Abstract
The force-generation and phosphate-release steps of the cross-bridge cycle in rabbit soleus slow-twitch muscle fibers (STF) were investigated using sinusoidal analysis, and the results were compared with those of rabbit psoas fast-twitch fibers (FTF). Single fiber preparations were activated at pCa 4.40 and ionic strength 180 mM at 20 degrees C. The effects of inorganic phosphate (Pi) concentrations on three exponential processes, B, C, and D, were studied. Results are consistent with the following cross-bridge scheme: [formula: see text] where A is actin, M is myosin, D is MgADP, and P is inorganic phosphate. The values determined are k4 = 5.7 +/- 0.5 s-1 (rate constant of isomerization step, N = 9, mean +/- SE), k-4 = 4.5 +/- 0.5 s-1 (rate constant of reverse isomerization), K4 = 1.37 +/- 0.13 (equilibrium constant of the isomerization), and K5 = 0.18 +/- 0.01 mM-1 (Pi association constant). The isomerization step (k4) in soleus STF is 20 times slower, and its reversal (k-4) is 20 times slower than psoas fibers. Consequently, the equilibrium constant of the isomerization step (K4) is the same in these two types of fibers. The Pi association constant (K5) is slightly higher in STF than in FTF, indicating that Pi binds to cross-bridges slightly more tightly in STF than FTF. By correlating the cross-bridge distribution with isometric tension, it was confirmed that force is generated during the isomerization (step 4) of the AMDP state and before Pi release in soleus STF.
Full text
PDF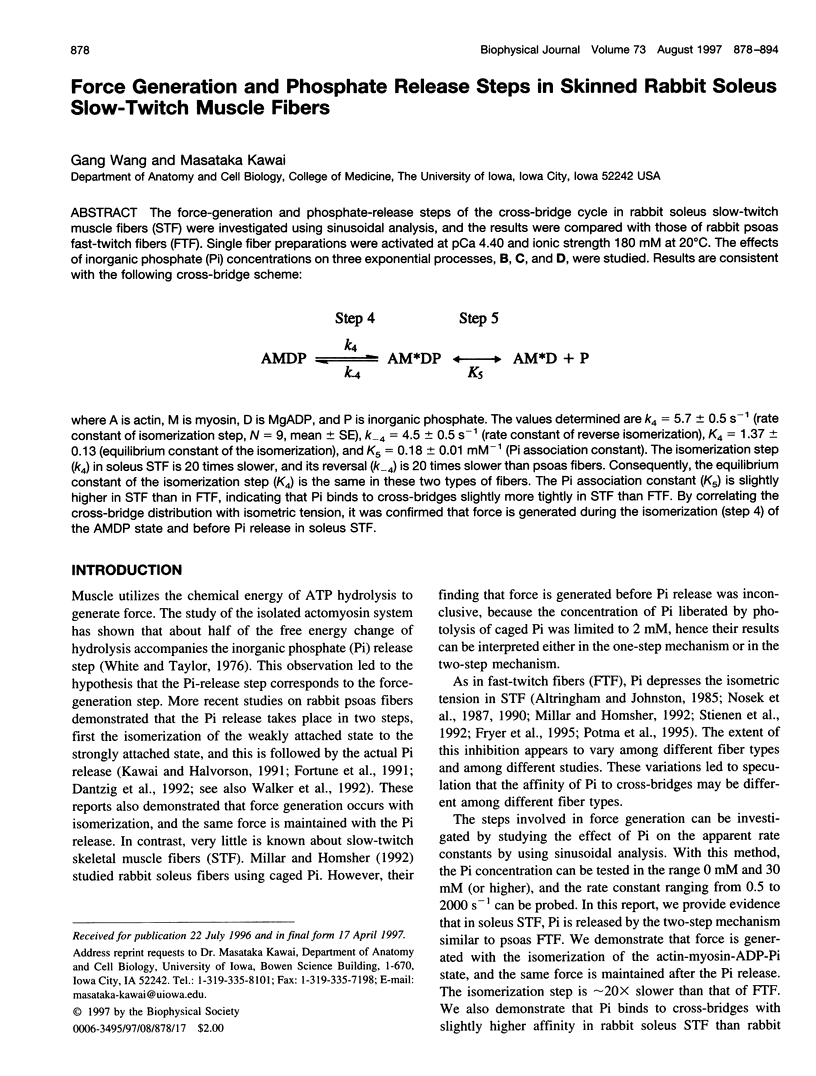
