Abstract
1. The highest blood concentrations of ketone bodies were found at 5 days of age, after which time the concentration fell to reach the adult value by 30 days of age. 2. Both mitochondrial and cytoplasmic hydroxymethylglutaryl-CoA synthase activities were detected, with highest activities being found in the mitochondria at all stages of development. Activity of the mitochondrial enzyme increases rapidly immediately after birth, showing a maximum at 15 days of age, thereafter falling to adult values. The cytoplasmic enzyme, on the other hand, increased steadily in activity after birth to reach a maximum at 40 days of age, after which time activity fell to adult values. 3. Both mitochondrial and cytoplasmic aceto-acetyl-CoA thiolase activities were detected, with the mitochondrial enzyme having considerably higher activities at all stages of development. The developmental patterns for both enzymes were very similar to those for the corresponding hydroxymethylglutaryl-CoA synthases. 4. The activity of heart acetoacetyl-CoA transferase remains constant from late foetal life until the end of the suckling period, after which time there is a gradual threefold increase in activity to reach the adult values. The activity of brain 3-oxo acid CoA-transferase increases steadily after birth, reaching a maximum at 30 days of age, thereafter decreasing to adult values, which are similar to foetal activities. Although at all stages of development the specific activity of the heart enzyme is higher than that of brain, the total enzymic capacity of the brain is higher than that of the heart during the suckling period.
