Abstract
1. The whole-cell configuration of the patch clamp technique was used to study inward and delayed outward currents in beta-cells isolated from human pancreatic islets. 2. The delayed outward current activated at about -20 mV and increased linearly with further depolarization. The instantaneous current-voltage (I-V) relation, measured by tail current analysis, reversed at -70 mV. This is close to the K+ equilibrium potential and suggests the outward current is carried primarily by potassium ions. In support of this idea, outward currents were abolished when internal K+ was replaced by the impermeant cation N-methyl-D-glucamine (NMG). 3. The voltage dependence of K+ current activation could be fitted by a sigmoidal function with a mid-point at +1 mV. K+ currents showed voltage-dependent inactivation which was half-maximal at -25 mV. 4. Inward currents were studied after outward currents were suppressed by replacing internal potassium with NMG. In 5 mM [Ca2+]o, the inward current activated between -50 and -40 mV, had a peak amplitude at -10 mV and reversed at potentials positive to +60 mV. The voltage dependence of inward current activation was sigmoidal with half-maximal activation at -10 mV in 5 mM [Ca2+]o and at -22 mV in 5 mM [Ba2+]o. 5. Inward currents were unaffected by tetrodotoxin (TTX), but could be blocked by cadmium ions. Barium was also capable of carrying inward current. This pharmacology is consistent with inward currents flowing through Ca2+ channels. 6. The inactivation of the inward current was dependent on calcium entry. In two-pulse experiments, the voltage dependence of inactivation was U-shaped, and resembled that of the calcium current. Barium currents showed little inactivation. 7. In two-pulse experiments the degree of inward current inactivation during the test pulse was related to