Abstract
1. Suction electrode and whole-cell recording were used to record membrane currents from defined regions of solitary olfactory receptor cells from Ambystoma tigrinum. 2. Under whole-cell current clamp, stimulation of cells with odorants activated an inward current in the cilia, an outward current in the soma, and induced a membrane depolarization. Clamping the membrane potential at its resting value of -70 mV increased the inward ciliary current 5- to 10-fold and abolished the outward somatic current. 3. Local odorant stimulation was accomplished by ejecting an odorant solution into a steady flow of Ringer solution. A suction electrode was used to immobilize a cell in the flow and to record the odorant-induced somatic current. The amplitude of the odorant response increased approximately linearly with the length of cilia exposed to the stimulus, but was independent of the length of dendrite exposed to the stimulus, indicating that odorant sensitivity is predominantly localized to the cilia. 4. The latencies of responses recorded under flow did not vary with the region of the cilia which was exposed to the stimulus. Also, the magnitude of the inward ciliary current activated by odorants was equal to that of the whole-cell current recorded under voltage clamp. These observations indicate that the odorant-induced inward current is predominantly localized to the ciliary membrane. 5. Under whole-cell current clamp, local application of a high-K+ solution generated an outward somatic current when applied to the dendrite, but had no effect when applied to the cilia. This indicates that the density of the resting K+ conductance is lower in the ciliary membrane than in the dendritic membrane. 