Abstract
The mathematical model of the compartmentalized energy transfer system in cardiac myocytes presented includes mitochondrial synthesis of ATP by ATP synthase, phosphocreatine production in the coupled mitochondrial creatine kinase reaction, the myofibrillar and cytoplasmic creatine kinase reactions, ATP utilization by actomyosin ATPase during the contraction cycle, and diffusional exchange of metabolites between different compartments. The model was used to calculate the changes in metabolite profiles during the cardiac cycle, metabolite and energy fluxes in different cellular compartments at high workload (corresponding to the rate of oxygen consumption of 46 mu atoms of O.(g wet mass)-1.min-1) under varying conditions of restricted ADP diffusion across mitochondrial outer membrane and creatine kinase isoenzyme "switchoff." In the complete system, restricted diffusion of ADP across the outer mitochondrial membrane stabilizes phosphocreatine production in cardiac mitochondria and increases the role of the phosphocreatine shuttle in energy transport and respiration regulation. Selective inhibition of myoplasmic or mitochondrial creatine kinase (modeling the experiments with transgenic animals) results in "takeover" of their function by another, active creatine kinase isoenzyme. This mathematical modeling also shows that assumption of the creatine kinase equilibrium in the cell may only be a very rough approximation to the reality at increased workload. The mathematical model developed can be used as a basis for further quantitative analyses of energy fluxes in the cell and their regulation, particularly by adding modules for adenylate kinase, the glycolytic system, and other reactions of energy metabolism of the cell.
Full text
