Abstract
Spectral evidence indicates that molar concentrations of K+ can induce aggregate formation in d(TGG)4. The 320-nm turbidity monitoring indicates that more than 1 M KCl is needed for the onset of aggregation to occur at 20 degrees C within the time span of 24 h. The kinetic profile is reminiscent of autocatalytic reactions that consist of a lag period followed by accelerative and levelling phases. Progressive shortening of lag periods and more rapid accelerative phases accompany further increases in [K+]. Interestingly, the presence of Mg2+ greatly facilitates the aggregate formation and results in the prominent appearance of an intense psi-type CD. For example, whereas 1 M K+ fails to induce aggregate formation of d(TGG)4 within 24 h, the addition of 1 mM Mg2+ to a 1 M K+ solution is sufficient to induce the onset of aggregation in approximately 12 h. Furthermore, adjustment of the buffer to 16 mM Mg2+/1 M KCl reduces the lag time to less than 10 min and aggregation is nearly complete in 2 h. The requirement of [K+] for aggregation is reduced to 2 mM in the presence of 16 mM Mg2+, a reduction of nearly three orders of magnitude when compared to solutions without Mg2+. The effects of K+ and Mg2+ ions are synergistic, because the presence of 16 mM Mg2+ alone does not induce aggregate formation in this oligomer. Thermal stabilities of the aggregates are strongly dependent on the concentrations of these two ions. Although aggregates formed in the presence of 2 M KCl alone melt around 55 degrees C, those formed with added 16 mM Mg2+ melt at approximately 90 degrees C, with some aggregates remaining unmelted even at 95 degrees C. The slow kinetics of aggregate formation led to the appearance of gross hystereses in the cooling profiles. The interplay of these two ions appears to be specific, because the replacement of K+ by Na+ or the replacement of Mg2+ by other divalent cations does not lead to the observed self-assembly phenomenon, although Sr2+ can substitute for K+. A possible mechanism for the formation of self-assembled structures is suggested.