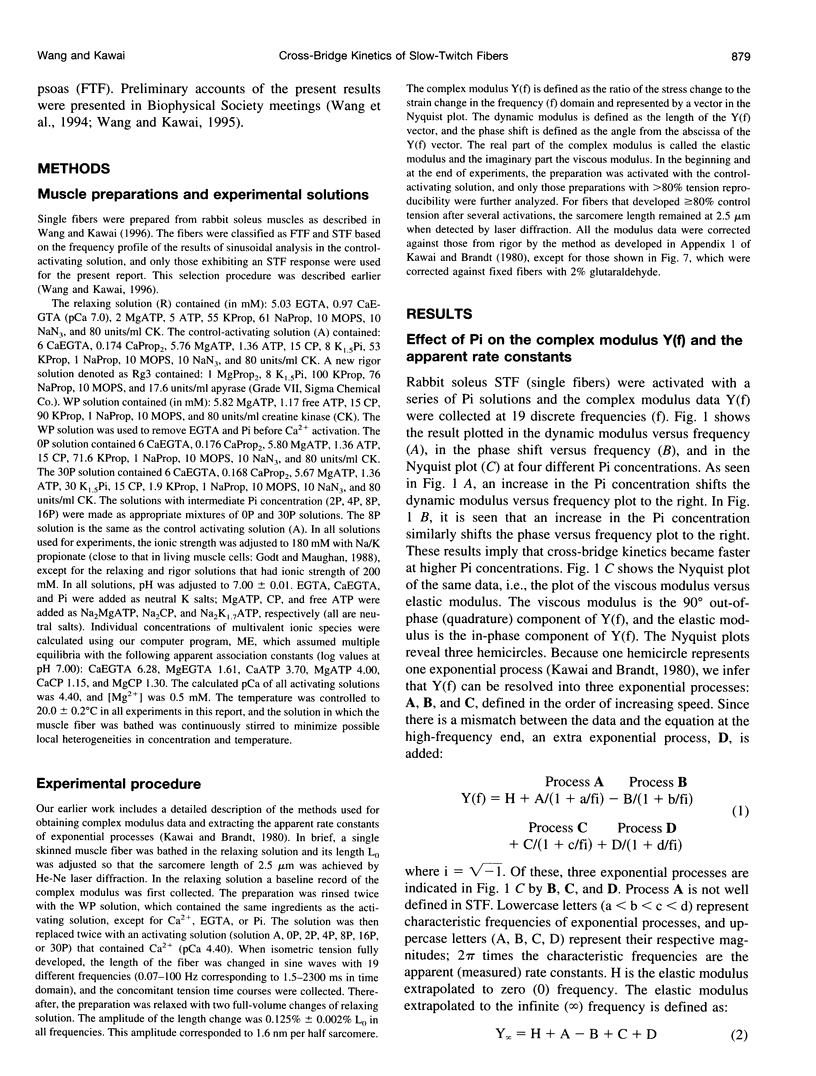
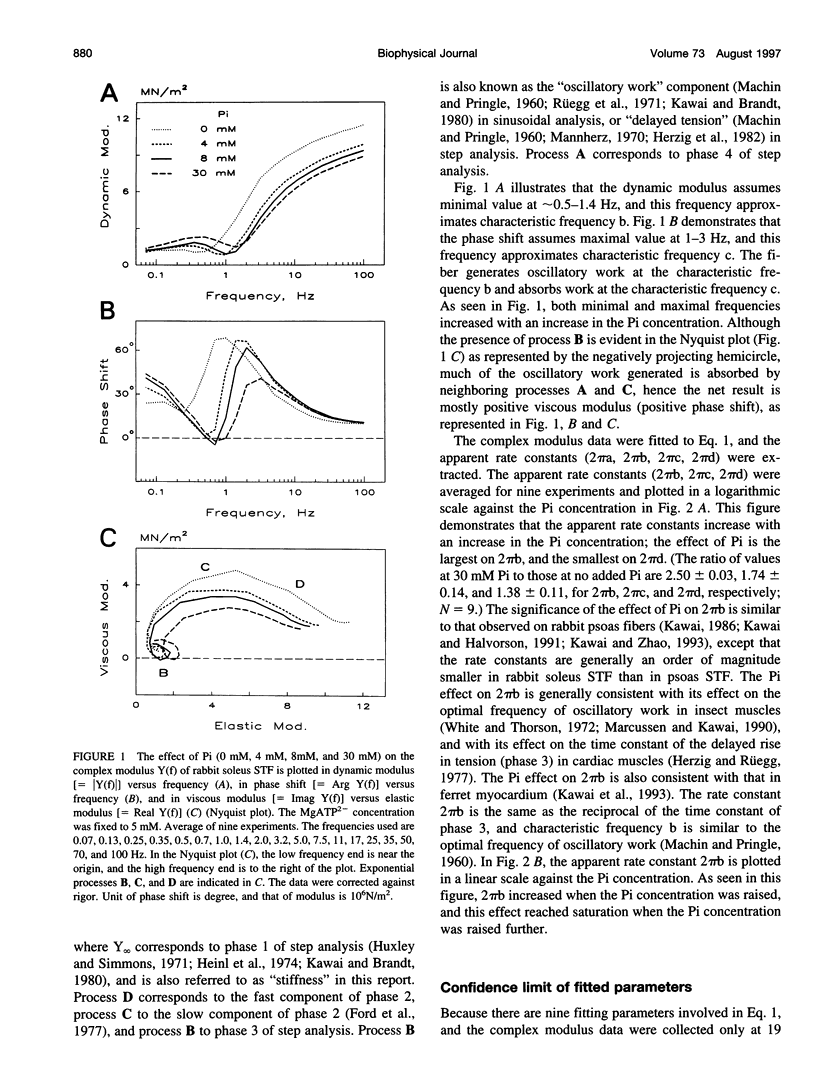
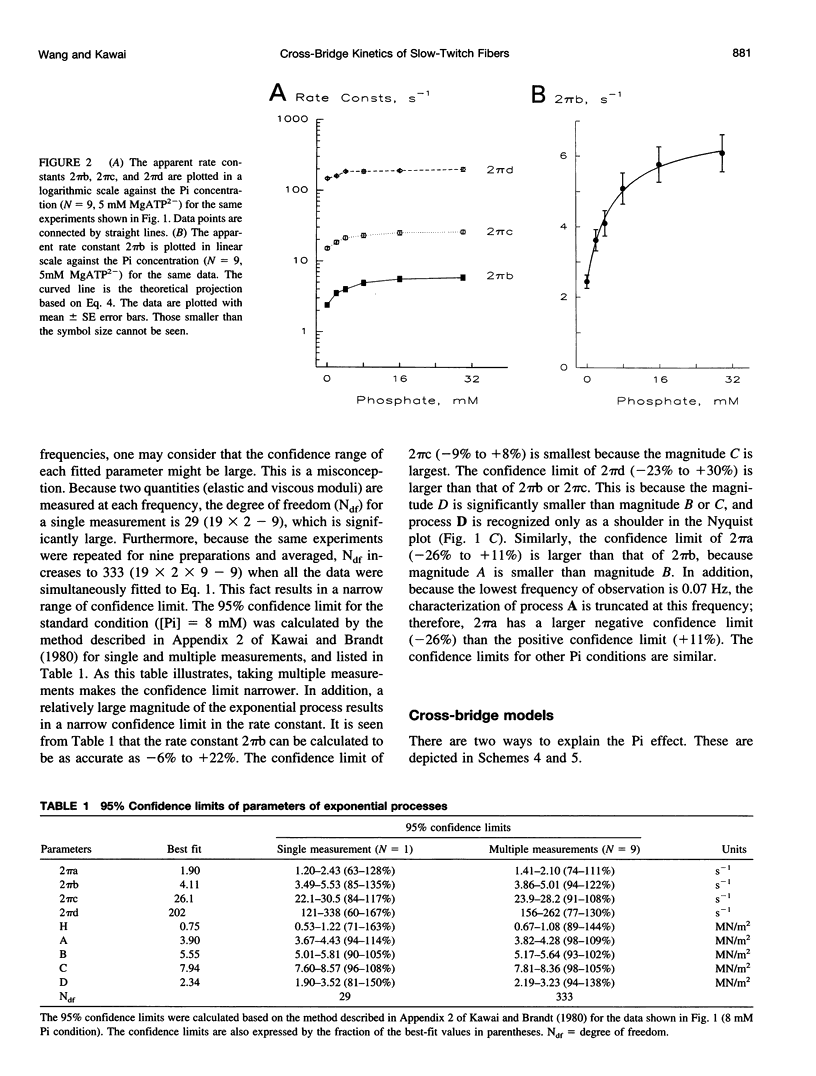
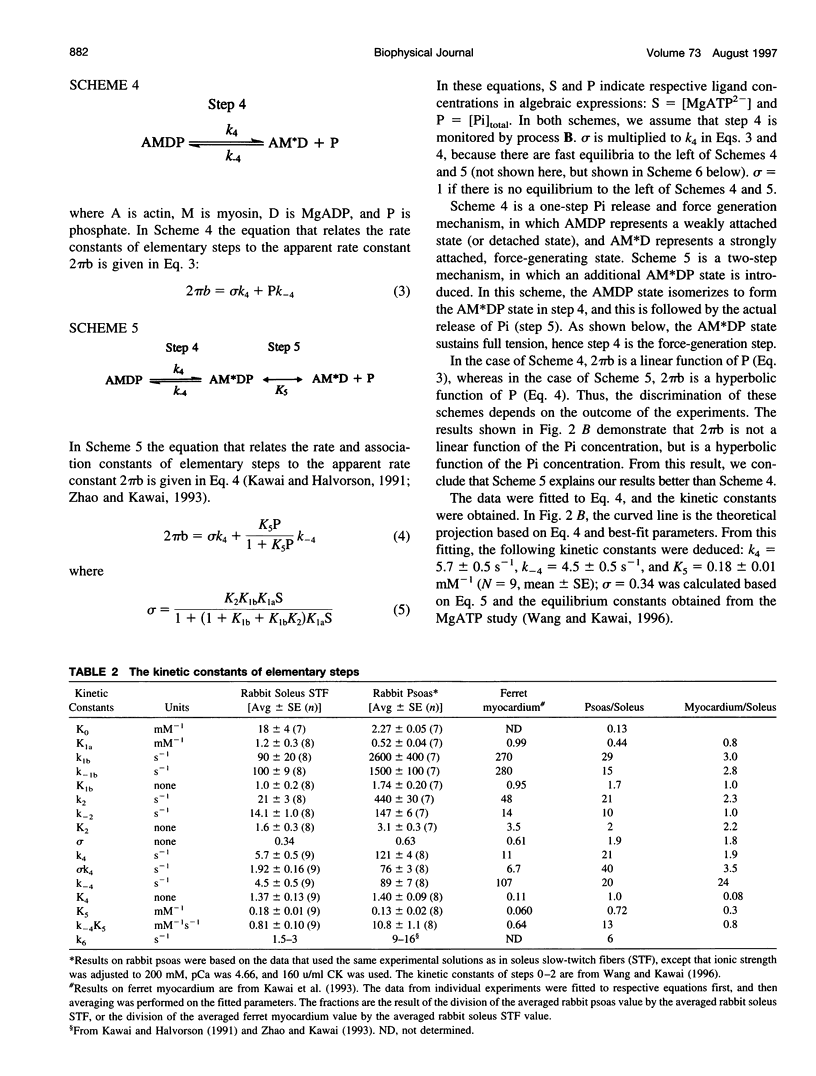
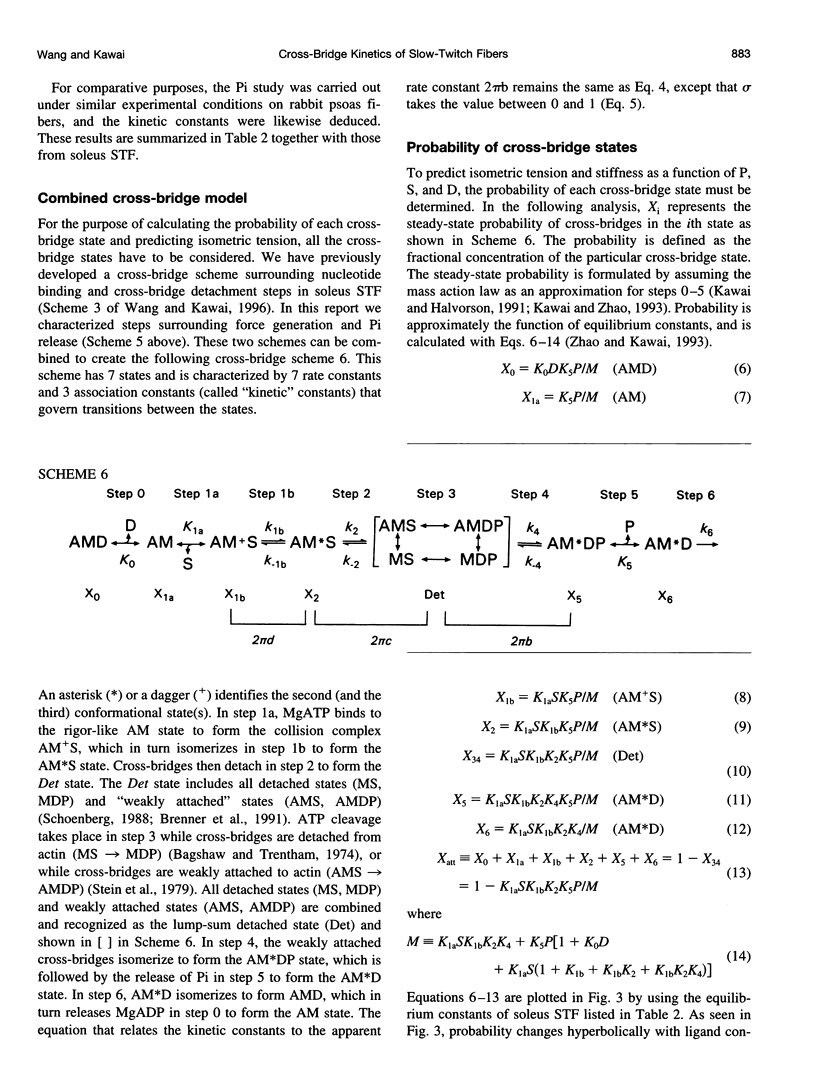
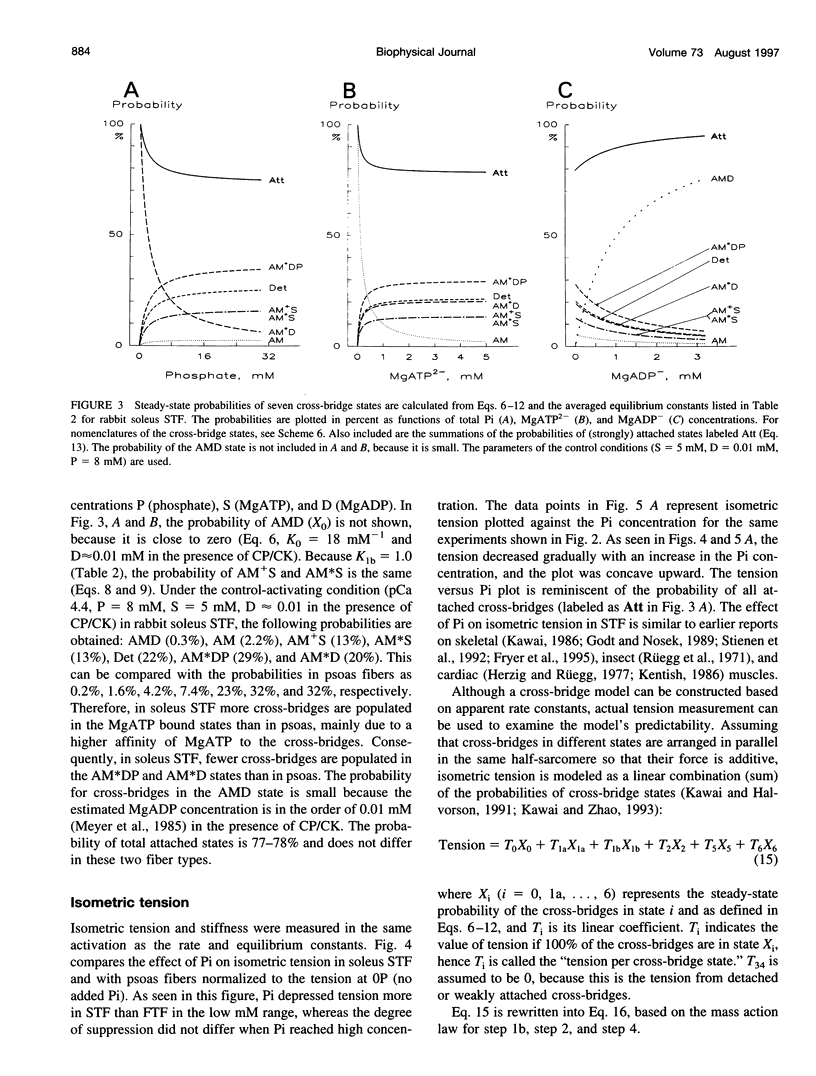
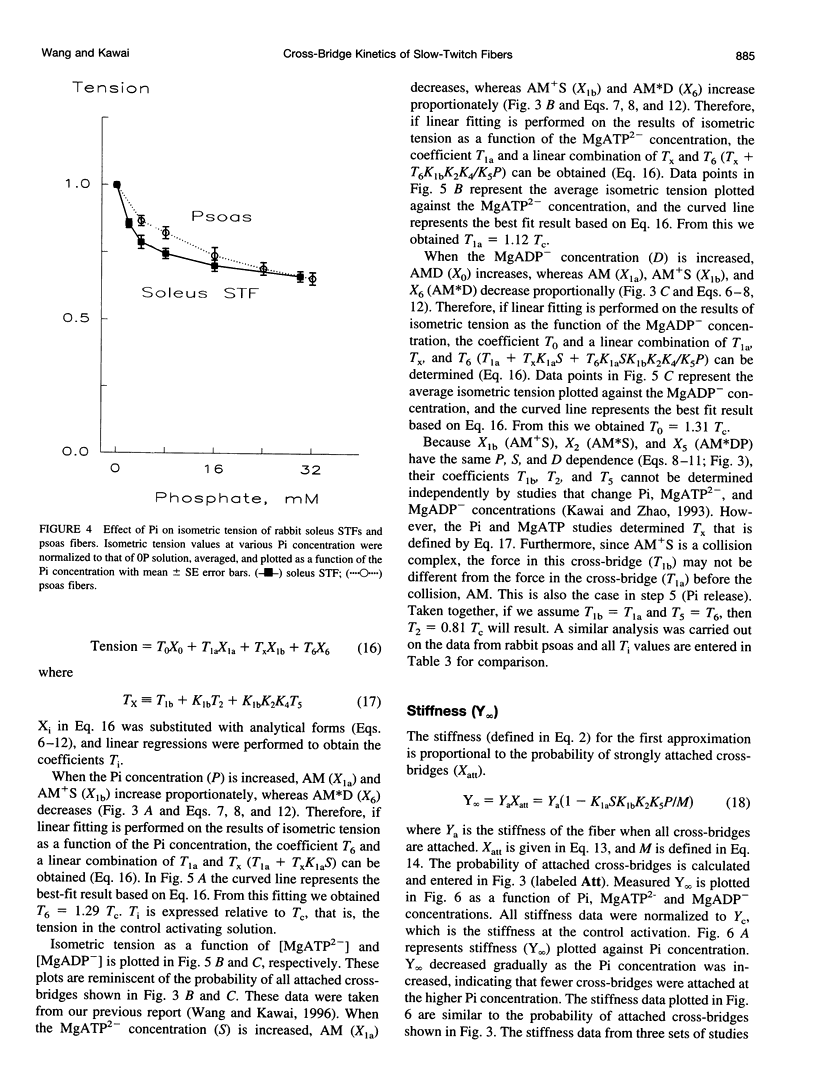
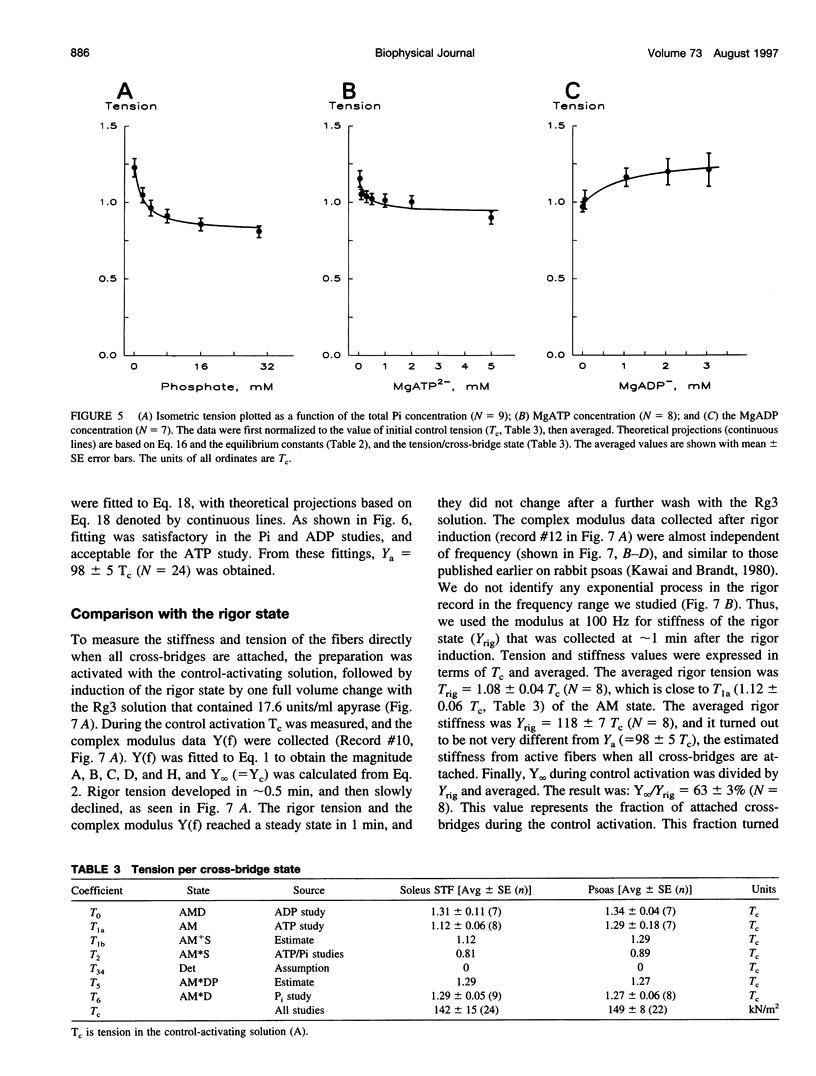
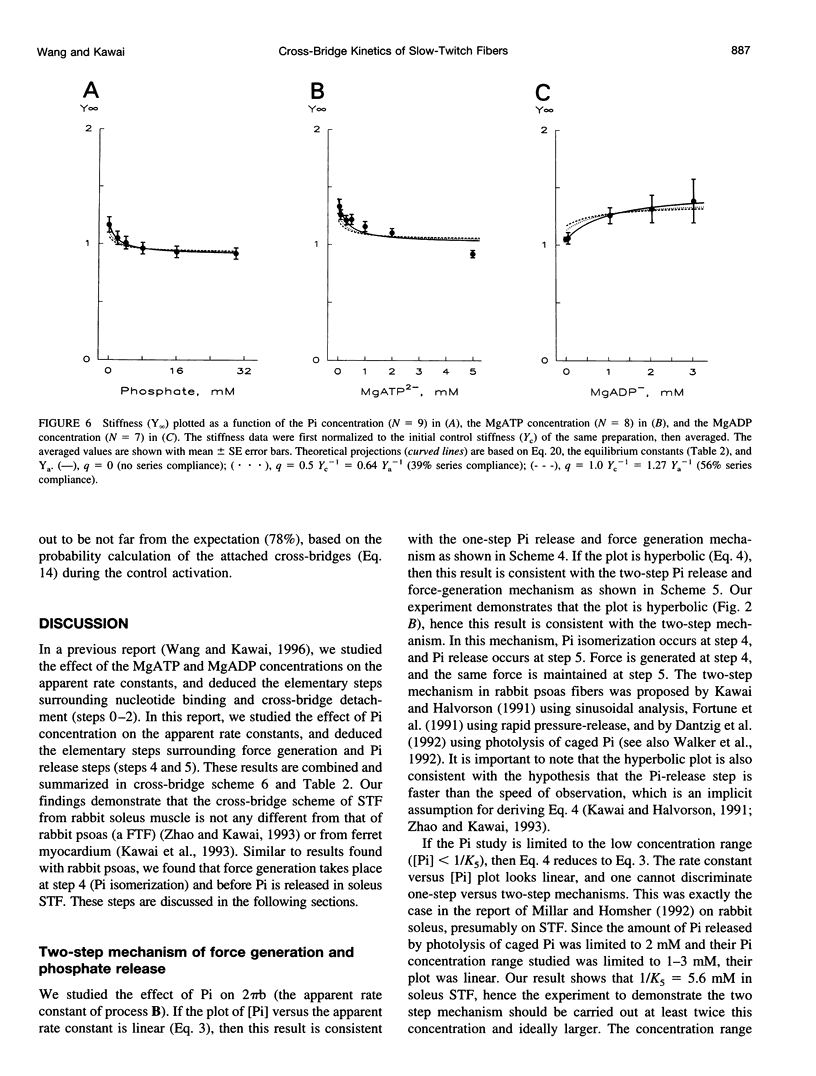
