Abstract
1. The mechanism of post-ischaemic ectopic impulse generation in nerve is not known, and previous measurements of excitability changes in human motor axons have appeared to conflict. We have used automatic threshold tracking and different stimulus-response combinations to follow the effects on excitability of brief (5-10 min) periods of ischaemia, too short to induce motor fasciculations. Excitability changes have been compared at different sites in axons innervating hand, arm and foot muscles. 2. Threshold was determined as the percutaneous stimulus current required to excite a single motor unit, or to evoke a constant multiunit response, after rectifying and integrating the electromyogram (EMG). Three different waveforms of stimulus current were compared: short (less than or equal to 2 ms) pulses, long (100-200 ms) pulses to measure rheobase, and 100 ms current ramps. We also measured accommodation by recording the effects of subthreshold depolarizing currents on excitability. 3. Ischaemic and post-ischaemic excitability changes were greatest in the proximal parts of the longest motor axons, and greater if the sphygmomanometer cuff was inflated over, rather than proximal to, the stimulating site. 4. Using integrated EMG responses from abductor digiti minimi, the ulnar nerve stimulated above the elbow became rapidly much less excitable after ischaemia when tested with short pulses, but more excitable when tested with current ramps. The rheobase rose briefly, but then fell, often below resting level, always staying below the pulse and ramp thresholds. 5. The latency of the response to a rheobasic stimulus altered in parallel with the threshold to short current pulses, and increased dramatically after ischaemia. This latency increase was associated with a prolonged phase of 'negative accommodation', i.e. the continued increase in excitability to a maintained subthreshold depolarizing current. 6. Changes in excitability and accommodation similar to those occurring after ischaemia were recorded following high frequency trains of stimuli. They were attributed primarily to hyperpolarization by the electrogenic sodium pump, since comparable changes could be induced by passing a steady hyperpolarizing current through the stimulating electrode. 