Abstract
1. Changes in intracellular Ca2+ ion concentrations ([Ca2+]i) during potassium-induced depolarizations were studied in cultured embryonic Xenopus spinal neurones using the Ca(2+)-sensitive dye Fura-2 and quantitative fluorescence microscopy. 2. Membrane voltages attained during exposure to bath solutions containing 3, 10, 20, 30, 40 and 50 mM-K+ were determined under current clamp. In 3 mM-K(+)-containing solution (normal saline), the resting potential was -65 mV. The threshold voltage required to observe a measurable rise in [Ca2+]i was -40 mV (external potassium concentration [K+]o = 20 mM). The depolarization-induced [Ca2+]i signal had two components: a non-relaxing component, and, at voltages positive to -40 mV, an additional transient component on the rising phase that decayed over tens of seconds. There was substantial variability in the magnitudes of resting and voltage-induced changes in [Ca2+]i, but [Ca2+]i responses were qualitatively consistent between neurons of similar ages. 3. External potassium (K+o)-induced increases in [Ca2+]i were spatially non-homogeneous. The largest increases were seen in the nucleus, near the base of a major neurite, and in growth cones. Increases occurred more rapidly in neurites and growth cones than in somas. T-type and high-voltage-activated (HVA) channels appeared to be present in all cell regions. 4. Increases in [Ca2+]i evoked by 50 mM-K+ (depolarization to approximately -15 mV) were sensitive to treatments demonstrated to inhibit Ca2+ currents in these cells (T-type, HVA-relaxing