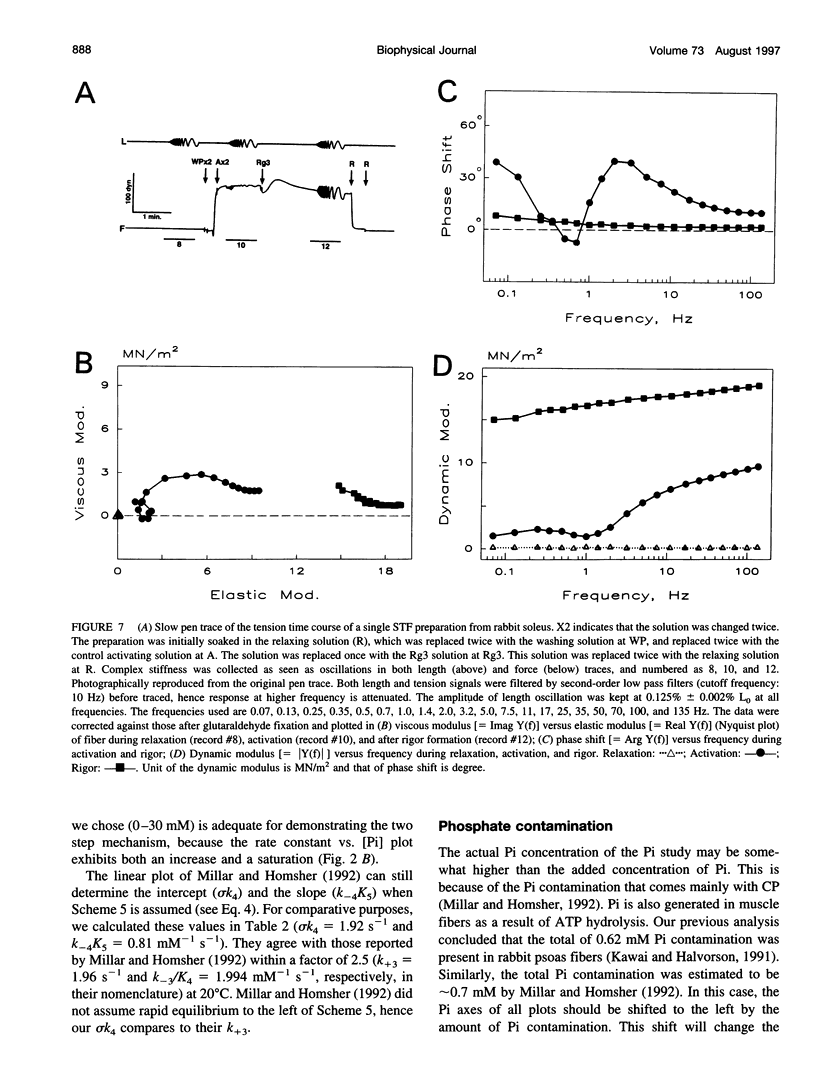
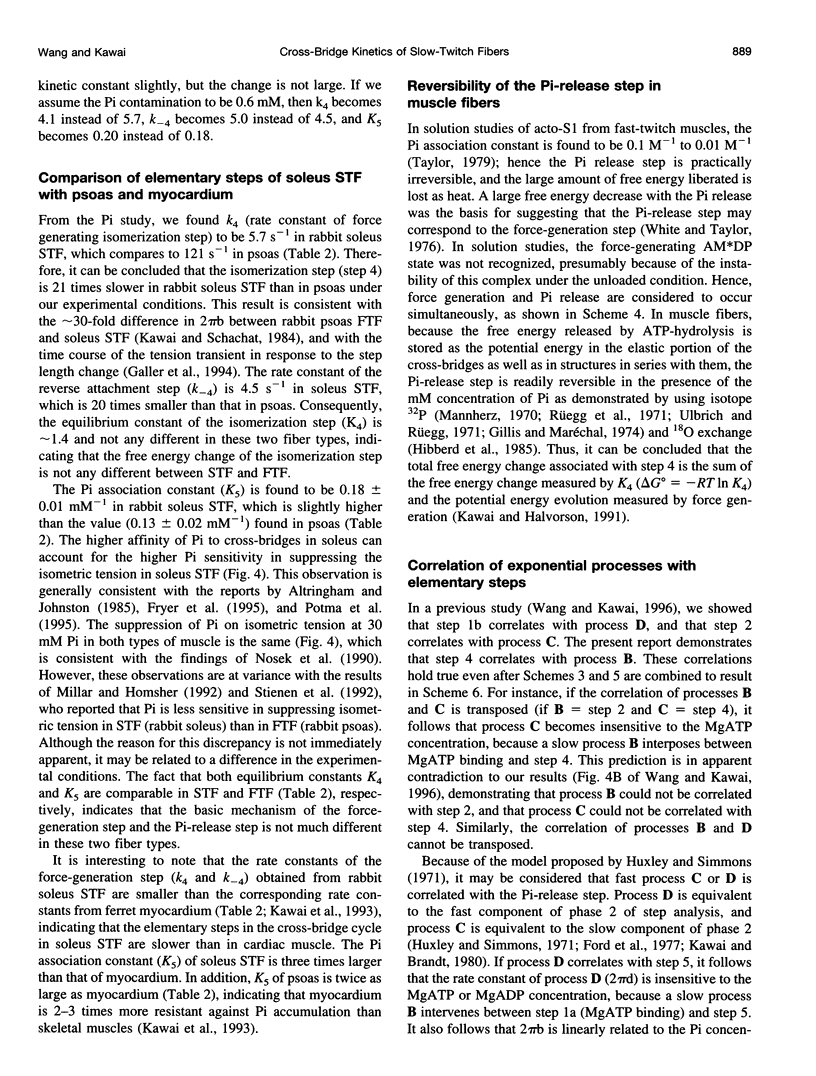
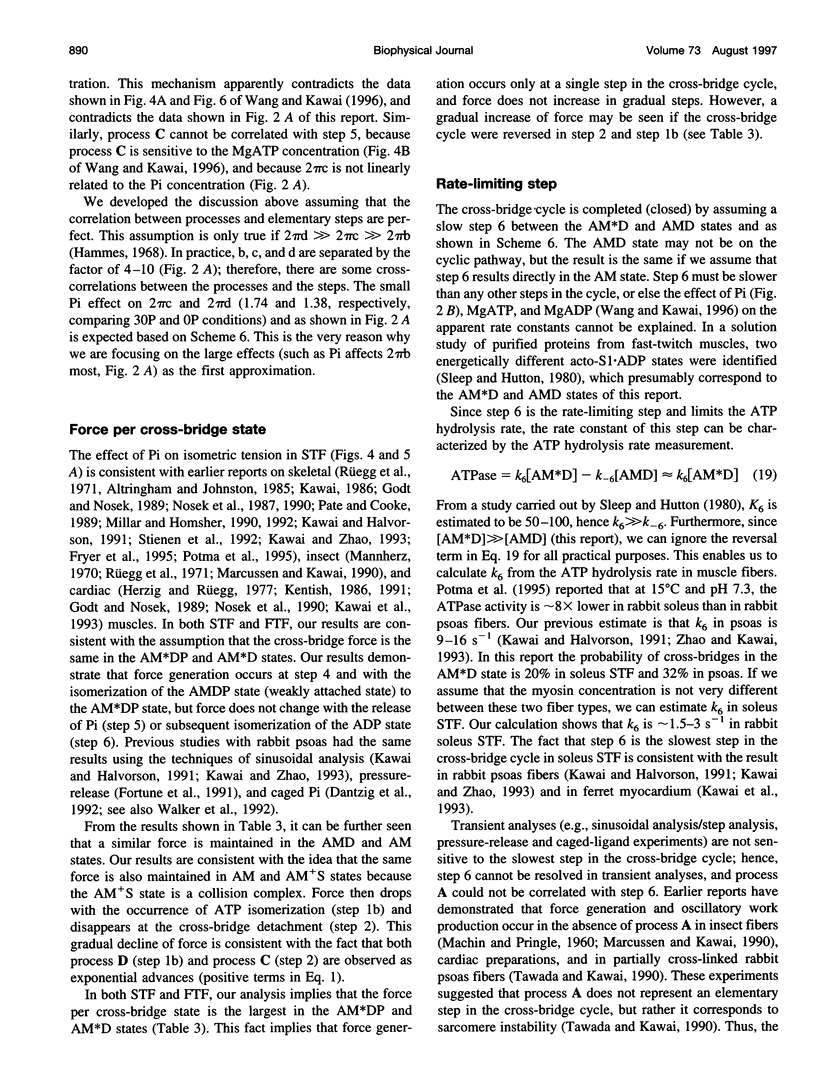
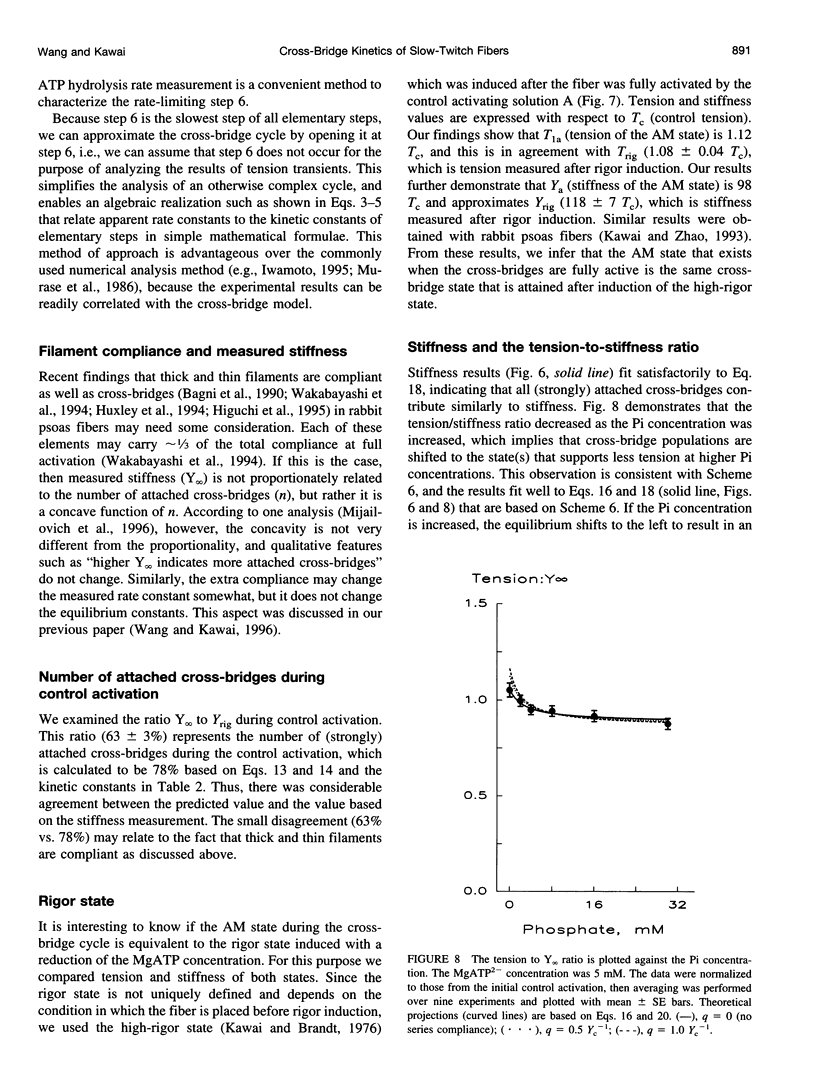
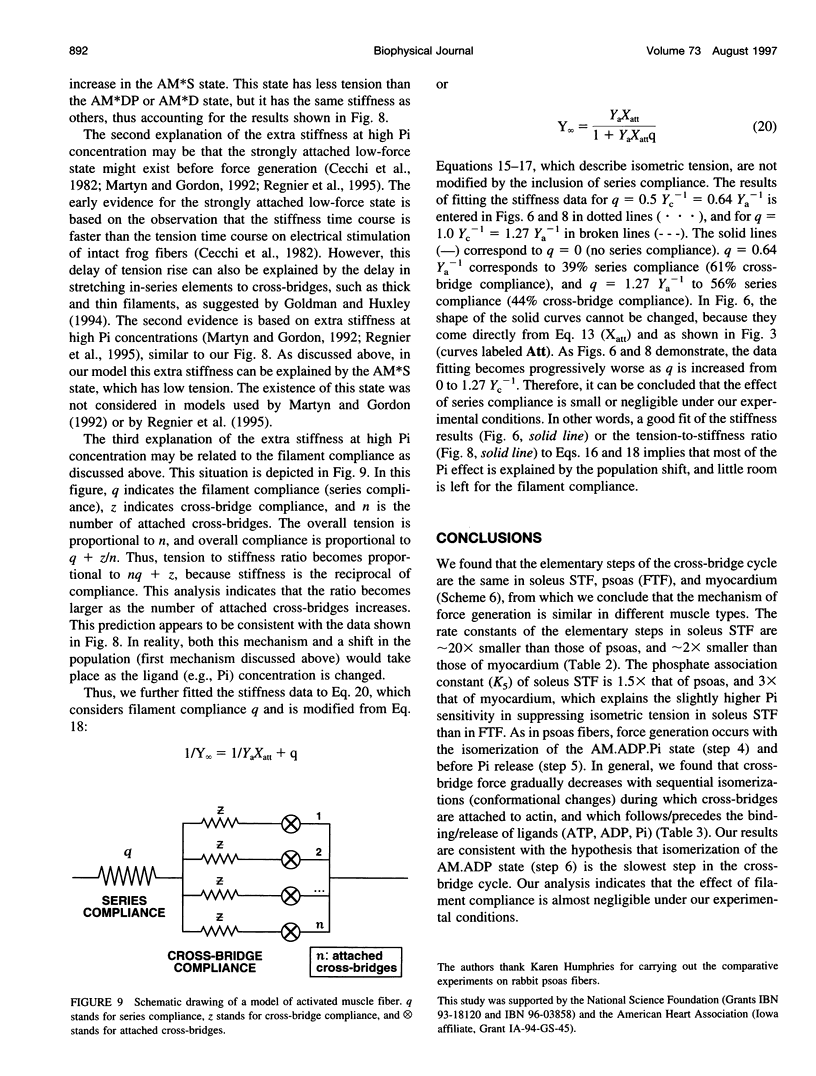
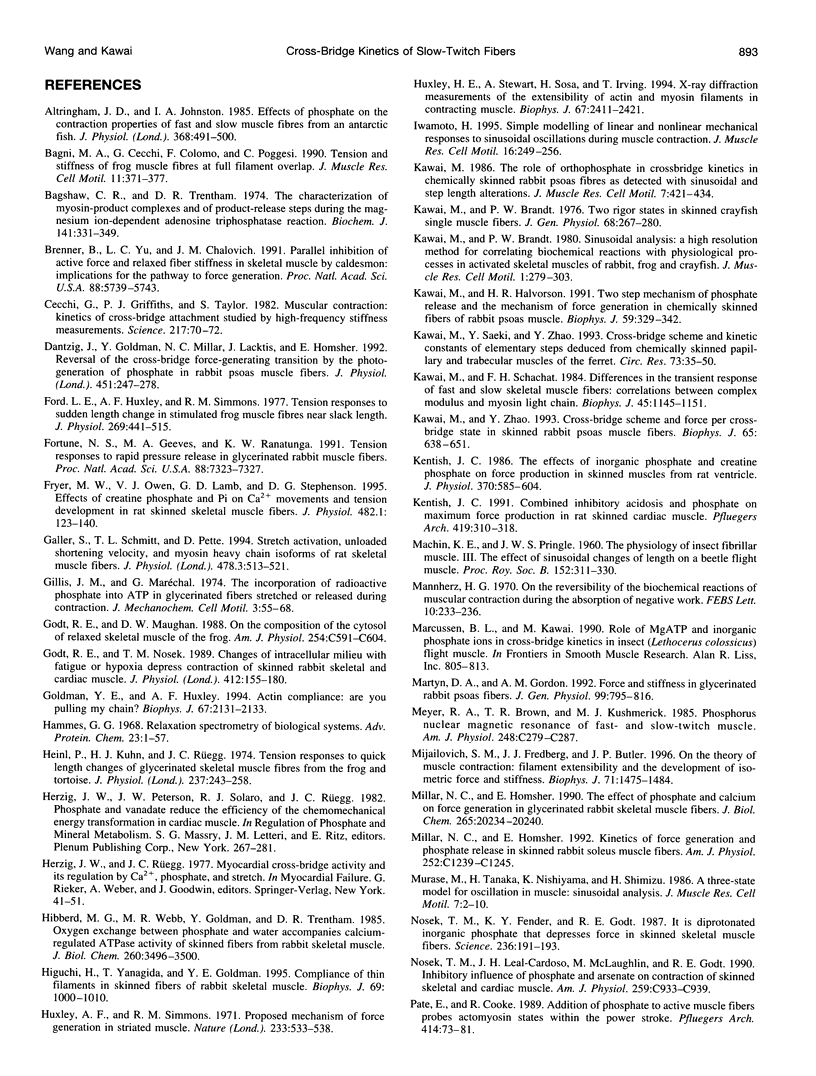
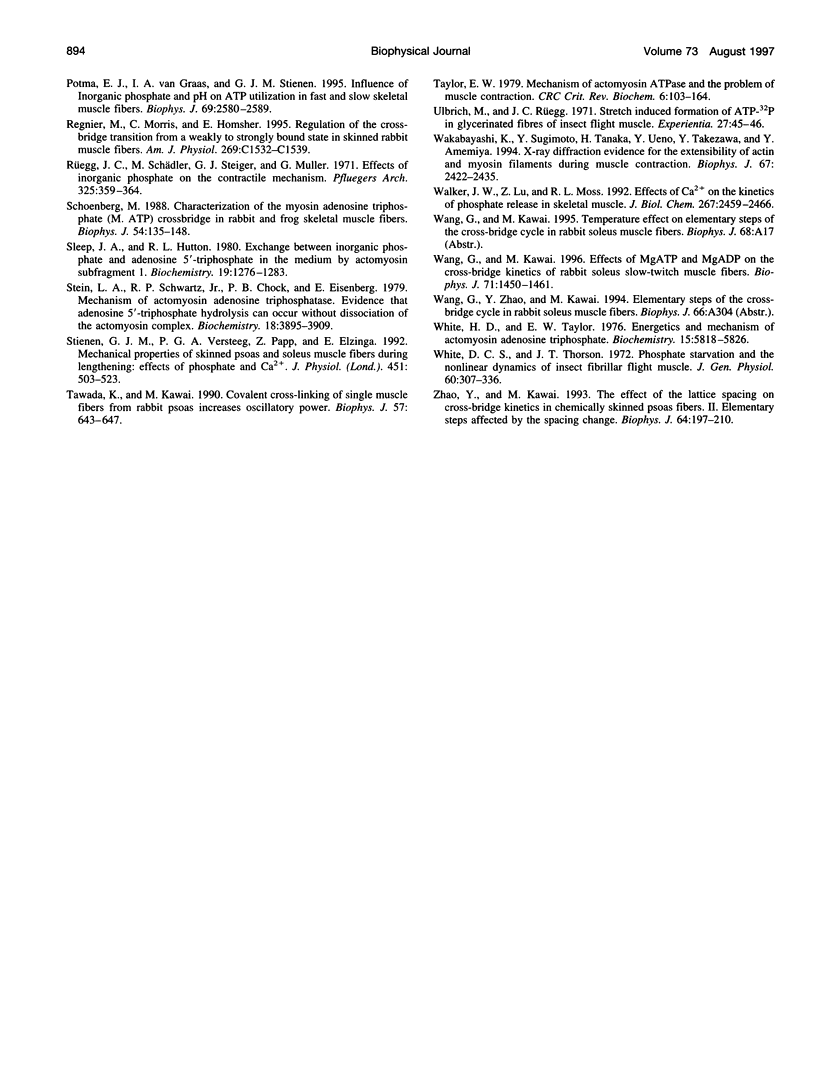
Selected References
These references are in PubMed. This may not be the complete list of references from this article.
