Abstract
1. Inward currents elicited by depolarization from holding potentials of -80 to -10 mV in single smooth muscle cells isolated from stomach fundus of the rat and ureter of the guinea-pig had two components. The initial fast component (Ifi) was activated and mostly inactivated within 1-2 and 10 ms, respectively, at 21 degrees C. The following sustained component (Isi) lasted over 50 and 500 ms in fundus and ureter cells, respectively. Ifi was blocked by tetrodotoxin but not affected by 0.5 microM-mu-conotoxin in both types of cells. Isi was abolished by the substitution of extracellular Ca2+ with Mn2+. 2. The sensitivity of Ifis to TTX was markedly different in fundus and ureter cells. The half-inhibition was obtained at 870 and 11 nM, respectively. The amplitude of Ifi was highly dependent on extracellular Na+ concentration in a solution containing 2.2 mM-Mn2+ and 0 mM-Ca2+ in both cells. It is concluded that Ifis in these cells are TTX-sensitive and mu-conotoxin-insensitive Na+ currents. 3. Some of the kinetics of INa measured at 10 degrees C were markedly different in fundus and ureter cells. The current-voltage relationships for Ifi in fundus and ureter cells had peaks at about -10 and 0 mV, respectively. The voltage dependence of the steady-state inactivation of Ifi was also significantly different in these cell types. The half-inactivation voltages were about -74 and -45 mV, respectively. The recovery time course from inactivation in fundus cells was about 10 times slower than that in ureter at -80 mV, where it was 