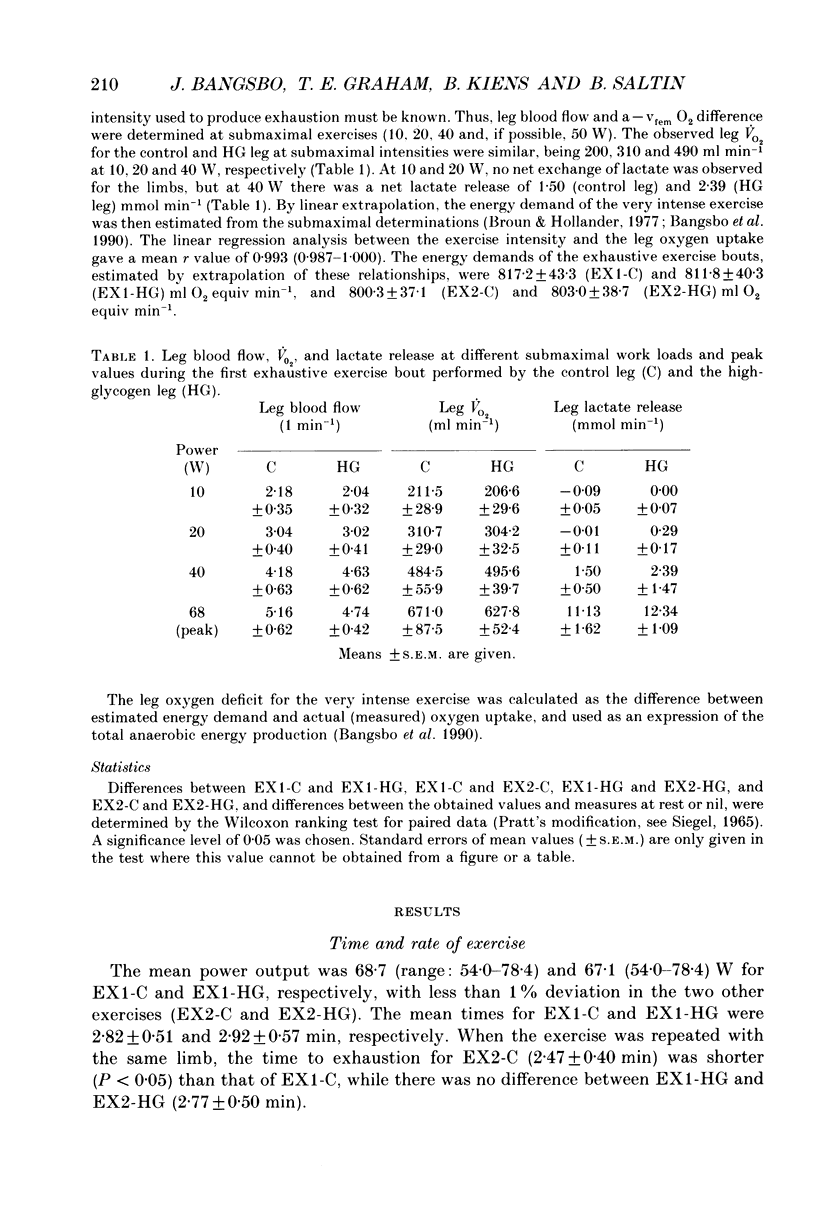
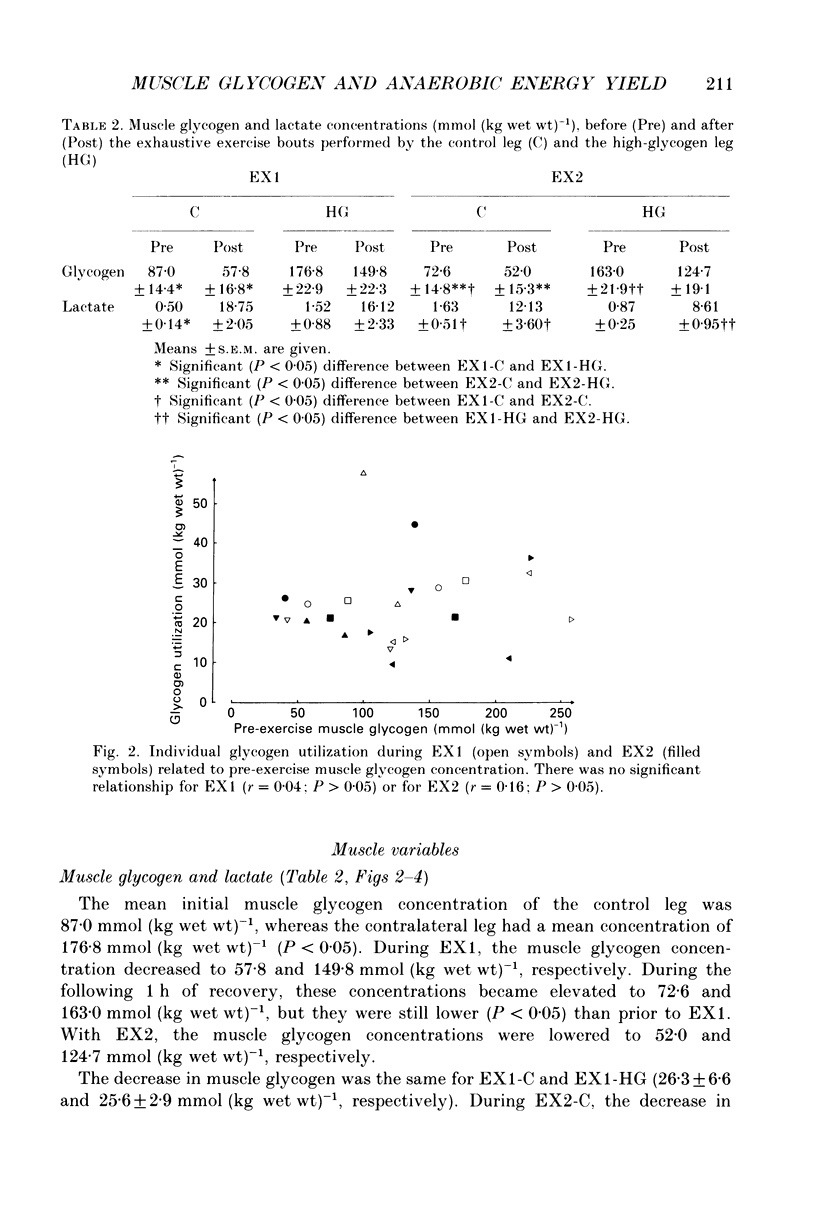
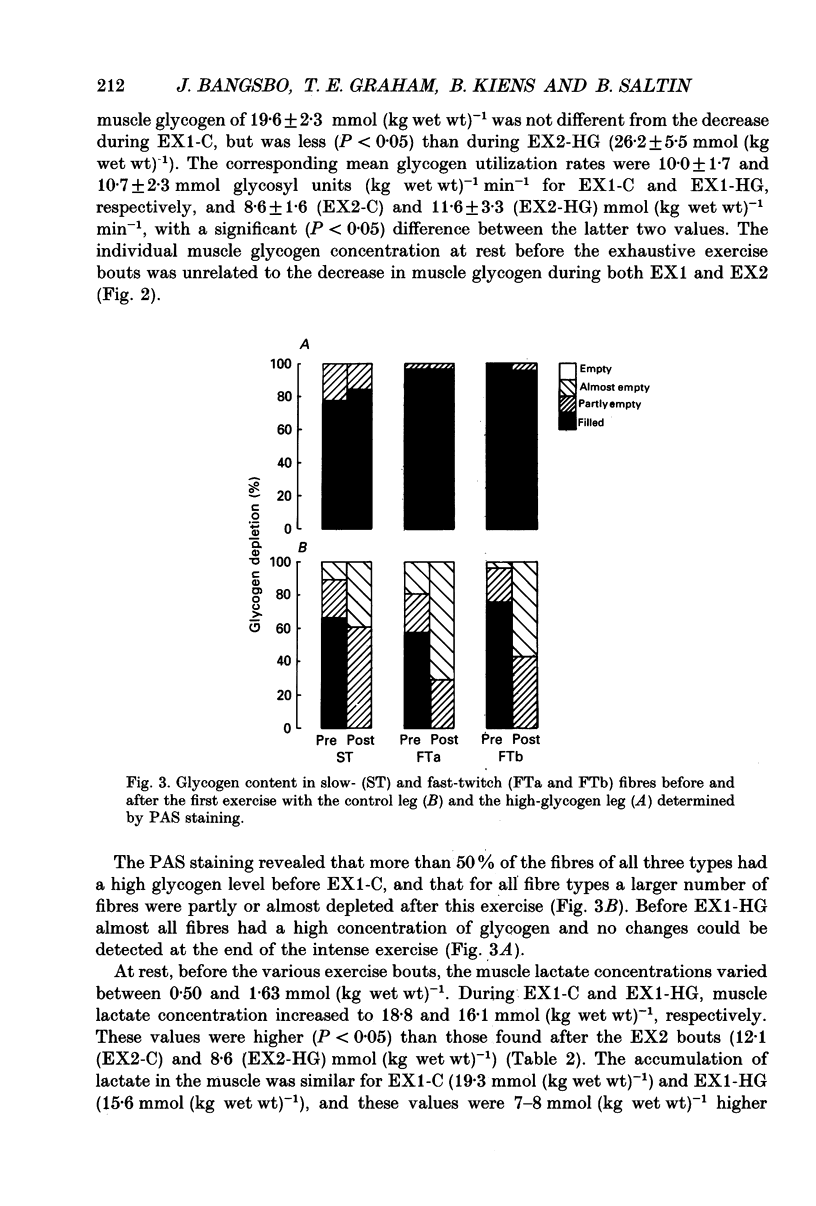