6. The results above are consistent with the hypothesis that all components of the transduction mechanism are uniformly distributed within the cilia, and that the cilia are electrotonically compact, even during an odorant-induced conductance increase.
Full text
PDF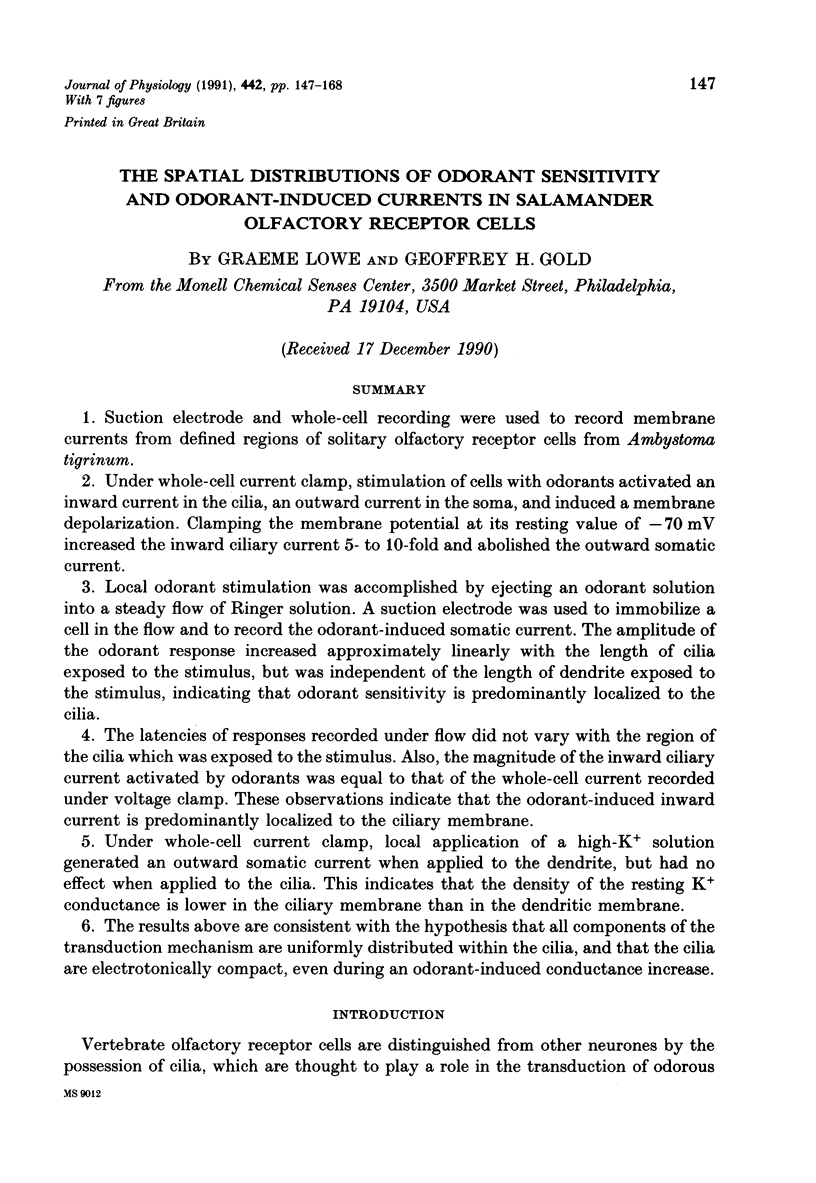
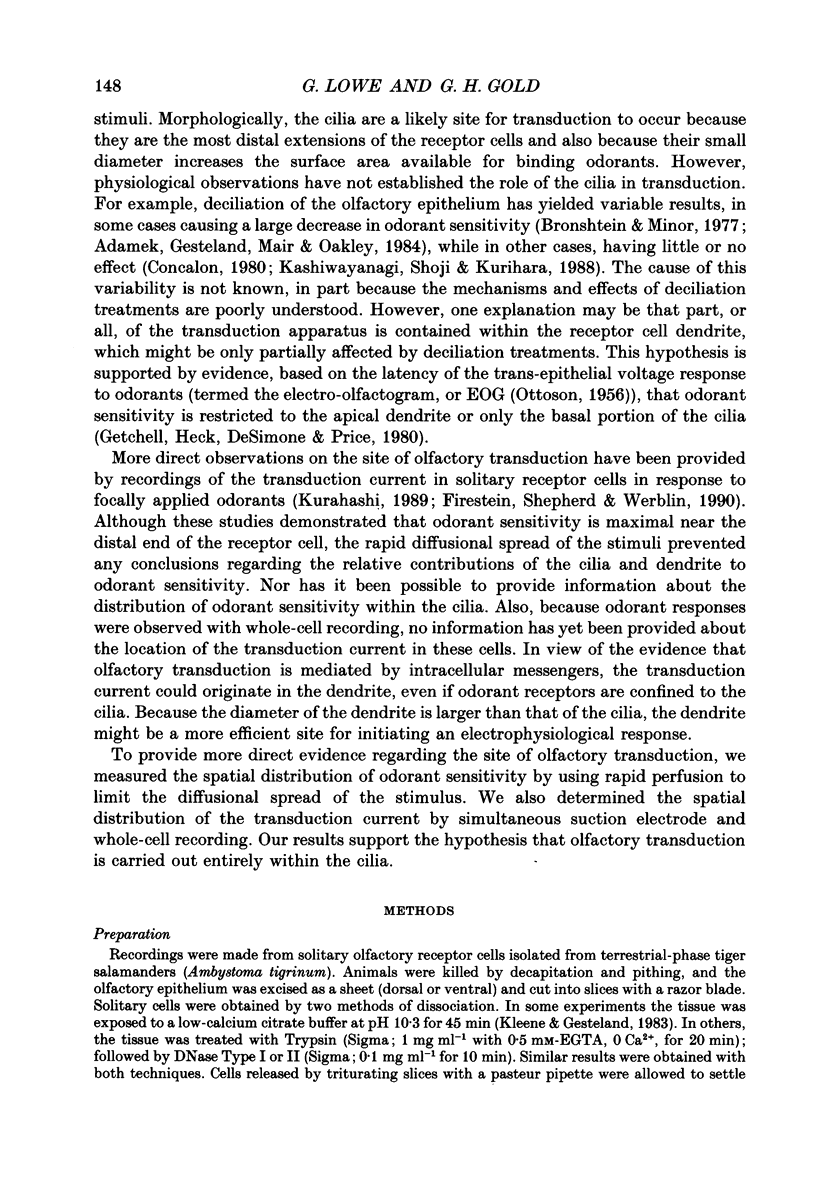
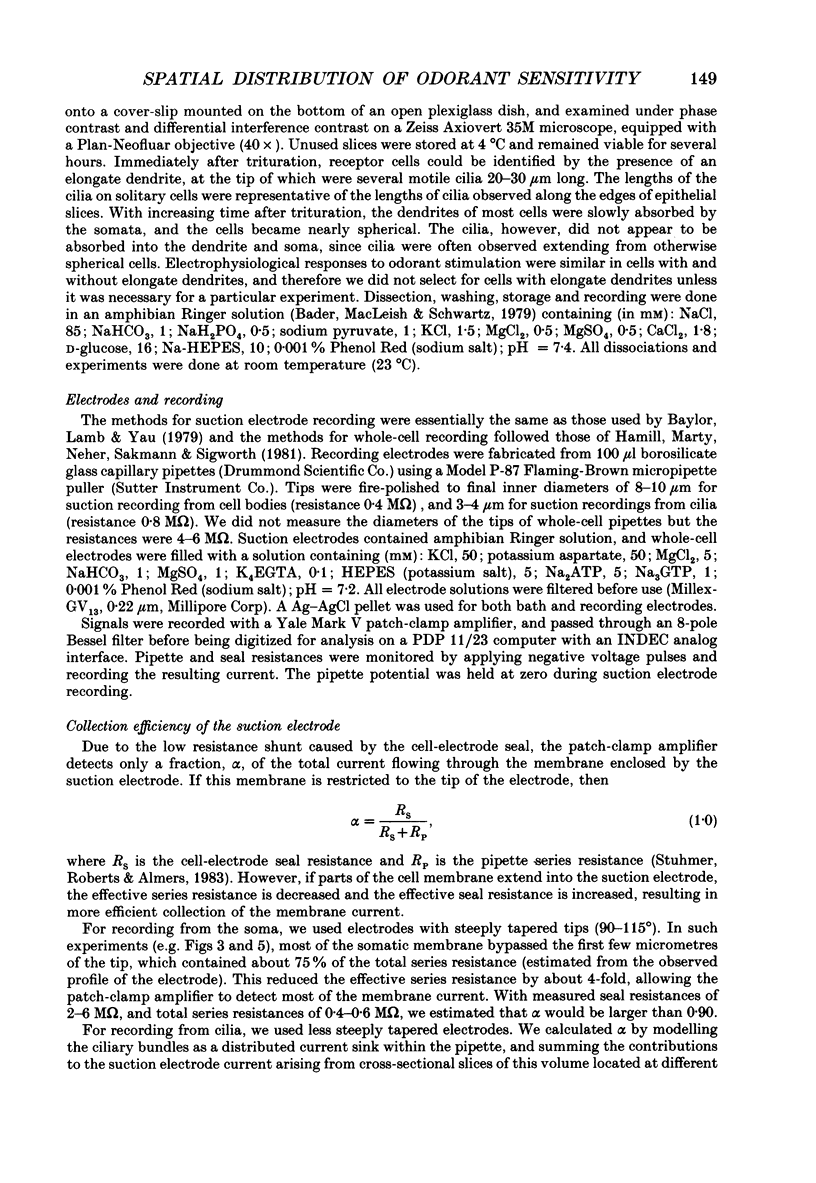
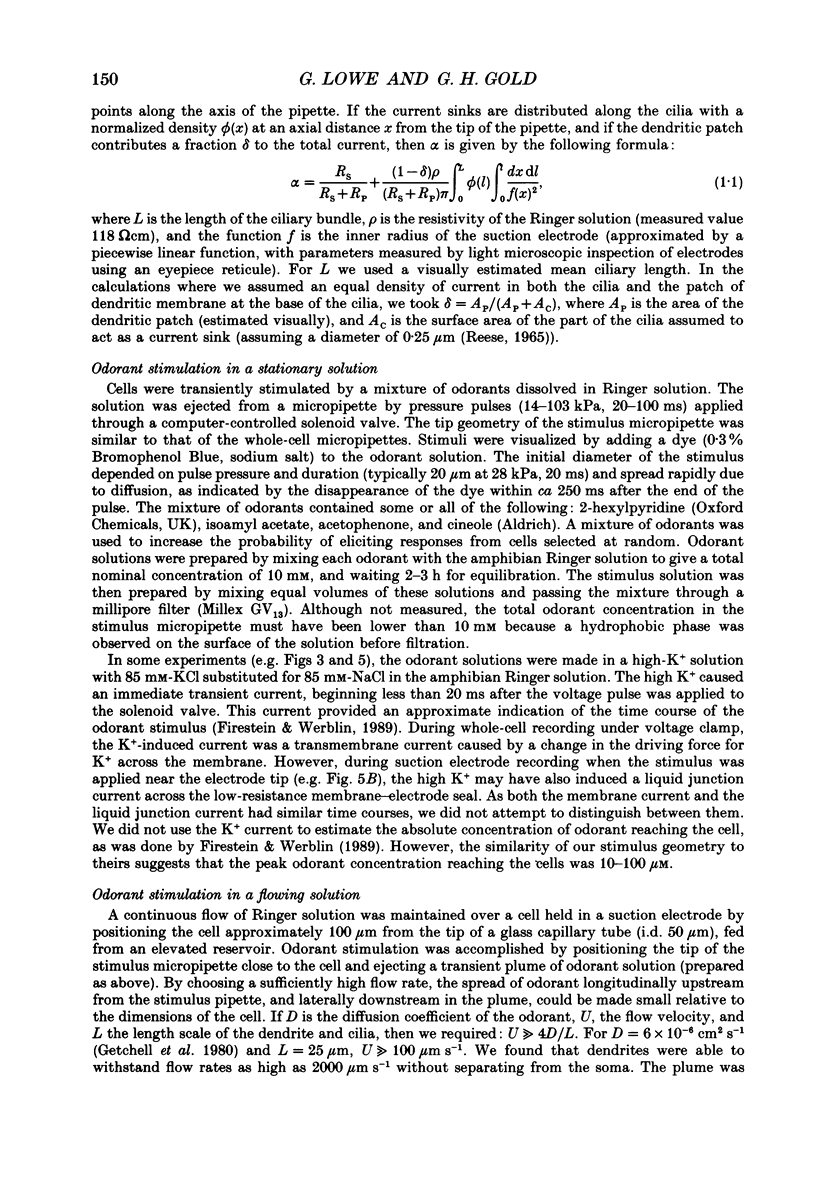