25 ms. 4. The contribution of Ifi to the rising phase of an action potential was examined using TTX under current clamp mode at 21 degrees C. A fast notch-like potential elicited by a subthreshold stimulus for action potential generation was blocked by TTX in both types of cells. Action potentials elicited by a stimulus around threshold were occasionally suppressed by TTX, whereas an action potential was never observed when extracellular Ca2+ was replaced with Mn2+. 5. In conclusion, the existence of at least two types of Na+ channel currents, which were distinguished by their TTX sensitivity and kinetics, was strongly suggested in smooth muscle cells from the rat fundus and the guinea-pig ureter. INa in these cells may have a physiological role to accelerate the generation of an action potential by triggering a rapid activation of ICa, while not being essential for activation of action potentials.
Full text
PDF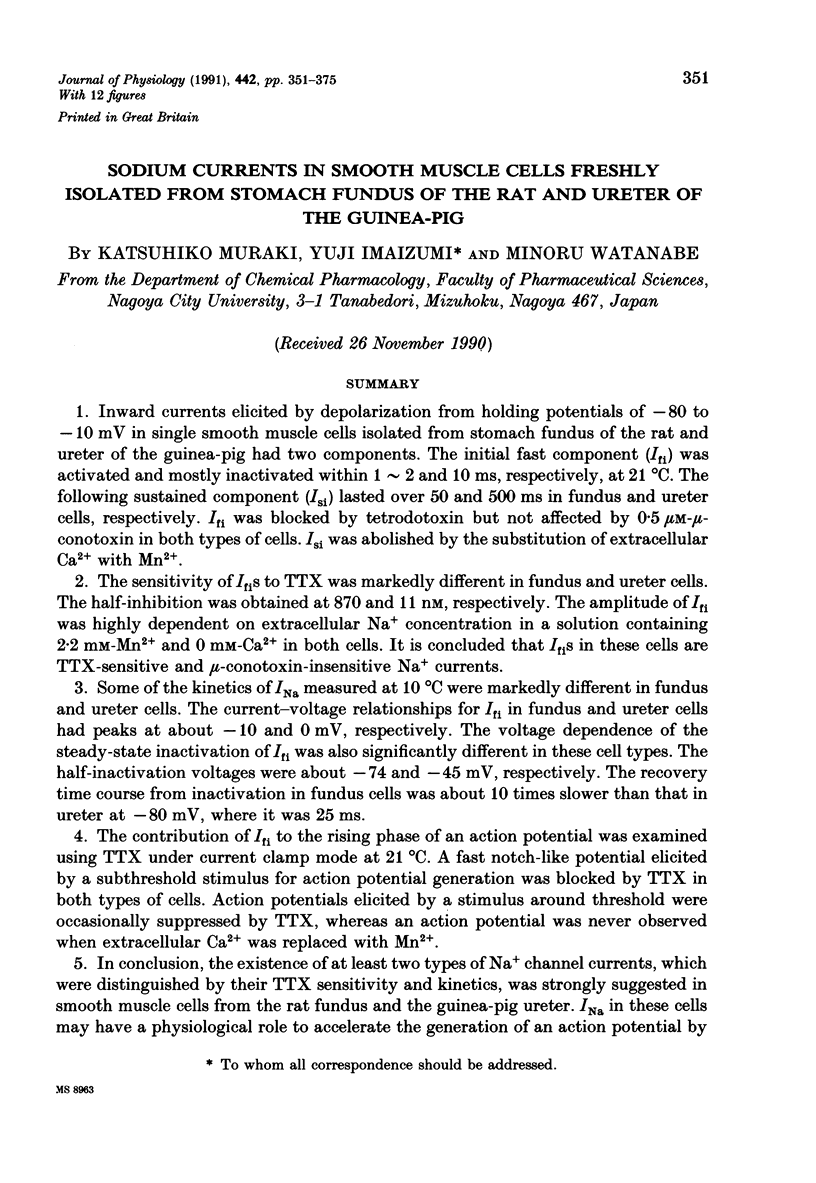
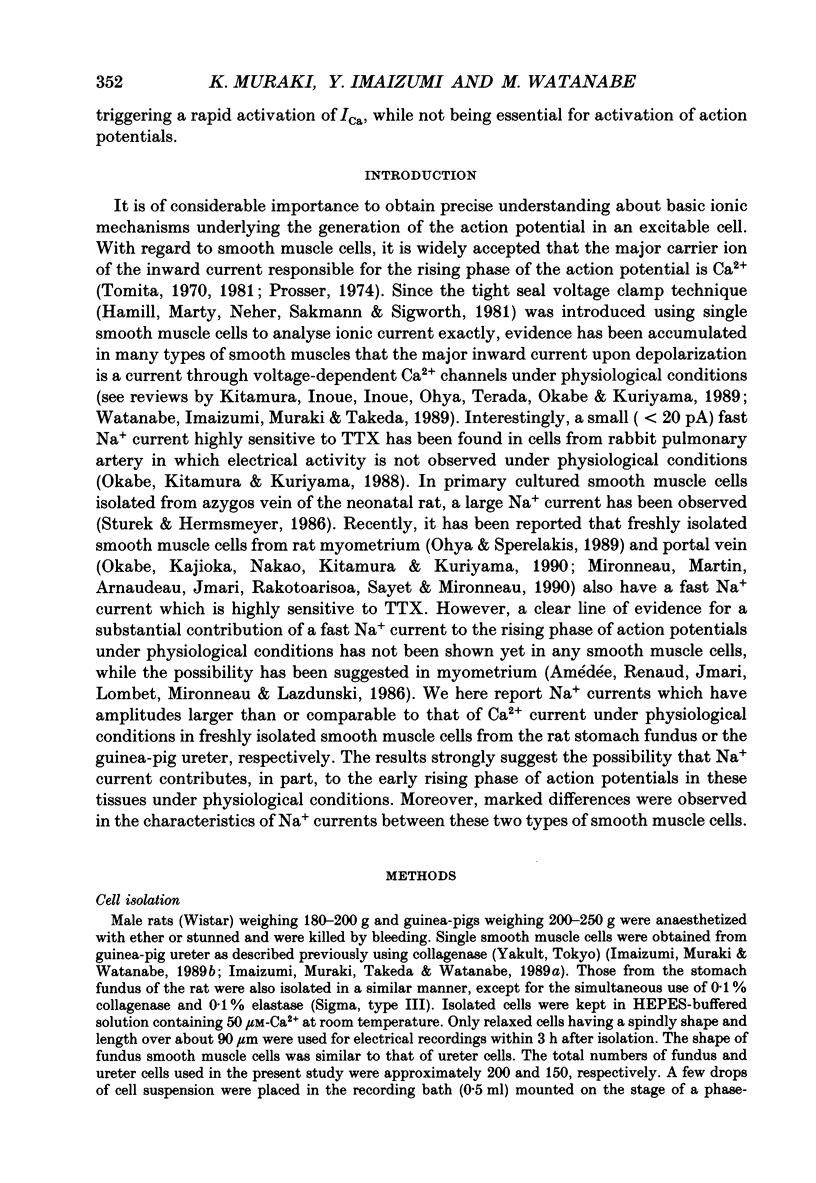
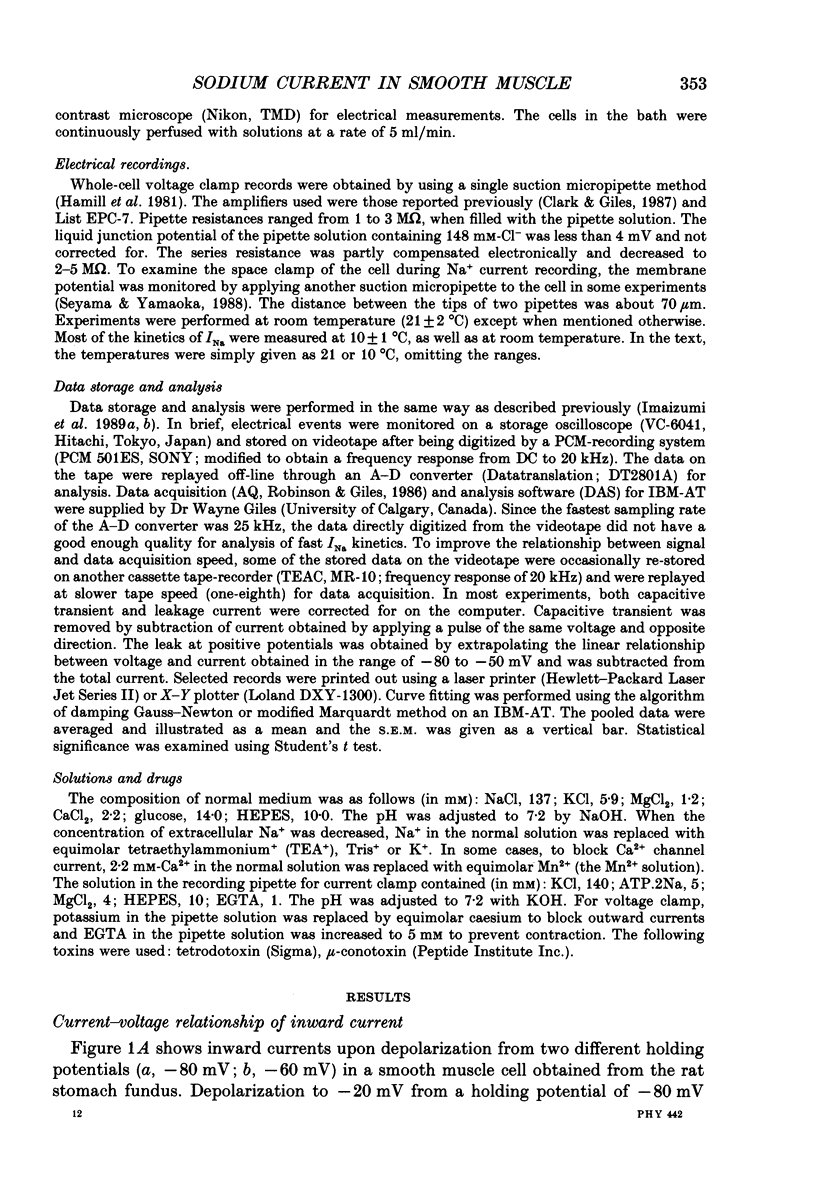