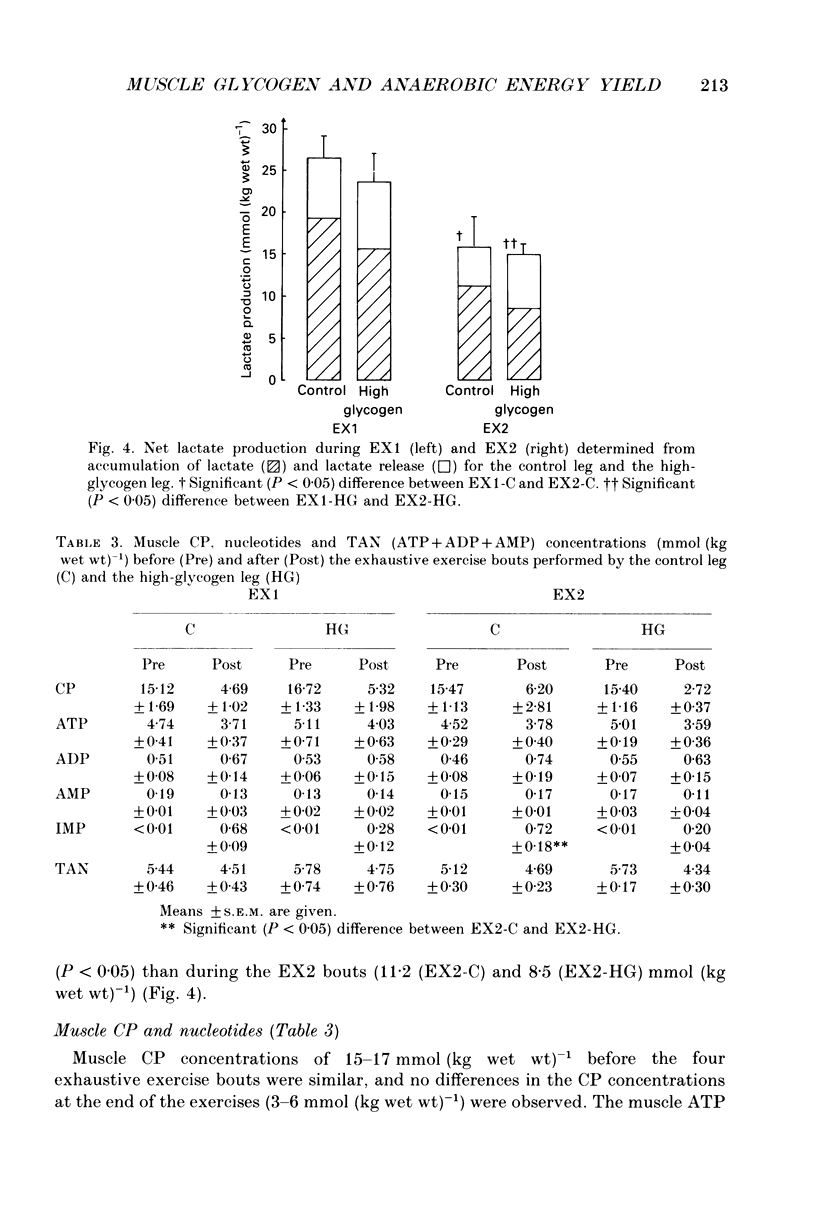
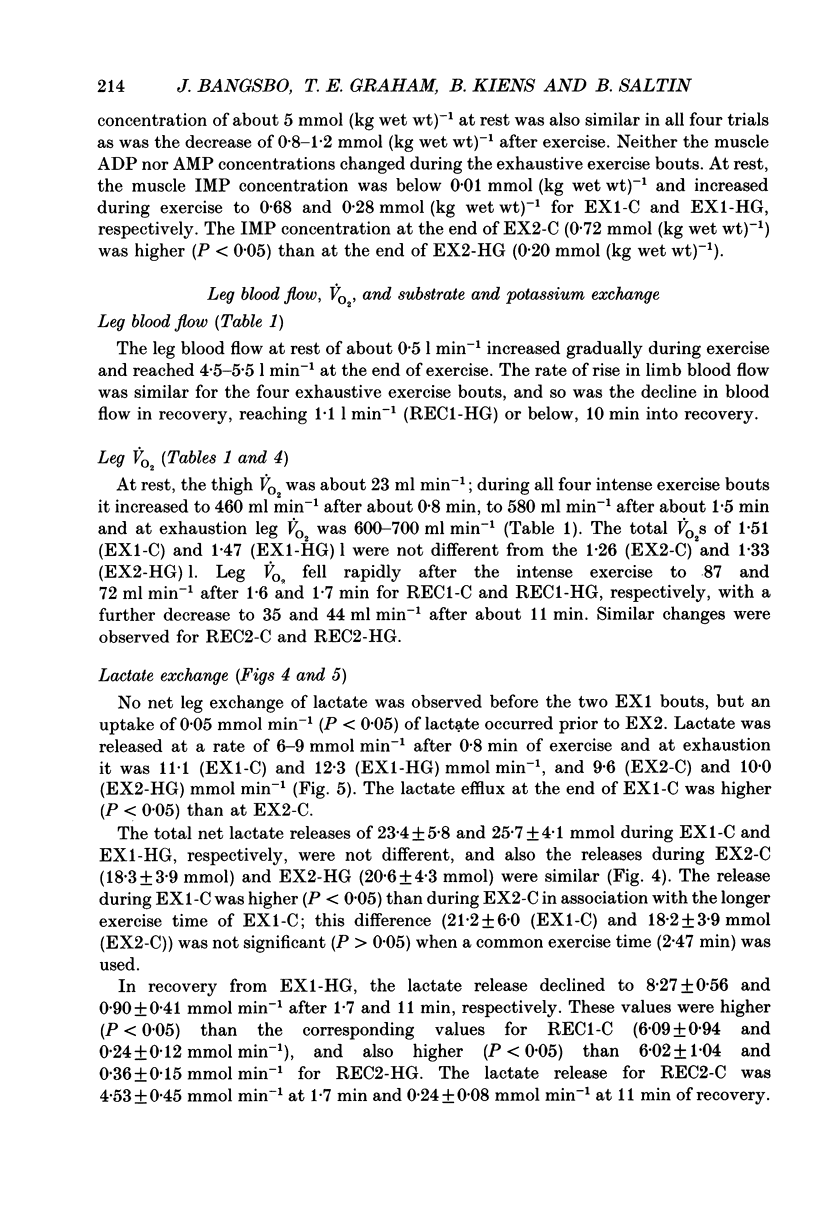
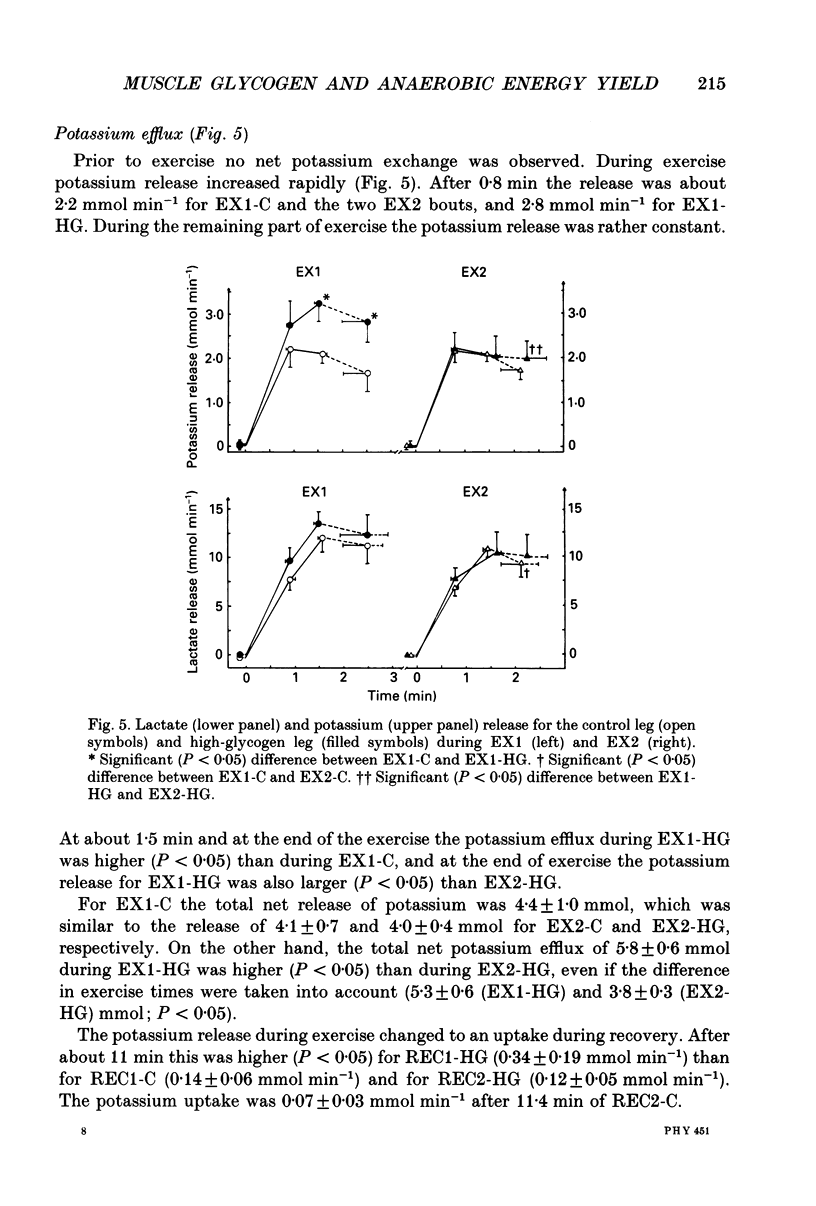
