Abstract
1. Experiments were done to test the hypothesis that parvalbumin (PA) promotes relaxation in frog skeletal muscle. Single fibres and purified PA from Rana temporaria skeletal muscle were used to determine the relationship between PA concentration ( [PA] ), Ca2+ and Mg2+ dissociation rates from PA and changes in rate of relaxation as a function of tetanus duration at 0 degrees C. 2. Total [PA] in fibres from tibialis anterior muscles is 0.76 +/- 0.20 mmol PA l-1 myoplasmic water (mean +/- S.D., n = 25) with 65% PA IVa and 35% PA IVb, where PA IVa and PA IVb are PA isoforms. 3. Relaxation rate from an isometric tetanus shows progressively as a function of tetanus duration with an exponential time course and a rate constant of 1.18 +/- 0.35 s-1 (n = 17). Time course of recovery of relaxation rate after a prolonged tetanus is exponential with a rate constant of 0.12 +/- 0.02 s-1 (n = 14). 4. The extent of recovery of relaxation rate after a prolonged tetanus was correlated with total [PA] in fibres (correlation coefficient (r) = 0.80, n = 7; P less than 0.05). 5. Dissociation rate constants for Mg2+ and Ca2+ from purified PA are 0.93 +/- 0.02 s-1 (n = 5) and 0.19 +/- 0.01 s-1 (n = 5), respectively. Dissociation rate constants were not significantly different for PA isoforms IVa and IVb. These rate constants are similar to the rate constants determined for the time courses of slowing and recovery of relaxation rate, respectively. 6. Results suggest that the time courses of slowing and recovery of relaxation rate may be controlled, to