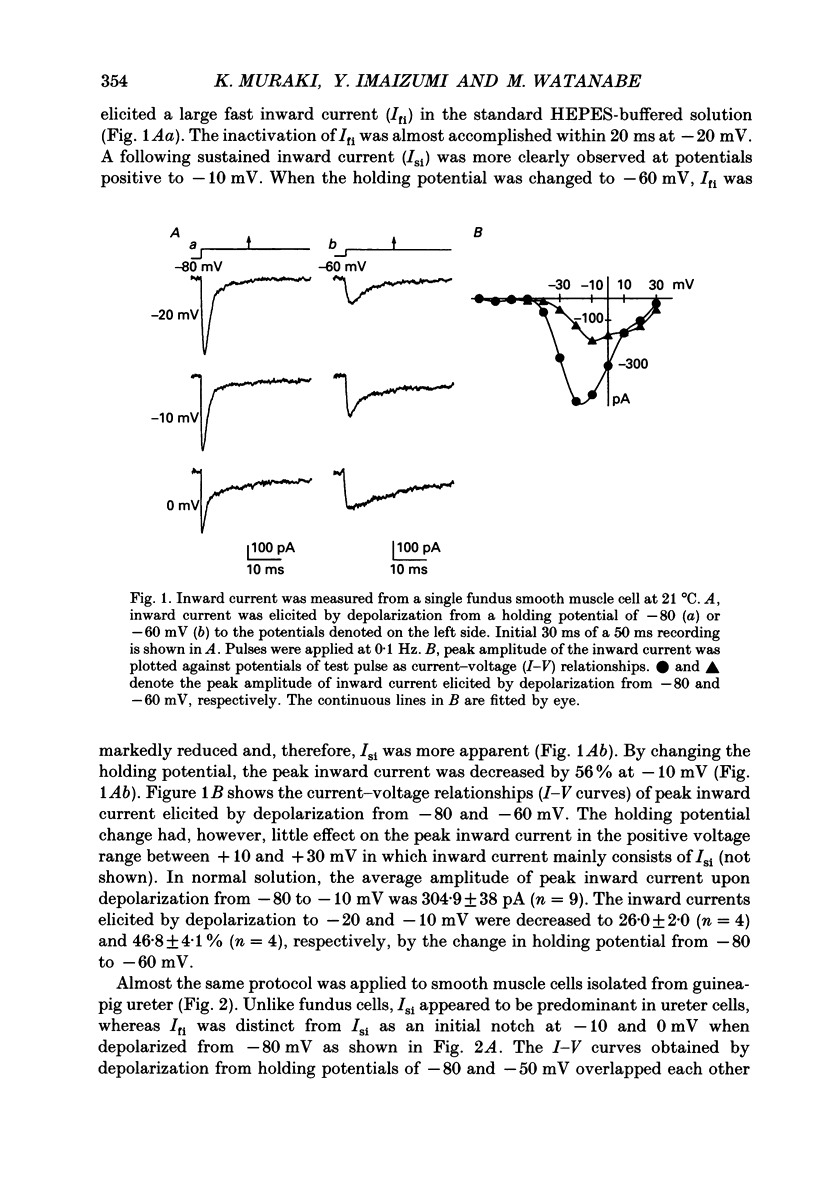
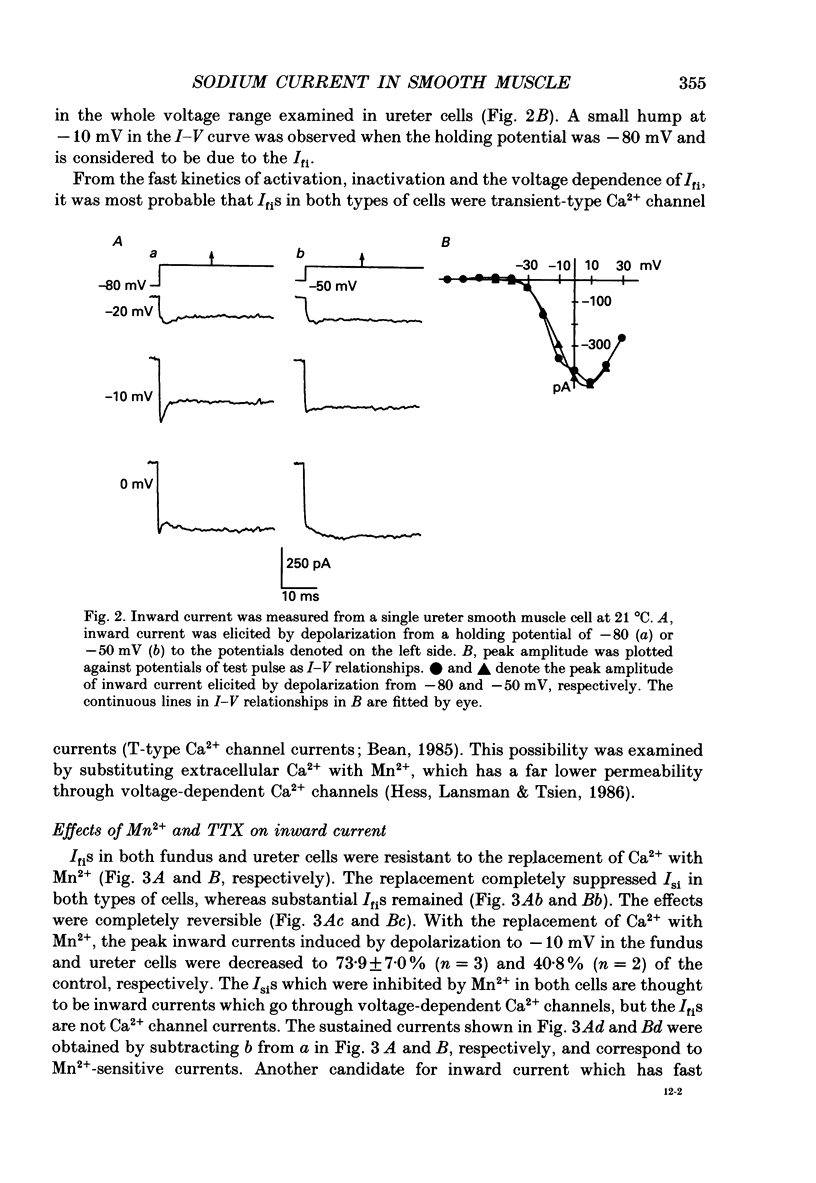
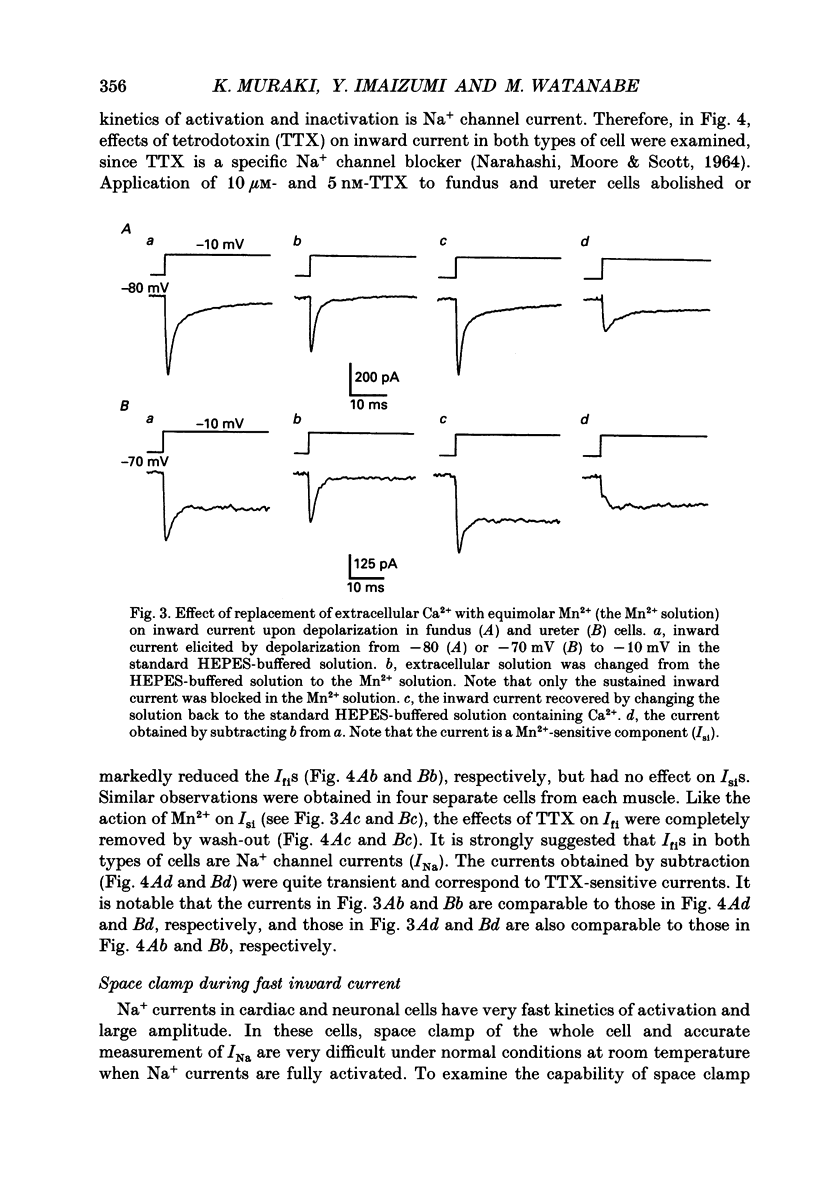
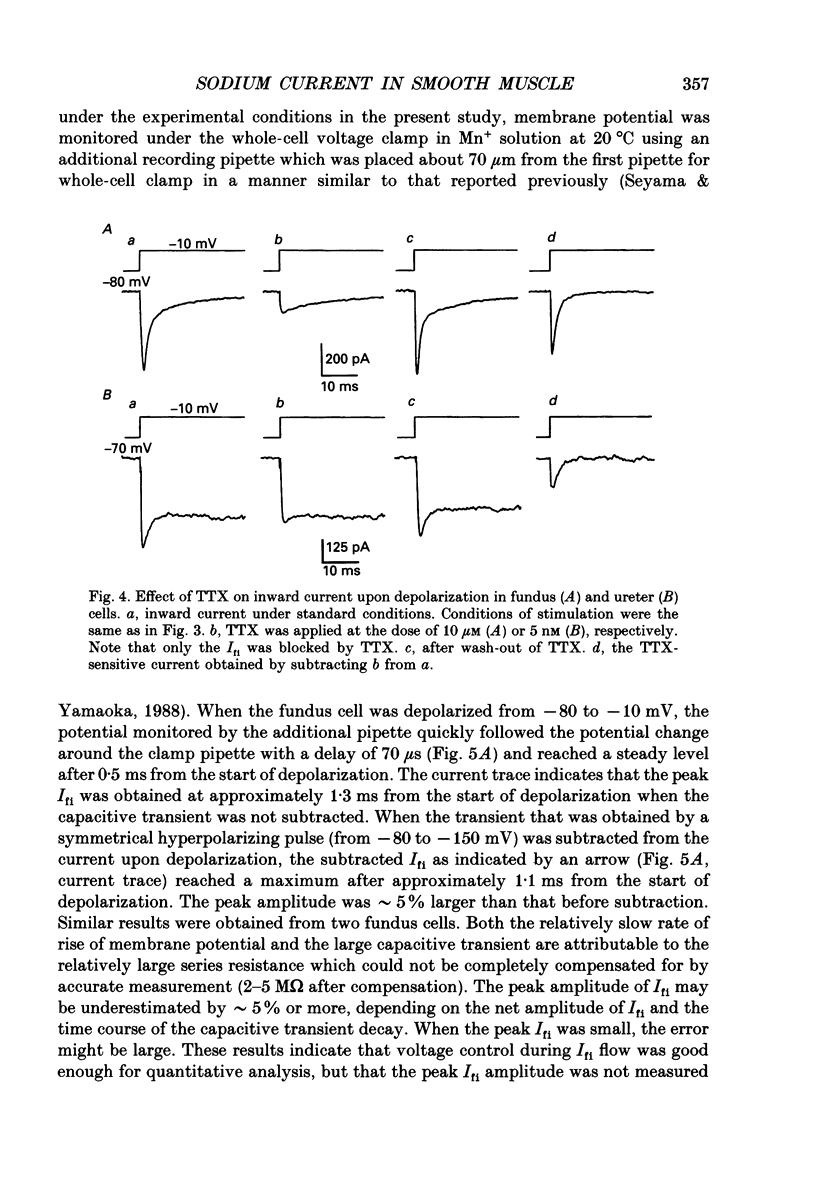
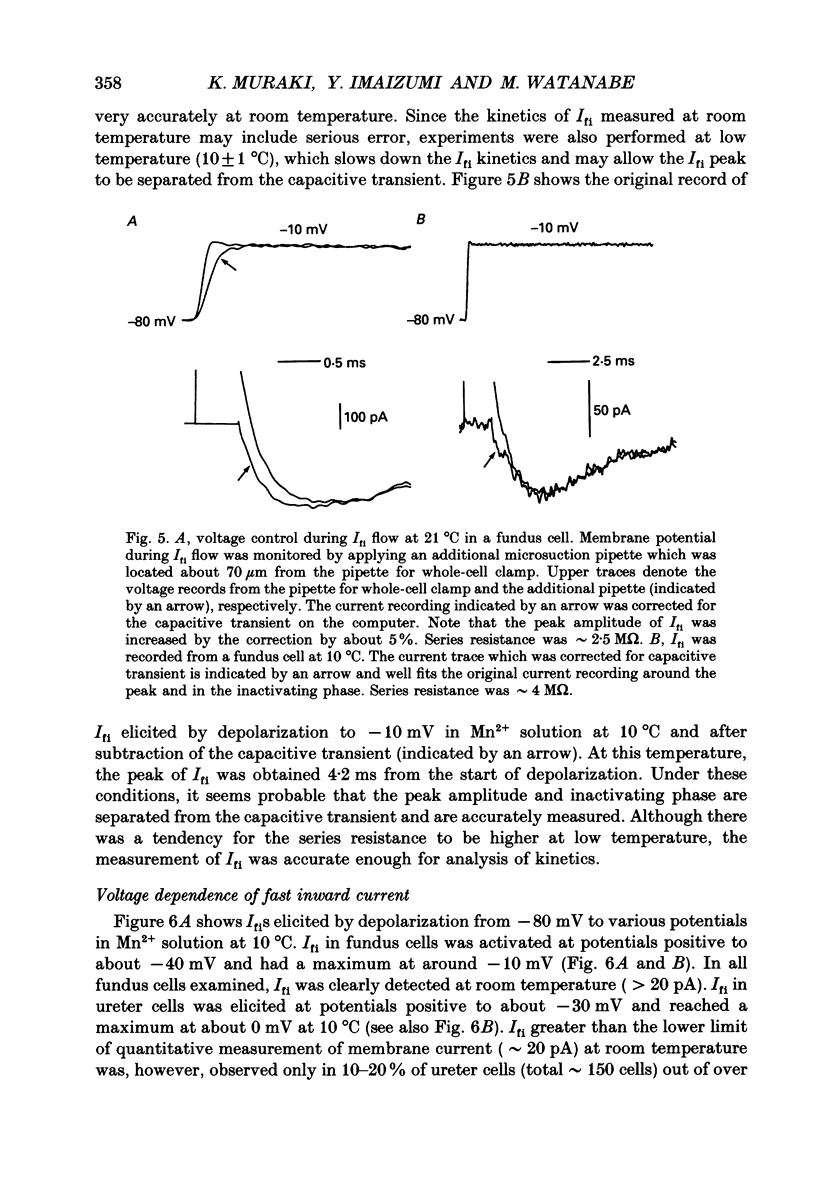
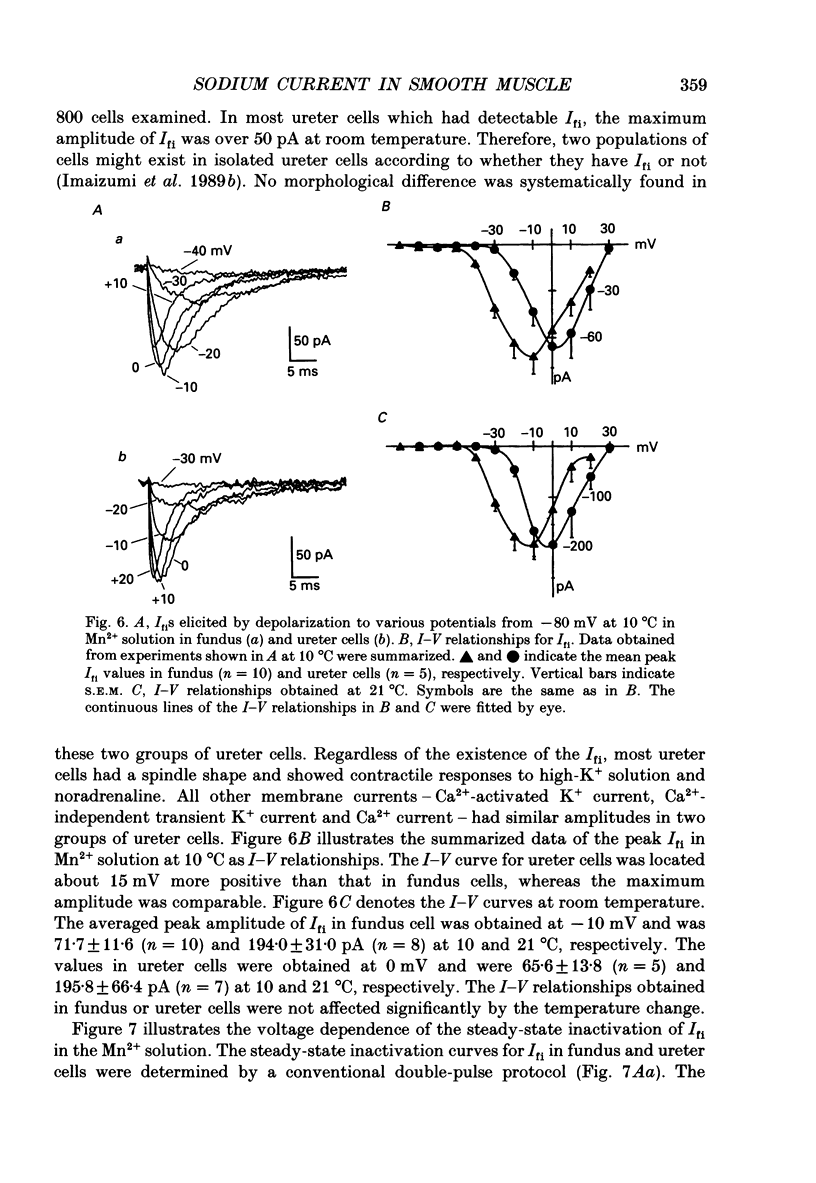
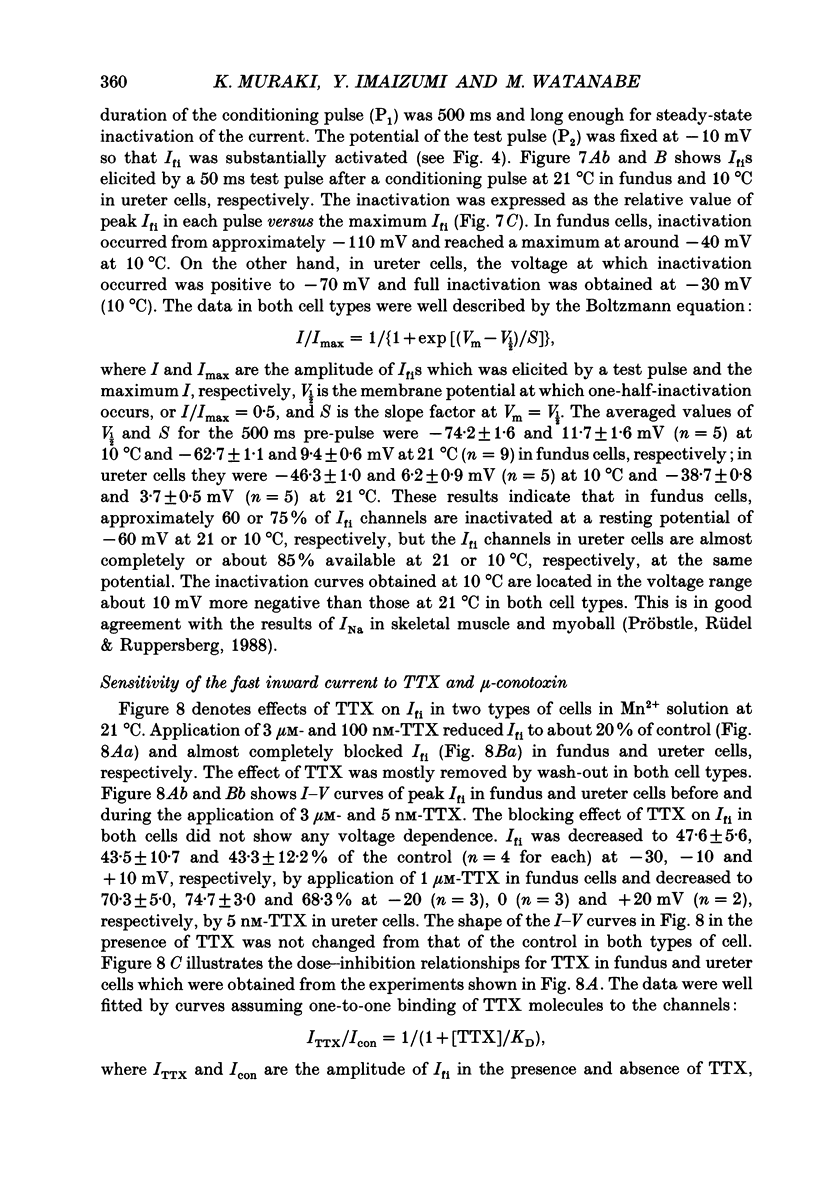
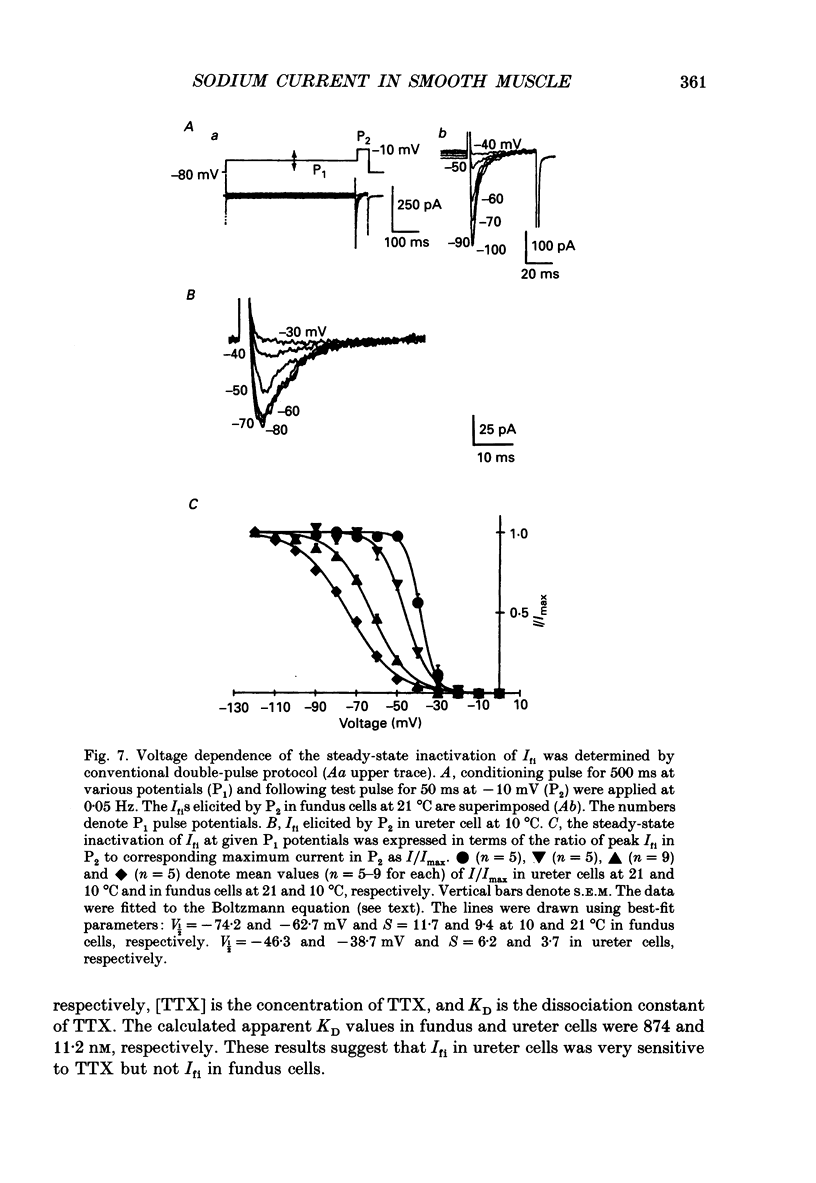
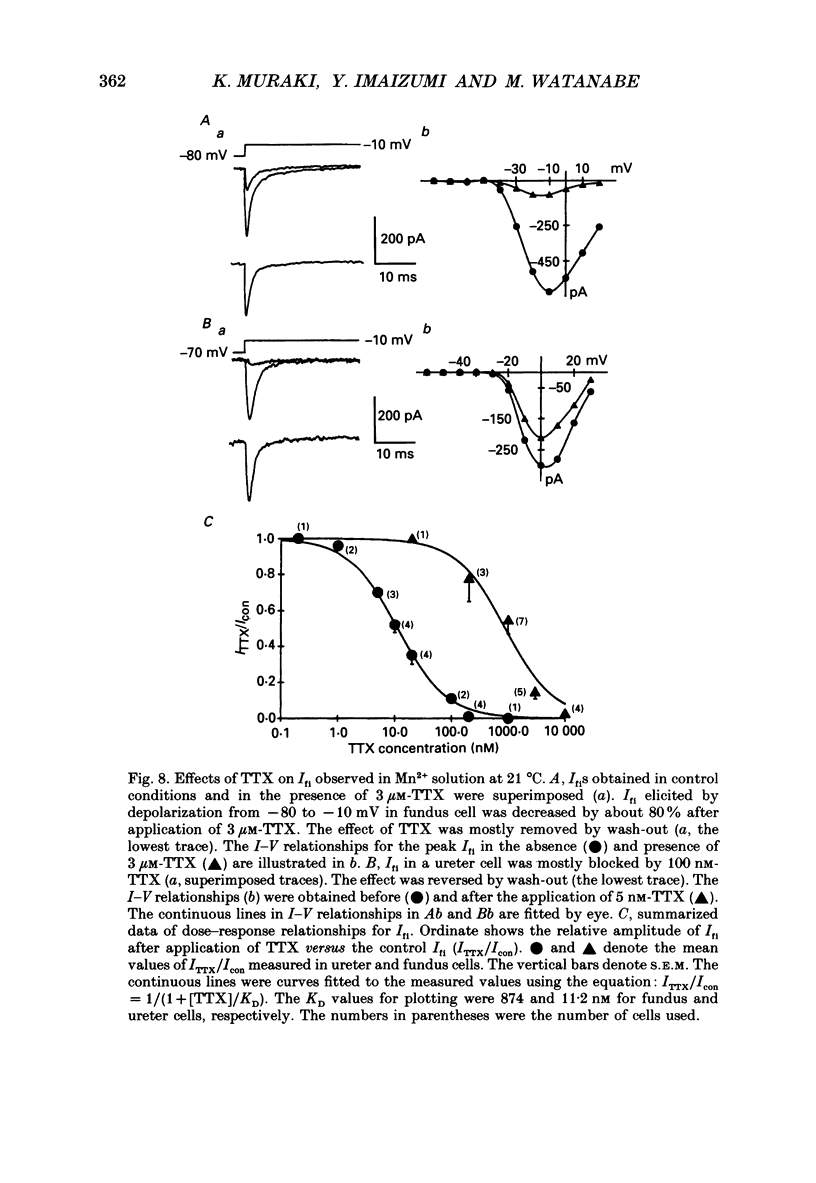
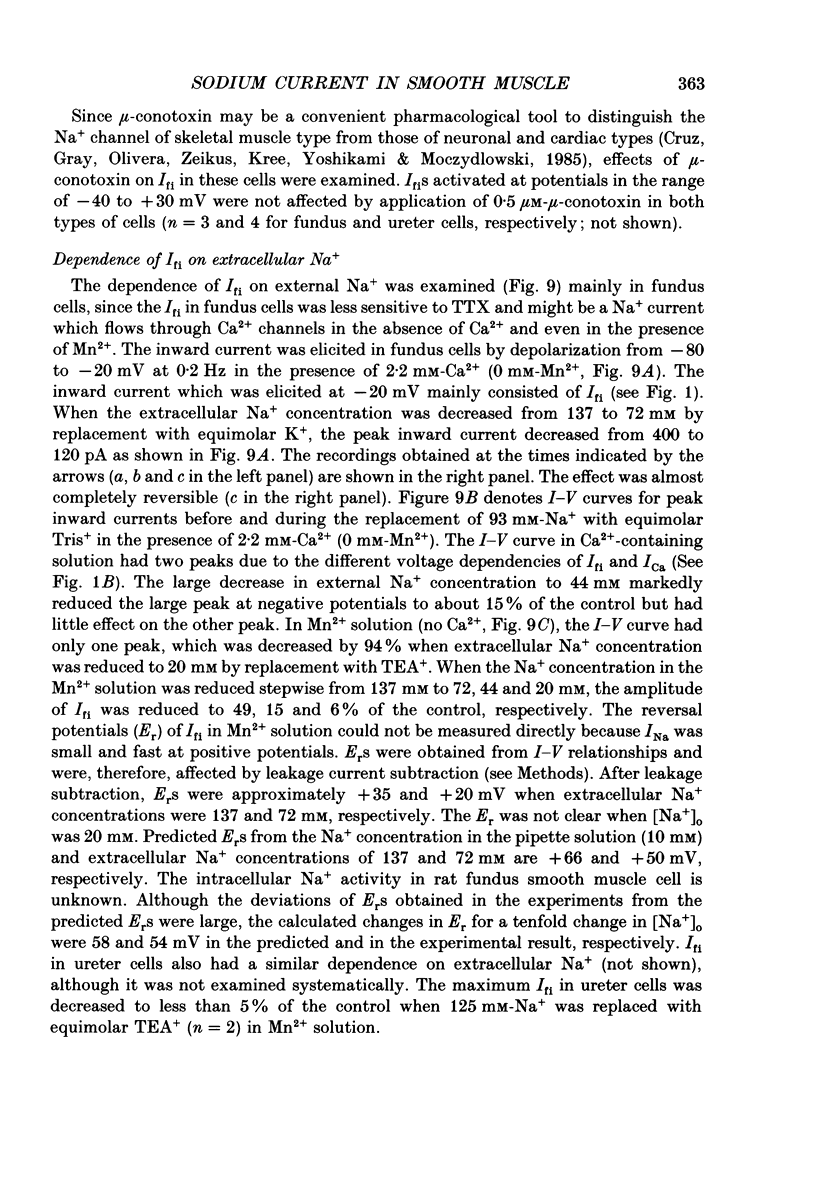
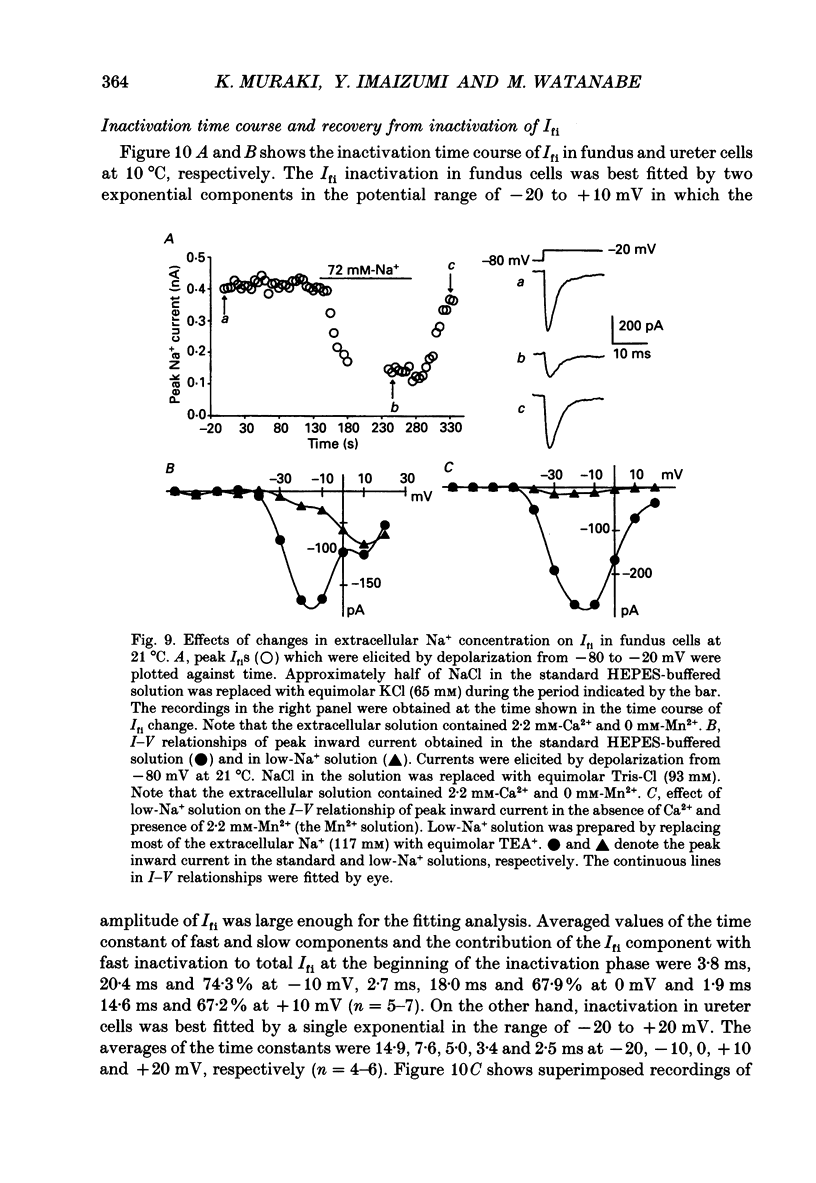
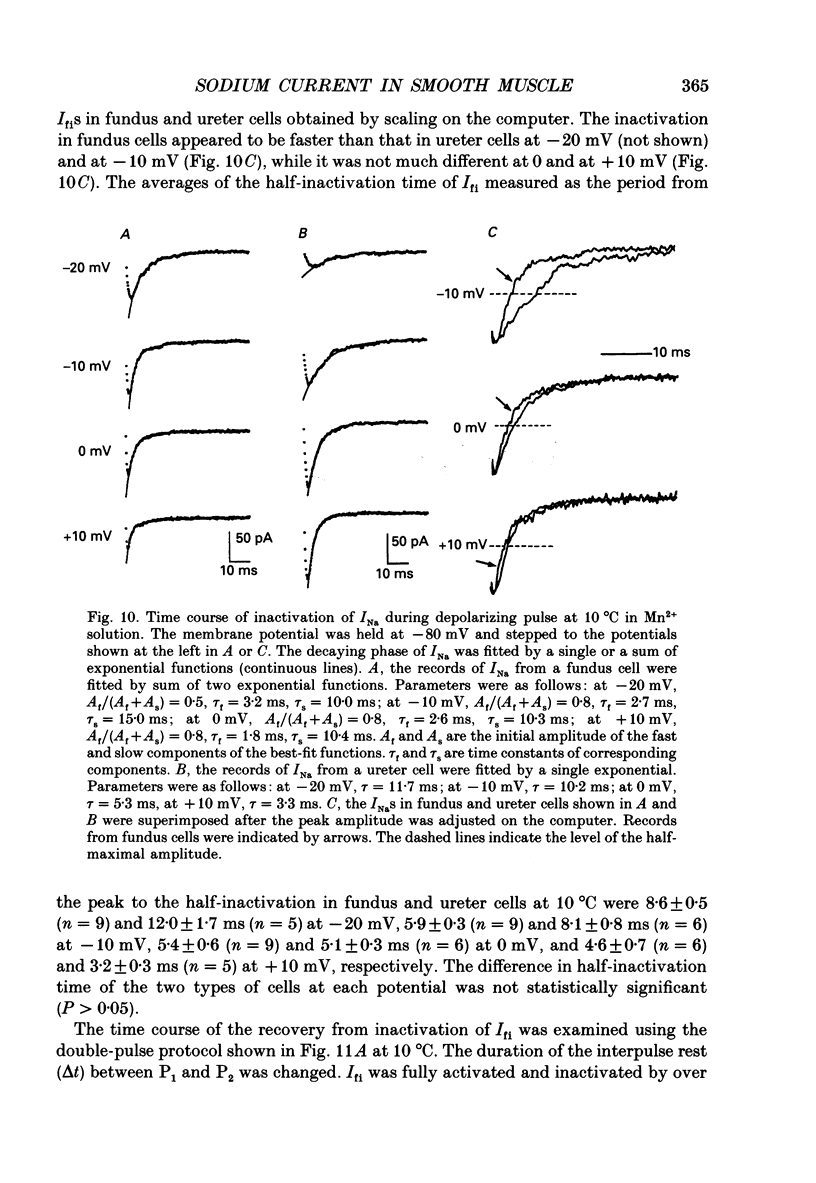