7. Threshold and latency recordings from single motor units during and after ischaemia resembled in most respects the multiunit responses, but single unit rheobase did not show a post-ischaemic fall below the resting level. Repetitive firing contributed to the low multiunit thresholds recorded with long current pulses during the post-ischaemic period. 8. We conclude that human motor nerves become simultaneously both more and less excitable than normal after 10 min of ischaemia, depending on the choice of stimulus and response.(ABSTRACT TRUNCATED AT 400 WORDS)
Full text
PDF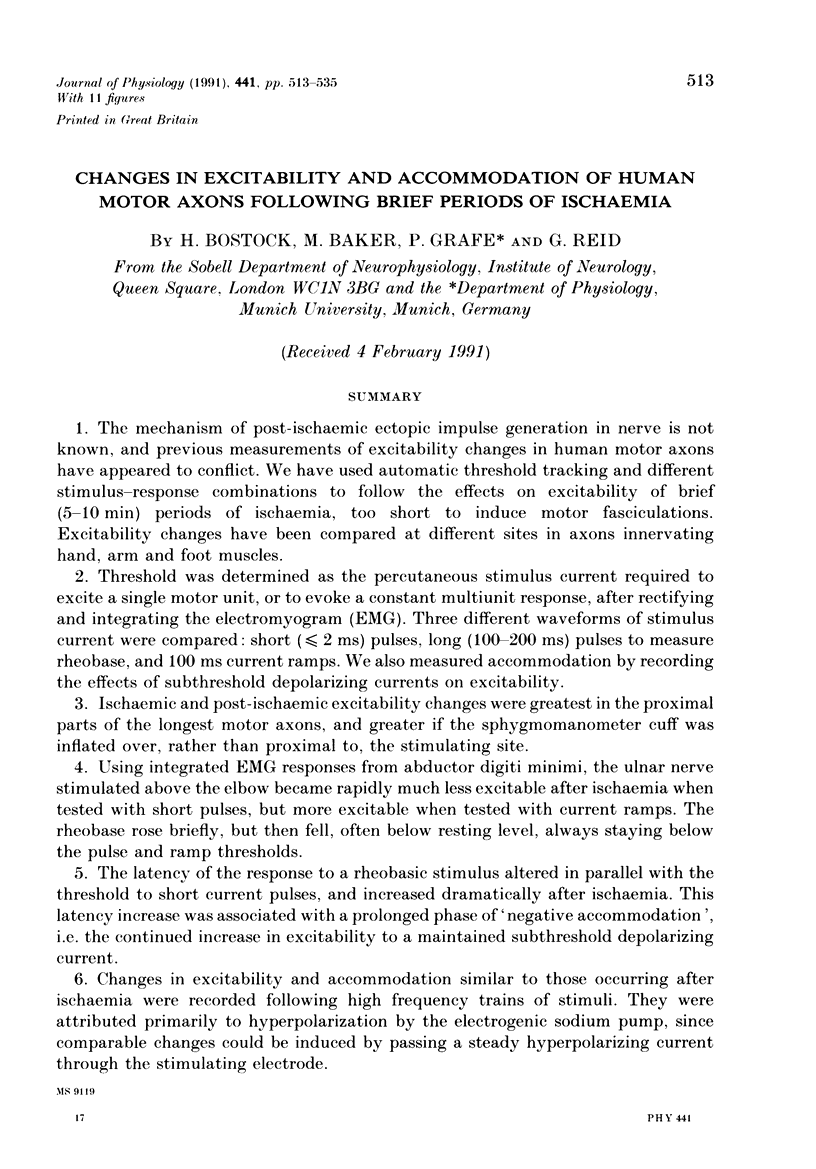
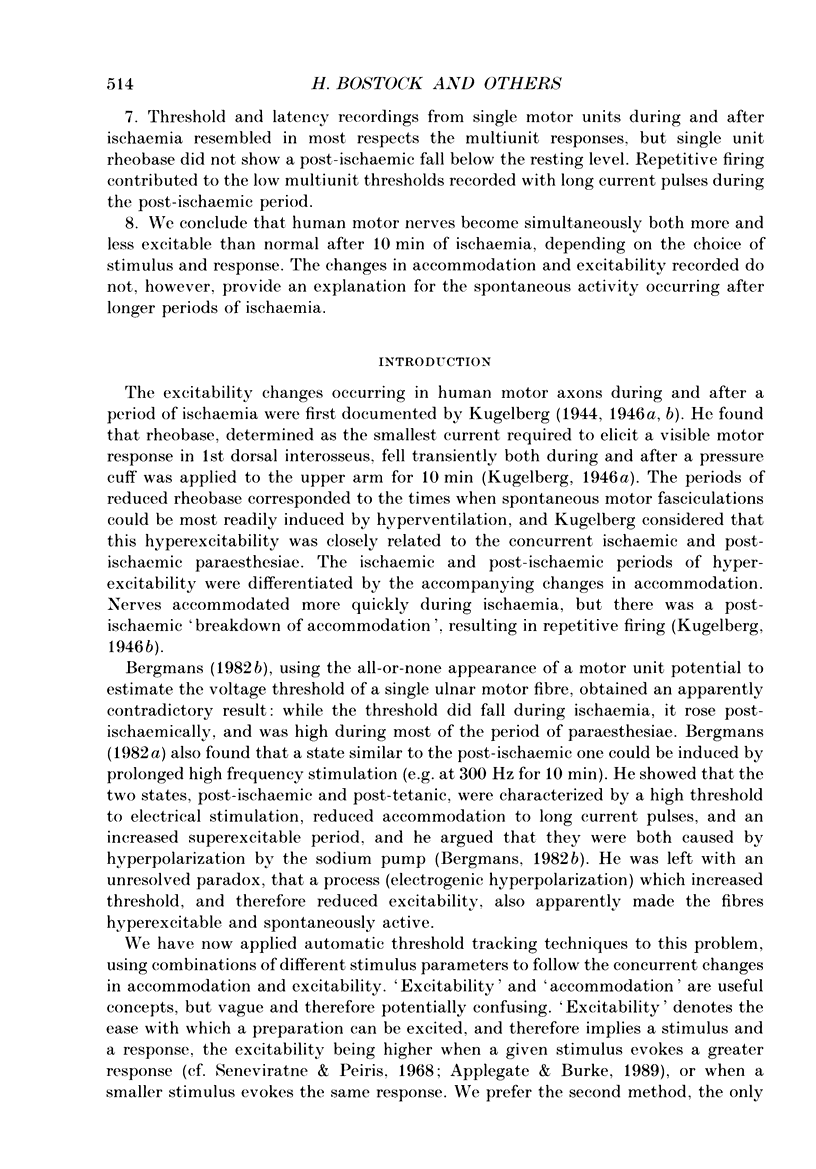
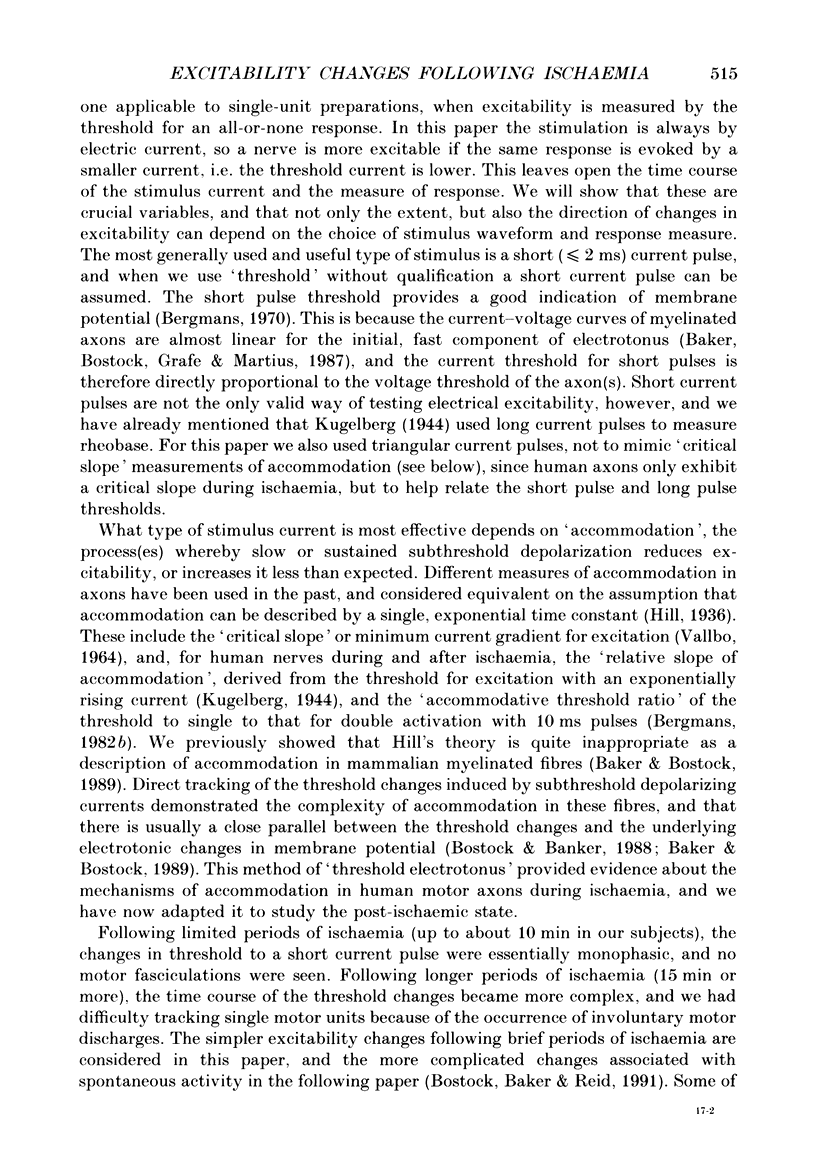
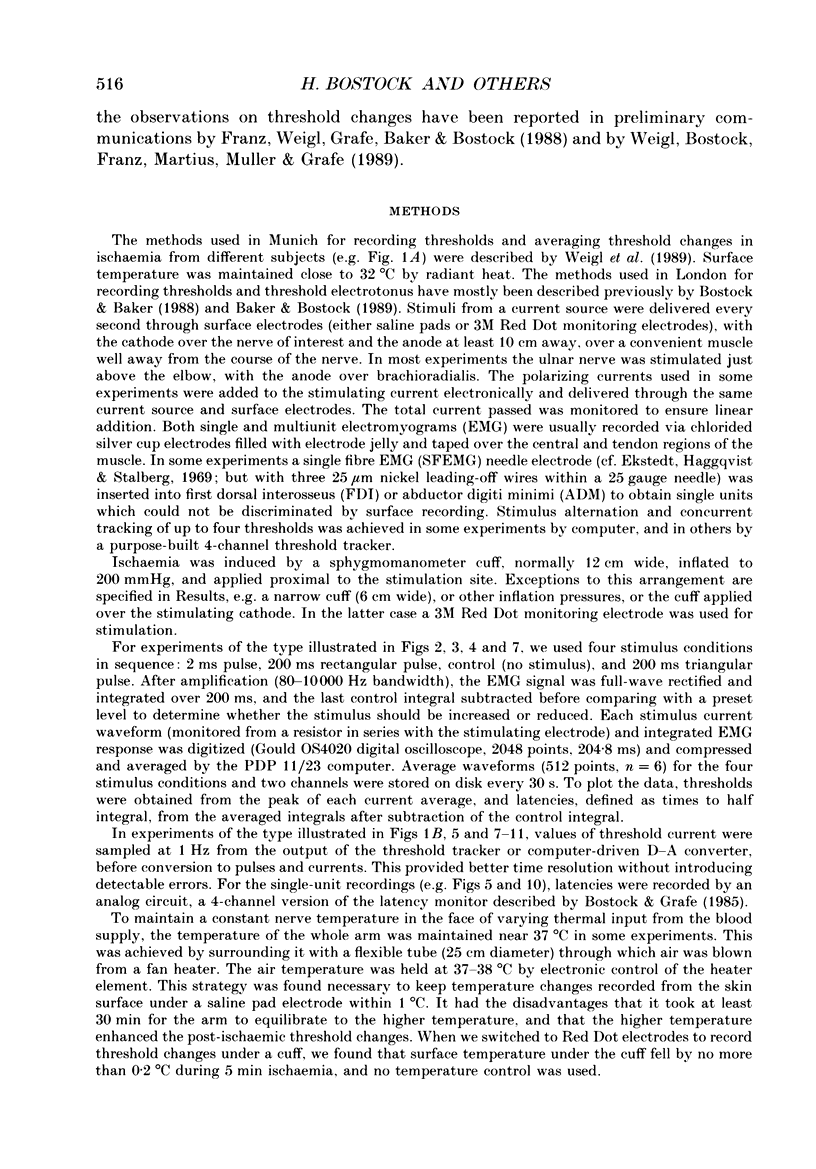
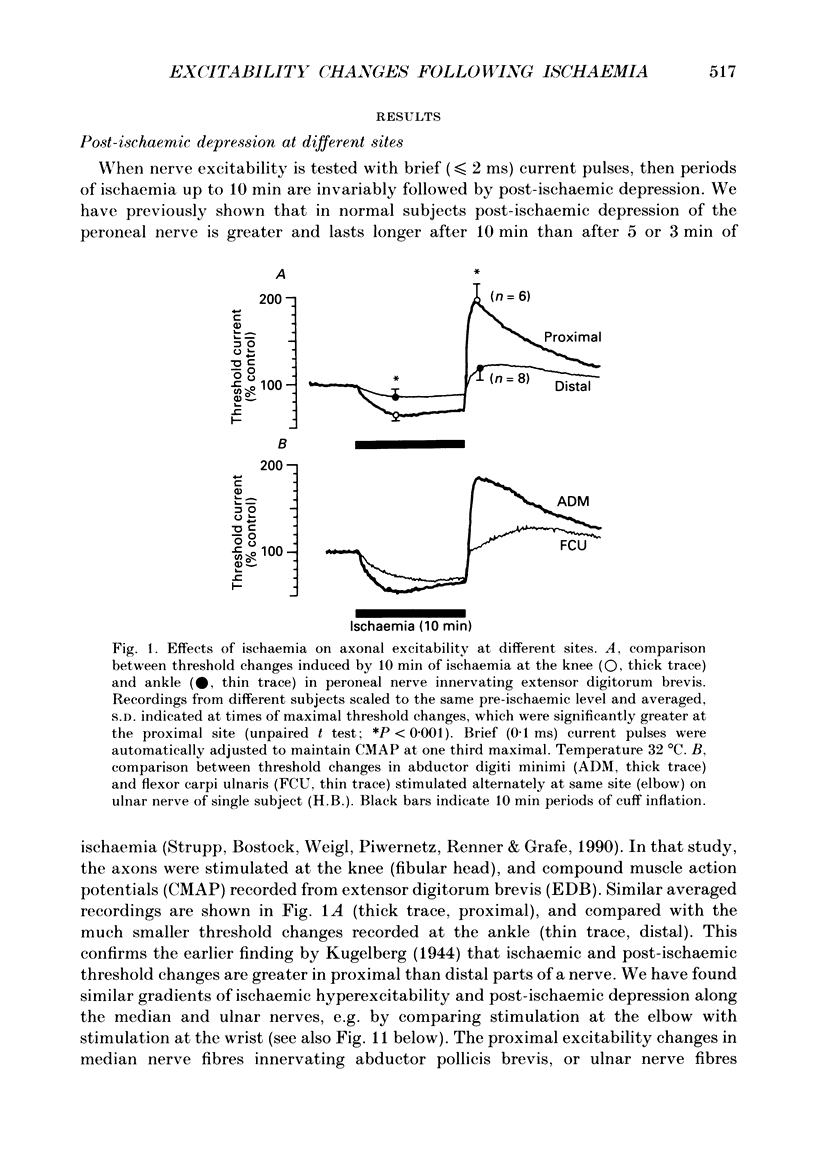
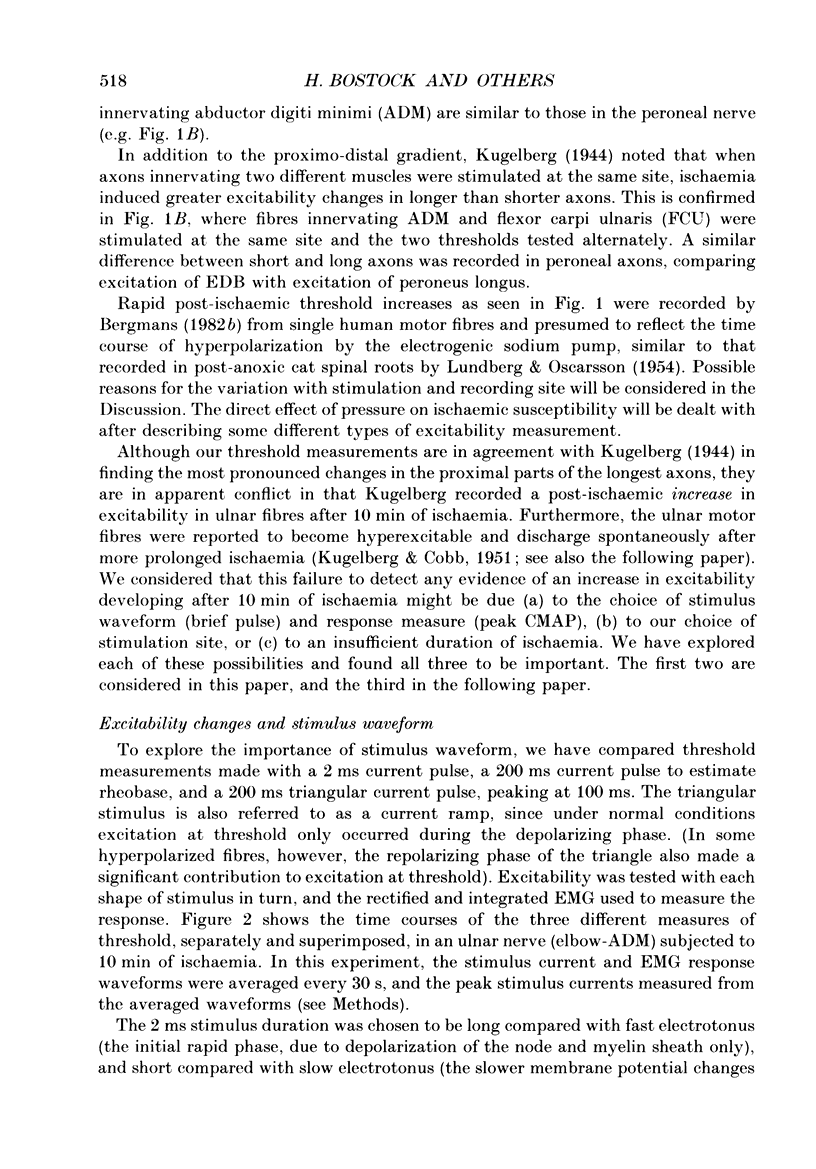
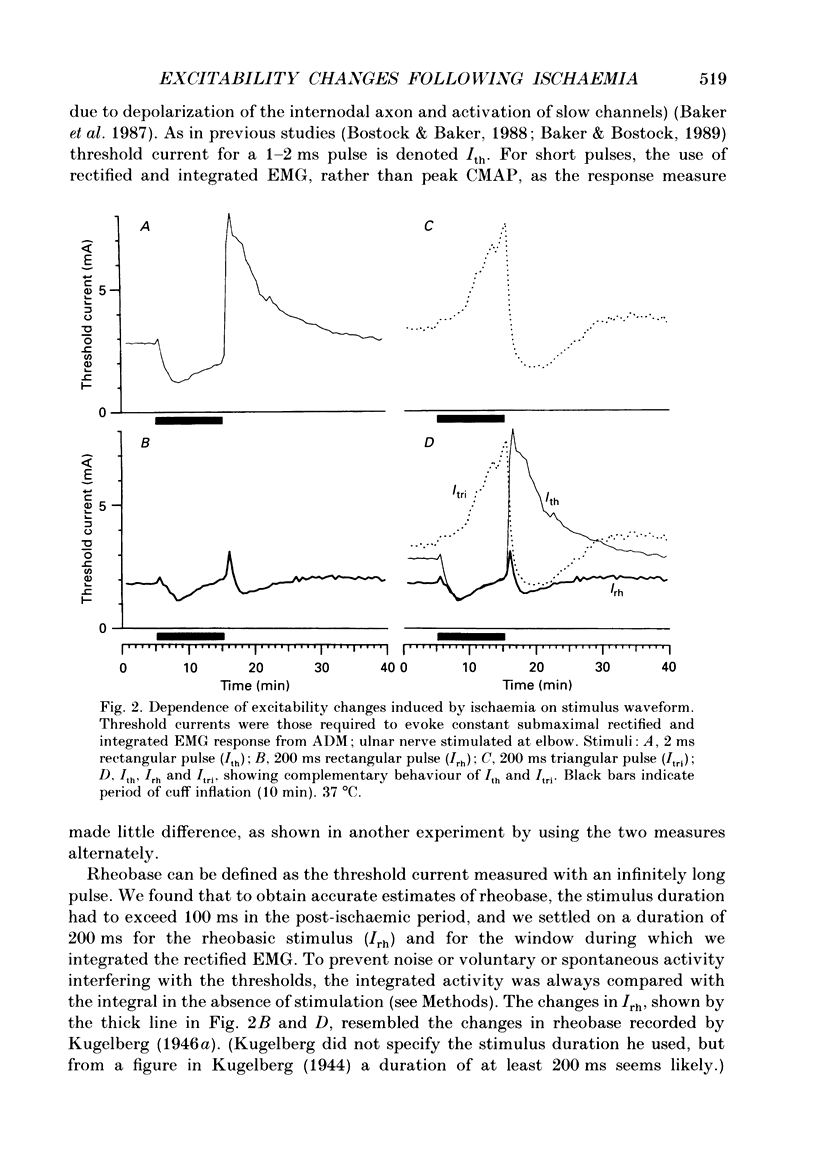
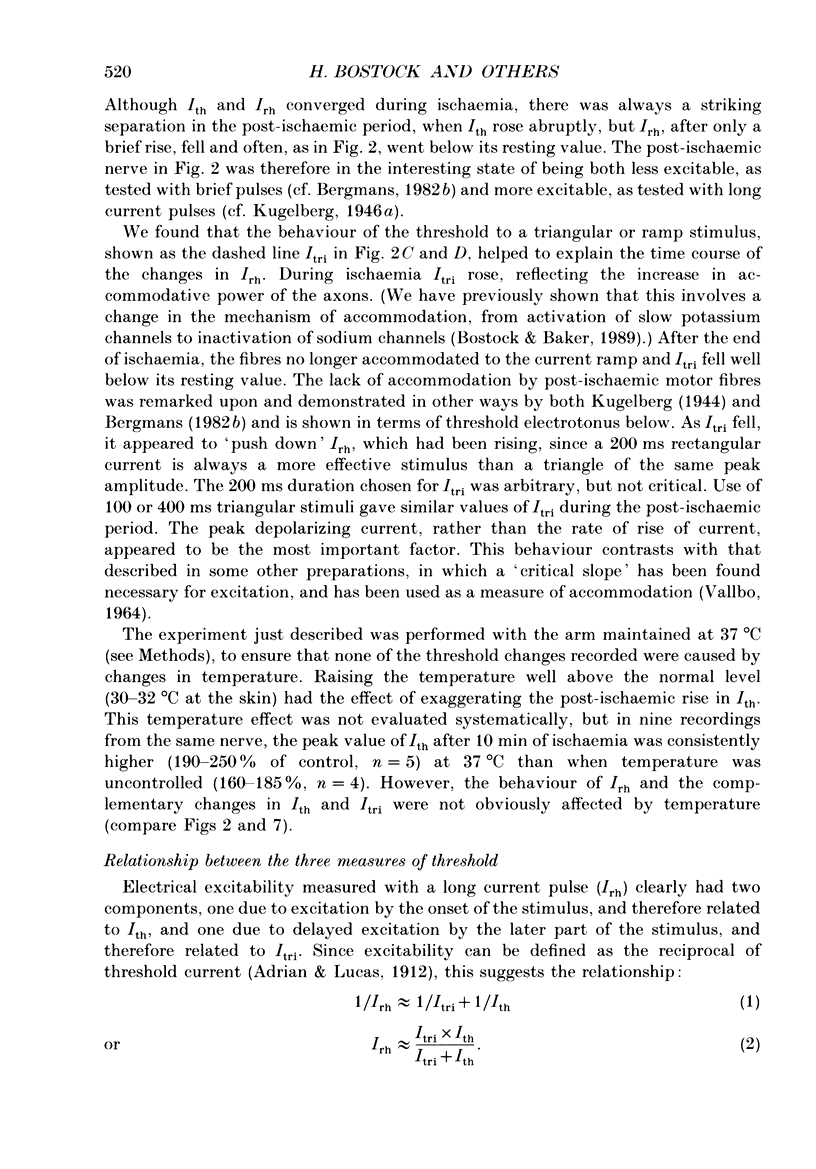
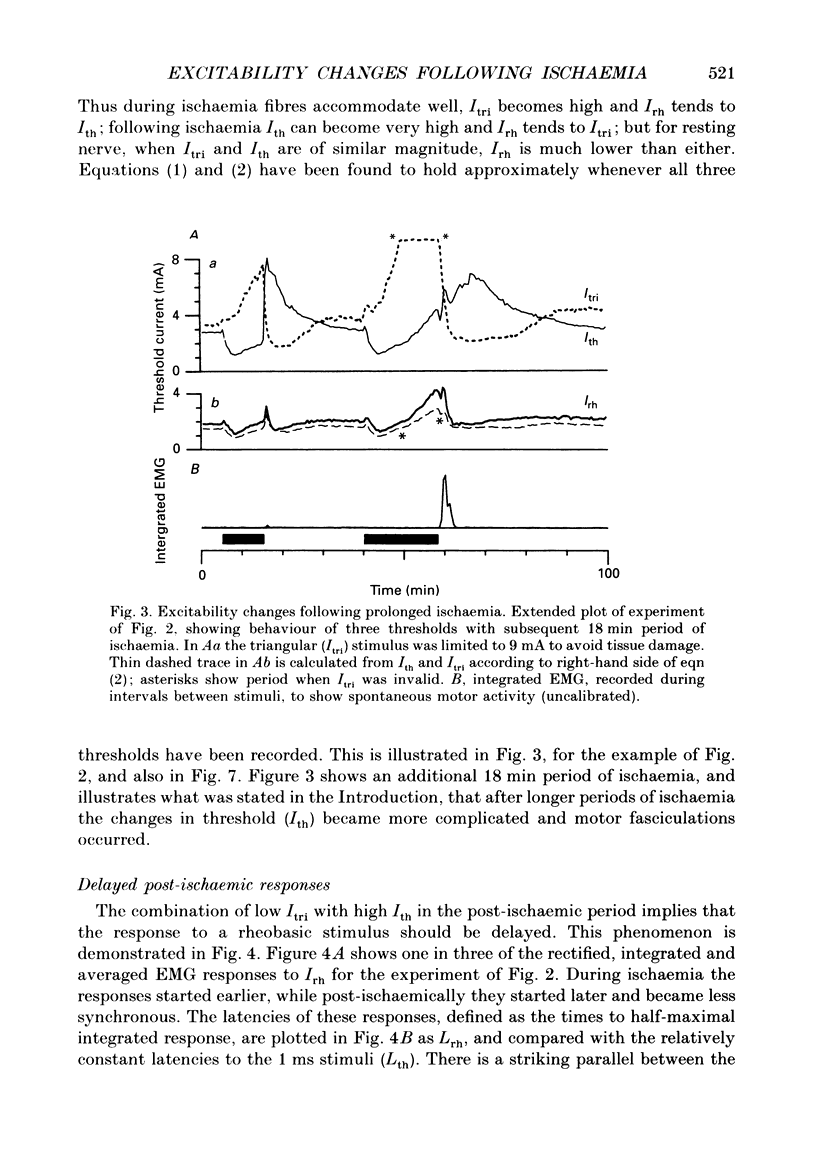
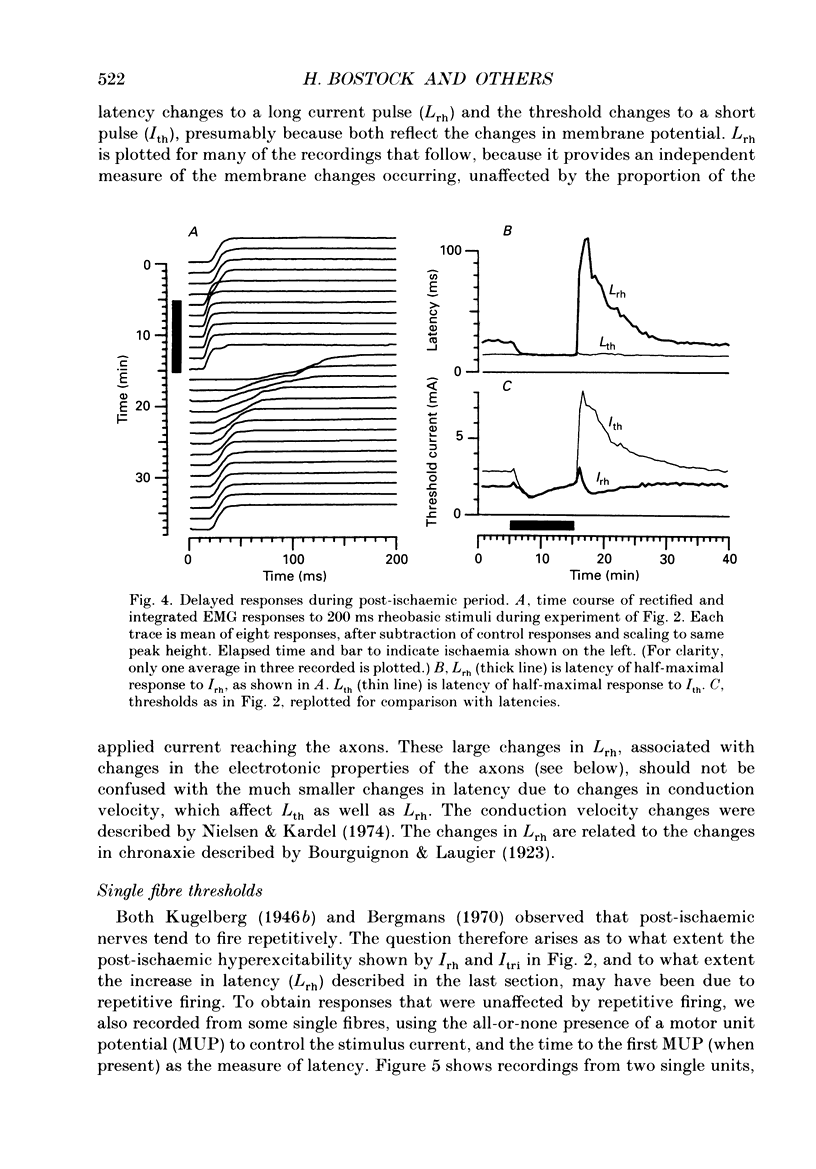
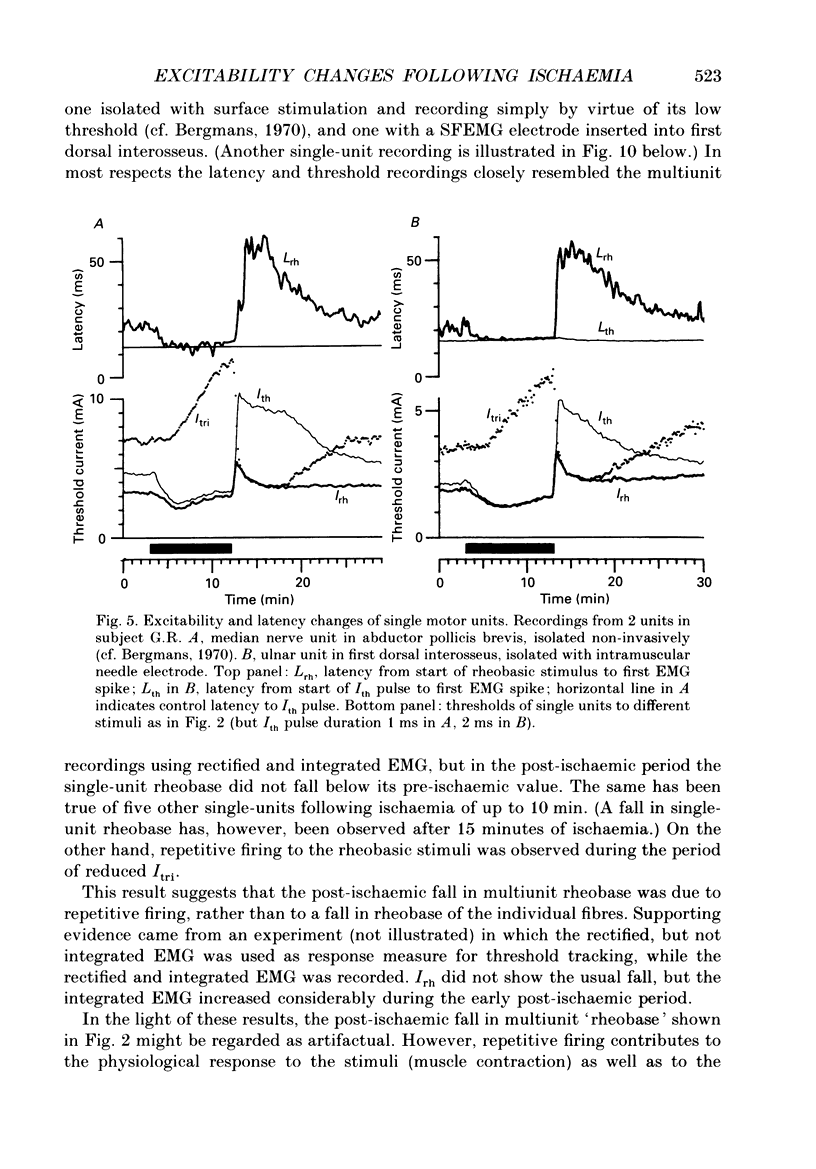
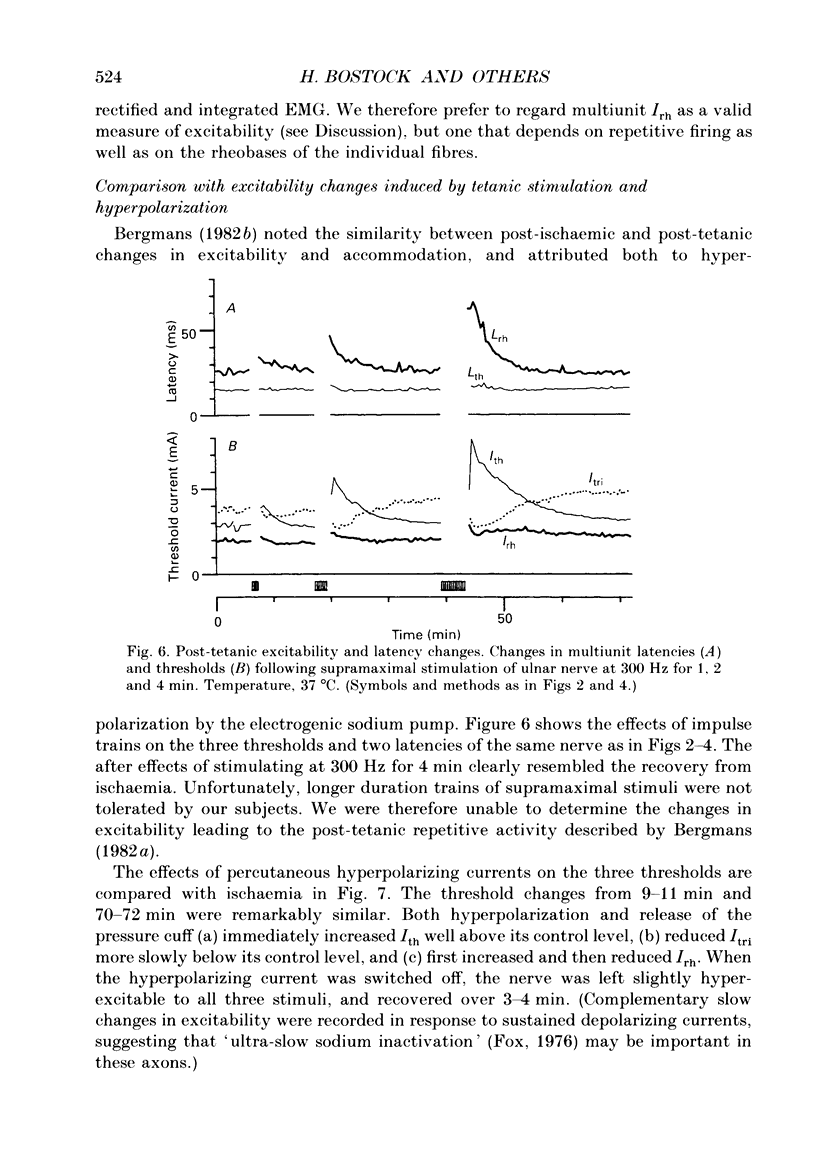
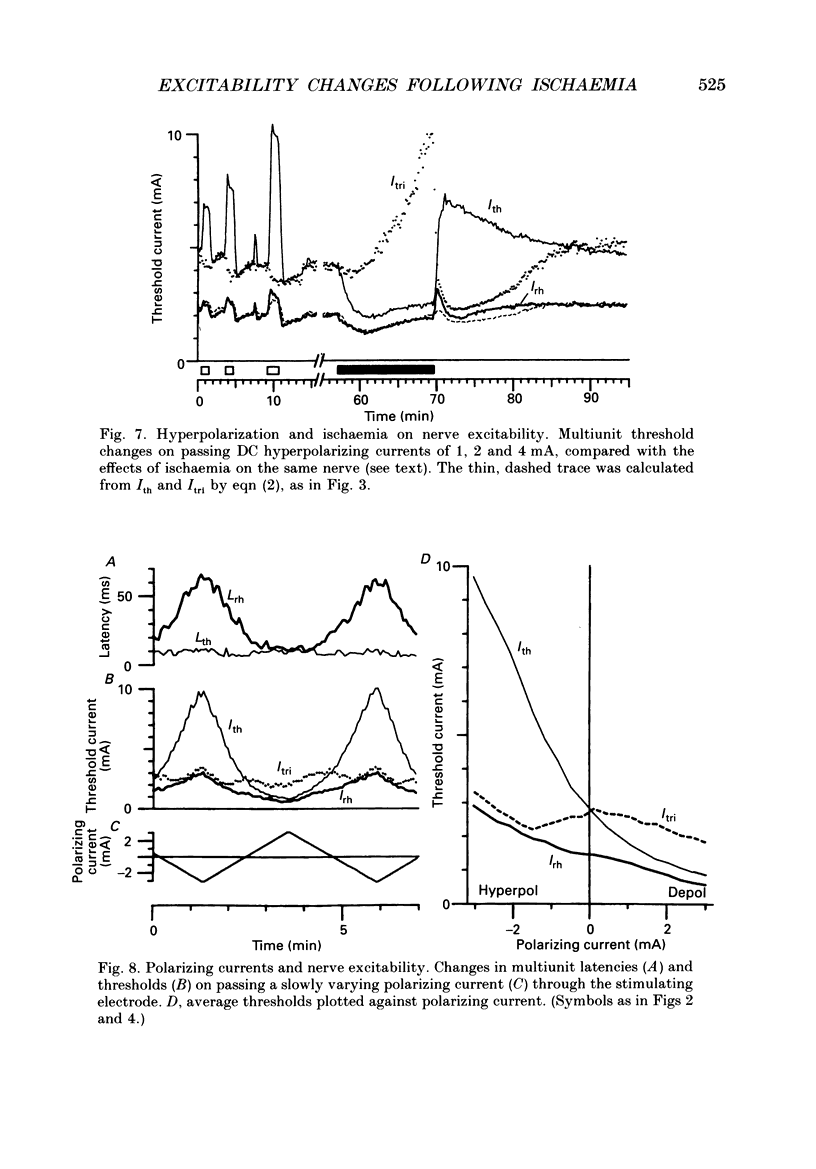
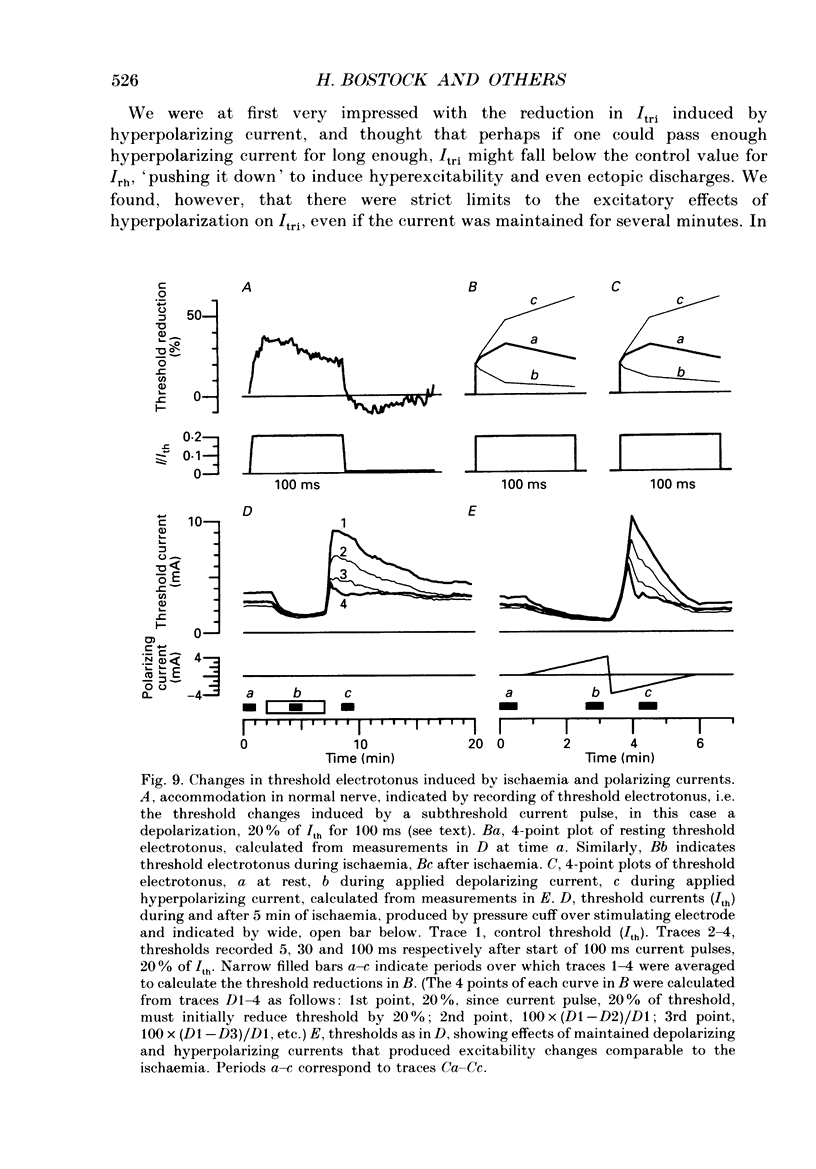
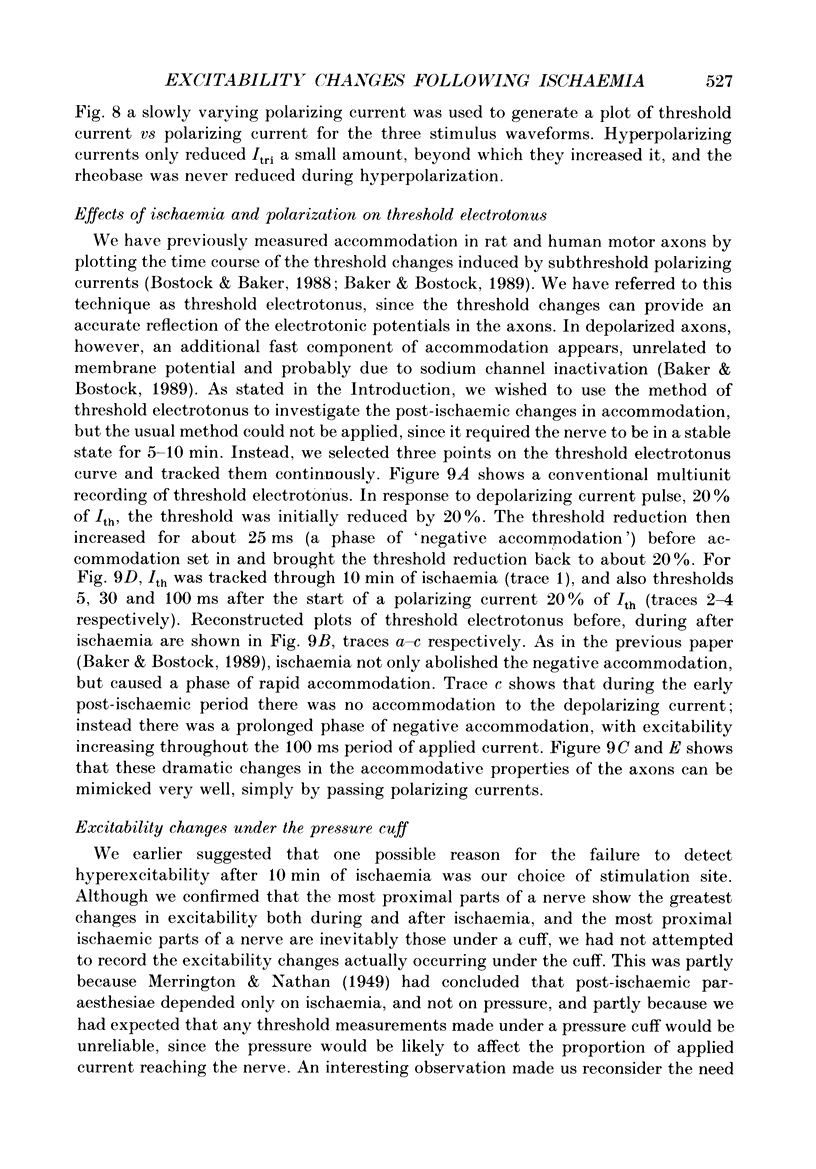
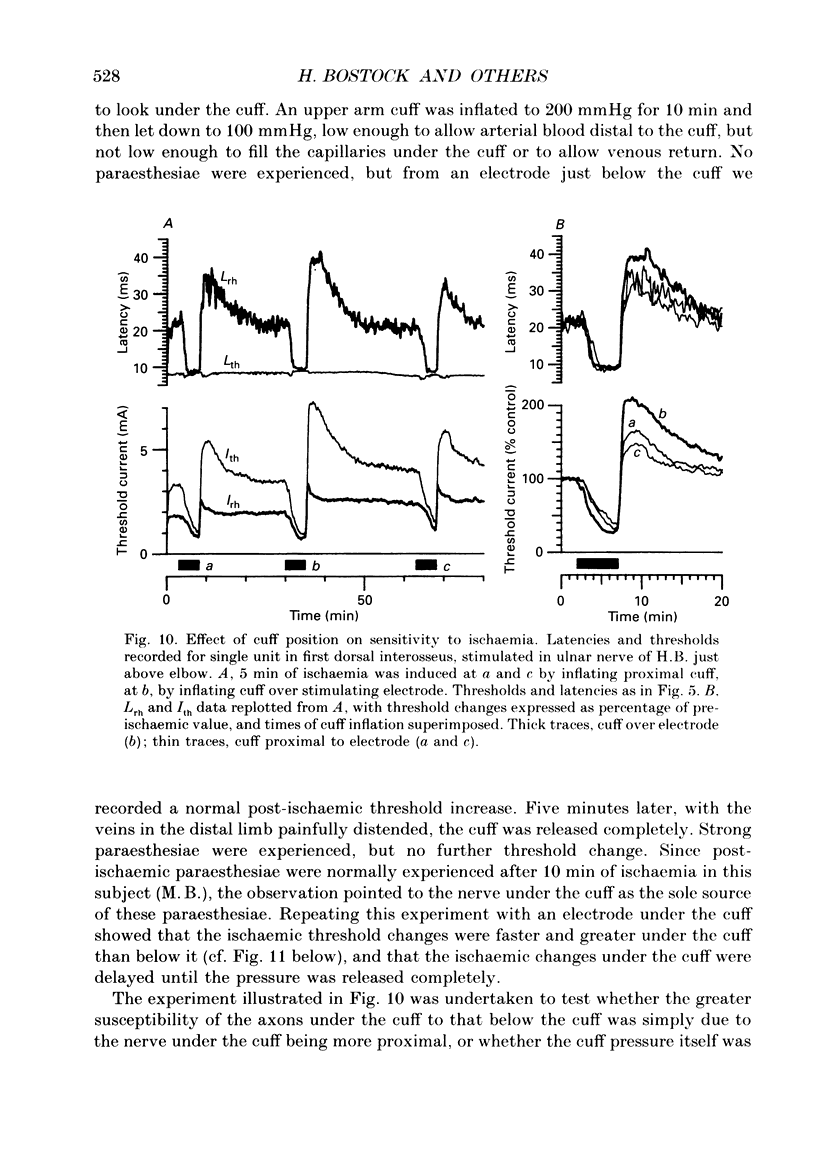
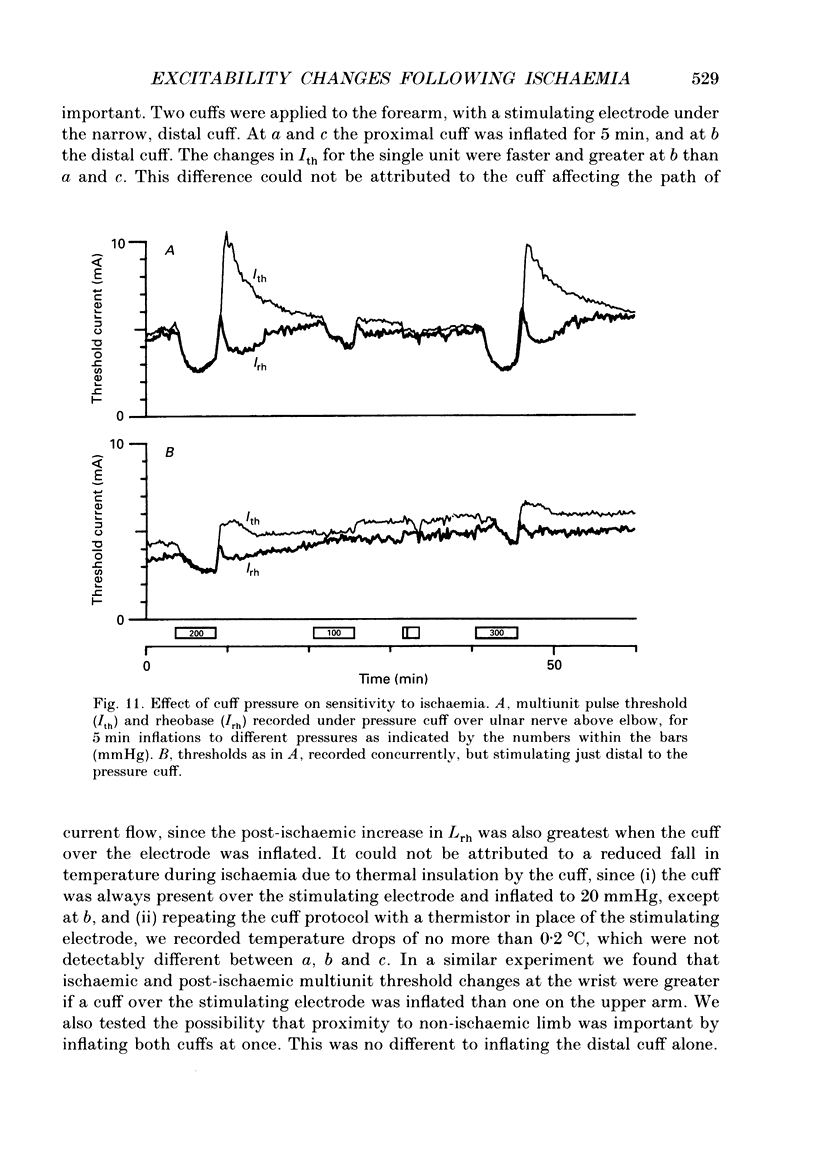
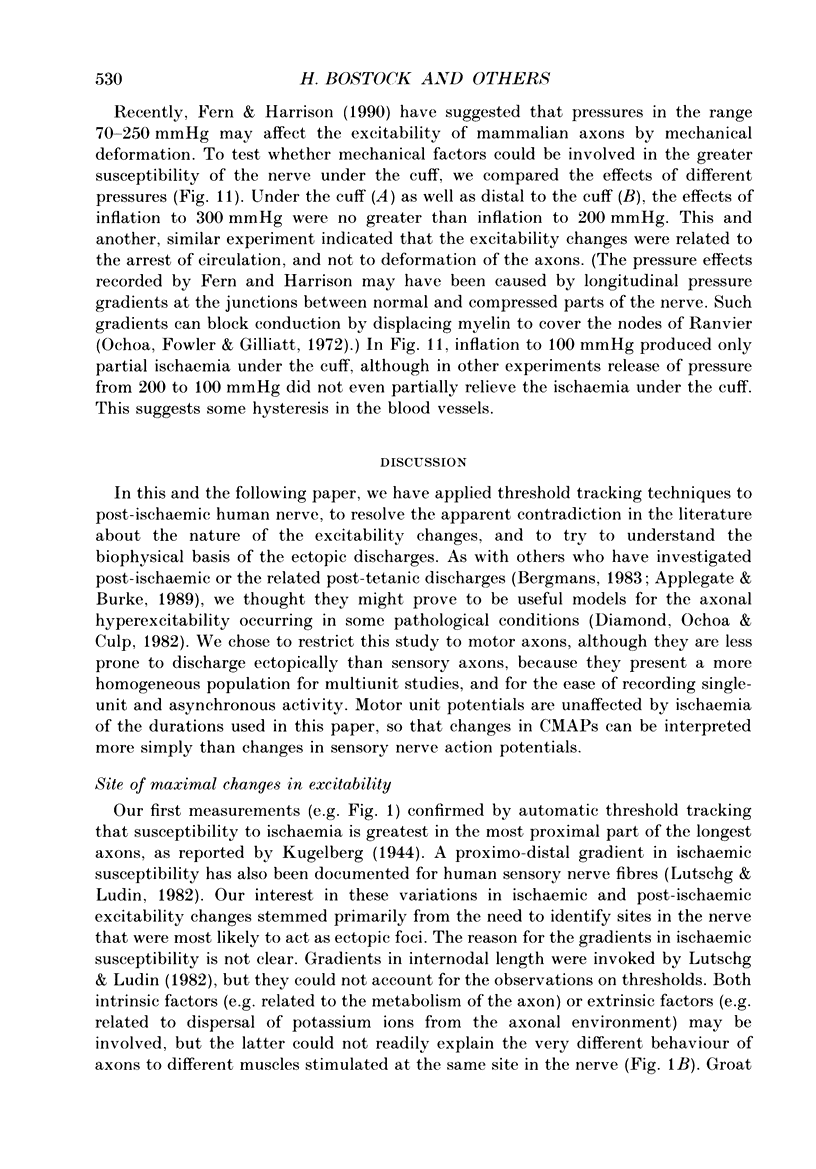