a large extent, by Mg2+ and Ca2+ dissociation from PA, respectively. This evidence supports a role for PA in facilitating relaxation during a tetanus in frog skeletal muscle at 0 degrees C.
Full text
PDF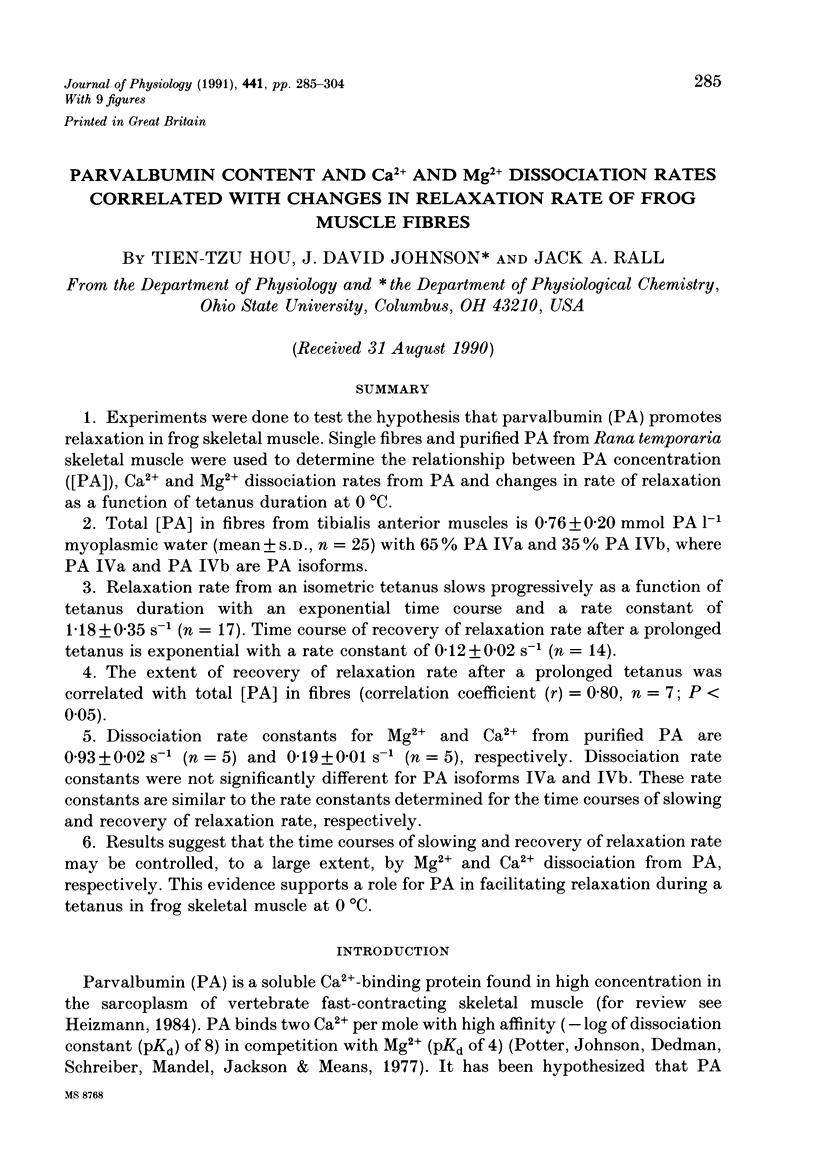
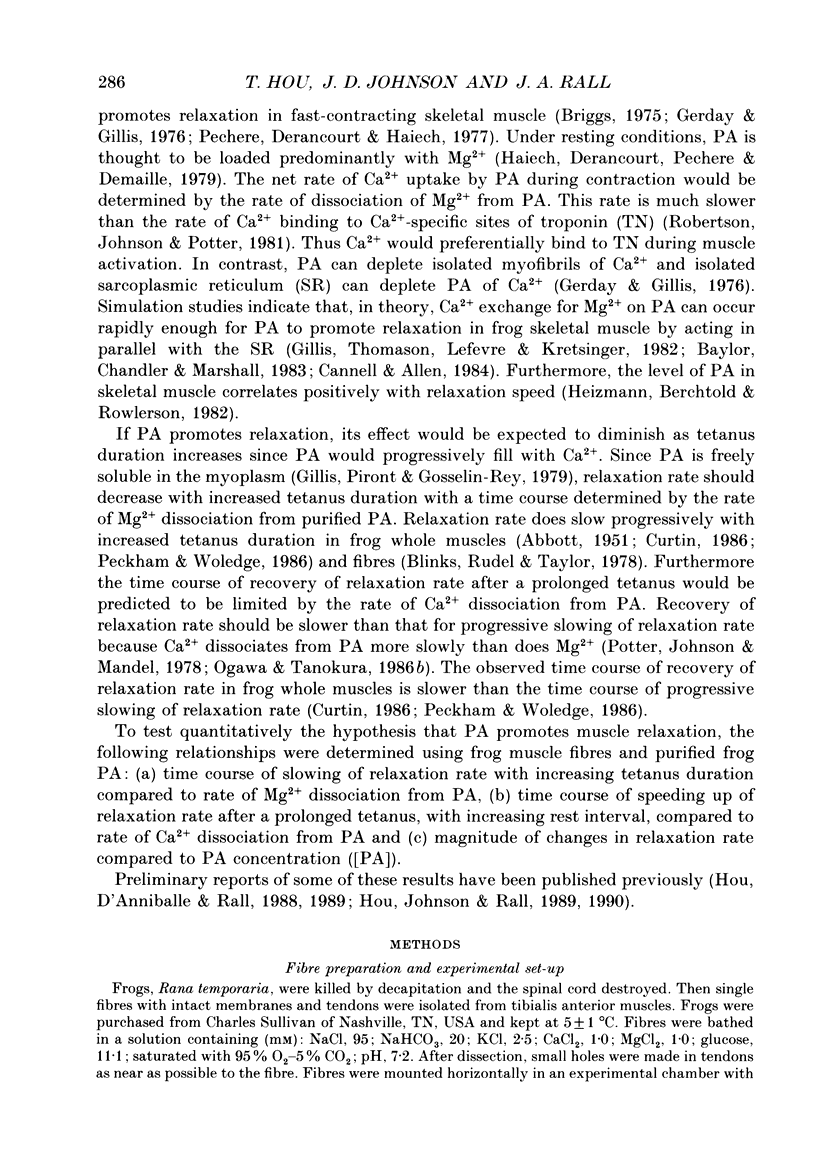
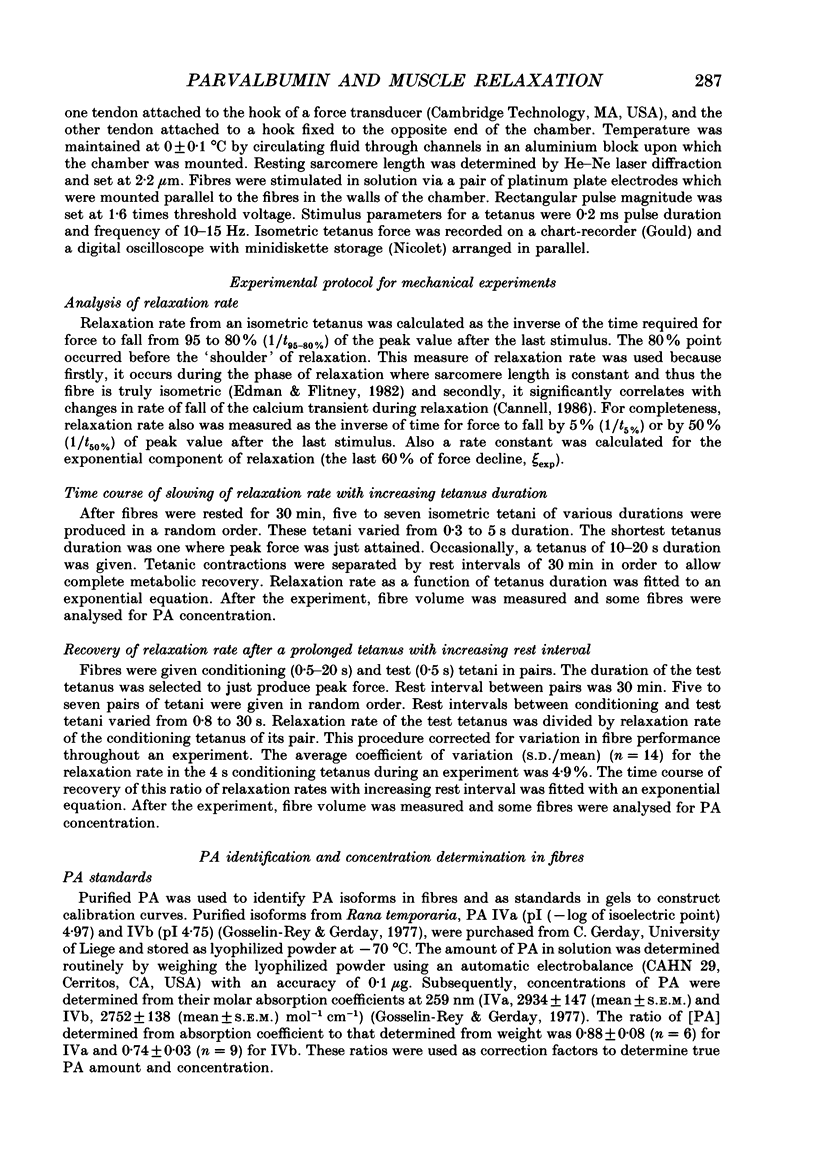
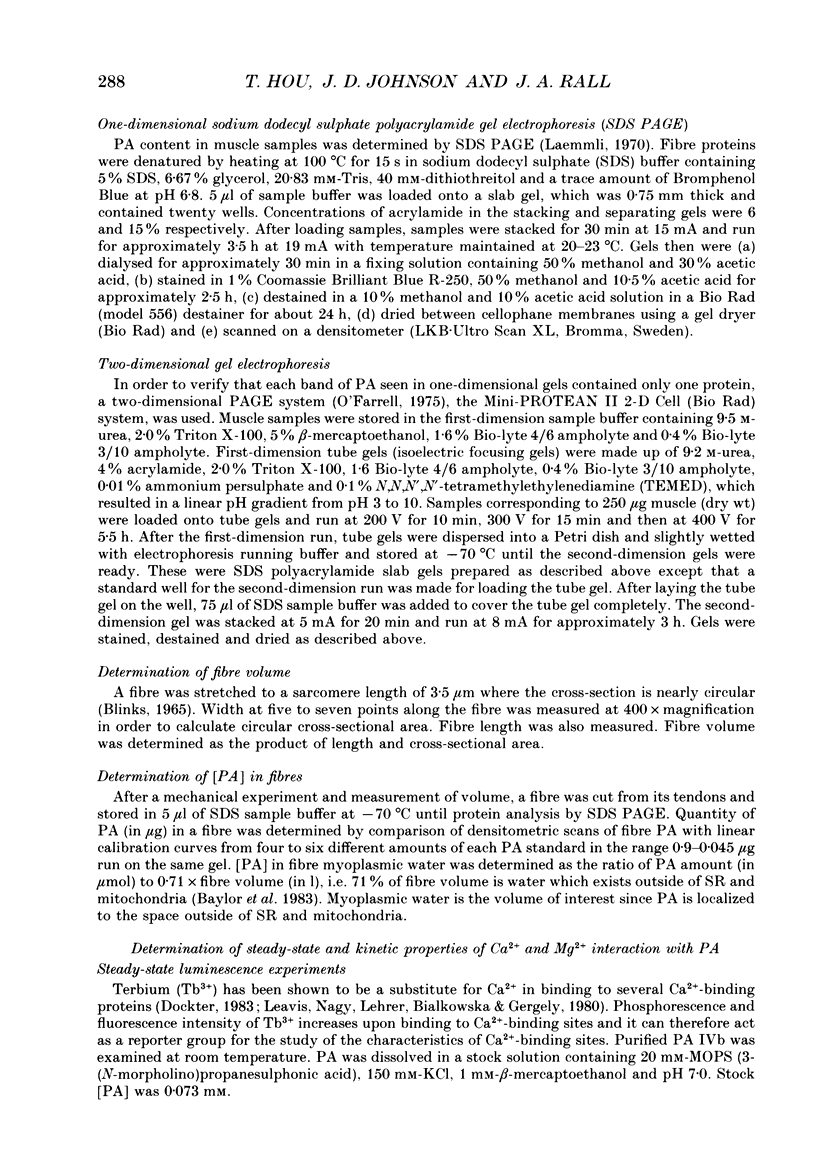