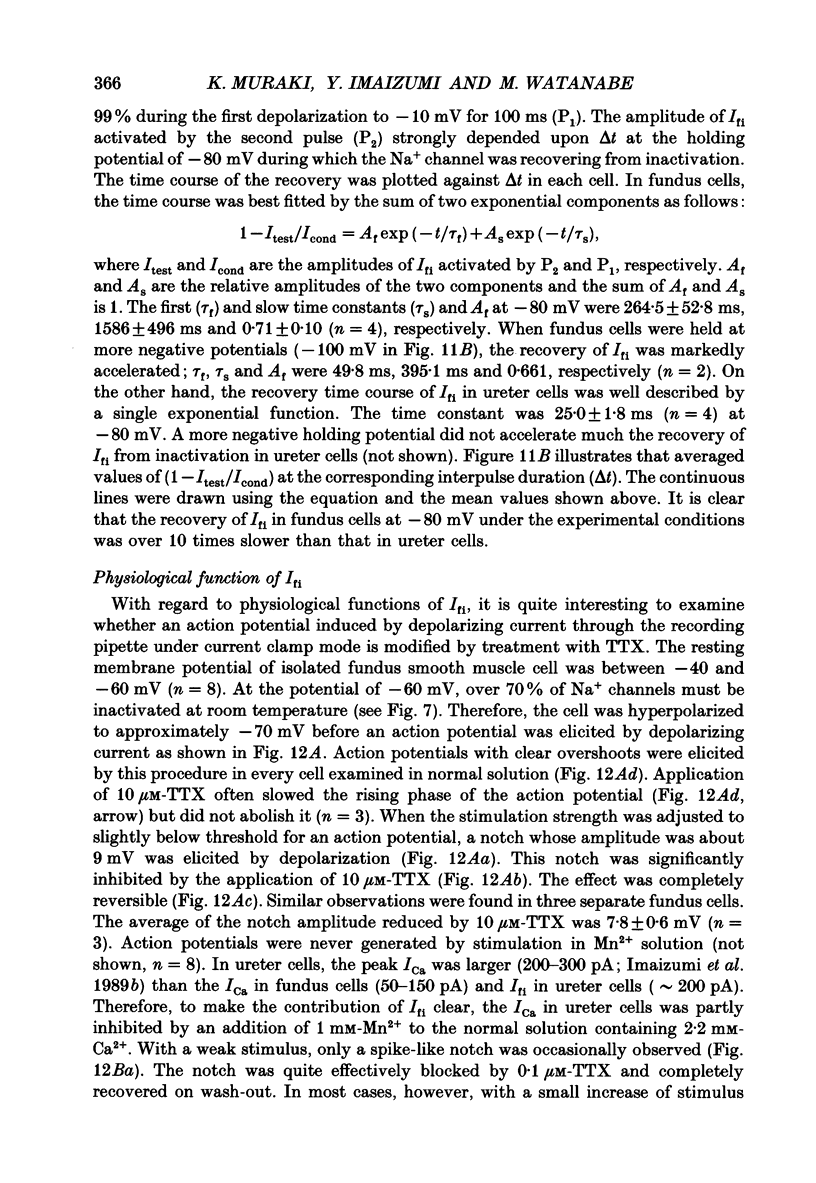
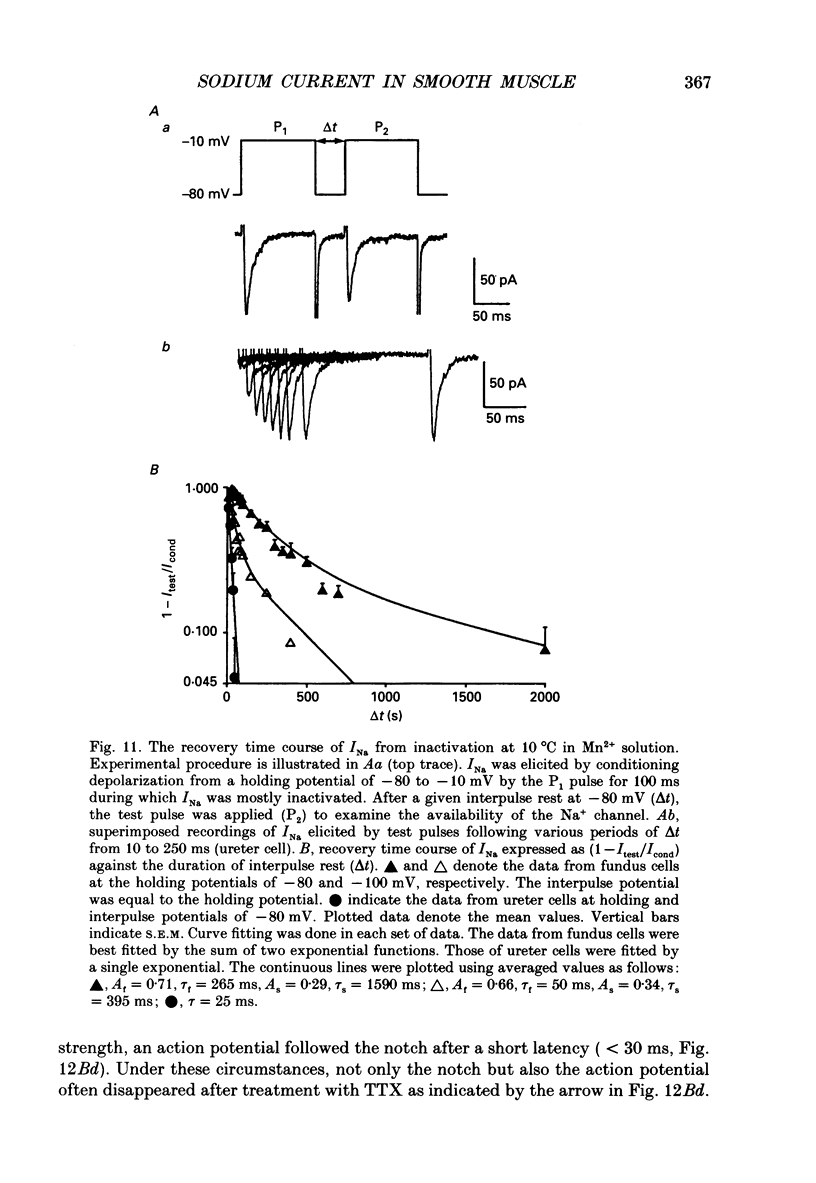
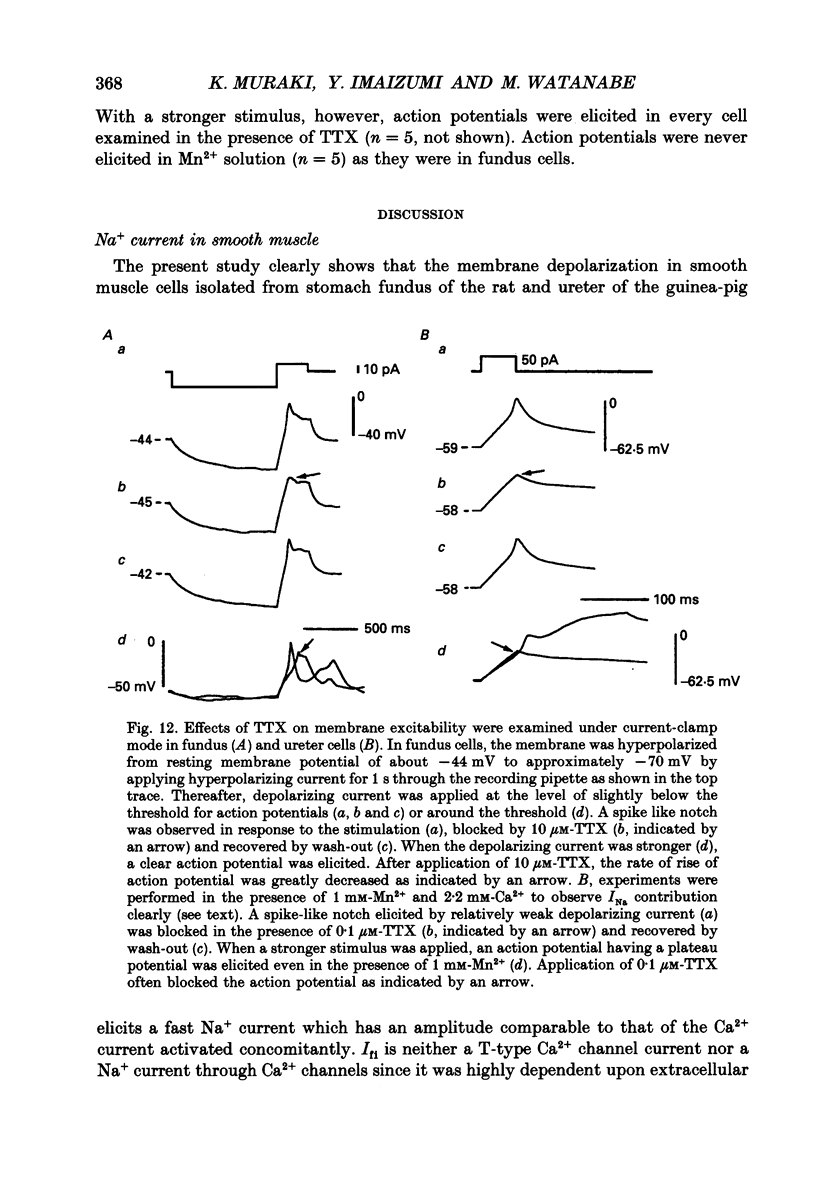
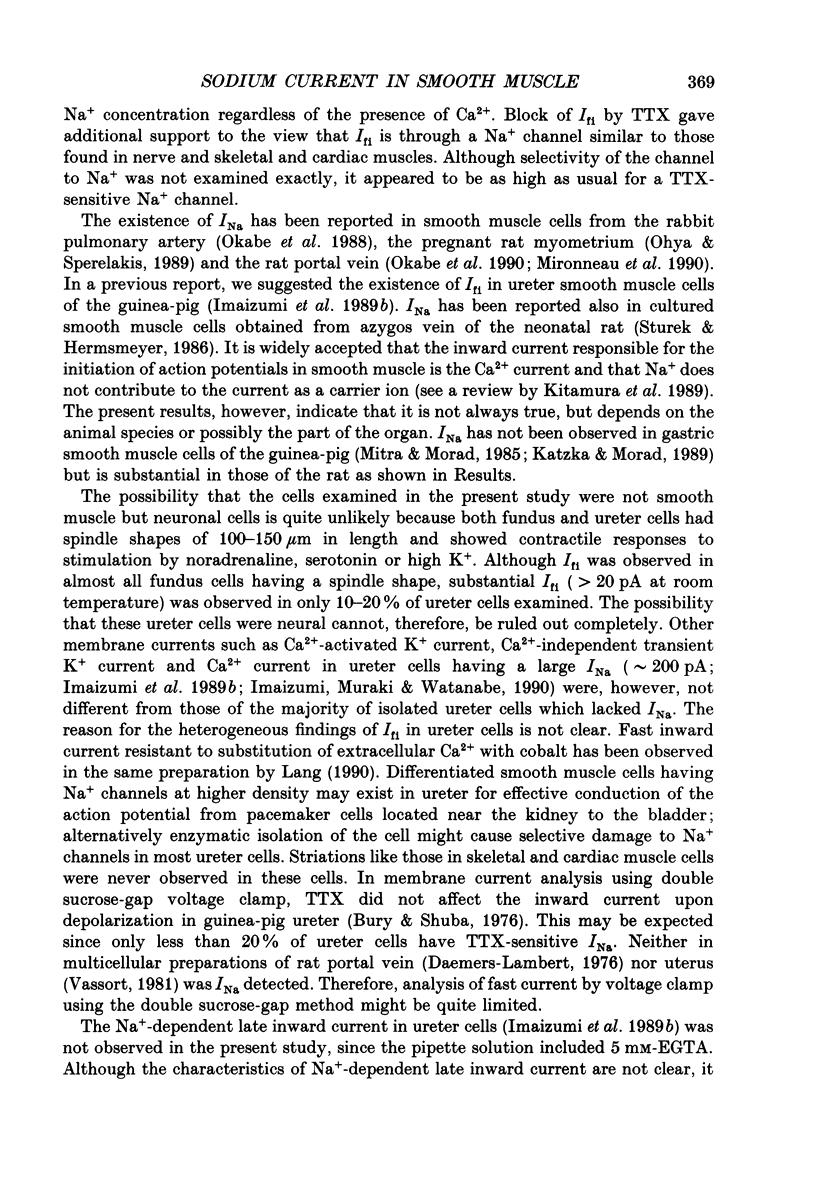
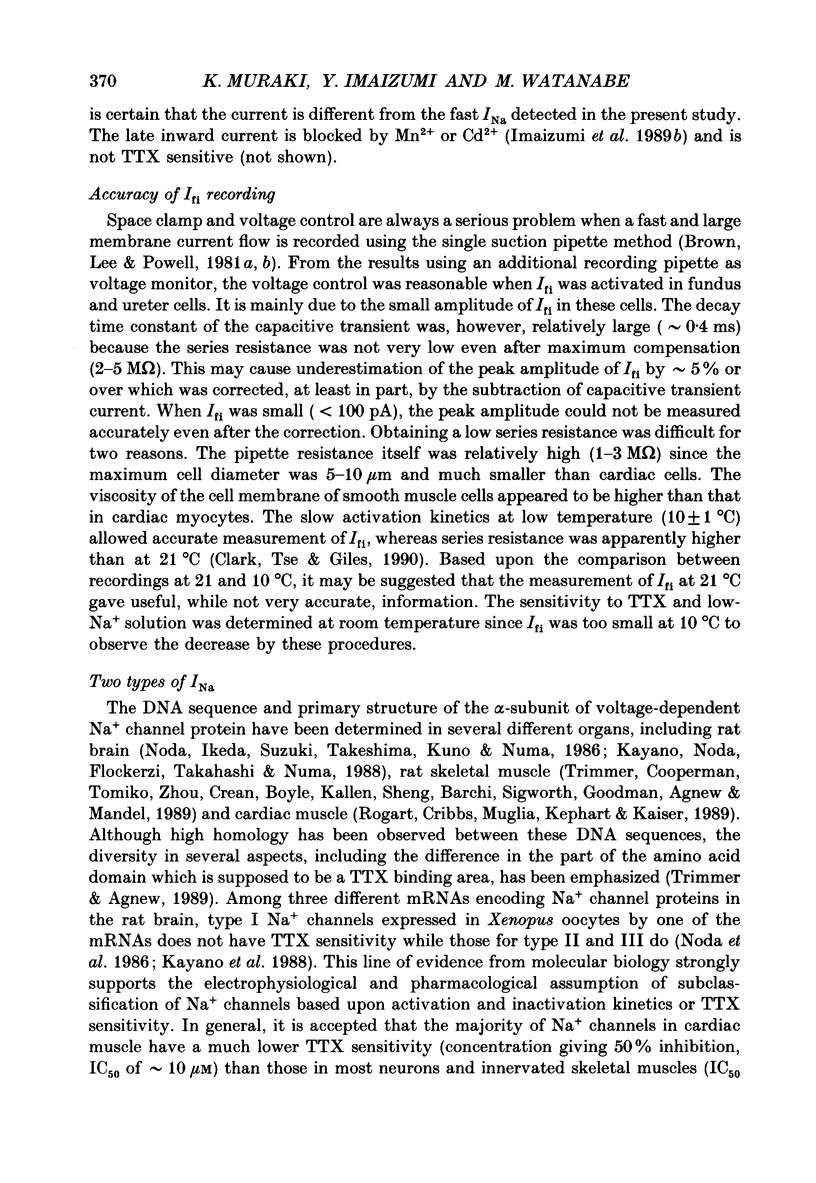
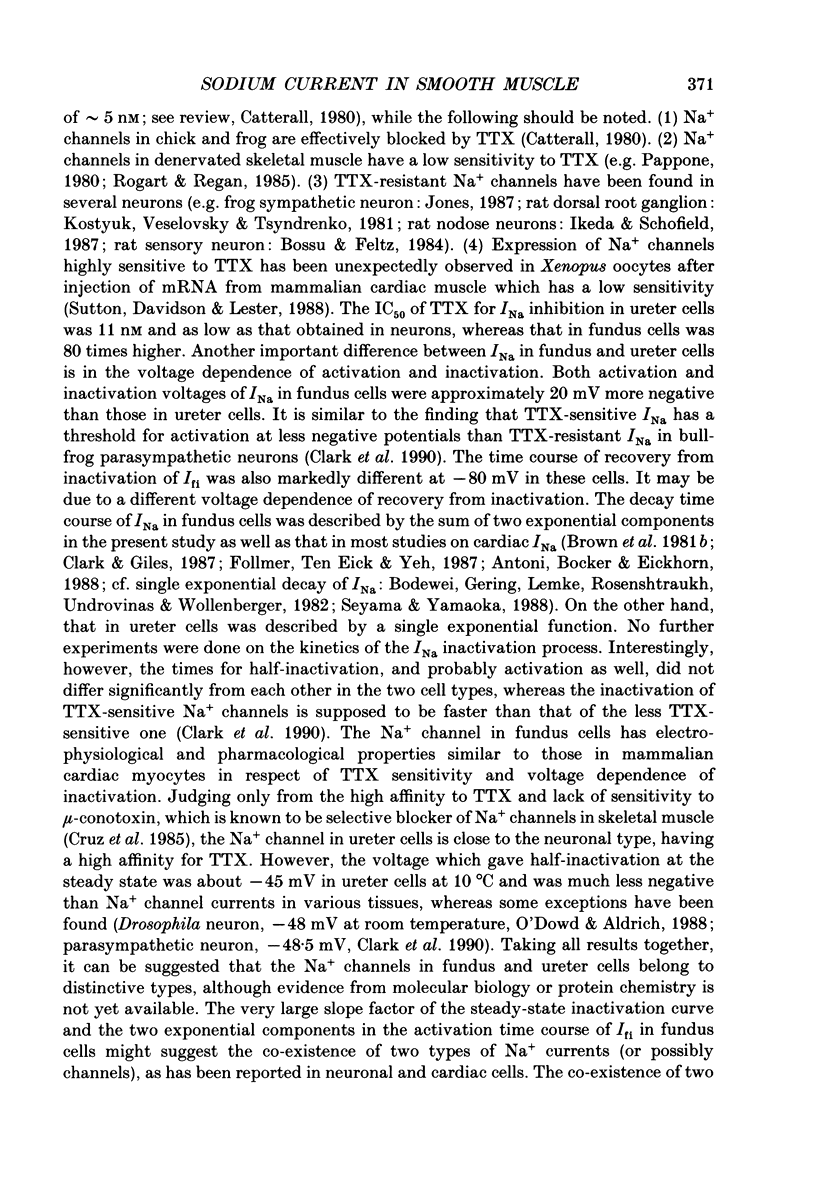
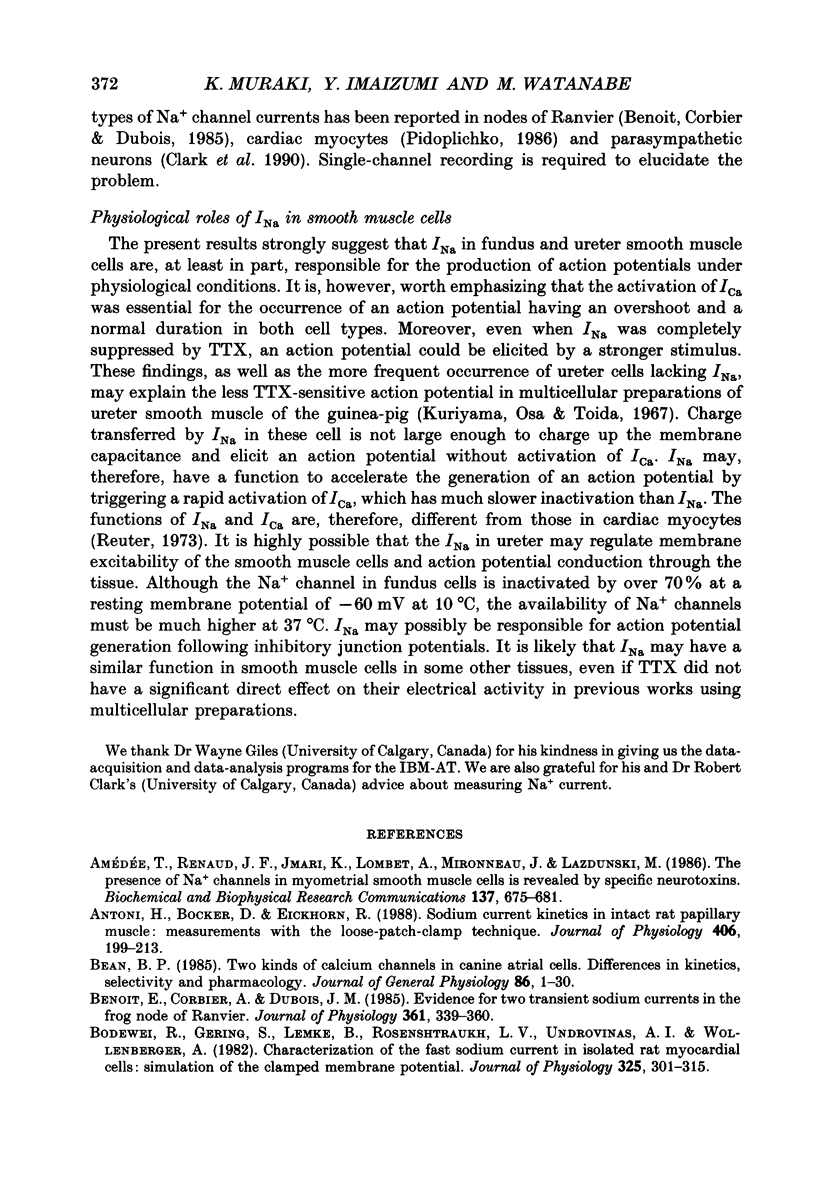
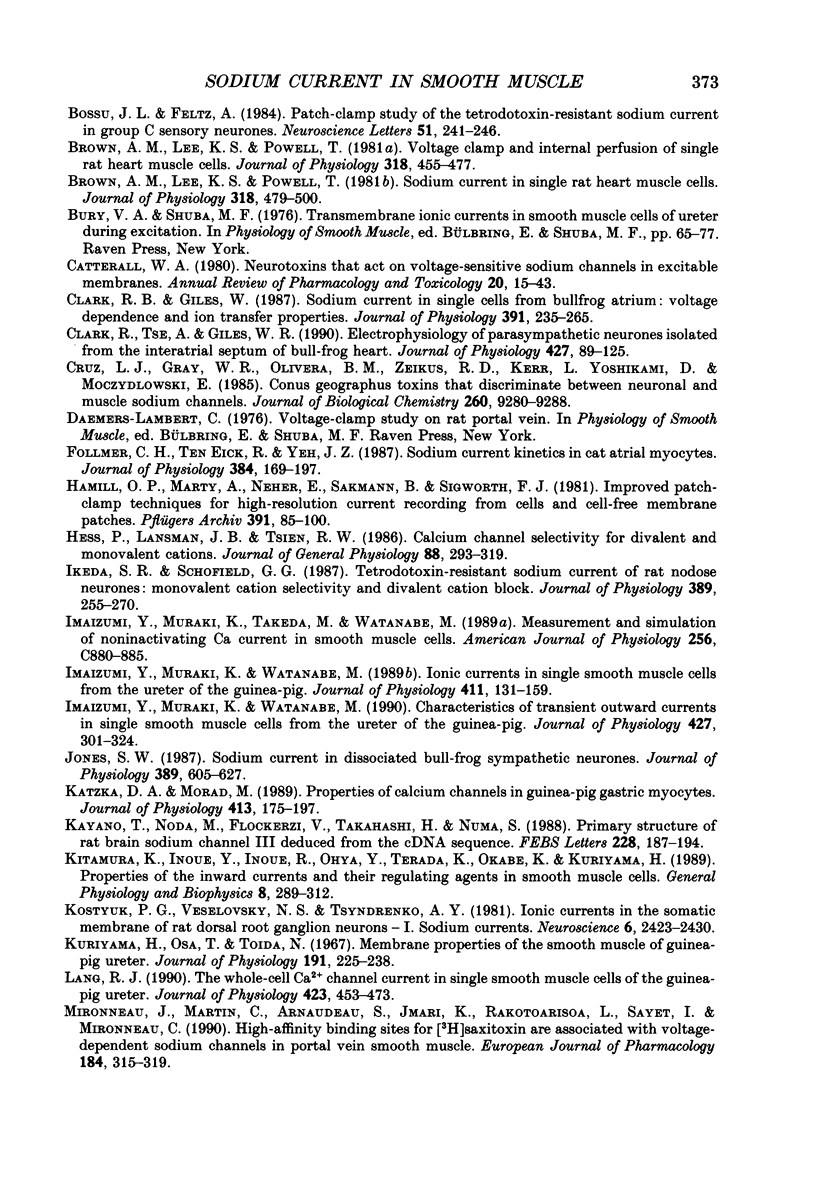
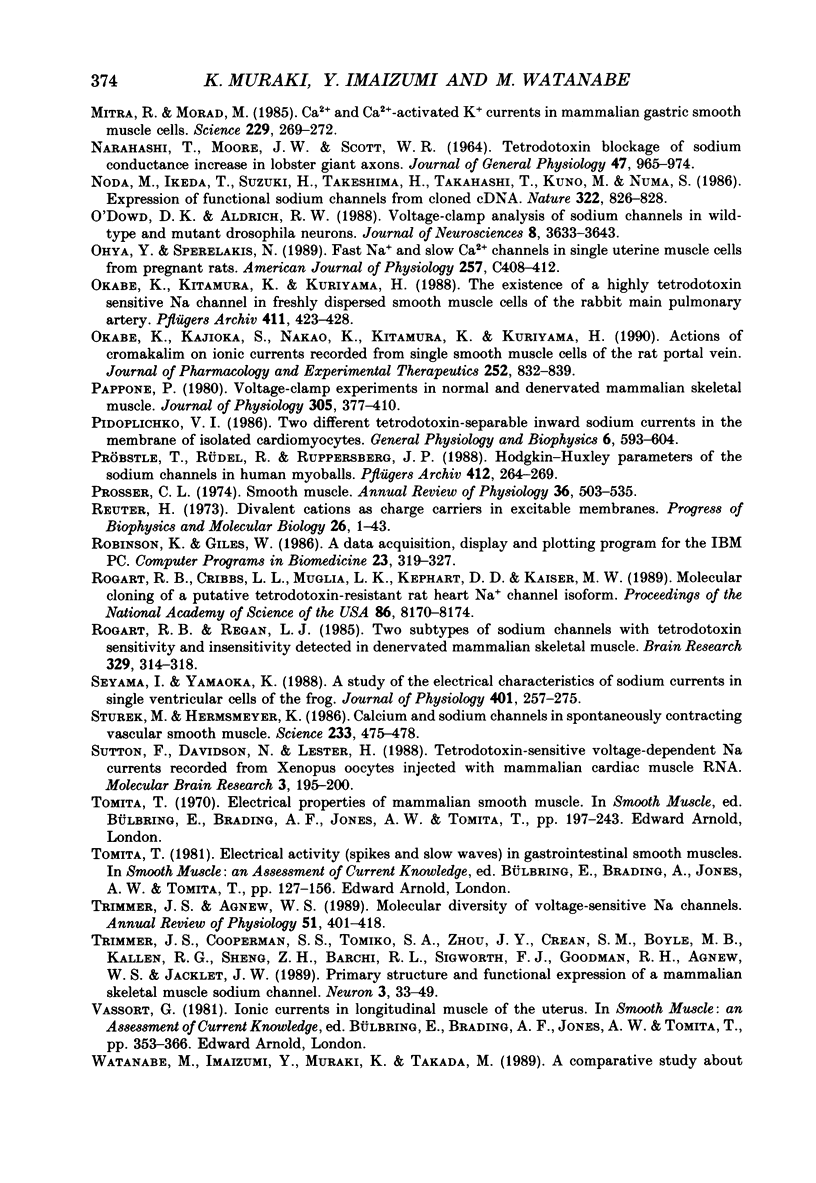
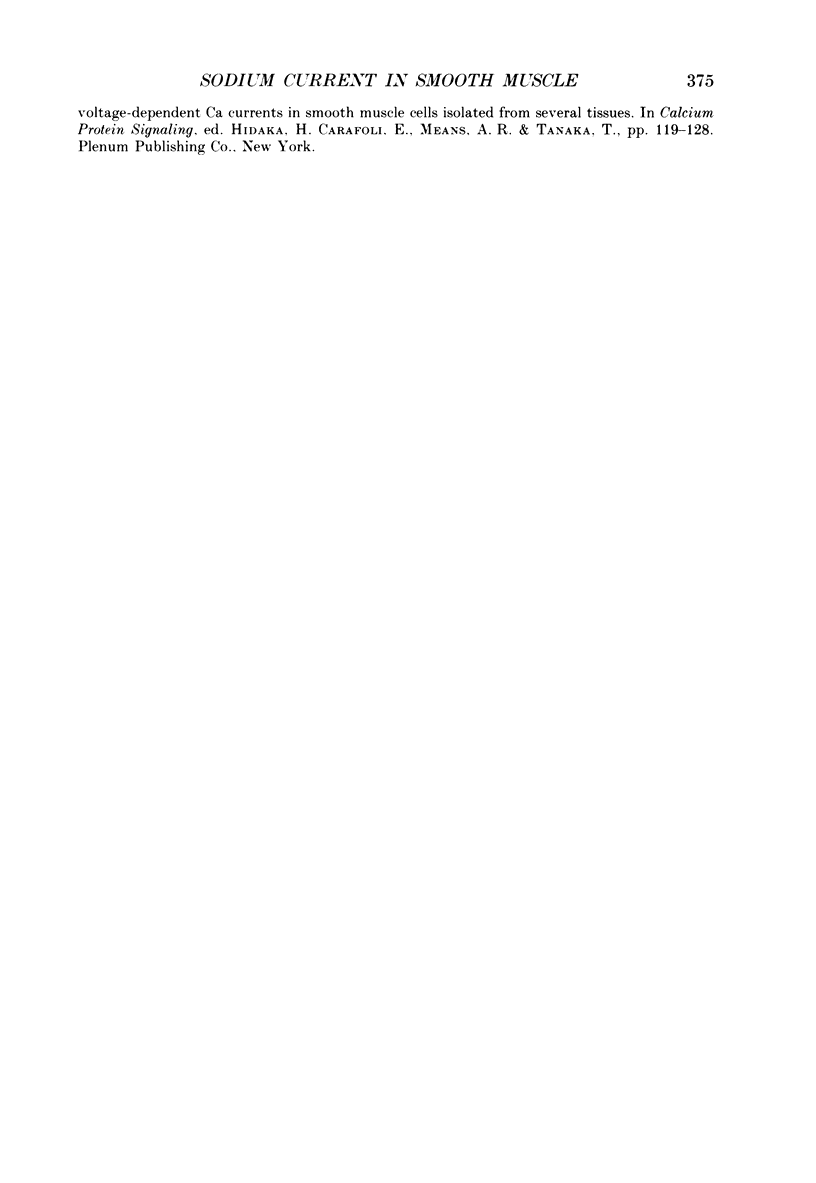
Selected References
These references are in PubMed. This may not be the complete list of references from this article.