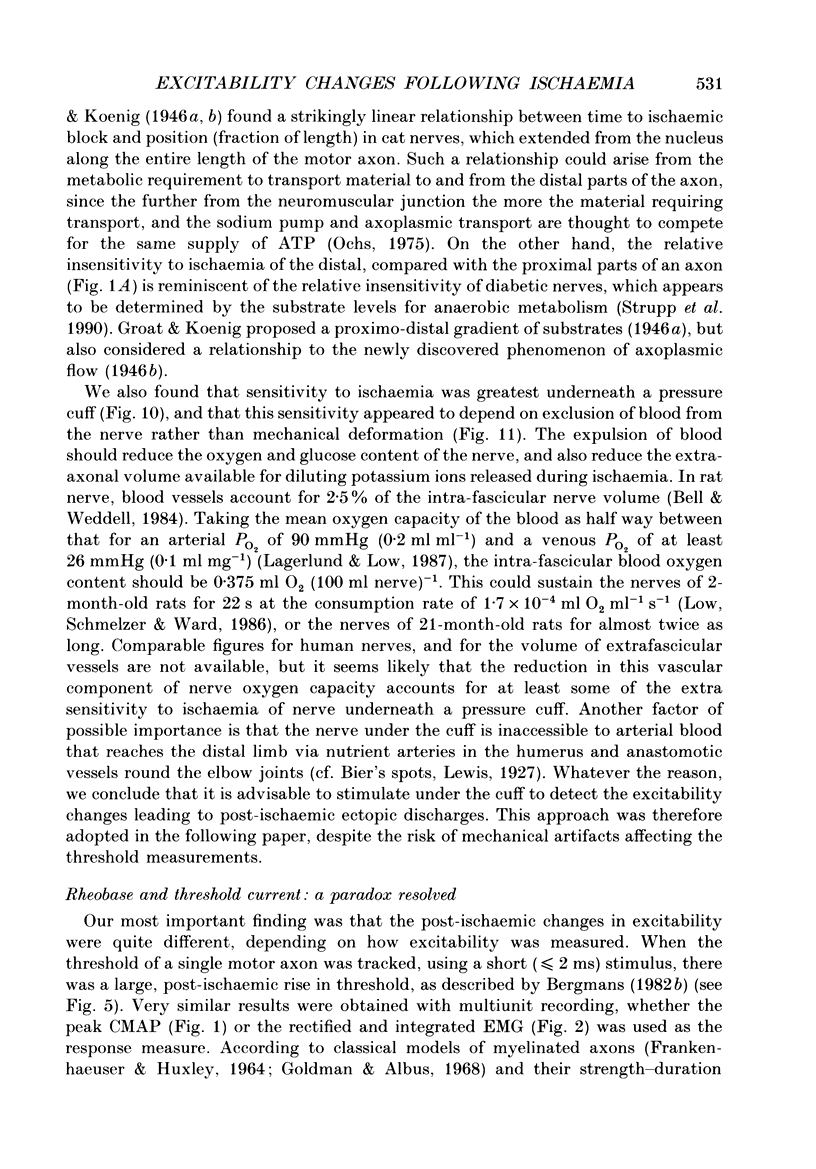
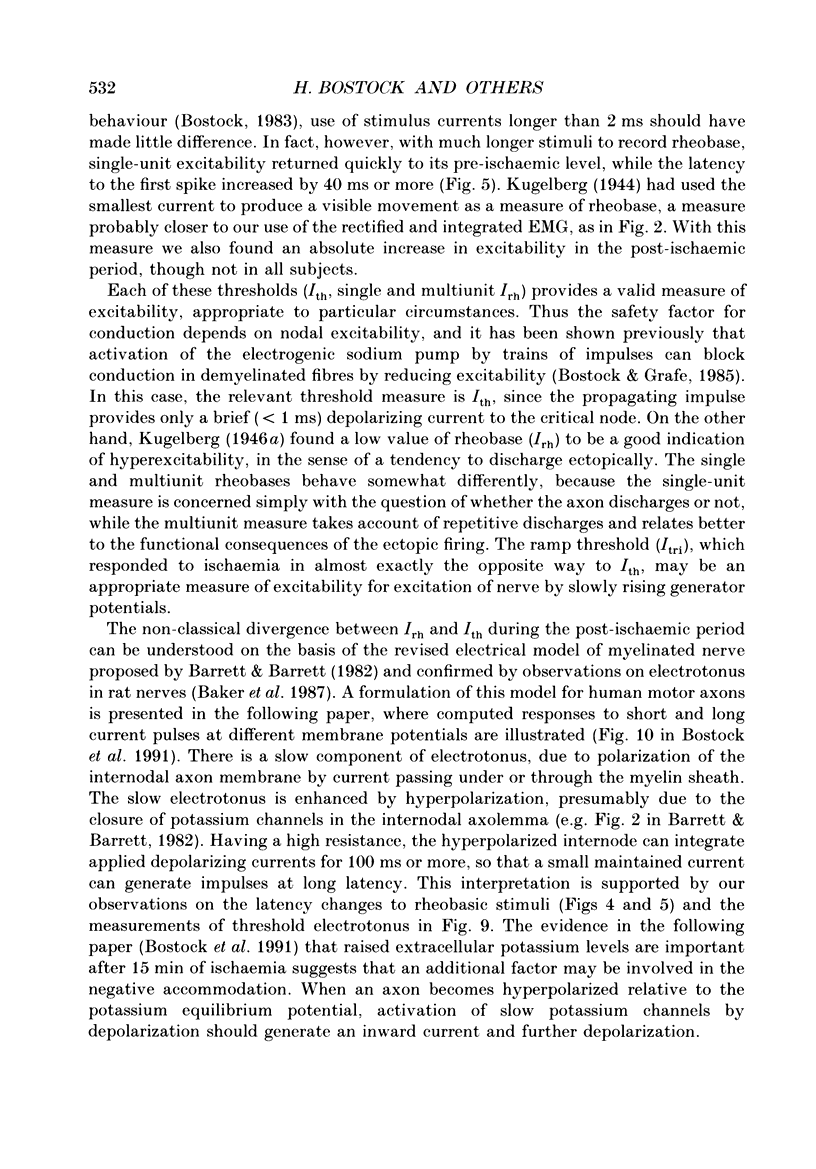
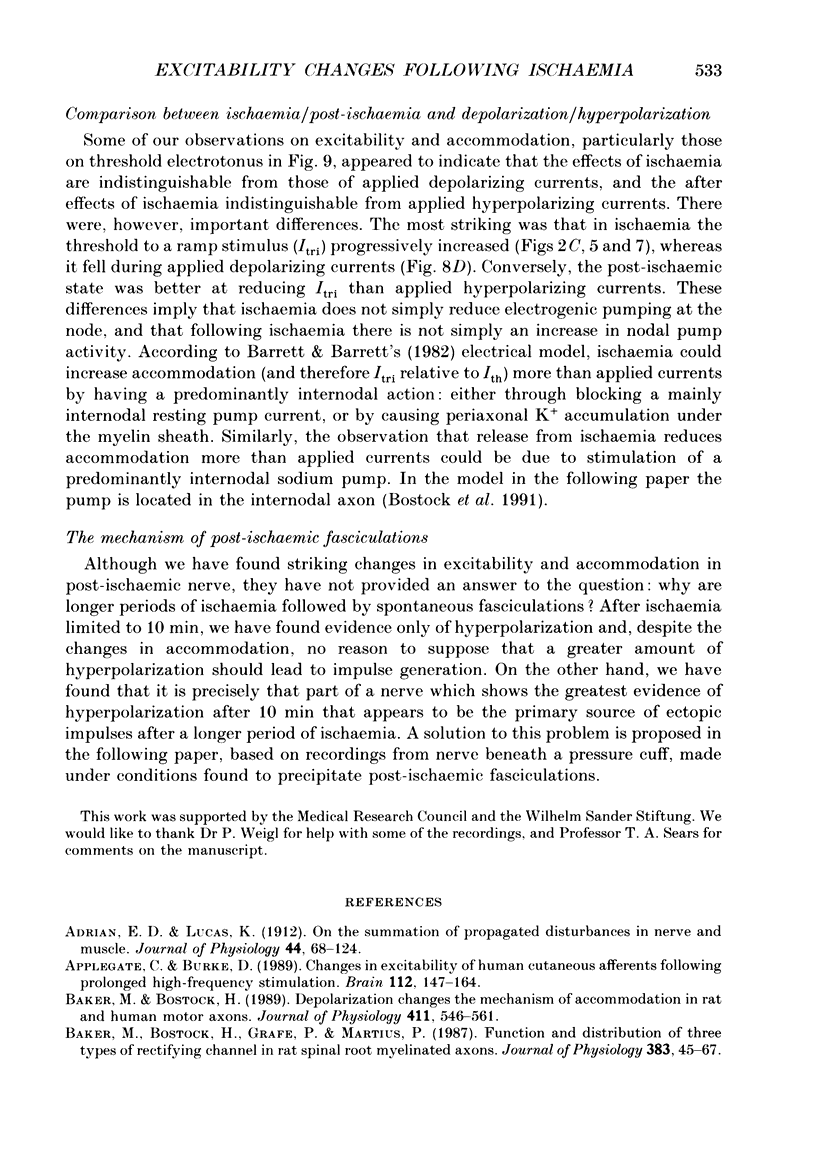
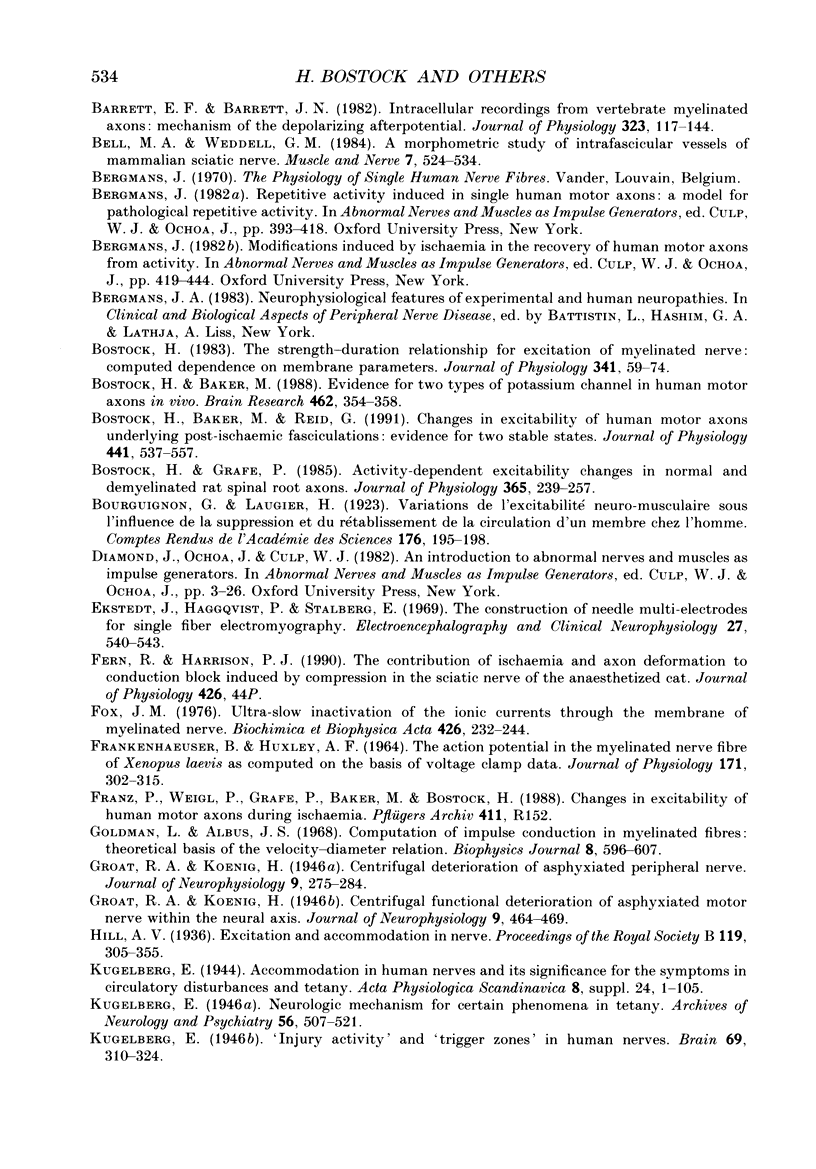
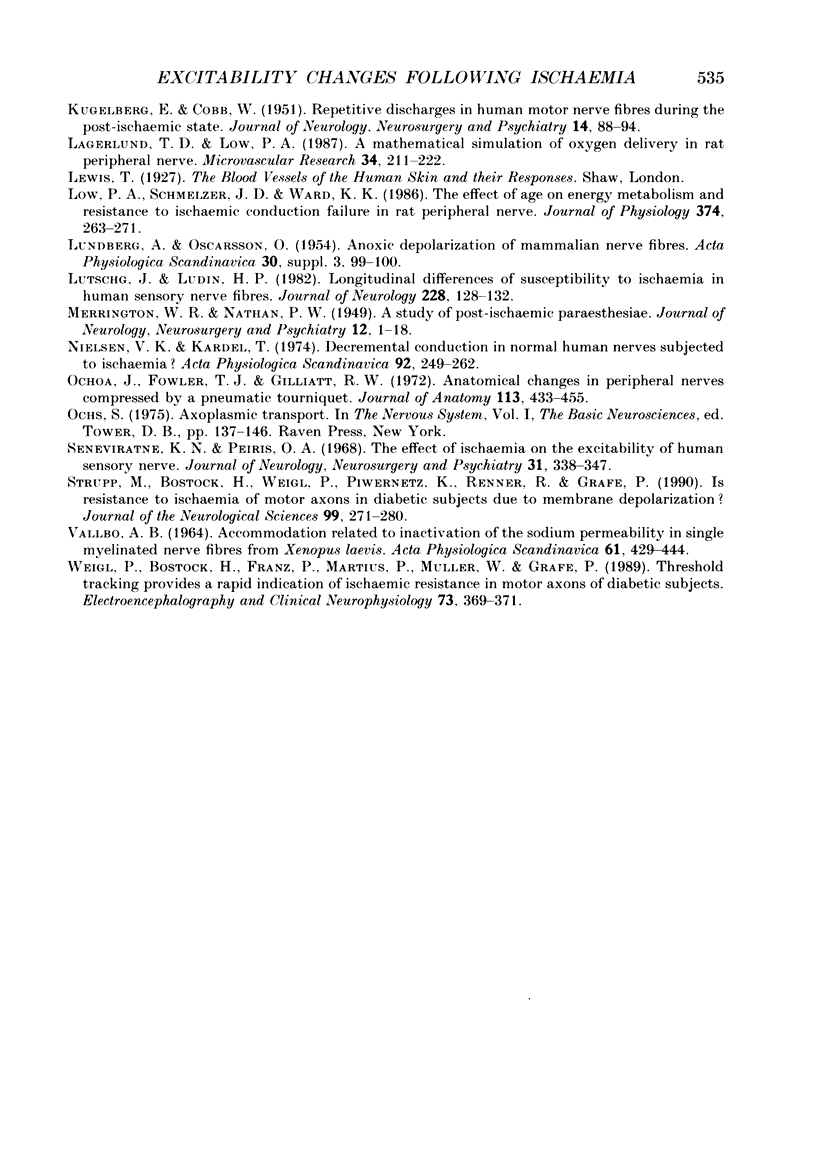
Selected References
These references are in PubMed. This may not be the complete list of references from this article.
- Adrian E. D. On the summation of propagated disturbances in nerve and muscle. J Physiol. 1912 Mar 29;44(1-2):68–124. doi: 10.1113/jphysiol.1912.sp001503. [DOI] [PMC free article] [PubMed] [Google Scholar]
- Applegate C., Burke D. Changes in excitability of human cutaneous afferents following prolonged high-frequency stimulation. Brain. 1989 Feb;112(Pt 1):147–164. doi: 10.1093/brain/112.1.147. [DOI] [PubMed] [Google Scholar]
- Baker M., Bostock H. Depolarization changes the mechanism of accommodation in rat and human motor axons. J Physiol. 1989 Apr;411:545–561. doi: 10.1113/jphysiol.1989.sp017589. [DOI] [PMC free article] [PubMed] [Google Scholar]
- Baker M., Bostock H., Grafe P., Martius P. Function and distribution of three types of rectifying channel in rat spinal root myelinated axons. J Physiol. 1987 Feb;383:45–67. doi: 10.1113/jphysiol.1987.sp016395. [DOI] [PMC free article] [PubMed] [Google Scholar]