and HVA-sustained), including Ni2+ (200 microM), Metenkephalin (17.5 microM), and omega-conotoxin (omega-CgTx; 5.5 microM). [Ca2+]i increases were reduced by caffeine (10 mM) and ryanodine (10-100 microM), agents that affect Ca2+ release from intracellular stores. 5. A sustained increase in [Ca2+]i observed at approximately -40 mV ([K+]o = 20 mM) was investigated in greater detail. Concentrations of Ni2+ sufficient to block T-type Ca2+ current slowed but did not block the rise in [Ca2+]i induced by 20 mM-K+. Met-enkephalin did not affect the [Ca2+]i response. omega-CgTx reduced the amplitude of the [Ca2+]i response, but did not eliminate the sustained component. Verapamil (100 microM), caffeine and ryanodine differentially reduced the sustained component as compared to the initial rising phase. These observations suggest that the rising phase was due to Ca2+ influx through T-type and other Ca2+ channels, and that the sustained phase was differentially sensitive to inhibition of internal Ca2+ release.(ABSTRACT TRUNCATED AT 400 WORDS)
Full text
PDF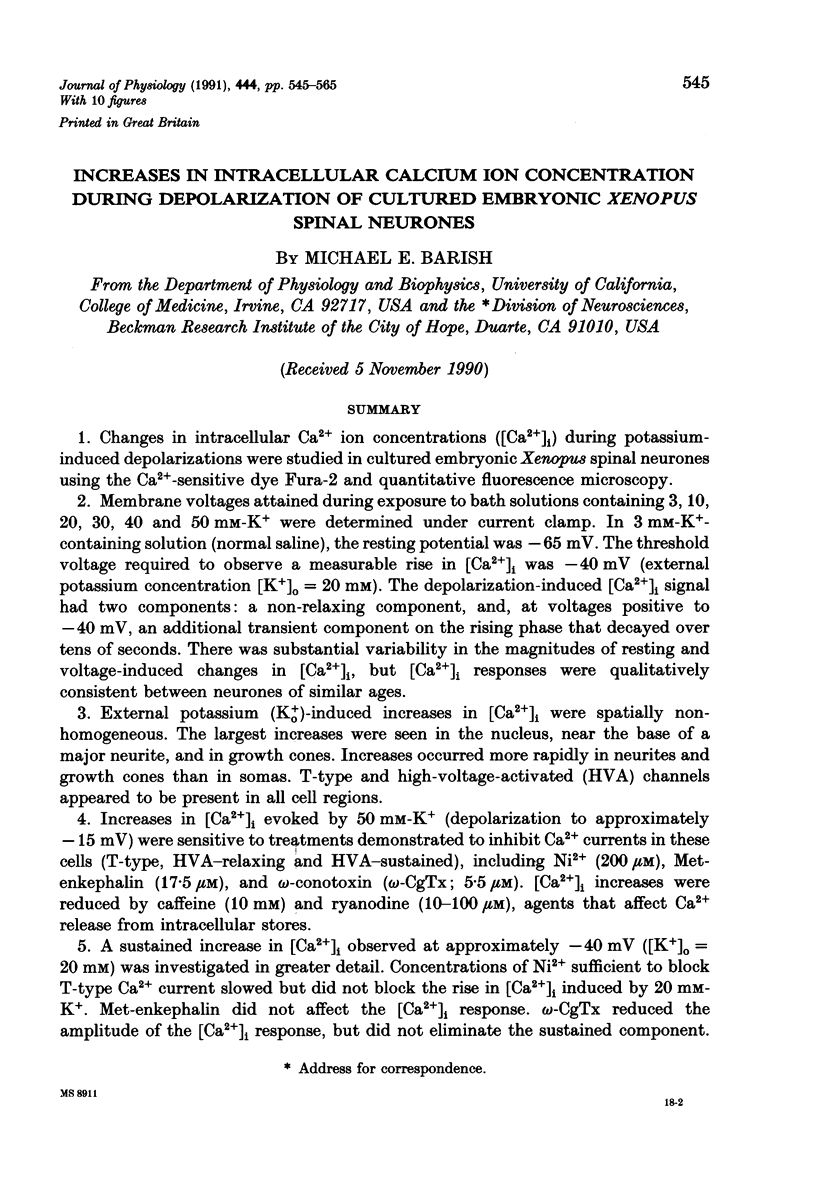
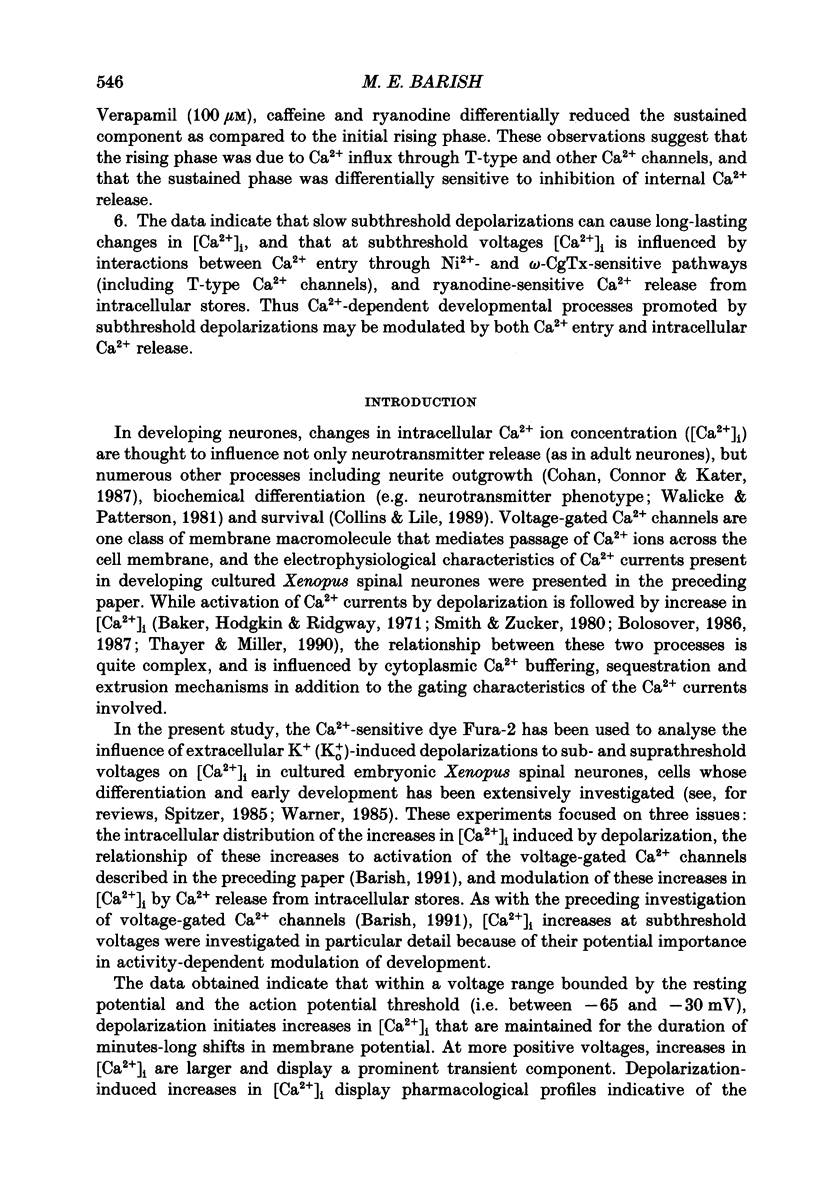
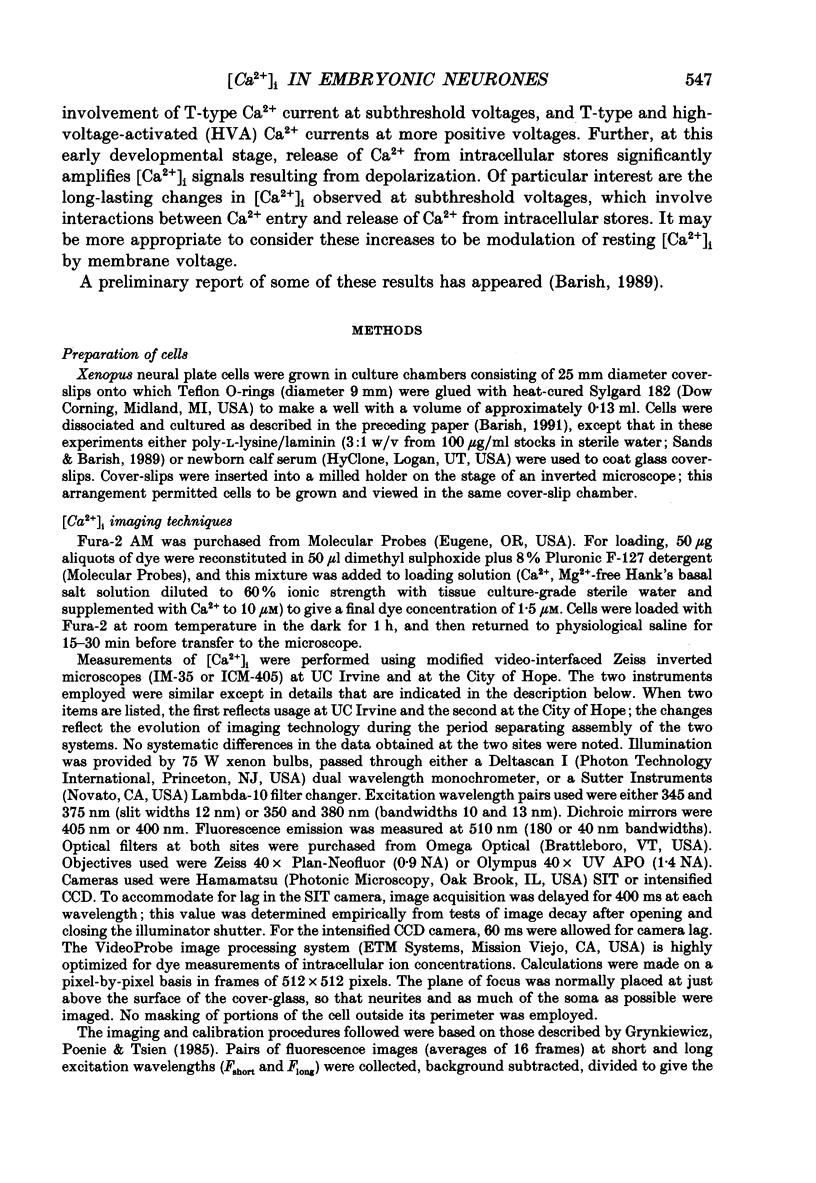
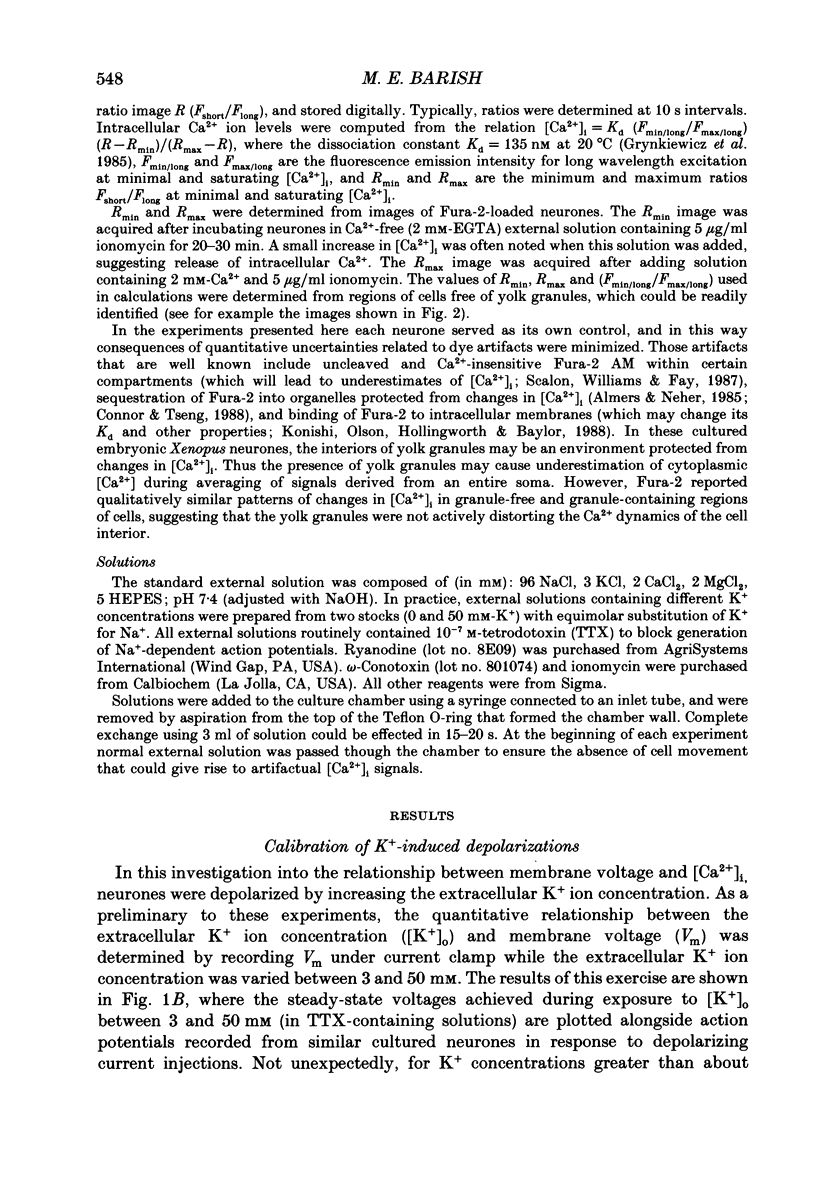
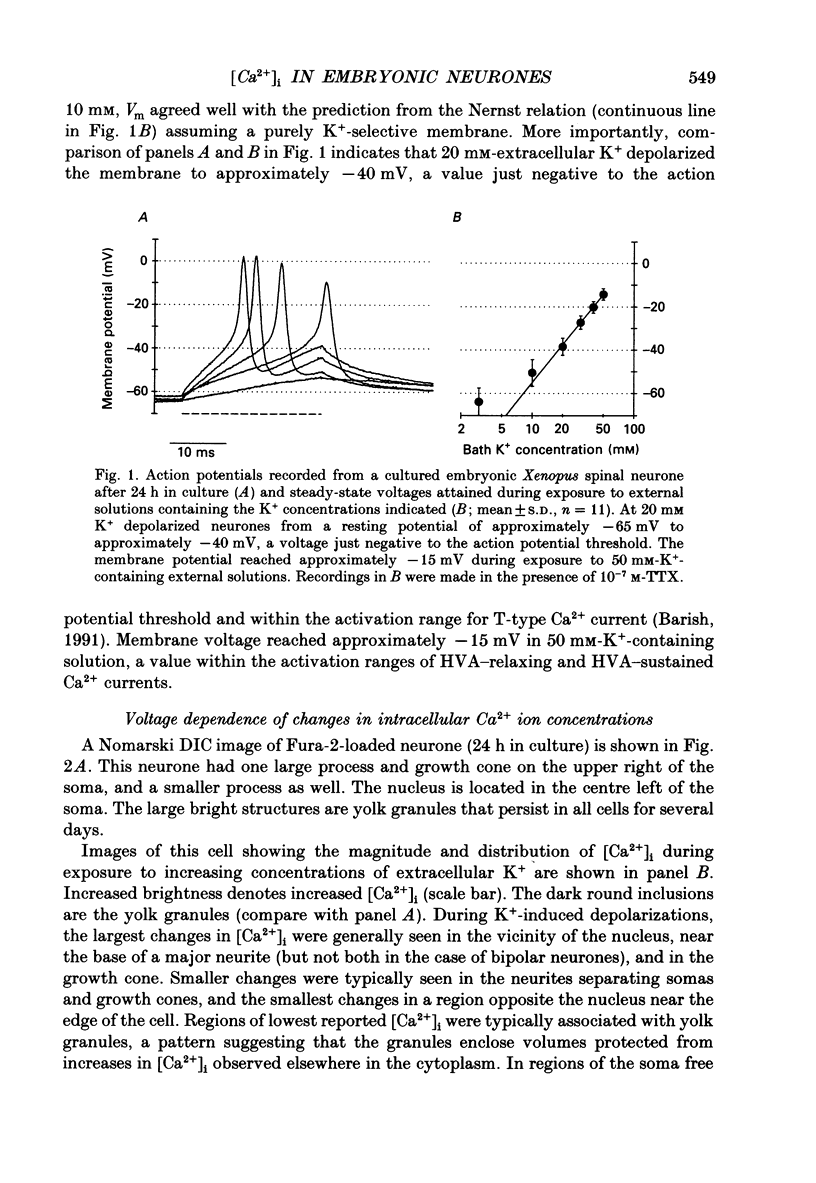
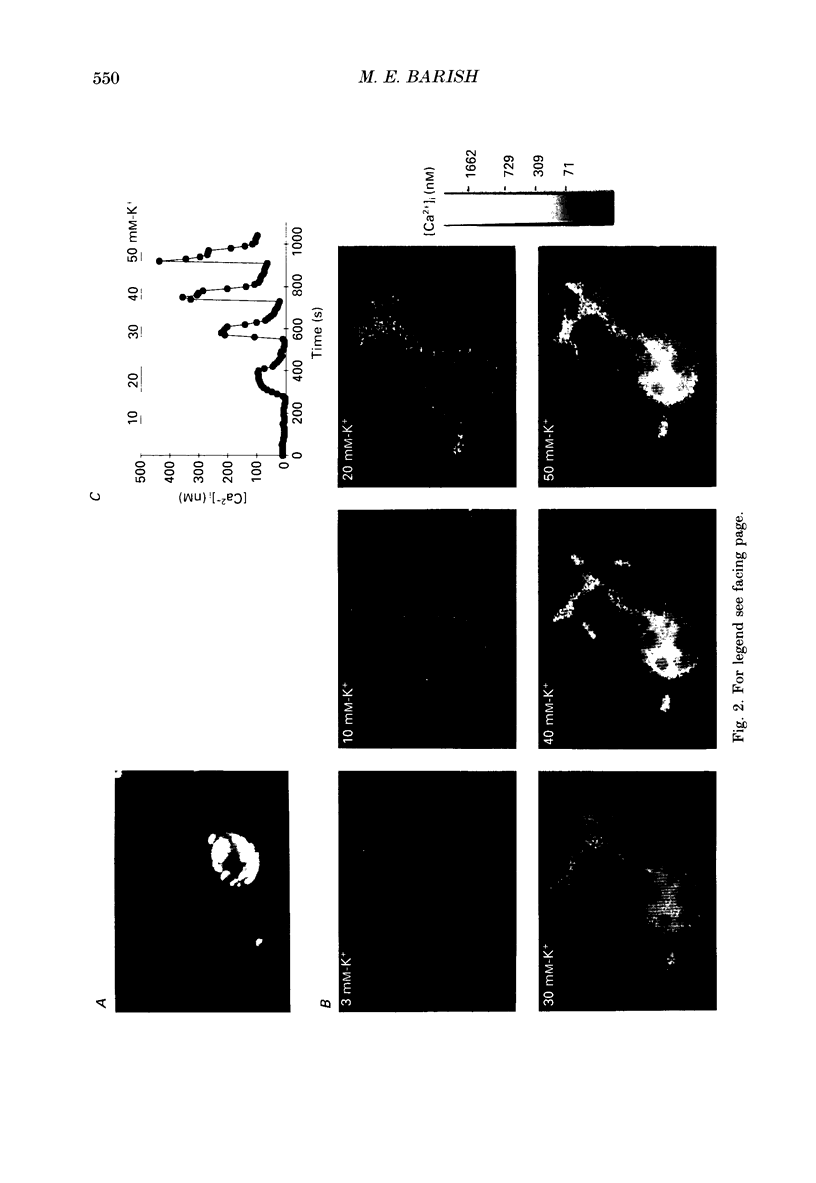
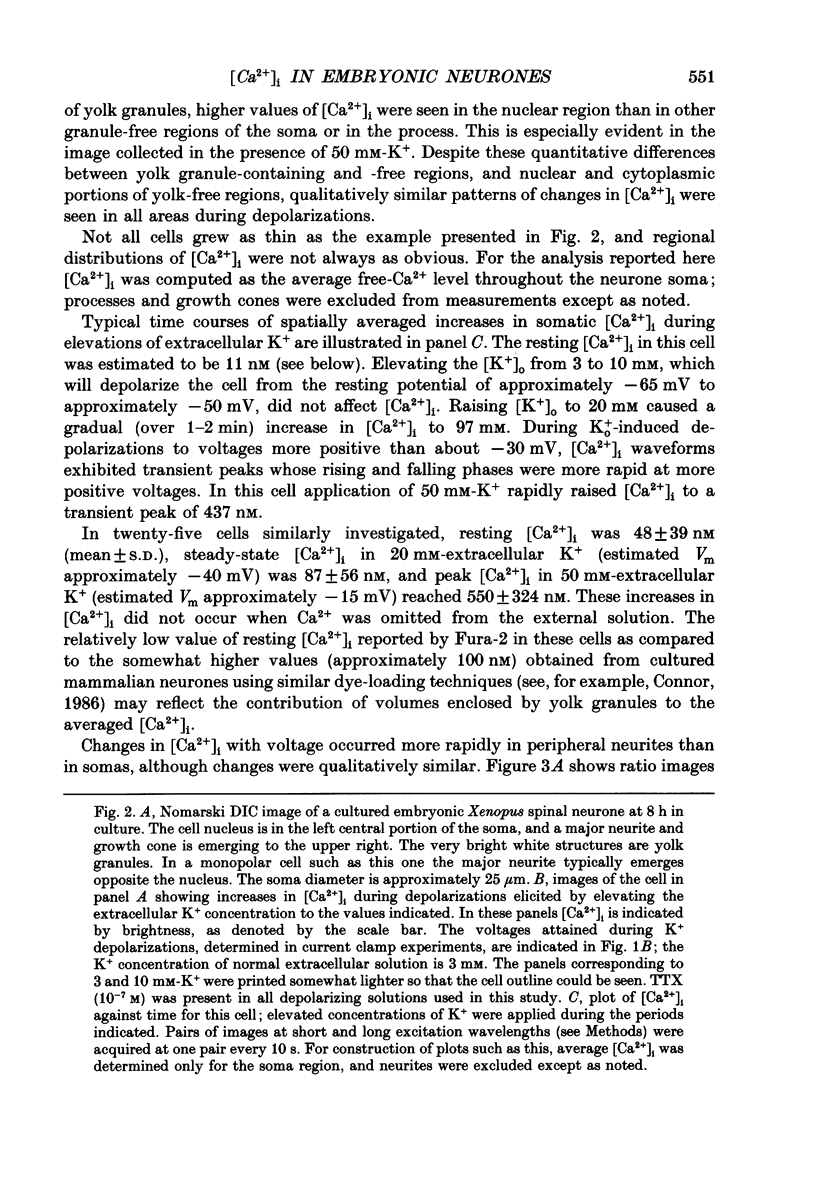
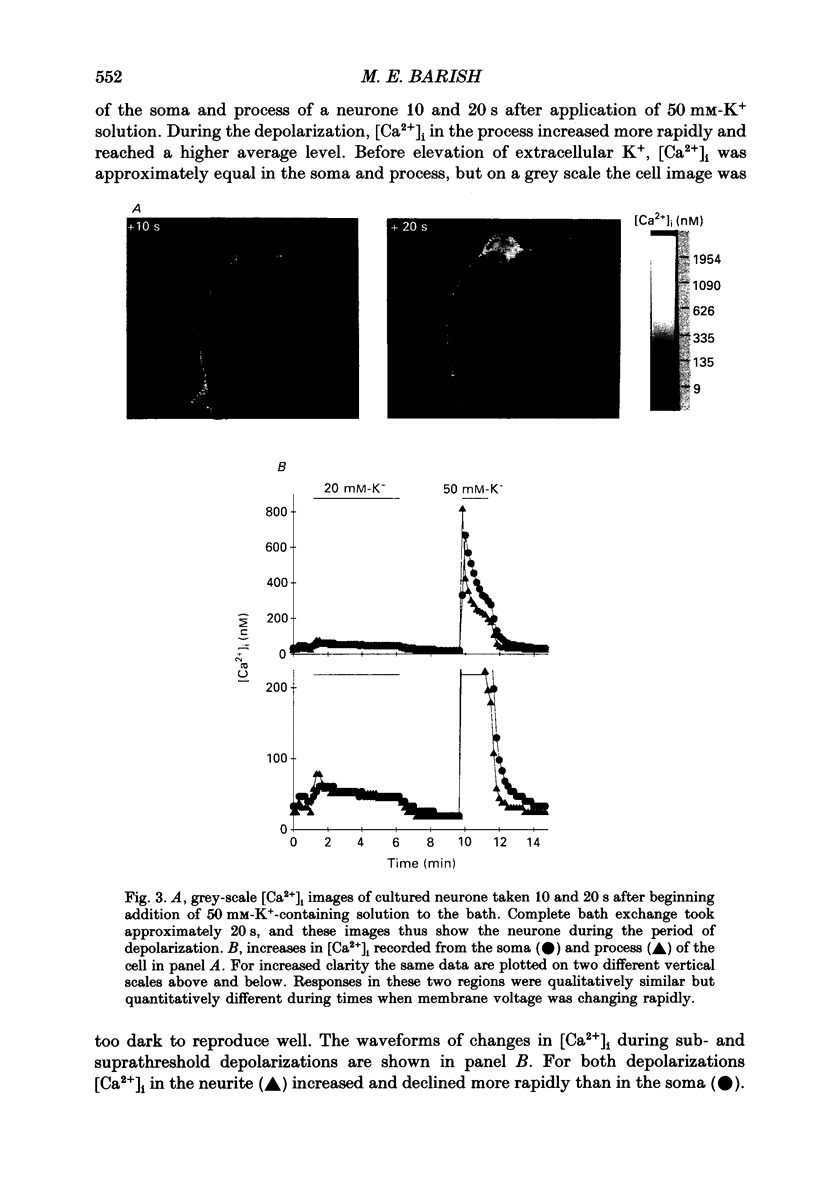
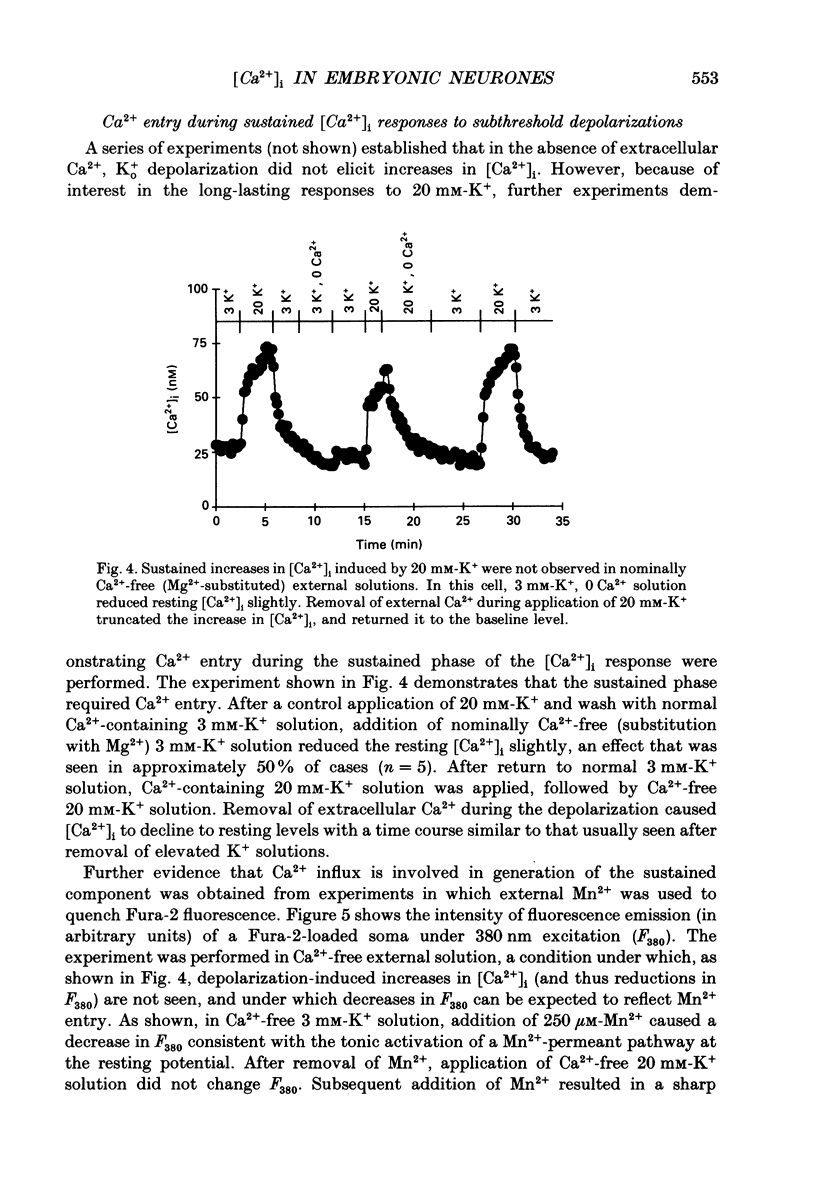
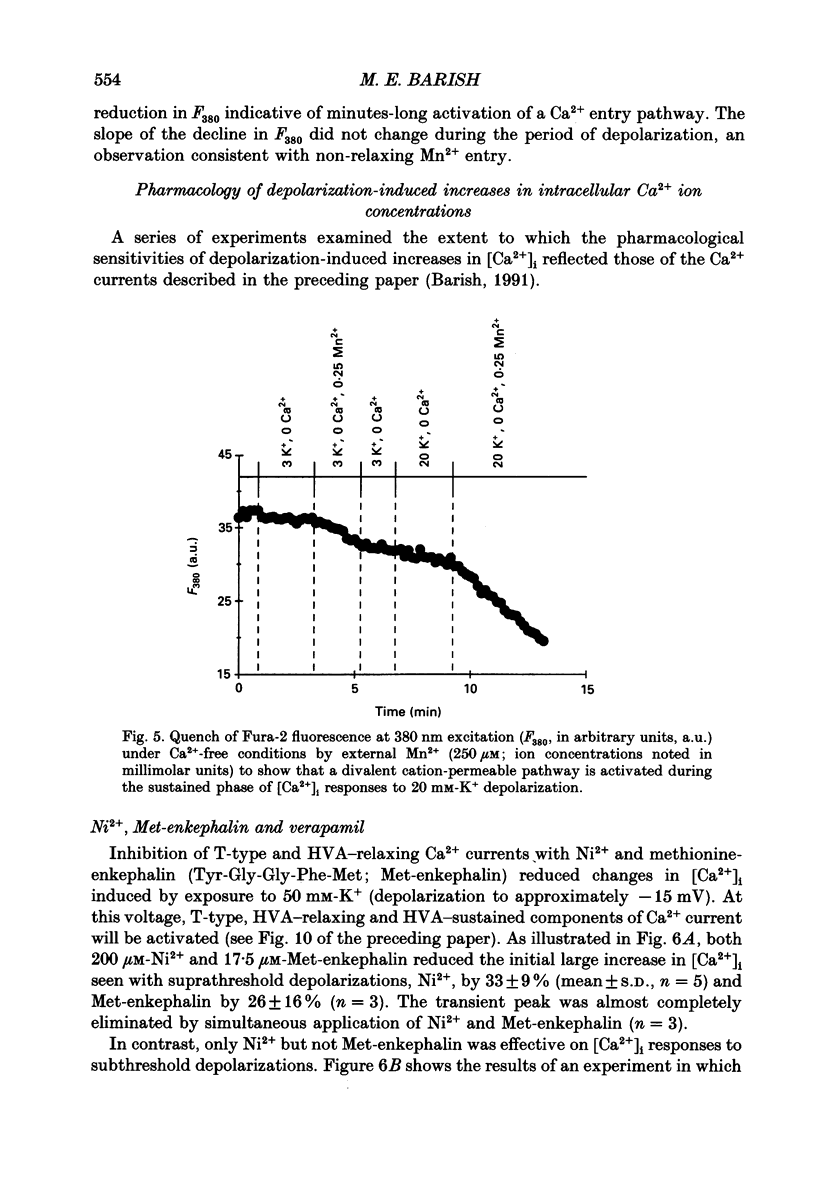
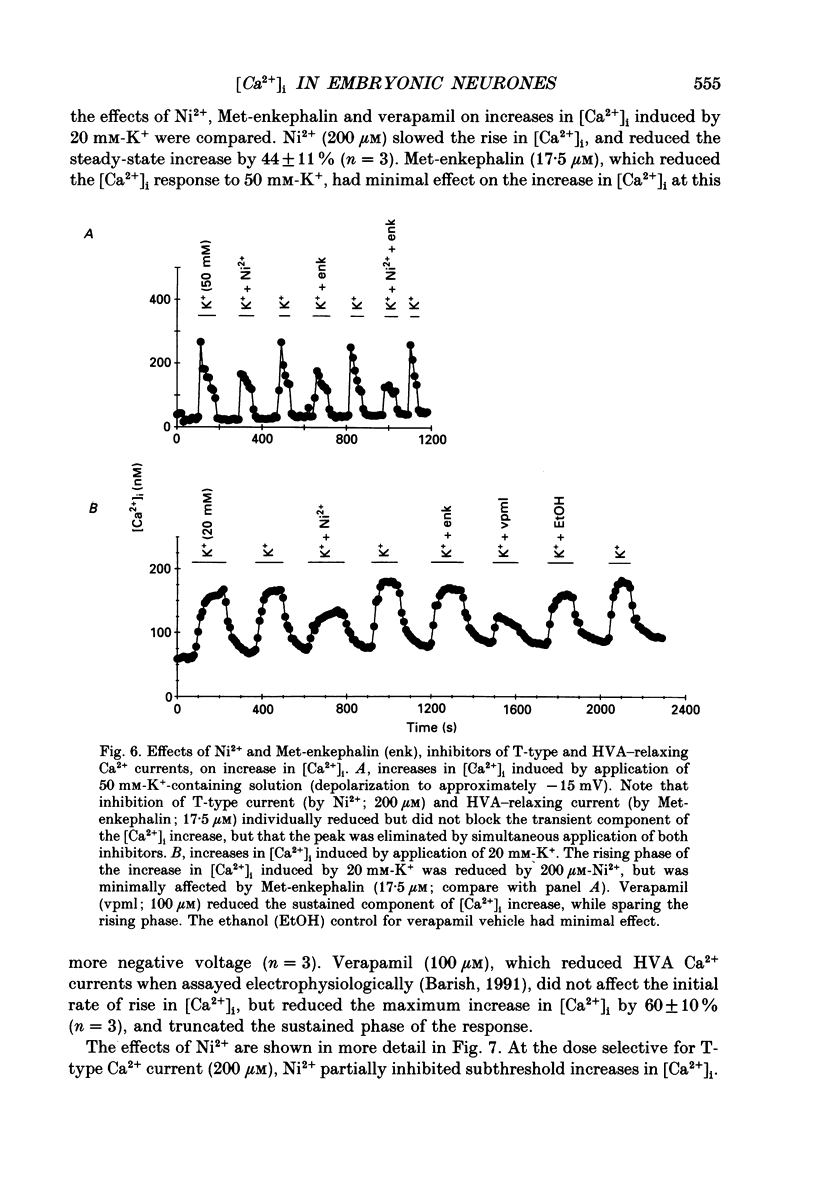
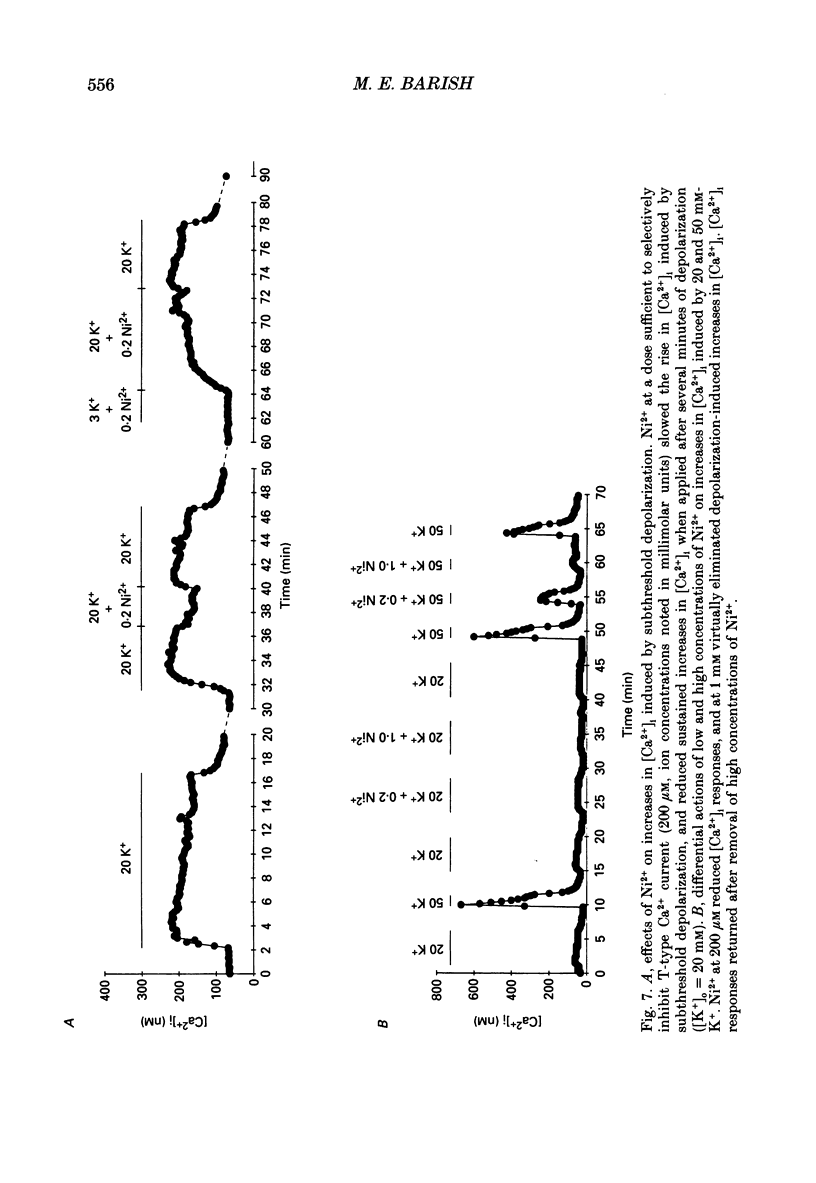