Full text
PDF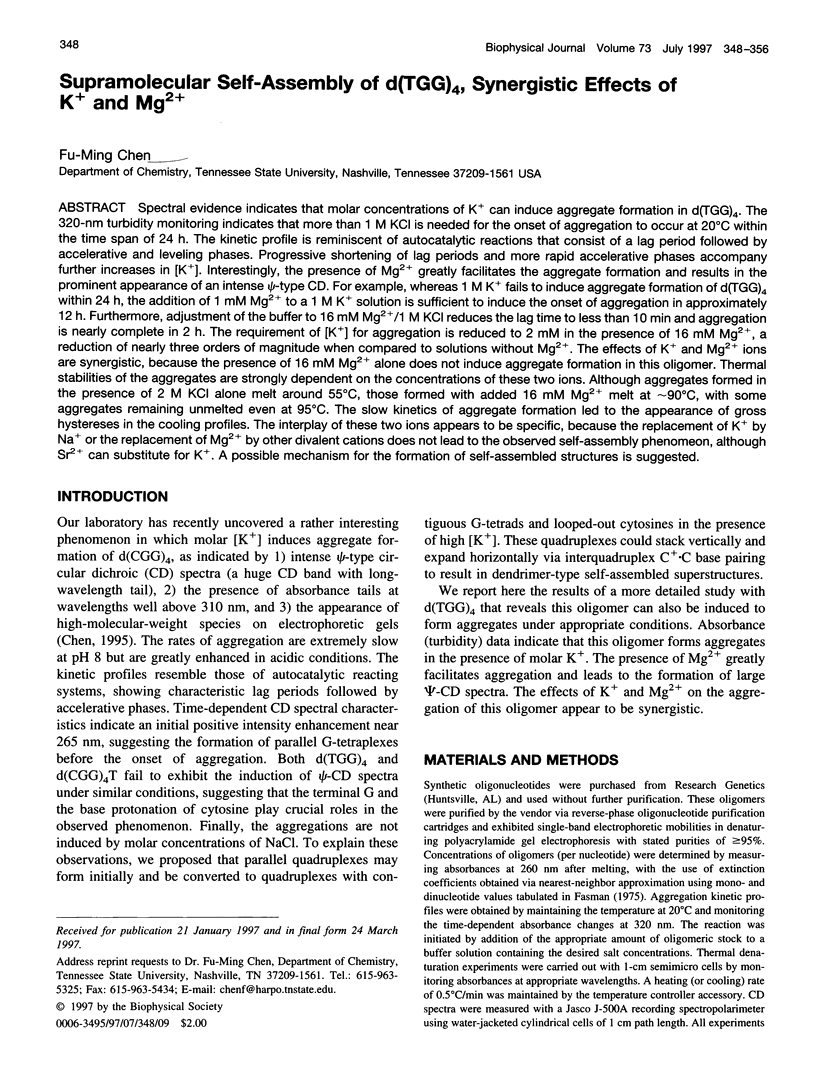
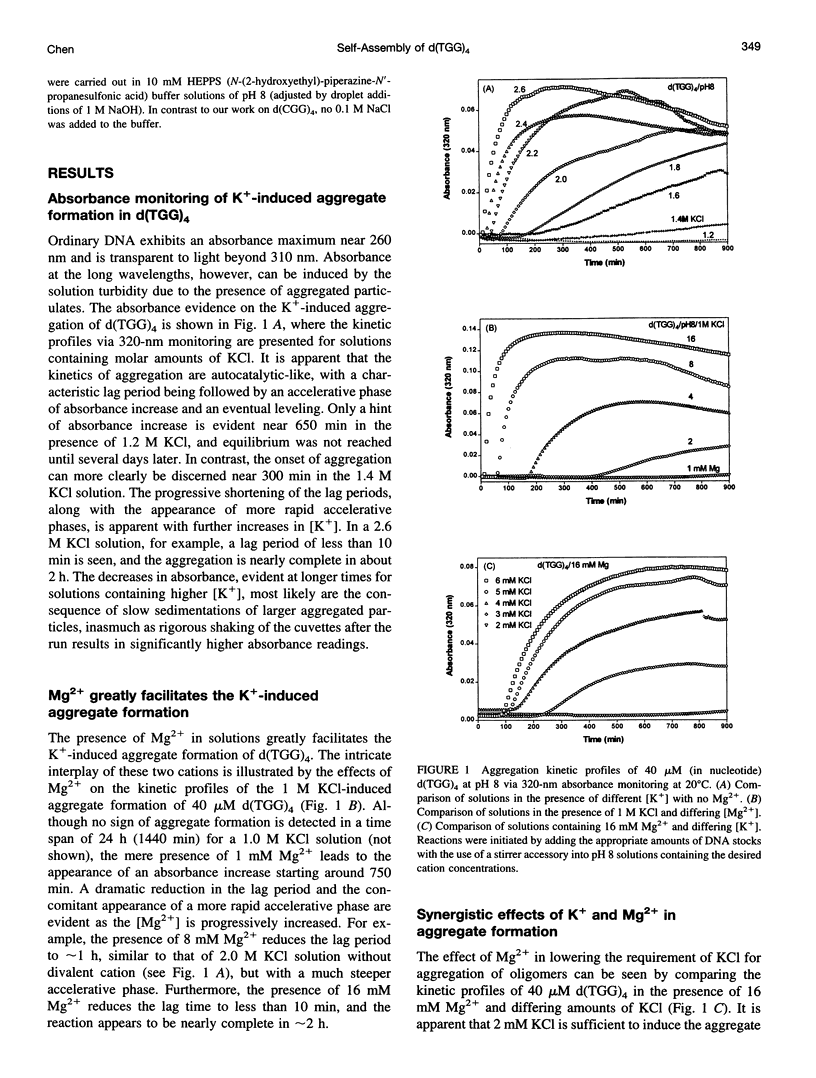
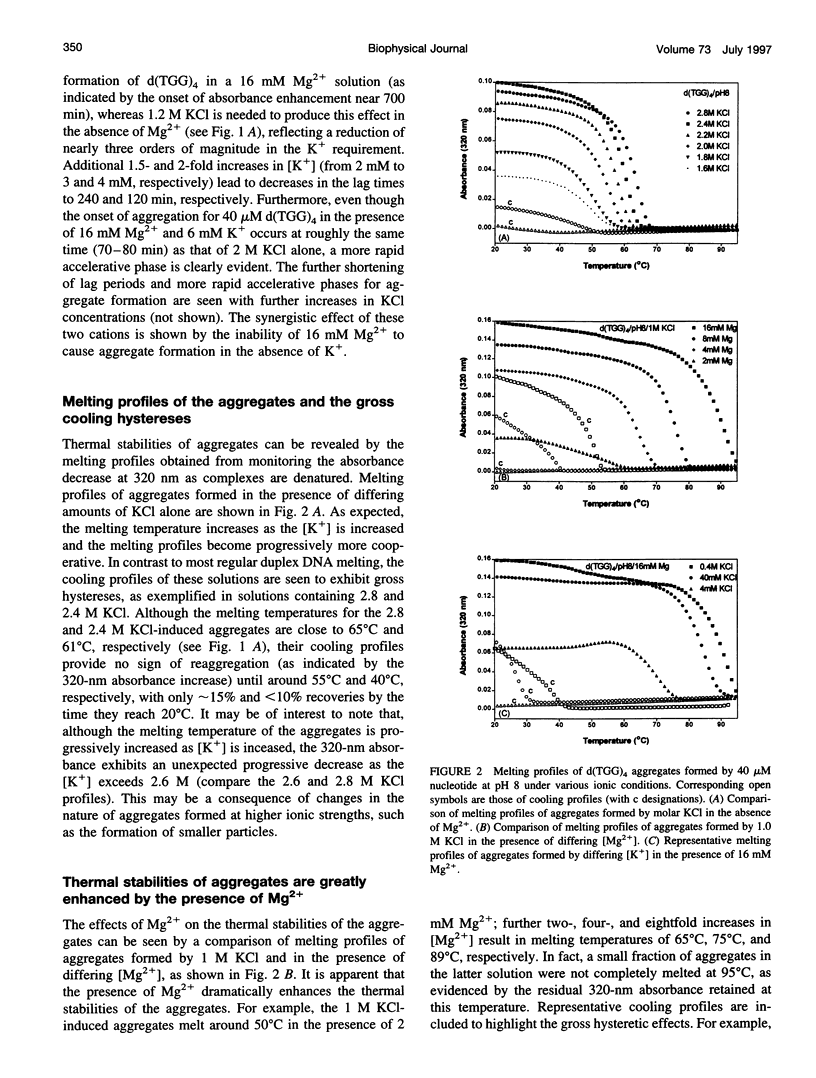
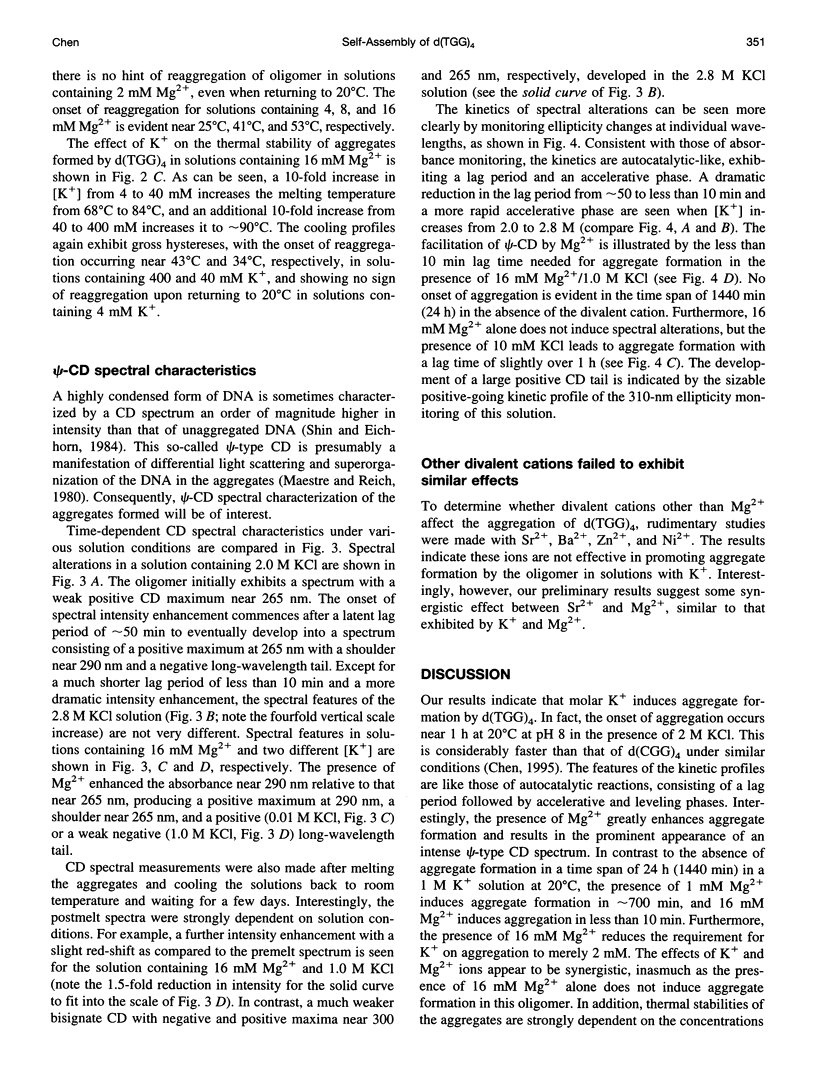
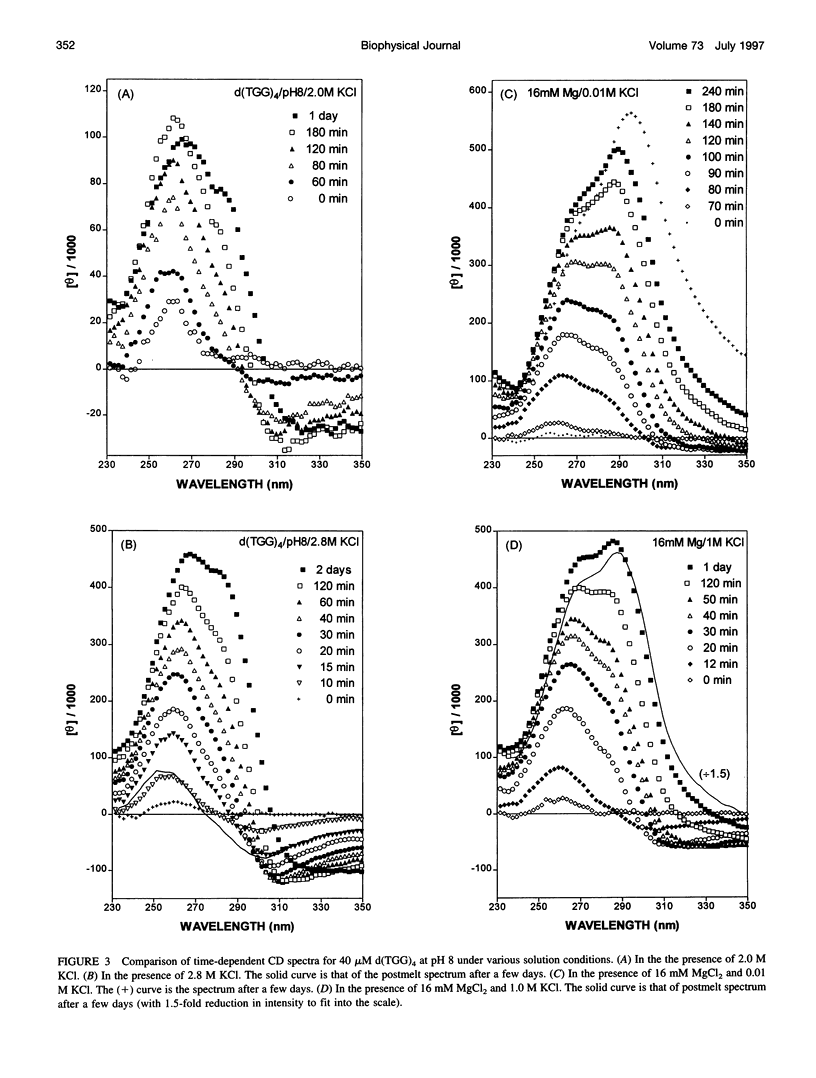
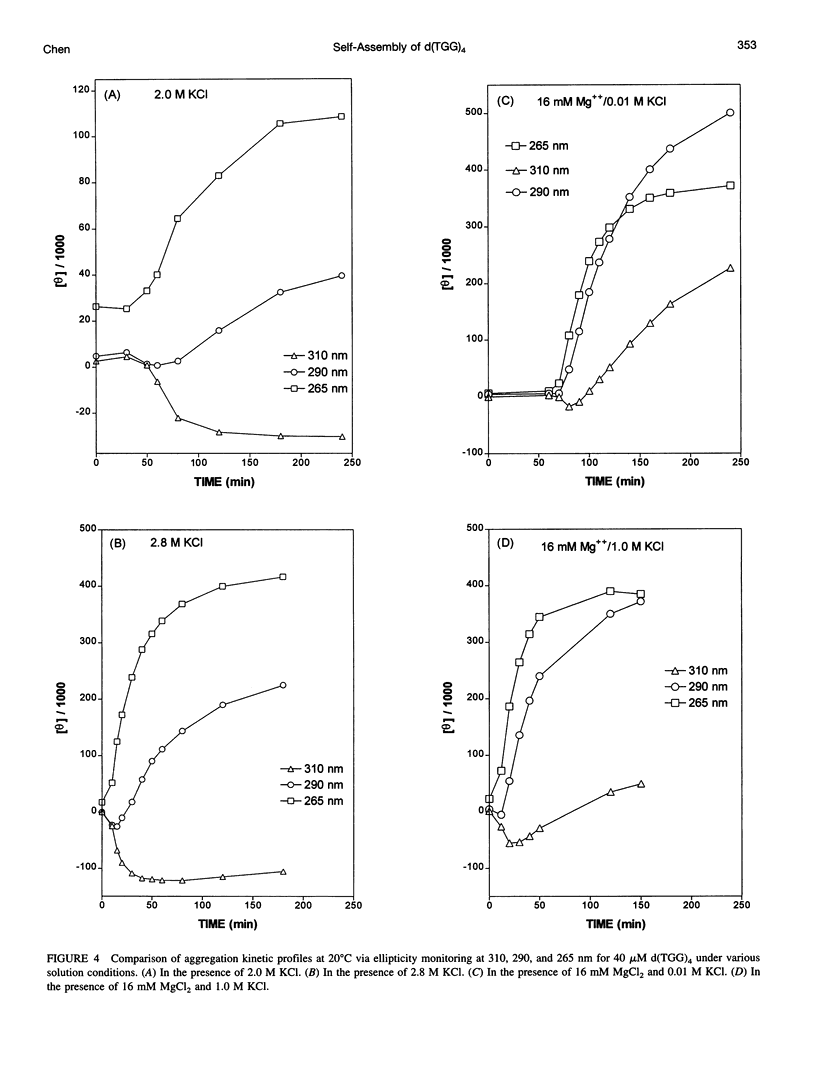
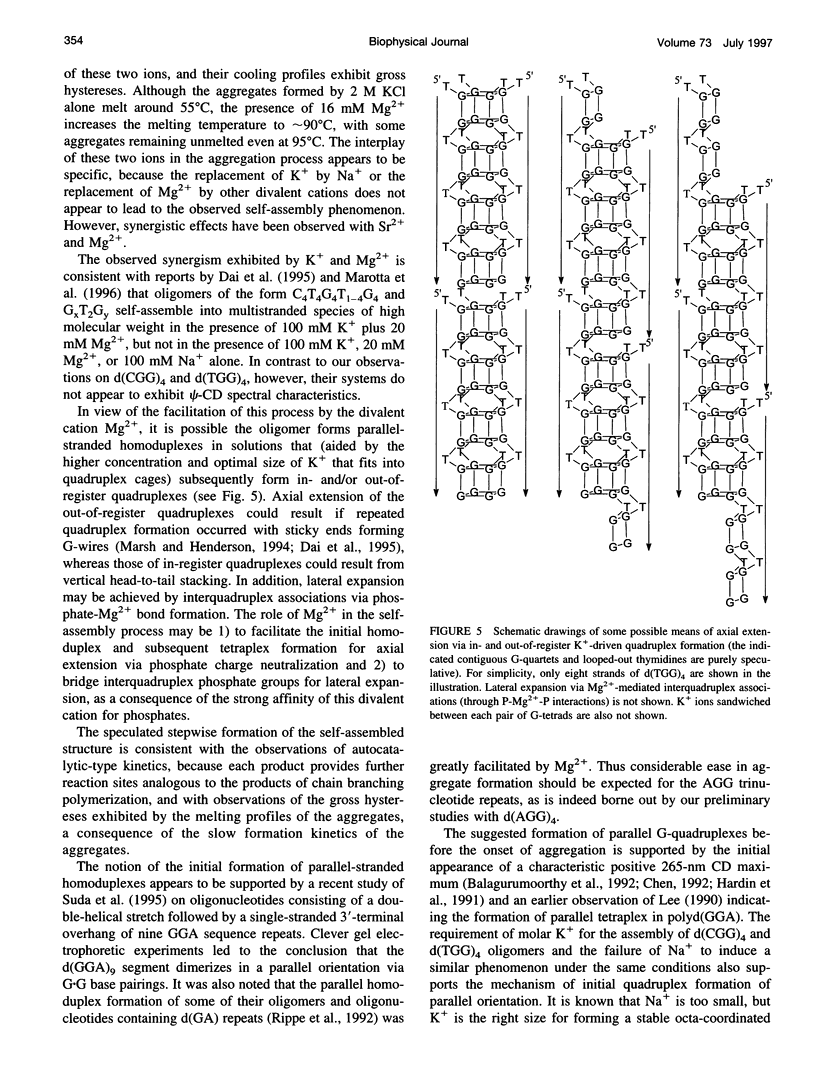
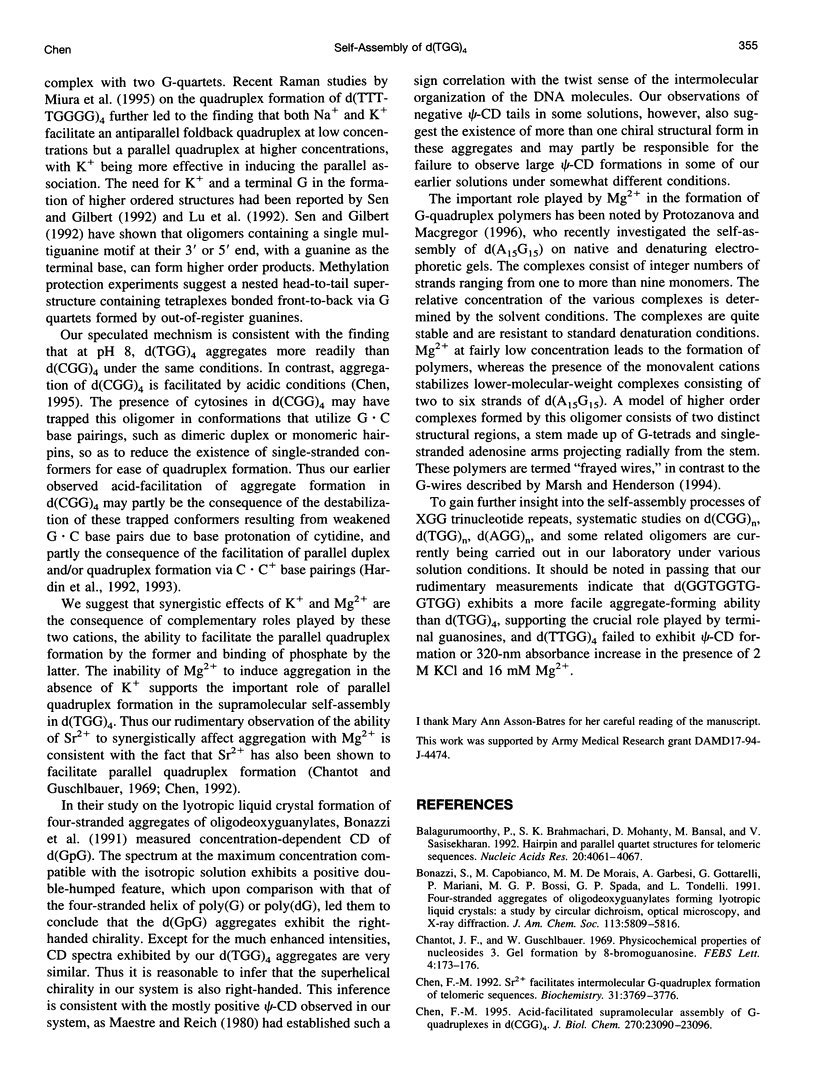
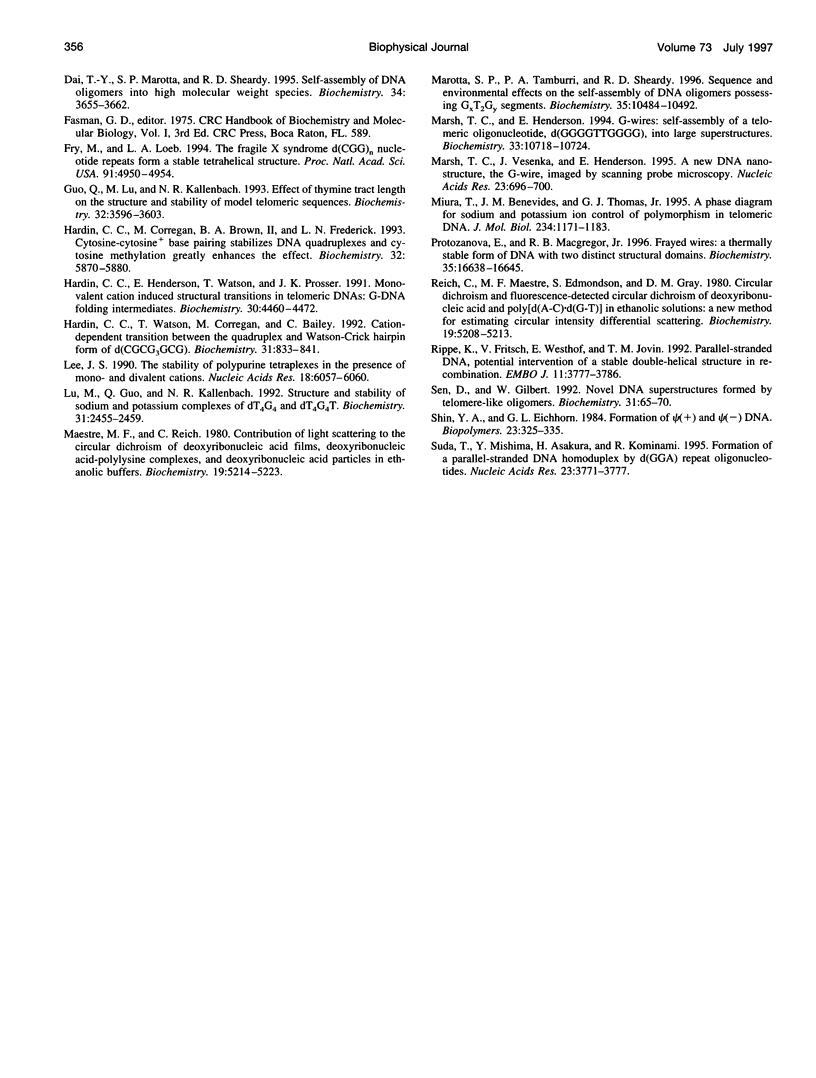
Selected References
These references are in PubMed. This may not be the complete list of references from this article.
- Balagurumoorthy P., Brahmachari S. K., Mohanty D., Bansal M., Sasisekharan V. Hairpin and parallel quartet structures for telomeric sequences. Nucleic Acids Res. 1992 Aug 11;20(15):4061–4067. doi: 10.1093/nar/20.15.4061. [DOI] [PMC free article] [PubMed] [Google Scholar]