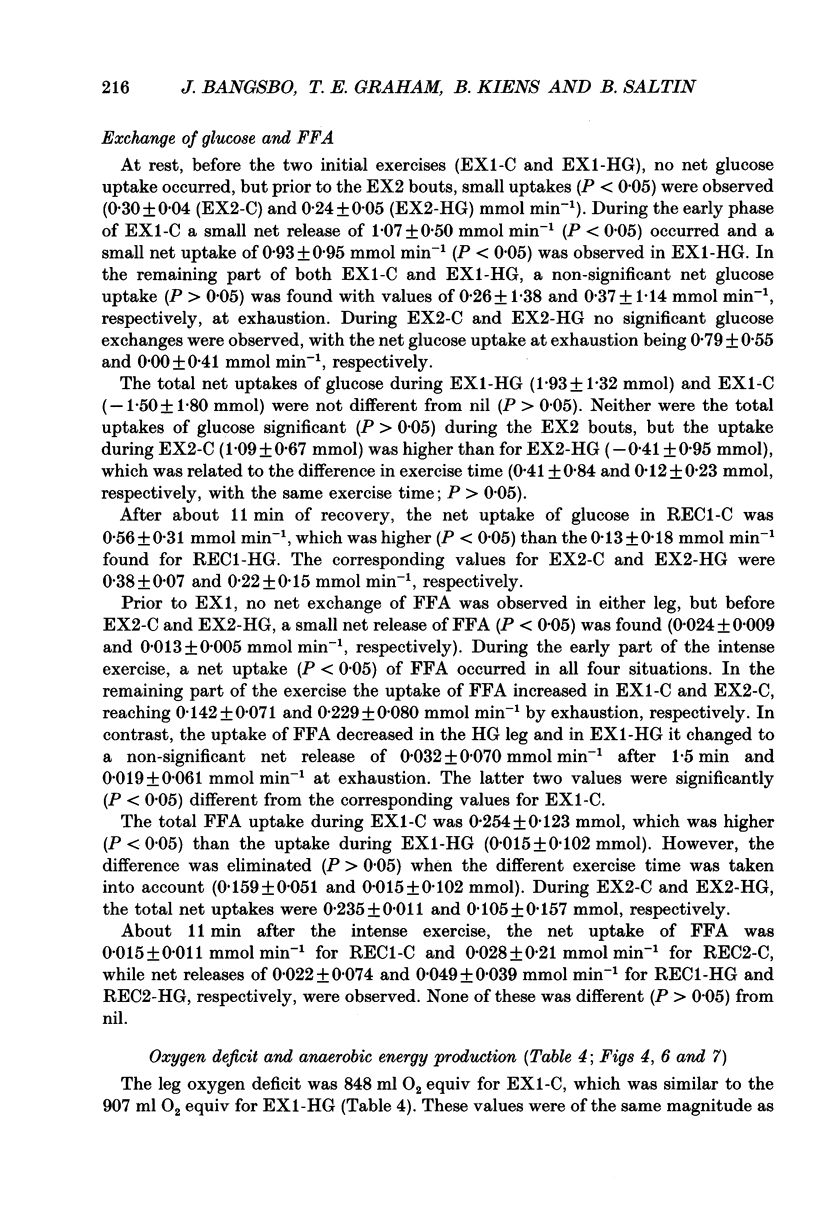
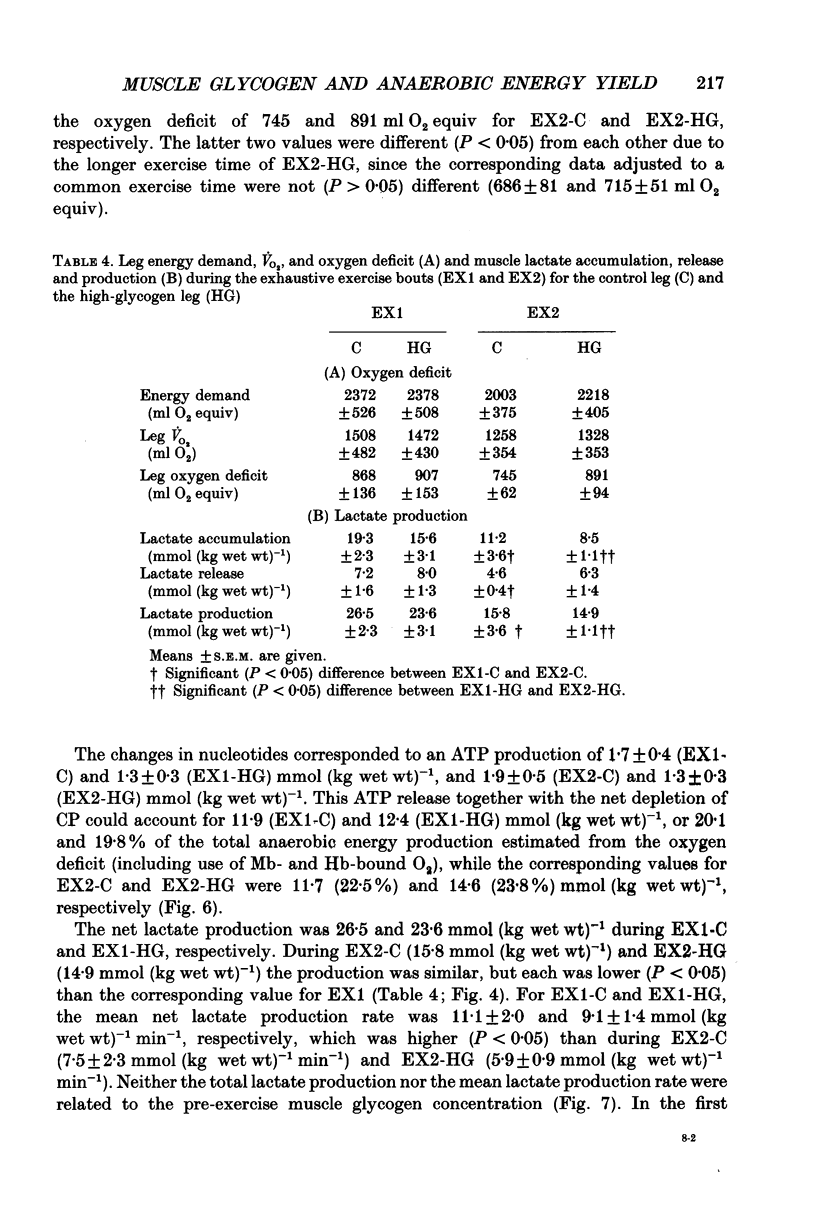
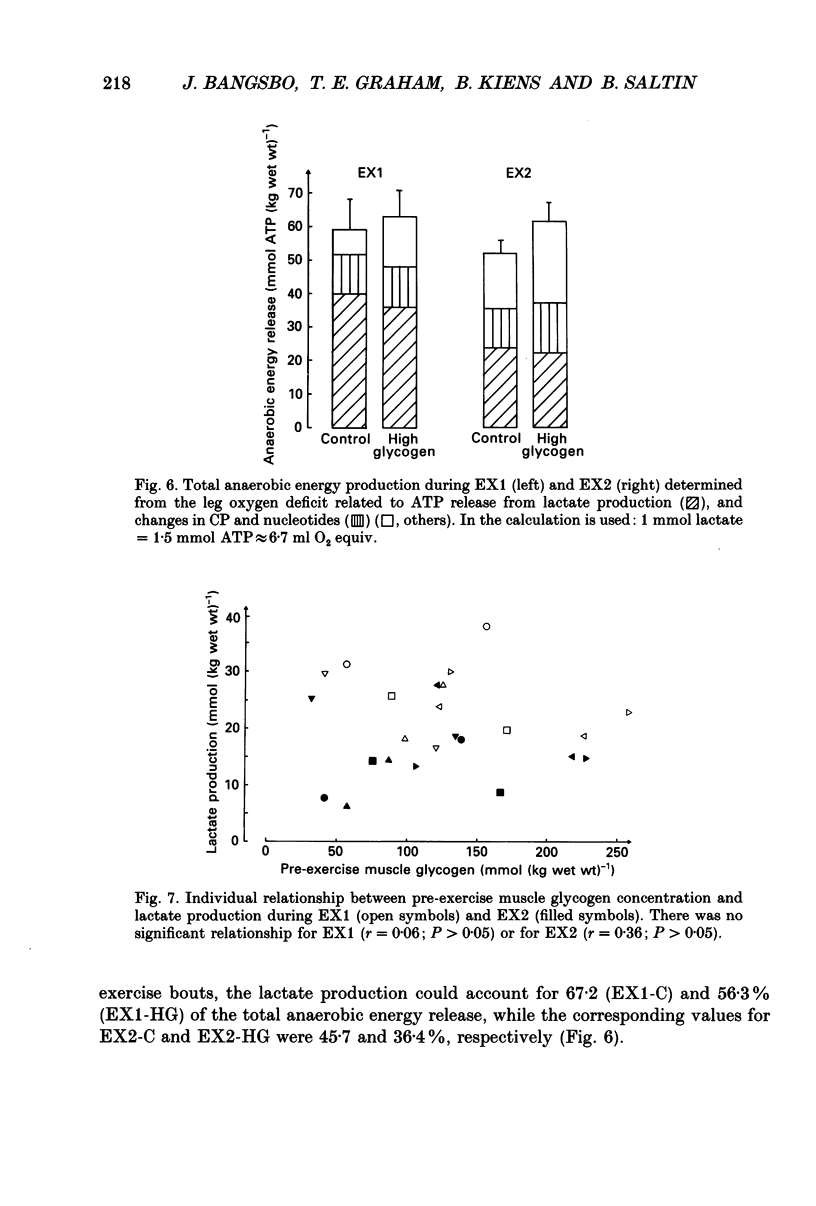