- Amédée T., Renaud J. F., Jmari K., Lombet A., Mironneau J., Lazdunski M. The presence of Na+ channels in myometrial smooth muscle cells is revealed by specific neurotoxins. Biochem Biophys Res Commun. 1986 Jun 13;137(2):675–681. doi: 10.1016/0006-291x(86)91131-9. [DOI] [PubMed] [Google Scholar]
- Antoni H., Böcker D., Eickhorn R. Sodium current kinetics in intact rat papillary muscle: measurements with the loose-patch-clamp technique. J Physiol. 1988 Dec;406:199–213. doi: 10.1113/jphysiol.1988.sp017376. [DOI] [PMC free article] [PubMed] [Google Scholar]
- Bean B. P. Two kinds of calcium channels in canine atrial cells. Differences in kinetics, selectivity, and pharmacology. J Gen Physiol. 1985 Jul;86(1):1–30. doi: 10.1085/jgp.86.1.1. [DOI] [PMC free article] [PubMed] [Google Scholar]
- Benoit E., Corbier A., Dubois J. M. Evidence for two transient sodium currents in the frog node of Ranvier. J Physiol. 1985 Apr;361:339–360. doi: 10.1113/jphysiol.1985.sp015649. [DOI] [PMC free article] [PubMed] [Google Scholar]
- Bodewei R., Hering S., Lemke B., Rosenshtraukh L. V., Undrovinas A. I., Wollenberger A. Characterization of the fast sodium current in isolated rat myocardial cells: simulation of the clamped membrane potential. J Physiol. 1982 Apr;325:301–315. doi: 10.1113/jphysiol.1982.sp014151. [DOI] [PMC free article] [PubMed] [Google Scholar]
- Bossu J. L., Feltz A. Patch-clamp study of the tetrodotoxin-resistant sodium current in group C sensory neurones. Neurosci Lett. 1984 Oct 12;51(2):241–246. doi: 10.1016/0304-3940(84)90558-5. [DOI] [PubMed] [Google Scholar]
- Brown A. M., Lee K. S., Powell T. Sodium current in single rat heart muscle cells. J Physiol. 1981 Sep;318:479–500. doi: 10.1113/jphysiol.1981.sp013879. [DOI] [PMC free article] [PubMed] [Google Scholar]
- Brown A. M., Lee K. S., Powell T. Voltage clamp and internal perfusion of single rat heart muscle cells. J Physiol. 1981 Sep;318:455–477. doi: 10.1113/jphysiol.1981.sp013878. [DOI] [PMC free article] [PubMed] [Google Scholar]
- Catterall W. A. Neurotoxins that act on voltage-sensitive sodium channels in excitable membranes. Annu Rev Pharmacol Toxicol. 1980;20:15–43. doi: 10.1146/annurev.pa.20.040180.000311. [DOI] [PubMed] [Google Scholar]
- Clark R. B., Giles W. Sodium current in single cells from bullfrog atrium: voltage dependence and ion transfer properties. J Physiol. 1987 Oct;391:235–265. doi: 10.1113/jphysiol.1987.sp016736. [DOI] [PMC free article] [PubMed] [Google Scholar]
- Clark R. B., Tse A., Giles W. R. Electrophysiology of parasympathetic neurones isolated from the interatrial septum of bull-frog heart. J Physiol. 1990 Aug;427:89–125. doi: 10.1113/jphysiol.1990.sp018163. [DOI] [PMC free article] [PubMed] [Google Scholar]
- Cruz L. J., Gray W. R., Olivera B. M., Zeikus R. D., Kerr L., Yoshikami D., Moczydlowski E. Conus geographus toxins that discriminate between neuronal and muscle sodium channels. J Biol Chem. 1985 Aug 5;260(16):9280–9288. [PubMed] [Google Scholar]
- Follmer C. H., ten Eick R. E., Yeh J. Z. Sodium current kinetics in cat atrial myocytes. J Physiol. 1987 Mar;384:169–197. doi: 10.1113/jphysiol.1987.sp016449. [DOI] [PMC free article] [PubMed] [Google Scholar]
- Hamill O. P., Marty A., Neher E., Sakmann B., Sigworth F. J. Improved patch-clamp techniques for high-resolution current recording from cells and cell-free membrane patches. Pflugers Arch. 1981 Aug;391(2):85–100. doi: 10.1007/BF00656997. [DOI] [PubMed] [Google Scholar]
- Hess P., Lansman J. B., Tsien R. W. Calcium channel selectivity for divalent and monovalent cations. Voltage and concentration dependence of single channel current in ventricular heart cells. J Gen Physiol. 1986 Sep;88(3):293–319. doi: 10.1085/jgp.88.3.293. [DOI] [PMC free article] [PubMed] [Google Scholar]
- Ikeda S. R., Schofield G. G. Tetrodotoxin-resistant sodium current of rat nodose neurones: monovalent cation selectivity and divalent cation block. J Physiol. 1987 Aug;389:255–270. doi: 10.1113/jphysiol.1987.sp016656. [DOI] [PMC free article] [PubMed] [Google Scholar]
- Imaizumi Y., Muraki K., Takeda M., Watanabe M. Measurement and simulation of noninactivating Ca current in smooth muscle cells. Am J Physiol. 1989 Apr;256(4 Pt 1):C880–C885. doi: 10.1152/ajpcell.1989.256.4.C880. [DOI] [PubMed] [Google Scholar]
- Imaizumi Y., Muraki K., Watanabe M. Characteristics of transient outward currents in single smooth muscle cells from the ureter of the guinea-pig. J Physiol. 1990 Aug;427:301–324. doi: 10.1113/jphysiol.1990.sp018173. [DOI] [PMC free article] [PubMed] [Google Scholar]
- Imaizumi Y., Muraki K., Watanabe M. Ionic currents in single smooth muscle cells from the ureter of the guinea-pig. J Physiol. 1989 Apr;411:131–159. doi: 10.1113/jphysiol.1989.sp017565. [DOI] [PMC free article] [PubMed] [Google Scholar]
- Jones S. W. Sodium currents in dissociated bull-frog sympathetic neurones. J Physiol. 1987 Aug;389:605–627. doi: 10.1113/jphysiol.1987.sp016674. [DOI] [PMC free article] [PubMed] [Google Scholar]
- Katzka D. A., Morad M. Properties of calcium channels in guinea-pig gastric myocytes. J Physiol. 1989 Jun;413:175–197. doi: 10.1113/jphysiol.1989.sp017648. [DOI] [PMC free article] [PubMed] [Google Scholar]
- Kayano T., Noda M., Flockerzi V., Takahashi H., Numa S. Primary structure of rat brain sodium channel III deduced from the cDNA sequence. FEBS Lett. 1988 Feb 8;228(1):187–194. doi: 10.1016/0014-5793(88)80614-8. [DOI] [PubMed] [Google Scholar]
- Kitamura K., Inoue Y., Inoue R., Ohya Y., Terada K., Okabe K., Kuriyama H. Properties of the inward ionic currents and their regulating agents in smooth muscle cells. Gen Physiol Biophys. 1989 Aug;8(4):289–312. [PubMed] [Google Scholar]
- Kostyuk P. G., Veselovsky N. S., Tsyndrenko A. Y. Ionic currents in the somatic membrane of rat dorsal root ganglion neurons-I. Sodium currents. Neuroscience. 1981;6(12):2423–2430. doi: 10.1016/0306-4522(81)90088-9. [DOI] [PubMed] [Google Scholar]
- Kuriyama H., Osa T., Toida N. Membrane properties of the smooth muscle of guinea-pig ureter. J Physiol. 1967 Jul;191(2):225–238. doi: 10.1113/jphysiol.1967.sp008247. [DOI] [PMC free article] [PubMed] [Google Scholar]
- Lang R. J. The whole-cell Ca2+ channel current in single smooth muscle cells of the guinea-pig ureter. J Physiol. 1990 Apr;423:453–473. doi: 10.1113/jphysiol.1990.sp018033. [DOI] [PMC free article] [PubMed] [Google Scholar]
- Mironneau J., Martin C., Arnaudeau S., Jmari K., Rakotoarisoa L., Sayet I., Mironneau C. High-affinity binding sites for [3H]saxitoxin are associated with voltage-dependent sodium channels in portal vein smooth muscle. Eur J Pharmacol. 1990 Aug 10;184(2-3):315–319. doi: 10.1016/0014-2999(90)90624-f. [DOI] [PubMed] [Google Scholar]
- Mitra R., Morad M. Ca2+ and Ca2+-activated K+ currents in mammalian gastric smooth muscle cells. Science. 1985 Jul 19;229(4710):269–272. doi: 10.1126/science.2409600. [DOI] [PubMed] [Google Scholar]
- NARAHASHI T., MOORE J. W., SCOTT W. R. TETRODOTOXIN BLOCKAGE OF SODIUM CONDUCTANCE INCREASE IN LOBSTER GIANT AXONS. J Gen Physiol. 1964 May;47:965–974. doi: 10.1085/jgp.47.5.965. [DOI] [PMC free article] [PubMed] [Google Scholar]
- Noda M., Ikeda T., Suzuki H., Takeshima H., Takahashi T., Kuno M., Numa S. Expression of functional sodium channels from cloned cDNA. 1986 Aug 28-Sep 3Nature. 322(6082):826–828. doi: 10.1038/322826a0. [DOI] [PubMed] [Google Scholar]
- O'Dowd D. K., Aldrich R. W. Voltage-clamp analysis of sodium channels in wild-type and mutant Drosophila neurons. J Neurosci. 1988 Oct;8(10):3633–3643. doi: 10.1523/JNEUROSCI.08-10-03633.1988. [DOI] [PMC free article] [PubMed] [Google Scholar]
- Ohya Y., Sperelakis N. Fast Na+ and slow Ca2+ channels in single uterine muscle cells from pregnant rats. Am J Physiol. 1989 Aug;257(2 Pt 1):C408–C412. doi: 10.1152/ajpcell.1989.257.2.C408. [DOI] [PubMed] [Google Scholar]
- Okabe K., Kajioka S., Nakao K., Kitamura K., Kuriyama H., Weston A. H. Actions of cromakalim on ionic currents recorded from single smooth muscle cells of the rat portal vein. J Pharmacol Exp Ther. 1990 Feb;252(2):832–839. [PubMed] [Google Scholar]
- Okabe K., Kitamura K., Kuriyama H. The existence of a highly tetrodotoxin sensitive Na channel in freshly dispersed smooth muscle cells of the rabbit main pulmonary artery. Pflugers Arch. 1988 Apr;411(4):423–428. doi: 10.1007/BF00587722. [DOI] [PubMed] [Google Scholar]
- Pappone P. A. Voltage-clamp experiments in normal and denervated mammalian skeletal muscle fibres. J Physiol. 1980 Sep;306:377–410. doi: 10.1113/jphysiol.1980.sp013403. [DOI] [PMC free article] [PubMed] [Google Scholar]
- Pidoplichko V. I. Two different tetrodotoxin-separable inward sodium currents in the membrane of isolated cardiomyocytes. Gen Physiol Biophys. 1986 Dec;5(6):593–604. [PubMed] [Google Scholar]
- Prosser C. L. Smooth muscle. Annu Rev Physiol. 1974;36:503–535. doi: 10.1146/annurev.ph.36.030174.002443. [DOI] [PubMed] [Google Scholar]
- Pröbstle T., Rüdel R., Ruppersberg J. P. Hodgkin-Huxley parameters of the sodium channels in human myoballs. Pflugers Arch. 1988 Aug;412(3):264–269. doi: 10.1007/BF00582507. [DOI] [PubMed] [Google Scholar]
- Reuter H. Divalent cations as charge carriers in excitable membranes. Prog Biophys Mol Biol. 1973;26:1–43. doi: 10.1016/0079-6107(73)90016-3. [DOI] [PubMed] [Google Scholar]
- Robinson K., Giles W. A data acquisition, display and plotting program for the IBM PC. Comput Methods Programs Biomed. 1986 Dec;23(3):319–327. doi: 10.1016/0169-2607(86)90067-2. [DOI] [PubMed] [Google Scholar]
- Rogart R. B., Cribbs L. L., Muglia L. K., Kephart D. D., Kaiser M. W. Molecular cloning of a putative tetrodotoxin-resistant rat heart Na+ channel isoform. Proc Natl Acad Sci U S A. 1989 Oct;86(20):8170–8174. doi: 10.1073/pnas.86.20.8170. [DOI] [PMC free article] [PubMed] [Google Scholar]
- Rogart R. B., Regan L. J. Two subtypes of sodium channel with tetrodotoxin sensitivity and insensitivity detected in denervated mammalian skeletal muscle. Brain Res. 1985 Mar 11;329(1-2):314–318. doi: 10.1016/0006-8993(85)90541-4. [DOI] [PubMed] [Google Scholar]
- Seyama I., Yamaoka K. A study of the electrical characteristics of sodium currents in single ventricular cells of the frog. J Physiol. 1988 Jul;401:257–275. doi: 10.1113/jphysiol.1988.sp017161. [DOI] [PMC free article] [PubMed] [Google Scholar]
- Sturek M., Hermsmeyer K. Calcium and sodium channels in spontaneously contracting vascular muscle cells. Science. 1986 Jul 25;233(4762):475–478. doi: 10.1126/science.2425434. [DOI] [PubMed] [Google Scholar]
- Trimmer J. S., Agnew W. S. Molecular diversity of voltage-sensitive Na channels. Annu Rev Physiol. 1989;51:401–418. doi: 10.1146/annurev.ph.51.030189.002153. [DOI] [PubMed] [Google Scholar]
- Trimmer J. S., Cooperman S. S., Tomiko S. A., Zhou J. Y., Crean S. M., Boyle M. B., Kallen R. G., Sheng Z. H., Barchi R. L., Sigworth F. J. Primary structure and functional expression of a mammalian skeletal muscle sodium channel. Neuron. 1989 Jul;3(1):33–49. doi: 10.1016/0896-6273(89)90113-x. [DOI] [PubMed] [Google Scholar]