- Chantot J. -F., Guschlbauer W. Physicochemical properties of nucleosides 3. Gel formation by 8-bromoguanosine. FEBS Lett. 1969 Aug;4(3):173–176. doi: 10.1016/0014-5793(69)80227-9. [DOI] [PubMed] [Google Scholar]
- Chen F. M. Acid-facilitated supramolecular assembly of G-quadruplexes in d(CGG)4. J Biol Chem. 1995 Sep 29;270(39):23090–23096. doi: 10.1074/jbc.270.39.23090. [DOI] [PubMed] [Google Scholar]
- Chen F. M. Sr2+ facilitates intermolecular G-quadruplex formation of telomeric sequences. Biochemistry. 1992 Apr 21;31(15):3769–3776. doi: 10.1021/bi00130a006. [DOI] [PubMed] [Google Scholar]
- Dai T. Y., Marotta S. P., Sheardy R. D. Self-assembly of DNA oligomers into high molecular weight species. Biochemistry. 1995 Mar 21;34(11):3655–3662. doi: 10.1021/bi00011a021. [DOI] [PubMed] [Google Scholar]
- Fry M., Loeb L. A. The fragile X syndrome d(CGG)n nucleotide repeats form a stable tetrahelical structure. Proc Natl Acad Sci U S A. 1994 May 24;91(11):4950–4954. doi: 10.1073/pnas.91.11.4950. [DOI] [PMC free article] [PubMed] [Google Scholar]