the amount of calcium entry during the first pulse. Calcium entering at more positive potentials was less effective at producing inactivation. 8. Calcium and barium currents also showed a slow, voltage-dependent inactivation when the holding potential was changed between -100 and -40 mV. This inactivation developed with a time course of seconds. 9. The Ca2+ and K+ currents described here are similar to those reported for rodent beta-cells and indicate the rodent beta-cell provides a good model for that of man.
Full text
PDF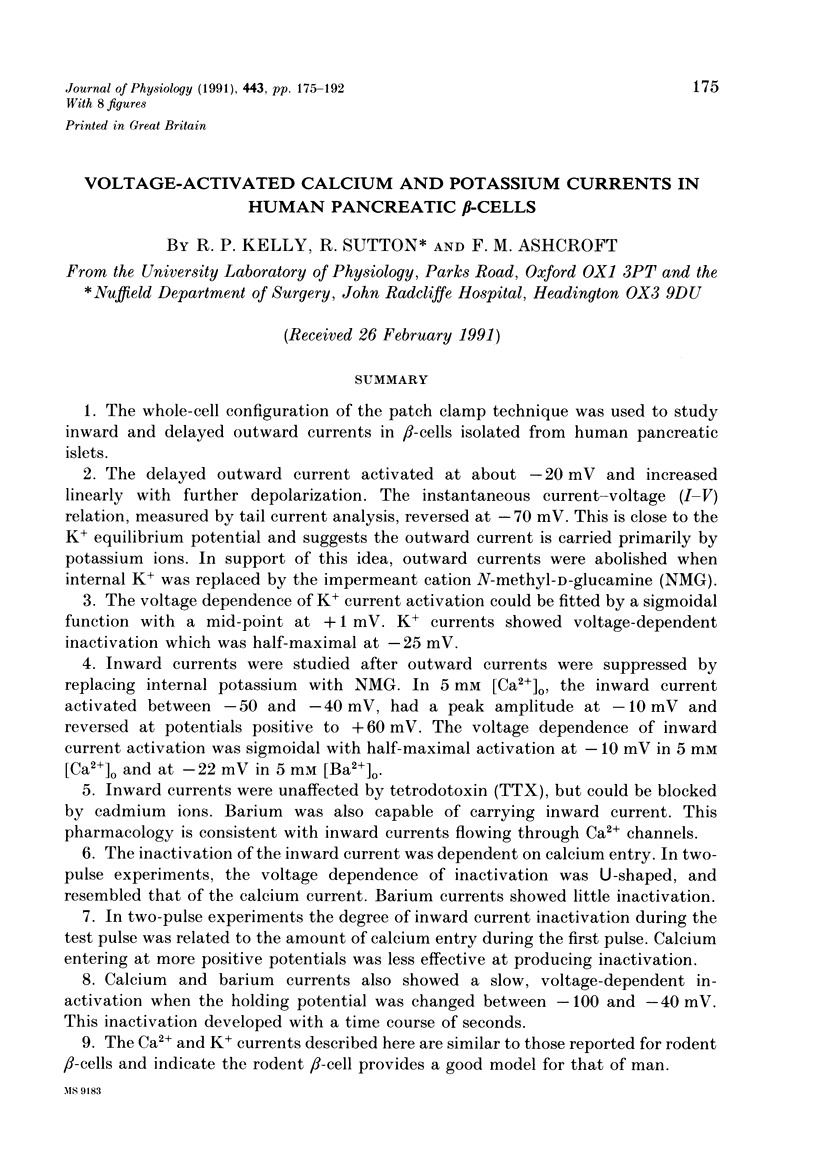
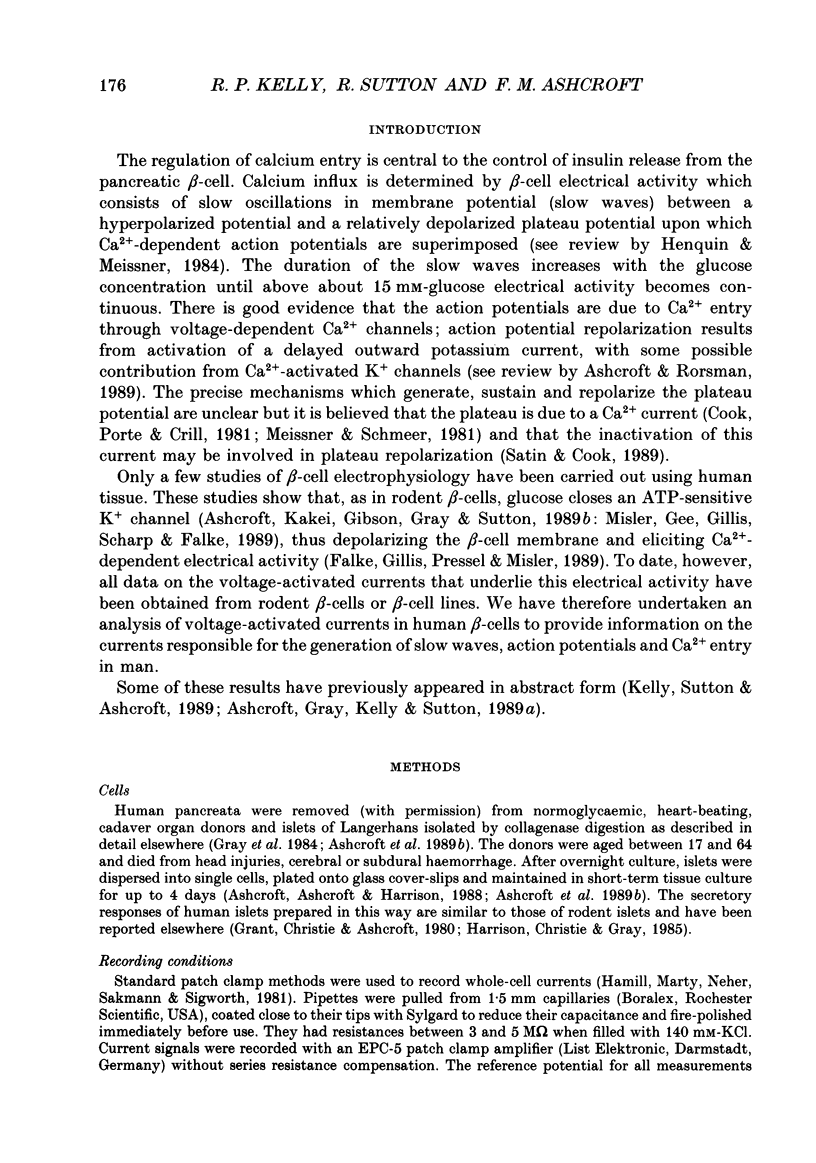
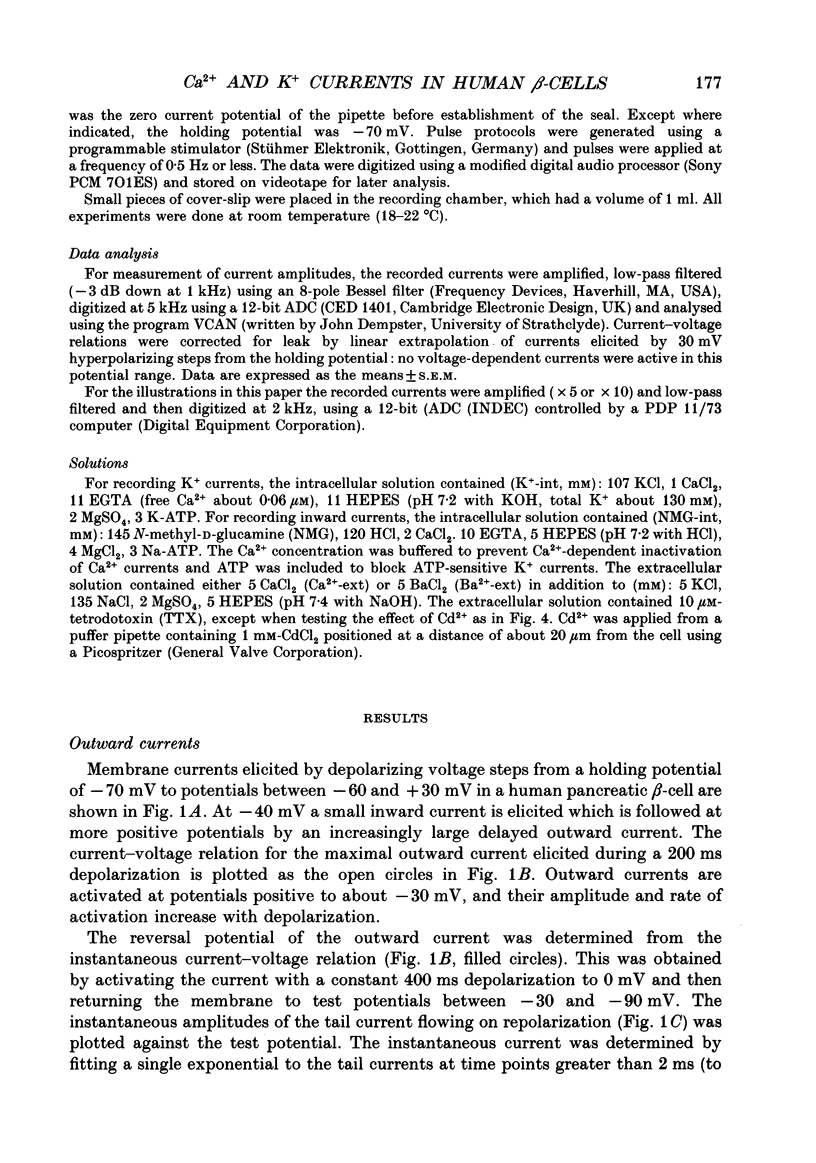
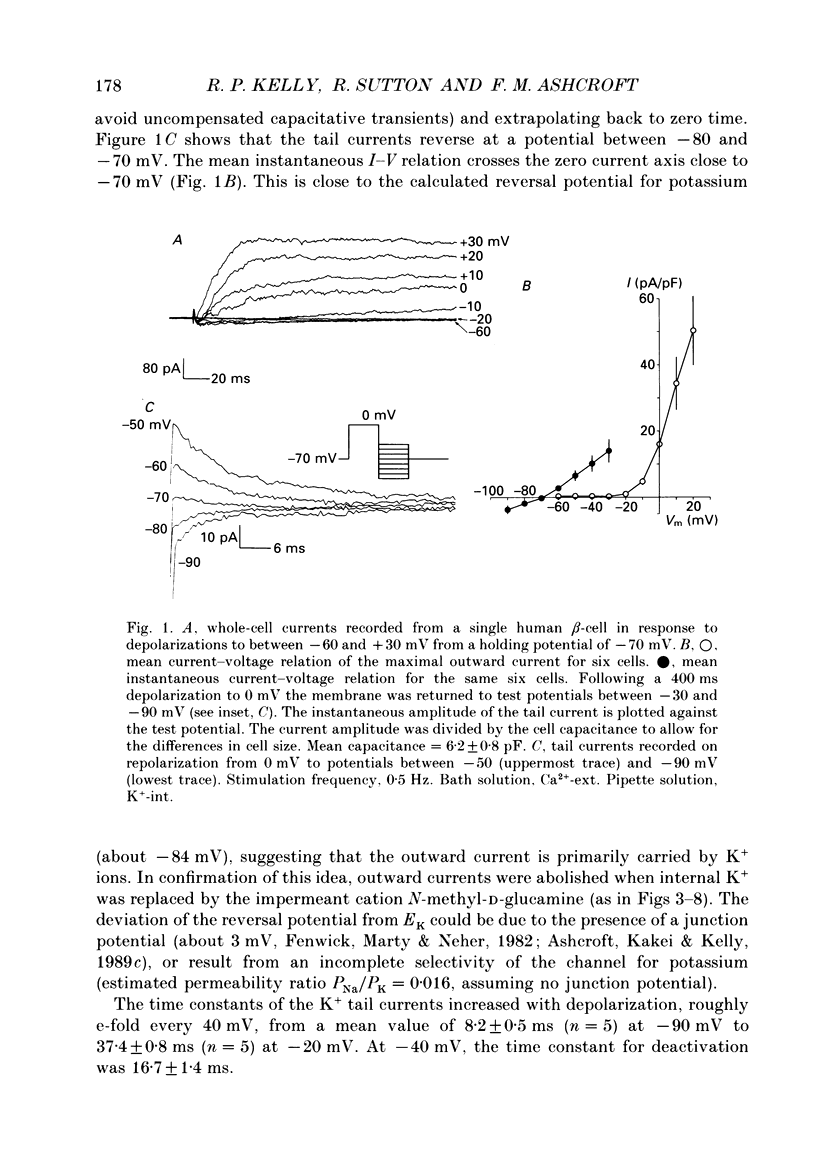
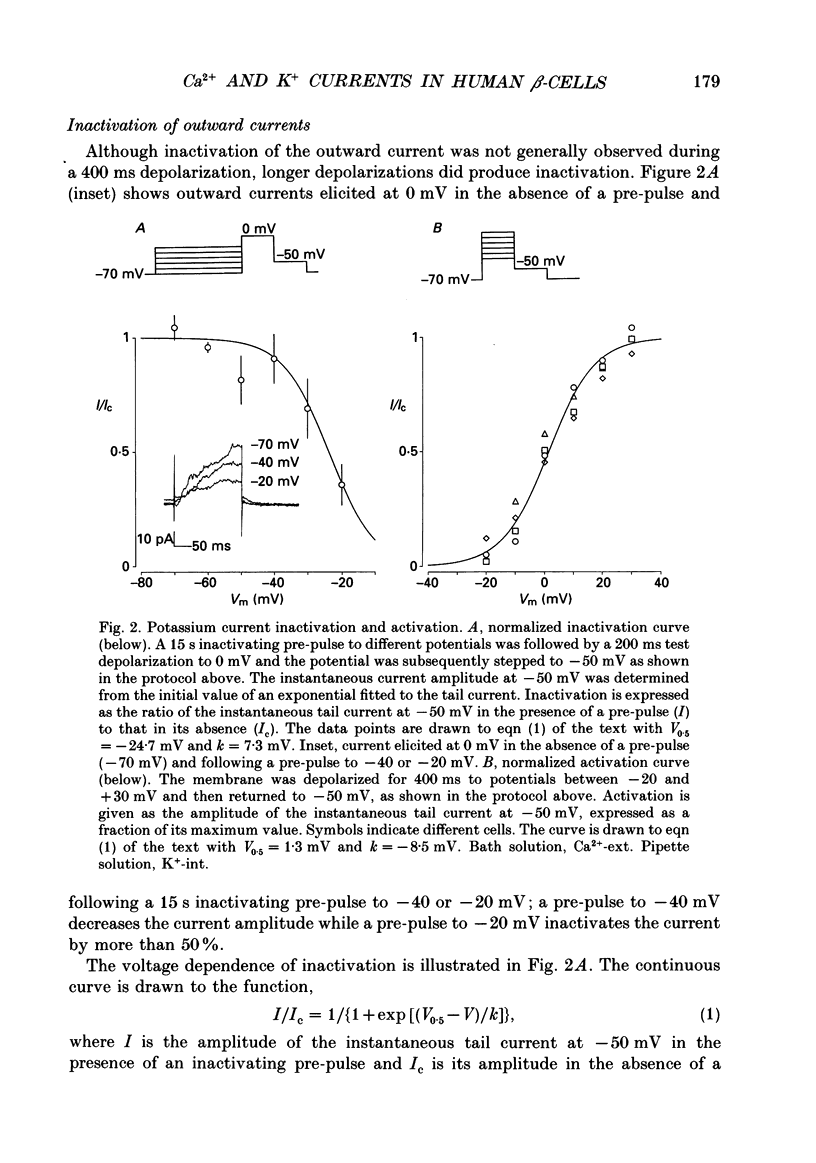
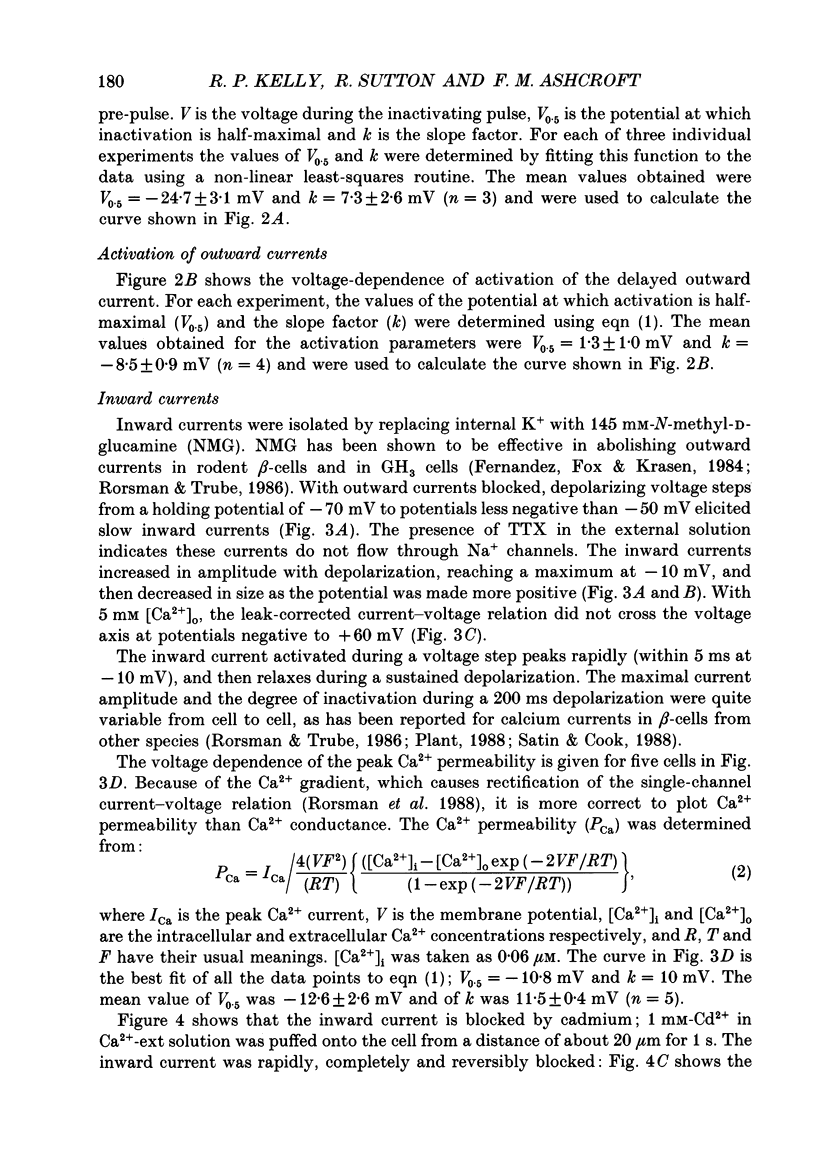
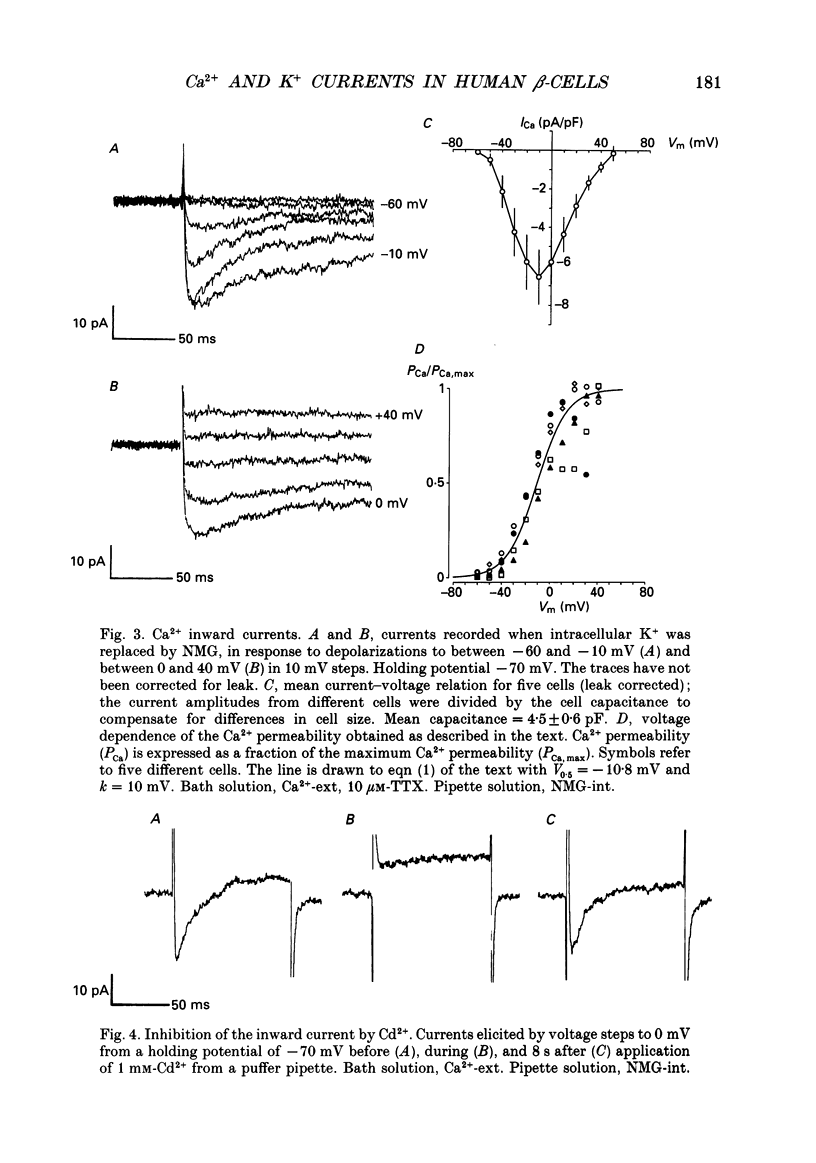
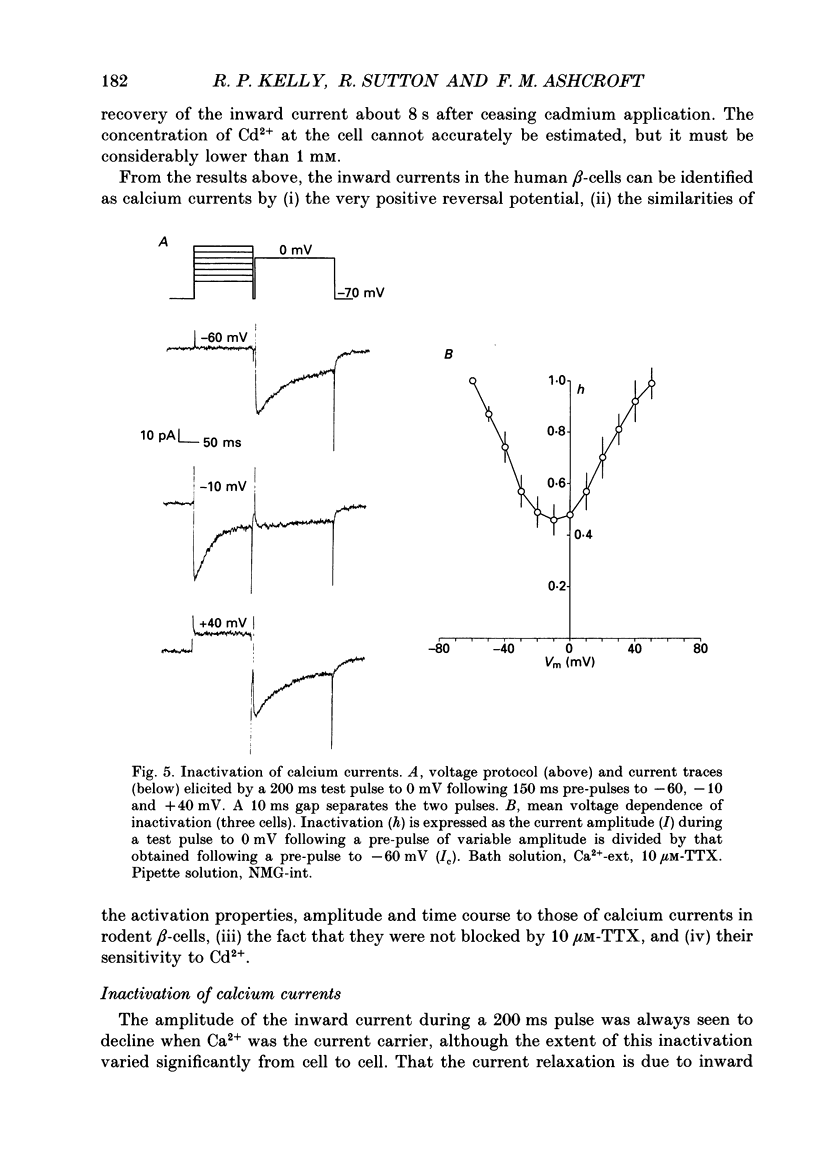
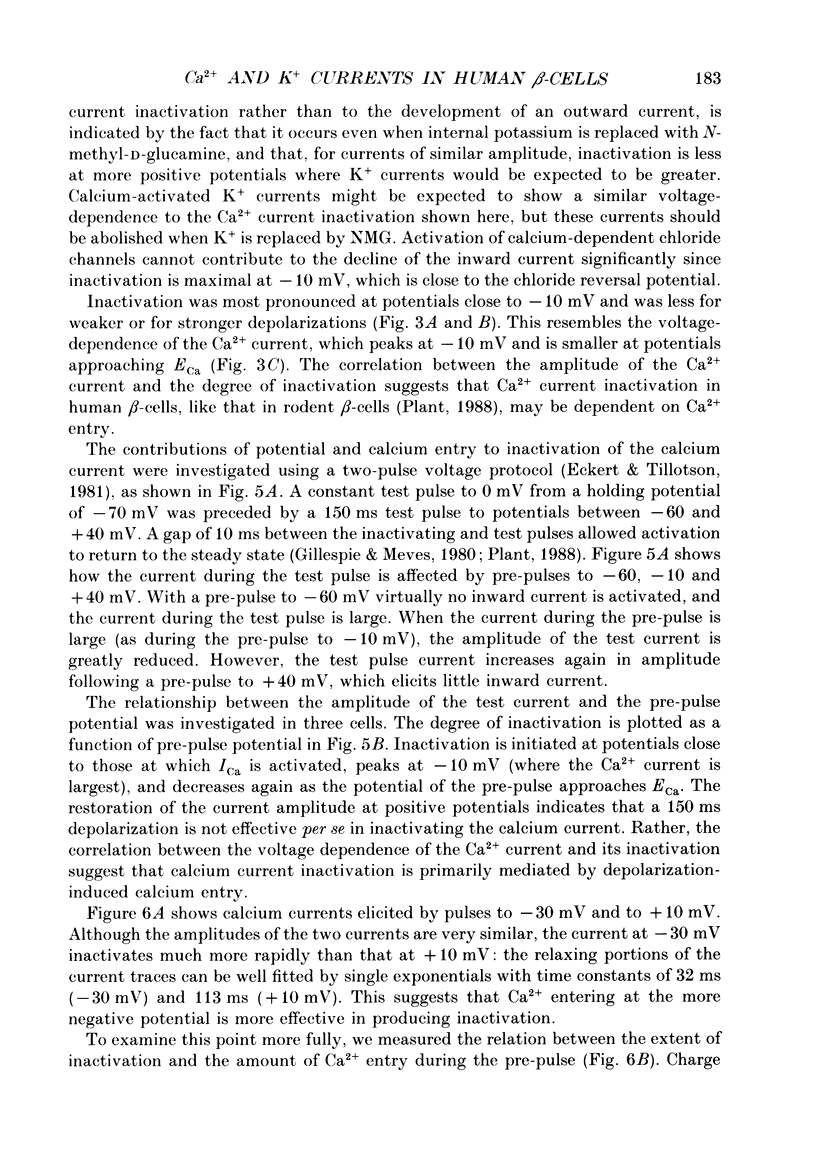
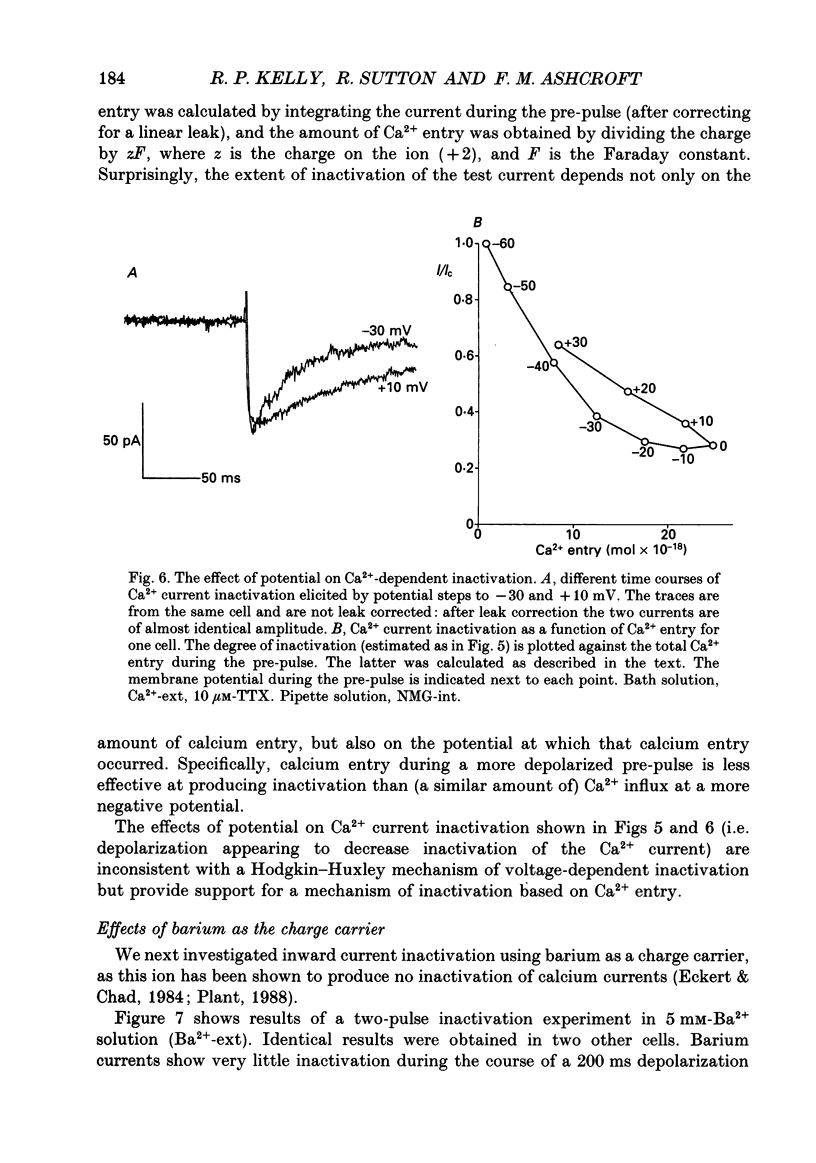