- Altringham J. D., Johnston I. A. Effects of phosphate on the contractile properties of fast and slow muscle fibres from an Antarctic fish. J Physiol. 1985 Nov;368:491–500. doi: 10.1113/jphysiol.1985.sp015871. [DOI] [PMC free article] [PubMed] [Google Scholar]
- Bagni M. A., Cecchi G., Colomo F., Poggesi C. Tension and stiffness of frog muscle fibres at full filament overlap. J Muscle Res Cell Motil. 1990 Oct;11(5):371–377. doi: 10.1007/BF01739758. [DOI] [PubMed] [Google Scholar]
- Bagshaw C. R., Trentham D. R. The characterization of myosin-product complexes and of product-release steps during the magnesium ion-dependent adenosine triphosphatase reaction. Biochem J. 1974 Aug;141(2):331–349. doi: 10.1042/bj1410331. [DOI] [PMC free article] [PubMed] [Google Scholar]
- Brenner B., Yu L. C., Chalovich J. M. Parallel inhibition of active force and relaxed fiber stiffness in skeletal muscle by caldesmon: implications for the pathway to force generation. Proc Natl Acad Sci U S A. 1991 Jul 1;88(13):5739–5743. doi: 10.1073/pnas.88.13.5739. [DOI] [PMC free article] [PubMed] [Google Scholar]
- Cecchi G., Griffiths P. J., Taylor S. Muscular contraction: kinetics of crossbridge attachment studied by high-frequency stiffness measurements. Science. 1982 Jul 2;217(4554):70–72. doi: 10.1126/science.6979780. [DOI] [PubMed] [Google Scholar]
- Dantzig J. A., Goldman Y. E., Millar N. C., Lacktis J., Homsher E. Reversal of the cross-bridge force-generating transition by photogeneration of phosphate in rabbit psoas muscle fibres. J Physiol. 1992;451:247–278. doi: 10.1113/jphysiol.1992.sp019163. [DOI] [PMC free article] [PubMed] [Google Scholar]
- Ford L. E., Huxley A. F., Simmons R. M. Tension responses to sudden length change in stimulated frog muscle fibres near slack length. J Physiol. 1977 Jul;269(2):441–515. doi: 10.1113/jphysiol.1977.sp011911. [DOI] [PMC free article] [PubMed] [Google Scholar]
- Fortune N. S., Geeves M. A., Ranatunga K. W. Tension responses to rapid pressure release in glycerinated rabbit muscle fibers. Proc Natl Acad Sci U S A. 1991 Aug 15;88(16):7323–7327. doi: 10.1073/pnas.88.16.7323. [DOI] [PMC free article] [PubMed] [Google Scholar]
- Fryer M. W., Owen V. J., Lamb G. D., Stephenson D. G. Effects of creatine phosphate and P(i) on Ca2+ movements and tension development in rat skinned skeletal muscle fibres. J Physiol. 1995 Jan 1;482(Pt 1):123–140. doi: 10.1113/jphysiol.1995.sp020504. [DOI] [PMC free article] [PubMed] [Google Scholar]
- Galler S., Schmitt T. L., Pette D. Stretch activation, unloaded shortening velocity, and myosin heavy chain isoforms of rat skeletal muscle fibres. J Physiol. 1994 Aug 1;478(Pt 3):513–521. doi: 10.1113/jphysiol.1994.sp020270. [DOI] [PMC free article] [PubMed] [Google Scholar]
- Gillis J. M., Maréchal G. The incorporation of radioactive phosphate into ATP in glycerinated fibres stretched or released during contraction. J Mechanochem Cell Motil. 1974;3(1):55–68. [PubMed] [Google Scholar]
- Godt R. E., Maughan D. W. On the composition of the cytosol of relaxed skeletal muscle of the frog. Am J Physiol. 1988 May;254(5 Pt 1):C591–C604. doi: 10.1152/ajpcell.1988.254.5.C591. [DOI] [PubMed] [Google Scholar]
- Godt R. E., Nosek T. M. Changes of intracellular milieu with fatigue or hypoxia depress contraction of skinned rabbit skeletal and cardiac muscle. J Physiol. 1989 May;412:155–180. doi: 10.1113/jphysiol.1989.sp017609. [DOI] [PMC free article] [PubMed] [Google Scholar]
- Goldman Y. E., Huxley A. F. Actin compliance: are you pulling my chain? Biophys J. 1994 Dec;67(6):2131–2133. doi: 10.1016/S0006-3495(94)80700-3. [DOI] [PMC free article] [PubMed] [Google Scholar]
- Hammes G. G. Relaxation spectrometry of biological systems. Adv Protein Chem. 1968;23:1–57. doi: 10.1016/s0065-3233(08)60399-x. [DOI] [PubMed] [Google Scholar]
- Heinl P., Kuhn H. J., Rüegg J. C. Tension responses to quick length changes of glycerinated skeletal muscle fibres from the frog and tortoise. J Physiol. 1974 Mar;237(2):243–258. doi: 10.1113/jphysiol.1974.sp010480. [DOI] [PMC free article] [PubMed] [Google Scholar]
- Herzig J. W., Peterson J. W., Solaro R. J., Rüegg J. C. Phosphate and vanadate reduce the efficiency of the chemo-mechanical energy transformation in cardiac muscle. Adv Exp Med Biol. 1982;151:267–281. doi: 10.1007/978-1-4684-4259-5_33. [DOI] [PubMed] [Google Scholar]
- Hibberd M. G., Webb M. R., Goldman Y. E., Trentham D. R. Oxygen exchange between phosphate and water accompanies calcium-regulated ATPase activity of skinned fibers from rabbit skeletal muscle. J Biol Chem. 1985 Mar 25;260(6):3496–3500. [PubMed] [Google Scholar]
- Higuchi H., Yanagida T., Goldman Y. E. Compliance of thin filaments in skinned fibers of rabbit skeletal muscle. Biophys J. 1995 Sep;69(3):1000–1010. doi: 10.1016/S0006-3495(95)79975-1. [DOI] [PMC free article] [PubMed] [Google Scholar]
- Huxley A. F., Simmons R. M. Proposed mechanism of force generation in striated muscle. Nature. 1971 Oct 22;233(5321):533–538. doi: 10.1038/233533a0. [DOI] [PubMed] [Google Scholar]
- Huxley H. E., Stewart A., Sosa H., Irving T. X-ray diffraction measurements of the extensibility of actin and myosin filaments in contracting muscle. Biophys J. 1994 Dec;67(6):2411–2421. doi: 10.1016/S0006-3495(94)80728-3. [DOI] [PMC free article] [PubMed] [Google Scholar]
- Iwamoto H. Simple modelling of linear and nonlinear mechanical responses to sinusoidal oscillations during muscle contraction. J Muscle Res Cell Motil. 1995 Jun;16(3):249–256. doi: 10.1007/BF00121134. [DOI] [PubMed] [Google Scholar]
- Kawai M., Brandt P. W. Sinusoidal analysis: a high resolution method for correlating biochemical reactions with physiological processes in activated skeletal muscles of rabbit, frog and crayfish. J Muscle Res Cell Motil. 1980 Sep;1(3):279–303. doi: 10.1007/BF00711932. [DOI] [PubMed] [Google Scholar]
- Kawai M., Brandt P. W. Two rigor states in skinned crayfish single muscle fibers. J Gen Physiol. 1976 Sep;68(3):267–280. doi: 10.1085/jgp.68.3.267. [DOI] [PMC free article] [PubMed] [Google Scholar]
- Kawai M., Halvorson H. R. Two step mechanism of phosphate release and the mechanism of force generation in chemically skinned fibers of rabbit psoas muscle. Biophys J. 1991 Feb;59(2):329–342. doi: 10.1016/S0006-3495(91)82227-5. [DOI] [PMC free article] [PubMed] [Google Scholar]
- Kawai M., Saeki Y., Zhao Y. Crossbridge scheme and the kinetic constants of elementary steps deduced from chemically skinned papillary and trabecular muscles of the ferret. Circ Res. 1993 Jul;73(1):35–50. doi: 10.1161/01.res.73.1.35. [DOI] [PubMed] [Google Scholar]
- Kawai M., Schachat F. H. Differences in the transient response of fast and slow skeletal muscle fibers. Correlations between complex modulus and myosin light chains. Biophys J. 1984 Jun;45(6):1145–1151. doi: 10.1016/S0006-3495(84)84262-9. [DOI] [PMC free article] [PubMed] [Google Scholar]
- Kawai M. The role of orthophosphate in crossbridge kinetics in chemically skinned rabbit psoas fibres as detected with sinusoidal and step length alterations. J Muscle Res Cell Motil. 1986 Oct;7(5):421–434. doi: 10.1007/BF01753585. [DOI] [PubMed] [Google Scholar]
- Kawai M., Zhao Y. Cross-bridge scheme and force per cross-bridge state in skinned rabbit psoas muscle fibers. Biophys J. 1993 Aug;65(2):638–651. doi: 10.1016/S0006-3495(93)81109-3. [DOI] [PMC free article] [PubMed] [Google Scholar]