Full text
PDF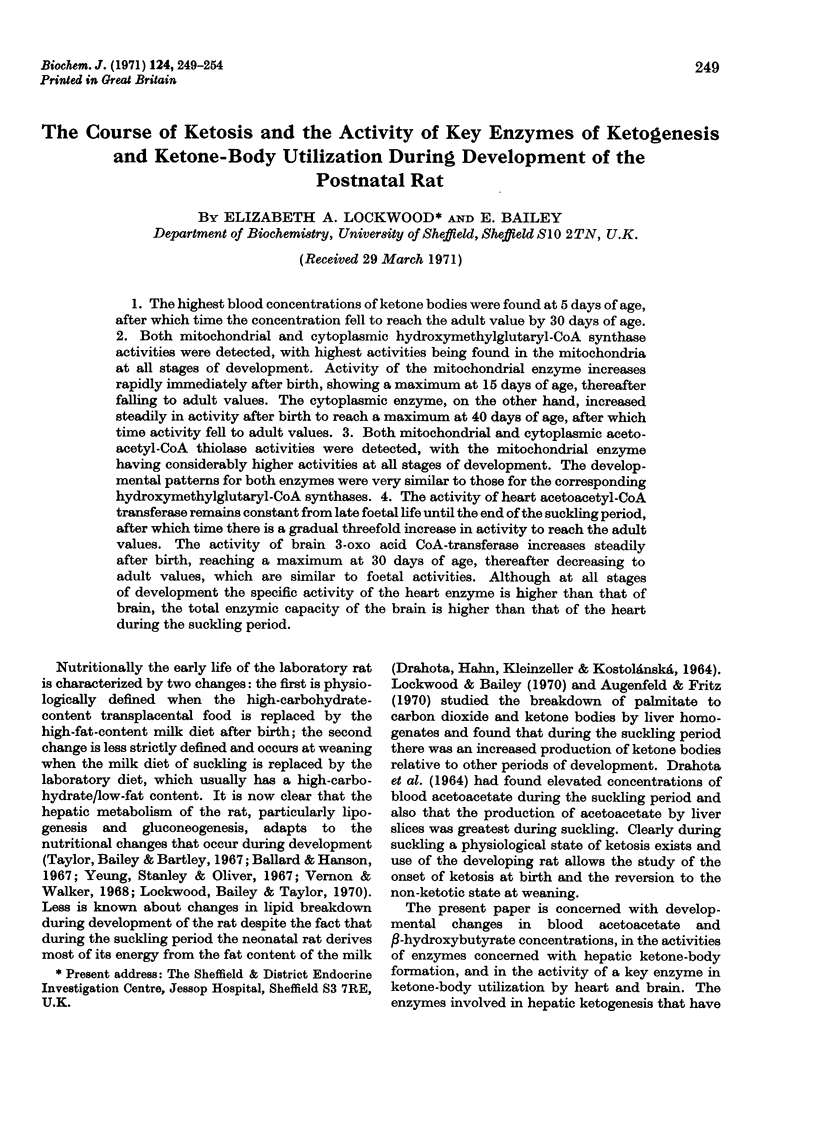
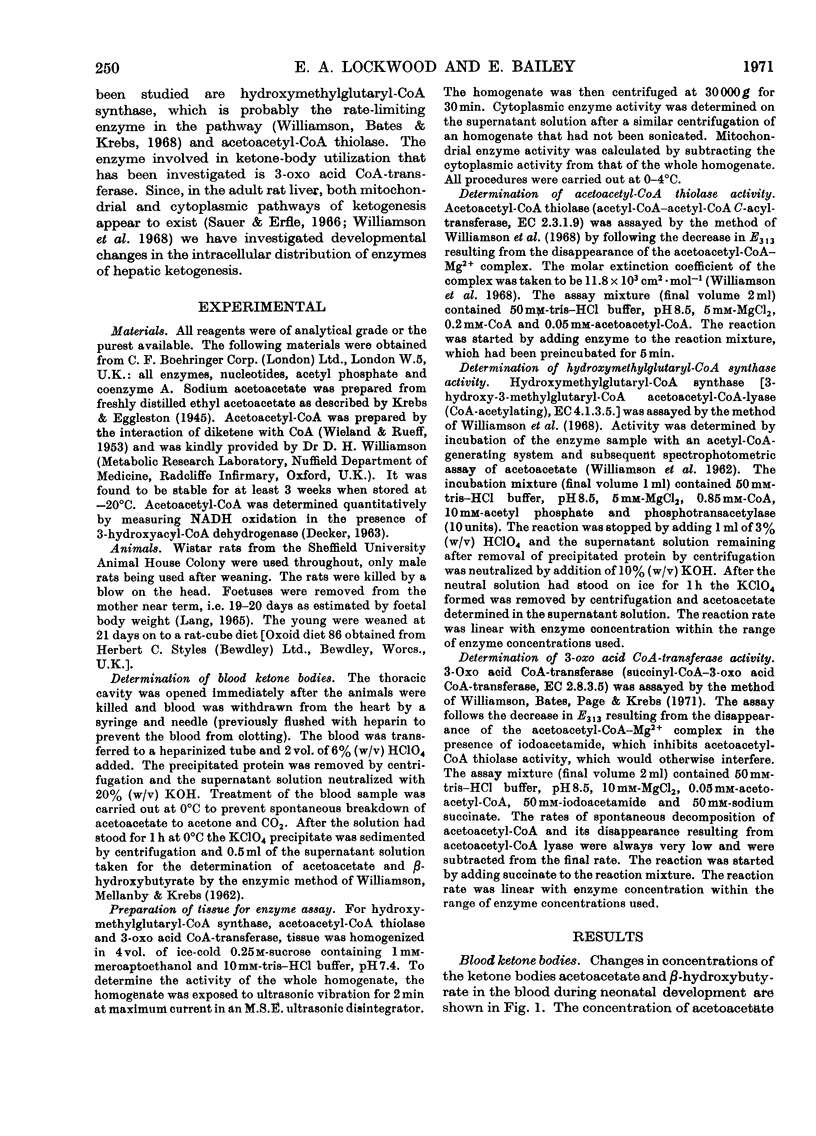
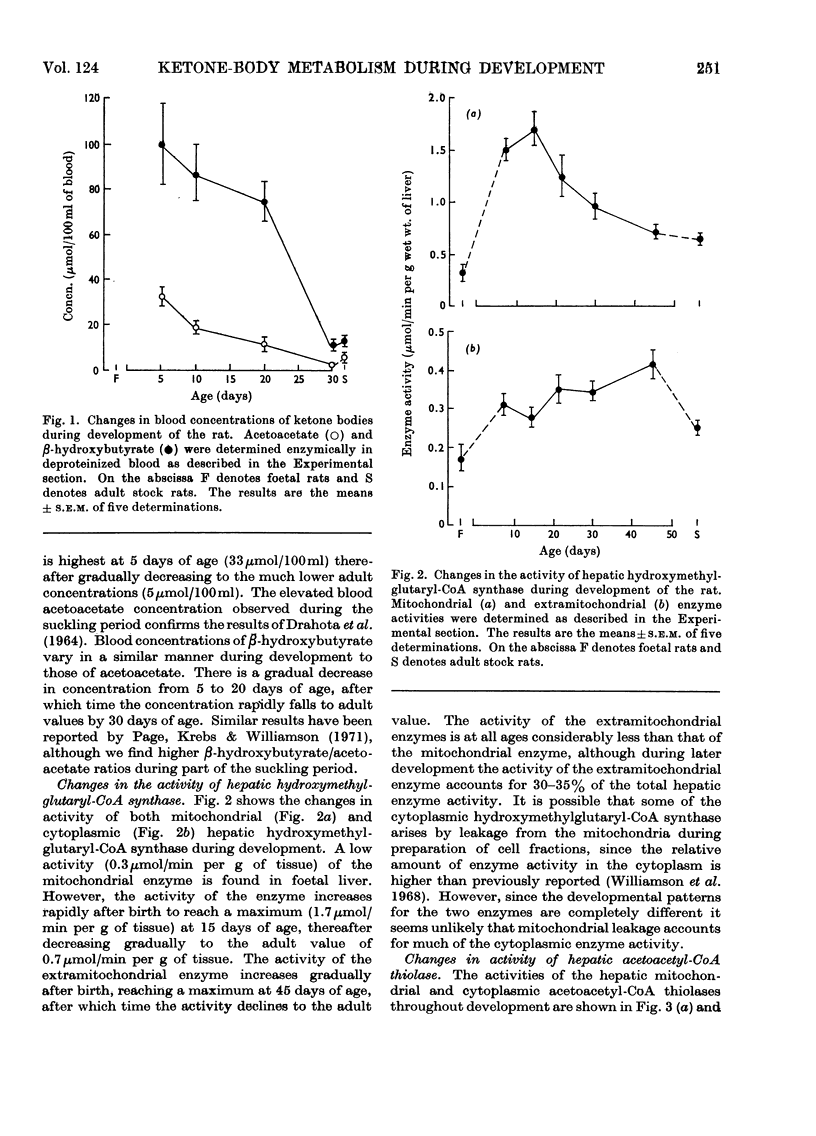
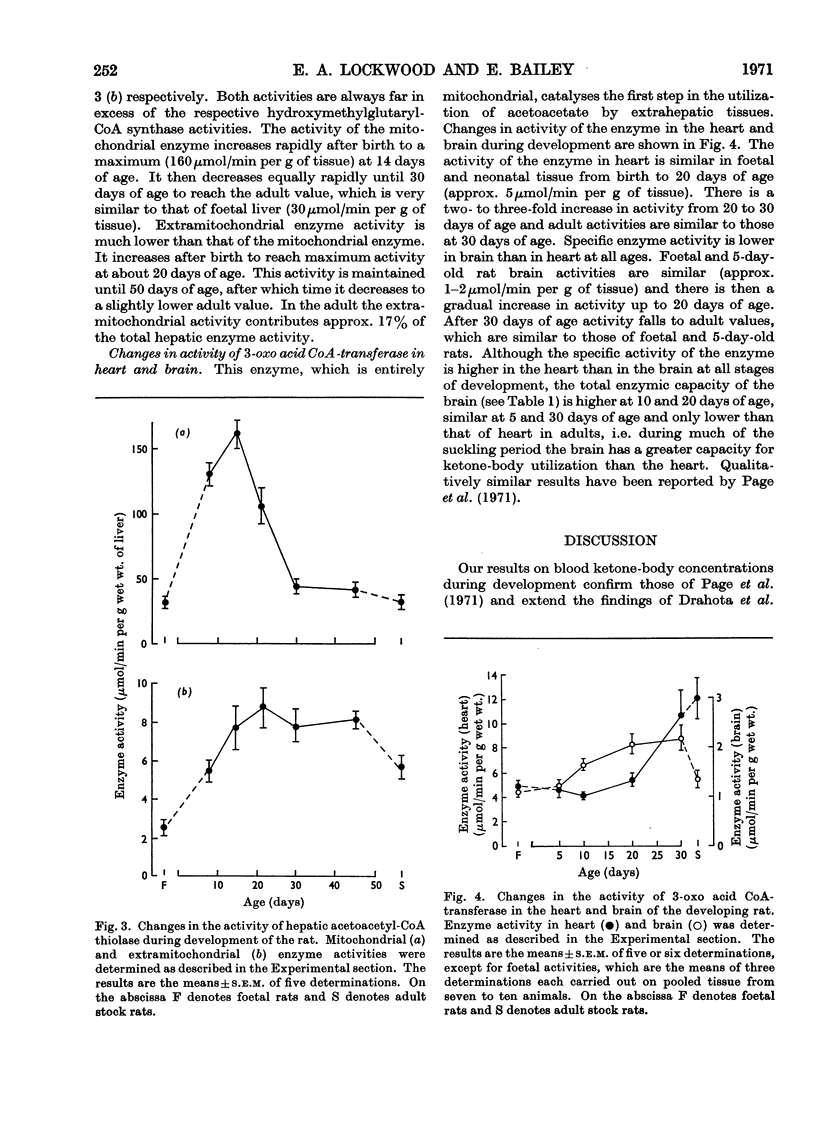
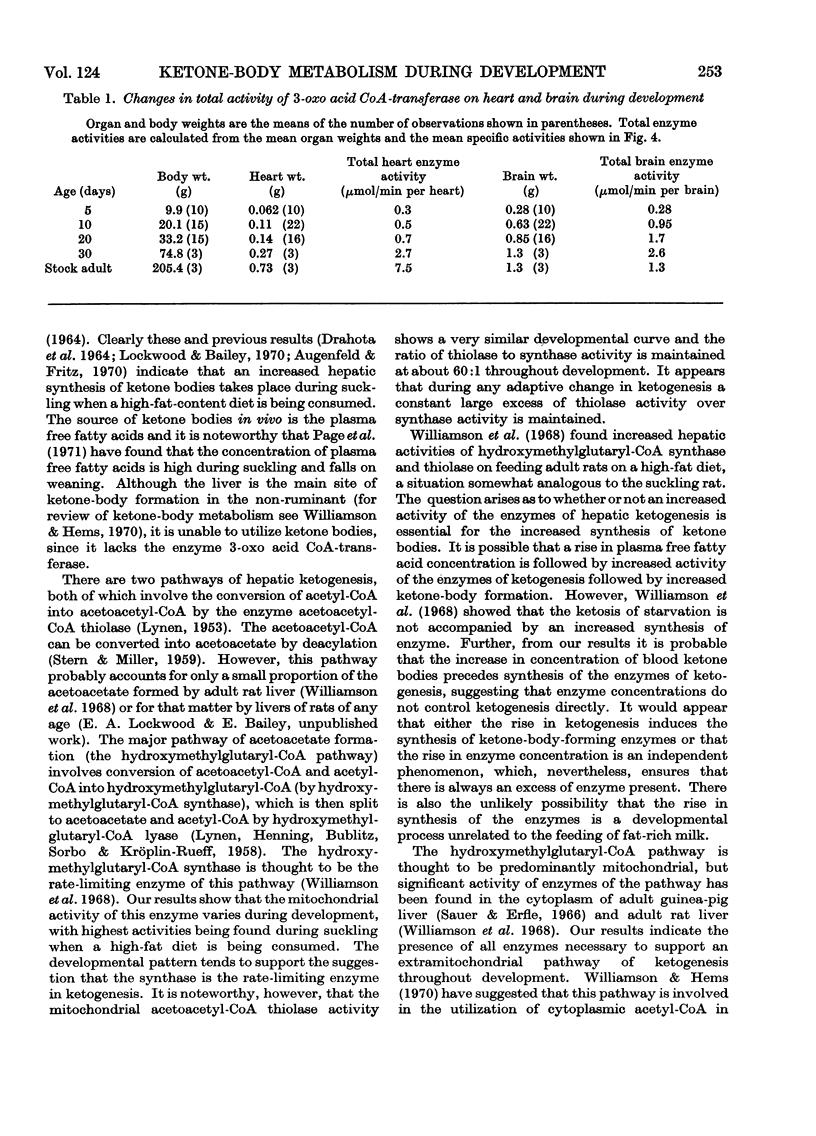
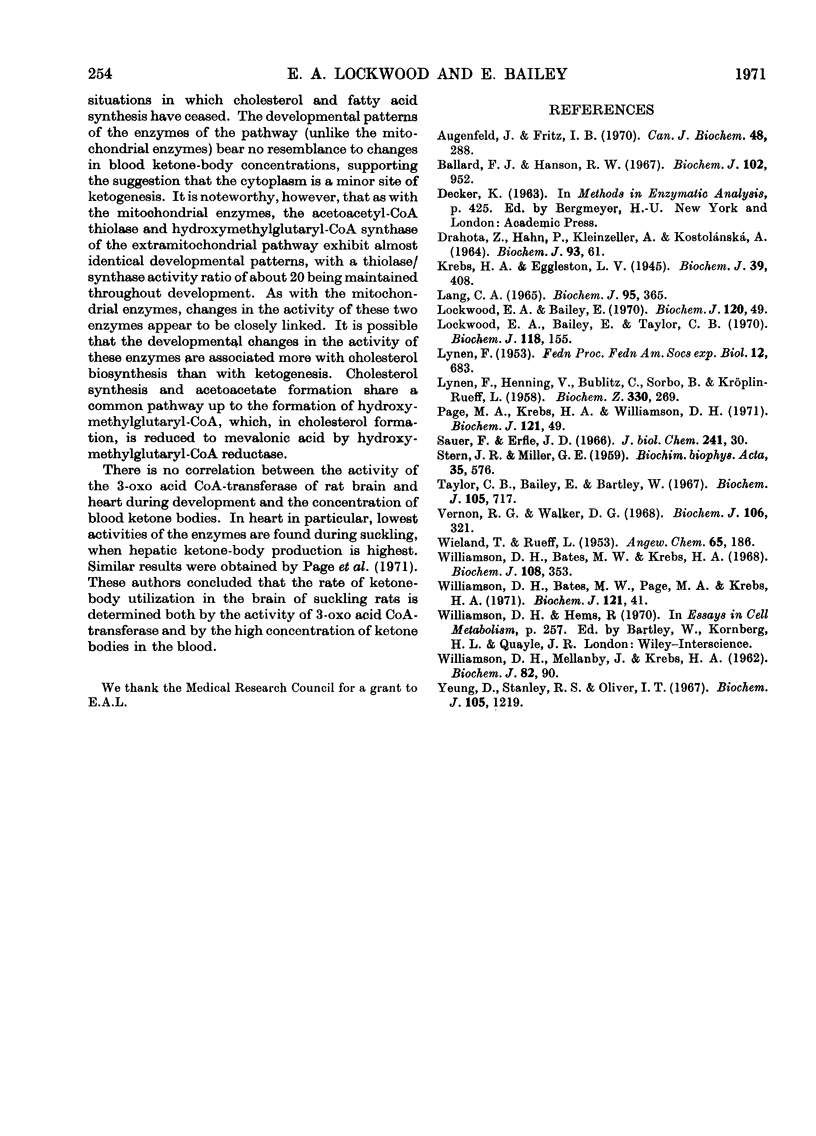
Selected References
These references are in PubMed. This may not be the complete list of references from this article.