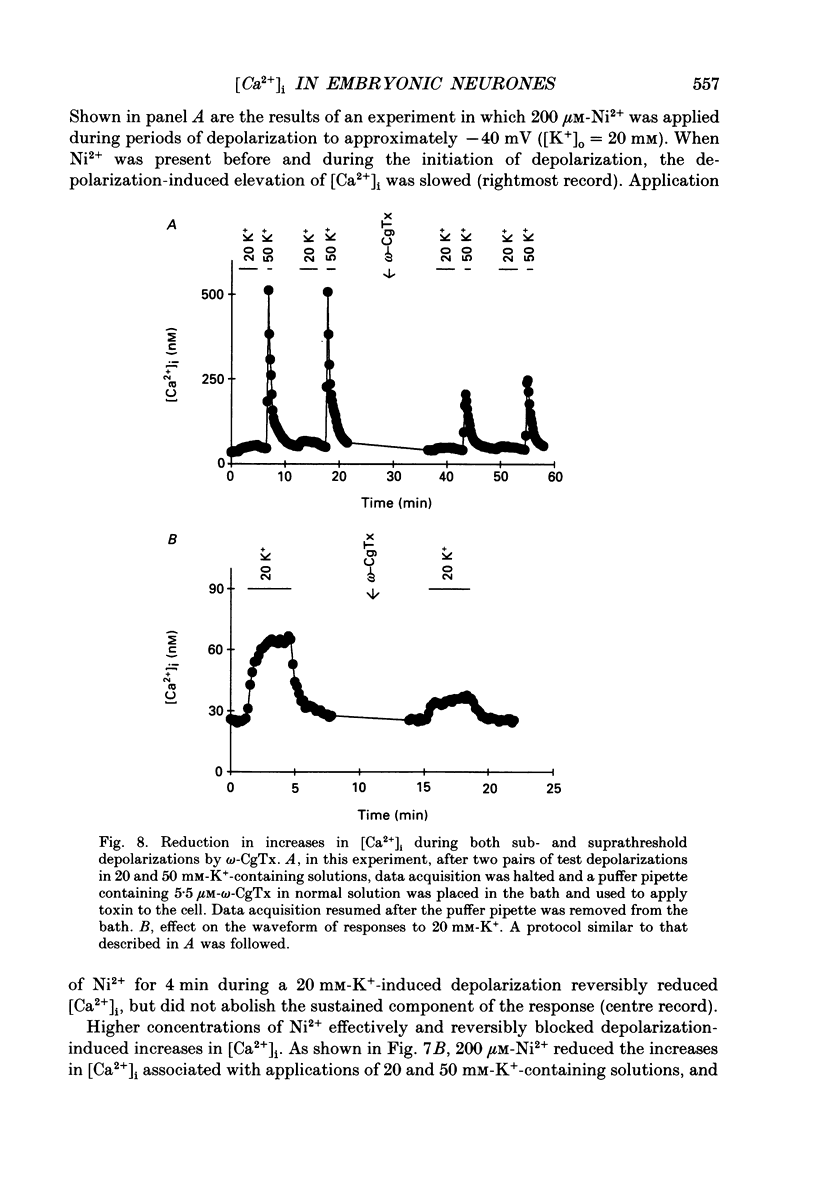
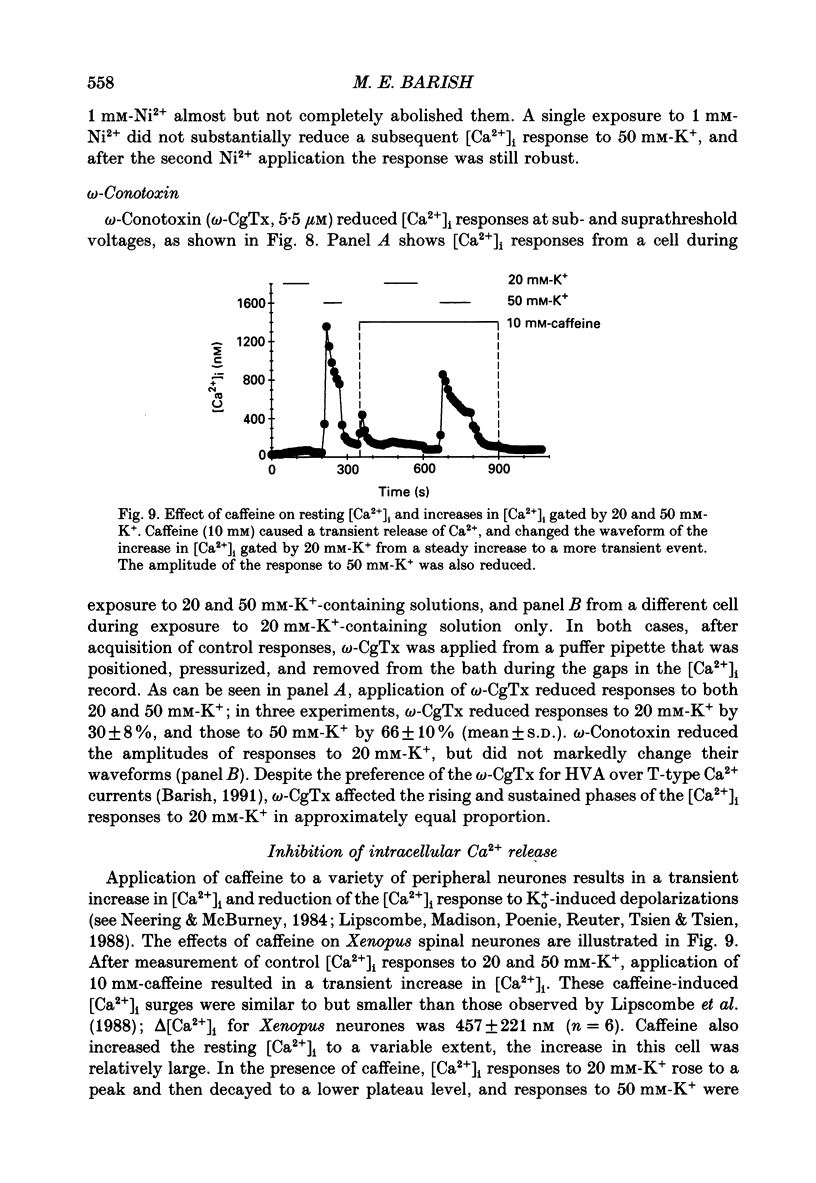
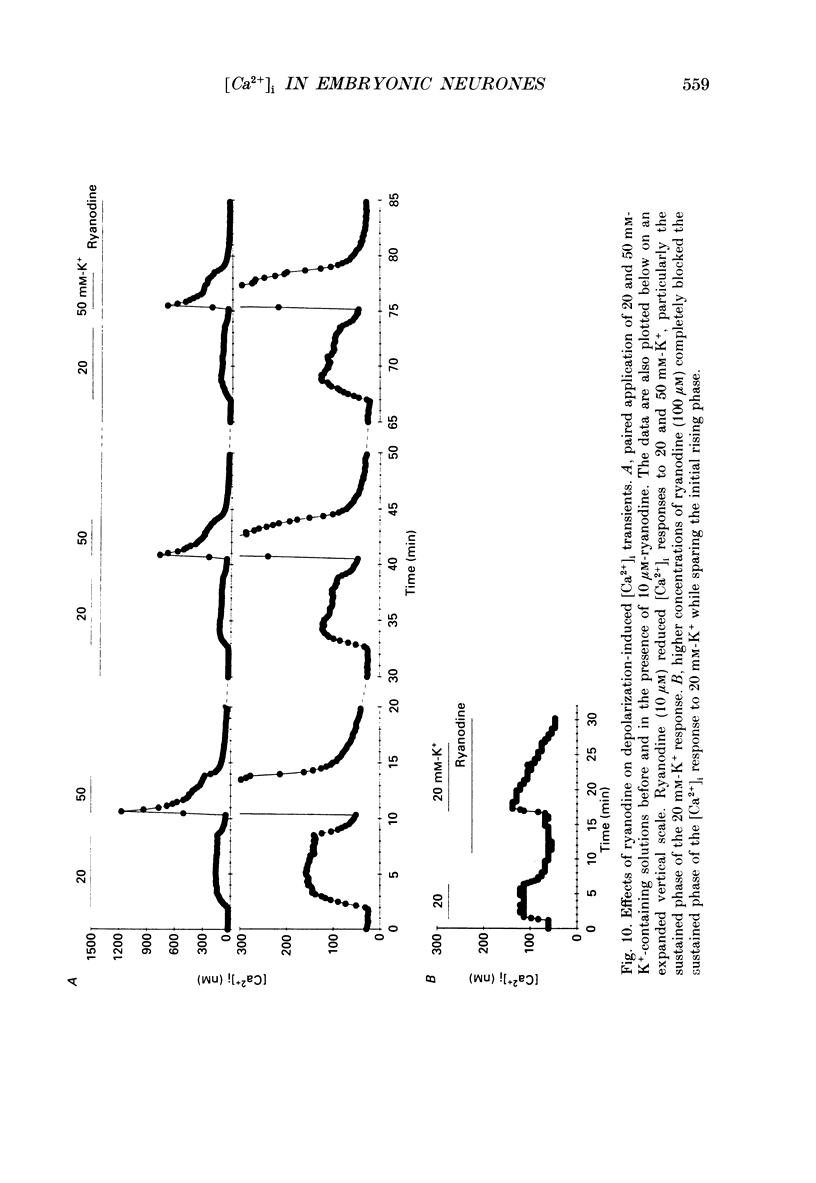
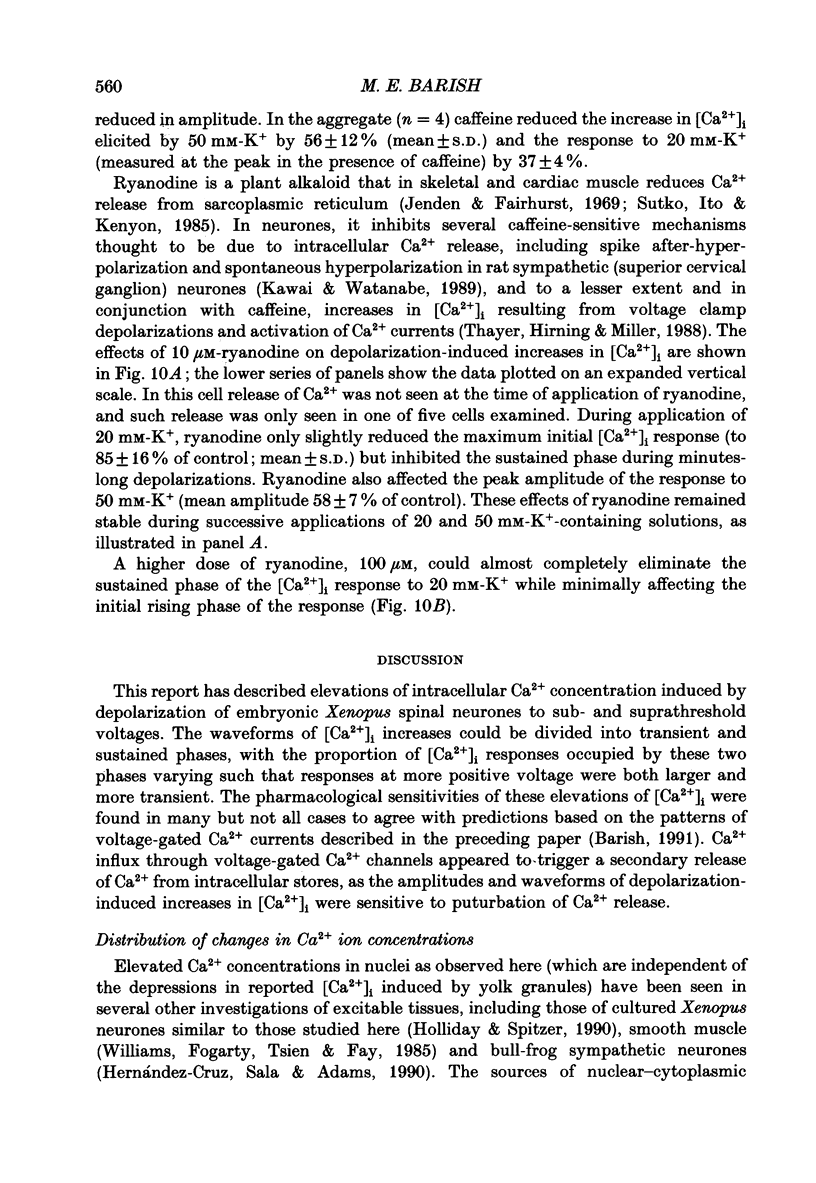
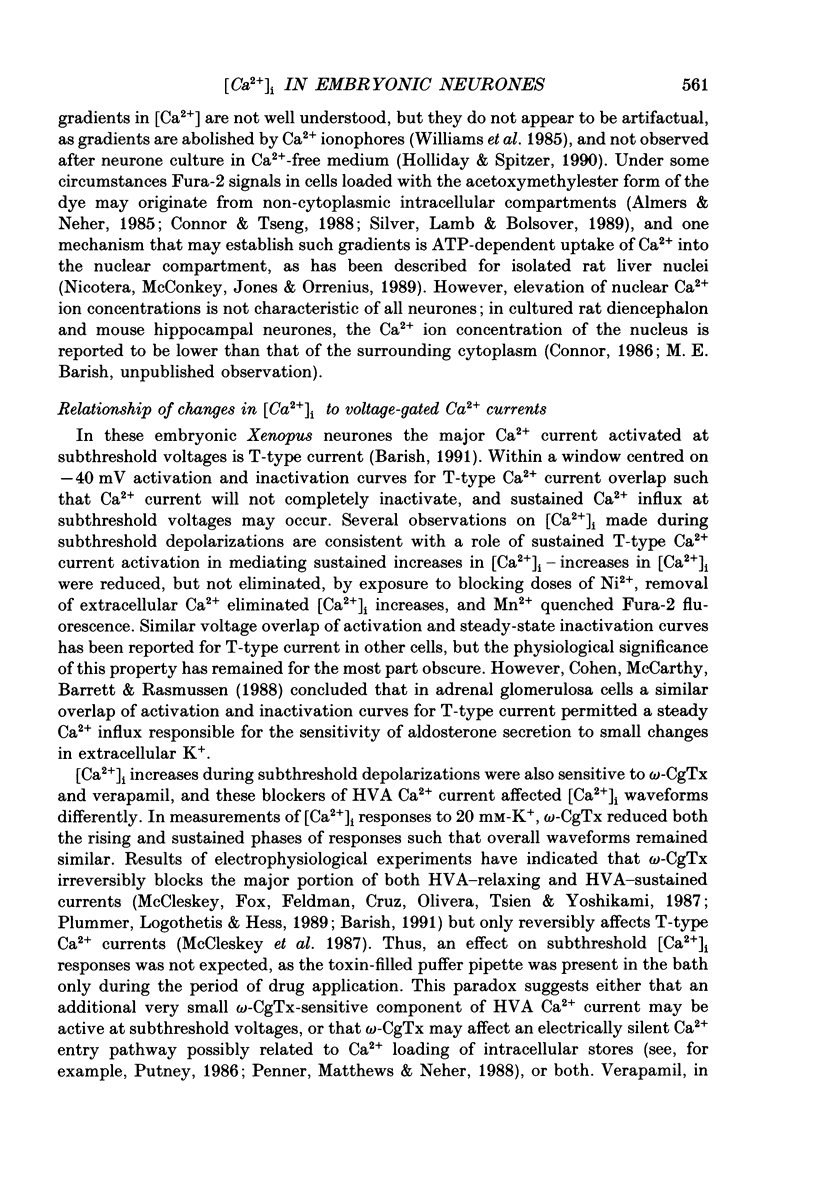
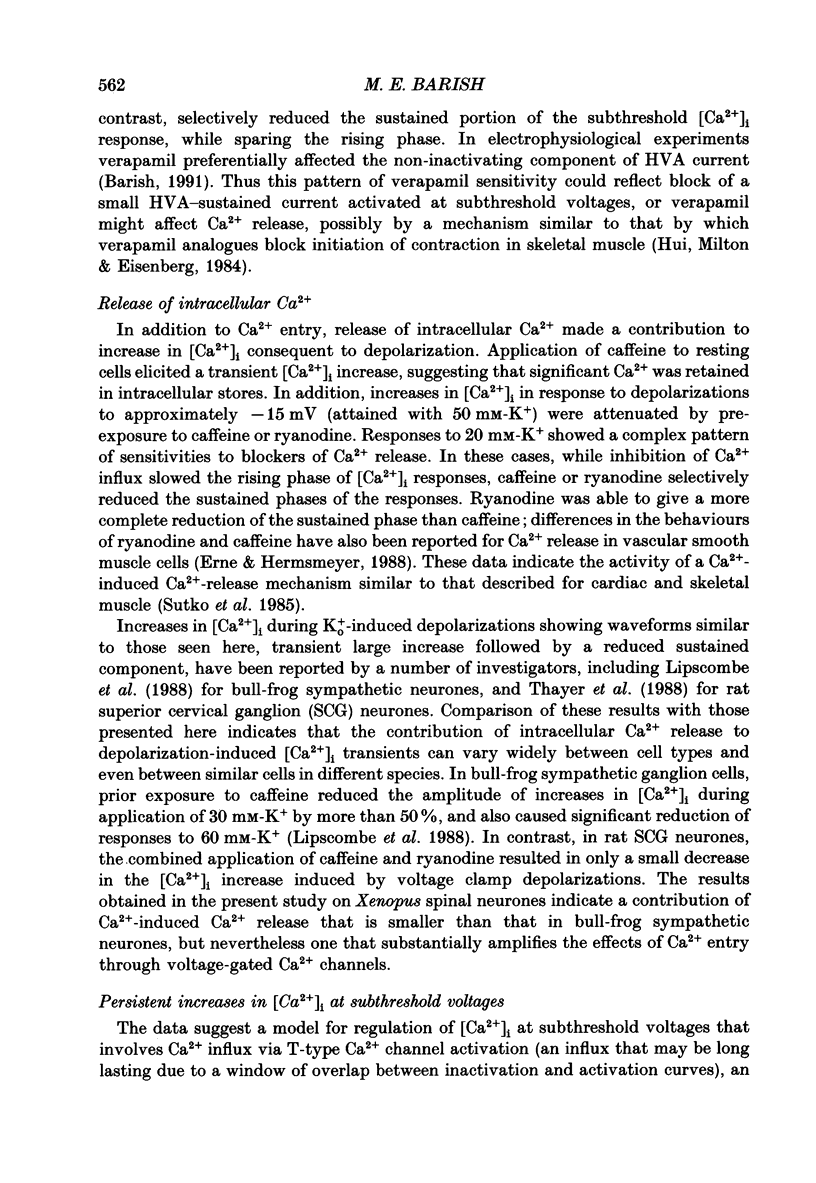
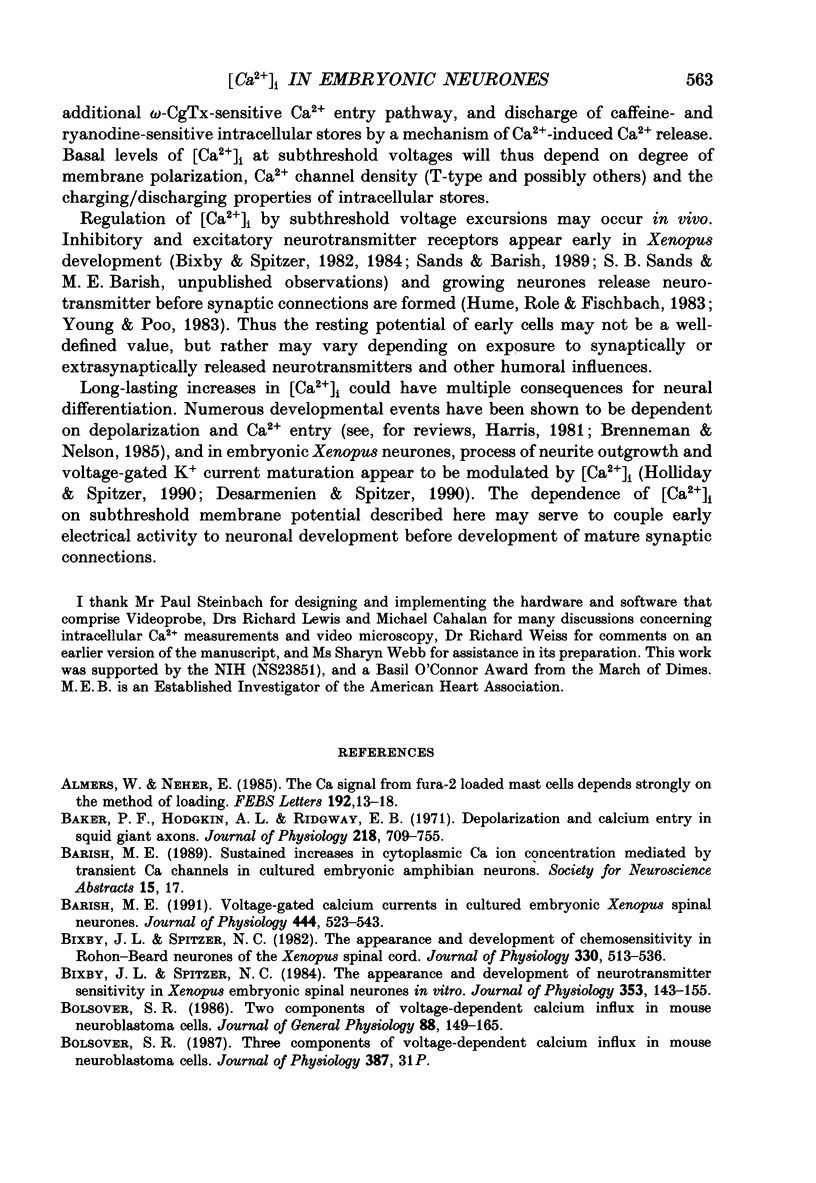
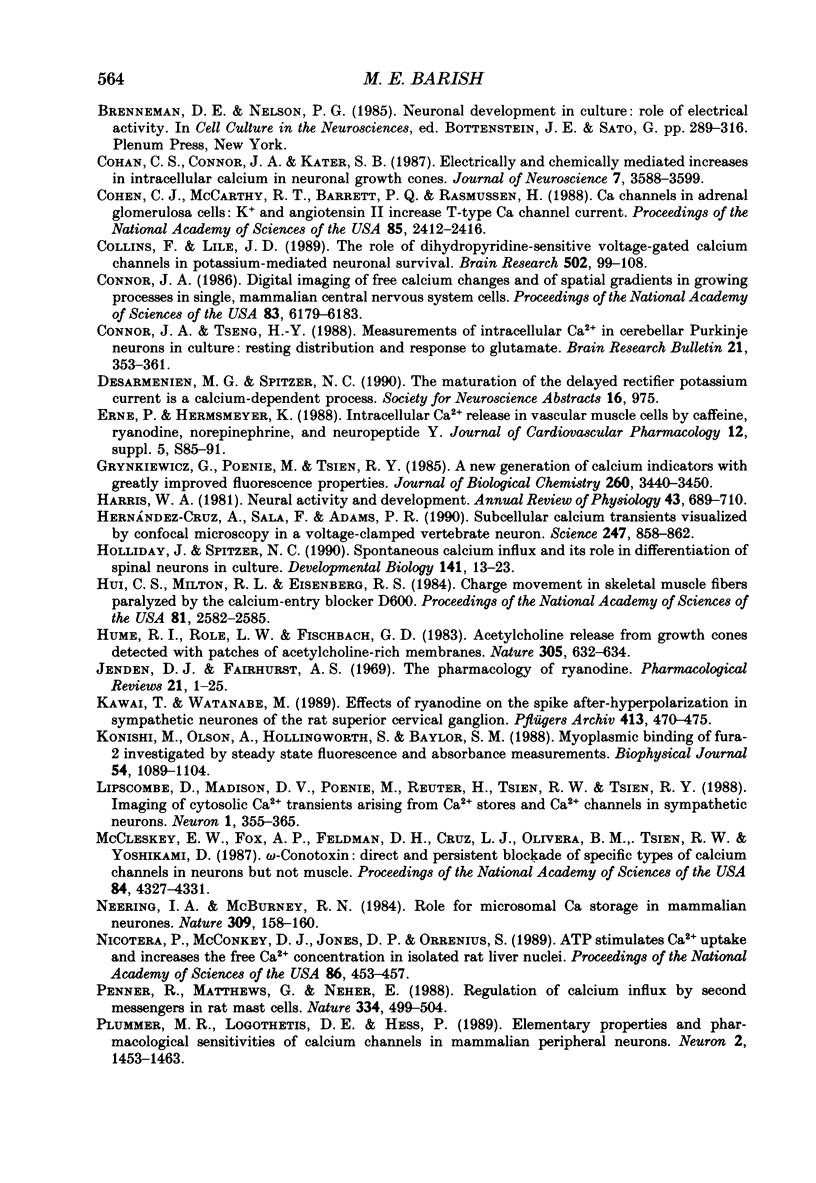
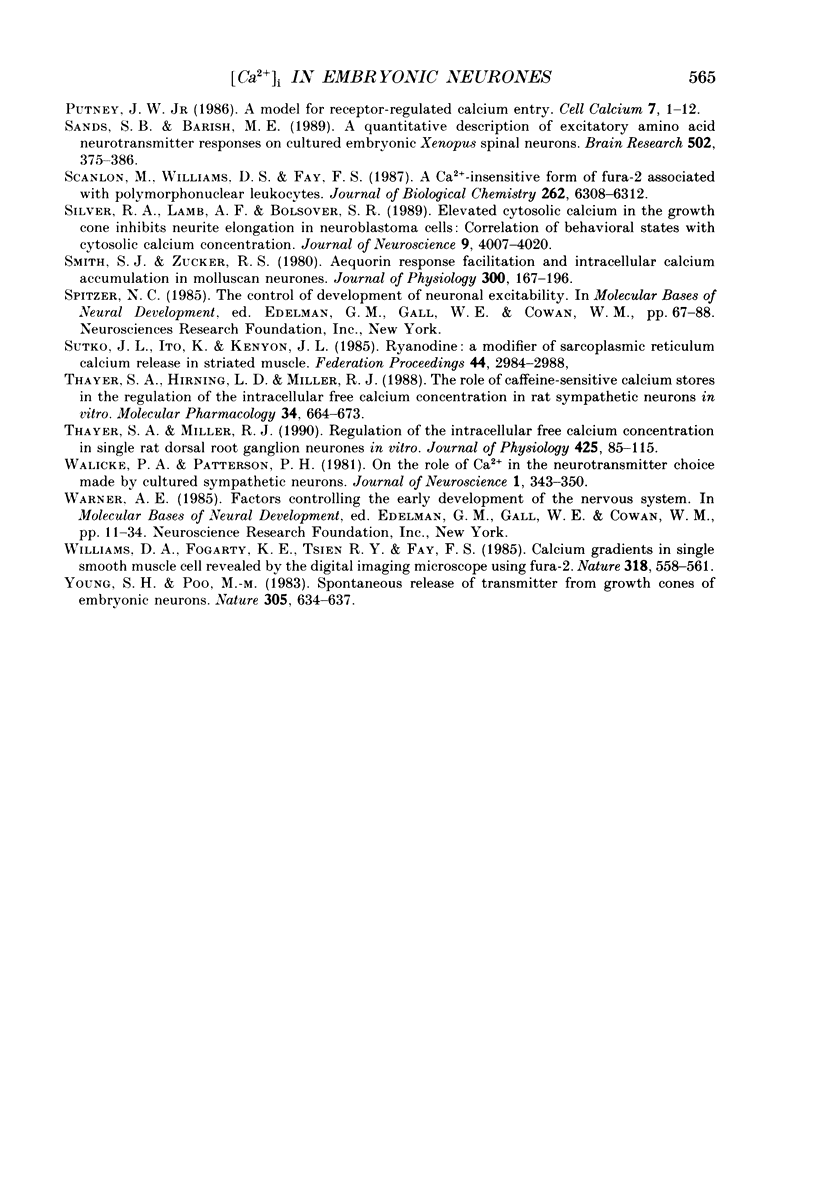
Images in this article
Selected References
These references are in PubMed. This may not be the complete list of references from this article.