- Kentish J. C. Combined inhibitory actions of acidosis and phosphate on maximum force production in rat skinned cardiac muscle. Pflugers Arch. 1991 Oct;419(3-4):310–318. doi: 10.1007/BF00371112. [DOI] [PubMed] [Google Scholar]
- Kentish J. C. The effects of inorganic phosphate and creatine phosphate on force production in skinned muscles from rat ventricle. J Physiol. 1986 Jan;370:585–604. doi: 10.1113/jphysiol.1986.sp015952. [DOI] [PMC free article] [PubMed] [Google Scholar]
- MACHIN K. E., PRINGLE J. W. The physiology of insect fibrillar muscle. III. The effect of sinusoidal changes of length on a beetle flight muscle. Proc R Soc Lond B Biol Sci. 1960 Jun 14;152:311–330. doi: 10.1098/rspb.1960.0041. [DOI] [PubMed] [Google Scholar]
- Mannherz Hans G. On the reversibility of the biochemical reactions of muscular contraction during the absorption of negative work. FEBS Lett. 1970 Oct 16;10(4):233–236. doi: 10.1016/0014-5793(70)80636-6. [DOI] [PubMed] [Google Scholar]
- Marcussen B. L., Kawai M. Role of MgATP and inorganic phosphate ions in cross-bridge kinetics in insect (Lethocerus colossicus) flight muscle. Prog Clin Biol Res. 1990;327:805–813. [PubMed] [Google Scholar]
- Martyn D. A., Gordon A. M. Force and stiffness in glycerinated rabbit psoas fibers. Effects of calcium and elevated phosphate. J Gen Physiol. 1992 May;99(5):795–816. doi: 10.1085/jgp.99.5.795. [DOI] [PMC free article] [PubMed] [Google Scholar]
- Meyer R. A., Brown T. R., Kushmerick M. J. Phosphorus nuclear magnetic resonance of fast- and slow-twitch muscle. Am J Physiol. 1985 Mar;248(3 Pt 1):C279–C287. doi: 10.1152/ajpcell.1985.248.3.C279. [DOI] [PubMed] [Google Scholar]
- Mijailovich S. M., Fredberg J. J., Butler J. P. On the theory of muscle contraction: filament extensibility and the development of isometric force and stiffness. Biophys J. 1996 Sep;71(3):1475–1484. doi: 10.1016/S0006-3495(96)79348-7. [DOI] [PMC free article] [PubMed] [Google Scholar]
- Millar N. C., Homsher E. Kinetics of force generation and phosphate release in skinned rabbit soleus muscle fibers. Am J Physiol. 1992 May;262(5 Pt 1):C1239–C1245. doi: 10.1152/ajpcell.1992.262.5.C1239. [DOI] [PubMed] [Google Scholar]
- Millar N. C., Homsher E. The effect of phosphate and calcium on force generation in glycerinated rabbit skeletal muscle fibers. A steady-state and transient kinetic study. J Biol Chem. 1990 Nov 25;265(33):20234–20240. [PubMed] [Google Scholar]
- Murase M., Tanaka H., Nishiyama K., Shimizu H. A three-state model for oscillation in muscle: sinusoidal analysis. J Muscle Res Cell Motil. 1986 Feb;7(1):2–10. doi: 10.1007/BF01756196. [DOI] [PubMed] [Google Scholar]
- Nosek T. M., Fender K. Y., Godt R. E. It is diprotonated inorganic phosphate that depresses force in skinned skeletal muscle fibers. Science. 1987 Apr 10;236(4798):191–193. doi: 10.1126/science.3563496. [DOI] [PubMed] [Google Scholar]
- Nosek T. M., Leal-Cardoso J. H., McLaughlin M., Godt R. E. Inhibitory influence of phosphate and arsenate on contraction of skinned skeletal and cardiac muscle. Am J Physiol. 1990 Dec;259(6 Pt 1):C933–C939. doi: 10.1152/ajpcell.1990.259.6.C933. [DOI] [PubMed] [Google Scholar]
- Pate E., Cooke R. Addition of phosphate to active muscle fibers probes actomyosin states within the powerstroke. Pflugers Arch. 1989 May;414(1):73–81. doi: 10.1007/BF00585629. [DOI] [PubMed] [Google Scholar]
- Potma E. J., van Graas I. A., Stienen G. J. Influence of inorganic phosphate and pH on ATP utilization in fast and slow skeletal muscle fibers. Biophys J. 1995 Dec;69(6):2580–2589. doi: 10.1016/S0006-3495(95)80129-3. [DOI] [PMC free article] [PubMed] [Google Scholar]
- Regnier M., Morris C., Homsher E. Regulation of the cross-bridge transition from a weakly to strongly bound state in skinned rabbit muscle fibers. Am J Physiol. 1995 Dec;269(6 Pt 1):C1532–C1539. doi: 10.1152/ajpcell.1995.269.6.C1532. [DOI] [PubMed] [Google Scholar]
- Rüegg J. C., Schädler M., Steiger G. J., Müller G. Effects of inorganic phosphate on the contractile mechanism. Pflugers Arch. 1971;325(4):359–364. doi: 10.1007/BF00592176. [DOI] [PubMed] [Google Scholar]
- Schoenberg M. Characterization of the myosin adenosine triphosphate (M.ATP) crossbridge in rabbit and frog skeletal muscle fibers. Biophys J. 1988 Jul;54(1):135–148. doi: 10.1016/S0006-3495(88)82938-2. [DOI] [PMC free article] [PubMed] [Google Scholar]
- Sleep J. A., Hutton R. L. Exchange between inorganic phosphate and adenosine 5'-triphosphate in the medium by actomyosin subfragment 1. Biochemistry. 1980 Apr 1;19(7):1276–1283. doi: 10.1021/bi00548a002. [DOI] [PubMed] [Google Scholar]
- Stein L. A., Schwarz R. P., Jr, Chock P. B., Eisenberg E. Mechanism of actomyosin adenosine triphosphatase. Evidence that adenosine 5'-triphosphate hydrolysis can occur without dissociation of the actomyosin complex. Biochemistry. 1979 Sep 4;18(18):3895–3909. doi: 10.1021/bi00585a009. [DOI] [PubMed] [Google Scholar]
- Stienen G. J., Versteeg P. G., Papp Z., Elzinga G. Mechanical properties of skinned rabbit psoas and soleus muscle fibres during lengthening: effects of phosphate and Ca2+. J Physiol. 1992;451:503–523. doi: 10.1113/jphysiol.1992.sp019176. [DOI] [PMC free article] [PubMed] [Google Scholar]
- Tawada K., Kawai M. Covalent cross-linking of single fibers from rabbit psoas increases oscillatory power. Biophys J. 1990 Mar;57(3):643–647. doi: 10.1016/S0006-3495(90)82582-0. [DOI] [PMC free article] [PubMed] [Google Scholar]
- Taylor E. W. Mechanism of actomyosin ATPase and the problem of muscle contraction. CRC Crit Rev Biochem. 1979;6(2):103–164. doi: 10.3109/10409237909102562. [DOI] [PubMed] [Google Scholar]
- Ulbrich M., Rüegg J. C. Stretch induced formation of ATP-32P in glycerinated fibres of insect flight muscle. Experientia. 1971 Jan 15;27(1):45–46. doi: 10.1007/BF02137732. [DOI] [PubMed] [Google Scholar]
- Wakabayashi K., Sugimoto Y., Tanaka H., Ueno Y., Takezawa Y., Amemiya Y. X-ray diffraction evidence for the extensibility of actin and myosin filaments during muscle contraction. Biophys J. 1994 Dec;67(6):2422–2435. doi: 10.1016/S0006-3495(94)80729-5. [DOI] [PMC free article] [PubMed] [Google Scholar]
- Walker J. W., Lu Z., Moss R. L. Effects of Ca2+ on the kinetics of phosphate release in skeletal muscle. J Biol Chem. 1992 Feb 5;267(4):2459–2466. [PubMed] [Google Scholar]
- Wang G., Kawai M. Effects of MgATP and MgADP on the cross-bridge kinetics of rabbit soleus slow-twitch muscle fibers. Biophys J. 1996 Sep;71(3):1450–1461. doi: 10.1016/S0006-3495(96)79346-3. [DOI] [PMC free article] [PubMed] [Google Scholar]
- White D. C., Thorson J. Phosphate starvation and the nonlinear dynamics of insect fibrillar flight muscle. J Gen Physiol. 1972 Sep;60(3):307–336. doi: 10.1085/jgp.60.3.307. [DOI] [PMC free article] [PubMed] [Google Scholar]
- White H. D., Taylor E. W. Energetics and mechanism of actomyosin adenosine triphosphatase. Biochemistry. 1976 Dec 28;15(26):5818–5826. doi: 10.1021/bi00671a020. [DOI] [PubMed] [Google Scholar]
- Zhao Y., Kawai M. The effect of the lattice spacing change on cross-bridge kinetics in chemically skinned rabbit psoas muscle fibers. II. Elementary steps affected by the spacing change. Biophys J. 1993 Jan;64(1):197–210. doi: 10.1016/S0006-3495(93)81357-2. [DOI] [PMC free article] [PubMed] [Google Scholar]