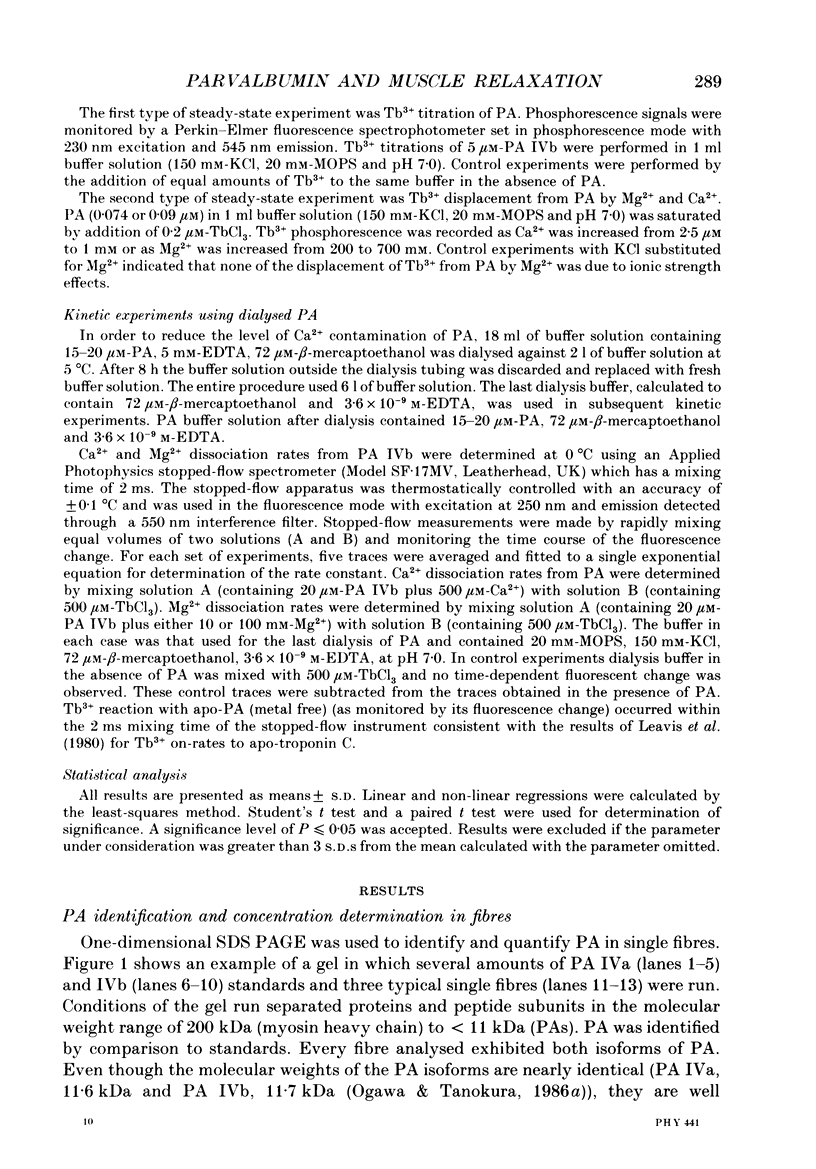
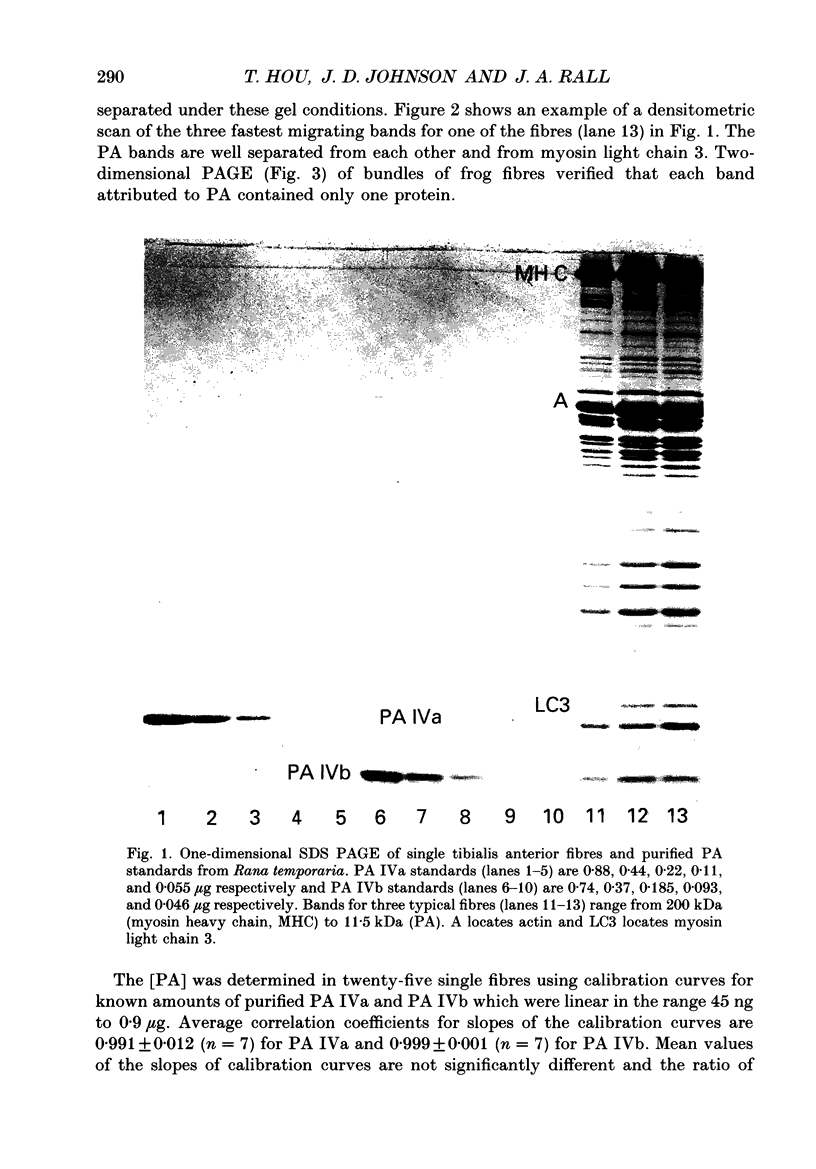
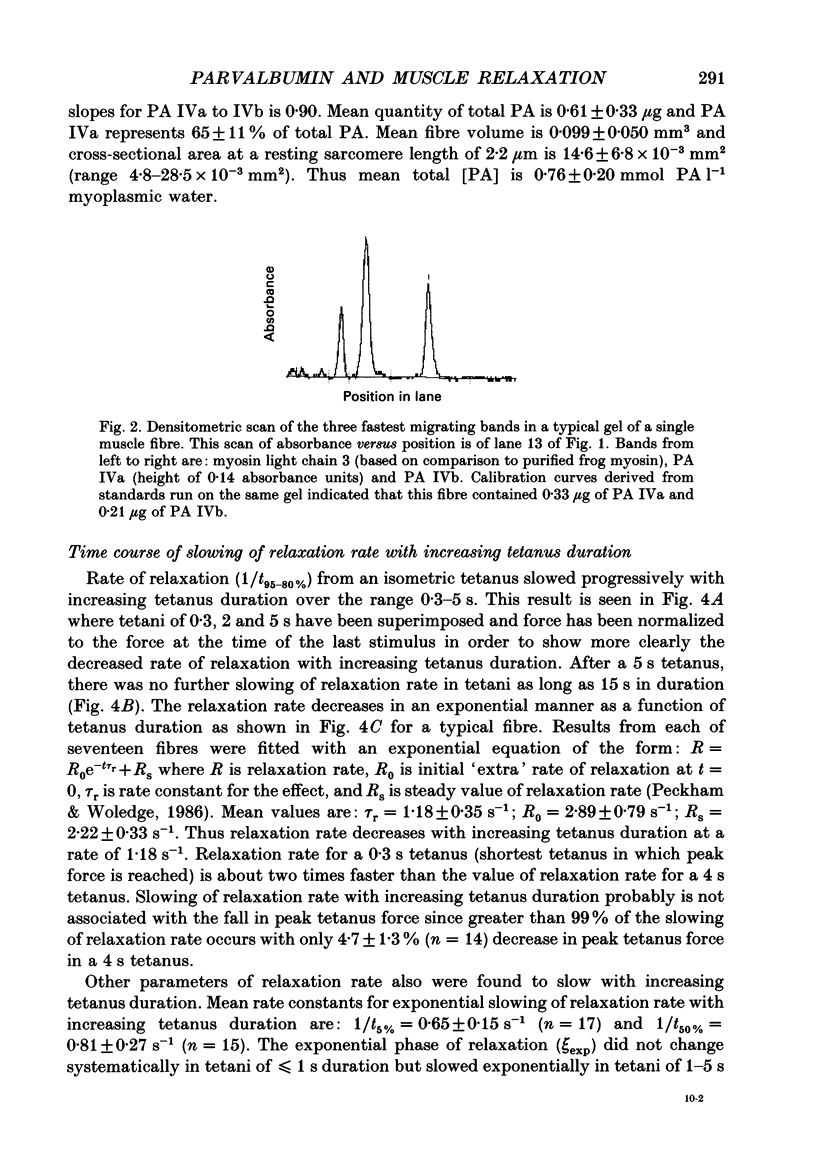
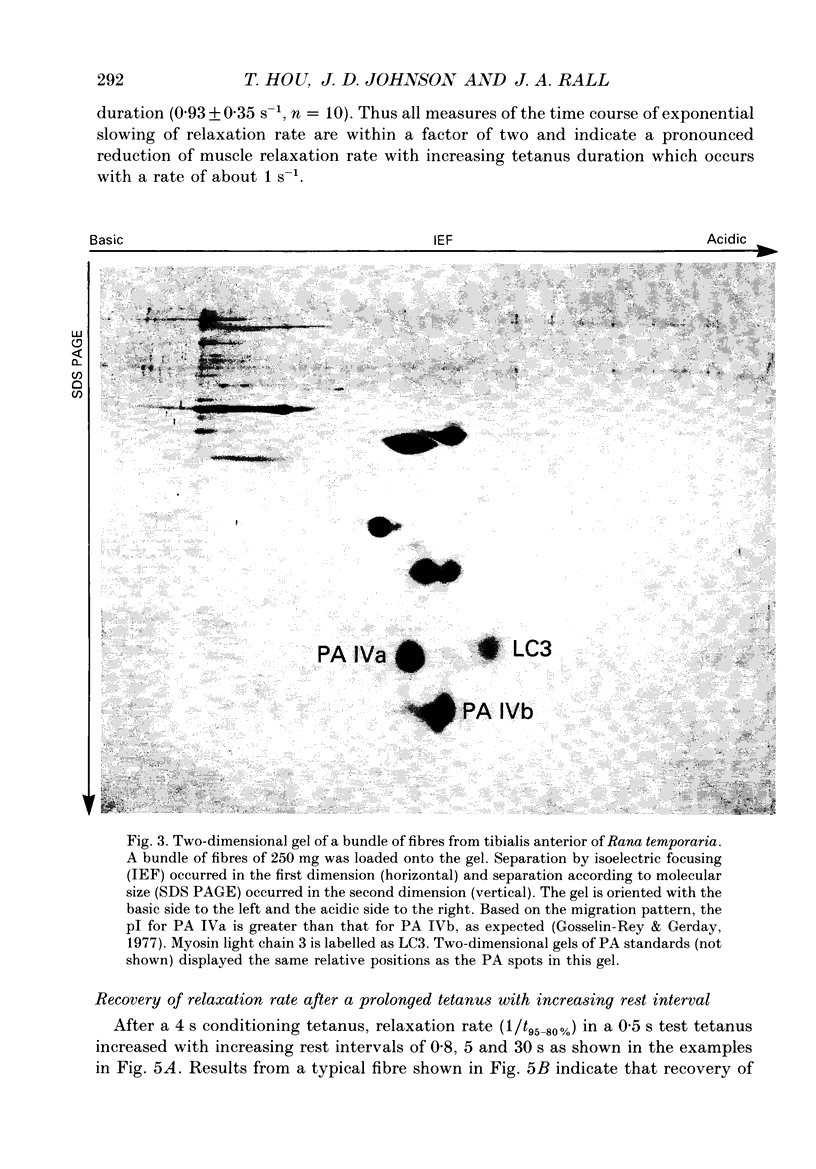
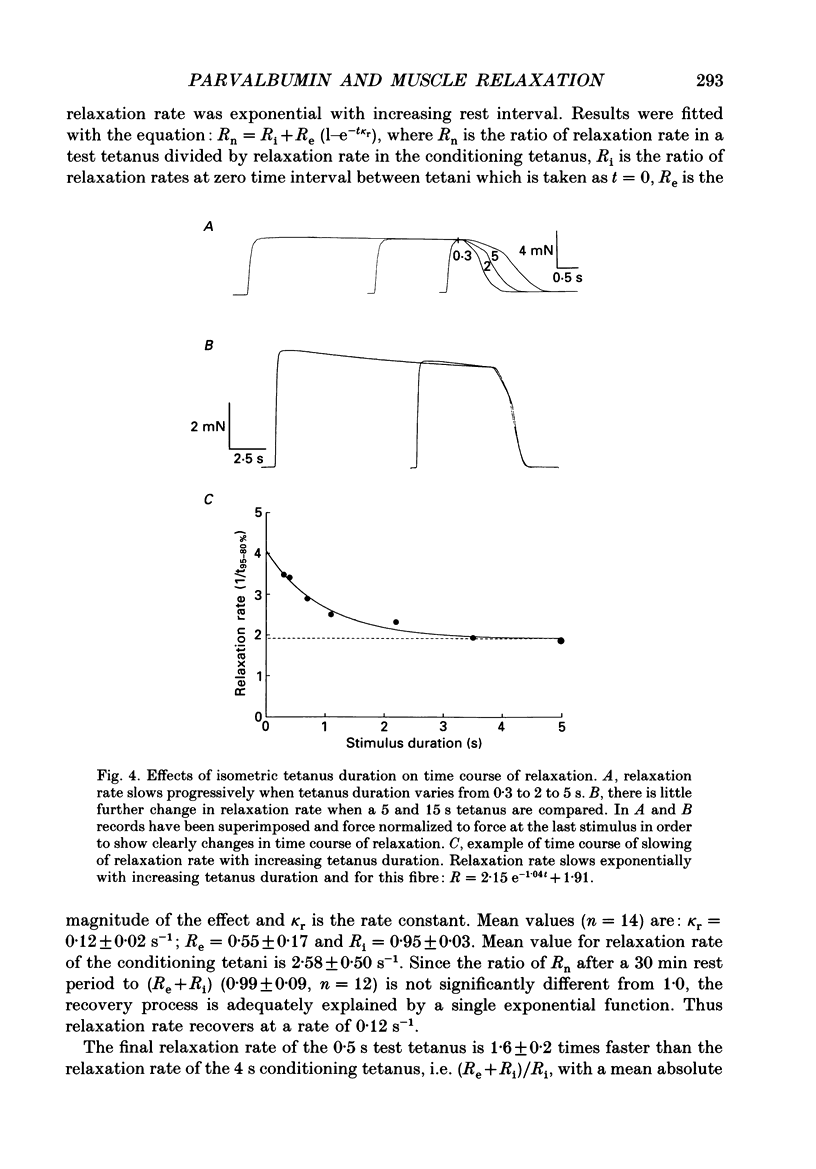
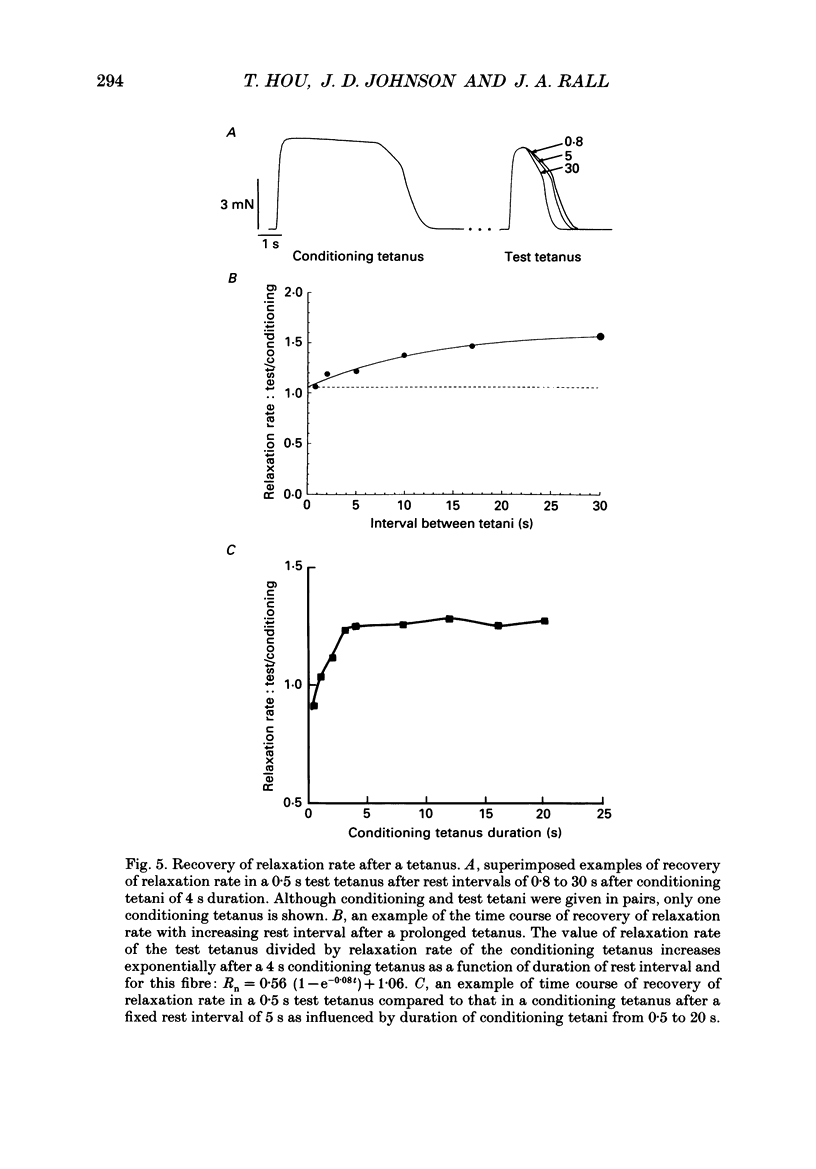
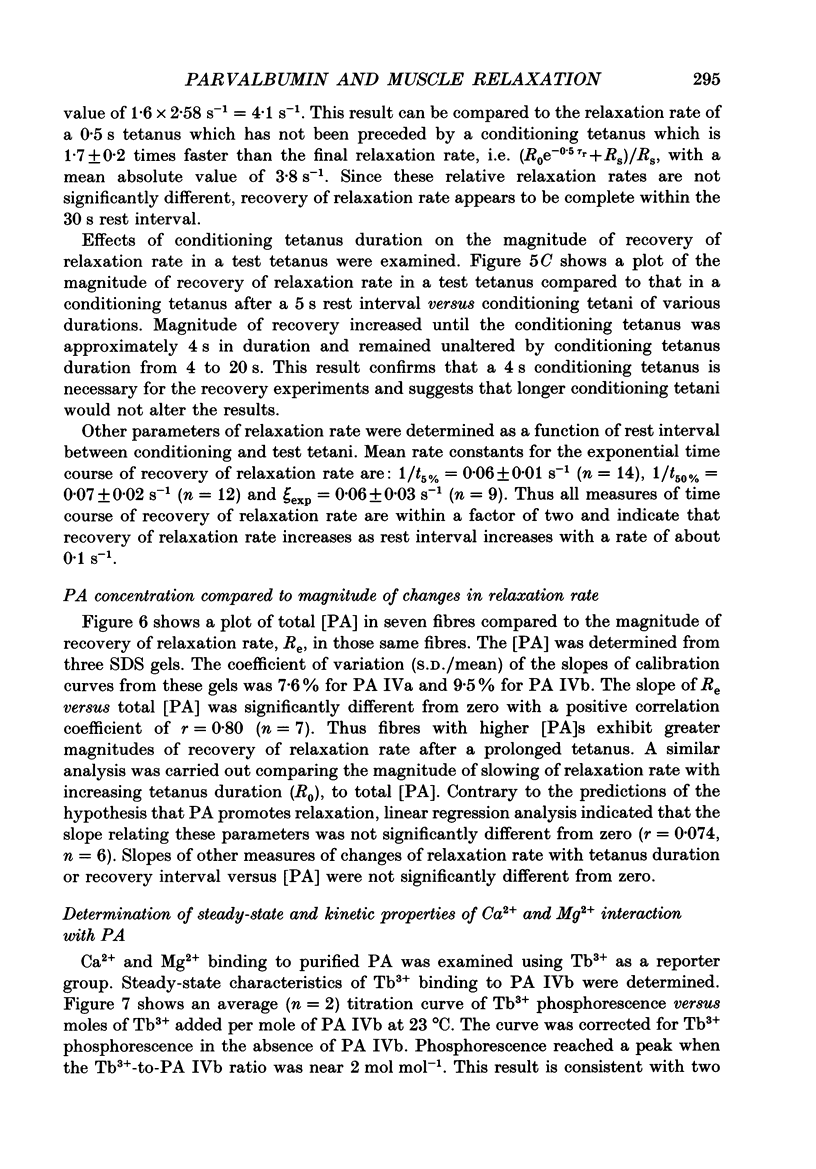
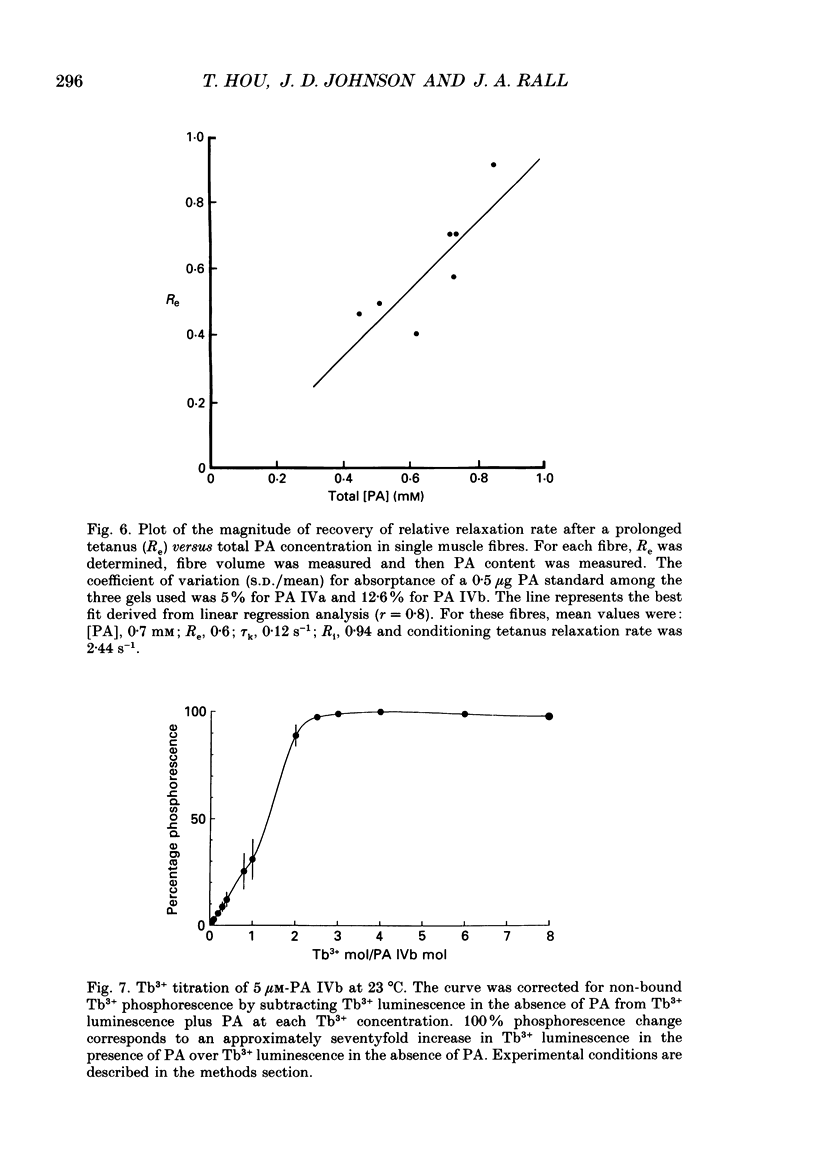
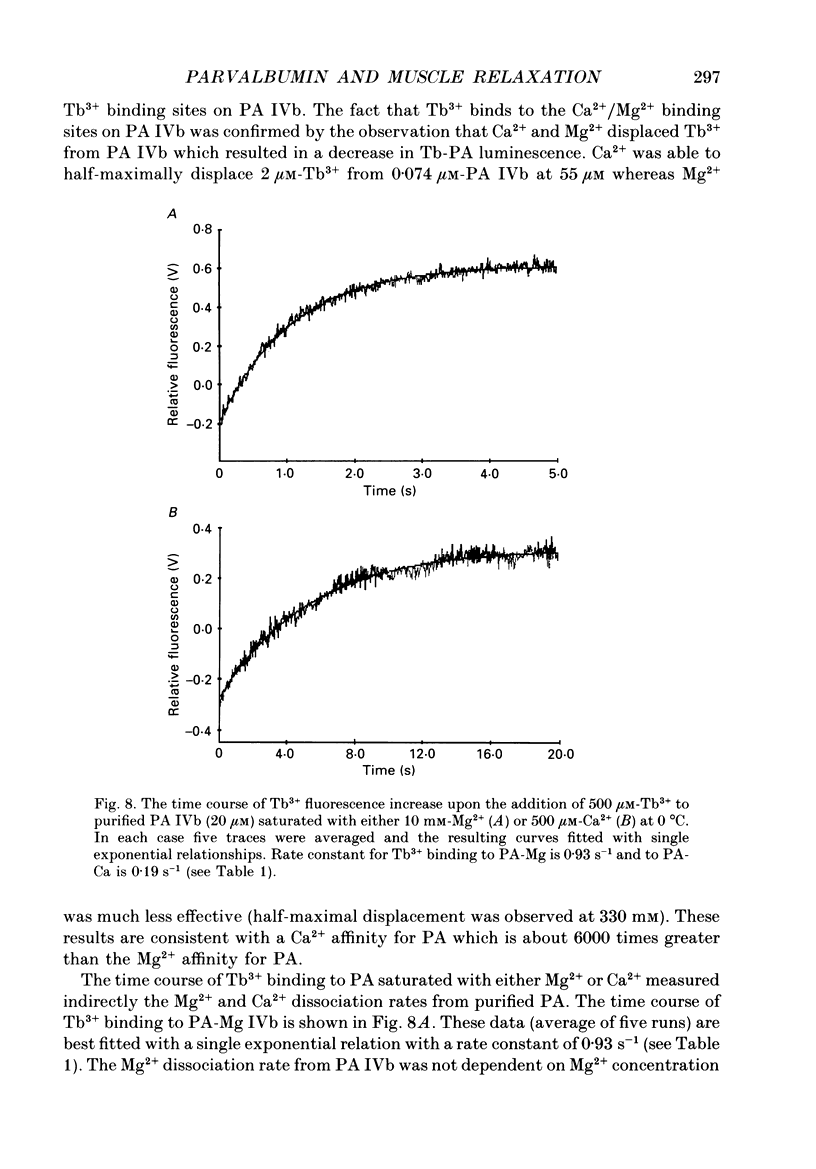
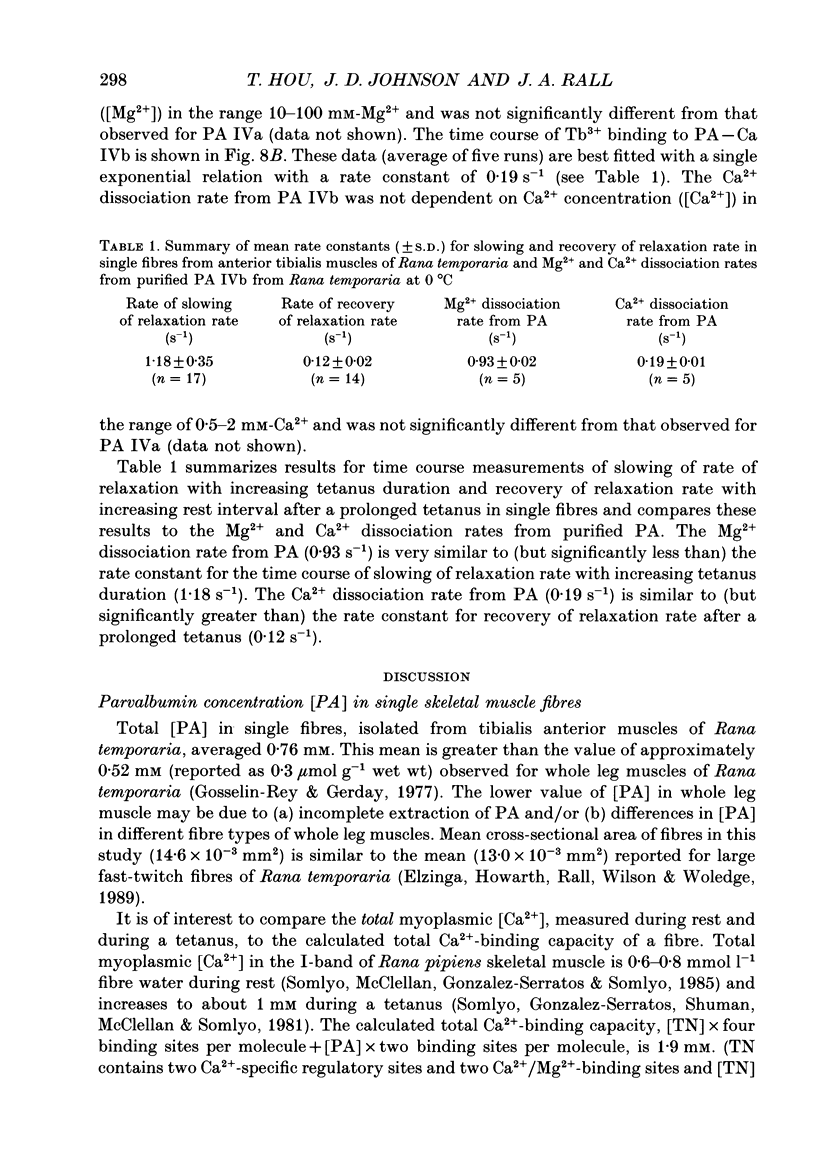