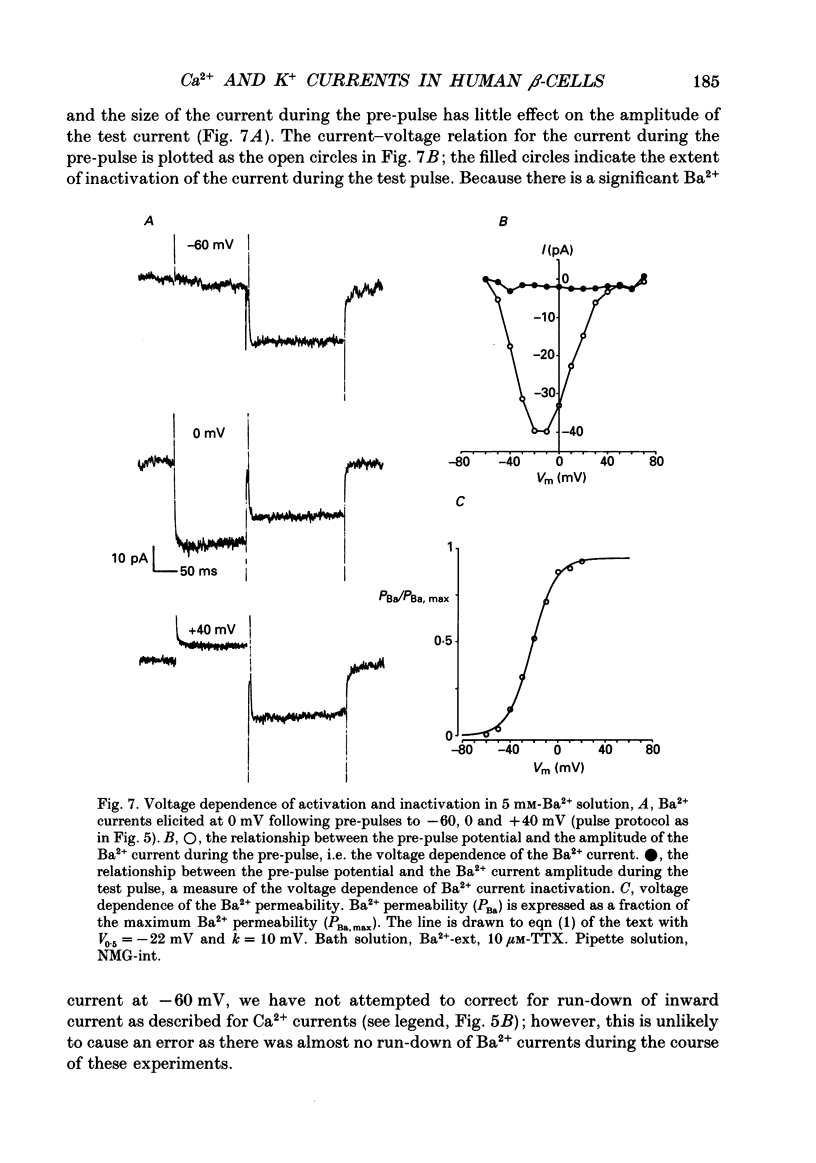
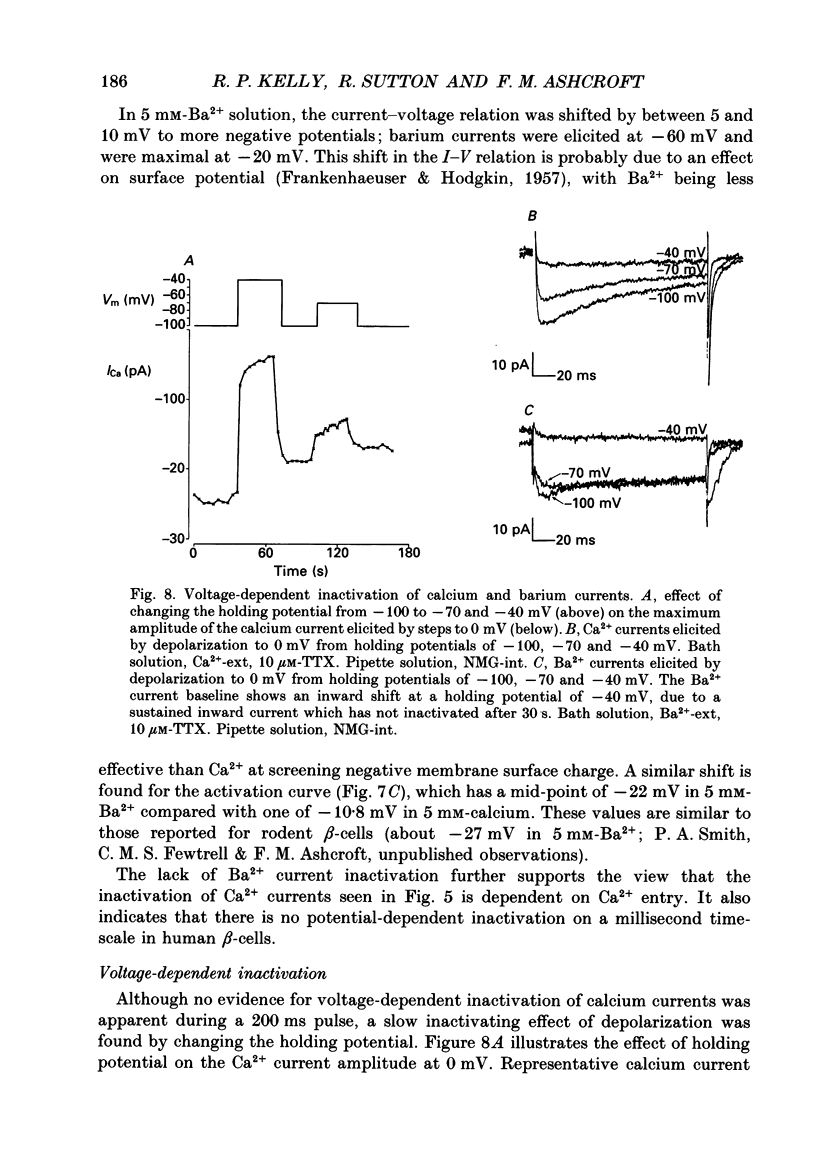
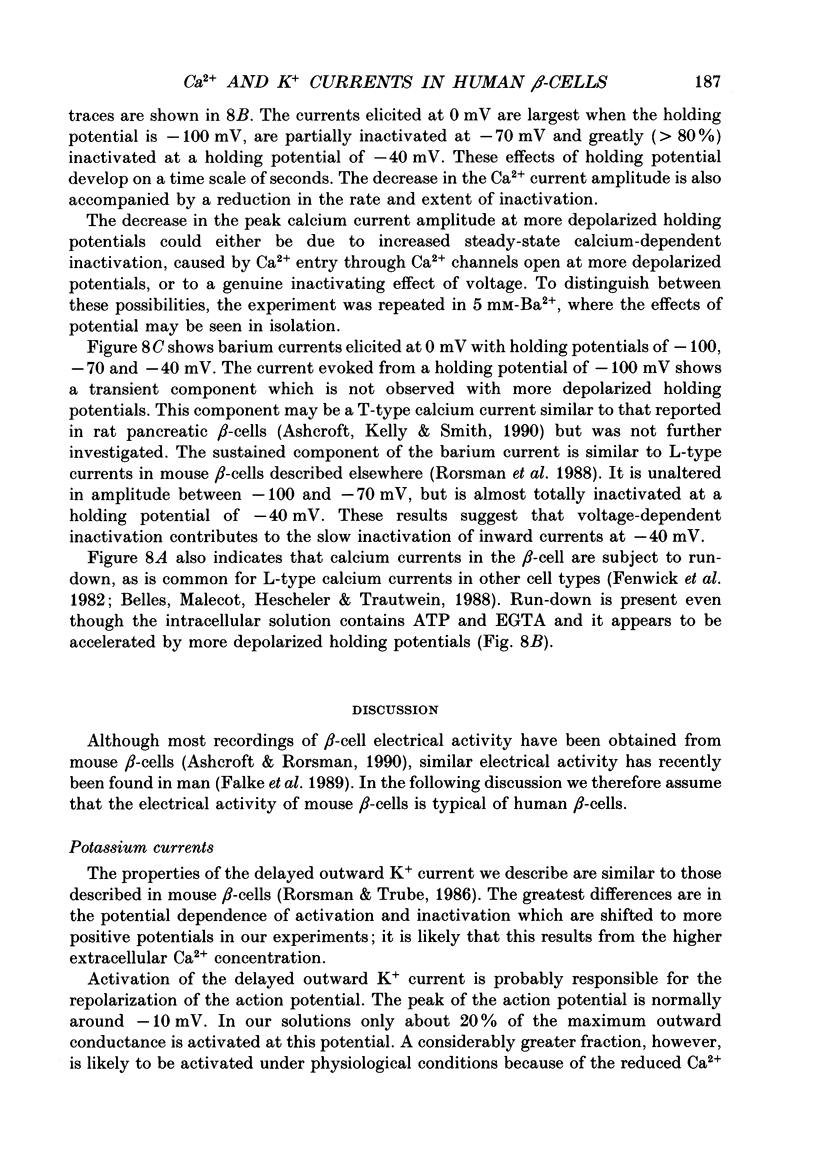
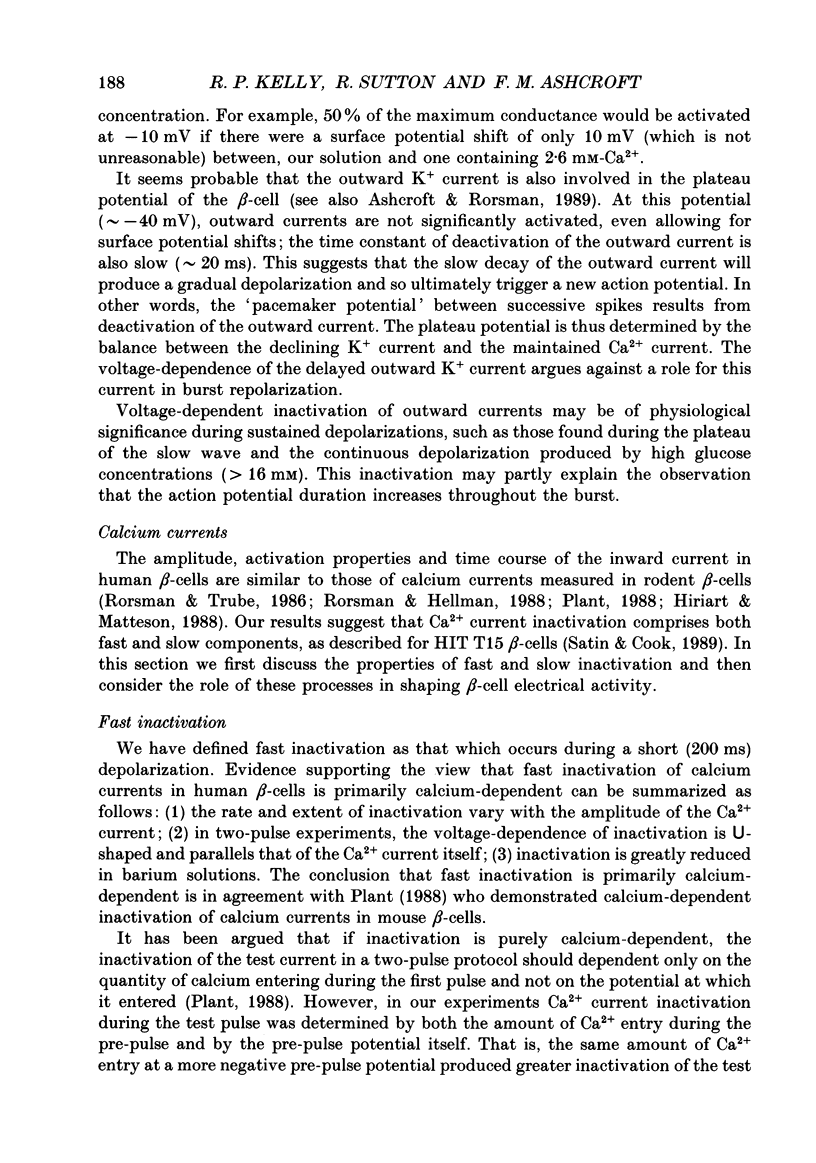
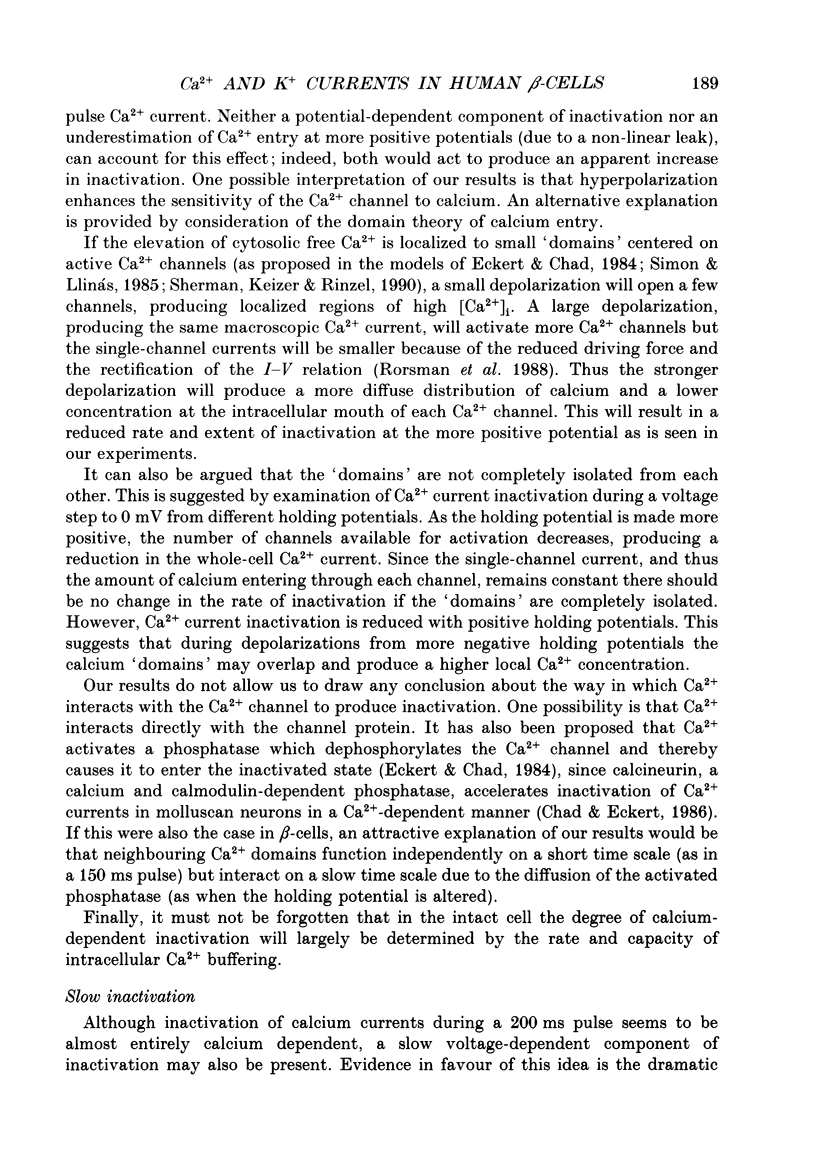
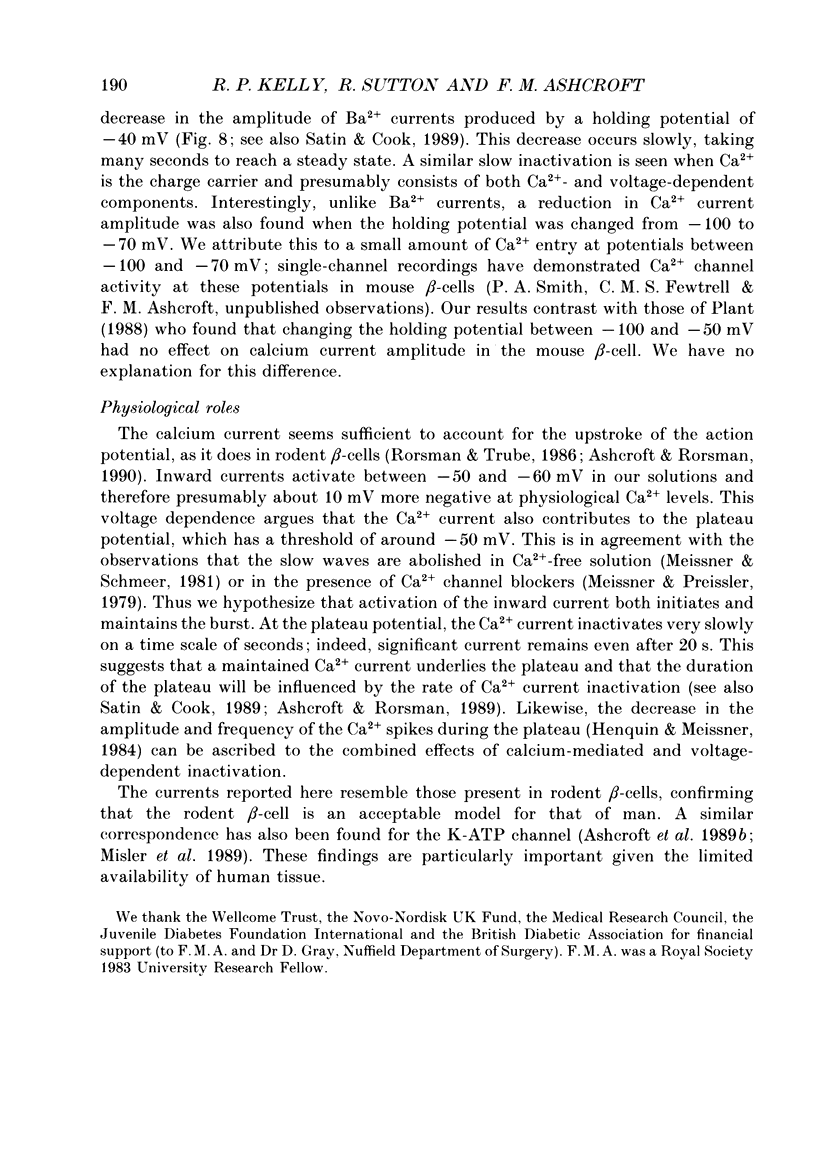
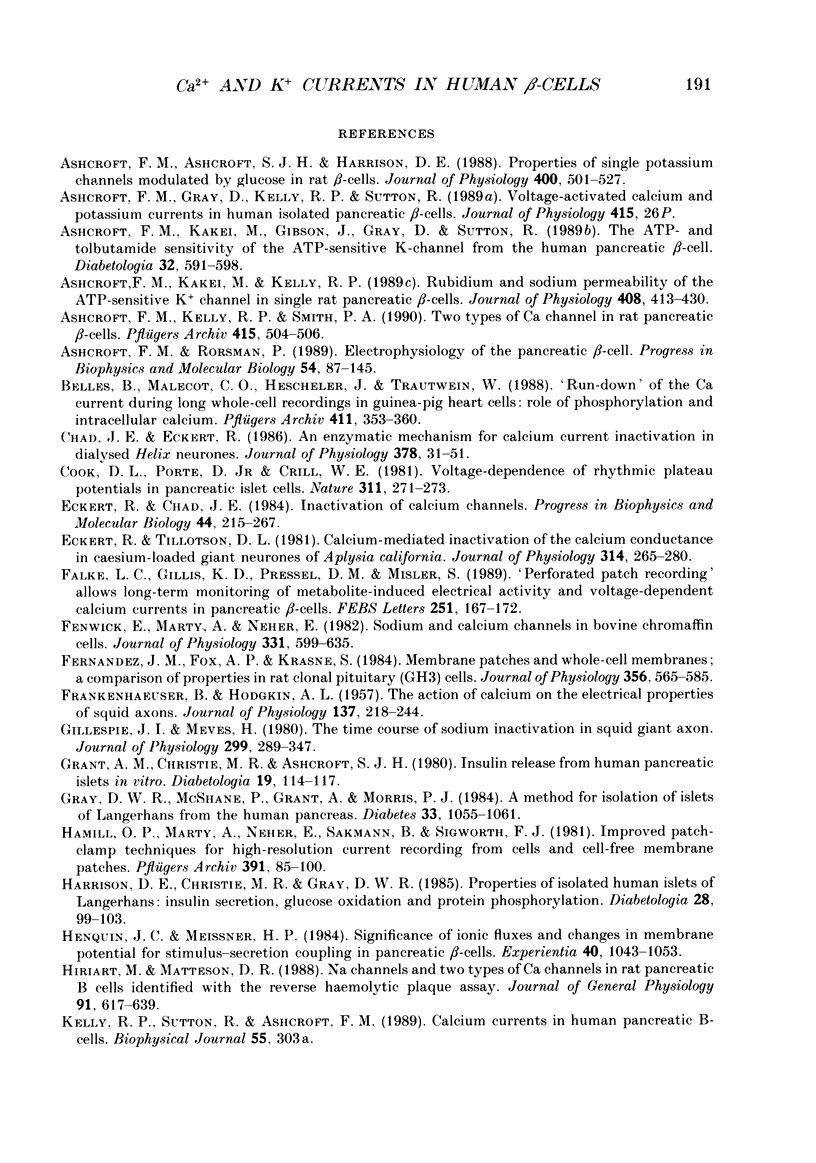
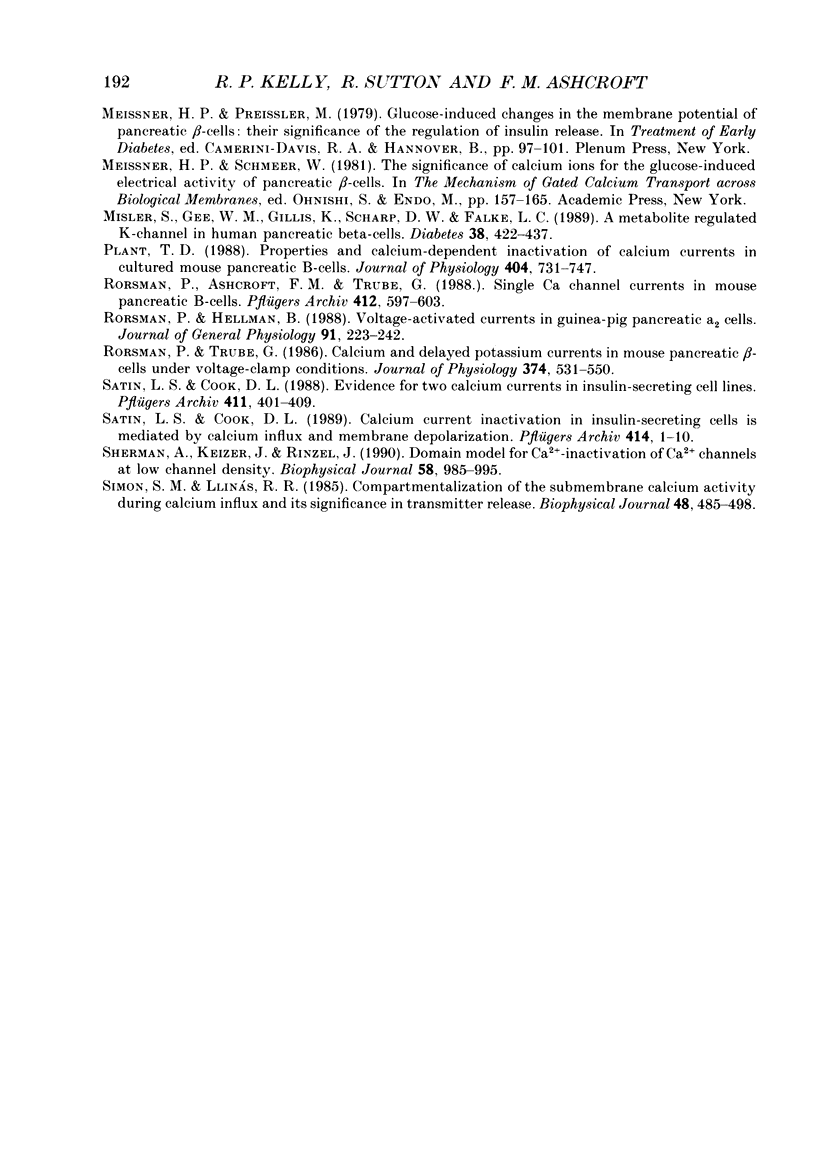
Selected References
These references are in PubMed. This may not be the complete list of references from this article.
