Abstract
The high stiffness of relaxed cardiac myofibrils is explainable mainly by the expression of a short-length titin (connectin), the giant elastic protein of the vertebrate myofibrillar cytoskeleton. However, additional molecular features could account for this high stiffness, such as interaction between titin and actin, which has previously been reported in vitro. To probe this finding for a possible physiological significance, isolated myofibrils from rat heart were subjected to selective removal of actin filaments by a calcium-independent gelsolin fragment, and the "passive" stiffness of the specimens was recorded. Upon actin extraction, stiffness decreased by nearly 60%, and to a similar degree after high-salt extraction of thick filaments. Thus actin-titin association indeed contributes to the stiffness of resting cardiac muscle. To identify possible sites of association, we employed a combination of different techniques. Immunofluorescence microscopy revealed that actin extraction increased the extensibility of the previously stiff Z-disc-flanking titin region. Actin-titin interaction within this region was confirmed in in vitro cosedimentation assays, in which multimodule recombinant titin fragments were tested for their ability to interact with F-actin. By contrast, such assays showed no actin-titin-binding propensity for sarcomeric regions outside the Z-disc comb. Accordingly, the results of mechanical measurements demonstrated that competition with native titin by recombinant titin fragments from Z-disc-remote, I-band or A-band regions did not affect passive myofibril stiffness. These results indicate that it is actin-titin association near the Z-disc, but not along the remainder of the sarcomere, that