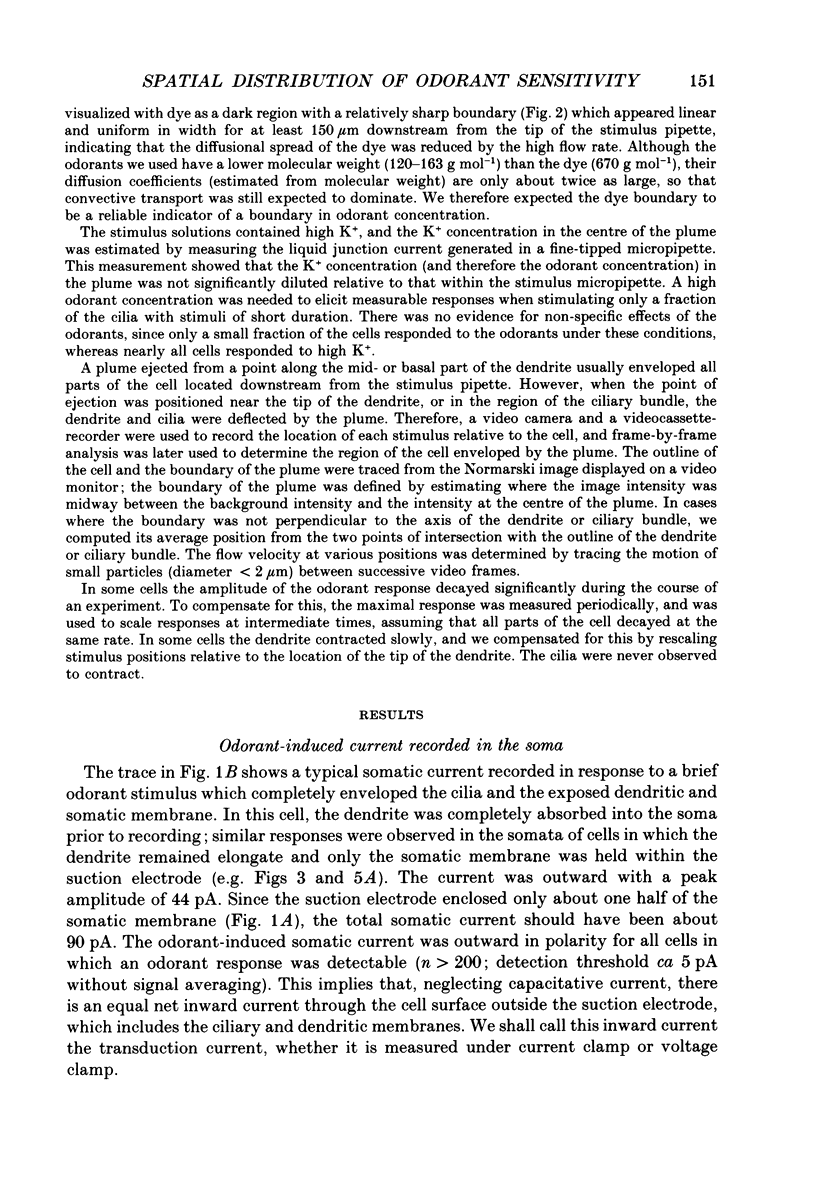
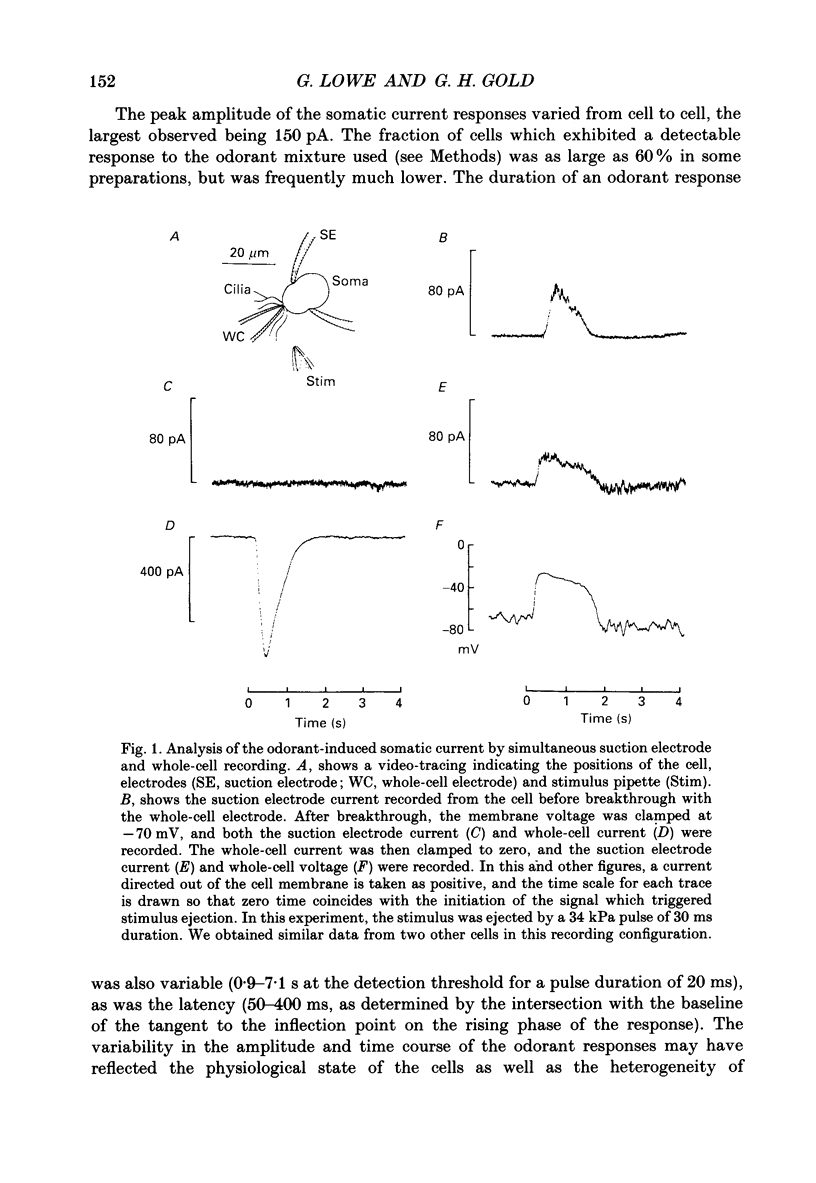
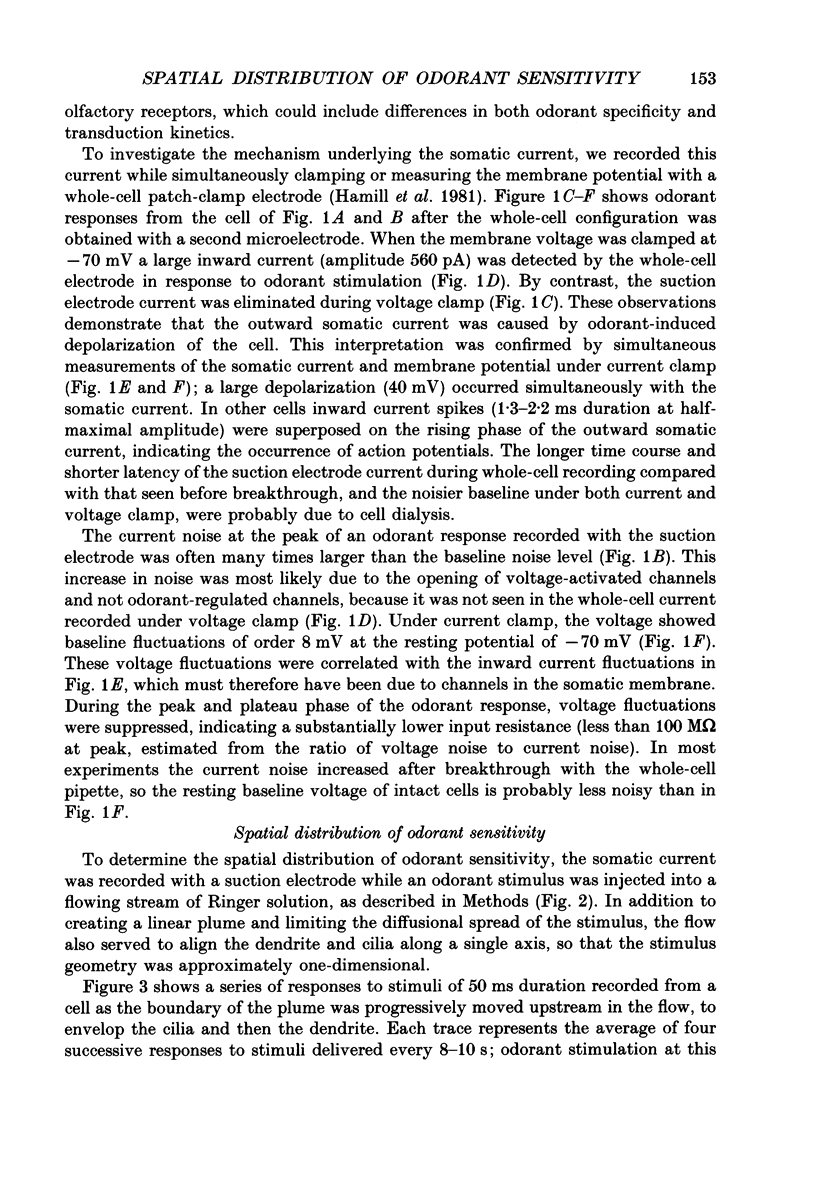
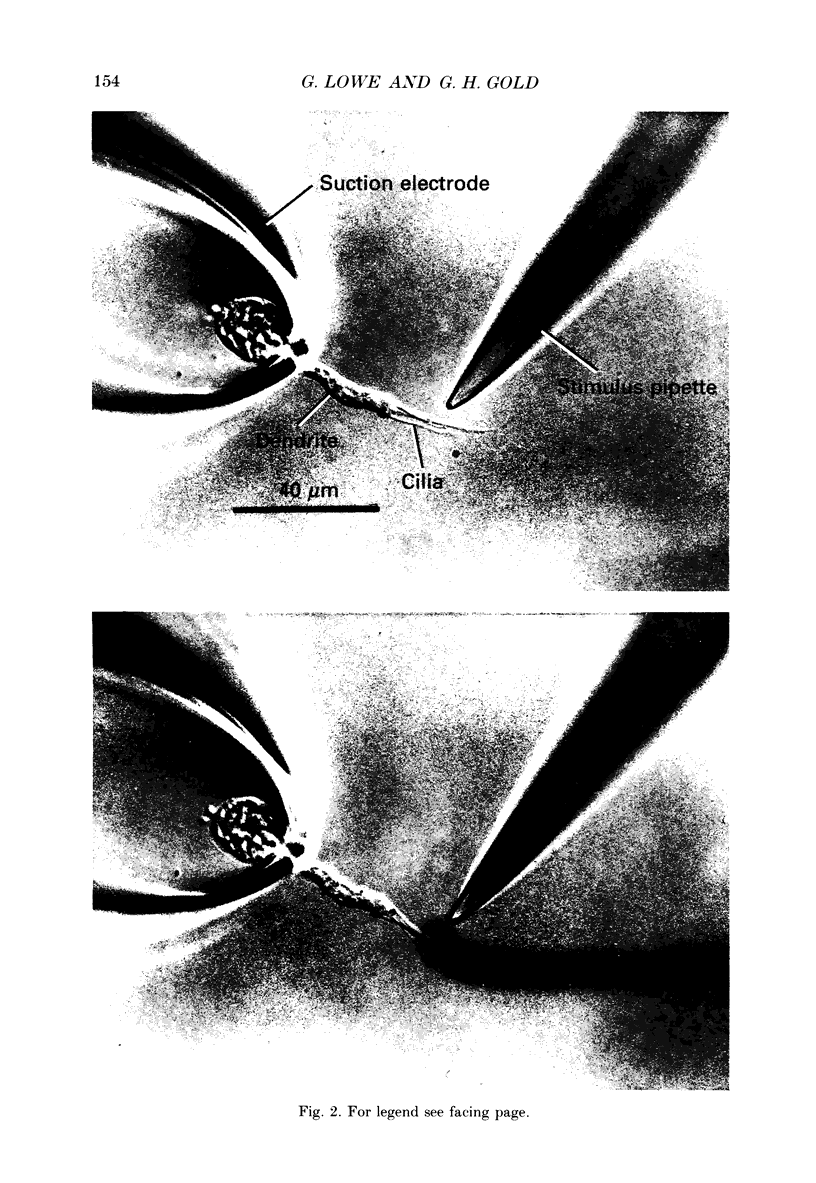
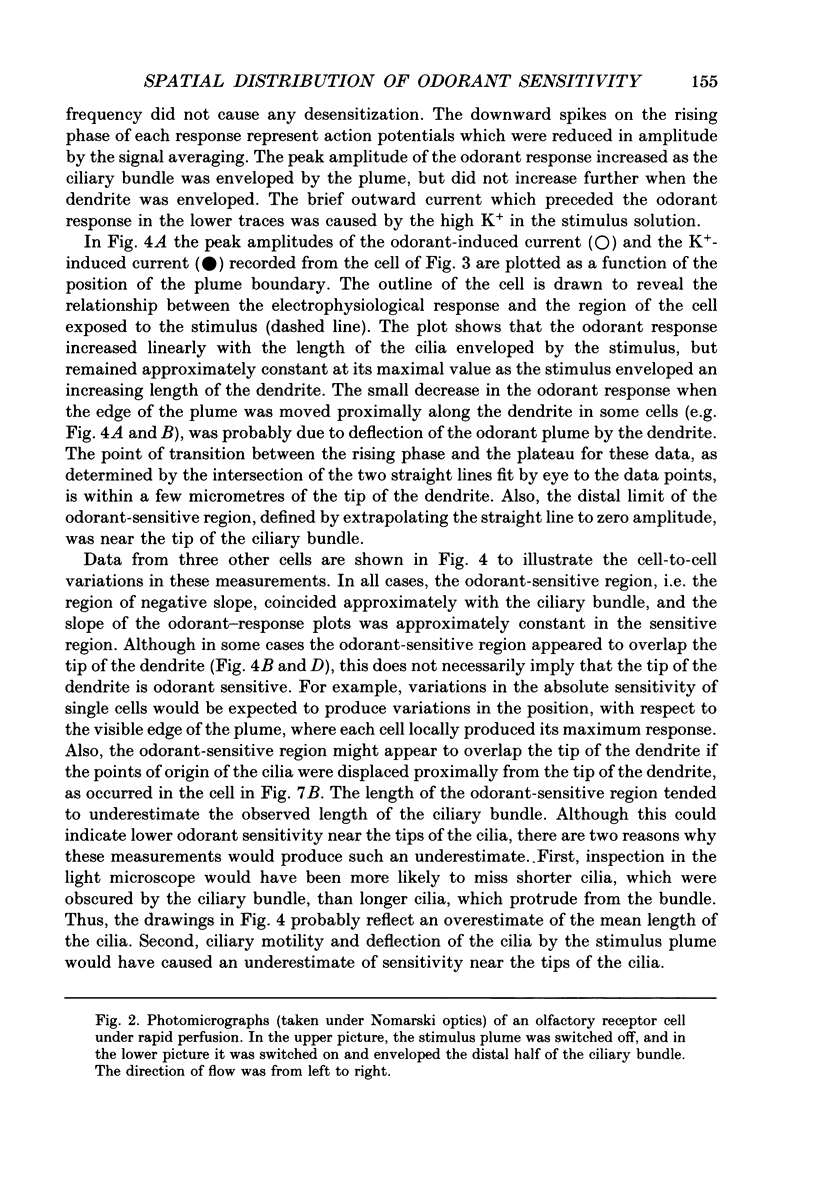
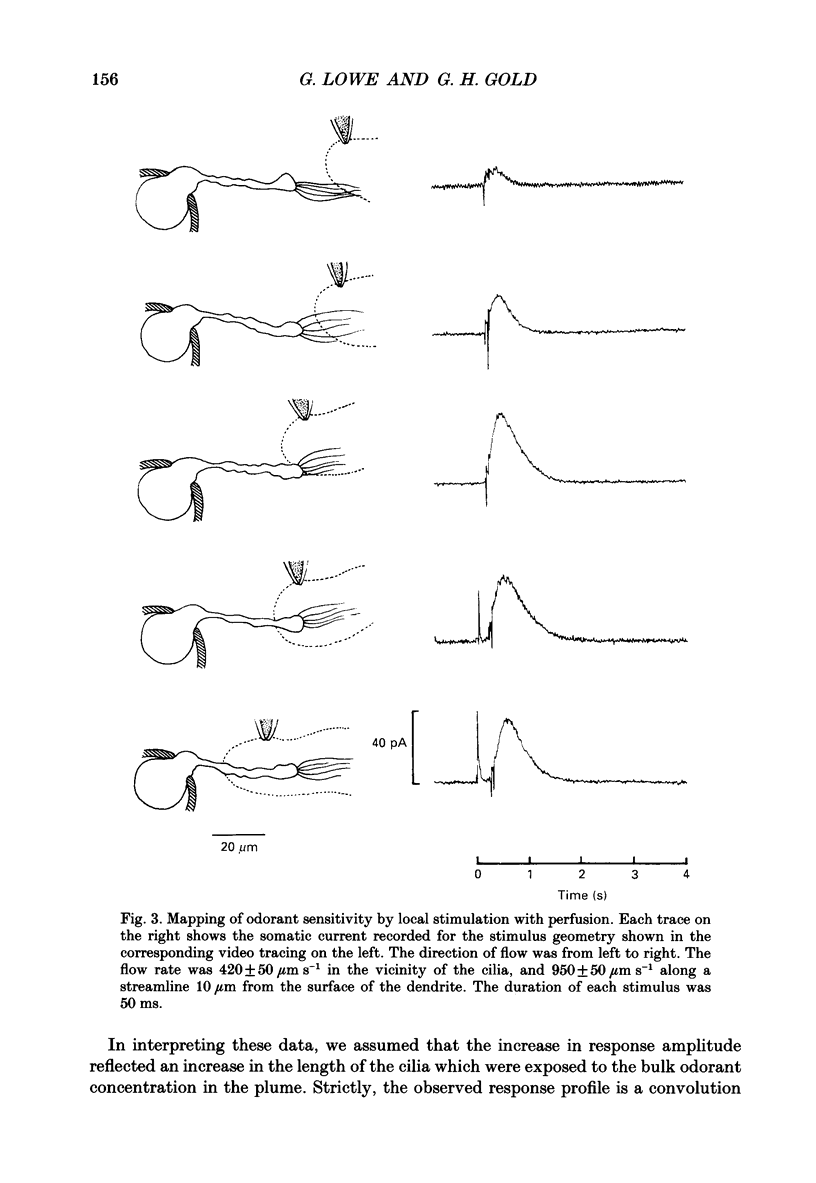
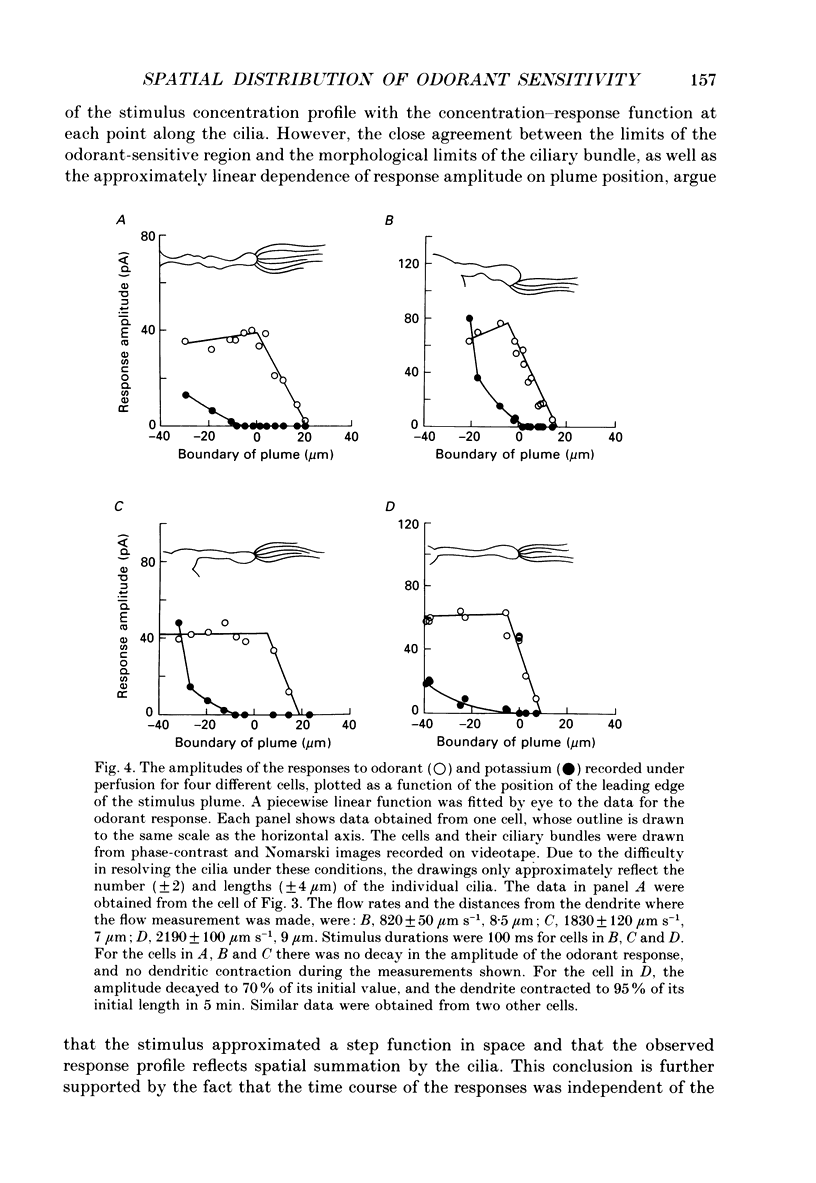
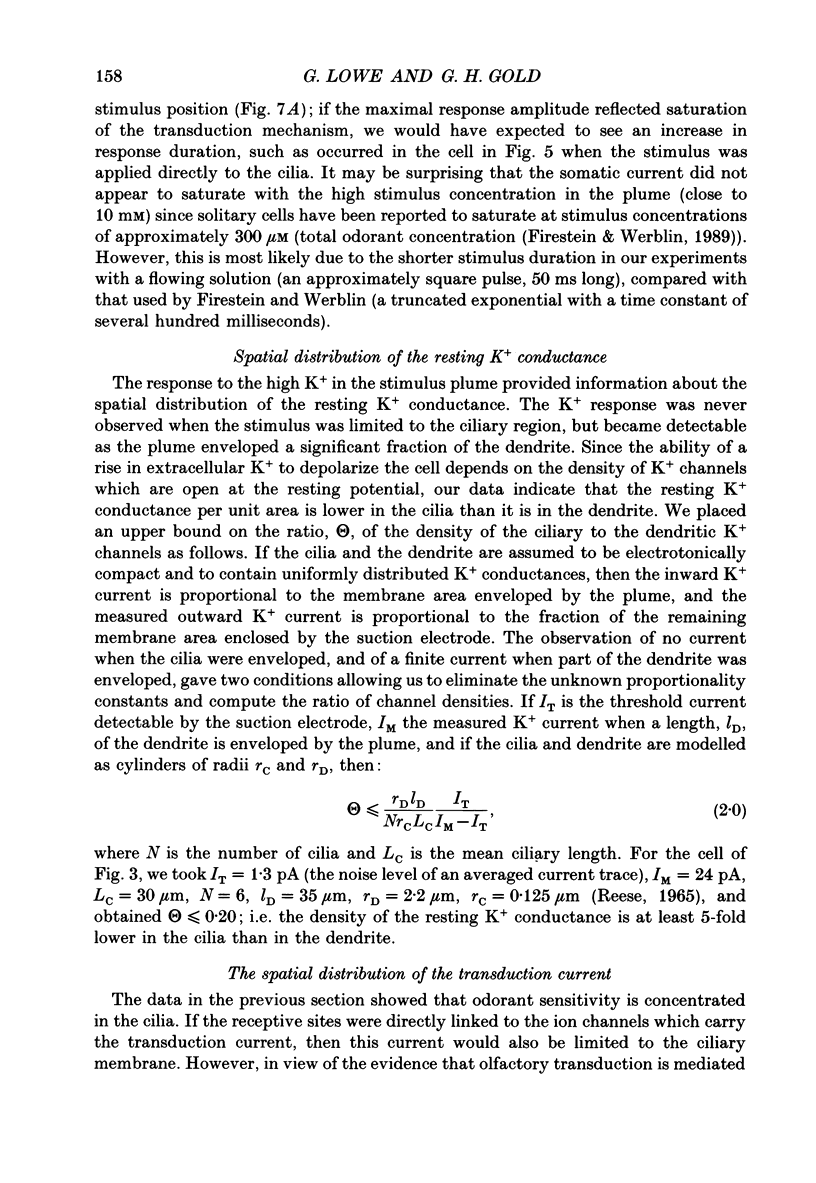
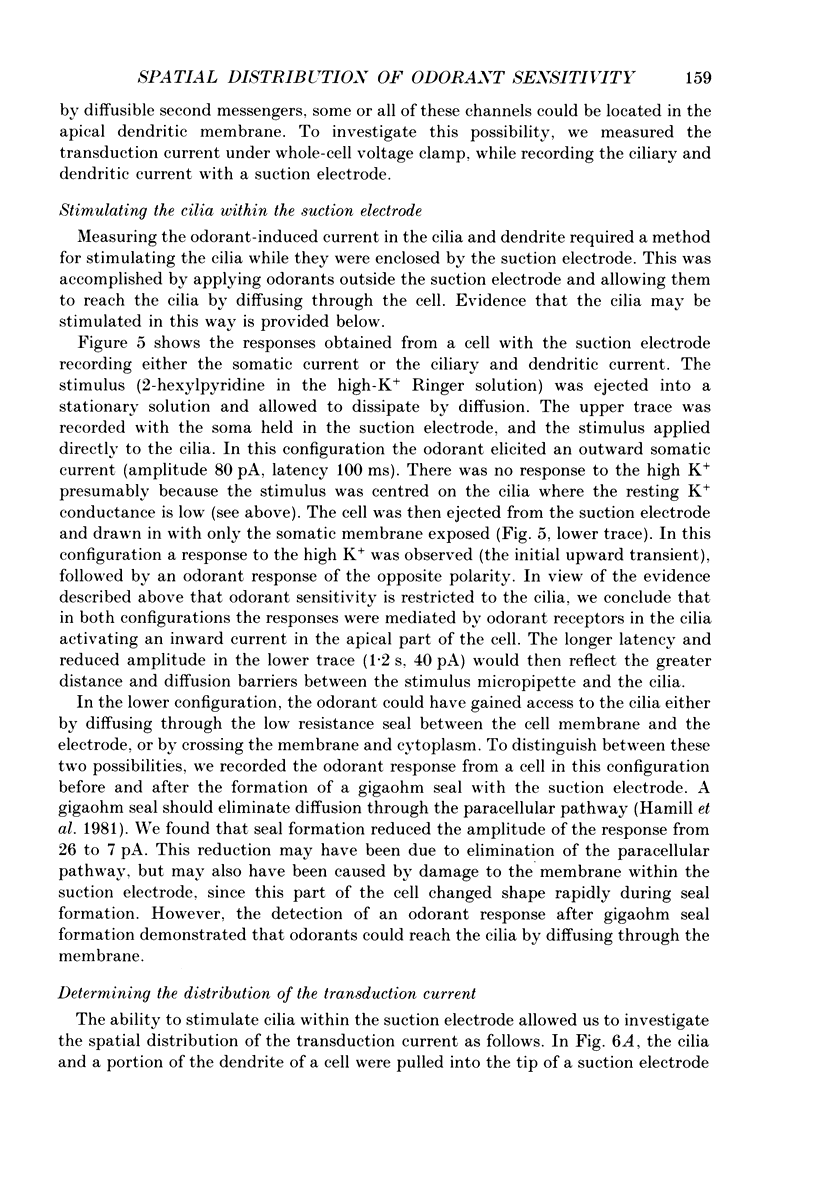
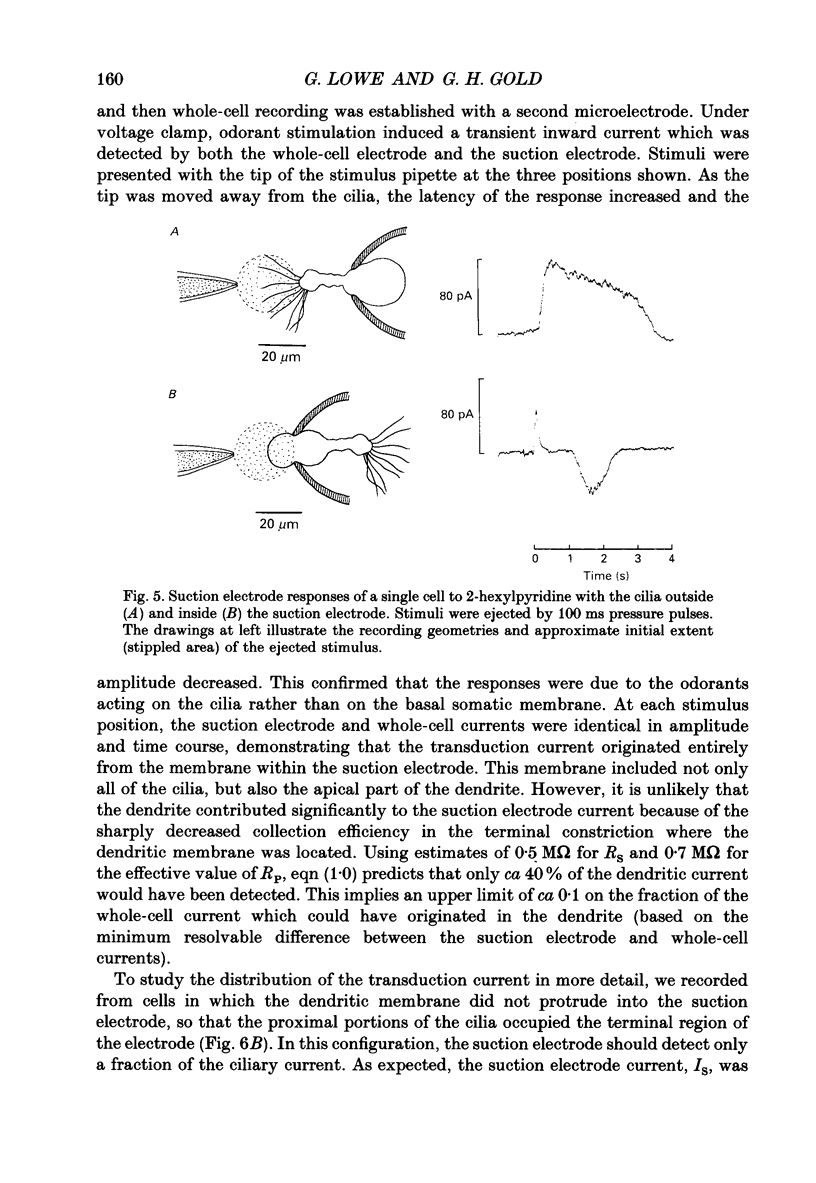
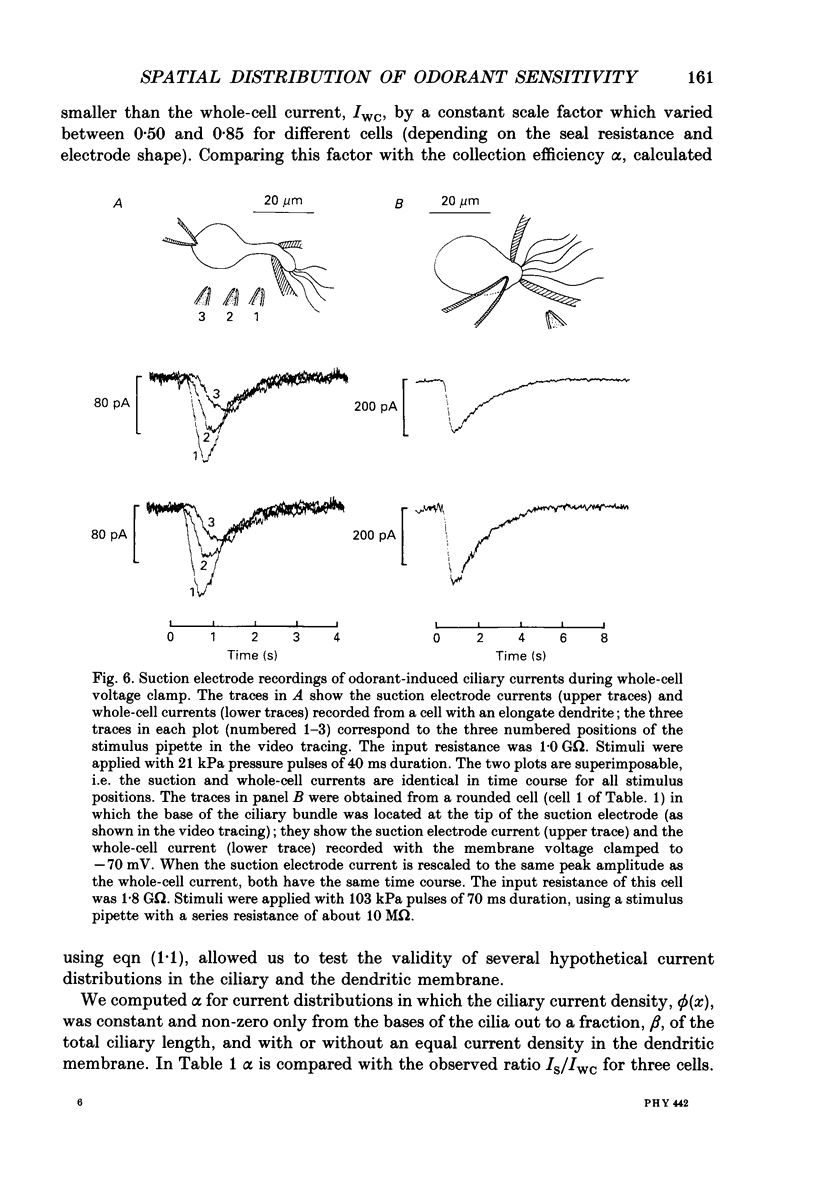