PDF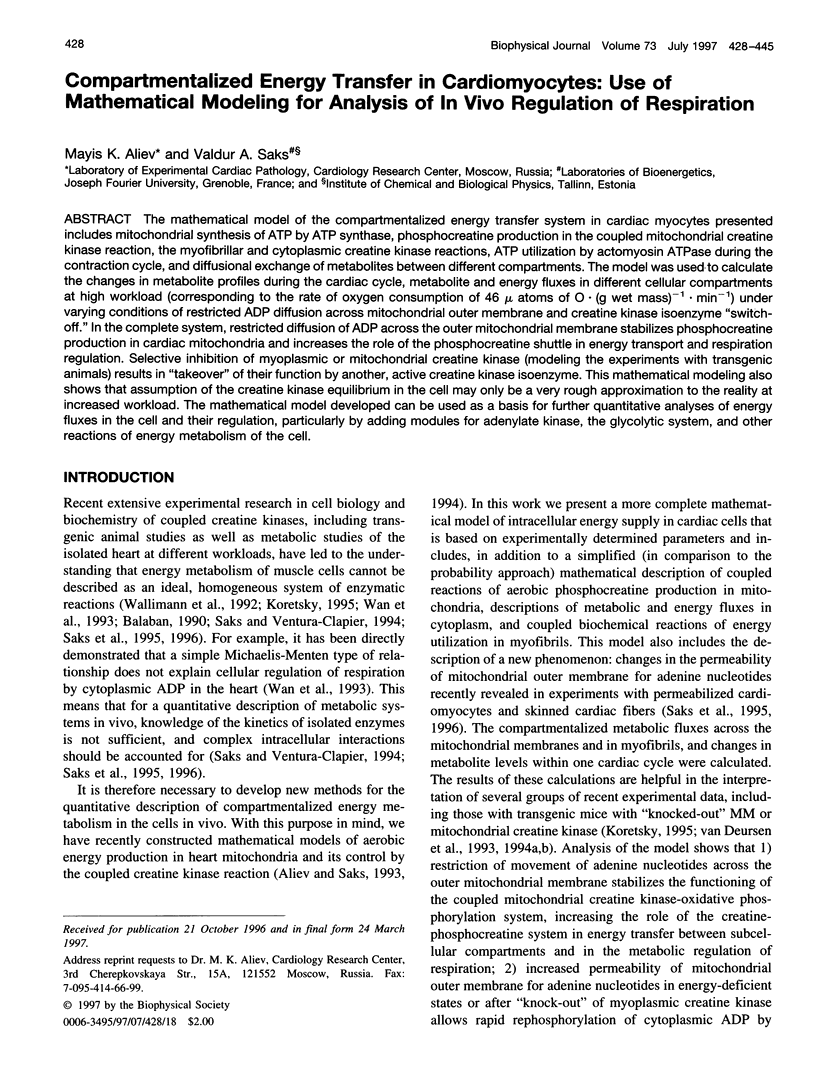
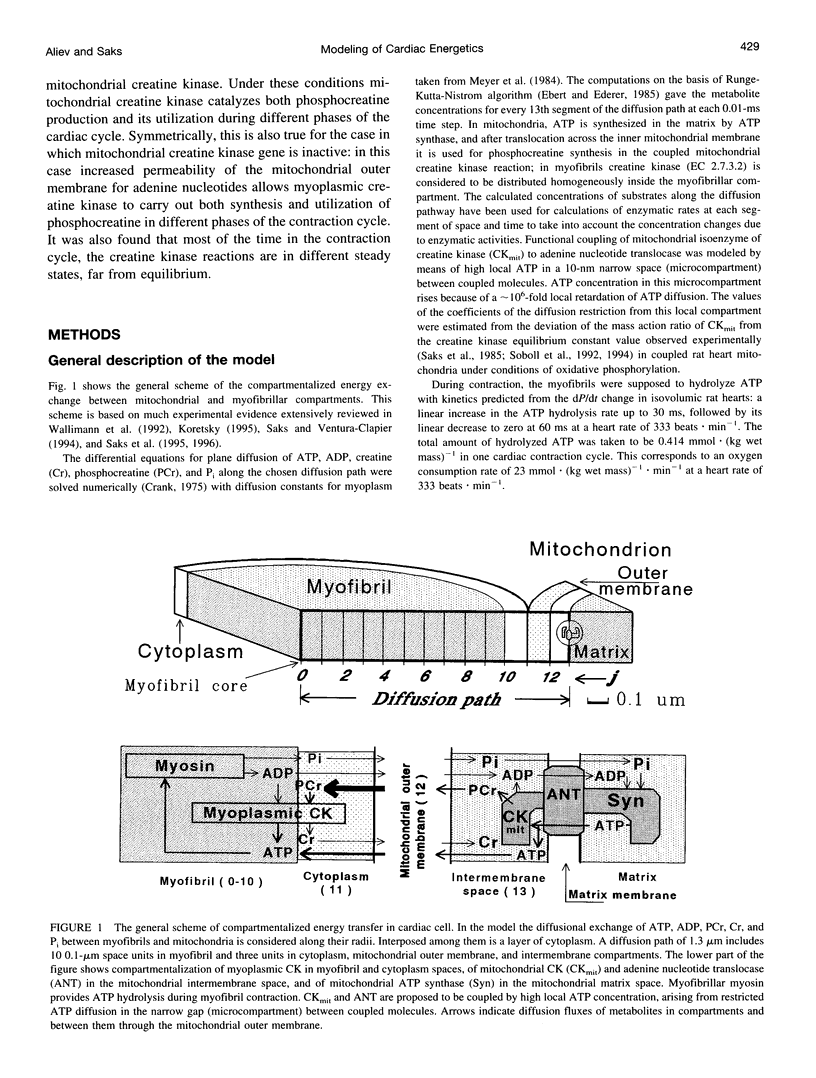
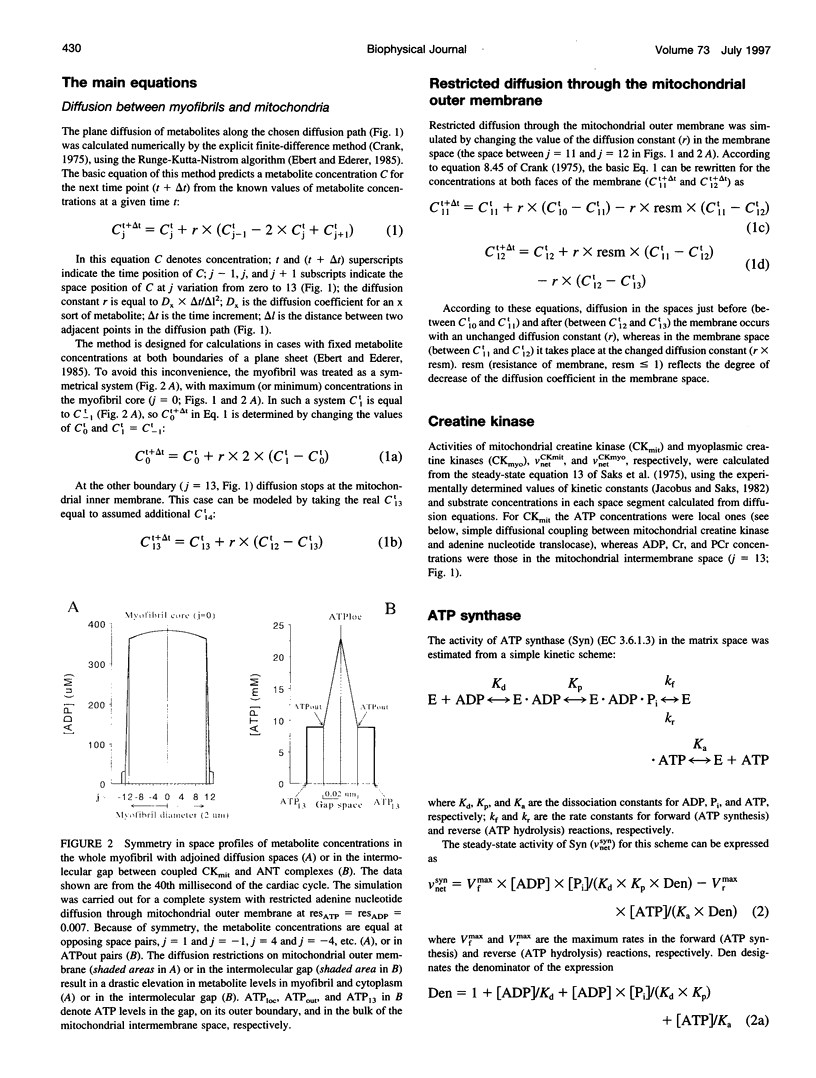
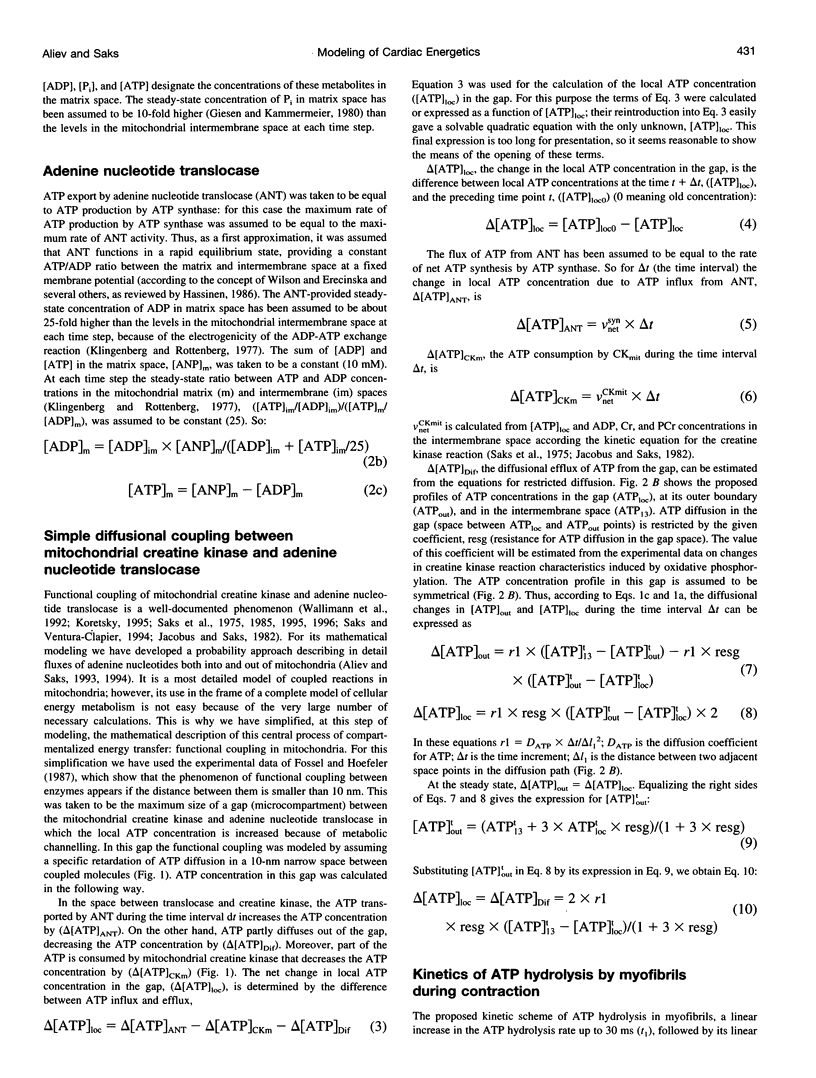
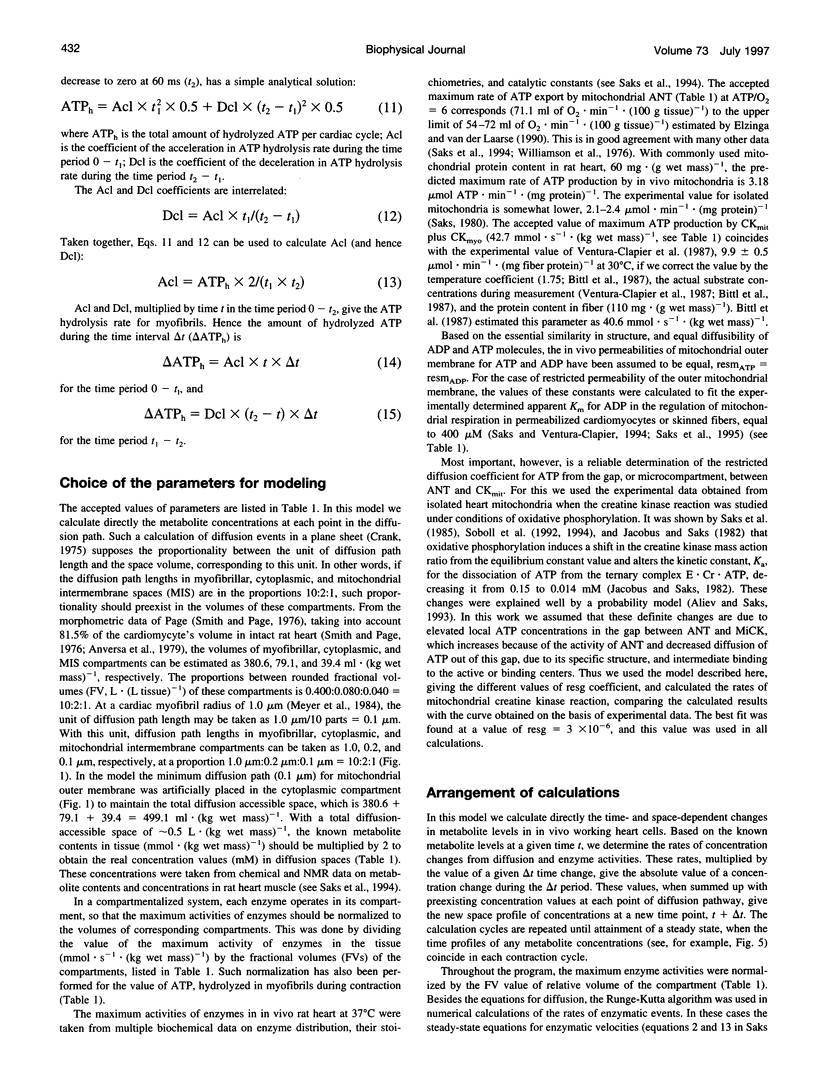
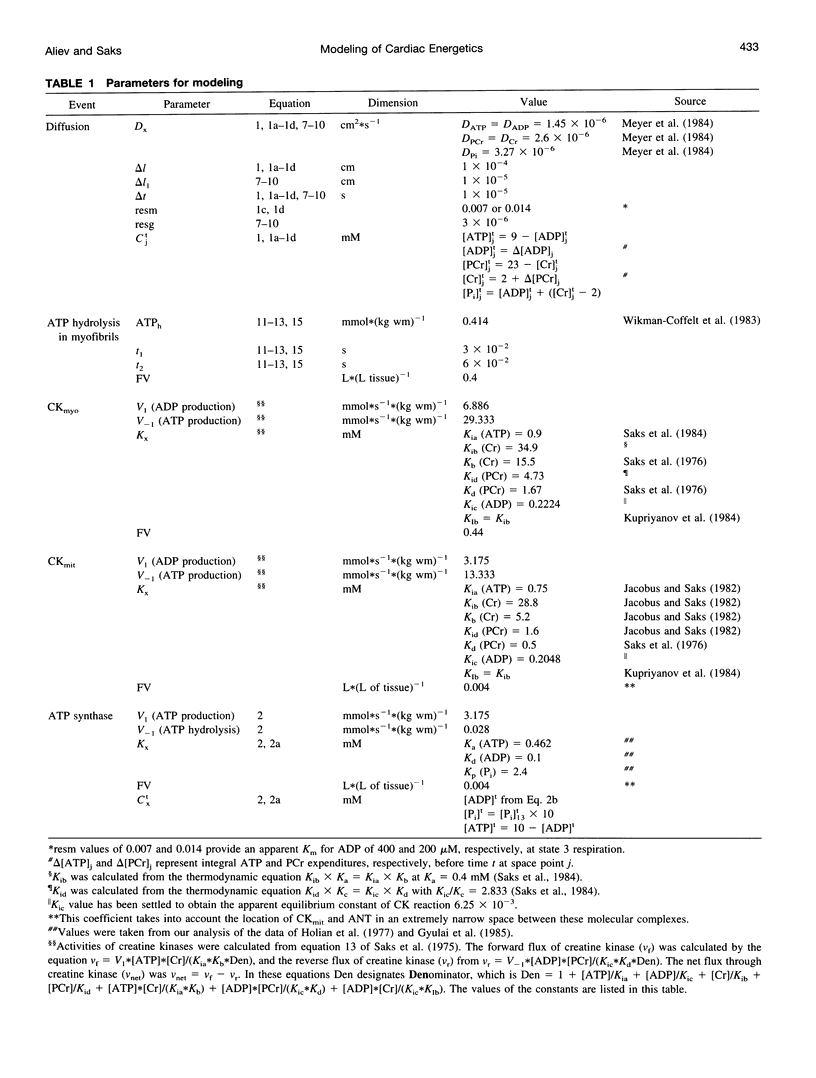
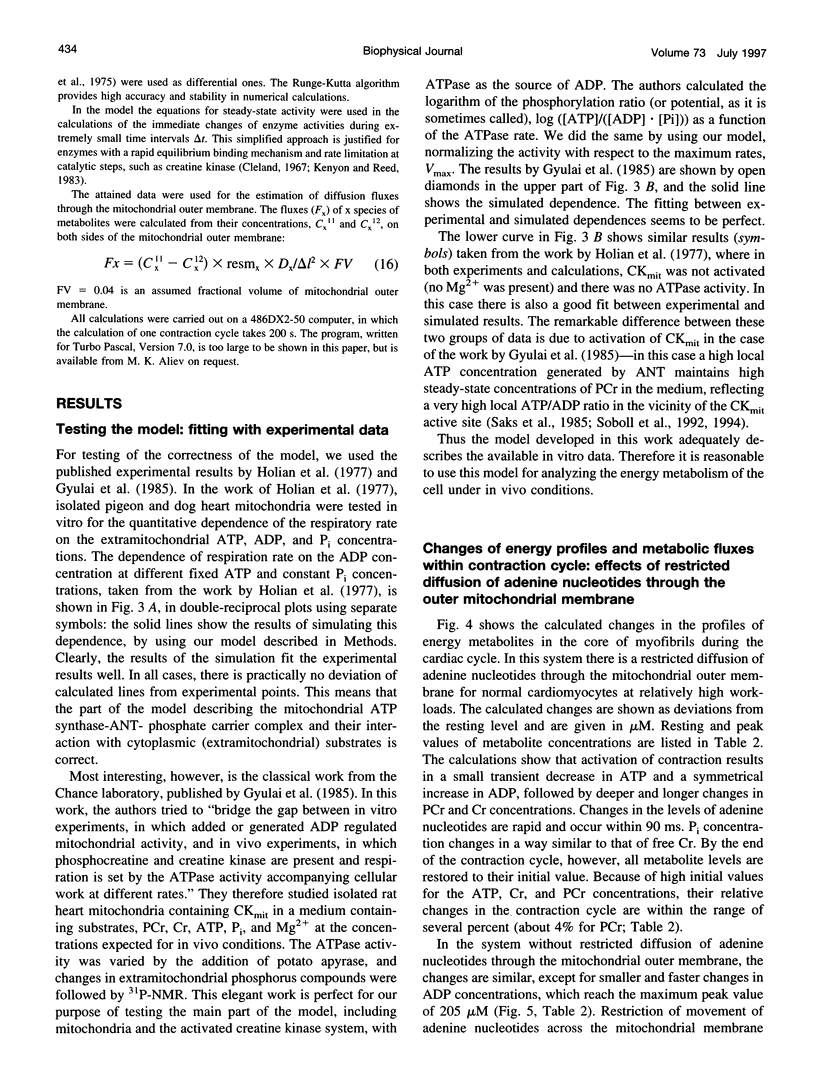
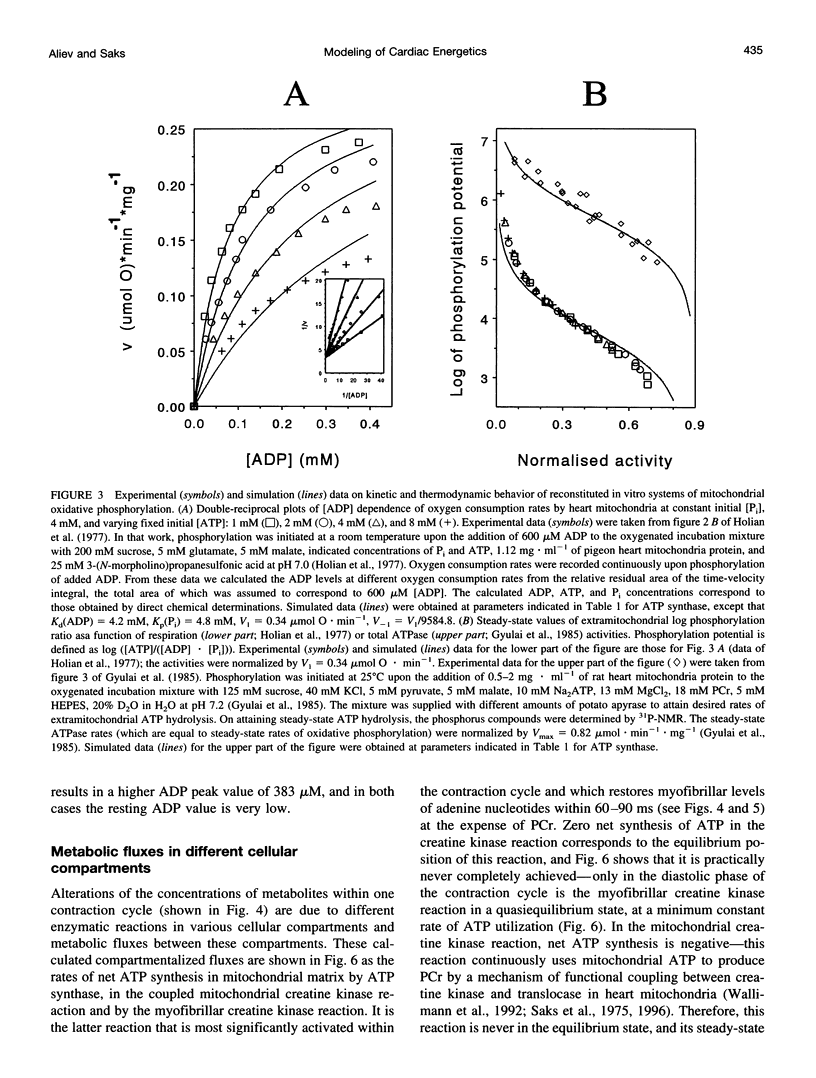
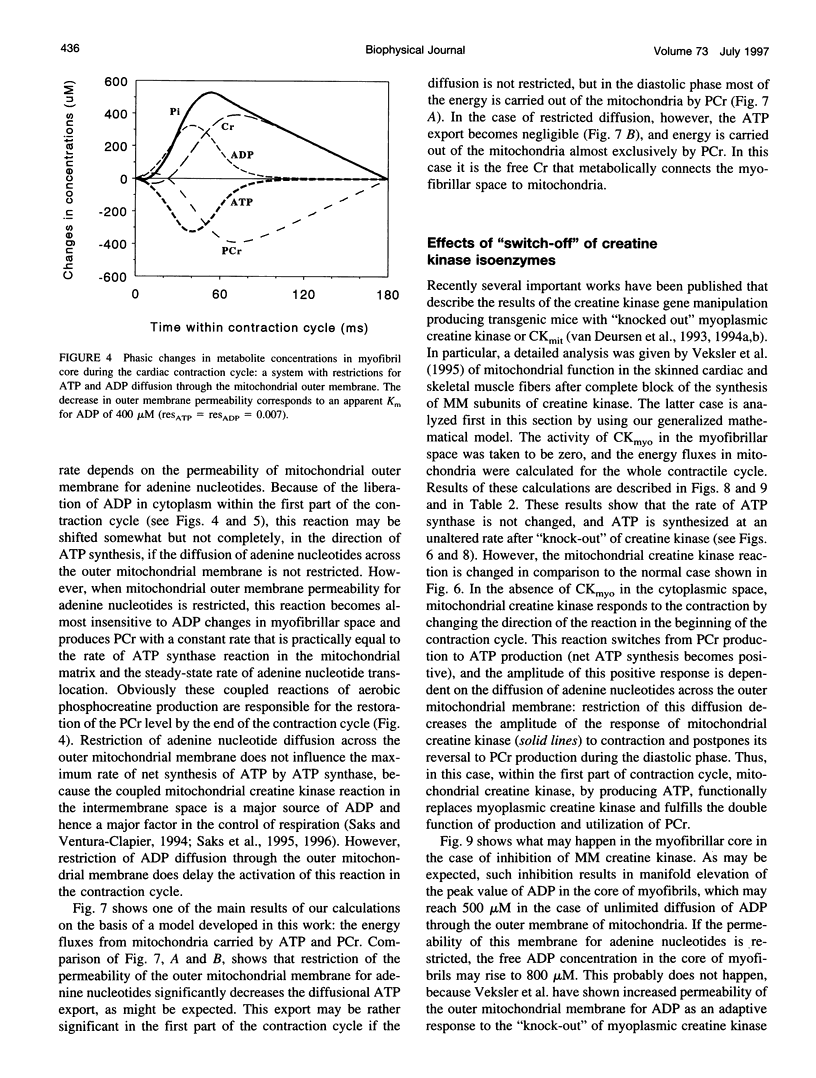
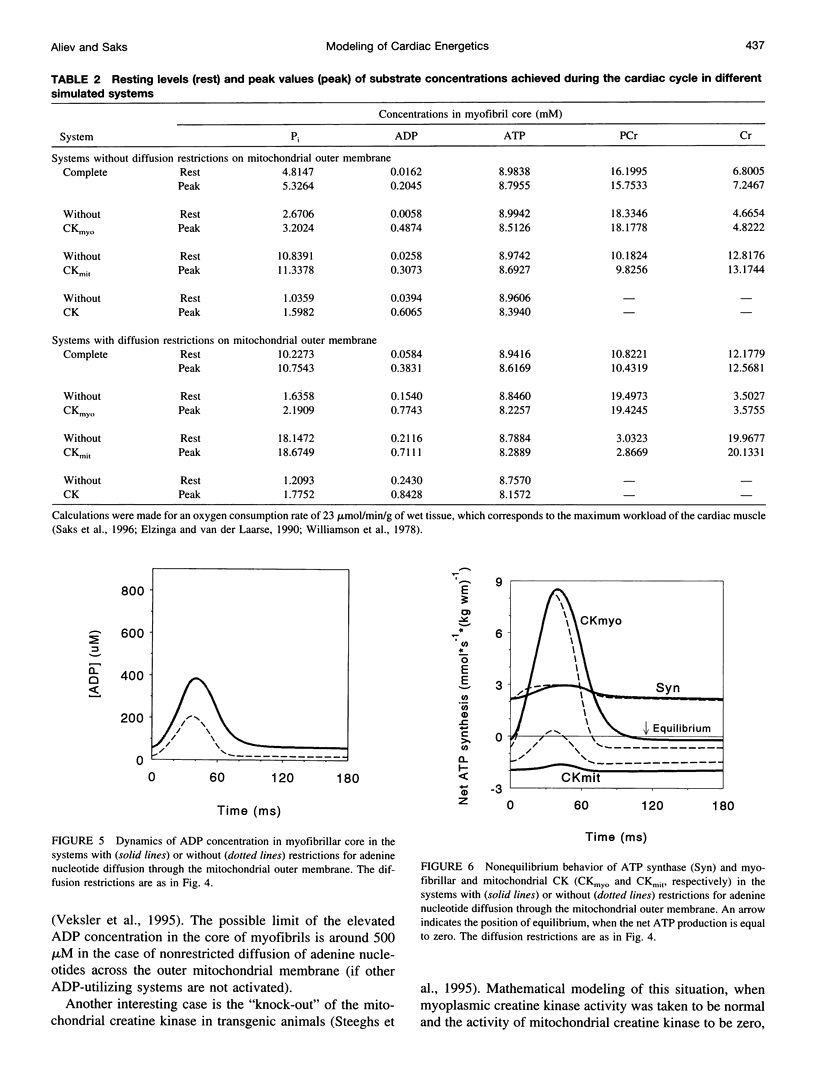
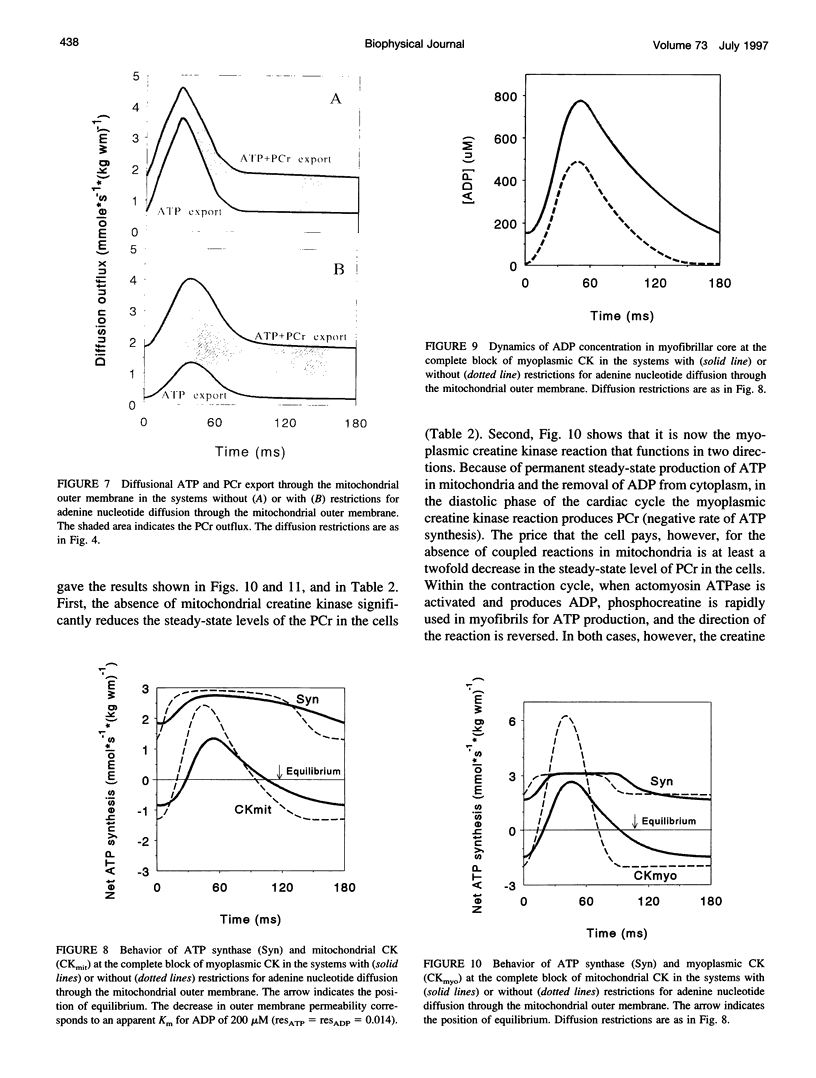