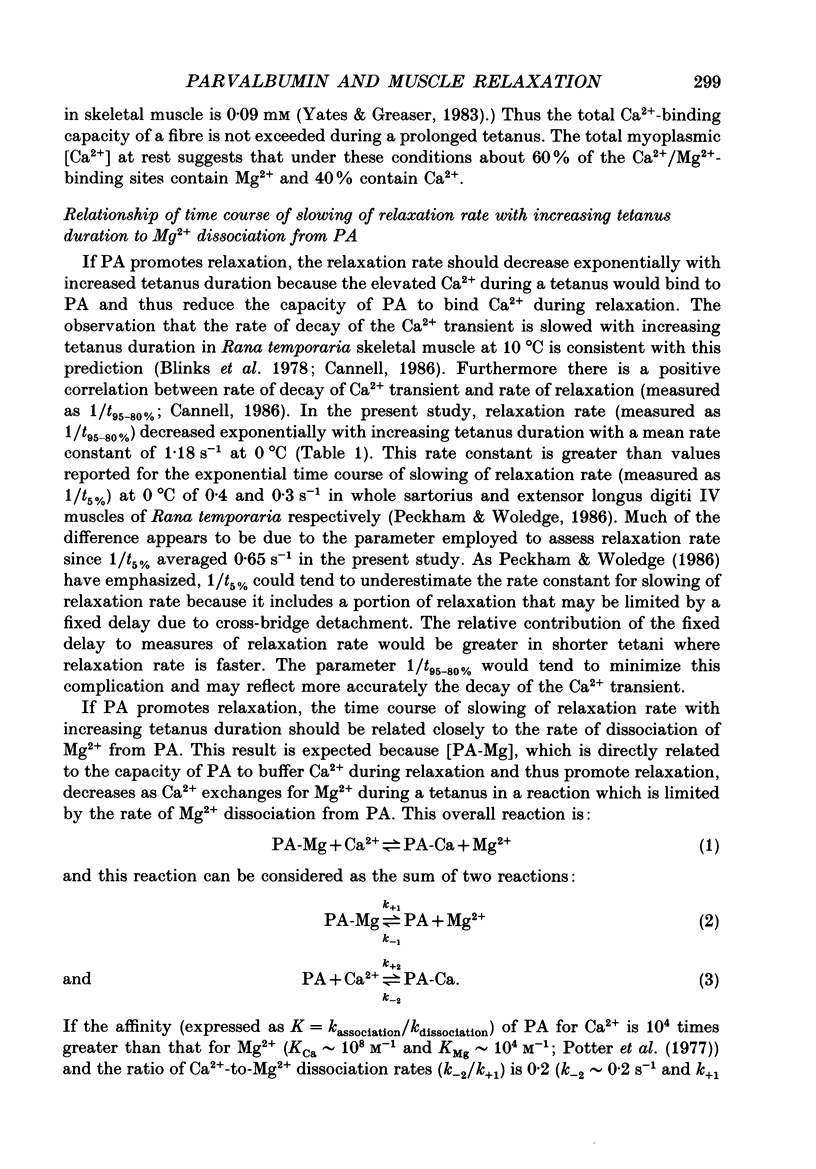
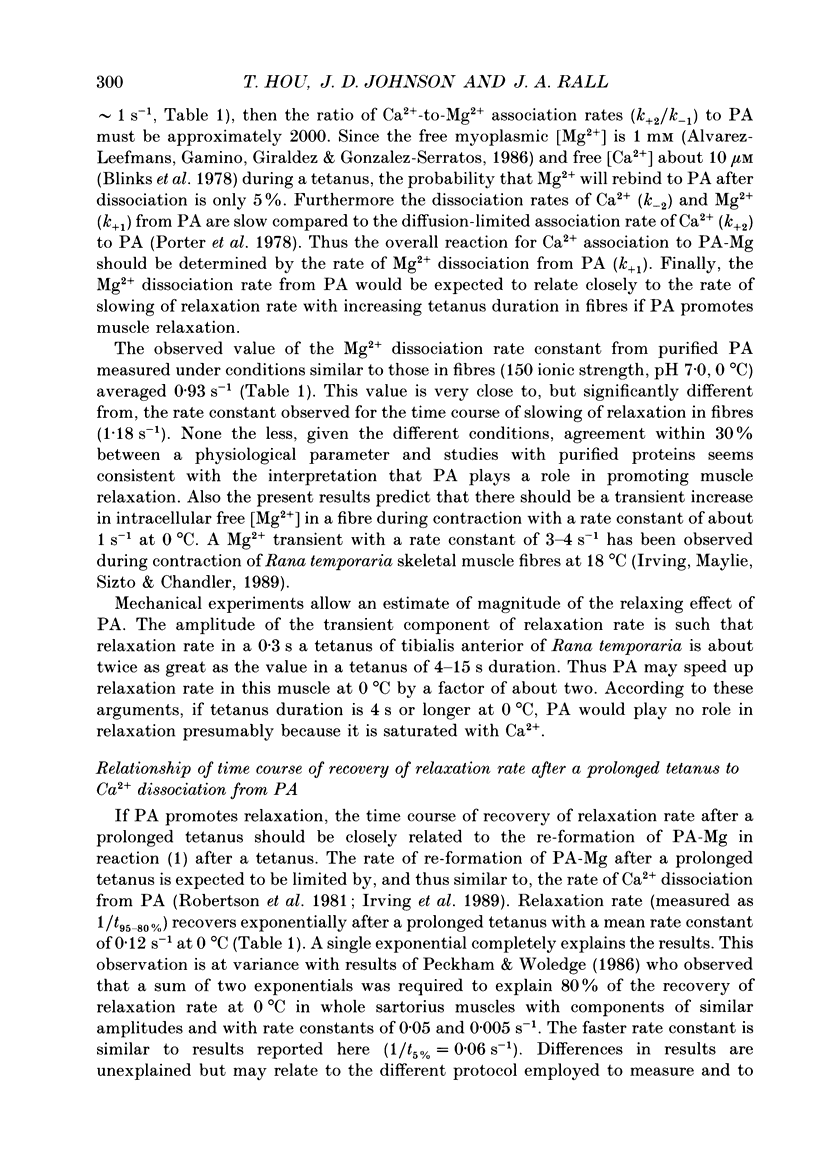
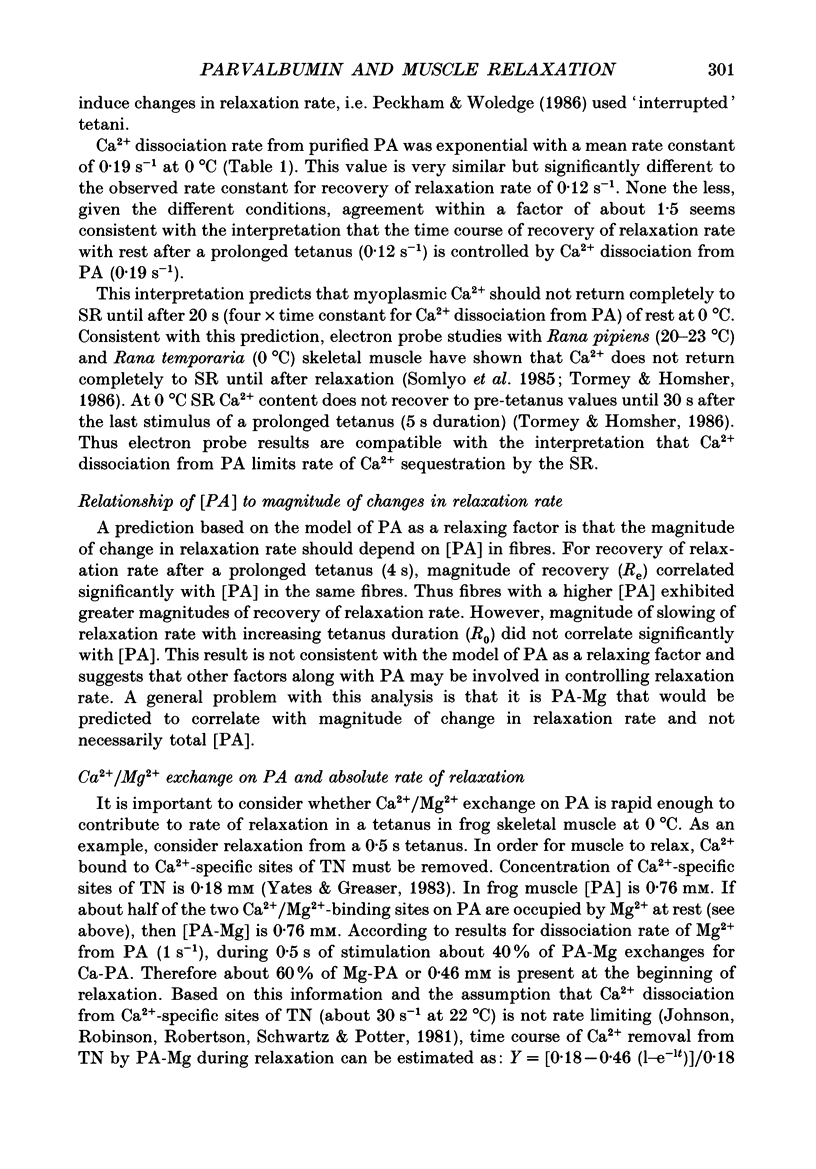
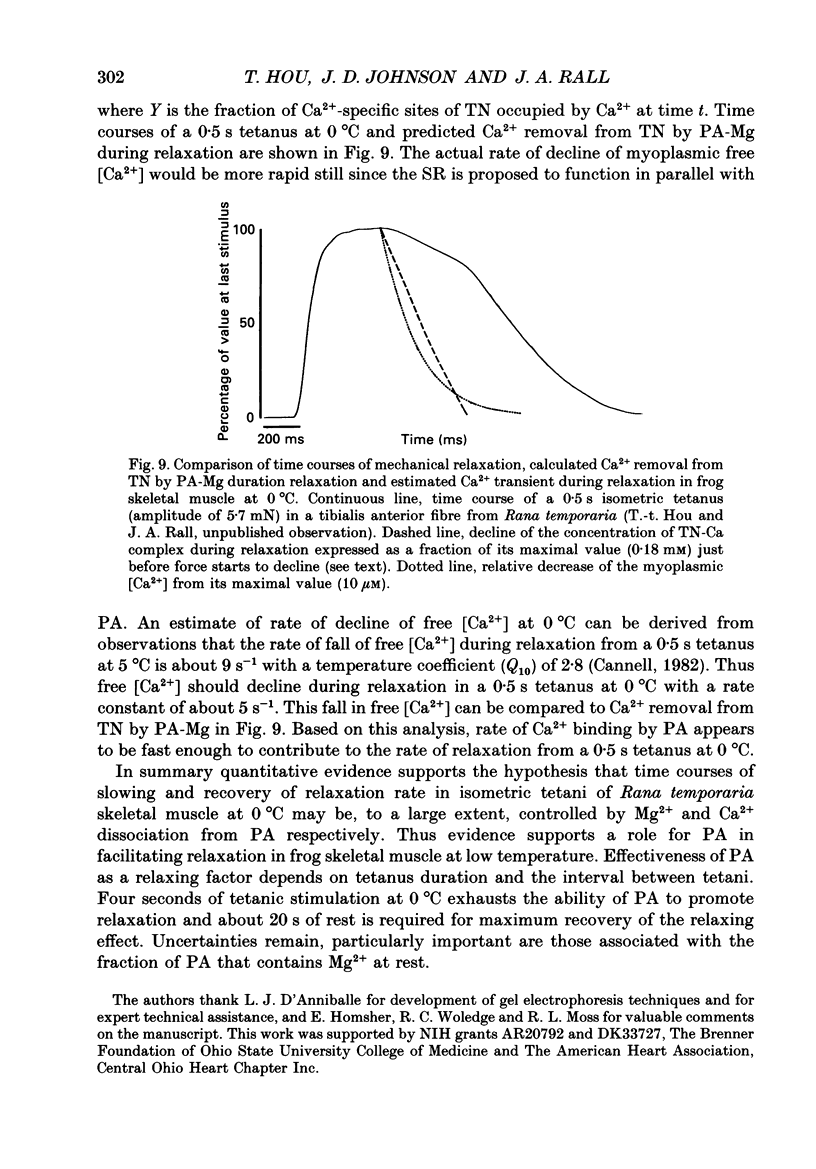
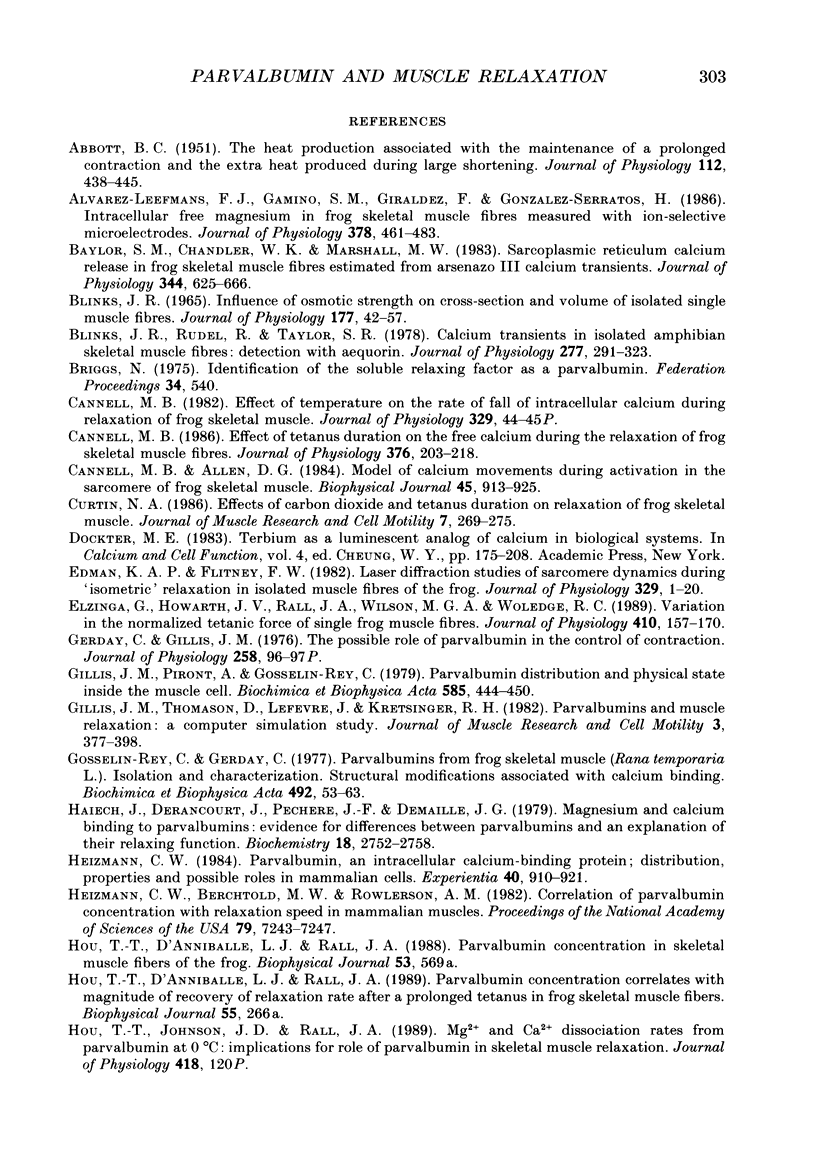
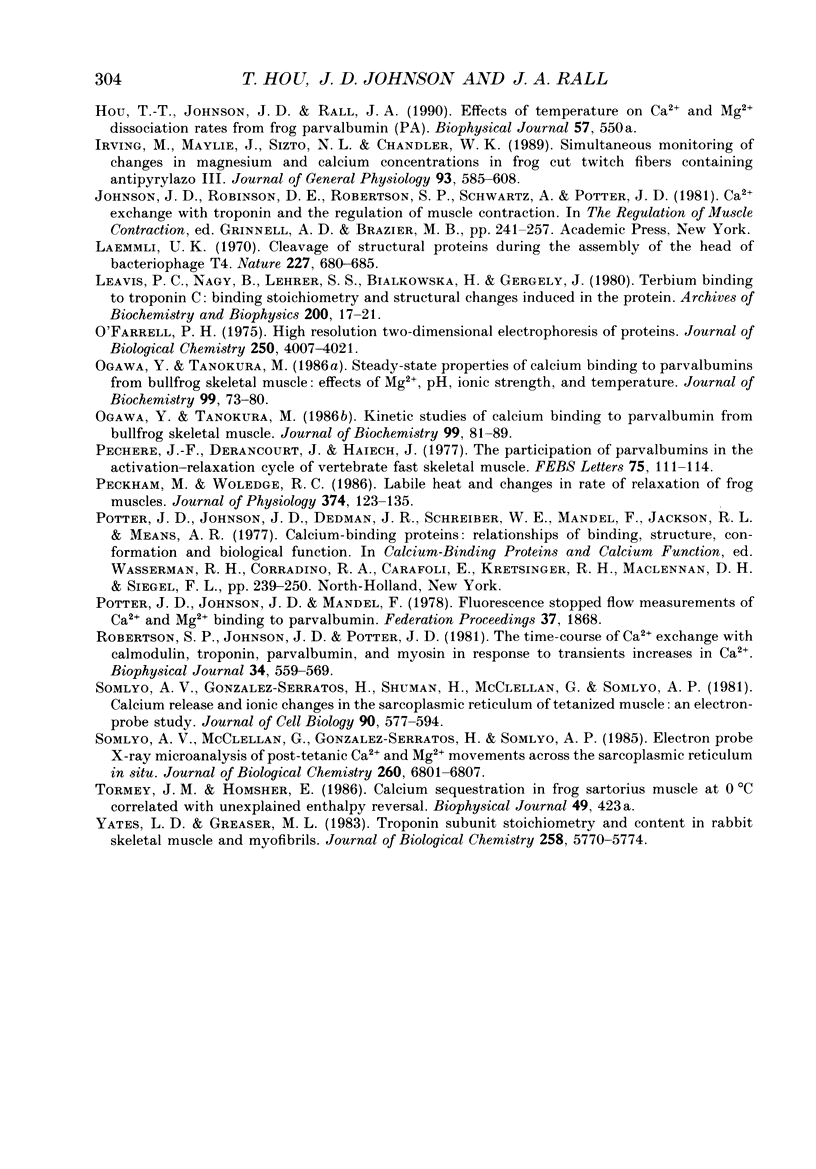
Images in this article
Selected References
These references are in PubMed. This may not be the complete list of references from this article.