- Barrett E. F., Barrett J. N. Intracellular recording from vertebrate myelinated axons: mechanism of the depolarizing afterpotential. J Physiol. 1982 Feb;323:117–144. doi: 10.1113/jphysiol.1982.sp014064. [DOI] [PMC free article] [PubMed] [Google Scholar]
- Bell M. A., Weddell A. G. A morphometric study of intrafascicular vessels of mammalian sciatic nerve. Muscle Nerve. 1984 Sep;7(7):524–534. doi: 10.1002/mus.880070703. [DOI] [PubMed] [Google Scholar]
- Bostock H., Baker M. Evidence for two types of potassium channel in human motor axons in vivo. Brain Res. 1988 Oct 18;462(2):354–358. doi: 10.1016/0006-8993(88)90564-1. [DOI] [PubMed] [Google Scholar]
- Bostock H., Baker M., Reid G. Changes in excitability of human motor axons underlying post-ischaemic fasciculations: evidence for two stable states. J Physiol. 1991 Sep;441:537–557. doi: 10.1113/jphysiol.1991.sp018766. [DOI] [PMC free article] [PubMed] [Google Scholar]
- Bostock H., Grafe P. Activity-dependent excitability changes in normal and demyelinated rat spinal root axons. J Physiol. 1985 Aug;365:239–257. doi: 10.1113/jphysiol.1985.sp015769. [DOI] [PMC free article] [PubMed] [Google Scholar]