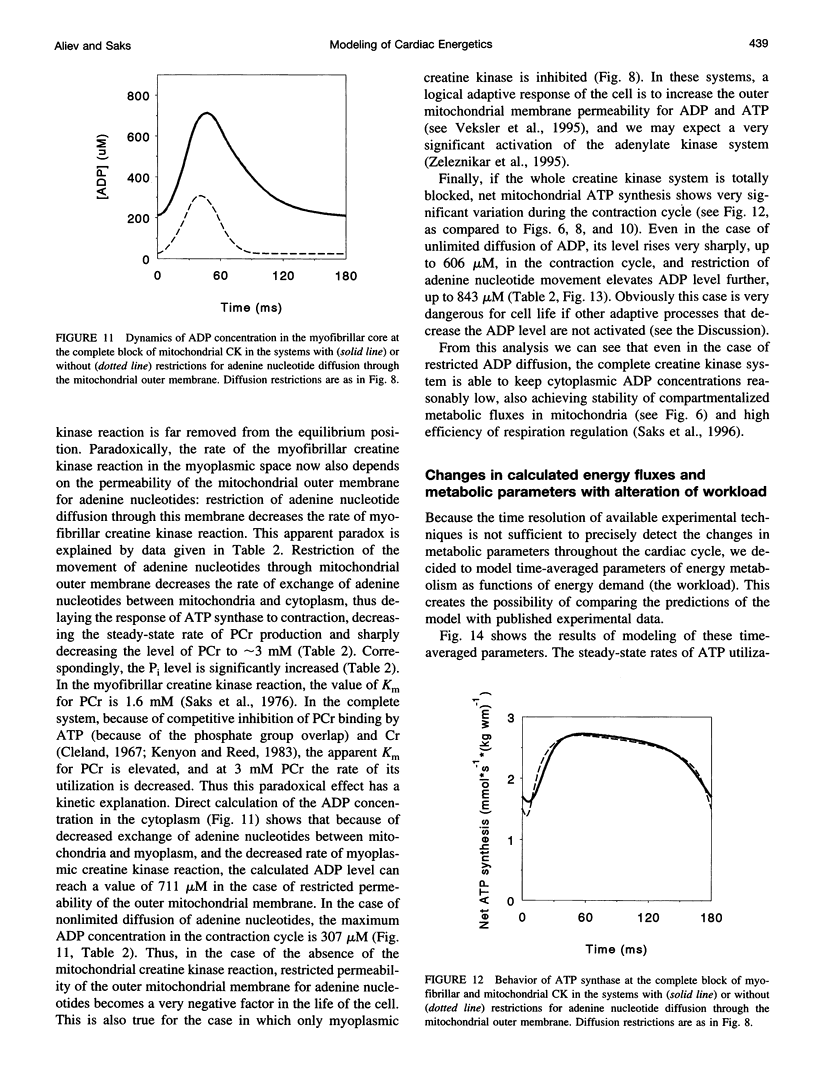
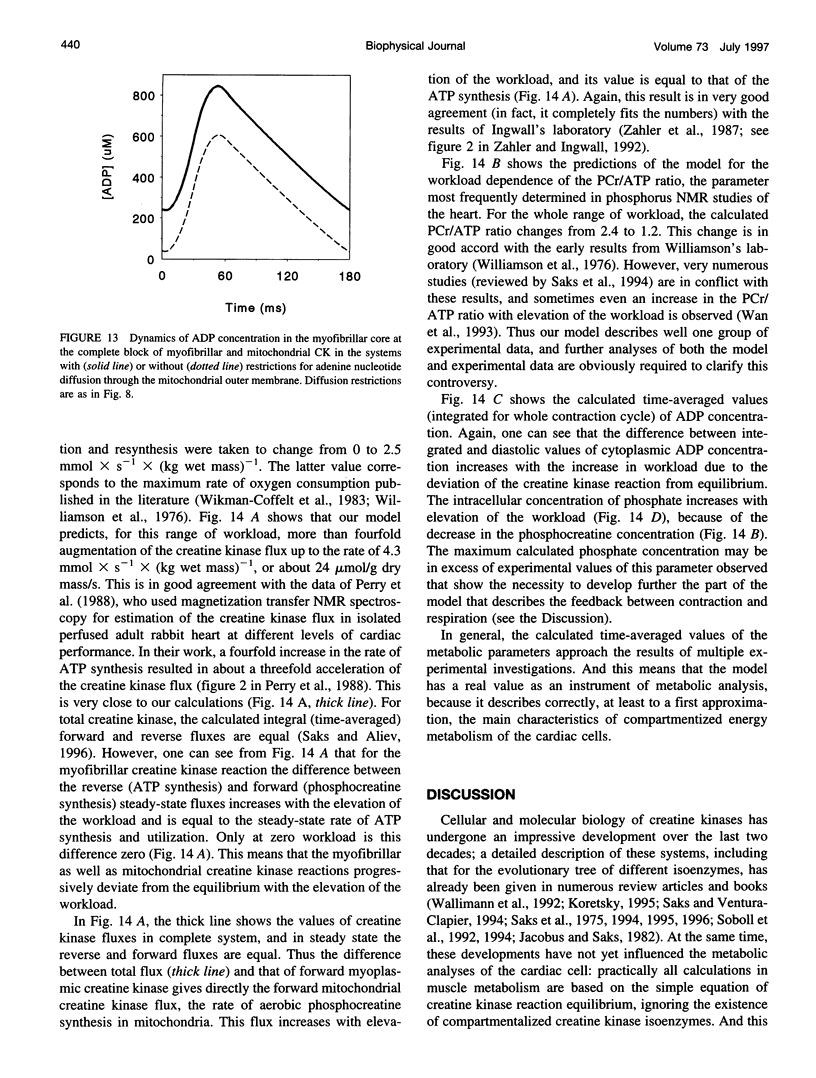
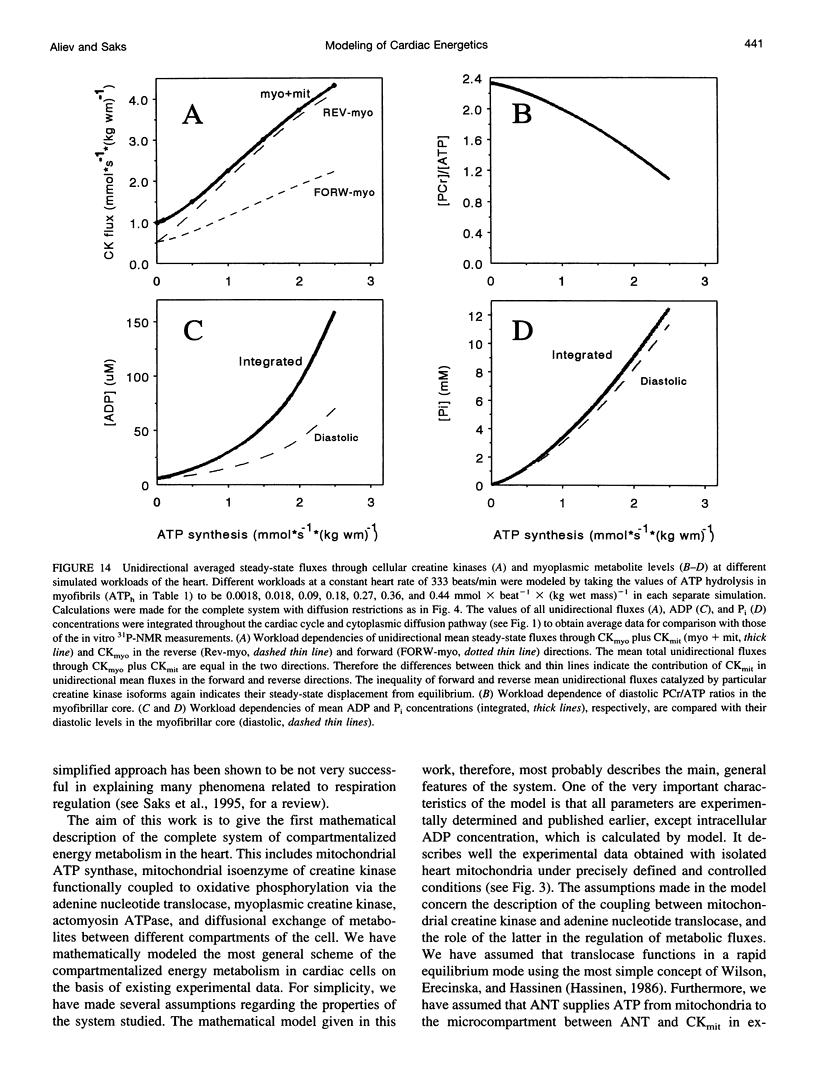
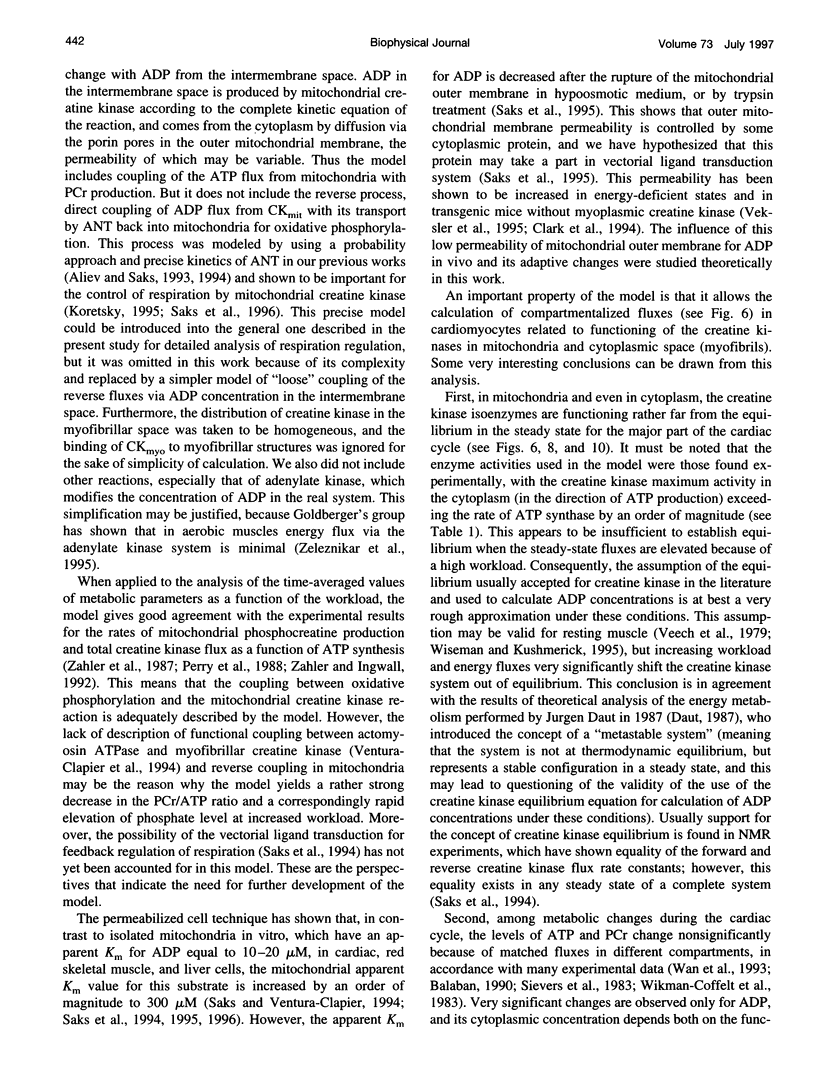
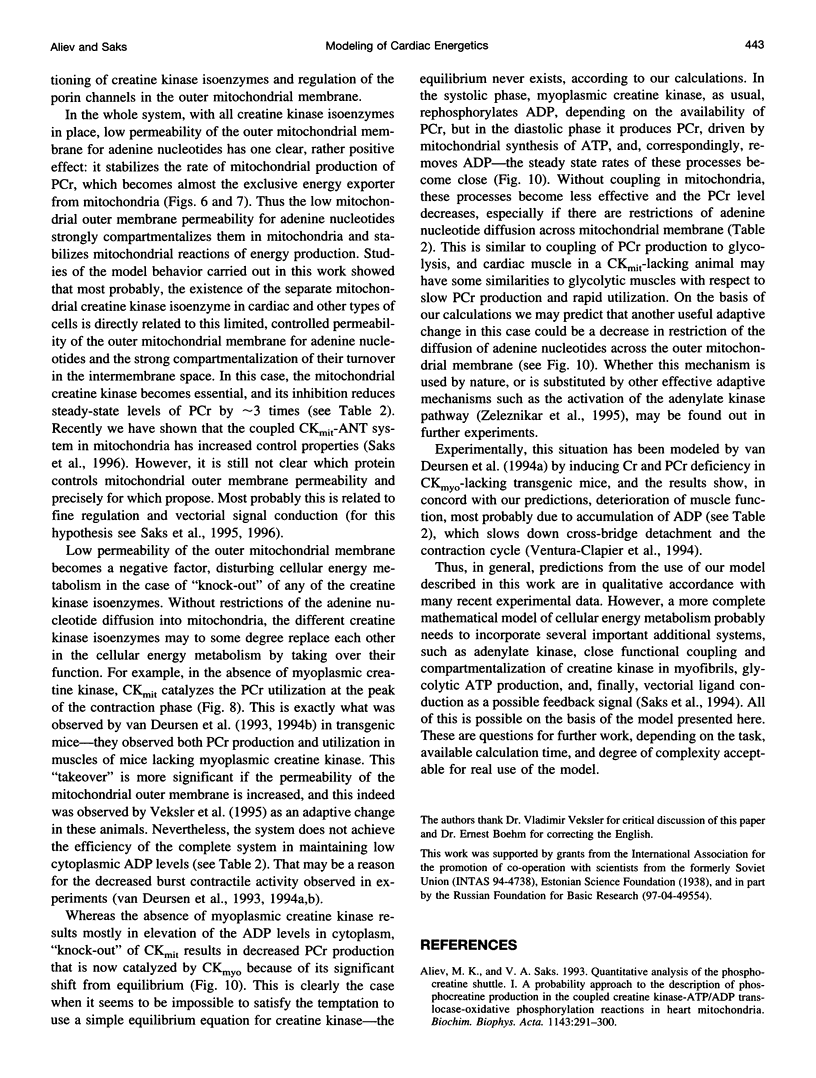
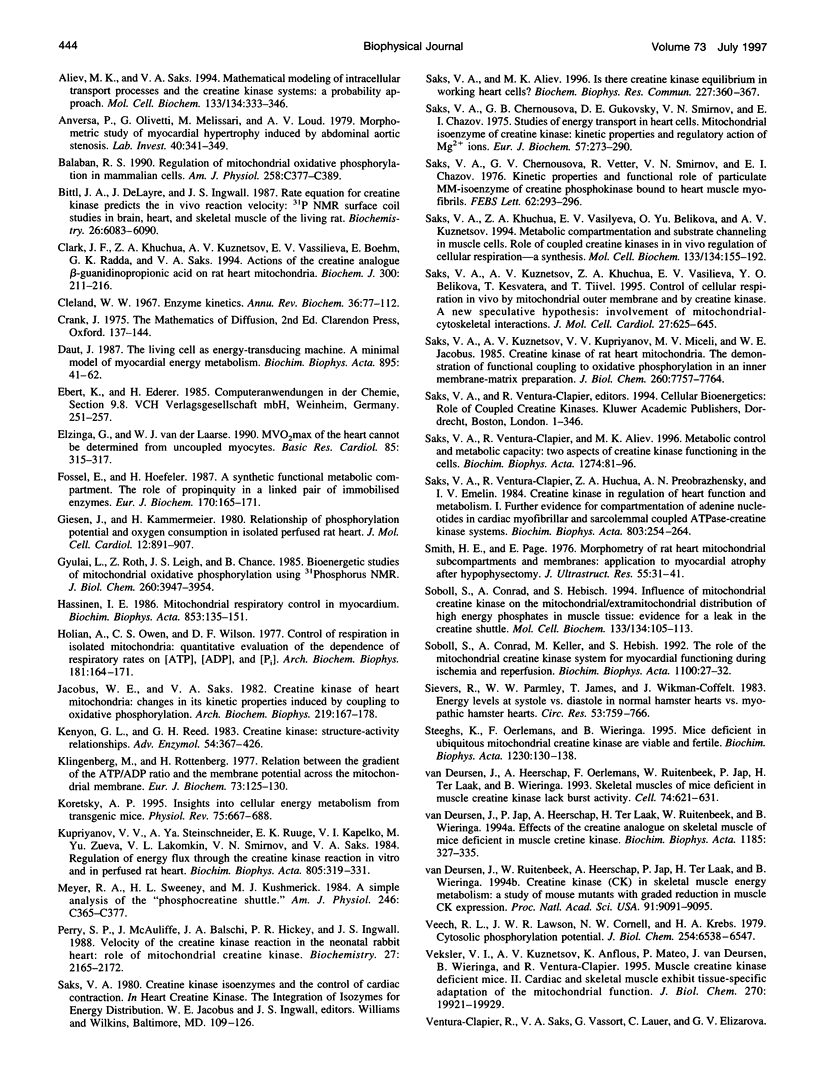
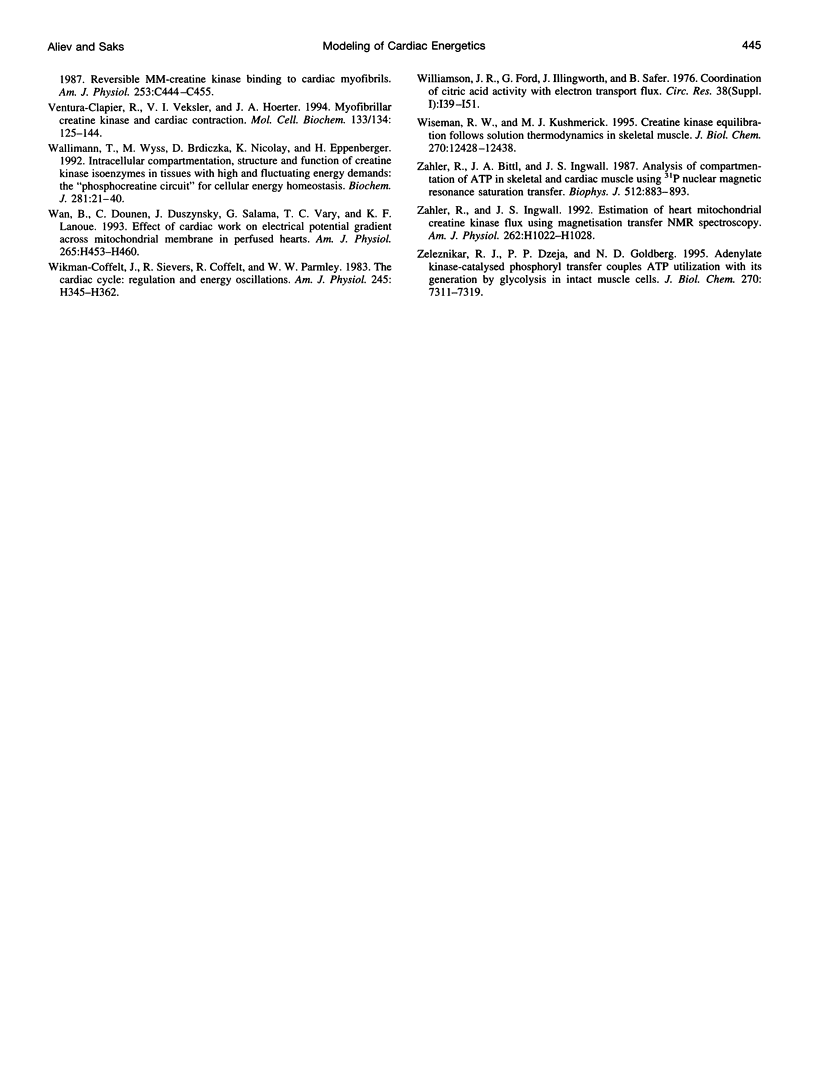
Images in this article
Selected References
These references are in PubMed. This may not be the complete list of references from this article.