- ABBOTT B. C. The heat production associated with the maintenance of a prolonged contraction and the extra heat produced during large shortening. J Physiol. 1951 Feb;112(3-4):438–445. doi: 10.1113/jphysiol.1951.sp004541. [DOI] [PMC free article] [PubMed] [Google Scholar]
- Alvarez-Leefmans F. J., Gamiño S. M., Giraldez F., González-Serratos H. Intracellular free magnesium in frog skeletal muscle fibres measured with ion-selective micro-electrodes. J Physiol. 1986 Sep;378:461–483. doi: 10.1113/jphysiol.1986.sp016230. [DOI] [PMC free article] [PubMed] [Google Scholar]
- BLINKS J. R. INFLUENCE OF OSMOTIC STRENGTH ON CROSS-SECTION AND VOLUME OF ISOLATED SINGLE MUSCLE FIBRES. J Physiol. 1965 Mar;177:42–57. doi: 10.1113/jphysiol.1965.sp007574. [DOI] [PMC free article] [PubMed] [Google Scholar]
- Baylor S. M., Chandler W. K., Marshall M. W. Sarcoplasmic reticulum calcium release in frog skeletal muscle fibres estimated from Arsenazo III calcium transients. J Physiol. 1983 Nov;344:625–666. doi: 10.1113/jphysiol.1983.sp014959. [DOI] [PMC free article] [PubMed] [Google Scholar]
- Blinks J. R., Rüdel R., Taylor S. R. Calcium transients in isolated amphibian skeletal muscle fibres: detection with aequorin. J Physiol. 1978 Apr;277:291–323. doi: 10.1113/jphysiol.1978.sp012273. [DOI] [PMC free article] [PubMed] [Google Scholar]
- Cannell M. B., Allen D. G. Model of calcium movements during activation in the sarcomere of frog skeletal muscle. Biophys J. 1984 May;45(5):913–925. doi: 10.1016/S0006-3495(84)84238-1. [DOI] [PMC free article] [PubMed] [Google Scholar]
- Cannell M. B. Effect of tetanus duration on the free calcium during the relaxation of frog skeletal muscle fibres. J Physiol. 1986 Jul;376:203–218. doi: 10.1113/jphysiol.1986.sp016149. [DOI] [PMC free article] [PubMed] [Google Scholar]
- Curtin N. A. Effects of carbon dioxide and tetanus duration on relaxation of frog skeletal muscle. J Muscle Res Cell Motil. 1986 Jun;7(3):269–275. doi: 10.1007/BF01753560. [DOI] [PubMed] [Google Scholar]
- Edman K. A., Flitney F. W. Laser diffraction studies of sarcomere dynamics during 'isometric' relaxation in isolated muscle fibres of the frog. J Physiol. 1982 Aug;329:1–20. doi: 10.1113/jphysiol.1982.sp014287. [DOI] [PMC free article] [PubMed] [Google Scholar]
- Elzinga G., Howarth J. V., Rall J. A., Wilson M. G., Woledge R. C. Variation in the normalized tetanic force of single frog muscle fibres. J Physiol. 1989 Mar;410:157–170. doi: 10.1113/jphysiol.1989.sp017526. [DOI] [PMC free article] [PubMed] [Google Scholar]
- Gillis J. M., Piront A., Gosselin-Rey C. Parvalbumins. Distribution and physical state inside the muscle cell. Biochim Biophys Acta. 1979 Jul 4;585(3):444–450. doi: 10.1016/0304-4165(79)90089-8. [DOI] [PubMed] [Google Scholar]
- Gillis J. M., Thomason D., Lefèvre J., Kretsinger R. H. Parvalbumins and muscle relaxation: a computer simulation study. J Muscle Res Cell Motil. 1982 Dec;3(4):377–398. doi: 10.1007/BF00712090. [DOI] [PubMed] [Google Scholar]
- Gosselin-rey C., Gerday C. Parvalbumins from frog skeletal muscle (Rana temporaria L.). Isolation and characterization. Structural modifications associated with calcium binding. Biochim Biophys Acta. 1977 May 27;492(1):53–63. doi: 10.1016/0005-2795(77)90213-6. [DOI] [PubMed] [Google Scholar]
- Haiech J., Derancourt J., Pechère J. F., Demaille J. G. Magnesium and calcium binding to parvalbumins: evidence for differences between parvalbumins and an explanation of their relaxing function. Biochemistry. 1979 Jun 26;18(13):2752–2758. doi: 10.1021/bi00580a010. [DOI] [PubMed] [Google Scholar]
- Heizmann C. W., Berchtold M. W., Rowlerson A. M. Correlation of parvalbumin concentration with relaxation speed in mammalian muscles. Proc Natl Acad Sci U S A. 1982 Dec;79(23):7243–7247. doi: 10.1073/pnas.79.23.7243. [DOI] [PMC free article] [PubMed] [Google Scholar]
- Heizmann C. W. Parvalbumin, an intracellular calcium-binding protein; distribution, properties and possible roles in mammalian cells. Experientia. 1984 Sep 15;40(9):910–921. doi: 10.1007/BF01946439. [DOI] [PubMed] [Google Scholar]
- Irving M., Maylie J., Sizto N. L., Chandler W. K. Simultaneous monitoring of changes in magnesium and calcium concentrations in frog cut twitch fibers containing antipyrylazo III. J Gen Physiol. 1989 Apr;93(4):585–608. doi: 10.1085/jgp.93.4.585. [DOI] [PMC free article] [PubMed] [Google Scholar]
- Laemmli U. K. Cleavage of structural proteins during the assembly of the head of bacteriophage T4. Nature. 1970 Aug 15;227(5259):680–685. doi: 10.1038/227680a0. [DOI] [PubMed] [Google Scholar]
- Leavis P. C., Nagy B., Lehrer S. S., Bialkowska H., Gergely J. Terbium binding to troponin C: binding stoichiometry and structural changes induced in the protein. Arch Biochem Biophys. 1980 Mar;200(1):17–21. doi: 10.1016/0003-9861(80)90324-0. [DOI] [PubMed] [Google Scholar]
- O'Farrell P. H. High resolution two-dimensional electrophoresis of proteins. J Biol Chem. 1975 May 25;250(10):4007–4021. [PMC free article] [PubMed] [Google Scholar]
- Ogawa Y., Tanokura M. Kinetic studies of calcium binding to parvalbumins from bullfrog skeletal muscle. J Biochem. 1986 Jan;99(1):81–89. doi: 10.1093/oxfordjournals.jbchem.a135482. [DOI] [PubMed] [Google Scholar]
- Ogawa Y., Tanokura M. Steady-state properties of calcium binding to parvalbumins from bullfrog skeletal muscle: effects of Mg2+, pH, ionic strength, and temperature. J Biochem. 1986 Jan;99(1):73–80. doi: 10.1093/oxfordjournals.jbchem.a135481. [DOI] [PubMed] [Google Scholar]
- Pechère J. F., Derancourt J., Haiech J. The participation of parvalbumins in the activation-relaxation cycle of vertebrate fast skeletal-muscle. FEBS Lett. 1977 Mar 15;75(1):111–114. doi: 10.1016/0014-5793(77)80064-1. [DOI] [PubMed] [Google Scholar]
- Peckham M., Woledge R. C. Labile heat and changes in rate of relaxation of frog muscles. J Physiol. 1986 May;374:123–135. doi: 10.1113/jphysiol.1986.sp016070. [DOI] [PMC free article] [PubMed] [Google Scholar]
- Robertson S. P., Johnson J. D., Potter J. D. The time-course of Ca2+ exchange with calmodulin, troponin, parvalbumin, and myosin in response to transient increases in Ca2+. Biophys J. 1981 Jun;34(3):559–569. doi: 10.1016/S0006-3495(81)84868-0. [DOI] [PMC free article] [PubMed] [Google Scholar]
- Somlyo A. V., Gonzalez-Serratos H. G., Shuman H., McClellan G., Somlyo A. P. Calcium release and ionic changes in the sarcoplasmic reticulum of tetanized muscle: an electron-probe study. J Cell Biol. 1981 Sep;90(3):577–594. doi: 10.1083/jcb.90.3.577. [DOI] [PMC free article] [PubMed] [Google Scholar]
- Somlyo A. V., McClellan G., Gonzalez-Serratos H., Somlyo A. P. Electron probe X-ray microanalysis of post-tetanic Ca2+ and Mg2+ movements across the sarcoplasmic reticulum in situ. J Biol Chem. 1985 Jun 10;260(11):6801–6807. [PubMed] [Google Scholar]
- Yates L. D., Greaser M. L. Troponin subunit stoichiometry and content in rabbit skeletal muscle and myofibrils. J Biol Chem. 1983 May 10;258(9):5770–5774. [PubMed] [Google Scholar]