- Bostock H. The strength-duration relationship for excitation of myelinated nerve: computed dependence on membrane parameters. J Physiol. 1983 Aug;341:59–74. doi: 10.1113/jphysiol.1983.sp014792. [DOI] [PMC free article] [PubMed] [Google Scholar]
- Ekstedt J., Häggqvist P., Stålberg E. The construction of needle multi-electrodes for single fiber electromyography. Electroencephalogr Clin Neurophysiol. 1969 Nov;27(5):540–543. doi: 10.1016/0013-4694(69)90196-5. [DOI] [PubMed] [Google Scholar]
- FRANKENHAEUSER B., HUXLEY A. F. THE ACTION POTENTIAL IN THE MYELINATED NERVE FIBER OF XENOPUS LAEVIS AS COMPUTED ON THE BASIS OF VOLTAGE CLAMP DATA. J Physiol. 1964 Jun;171:302–315. doi: 10.1113/jphysiol.1964.sp007378. [DOI] [PMC free article] [PubMed] [Google Scholar]
- Fox J. M. Ultra-slow inactivation of the ionic currents through the membrane of myelinated nerve. Biochim Biophys Acta. 1976 Mar 5;426(2):232–244. doi: 10.1016/0005-2736(76)90334-5. [DOI] [PubMed] [Google Scholar]
- Goldman L., Albus J. S. Computation of impulse conduction in myelinated fibers; theoretical basis of the velocity-diameter relation. Biophys J. 1968 May;8(5):596–607. doi: 10.1016/S0006-3495(68)86510-5. [DOI] [PMC free article] [PubMed] [Google Scholar]