- Aliev M. K., Saks V. A. Mathematical modeling of intracellular transport processes and the creatine kinase systems: a probability approach. Mol Cell Biochem. 1994 Apr-May;133-134:333–346. [PubMed] [Google Scholar]
- Aliev M. K., Saks V. A. Quantitative analysis of the 'phosphocreatine shuttle': I. A probability approach to the description of phosphocreatine production in the coupled creatine kinase-ATP/ADP translocase-oxidative phosphorylation reactions in heart mitochondria. Biochim Biophys Acta. 1993 Jul 26;1143(3):291–300. doi: 10.1016/0005-2728(93)90200-y. [DOI] [PubMed] [Google Scholar]
- Anversa P., Olivetti G., Melissari M., Loud A. V. Morphometric study of myocardial hypertrophy induced by abdominal aortic stenosis. Lab Invest. 1979 Mar;40(3):341–349. [PubMed] [Google Scholar]
- Balaban R. S. Regulation of oxidative phosphorylation in the mammalian cell. Am J Physiol. 1990 Mar;258(3 Pt 1):C377–C389. doi: 10.1152/ajpcell.1990.258.3.C377. [DOI] [PubMed] [Google Scholar]
- Bittl J. A., DeLayre J., Ingwall J. S. Rate equation for creatine kinase predicts the in vivo reaction velocity: 31P NMR surface coil studies in brain, heart, and skeletal muscle of the living rat. Biochemistry. 1987 Sep 22;26(19):6083–6090. doi: 10.1021/bi00393a021. [DOI] [PubMed] [Google Scholar]
- Clark J. F., Khuchua Z., Kuznetsov A. V., Vassil'eva E., Boehm E., Radda G. K., Saks V. Actions of the creatine analogue beta-guanidinopropionic acid on rat heart mitochondria. Biochem J. 1994 May 15;300(Pt 1):211–216. doi: 10.1042/bj3000211. [DOI] [PMC free article] [PubMed] [Google Scholar]
- Daut J. The living cell as an energy-transducing machine. A minimal model of myocardial metabolism. Biochim Biophys Acta. 1987;895(1):41–62. doi: 10.1016/s0304-4173(87)80016-2. [DOI] [PubMed] [Google Scholar]
- Elzinga G., van der Laarse W. J. MVO2max of the heart cannot be determined from uncoupled myocytes. Basic Res Cardiol. 1990 Jul-Aug;85(4):315–317. doi: 10.1007/BF01907124. [DOI] [PubMed] [Google Scholar]
- Fossel E. T., Hoefeler H. A synthetic functional metabolic compartment. The role of propinquity in a linked pair of immobilized enzymes. Eur J Biochem. 1987 Dec 30;170(1-2):165–171. doi: 10.1111/j.1432-1033.1987.tb13682.x. [DOI] [PubMed] [Google Scholar]
- Giesen J., Kammermeier H. Relationship of phosphorylation potential and oxygen consumption in isolated perfused rat hearts. J Mol Cell Cardiol. 1980 Sep;12(9):891–907. doi: 10.1016/0022-2828(80)90058-9. [DOI] [PubMed] [Google Scholar]
- Gyulai L., Roth Z., Leigh J. S., Jr, Chance B. Bioenergetic studies of mitochondrial oxidative phosphorylation using 31phosphorus NMR. J Biol Chem. 1985 Apr 10;260(7):3947–3954. [PubMed] [Google Scholar]
- Hassinen I. E. Mitochondrial respiratory control in the myocardium. Biochim Biophys Acta. 1986;853(2):135–151. doi: 10.1016/0304-4173(86)90008-x. [DOI] [PubMed] [Google Scholar]
- Holian A., Owen C. S., Wilson D. F. Control of respiration in isolated mitochondria: quantitative evaluation of the dependence of respiratory rates on [ATP], [ADP], and [Pi]. Arch Biochem Biophys. 1977 May;181(1):164–171. doi: 10.1016/0003-9861(77)90494-5. [DOI] [PubMed] [Google Scholar]
- Jacobus W. E., Saks V. A. Creatine kinase of heart mitochondria: changes in its kinetic properties induced by coupling to oxidative phosphorylation. Arch Biochem Biophys. 1982 Nov;219(1):167–178. doi: 10.1016/0003-9861(82)90146-1. [DOI] [PubMed] [Google Scholar]
- Kenyon G. L., Reed G. H. Creatine kinase: structure-activity relationships. Adv Enzymol Relat Areas Mol Biol. 1983;54:367–426. doi: 10.1002/9780470122990.ch6. [DOI] [PubMed] [Google Scholar]
- Klingenberg M., Rottenberg H. Relation between the gradient of the ATP/ADP ratio and the membrane potential across the mitochondrial membrane. Eur J Biochem. 1977 Feb 15;73(1):125–130. doi: 10.1111/j.1432-1033.1977.tb11298.x. [DOI] [PubMed] [Google Scholar]
- Koretsky A. P. Insights into cellular energy metabolism from transgenic mice. Physiol Rev. 1995 Oct;75(4):667–688. doi: 10.1152/physrev.1995.75.4.667. [DOI] [PubMed] [Google Scholar]
- Kupriyanov V. V., Ya Steinschneider A., Ruuge E. K., Kapel'ko V. I., Yu Zueva M., Lakomkin V. L., Smirnov V. N., Saks V. A. Regulation of energy flux through the creatine kinase reaction in vitro and in perfused rat heart. 31P-NMR studies. Biochim Biophys Acta. 1984 Dec 11;805(4):319–331. doi: 10.1016/0167-4889(84)90014-4. [DOI] [PubMed] [Google Scholar]
- Meyer R. A., Sweeney H. L., Kushmerick M. J. A simple analysis of the "phosphocreatine shuttle". Am J Physiol. 1984 May;246(5 Pt 1):C365–C377. doi: 10.1152/ajpcell.1984.246.5.C365. [DOI] [PubMed] [Google Scholar]
- Perry S. B., McAuliffe J., Balschi J. A., Hickey P. R., Ingwall J. S. Velocity of the creatine kinase reaction in the neonatal rabbit heart: role of mitochondrial creatine kinase. Biochemistry. 1988 Mar 22;27(6):2165–2172. doi: 10.1021/bi00406a052. [DOI] [PubMed] [Google Scholar]
- Saks V. A., Aliev M. K. Is there the creatine kinase equilibrium in working heart cells? Biochem Biophys Res Commun. 1996 Oct 14;227(2):360–367. doi: 10.1006/bbrc.1996.1513. [DOI] [PubMed] [Google Scholar]
- Saks V. A., Chernousova G. B., Gukovsky D. E., Smirnov V. N., Chazov E. I. Studies of energy transport in heart cells. Mitochondrial isoenzyme of creatine phosphokinase: kinetic properties and regulatory action of Mg2+ ions. Eur J Biochem. 1975 Sep 1;57(1):273–290. doi: 10.1111/j.1432-1033.1975.tb02299.x. [DOI] [PubMed] [Google Scholar]
- Saks V. A., Chernousova G. B., Vetter R., Smirnov V. N., Chazov E. I. Kinetic properties and the functional role of particulate MM-isoenzyme of creatine phosphokinase bound to heart muscle myofibrils. FEBS Lett. 1976 Mar 1;62(3):293–296. doi: 10.1016/0014-5793(76)80078-6. [DOI] [PubMed] [Google Scholar]
- Saks V. A., Khuchua Z. A., Vasilyeva E. V., Belikova OYu, Kuznetsov A. V. Metabolic compartmentation and substrate channelling in muscle cells. Role of coupled creatine kinases in in vivo regulation of cellular respiration--a synthesis. Mol Cell Biochem. 1994 Apr-May;133-134:155–192. doi: 10.1007/BF01267954. [DOI] [PubMed] [Google Scholar]
- Saks V. A., Kuznetsov A. V., Khuchua Z. A., Vasilyeva E. V., Belikova J. O., Kesvatera T., Tiivel T. Control of cellular respiration in vivo by mitochondrial outer membrane and by creatine kinase. A new speculative hypothesis: possible involvement of mitochondrial-cytoskeleton interactions. J Mol Cell Cardiol. 1995 Jan;27(1):625–645. doi: 10.1016/s0022-2828(08)80056-9. [DOI] [PubMed] [Google Scholar]