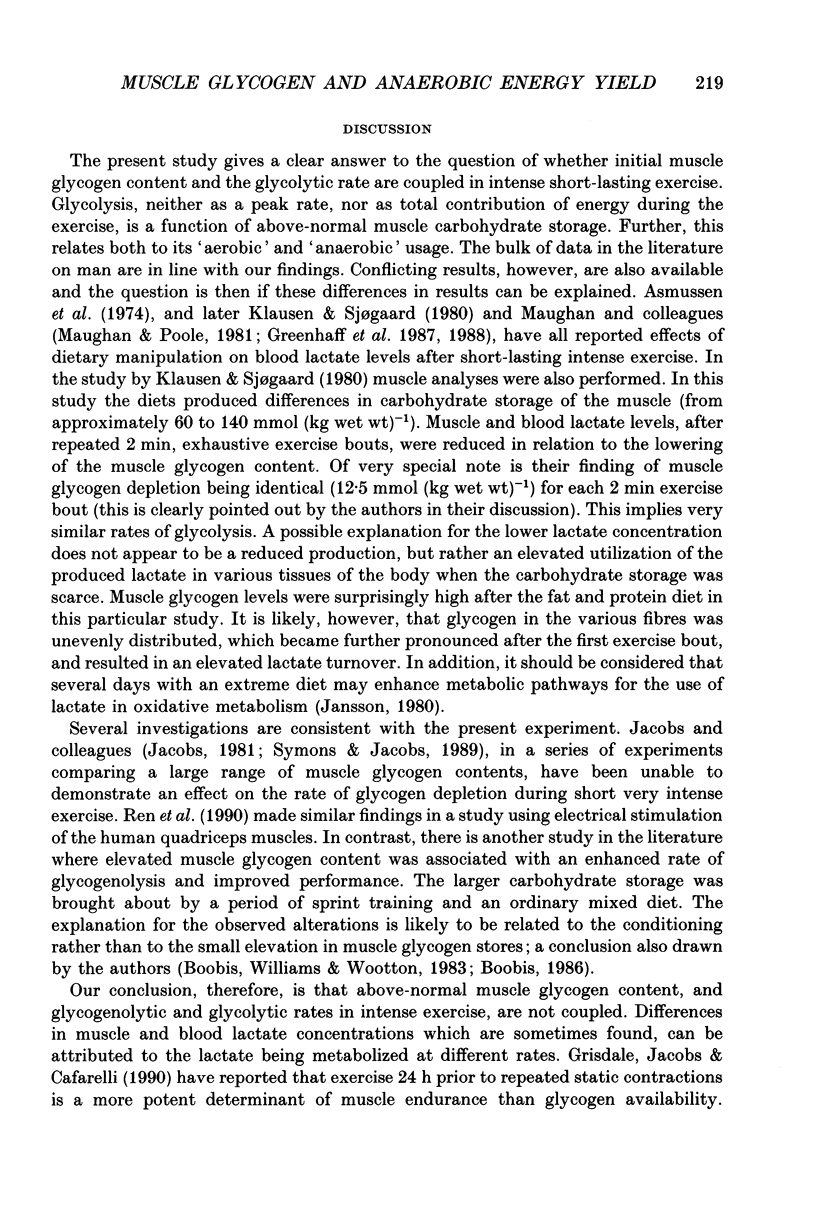
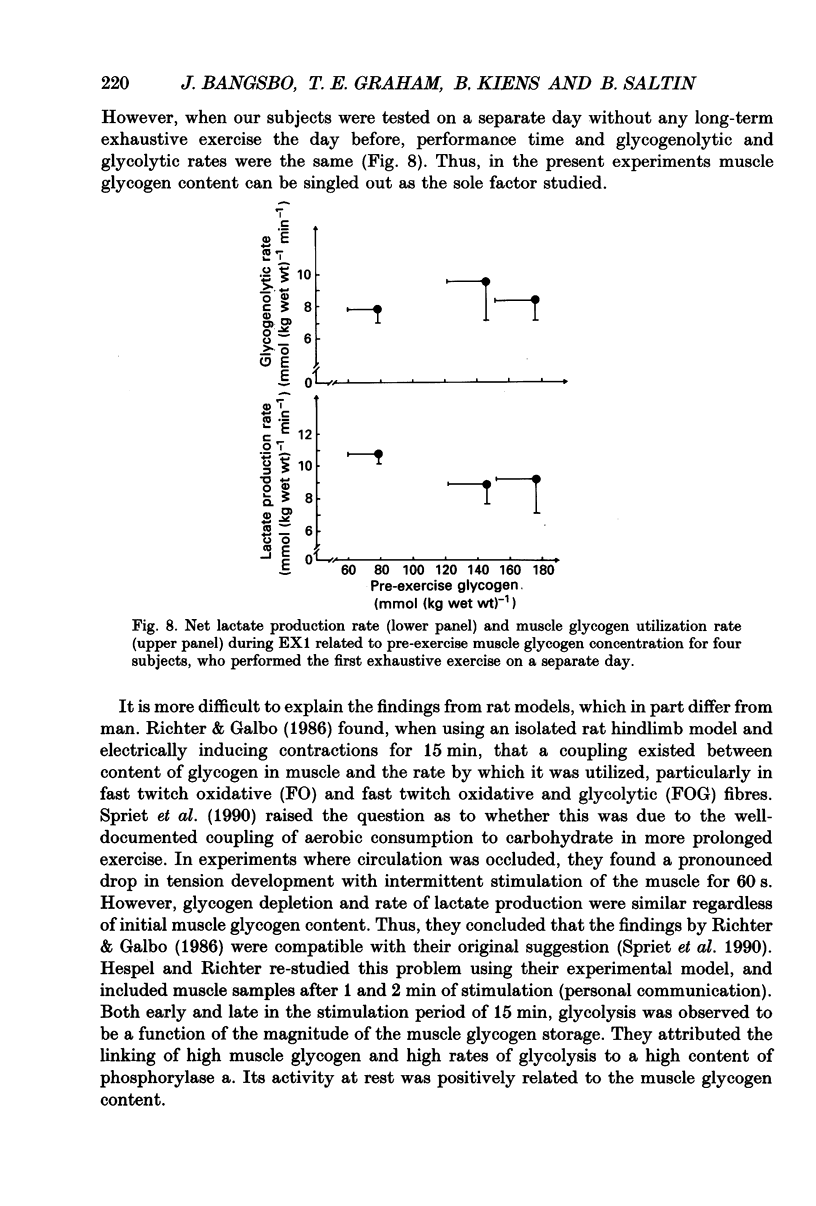
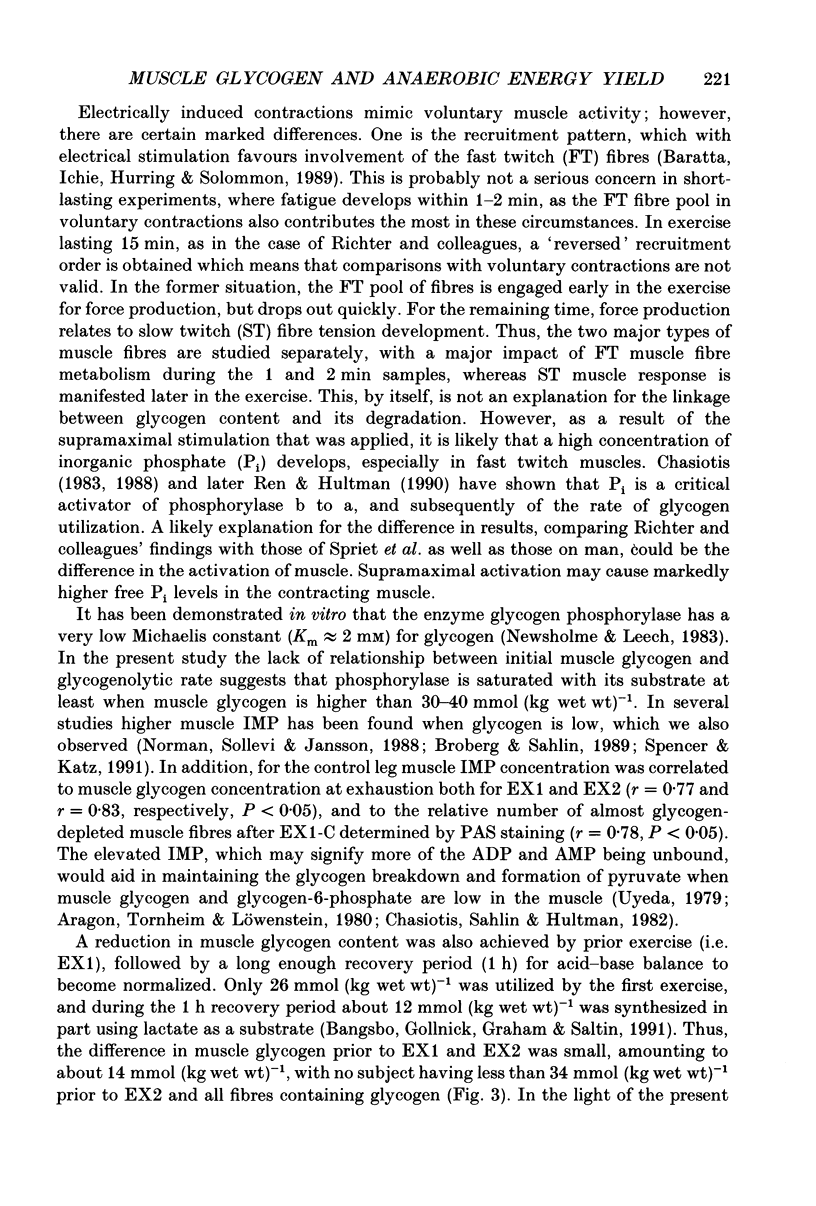