helps to anchor the titin molecule at its N-terminus and maintain a high stiffness of the relaxed cardiac myofibril.
Full text
PDF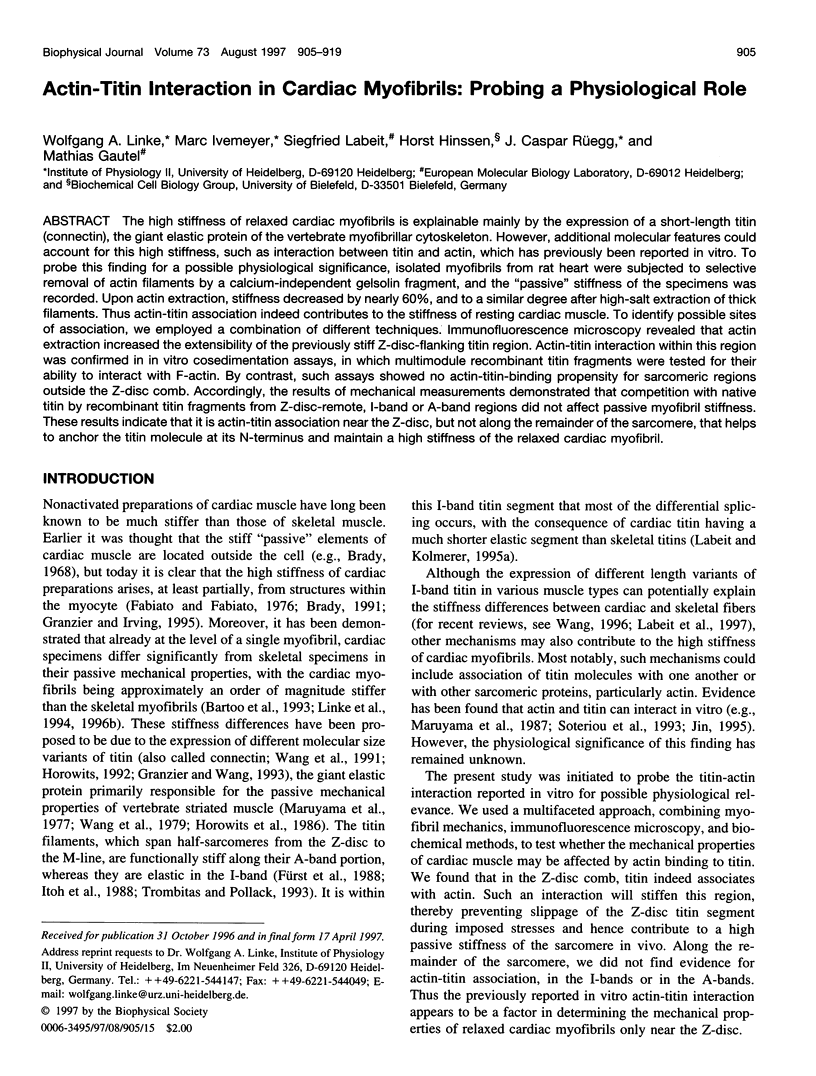
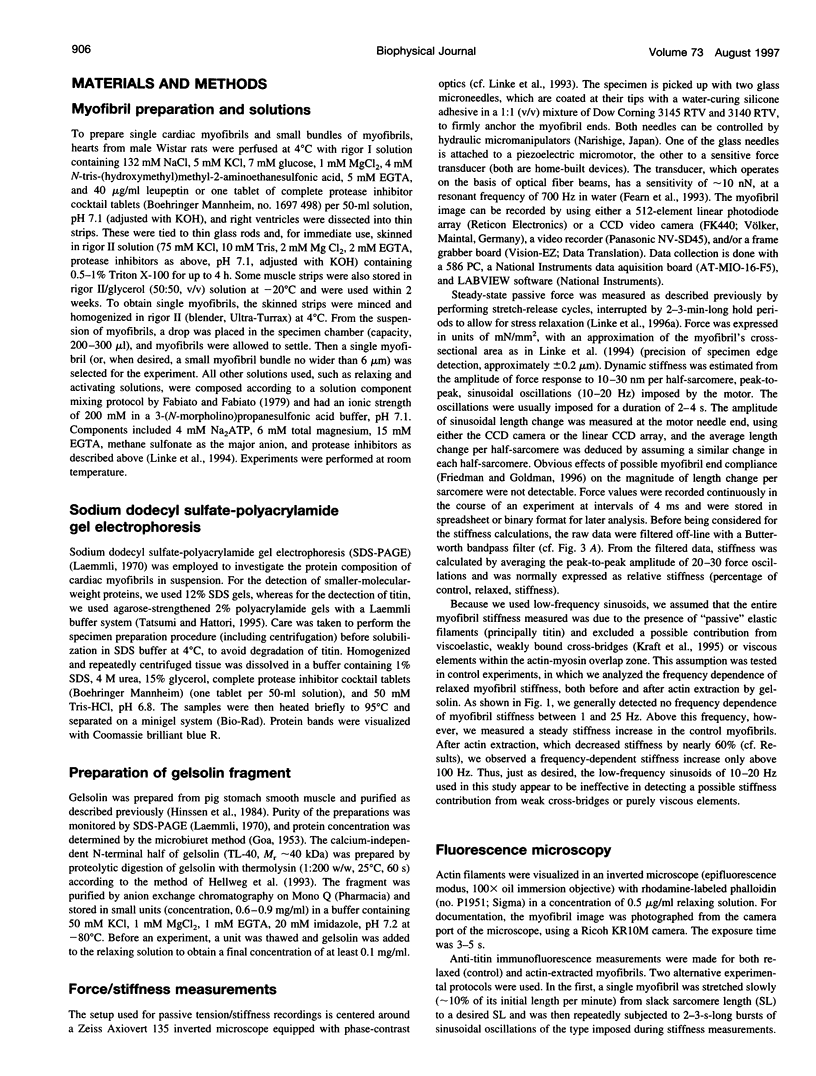
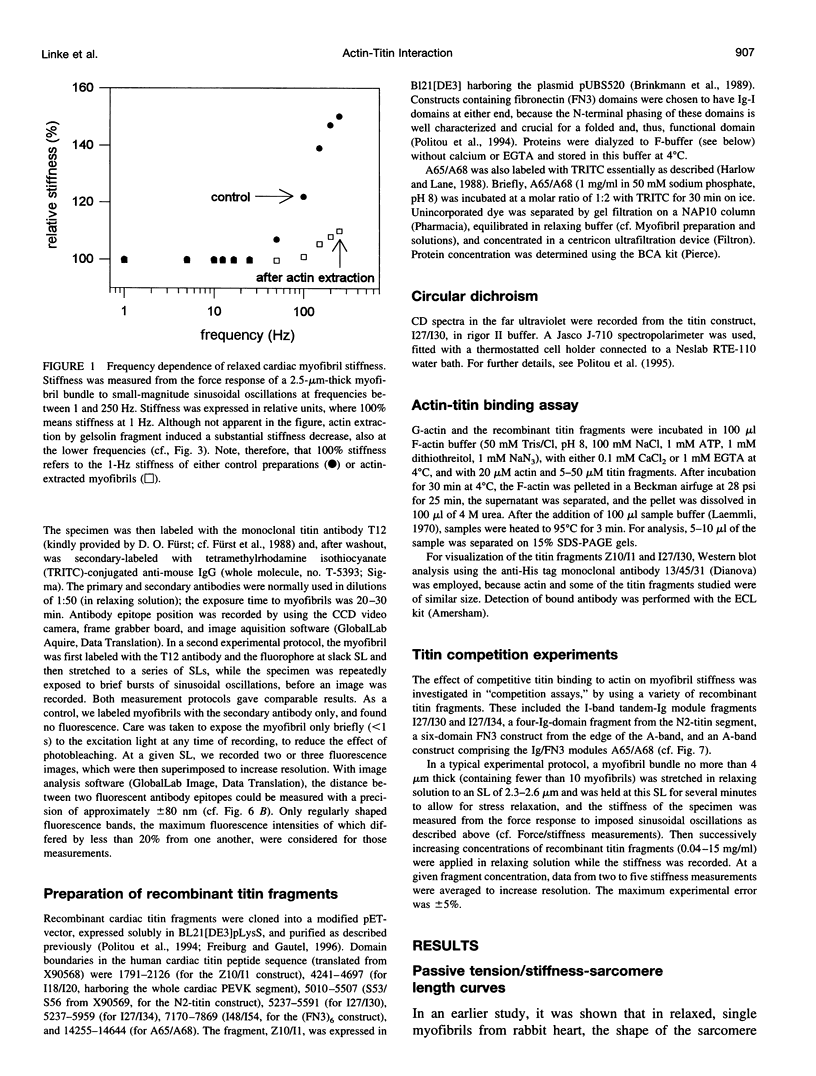
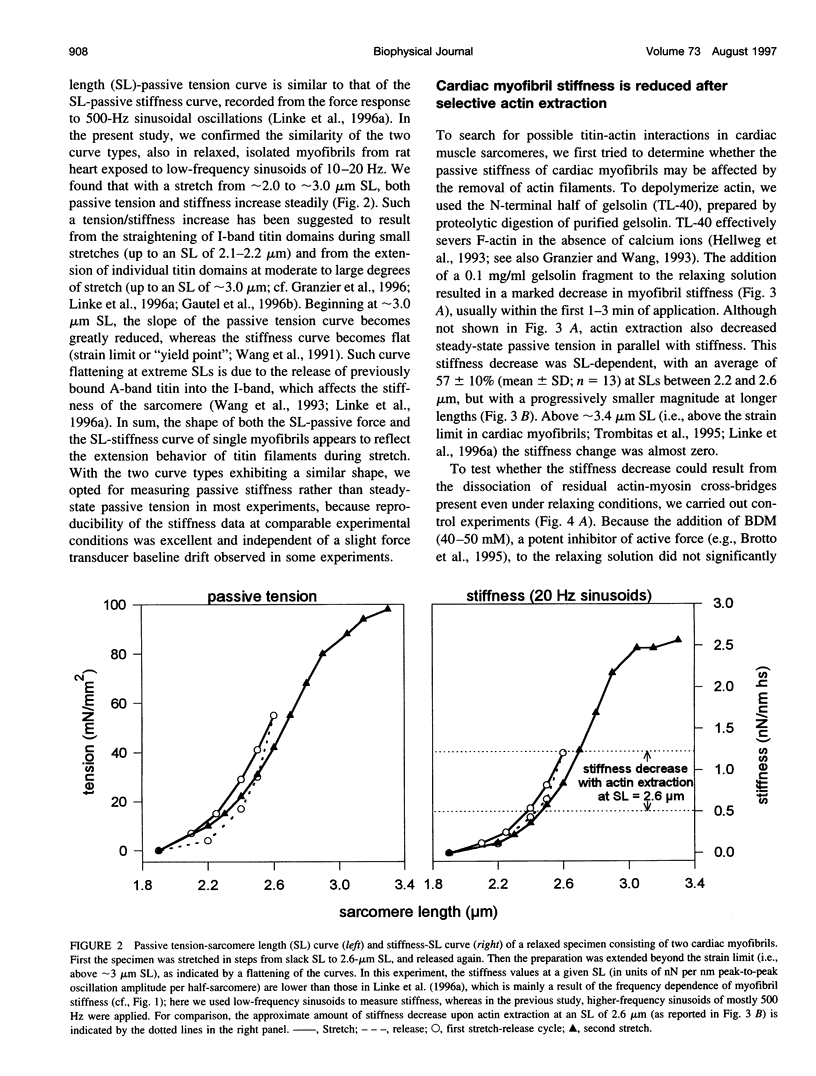