- Augenfeld J., Fritz I. B. Carnitine palmitolyltransferase activity and fatty acid oxidation by livers from fetal and neonatal rats. Can J Biochem. 1970 Mar;48(3):288–294. doi: 10.1139/o70-050. [DOI] [PubMed] [Google Scholar]
- Ballard F. J., Hanson R. W. Changes in lipid synthesis in rat liver during development. Biochem J. 1967 Mar;102(3):952–958. doi: 10.1042/bj1020952. [DOI] [PMC free article] [PubMed] [Google Scholar]
- Drahota Z., Hahn P., Kleinzeller A., Kostolánská A. Acetoacetate formation by liver slices from adult and infant rats. Biochem J. 1964 Oct;93(1):61–65. doi: 10.1042/bj0930061. [DOI] [PMC free article] [PubMed] [Google Scholar]
- Krebs H. A., Eggleston L. V. Metabolism of acetoacetate in animal tissues. 1. Biochem J. 1945;39(5):408–419. [PMC free article] [PubMed] [Google Scholar]
- LANG C. A. RESPIRATORY ENZYMES IN THE HEART AND LIVER OF THE PRENATAL AND POSTNATAL RAT. Biochem J. 1965 May;95:365–371. doi: 10.1042/bj0950365. [DOI] [PMC free article] [PubMed] [Google Scholar]
- LYNEN F., HENNING U., BUBLITZ C., SORBO B., KROPLIN-RUEFF L. Der chemische Mechanismus der Acetessigsäurebildung in der Leber. Biochem Z. 1958;330(4):269–295. [PubMed] [Google Scholar]
- Lockwood E. A., Bailey E. Fatty acid utilization during development of the rat. Biochem J. 1970 Nov;120(1):49–54. doi: 10.1042/bj1200049. [DOI] [PMC free article] [PubMed] [Google Scholar]
- Lockwood E. A., Bailey E., Taylor C. B. Factors involved in changes in hepatic lipogenesis during development of the rat. Biochem J. 1970 Jun;118(1):155–162. doi: 10.1042/bj1180155. [DOI] [PMC free article] [PubMed] [Google Scholar]
- Page M. A., Krebs H. A., Williamson D. H. Activities of enzymes of ketone-body utilization in brain and other tissues of suckling rats. Biochem J. 1971 Jan;121(1):49–53. doi: 10.1042/bj1210049. [DOI] [PMC free article] [PubMed] [Google Scholar]
- STERN J. R., MILLER G. E. On the enzymic mechanism of acetoacetate synthesis. Biochim Biophys Acta. 1959 Oct;35:576–577. doi: 10.1016/0006-3002(59)90424-x. [DOI] [PubMed] [Google Scholar]
- Sauer F., Erfle J. D. On the mechanism of acetoacetate synthesis by guinea pig liver fractions. J Biol Chem. 1966 Jan 10;241(1):30–37. [PubMed] [Google Scholar]
- Taylor C. B., Bailey E., Bartley W. Changes in hepatic lipigenesis during development of the rat. Biochem J. 1967 Nov;105(2):717–722. doi: 10.1042/bj1050717. [DOI] [PMC free article] [PubMed] [Google Scholar]
- Vernon R. G., Walker D. G. Changes in activity of some enzymes involved in glucose utilization and formation in developing rat liver. Biochem J. 1968 Jan;106(2):321–329. doi: 10.1042/bj1060321. [DOI] [PMC free article] [PubMed] [Google Scholar]
- WILLIAMSON D. H., MELLANBY J., KREBS H. A. Enzymic determination of D(-)-beta-hydroxybutyric acid and acetoacetic acid in blood. Biochem J. 1962 Jan;82:90–96. doi: 10.1042/bj0820090. [DOI] [PMC free article] [PubMed] [Google Scholar]
- Williamson D. H., Bates M. W., Krebs H. A. Activity and intracellular distribution of enzymes of ketone-body metabolism in rat liver. Biochem J. 1968 Jul;108(3):353–361. doi: 10.1042/bj1080353. [DOI] [PMC free article] [PubMed] [Google Scholar]
- Yeung D., Stanley R. S., Oliver I. T. Development of gluconeogenesis in neonatal rat liver. Effect of triamcinolone. Biochem J. 1967 Dec;105(3):1219–1227. doi: 10.1042/bj1051219. [DOI] [PMC free article] [PubMed] [Google Scholar]