- Ashcroft F. M., Ashcroft S. J., Harrison D. E. Properties of single potassium channels modulated by glucose in rat pancreatic beta-cells. J Physiol. 1988 Jun;400:501–527. doi: 10.1113/jphysiol.1988.sp017134. [DOI] [PMC free article] [PubMed] [Google Scholar]
- Ashcroft F. M., Kakei M., Gibson J. S., Gray D. W., Sutton R. The ATP- and tolbutamide-sensitivity of the ATP-sensitive K-channel from human pancreatic B cells. Diabetologia. 1989 Aug;32(8):591–598. doi: 10.1007/BF00285333. [DOI] [PubMed] [Google Scholar]
- Ashcroft F. M., Kakei M., Kelly R. P. Rubidium and sodium permeability of the ATP-sensitive K+ channel in single rat pancreatic beta-cells. J Physiol. 1989 Jan;408:413–429. doi: 10.1113/jphysiol.1989.sp017467. [DOI] [PMC free article] [PubMed] [Google Scholar]
- Ashcroft F. M., Kelly R. P., Smith P. A. Two types of Ca channel in rat pancreatic beta-cells. Pflugers Arch. 1990 Jan;415(4):504–506. doi: 10.1007/BF00373633. [DOI] [PubMed] [Google Scholar]
- Ashcroft F. M., Rorsman P. Electrophysiology of the pancreatic beta-cell. Prog Biophys Mol Biol. 1989;54(2):87–143. doi: 10.1016/0079-6107(89)90013-8. [DOI] [PubMed] [Google Scholar]
- Belles B., Malécot C. O., Hescheler J., Trautwein W. "Run-down" of the Ca current during long whole-cell recordings in guinea pig heart cells: role of phosphorylation and intracellular calcium. Pflugers Arch. 1988 Apr;411(4):353–360. doi: 10.1007/BF00587713. [DOI] [PubMed] [Google Scholar]
- Chad J. E., Eckert R. An enzymatic mechanism for calcium current inactivation in dialysed Helix neurones. J Physiol. 1986 Sep;378:31–51. doi: 10.1113/jphysiol.1986.sp016206. [DOI] [PMC free article] [PubMed] [Google Scholar]
- Cook D. L., Hales C. N. Intracellular ATP directly blocks K+ channels in pancreatic B-cells. Nature. 1984 Sep 20;311(5983):271–273. doi: 10.1038/311271a0. [DOI] [PubMed] [Google Scholar]
- Eckert R., Chad J. E. Inactivation of Ca channels. Prog Biophys Mol Biol. 1984;44(3):215–267. doi: 10.1016/0079-6107(84)90009-9. [DOI] [PubMed] [Google Scholar]
- Eckert R., Tillotson D. L. Calcium-mediated inactivation of the calcium conductance in caesium-loaded giant neurones of Aplysia californica. J Physiol. 1981 May;314:265–280. doi: 10.1113/jphysiol.1981.sp013706. [DOI] [PMC free article] [PubMed] [Google Scholar]
- FRANKENHAEUSER B., HODGKIN A. L. The action of calcium on the electrical properties of squid axons. J Physiol. 1957 Jul 11;137(2):218–244. doi: 10.1113/jphysiol.1957.sp005808. [DOI] [PMC free article] [PubMed] [Google Scholar]
- Falke L. C., Gillis K. D., Pressel D. M., Misler S. 'Perforated patch recording' allows long-term monitoring of metabolite-induced electrical activity and voltage-dependent Ca2+ currents in pancreatic islet B cells. FEBS Lett. 1989 Jul 17;251(1-2):167–172. doi: 10.1016/0014-5793(89)81448-6. [DOI] [PubMed] [Google Scholar]
- Fenwick E. M., Marty A., Neher E. Sodium and calcium channels in bovine chromaffin cells. J Physiol. 1982 Oct;331:599–635. doi: 10.1113/jphysiol.1982.sp014394. [DOI] [PMC free article] [PubMed] [Google Scholar]
- Fernandez J. M., Fox A. P., Krasne S. Membrane patches and whole-cell membranes: a comparison of electrical properties in rat clonal pituitary (GH3) cells. J Physiol. 1984 Nov;356:565–585. doi: 10.1113/jphysiol.1984.sp015483. [DOI] [PMC free article] [PubMed] [Google Scholar]
- Gillespie J. I., Meves H. The time course of sodium inactivation in squid giant axons. J Physiol. 1980 Feb;299:289–307. doi: 10.1113/jphysiol.1980.sp013125. [DOI] [PMC free article] [PubMed] [Google Scholar]
- Grant A. M., Christie M. R., Ashcroft S. J. Insulin release from human pancreatic islets in vitro. Diabetologia. 1980 Aug;19(2):114–117. doi: 10.1007/BF00421856. [DOI] [PubMed] [Google Scholar]
- Gray D. W., McShane P., Grant A., Morris P. J. A method for isolation of islets of Langerhans from the human pancreas. Diabetes. 1984 Nov;33(11):1055–1061. doi: 10.2337/diab.33.11.1055. [DOI] [PubMed] [Google Scholar]
- Hamill O. P., Marty A., Neher E., Sakmann B., Sigworth F. J. Improved patch-clamp techniques for high-resolution current recording from cells and cell-free membrane patches. Pflugers Arch. 1981 Aug;391(2):85–100. doi: 10.1007/BF00656997. [DOI] [PubMed] [Google Scholar]
- Harrison D. E., Christie M. R., Gray D. W. Properties of isolated human islets of Langerhans: insulin secretion, glucose oxidation and protein phosphorylation. Diabetologia. 1985 Feb;28(2):99–103. doi: 10.1007/BF00279924. [DOI] [PubMed] [Google Scholar]
- Henquin J. C., Meissner H. P. Significance of ionic fluxes and changes in membrane potential for stimulus-secretion coupling in pancreatic B-cells. Experientia. 1984 Oct 15;40(10):1043–1052. doi: 10.1007/BF01971450. [DOI] [PubMed] [Google Scholar]
- Hiriart M., Matteson D. R. Na channels and two types of Ca channels in rat pancreatic B cells identified with the reverse hemolytic plaque assay. J Gen Physiol. 1988 May;91(5):617–639. doi: 10.1085/jgp.91.5.617. [DOI] [PMC free article] [PubMed] [Google Scholar]
- Misler S., Gee W. M., Gillis K. D., Scharp D. W., Falke L. C. Metabolite-regulated ATP-sensitive K+ channel in human pancreatic islet cells. Diabetes. 1989 Apr;38(4):422–427. doi: 10.2337/diab.38.4.422. [DOI] [PubMed] [Google Scholar]
- Plant T. D. Properties and calcium-dependent inactivation of calcium currents in cultured mouse pancreatic B-cells. J Physiol. 1988 Oct;404:731–747. doi: 10.1113/jphysiol.1988.sp017316. [DOI] [PMC free article] [PubMed] [Google Scholar]
- Rorsman P., Ashcroft F. M., Trube G. Single Ca channel currents in mouse pancreatic B-cells. Pflugers Arch. 1988 Oct;412(6):597–603. doi: 10.1007/BF00583760. [DOI] [PubMed] [Google Scholar]
- Rorsman P., Hellman B. Voltage-activated currents in guinea pig pancreatic alpha 2 cells. Evidence for Ca2+-dependent action potentials. J Gen Physiol. 1988 Feb;91(2):223–242. doi: 10.1085/jgp.91.2.223. [DOI] [PMC free article] [PubMed] [Google Scholar]
- Rorsman P., Trube G. Calcium and delayed potassium currents in mouse pancreatic beta-cells under voltage-clamp conditions. J Physiol. 1986 May;374:531–550. doi: 10.1113/jphysiol.1986.sp016096. [DOI] [PMC free article] [PubMed] [Google Scholar]
- Satin L. S., Cook D. L. Calcium current inactivation in insulin-secreting cells is mediated by calcium influx and membrane depolarization. Pflugers Arch. 1989 May;414(1):1–10. doi: 10.1007/BF00585619. [DOI] [PubMed] [Google Scholar]
- Satin L. S., Cook D. L. Evidence for two calcium currents in insulin-secreting cells. Pflugers Arch. 1988 Apr;411(4):401–409. doi: 10.1007/BF00587719. [DOI] [PubMed] [Google Scholar]
- Sherman A., Keizer J., Rinzel J. Domain model for Ca2(+)-inactivation of Ca2+ channels at low channel density. Biophys J. 1990 Oct;58(4):985–995. doi: 10.1016/S0006-3495(90)82443-7. [DOI] [PMC free article] [PubMed] [Google Scholar]
- Simon S. M., Llinás R. R. Compartmentalization of the submembrane calcium activity during calcium influx and its significance in transmitter release. Biophys J. 1985 Sep;48(3):485–498. doi: 10.1016/S0006-3495(85)83804-2. [DOI] [PMC free article] [PubMed] [Google Scholar]