- Almers W., Neher E. The Ca signal from fura-2 loaded mast cells depends strongly on the method of dye-loading. FEBS Lett. 1985 Nov 11;192(1):13–18. doi: 10.1016/0014-5793(85)80033-8. [DOI] [PubMed] [Google Scholar]
- Baker P. F., Hodgkin A. L., Ridgway E. B. Depolarization and calcium entry in squid giant axons. J Physiol. 1971 Nov;218(3):709–755. doi: 10.1113/jphysiol.1971.sp009641. [DOI] [PMC free article] [PubMed] [Google Scholar]
- Barish M. E. Voltage-gated calcium currents in cultured embryonic Xenopus spinal neurones. J Physiol. 1991 Dec;444:523–543. doi: 10.1113/jphysiol.1991.sp018892. [DOI] [PMC free article] [PubMed] [Google Scholar]
- Bixby J. L., Spitzer N. C. The appearance and development of chemosensitivity in Rohon-Beard neurones of the Xenopus spinal cord. J Physiol. 1982 Sep;330:513–536. doi: 10.1113/jphysiol.1982.sp014356. [DOI] [PMC free article] [PubMed] [Google Scholar]
- Bixby J. L., Spitzer N. C. The appearance and development of neurotransmitter sensitivity in Xenopus embryonic spinal neurones in vitro. J Physiol. 1984 Aug;353:143–155. doi: 10.1113/jphysiol.1984.sp015328. [DOI] [PMC free article] [PubMed] [Google Scholar]
- Bolsover S. R. Two components of voltage-dependent calcium influx in mouse neuroblastoma cells. Measurement with arsenazo III. J Gen Physiol. 1986 Aug;88(2):149–165. doi: 10.1085/jgp.88.2.149. [DOI] [PMC free article] [PubMed] [Google Scholar]
- Cohan C. S., Connor J. A., Kater S. B. Electrically and chemically mediated increases in intracellular calcium in neuronal growth cones. J Neurosci. 1987 Nov;7(11):3588–3599. doi: 10.1523/JNEUROSCI.07-11-03588.1987. [DOI] [PMC free article] [PubMed] [Google Scholar]
- Cohen C. J., McCarthy R. T., Barrett P. Q., Rasmussen H. Ca channels in adrenal glomerulosa cells: K+ and angiotensin II increase T-type Ca channel current. Proc Natl Acad Sci U S A. 1988 Apr;85(7):2412–2416. doi: 10.1073/pnas.85.7.2412. [DOI] [PMC free article] [PubMed] [Google Scholar]
- Collins F., Lile J. D. The role of dihydropyridine-sensitive voltage-gated calcium channels in potassium-mediated neuronal survival. Brain Res. 1989 Nov 13;502(1):99–108. doi: 10.1016/0006-8993(89)90465-4. [DOI] [PubMed] [Google Scholar]
- Connor J. A. Digital imaging of free calcium changes and of spatial gradients in growing processes in single, mammalian central nervous system cells. Proc Natl Acad Sci U S A. 1986 Aug;83(16):6179–6183. doi: 10.1073/pnas.83.16.6179. [DOI] [PMC free article] [PubMed] [Google Scholar]
- Connor J. A., Tseng H. Y. Measurement of intracellular Ca2+ in cerebellar Purkinje neurons in culture: resting distribution and response to glutamate. Brain Res Bull. 1988 Sep;21(3):353–361. doi: 10.1016/0361-9230(88)90147-5. [DOI] [PubMed] [Google Scholar]
- Grynkiewicz G., Poenie M., Tsien R. Y. A new generation of Ca2+ indicators with greatly improved fluorescence properties. J Biol Chem. 1985 Mar 25;260(6):3440–3450. [PubMed] [Google Scholar]
- Hernández-Cruz A., Sala F., Adams P. R. Subcellular calcium transients visualized by confocal microscopy in a voltage-clamped vertebrate neuron. Science. 1990 Feb 16;247(4944):858–862. doi: 10.1126/science.2154851. [DOI] [PubMed] [Google Scholar]
- Holliday J., Spitzer N. C. Spontaneous calcium influx and its roles in differentiation of spinal neurons in culture. Dev Biol. 1990 Sep;141(1):13–23. doi: 10.1016/0012-1606(90)90098-4. [DOI] [PubMed] [Google Scholar]
- Hui C. S., Milton R. L., Eisenberg R. S. Charge movement in skeletal muscle fibers paralyzed by the calcium-entry blocker D600. Proc Natl Acad Sci U S A. 1984 Apr;81(8):2582–2585. doi: 10.1073/pnas.81.8.2582. [DOI] [PMC free article] [PubMed] [Google Scholar]
- Hume R. I., Role L. W., Fischbach G. D. Acetylcholine release from growth cones detected with patches of acetylcholine receptor-rich membranes. Nature. 1983 Oct 13;305(5935):632–634. doi: 10.1038/305632a0. [DOI] [PubMed] [Google Scholar]
- Jenden D. J., Fairhurst A. S. The pharmacology of ryanodine. Pharmacol Rev. 1969 Mar;21(1):1–25. [PubMed] [Google Scholar]
- Kawai T., Watanabe M. Effects of ryanodine on the spike after-hyperpolarization in sympathetic neurones of the rat superior cervical ganglion. Pflugers Arch. 1989 Mar;413(5):470–475. doi: 10.1007/BF00594175. [DOI] [PubMed] [Google Scholar]
- Konishi M., Olson A., Hollingworth S., Baylor S. M. Myoplasmic binding of fura-2 investigated by steady-state fluorescence and absorbance measurements. Biophys J. 1988 Dec;54(6):1089–1104. doi: 10.1016/S0006-3495(88)83045-5. [DOI] [PMC free article] [PubMed] [Google Scholar]
- Lipscombe D., Madison D. V., Poenie M., Reuter H., Tsien R. W., Tsien R. Y. Imaging of cytosolic Ca2+ transients arising from Ca2+ stores and Ca2+ channels in sympathetic neurons. Neuron. 1988 Jul;1(5):355–365. doi: 10.1016/0896-6273(88)90185-7. [DOI] [PubMed] [Google Scholar]
- McCleskey E. W., Fox A. P., Feldman D. H., Cruz L. J., Olivera B. M., Tsien R. W., Yoshikami D. Omega-conotoxin: direct and persistent blockade of specific types of calcium channels in neurons but not muscle. Proc Natl Acad Sci U S A. 1987 Jun;84(12):4327–4331. doi: 10.1073/pnas.84.12.4327. [DOI] [PMC free article] [PubMed] [Google Scholar]
- Neering I. R., McBurney R. N. Role for microsomal Ca storage in mammalian neurones? Nature. 1984 May 10;309(5964):158–160. doi: 10.1038/309158a0. [DOI] [PubMed] [Google Scholar]
- Nicotera P., McConkey D. J., Jones D. P., Orrenius S. ATP stimulates Ca2+ uptake and increases the free Ca2+ concentration in isolated rat liver nuclei. Proc Natl Acad Sci U S A. 1989 Jan;86(2):453–457. doi: 10.1073/pnas.86.2.453. [DOI] [PMC free article] [PubMed] [Google Scholar]
- Penner R., Matthews G., Neher E. Regulation of calcium influx by second messengers in rat mast cells. Nature. 1988 Aug 11;334(6182):499–504. doi: 10.1038/334499a0. [DOI] [PubMed] [Google Scholar]
- Plummer M. R., Logothetis D. E., Hess P. Elementary properties and pharmacological sensitivities of calcium channels in mammalian peripheral neurons. Neuron. 1989 May;2(5):1453–1463. doi: 10.1016/0896-6273(89)90191-8. [DOI] [PubMed] [Google Scholar]
- Putney J. W., Jr A model for receptor-regulated calcium entry. Cell Calcium. 1986 Feb;7(1):1–12. doi: 10.1016/0143-4160(86)90026-6. [DOI] [PubMed] [Google Scholar]
- Sands S. B., Barish M. E. A quantitative description of excitatory amino acid neurotransmitter responses on cultured embryonic Xenopus spinal neurons. Brain Res. 1989 Nov 20;502(2):375–386. doi: 10.1016/0006-8993(89)90634-3. [DOI] [PubMed] [Google Scholar]
- Scanlon M., Williams D. A., Fay F. S. A Ca2+-insensitive form of fura-2 associated with polymorphonuclear leukocytes. Assessment and accurate Ca2+ measurement. J Biol Chem. 1987 May 5;262(13):6308–6312. [PubMed] [Google Scholar]
- Silver R. A., Lamb A. G., Bolsover S. R. Elevated cytosolic calcium in the growth cone inhibits neurite elongation in neuroblastoma cells: correlation of behavioral states with cytosolic calcium concentration. J Neurosci. 1989 Nov;9(11):4007–4020. doi: 10.1523/JNEUROSCI.09-11-04007.1989. [DOI] [PMC free article] [PubMed] [Google Scholar]
- Smith S. J., Zucker R. S. Aequorin response facilitation and intracellular calcium accumulation in molluscan neurones. J Physiol. 1980 Mar;300:167–196. doi: 10.1113/jphysiol.1980.sp013157. [DOI] [PMC free article] [PubMed] [Google Scholar]
- Sutko J. L., Ito K., Kenyon J. L. Ryanodine: a modifier of sarcoplasmic reticulum calcium release in striated muscle. Fed Proc. 1985 Dec;44(15):2984–2988. [PubMed] [Google Scholar]
- Thayer S. A., Hirning L. D., Miller R. J. The role of caffeine-sensitive calcium stores in the regulation of the intracellular free calcium concentration in rat sympathetic neurons in vitro. Mol Pharmacol. 1988 Nov;34(5):664–673. [PubMed] [Google Scholar]
- Thayer S. A., Miller R. J. Regulation of the intracellular free calcium concentration in single rat dorsal root ganglion neurones in vitro. J Physiol. 1990 Jun;425:85–115. doi: 10.1113/jphysiol.1990.sp018094. [DOI] [PMC free article] [PubMed] [Google Scholar]
- Walicke P. A., Patterson P. H. On the role of Ca2+ in the transmitter choice made by cultured sympathetic neurons. J Neurosci. 1981 Apr;1(4):343–350. doi: 10.1523/JNEUROSCI.01-04-00343.1981. [DOI] [PMC free article] [PubMed] [Google Scholar]
- Williams D. A., Fogarty K. E., Tsien R. Y., Fay F. S. Calcium gradients in single smooth muscle cells revealed by the digital imaging microscope using Fura-2. Nature. 1985 Dec 12;318(6046):558–561. doi: 10.1038/318558a0. [DOI] [PubMed] [Google Scholar]
- Young S. H., Poo M. M. Spontaneous release of transmitter from growth cones of embryonic neurones. Nature. 1983 Oct 13;305(5935):634–637. doi: 10.1038/305634a0. [DOI] [PubMed] [Google Scholar]