- Saks V. A., Kuznetsov A. V., Kupriyanov V. V., Miceli M. V., Jacobus W. E. Creatine kinase of rat heart mitochondria. The demonstration of functional coupling to oxidative phosphorylation in an inner membrane-matrix preparation. J Biol Chem. 1985 Jun 25;260(12):7757–7764. [PubMed] [Google Scholar]
- Saks V. A., Ventura-Clapier R., Aliev M. K. Metabolic control and metabolic capacity: two aspects of creatine kinase functioning in the cells. Biochim Biophys Acta. 1996 Jun 13;1274(3):81–88. doi: 10.1016/0005-2728(96)00011-4. [DOI] [PubMed] [Google Scholar]
- Saks V. A., Ventura-Clapier R., Huchua Z. A., Preobrazhensky A. N., Emelin I. V. Creatine kinase in regulation of heart function and metabolism. I. Further evidence for compartmentation of adenine nucleotides in cardiac myofibrillar and sarcolemmal coupled ATPase-creatine kinase systems. Biochim Biophys Acta. 1984 Apr 16;803(4):254–264. doi: 10.1016/0167-4889(84)90115-0. [DOI] [PubMed] [Google Scholar]
- Sievers R., Parmley W. W., James T., Wikman-Coffelt J. Energy levels at systole vs. diastole in normal hamster hearts vs. myopathic hamster hearts. Circ Res. 1983 Dec;53(6):759–766. doi: 10.1161/01.res.53.6.759. [DOI] [PubMed] [Google Scholar]
- Smith H. E., Page E. Morphometry of rat heart mitochondrial subcompartments and membranes: application to myocardial cell atrophy after hypophysectomy. J Ultrastruct Res. 1976 Apr;55(1):31–41. doi: 10.1016/s0022-5320(76)80079-2. [DOI] [PubMed] [Google Scholar]
- Soboll S., Conrad A., Hebisch S. Influence of mitochondrial creatine kinase on the mitochondrial/extramitochondrial distribution of high energy phosphates in muscle tissue: evidence for a leak in the creatine shuttle. Mol Cell Biochem. 1994 Apr-May;133-134:105–113. doi: 10.1007/BF01267950. [DOI] [PubMed] [Google Scholar]
- Soboll S., Conrad A., Keller M., Hebisch S. The role of the mitochondrial creatine kinase system for myocardial function during ischemia and reperfusion. Biochim Biophys Acta. 1992 Apr 10;1100(1):27–32. doi: 10.1016/0005-2728(92)90122-i. [DOI] [PubMed] [Google Scholar]
- Steeghs K., Oerlemans F., Wieringa B. Mice deficient in ubiquitous mitochondrial creatine kinase are viable and fertile. Biochim Biophys Acta. 1995 Jun 30;1230(3):130–138. doi: 10.1016/0005-2728(95)00044-j. [DOI] [PubMed] [Google Scholar]
- Veech R. L., Lawson J. W., Cornell N. W., Krebs H. A. Cytosolic phosphorylation potential. J Biol Chem. 1979 Jul 25;254(14):6538–6547. [PubMed] [Google Scholar]
- Veksler V. I., Kuznetsov A. V., Anflous K., Mateo P., van Deursen J., Wieringa B., Ventura-Clapier R. Muscle creatine kinase-deficient mice. II. Cardiac and skeletal muscles exhibit tissue-specific adaptation of the mitochondrial function. J Biol Chem. 1995 Aug 25;270(34):19921–19929. doi: 10.1074/jbc.270.34.19921. [DOI] [PubMed] [Google Scholar]
- Ventura-Clapier R., Saks V. A., Vassort G., Lauer C., Elizarova G. V. Reversible MM-creatine kinase binding to cardiac myofibrils. Am J Physiol. 1987 Sep;253(3 Pt 1):C444–C455. doi: 10.1152/ajpcell.1987.253.3.C444. [DOI] [PubMed] [Google Scholar]
- Ventura-Clapier R., Veksler V., Hoerter J. A. Myofibrillar creatine kinase and cardiac contraction. Mol Cell Biochem. 1994 Apr-May;133-134:125–144. doi: 10.1007/BF01267952. [DOI] [PubMed] [Google Scholar]
- Wallimann T., Wyss M., Brdiczka D., Nicolay K., Eppenberger H. M. Intracellular compartmentation, structure and function of creatine kinase isoenzymes in tissues with high and fluctuating energy demands: the 'phosphocreatine circuit' for cellular energy homeostasis. Biochem J. 1992 Jan 1;281(Pt 1):21–40. doi: 10.1042/bj2810021. [DOI] [PMC free article] [PubMed] [Google Scholar]
- Wan B., Doumen C., Duszynski J., Salama G., Vary T. C., LaNoue K. F. Effects of cardiac work on electrical potential gradient across mitochondrial membrane in perfused rat hearts. Am J Physiol. 1993 Aug;265(2 Pt 2):H453–H460. doi: 10.1152/ajpheart.1993.265.2.H453. [DOI] [PubMed] [Google Scholar]
- Wikman-Coffelt J., Sievers R., Coffelt R. J., Parmley W. W. The cardiac cycle: regulation and energy oscillations. Am J Physiol. 1983 Aug;245(2):H354–H362. doi: 10.1152/ajpheart.1983.245.2.H354. [DOI] [PubMed] [Google Scholar]
- Williamson J. R., Ford C., Illingworth J., Safer B. Coordination of citric acid cycle activity with electron transport flux. Circ Res. 1976 May;38(5 Suppl 1):I39–I51. [PubMed] [Google Scholar]
- Wiseman R. W., Kushmerick M. J. Creatine kinase equilibration follows solution thermodynamics in skeletal muscle. 31P NMR studies using creatine analogs. J Biol Chem. 1995 May 26;270(21):12428–12438. doi: 10.1074/jbc.270.21.12428. [DOI] [PubMed] [Google Scholar]
- Zahler R., Bittl J. A., Ingwall J. S. Analysis of compartmentation of ATP in skeletal and cardiac muscle using 31P nuclear magnetic resonance saturation transfer. Biophys J. 1987 Jun;51(6):883–893. doi: 10.1016/S0006-3495(87)83416-1. [DOI] [PMC free article] [PubMed] [Google Scholar]
- Zahler R., Ingwall J. S. Estimation of heart mitochondrial creatine kinase flux using magnetization transfer NMR spectroscopy. Am J Physiol. 1992 Apr;262(4 Pt 2):H1022–H1028. doi: 10.1152/ajpheart.1992.262.4.H1022. [DOI] [PubMed] [Google Scholar]
- Zeleznikar R. J., Dzeja P. P., Goldberg N. D. Adenylate kinase-catalyzed phosphoryl transfer couples ATP utilization with its generation by glycolysis in intact muscle. J Biol Chem. 1995 Mar 31;270(13):7311–7319. doi: 10.1074/jbc.270.13.7311. [DOI] [PubMed] [Google Scholar]
- van Deursen J., Heerschap A., Oerlemans F., Ruitenbeek W., Jap P., ter Laak H., Wieringa B. Skeletal muscles of mice deficient in muscle creatine kinase lack burst activity. Cell. 1993 Aug 27;74(4):621–631. doi: 10.1016/0092-8674(93)90510-w. [DOI] [PubMed] [Google Scholar]
- van Deursen J., Jap P., Heerschap A., ter Laak H., Ruitenbeek W., Wieringa B. Effects of the creatine analogue beta-guanidinopropionic acid on skeletal muscles of mice deficient in muscle creatine kinase. Biochim Biophys Acta. 1994 May 18;1185(3):327–335. doi: 10.1016/0005-2728(94)90248-8. [DOI] [PubMed] [Google Scholar]
- van Deursen J., Ruitenbeek W., Heerschap A., Jap P., ter Laak H., Wieringa B. Creatine kinase (CK) in skeletal muscle energy metabolism: a study of mouse mutants with graded reduction in muscle CK expression. Proc Natl Acad Sci U S A. 1994 Sep 13;91(19):9091–9095. doi: 10.1073/pnas.91.19.9091. [DOI] [PMC free article] [PubMed] [Google Scholar]