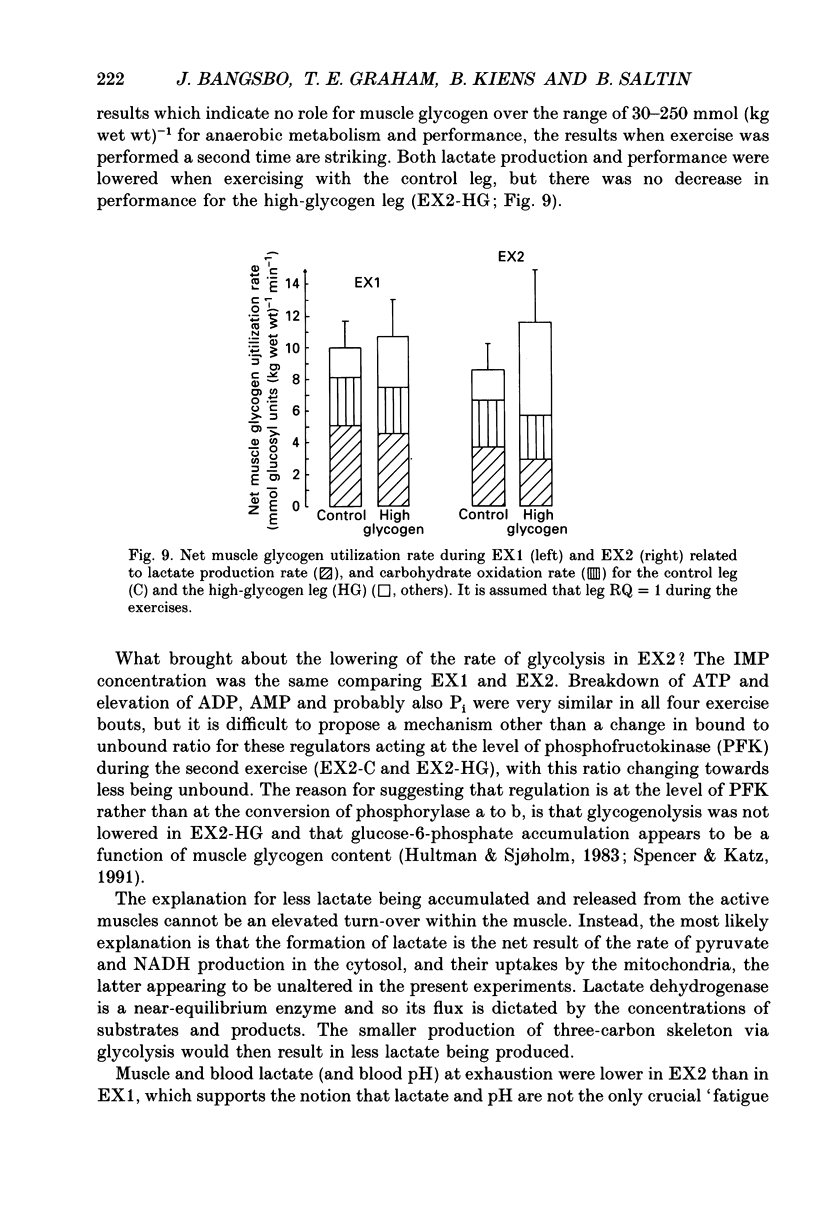
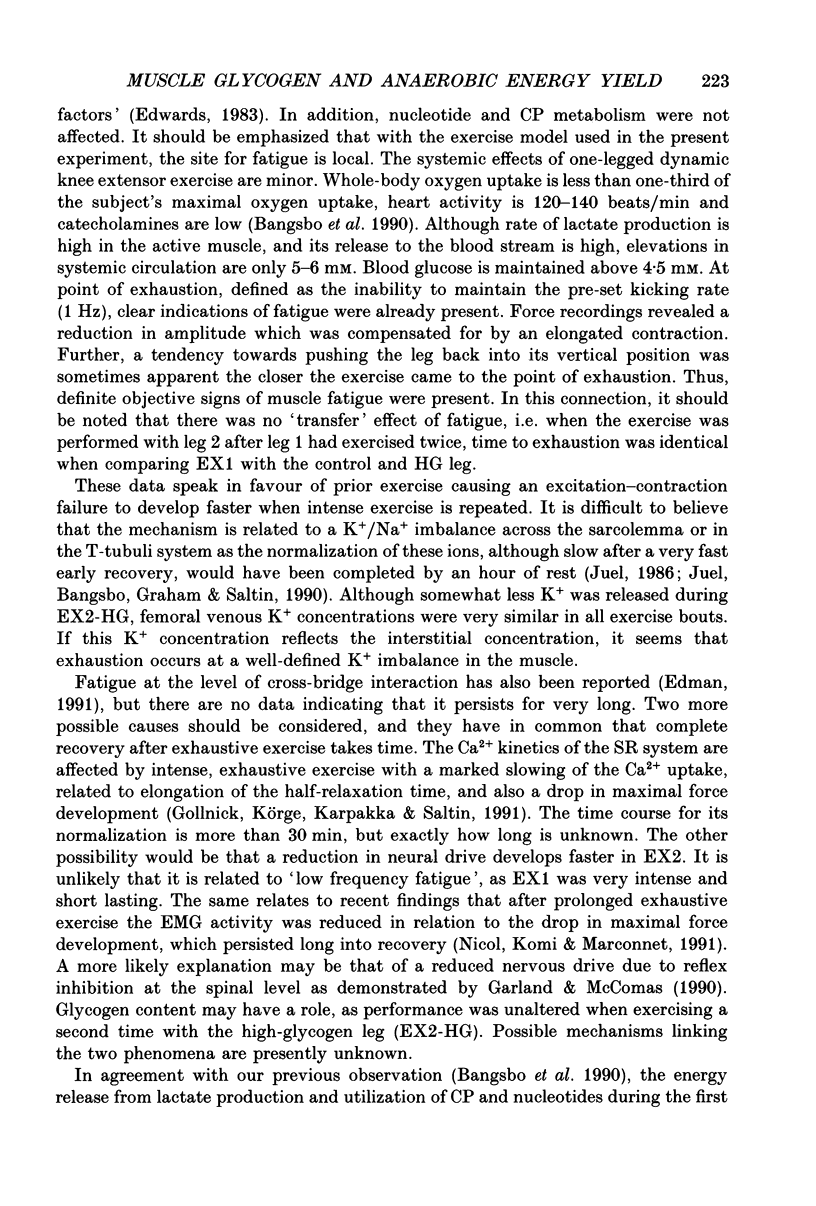
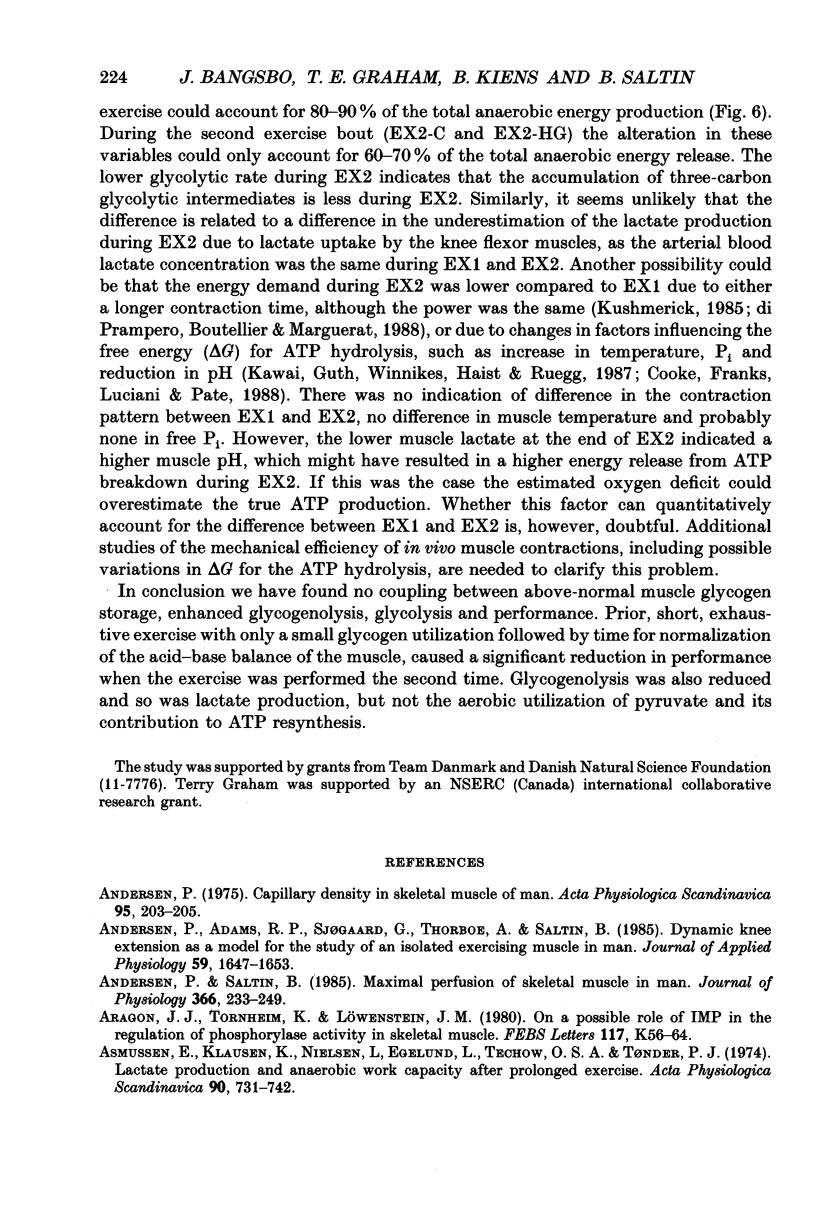