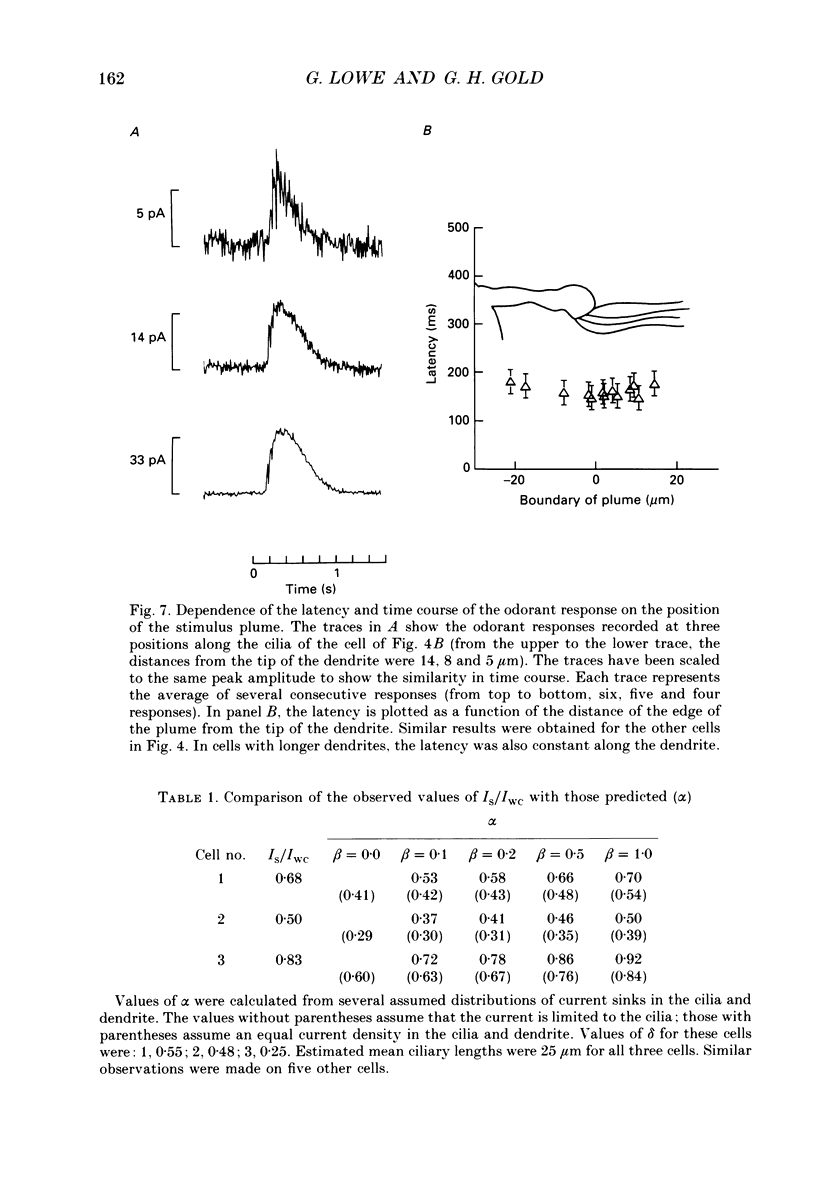
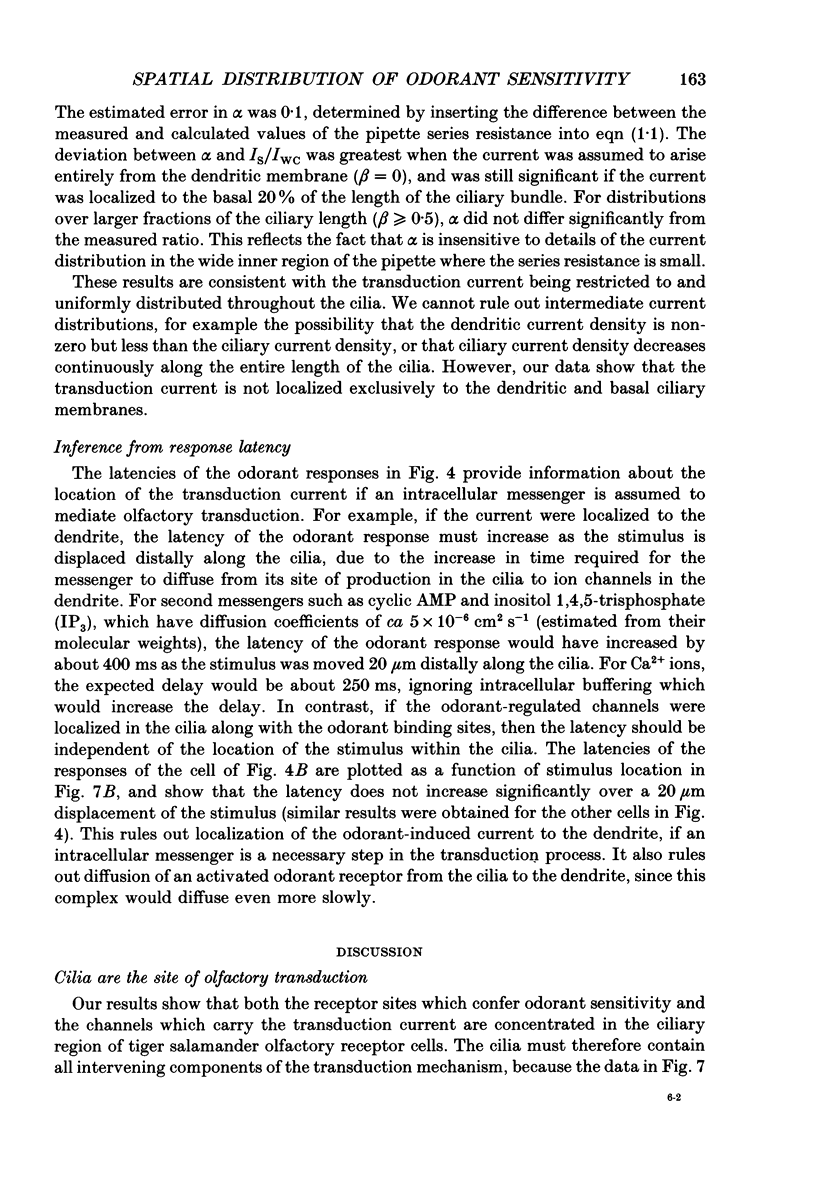
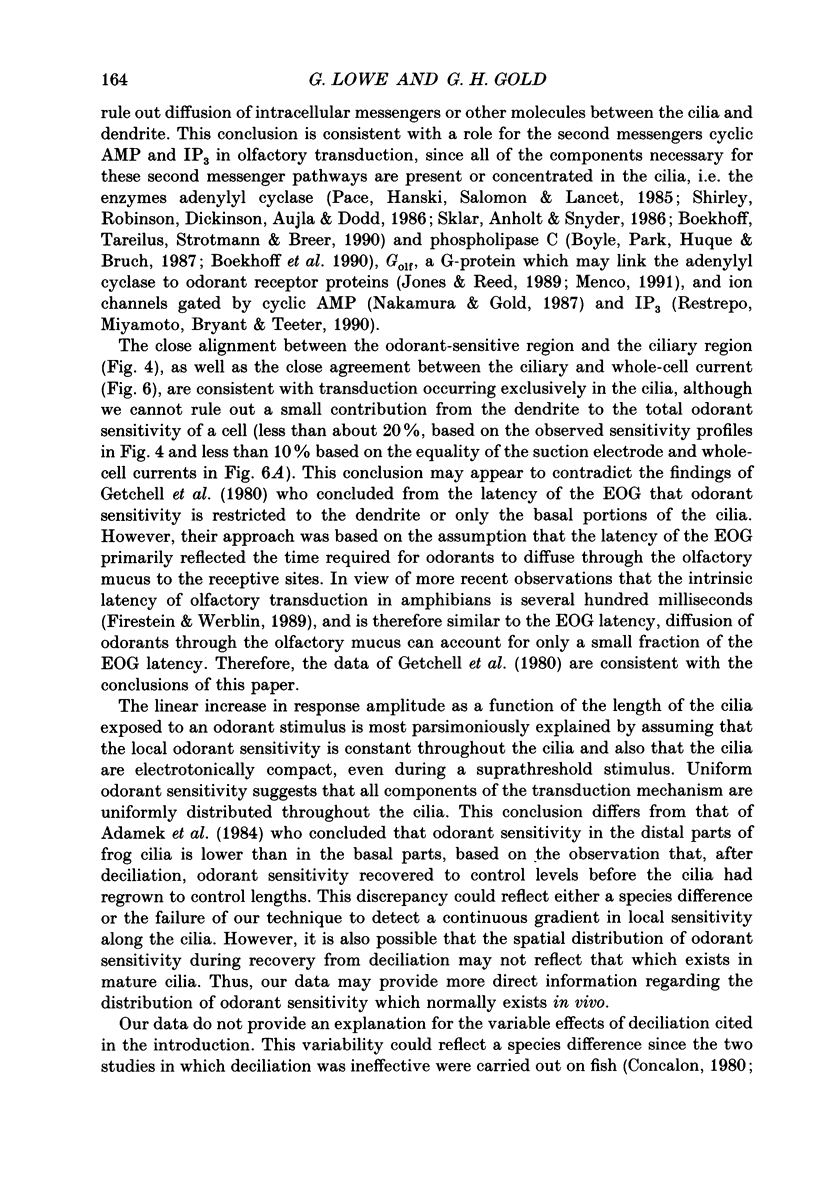
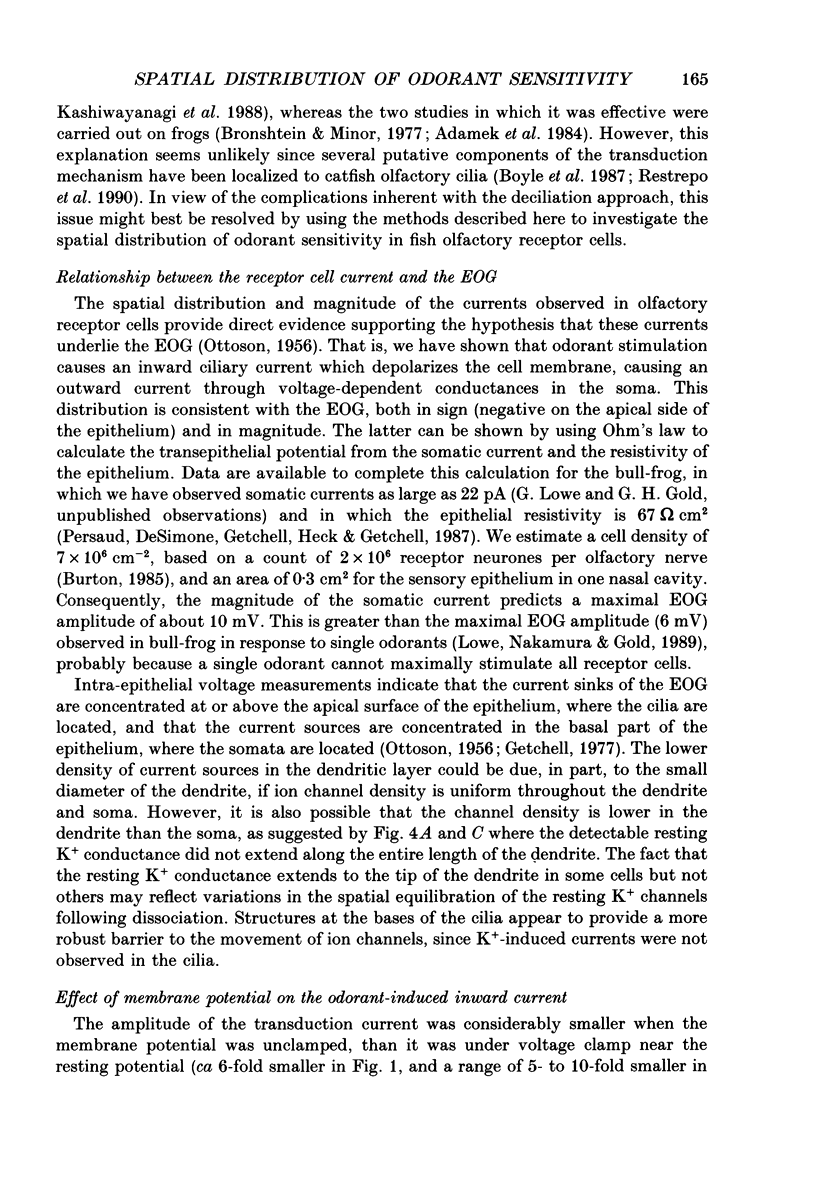
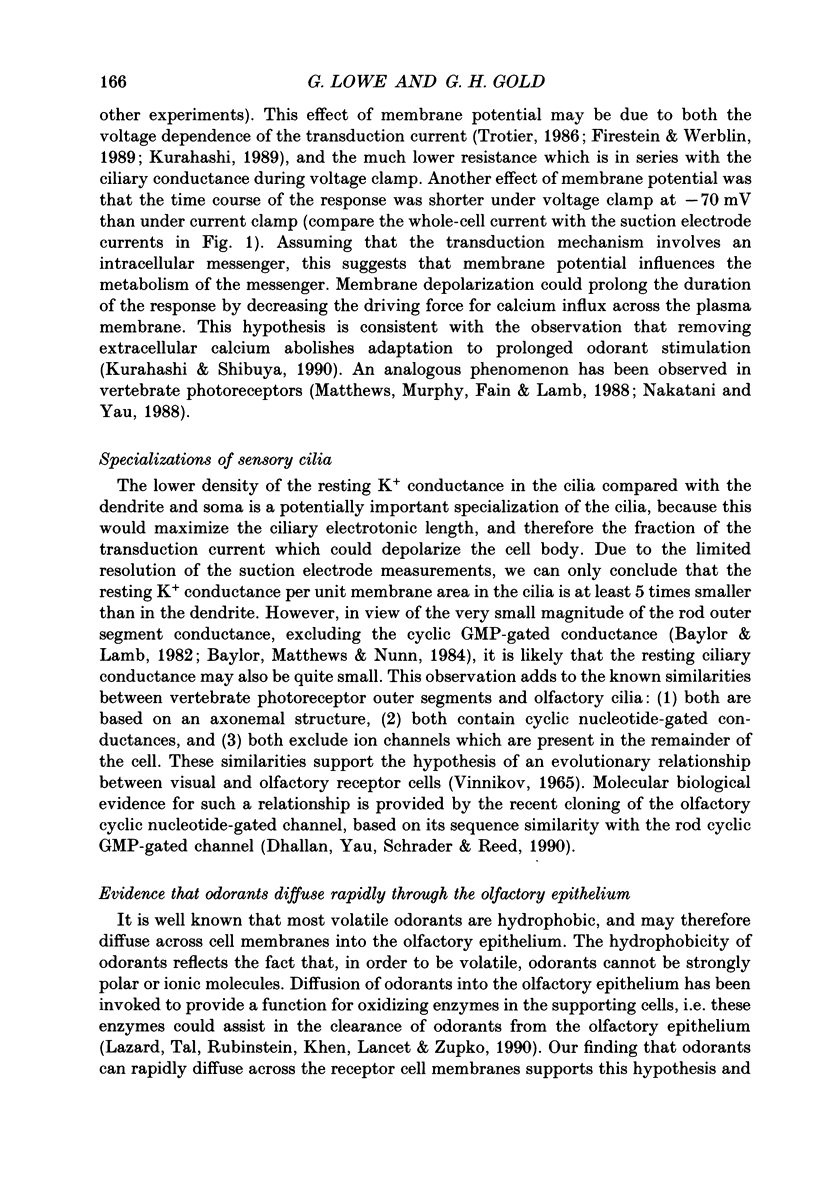
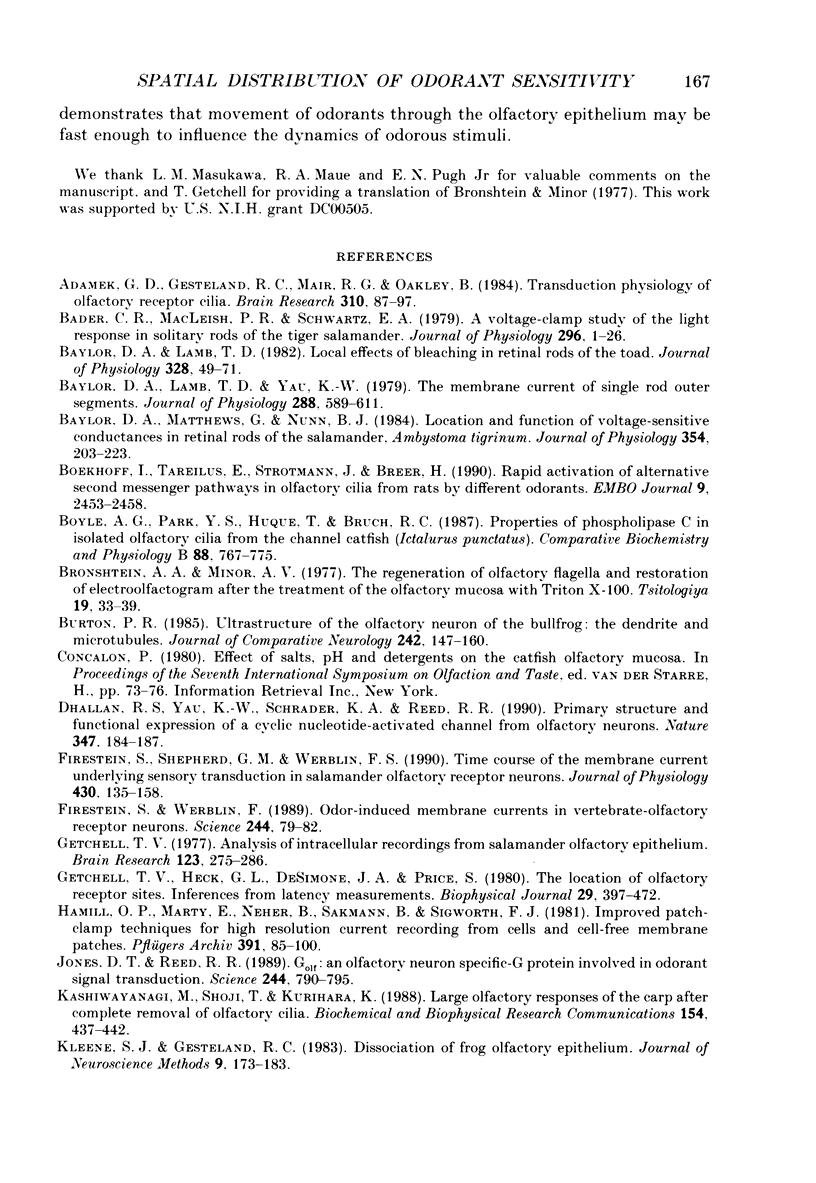
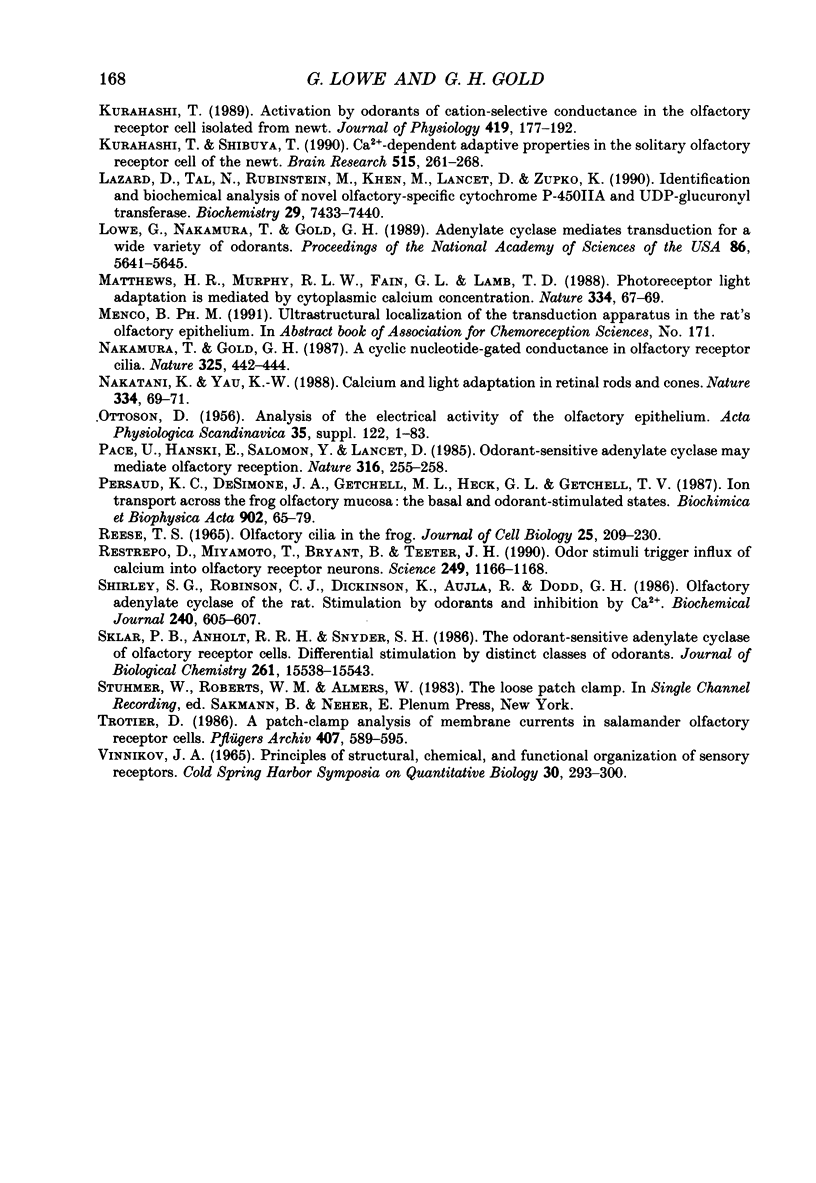
Images in this article
Selected References
These references are in PubMed. This may not be the complete list of references from this article.