- Guo Q., Lu M., Kallenbach N. R. Effect of thymine tract length on the structure and stability of model telomeric sequences. Biochemistry. 1993 Apr 13;32(14):3596–3603. doi: 10.1021/bi00065a010. [DOI] [PubMed] [Google Scholar]
- Hardin C. C., Corregan M., Brown B. A., 2nd, Frederick L. N. Cytosine-cytosine+ base pairing stabilizes DNA quadruplexes and cytosine methylation greatly enhances the effect. Biochemistry. 1993 Jun 8;32(22):5870–5880. doi: 10.1021/bi00073a021. [DOI] [PubMed] [Google Scholar]
- Hardin C. C., Henderson E., Watson T., Prosser J. K. Monovalent cation induced structural transitions in telomeric DNAs: G-DNA folding intermediates. Biochemistry. 1991 May 7;30(18):4460–4472. doi: 10.1021/bi00232a013. [DOI] [PubMed] [Google Scholar]
- Hardin C. C., Watson T., Corregan M., Bailey C. Cation-dependent transition between the quadruplex and Watson-Crick hairpin forms of d(CGCG3GCG). Biochemistry. 1992 Jan 28;31(3):833–841. doi: 10.1021/bi00118a028. [DOI] [PubMed] [Google Scholar]