- KUGELBERG E., COBB W. Repetitive discharges in human motor nerve fibers during the post-ischaemic state. J Neurol Neurosurg Psychiatry. 1951 May;14(2):88–94. doi: 10.1136/jnnp.14.2.88. [DOI] [PMC free article] [PubMed] [Google Scholar]
- Lagerlund T. D., Low P. A. A mathematical simulation of oxygen delivery in rat peripheral nerve. Microvasc Res. 1987 Sep;34(2):211–222. doi: 10.1016/0026-2862(87)90054-9. [DOI] [PubMed] [Google Scholar]
- Low P. A., Schmelzer J. D., Ward K. K. The effect of age on energy metabolism and resistance to ischaemic conduction failure in rat peripheral nerve. J Physiol. 1986 May;374:263–271. doi: 10.1113/jphysiol.1986.sp016078. [DOI] [PMC free article] [PubMed] [Google Scholar]
- Lütschg J., Ludin H. P. Longitudinal differences of susceptibility to ischaemia in human sensory nerve fibres. J Neurol. 1982;228(2):123–132. doi: 10.1007/BF00313757. [DOI] [PubMed] [Google Scholar]
- Nielsen V. K., Kardel T. Decremental conduction in normal human nerves subjected to ischemia? Acta Physiol Scand. 1974 Oct;92(2):249–262. doi: 10.1111/j.1748-1716.1974.tb05742.x. [DOI] [PubMed] [Google Scholar]