- Adamek G. D., Gesteland R. C., Mair R. G., Oakley B. Transduction physiology of olfactory receptor cilia. Brain Res. 1984 Sep 17;310(1):87–97. doi: 10.1016/0006-8993(84)90012-x. [DOI] [PubMed] [Google Scholar]
- Bader C. R., Macleish P. R., Schwartz E. A. A voltage-clamp study of the light response in solitary rods of the tiger salamander. J Physiol. 1979 Nov;296:1–26. doi: 10.1113/jphysiol.1979.sp012988. [DOI] [PMC free article] [PubMed] [Google Scholar]
- Baylor D. A., Lamb T. D. Local effects of bleaching in retinal rods of the toad. J Physiol. 1982 Jul;328:49–71. doi: 10.1113/jphysiol.1982.sp014252. [DOI] [PMC free article] [PubMed] [Google Scholar]
- Baylor D. A., Lamb T. D., Yau K. W. The membrane current of single rod outer segments. J Physiol. 1979 Mar;288:589–611. [PMC free article] [PubMed] [Google Scholar]
- Baylor D. A., Matthews G., Nunn B. J. Location and function of voltage-sensitive conductances in retinal rods of the salamander, Ambystoma tigrinum. J Physiol. 1984 Sep;354:203–223. doi: 10.1113/jphysiol.1984.sp015372. [DOI] [PMC free article] [PubMed] [Google Scholar]
- Boekhoff I., Tareilus E., Strotmann J., Breer H. Rapid activation of alternative second messenger pathways in olfactory cilia from rats by different odorants. EMBO J. 1990 Aug;9(8):2453–2458. doi: 10.1002/j.1460-2075.1990.tb07422.x. [DOI] [PMC free article] [PubMed] [Google Scholar]
- Boyle A. G., Park Y. S., Huque T., Bruch R. C. Properties of phospholipase C in isolated olfactory cilia from the channel catfish (Ictalurus punctatus). Comp Biochem Physiol B. 1987;88(3):767–775. doi: 10.1016/0305-0491(87)90242-2. [DOI] [PubMed] [Google Scholar]
- Bronshteín A. A., Minor A. V. Regeneratsiia oboniatel'nykh zhgutikov i vosstanovlenie élektrool'faktogrammy posle deistviia tritona X-100 na oboniatel'nuiu vystilku liagushki. Tsitologiia. 1977;19(1):33–39. [PubMed] [Google Scholar]
- Burton P. R. Ultrastructure of the olfactory neuron of the bullfrog: the dendrite and its microtubules. J Comp Neurol. 1985 Dec 8;242(2):147–160. doi: 10.1002/cne.902420202. [DOI] [PubMed] [Google Scholar]
- Dhallan R. S., Yau K. W., Schrader K. A., Reed R. R. Primary structure and functional expression of a cyclic nucleotide-activated channel from olfactory neurons. Nature. 1990 Sep 13;347(6289):184–187. doi: 10.1038/347184a0. [DOI] [PubMed] [Google Scholar]
- Firestein S., Shepherd G. M., Werblin F. S. Time course of the membrane current underlying sensory transduction in salamander olfactory receptor neurones. J Physiol. 1990 Nov;430:135–158. doi: 10.1113/jphysiol.1990.sp018286. [DOI] [PMC free article] [PubMed] [Google Scholar]
- Firestein S., Werblin F. Odor-induced membrane currents in vertebrate-olfactory receptor neurons. Science. 1989 Apr 7;244(4900):79–82. doi: 10.1126/science.2704991. [DOI] [PubMed] [Google Scholar]
- Getchell T. V. Analysis of intracellular recordings from salamander olfactory epithelium. Brain Res. 1977 Mar 11;123(2):275–286. doi: 10.1016/0006-8993(77)90479-6. [DOI] [PubMed] [Google Scholar]
- Getchell T. V., Heck G. L., DeSimone J. A., Price S. The location of olfactory receptor sites. Inferences from latency measurements. Biophys J. 1980 Mar;29(3):397–411. doi: 10.1016/S0006-3495(80)85142-3. [DOI] [PMC free article] [PubMed] [Google Scholar]
- Hamill O. P., Marty A., Neher E., Sakmann B., Sigworth F. J. Improved patch-clamp techniques for high-resolution current recording from cells and cell-free membrane patches. Pflugers Arch. 1981 Aug;391(2):85–100. doi: 10.1007/BF00656997. [DOI] [PubMed] [Google Scholar]
- Jones D. T., Reed R. R. Golf: an olfactory neuron specific-G protein involved in odorant signal transduction. Science. 1989 May 19;244(4906):790–795. doi: 10.1126/science.2499043. [DOI] [PubMed] [Google Scholar]
- Kashiwayanagi M., Shoji T., Kurihara K. Large olfactory responses of the carp after complete removal of olfactory cilia. Biochem Biophys Res Commun. 1988 Jul 15;154(1):437–442. doi: 10.1016/0006-291x(88)90705-x. [DOI] [PubMed] [Google Scholar]
- Kleene S. J., Gesteland R. C. Dissociation of frog olfactory epithelium. J Neurosci Methods. 1983 Oct;9(2):173–183. doi: 10.1016/0165-0270(83)90130-9. [DOI] [PubMed] [Google Scholar]
- Kurahashi T. Activation by odorants of cation-selective conductance in the olfactory receptor cell isolated from the newt. J Physiol. 1989 Dec;419:177–192. doi: 10.1113/jphysiol.1989.sp017868. [DOI] [PMC free article] [PubMed] [Google Scholar]
- Kurahashi T., Shibuya T. Ca2(+)-dependent adaptive properties in the solitary olfactory receptor cell of the newt. Brain Res. 1990 May 7;515(1-2):261–268. doi: 10.1016/0006-8993(90)90605-b. [DOI] [PubMed] [Google Scholar]
- Lazard D., Tal N., Rubinstein M., Khen M., Lancet D., Zupko K. Identification and biochemical analysis of novel olfactory-specific cytochrome P-450IIA and UDP-glucuronosyl transferase. Biochemistry. 1990 Aug 14;29(32):7433–7440. doi: 10.1021/bi00484a012. [DOI] [PubMed] [Google Scholar]
- Lowe G., Nakamura T., Gold G. H. Adenylate cyclase mediates olfactory transduction for a wide variety of odorants. Proc Natl Acad Sci U S A. 1989 Jul;86(14):5641–5645. doi: 10.1073/pnas.86.14.5641. [DOI] [PMC free article] [PubMed] [Google Scholar]
- Matthews H. R., Murphy R. L., Fain G. L., Lamb T. D. Photoreceptor light adaptation is mediated by cytoplasmic calcium concentration. Nature. 1988 Jul 7;334(6177):67–69. doi: 10.1038/334067a0. [DOI] [PubMed] [Google Scholar]
- Nakamura T., Gold G. H. A cyclic nucleotide-gated conductance in olfactory receptor cilia. 1987 Jan 29-Feb 4Nature. 325(6103):442–444. doi: 10.1038/325442a0. [DOI] [PubMed] [Google Scholar]
- Nakatani K., Yau K. W. Calcium and light adaptation in retinal rods and cones. Nature. 1988 Jul 7;334(6177):69–71. doi: 10.1038/334069a0. [DOI] [PubMed] [Google Scholar]
- OTTOSON D. Analysis of the electrical activity of the olfactory epithelium. Acta Physiol Scand Suppl. 1955;35(122):1–83. [PubMed] [Google Scholar]
- Pace U., Hanski E., Salomon Y., Lancet D. Odorant-sensitive adenylate cyclase may mediate olfactory reception. Nature. 1985 Jul 18;316(6025):255–258. doi: 10.1038/316255a0. [DOI] [PubMed] [Google Scholar]
- Peachey L. D. The sarcoplasmic reticulum and transverse tubules of the frog's sartorius. J Cell Biol. 1965 Jun;25(3 Suppl):209–231. doi: 10.1083/jcb.25.3.209. [DOI] [PubMed] [Google Scholar]
- Persaud K. C., DeSimone J. A., Getchell M. L., Heck G. L., Getchell T. V. Ion transport across the frog olfactory mucosa: the basal and odorant-stimulated states. Biochim Biophys Acta. 1987 Aug 7;902(1):65–79. doi: 10.1016/0005-2736(87)90136-2. [DOI] [PubMed] [Google Scholar]
- Restrepo D., Miyamoto T., Bryant B. P., Teeter J. H. Odor stimuli trigger influx of calcium into olfactory neurons of the channel catfish. Science. 1990 Sep 7;249(4973):1166–1168. doi: 10.1126/science.2168580. [DOI] [PubMed] [Google Scholar]
- Shirley S. G., Robinson C. J., Dickinson K., Aujla R., Dodd G. H. Olfactory adenylate cyclase of the rat. Stimulation by odorants and inhibition by Ca2+. Biochem J. 1986 Dec 1;240(2):605–607. doi: 10.1042/bj2400605. [DOI] [PMC free article] [PubMed] [Google Scholar]
- Sklar P. B., Anholt R. R., Snyder S. H. The odorant-sensitive adenylate cyclase of olfactory receptor cells. Differential stimulation by distinct classes of odorants. J Biol Chem. 1986 Nov 25;261(33):15538–15543. [PubMed] [Google Scholar]
- Trotier D. A patch-clamp analysis of membrane currents in salamander olfactory receptor cells. Pflugers Arch. 1986 Dec;407(6):589–595. doi: 10.1007/BF00582636. [DOI] [PubMed] [Google Scholar]
- Vinnikov J. A. Principles of structural, chemical, and functional organization of sensory receptors. Cold Spring Harb Symp Quant Biol. 1965;30:293–299. doi: 10.1101/sqb.1965.030.01.031. [DOI] [PubMed] [Google Scholar]