- Lee J. S. The stability of polypurine tetraplexes in the presence of mono- and divalent cations. Nucleic Acids Res. 1990 Oct 25;18(20):6057–6060. doi: 10.1093/nar/18.20.6057. [DOI] [PMC free article] [PubMed] [Google Scholar]
- Lu M., Guo Q., Kallenbach N. R. Structure and stability of sodium and potassium complexes of dT4G4 and dT4G4T. Biochemistry. 1992 Mar 10;31(9):2455–2459. doi: 10.1021/bi00124a003. [DOI] [PubMed] [Google Scholar]
- Maestre M. F., Reich C. Contribution of light scattering to the circular dichroism of deoxyribonucleic acid films, deoxyribonucleic acid-polylysine complexes, and deoxyribonucleic acid particles in ethanolic buffers. Biochemistry. 1980 Nov 11;19(23):5214–5223. doi: 10.1021/bi00564a010. [DOI] [PubMed] [Google Scholar]
- Marotta S. P., Tamburri P. A., Sheardy R. D. Sequence and environmental effects on the self-assembly of DNA oligomers possessing GxT2Gy segments. Biochemistry. 1996 Aug 13;35(32):10484–10492. doi: 10.1021/bi960979u. [DOI] [PubMed] [Google Scholar]
- Marsh T. C., Henderson E. G-wires: self-assembly of a telomeric oligonucleotide, d(GGGGTTGGGG), into large superstructures. Biochemistry. 1994 Sep 6;33(35):10718–10724. doi: 10.1021/bi00201a020. [DOI] [PubMed] [Google Scholar]
- Marsh T. C., Vesenka J., Henderson E. A new DNA nanostructure, the G-wire, imaged by scanning probe microscopy. Nucleic Acids Res. 1995 Feb 25;23(4):696–700. doi: 10.1093/nar/23.4.696. [DOI] [PMC free article] [PubMed] [Google Scholar]
- Protozanova E., Macgregor R. B., Jr Frayed wires: a thermally stable form of DNA with two distinct structural domains. Biochemistry. 1996 Dec 24;35(51):16638–16645. doi: 10.1021/bi960412d. [DOI] [PubMed] [Google Scholar]
- Reich C., Maestre M. F., Edmondson S., Gray D. M. Circular dichroism and fluorescence-detected circular dichroism of deoxyribonucleic acid and poly[d(A-C).d(G-T)] in ethanolic solutions: a new method for estimating circular intensity differential scattering. Biochemistry. 1980 Nov 11;19(23):5208–5213. doi: 10.1021/bi00564a009. [DOI] [PubMed] [Google Scholar]
- Rippe K., Fritsch V., Westhof E., Jovin T. M. Alternating d(G-A) sequences form a parallel-stranded DNA homoduplex. EMBO J. 1992 Oct;11(10):3777–3786. doi: 10.1002/j.1460-2075.1992.tb05463.x. [DOI] [PMC free article] [PubMed] [Google Scholar]
- Sen D., Gilbert W. Novel DNA superstructures formed by telomere-like oligomers. Biochemistry. 1992 Jan 14;31(1):65–70. doi: 10.1021/bi00116a011. [DOI] [PubMed] [Google Scholar]
- Shin Y. A., Eichhorn G. L. Formation of psi (+) and psi (-) DNA. Biopolymers. 1984 Feb;23(2):325–335. doi: 10.1002/bip.360230211. [DOI] [PubMed] [Google Scholar]
- Suda T., Mishima Y., Asakura H., Kominami R. Formation of a parallel-stranded DNA homoduplex by d(GGA) repeat oligonucleotides. Nucleic Acids Res. 1995 Sep 25;23(18):3771–3777. doi: 10.1093/nar/23.18.3771. [DOI] [PMC free article] [PubMed] [Google Scholar]