- Ochoa J., Fowler T. J., Gilliatt R. W. Anatomical changes in peripheral nerves compressed by a pneumatic tourniquet. J Anat. 1972 Dec;113(Pt 3):433–455. [PMC free article] [PubMed] [Google Scholar]
- Seneviratne K. N., Peiris O. A. The effect of ischaemia on the excitability of human sensory nerve. J Neurol Neurosurg Psychiatry. 1968 Aug;31(4):338–347. doi: 10.1136/jnnp.31.4.338. [DOI] [PMC free article] [PubMed] [Google Scholar]
- Strupp M., Bostock H., Weigl P., Piwernetz K., Renner R., Grafe P. Is resistance to ischaemia of motor axons in diabetic subjects due to membrane depolarization? J Neurol Sci. 1990 Nov;99(2-3):271–280. doi: 10.1016/0022-510x(90)90161-f. [DOI] [PubMed] [Google Scholar]
- VALLBO A. B. ACCOMMODATION RELATED TO INACTIVATION OF THE SODIUM PERMEABILITY IN SINGLE MYELINATED NERVE FIBRES FROM XENOPUS LAEVIS. Acta Physiol Scand. 1964 Aug;61:429–444. [PubMed] [Google Scholar]
- Weigl P., Bostock H., Franz P., Martius P., Müller W., Grafe P. Threshold tracking provides a rapid indication of ischaemic resistance in motor axons of diabetic subjects. Electroencephalogr Clin Neurophysiol. 1989 Oct;73(4):369–371. doi: 10.1016/0013-4694(89)90115-6. [DOI] [PubMed] [Google Scholar]