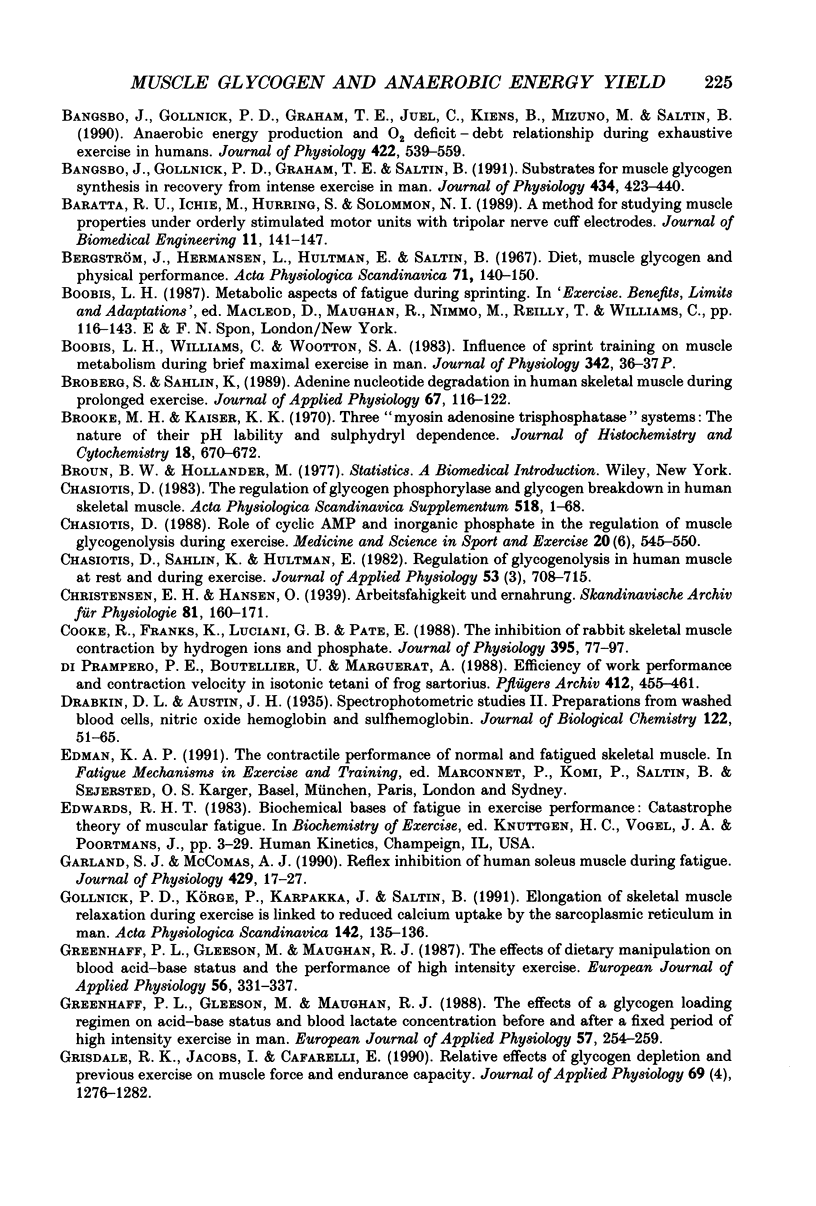
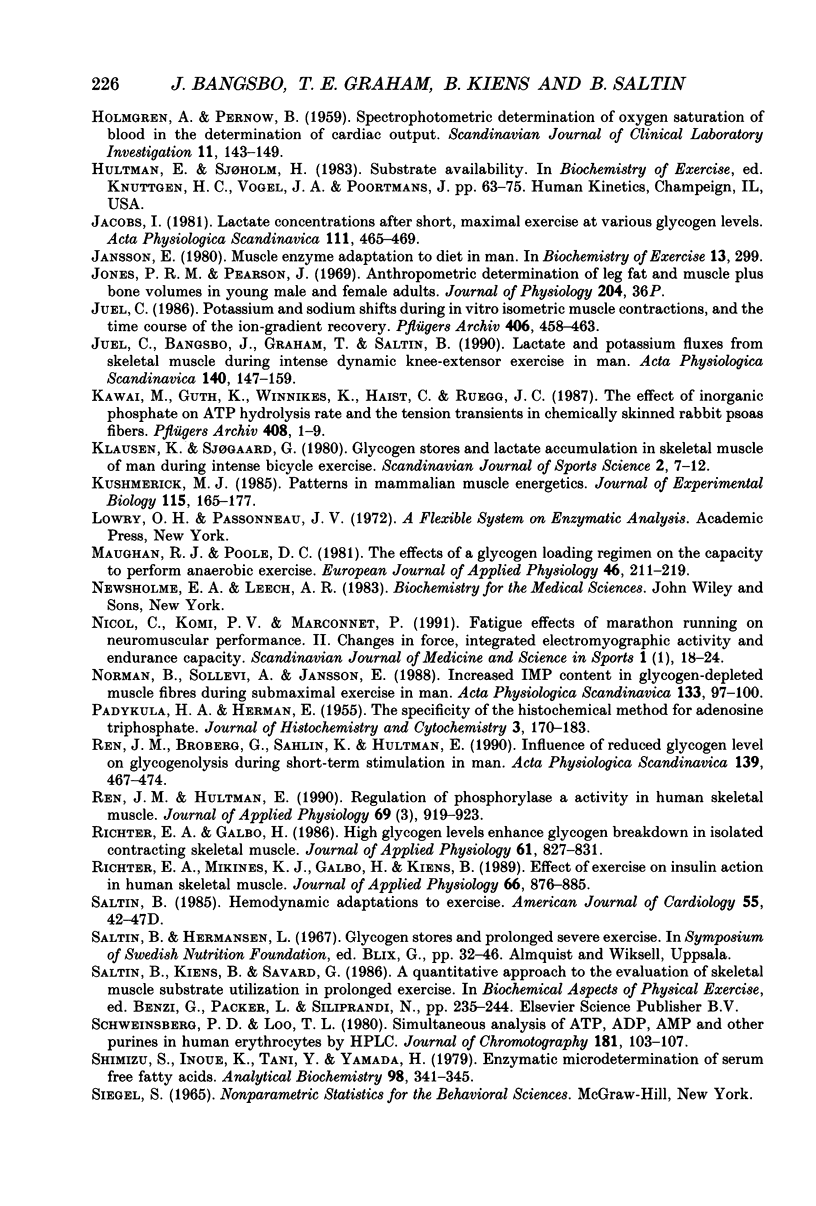
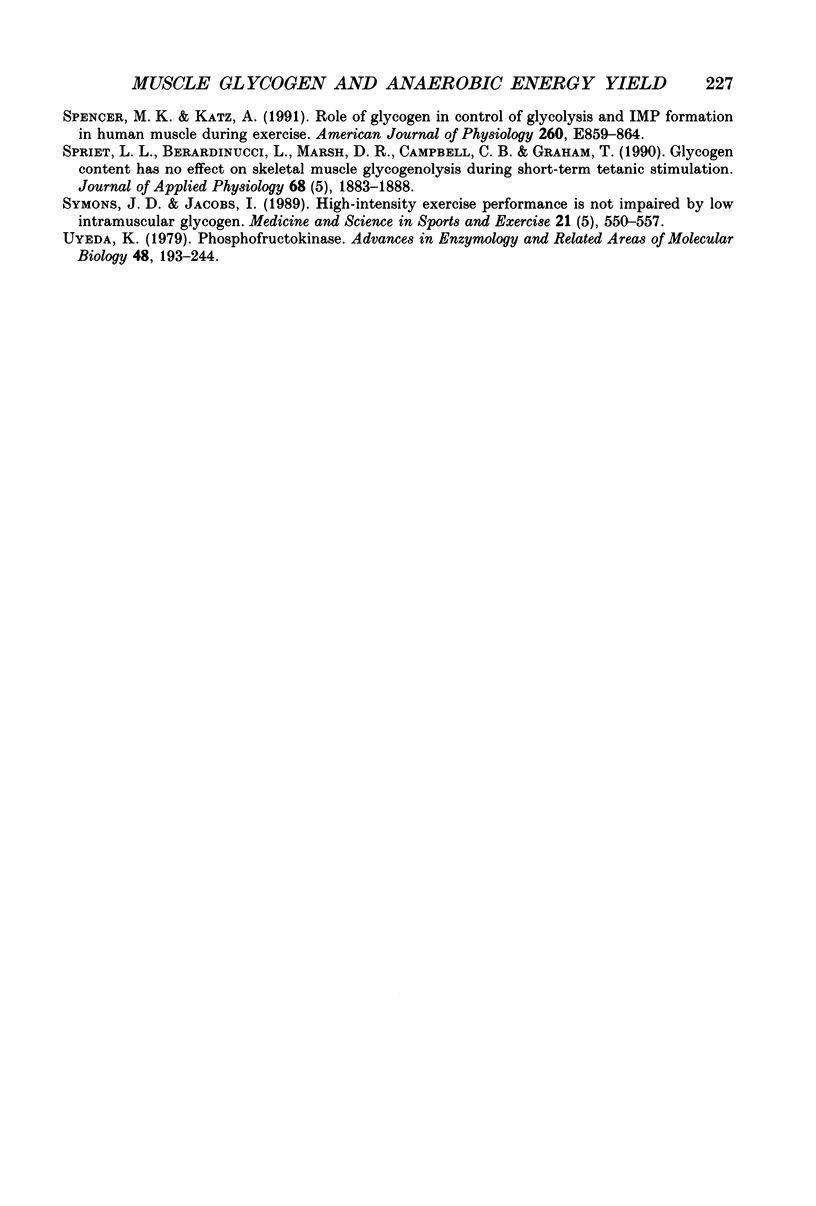
Selected References
These references are in PubMed. This may not be the complete list of references from this article.
- Andersen P., Adams R. P., Sjøgaard G., Thorboe A., Saltin B. Dynamic knee extension as model for study of isolated exercising muscle in humans. J Appl Physiol (1985) 1985 Nov;59(5):1647–1653. doi: 10.1152/jappl.1985.59.5.1647. [DOI] [PubMed] [Google Scholar]
- Andersen P. Capillary density in skeletal muscle of man. Acta Physiol Scand. 1975 Oct;95(2):203–205. doi: 10.1111/j.1748-1716.1975.tb10043.x. [DOI] [PubMed] [Google Scholar]
- Andersen P., Saltin B. Maximal perfusion of skeletal muscle in man. J Physiol. 1985 Sep;366:233–249. doi: 10.1113/jphysiol.1985.sp015794. [DOI] [PMC free article] [PubMed] [Google Scholar]
- Asmussen E., Klausen K., Nielsen L. E., Techow O. S., Tonder P. J. Lactate production and anaerobic work capacity after prolonged exercise. Acta Physiol Scand. 1974 Apr;90(4):731–742. doi: 10.1111/j.1748-1716.1974.tb05641.x. [DOI] [PubMed] [Google Scholar]
- Bangsbo J., Gollnick P. D., Graham T. E., Juel C., Kiens B., Mizuno M., Saltin B. Anaerobic energy production and O2 deficit-debt relationship during exhaustive exercise in humans. J Physiol. 1990 Mar;422:539–559. doi: 10.1113/jphysiol.1990.sp018000. [DOI] [PMC free article] [PubMed] [Google Scholar]
- Bangsbo J., Gollnick P. D., Graham T. E., Saltin B. Substrates for muscle glycogen synthesis in recovery from intense exercise in man. J Physiol. 1991 Mar;434:423–440. doi: 10.1113/jphysiol.1991.sp018478. [DOI] [PMC free article] [PubMed] [Google Scholar]
- Baratta R., Ichie M., Hwang S., Solomonow M. Method for studying muscle properties under orderly stimulated motor units with tripolar nerve cuff electrode. J Biomed Eng. 1989 Mar;11(2):141–147. doi: 10.1016/0141-5425(89)90125-8. [DOI] [PubMed] [Google Scholar]
- Bergström J., Hermansen L., Hultman E., Saltin B. Diet, muscle glycogen and physical performance. Acta Physiol Scand. 1967 Oct-Nov;71(2):140–150. doi: 10.1111/j.1748-1716.1967.tb03720.x. [DOI] [PubMed] [Google Scholar]
- Broberg S., Sahlin K. Adenine nucleotide degradation in human skeletal muscle during prolonged exercise. J Appl Physiol (1985) 1989 Jul;67(1):116–122. doi: 10.1152/jappl.1989.67.1.116. [DOI] [PubMed] [Google Scholar]
- Brooke M. H., Kaiser K. K. Three "myosin adenosine triphosphatase" systems: the nature of their pH lability and sulfhydryl dependence. J Histochem Cytochem. 1970 Sep;18(9):670–672. doi: 10.1177/18.9.670. [DOI] [PubMed] [Google Scholar]
- Chasiotis D. Role of cyclic AMP and inorganic phosphate in the regulation of muscle glycogenolysis during exercise. Med Sci Sports Exerc. 1988 Dec;20(6):545–550. [PubMed] [Google Scholar]
- Chasiotis D., Sahlin K., Hultman E. Regulation of glycogenolysis in human muscle at rest and during exercise. J Appl Physiol Respir Environ Exerc Physiol. 1982 Sep;53(3):708–715. doi: 10.1152/jappl.1982.53.3.708. [DOI] [PubMed] [Google Scholar]
- Chasiotis D. The regulation of glycogen phosphorylase and glycogen breakdown in human skeletal muscle. Acta Physiol Scand Suppl. 1983;518:1–68. [PubMed] [Google Scholar]
- Cooke R., Franks K., Luciani G. B., Pate E. The inhibition of rabbit skeletal muscle contraction by hydrogen ions and phosphate. J Physiol. 1988 Jan;395:77–97. doi: 10.1113/jphysiol.1988.sp016909. [DOI] [PMC free article] [PubMed] [Google Scholar]
- Garland S. J., McComas A. J. Reflex inhibition of human soleus muscle during fatigue. J Physiol. 1990 Oct;429:17–27. doi: 10.1113/jphysiol.1990.sp018241. [DOI] [PMC free article] [PubMed] [Google Scholar]
- Gollnick P. D., Körge P., Karpakka J., Saltin B. Elongation of skeletal muscle relaxation during exercise is linked to reduced calcium uptake by the sarcoplasmic reticulum in man. Acta Physiol Scand. 1991 May;142(1):135–136. doi: 10.1111/j.1748-1716.1991.tb09139.x. [DOI] [PubMed] [Google Scholar]
- Greenhaff P. L., Gleeson M., Maughan R. J. The effects of a glycogen loading regimen on acid-base status and blood lactate concentration before and after a fixed period of high intensity exercise in man. Eur J Appl Physiol Occup Physiol. 1988;57(2):254–259. doi: 10.1007/BF00640672. [DOI] [PubMed] [Google Scholar]
- Greenhaff P. L., Gleeson M., Maughan R. J. The effects of dietary manipulation on blood acid-base status and the performance of high intensity exercise. Eur J Appl Physiol Occup Physiol. 1987;56(3):331–337. doi: 10.1007/BF00690901. [DOI] [PubMed] [Google Scholar]
- Grisdale R. K., Jacobs I., Cafarelli E. Relative effects of glycogen depletion and previous exercise on muscle force and endurance capacity. J Appl Physiol (1985) 1990 Oct;69(4):1276–1282. doi: 10.1152/jappl.1990.69.4.1276. [DOI] [PubMed] [Google Scholar]
- Jacobs I. Lactate concentrations after short, maximal exercise at various glycogen levels. Acta Physiol Scand. 1981 Apr;111(4):465–469. doi: 10.1111/j.1748-1716.1981.tb06764.x. [DOI] [PubMed] [Google Scholar]
- Juel C., Bangsbo J., Graham T., Saltin B. Lactate and potassium fluxes from human skeletal muscle during and after intense, dynamic, knee extensor exercise. Acta Physiol Scand. 1990 Oct;140(2):147–159. doi: 10.1111/j.1748-1716.1990.tb08986.x. [DOI] [PubMed] [Google Scholar]
- Juel C. Potassium and sodium shifts during in vitro isometric muscle contraction, and the time course of the ion-gradient recovery. Pflugers Arch. 1986 May;406(5):458–463. doi: 10.1007/BF00583367. [DOI] [PubMed] [Google Scholar]
- Kawai M., Güth K., Winnikes K., Haist C., Rüegg J. C. The effect of inorganic phosphate on the ATP hydrolysis rate and the tension transients in chemically skinned rabbit psoas fibers. Pflugers Arch. 1987 Jan;408(1):1–9. doi: 10.1007/BF00581833. [DOI] [PubMed] [Google Scholar]
- Kushmerick M. J. Patterns in mammalian muscle energetics. J Exp Biol. 1985 Mar;115:165–177. doi: 10.1242/jeb.115.1.165. [DOI] [PubMed] [Google Scholar]
- Maughan R. J., Poole D. C. The effects of a glycogen-loading regimen on the capacity to perform anaerobic exercise. Eur J Appl Physiol Occup Physiol. 1981;46(3):211–219. doi: 10.1007/BF00423397. [DOI] [PubMed] [Google Scholar]
- Norman B., Sollevi A., Jansson E. Increased IMP content in glycogen-depleted muscle fibres during submaximal exercise in man. Acta Physiol Scand. 1988 May;133(1):97–100. doi: 10.1111/j.1748-1716.1988.tb08385.x. [DOI] [PubMed] [Google Scholar]
- PADYKULA H. A., HERMAN E. The specificity of the histochemical method for adenosine triphosphatase. J Histochem Cytochem. 1955 May;3(3):170–195. doi: 10.1177/3.3.170. [DOI] [PubMed] [Google Scholar]
- Ren J. M., Broberg S., Sahlin K., Hultman E. Influence of reduced glycogen level on glycogenolysis during short-term stimulation in man. Acta Physiol Scand. 1990 Jul;139(3):467–474. doi: 10.1111/j.1748-1716.1990.tb08948.x. [DOI] [PubMed] [Google Scholar]
- Ren J. M., Hultman E. Regulation of phosphorylase a activity in human skeletal muscle. J Appl Physiol (1985) 1990 Sep;69(3):919–923. doi: 10.1152/jappl.1990.69.3.919. [DOI] [PubMed] [Google Scholar]
- Richter E. A., Galbo H. High glycogen levels enhance glycogen breakdown in isolated contracting skeletal muscle. J Appl Physiol (1985) 1986 Sep;61(3):827–831. doi: 10.1152/jappl.1986.61.3.827. [DOI] [PubMed] [Google Scholar]
- Richter E. A., Mikines K. J., Galbo H., Kiens B. Effect of exercise on insulin action in human skeletal muscle. J Appl Physiol (1985) 1989 Feb;66(2):876–885. doi: 10.1152/jappl.1989.66.2.876. [DOI] [PubMed] [Google Scholar]
- Schweinsberg P. D., Loo T. L. Simultaneous analysis of ATP, ADP, AMP, and other purines in human erythrocytes by high-performance liquid chromatography. J Chromatogr. 1980 Jan 11;181(1):103–107. doi: 10.1016/s0378-4347(00)81276-1. [DOI] [PubMed] [Google Scholar]
- Shimizu S., Inoue K., Tani Y., Yamada H. Enzymatic microdetermination of serum free fatty acids. Anal Biochem. 1979 Oct 1;98(2):341–345. doi: 10.1016/0003-2697(79)90151-9. [DOI] [PubMed] [Google Scholar]
- Spencer M. K., Katz A. Role of glycogen in control of glycolysis and IMP formation in human muscle during exercise. Am J Physiol. 1991 Jun;260(6 Pt 1):E859–E864. doi: 10.1152/ajpendo.1991.260.6.E859. [DOI] [PubMed] [Google Scholar]
- Spriet L. L., Berardinucci L., Marsh D. R., Campbell C. B., Graham T. E. Glycogen content has no effect on skeletal muscle glycogenolysis during short-term tetanic stimulation. J Appl Physiol (1985) 1990 May;68(5):1883–1888. doi: 10.1152/jappl.1990.68.5.1883. [DOI] [PubMed] [Google Scholar]
- Symons J. D., Jacobs I. High-intensity exercise performance is not impaired by low intramuscular glycogen. Med Sci Sports Exerc. 1989 Oct;21(5):550–557. [PubMed] [Google Scholar]
- Uyeda K. Phosphofructokinase. Adv Enzymol Relat Areas Mol Biol. 1979;48:193–244. doi: 10.1002/9780470122938.ch4. [DOI] [PubMed] [Google Scholar]
- di Prampero P. E., Boutellier U., Marguerat A. Efficiency of work performance and contraction velocity in isotonic tetani of frog sartorius. Pflugers Arch. 1988 Oct;412(5):455–461. doi: 10.1007/BF00582533. [DOI] [PubMed] [Google Scholar]