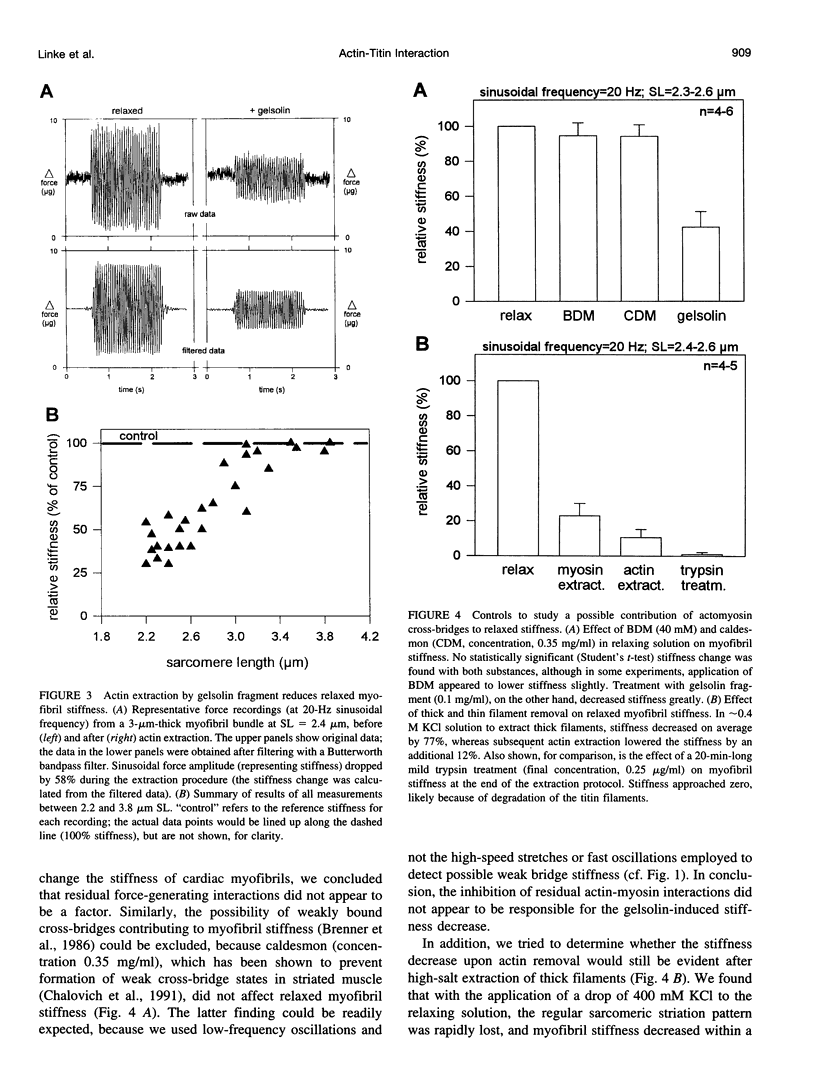
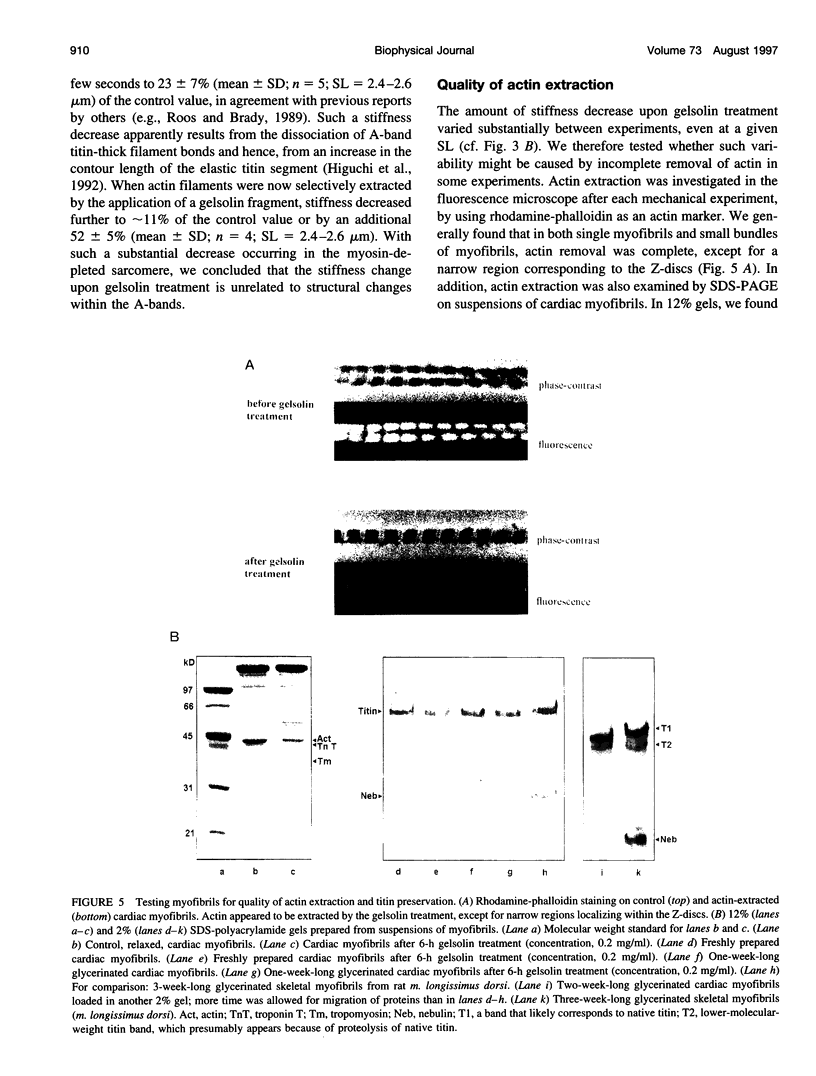
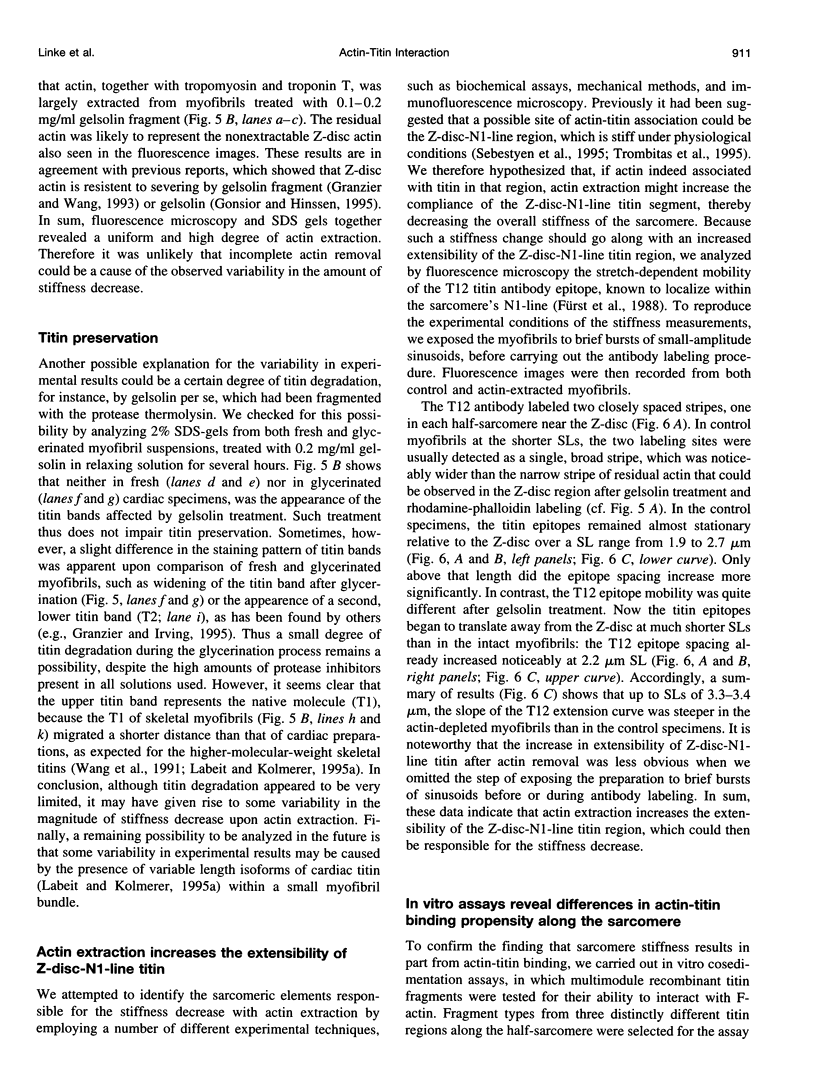
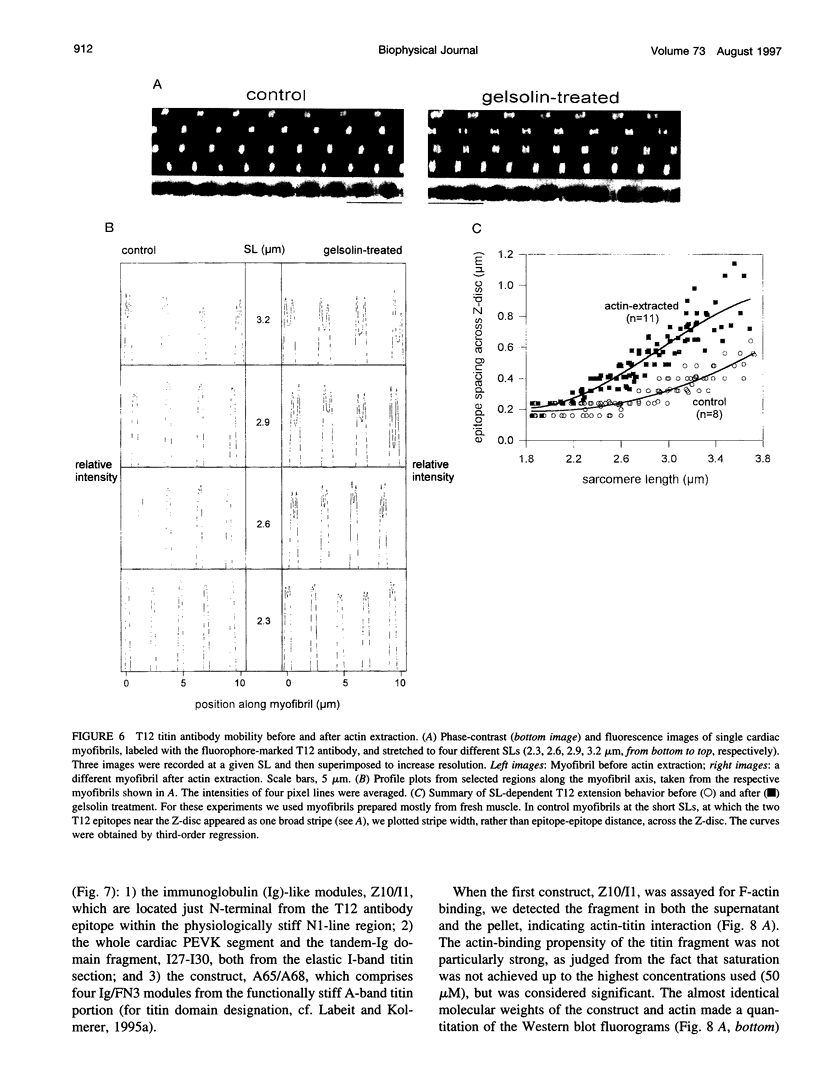
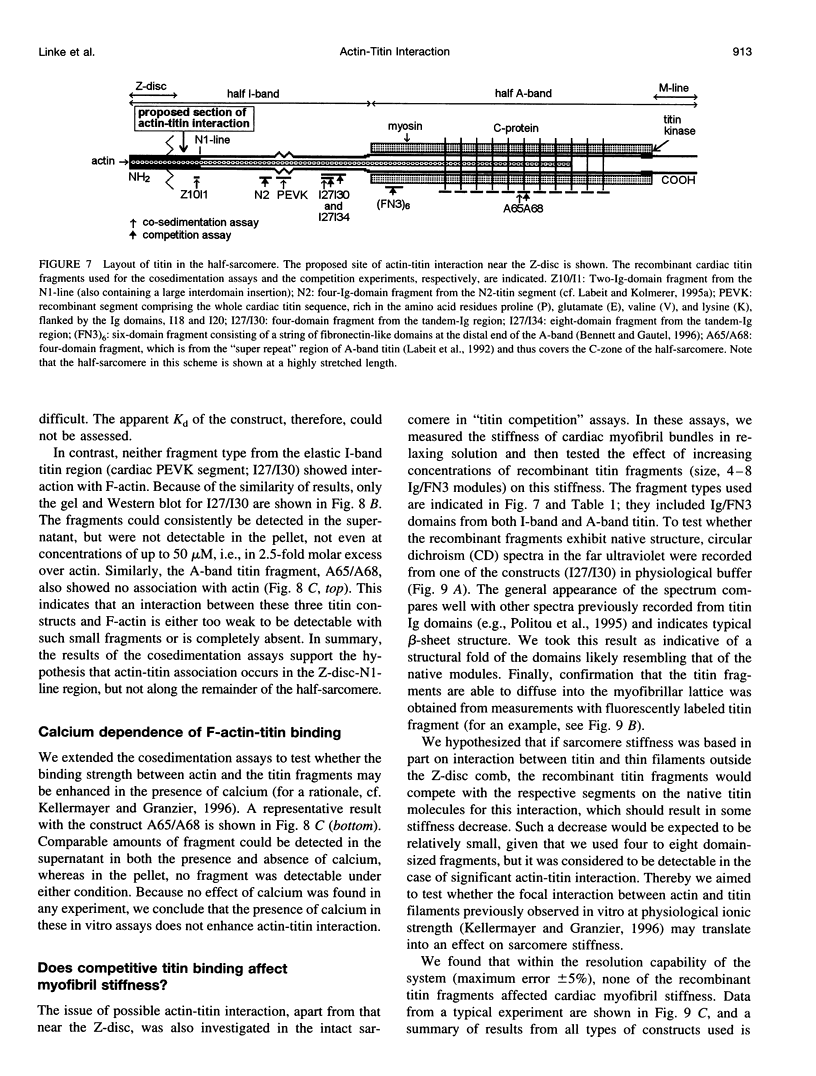
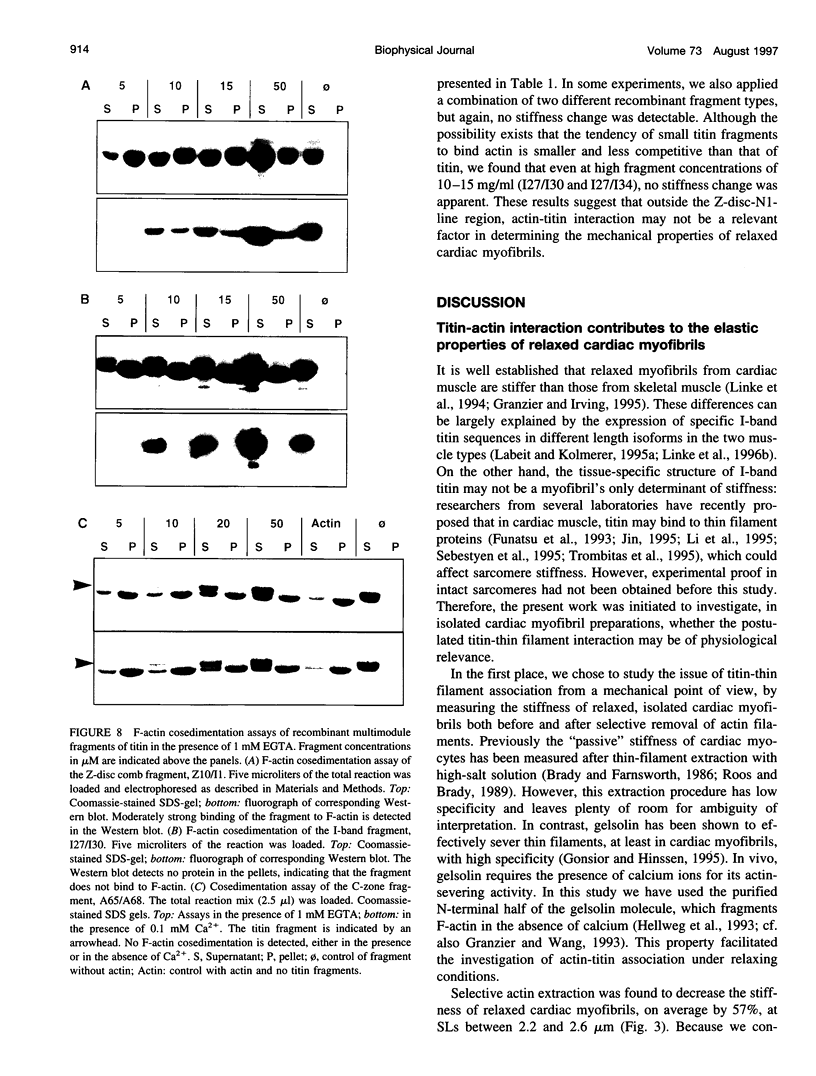
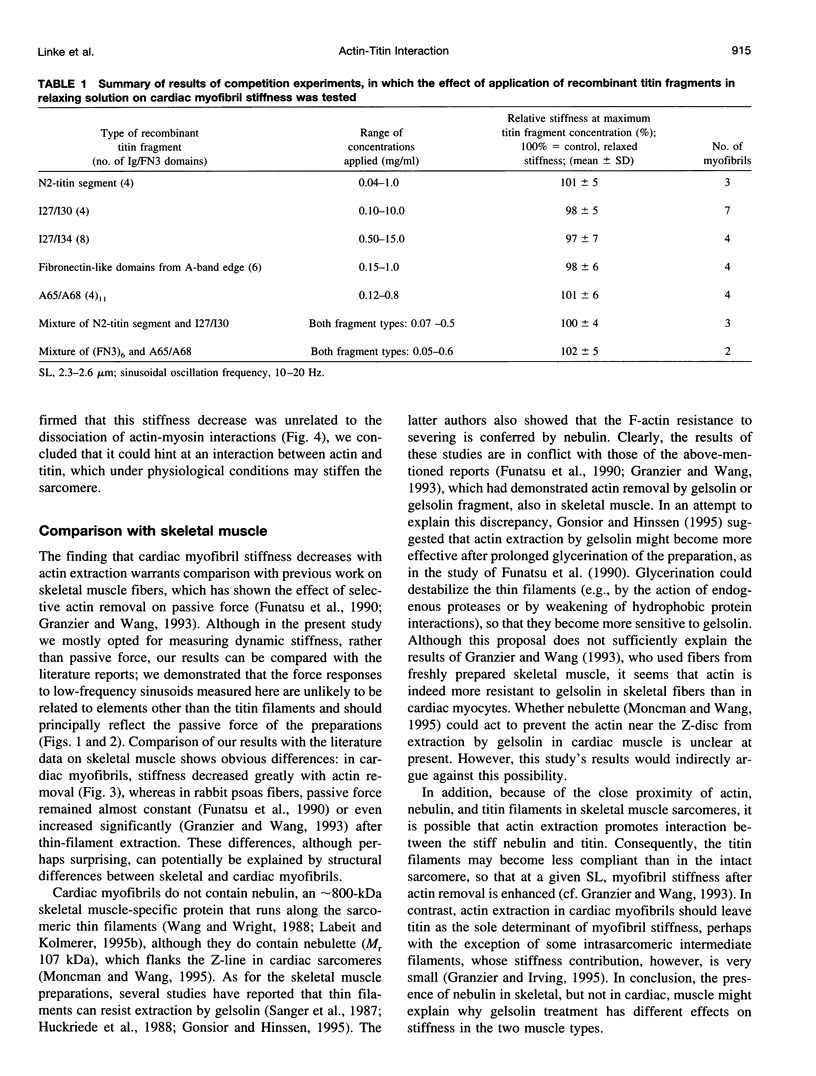
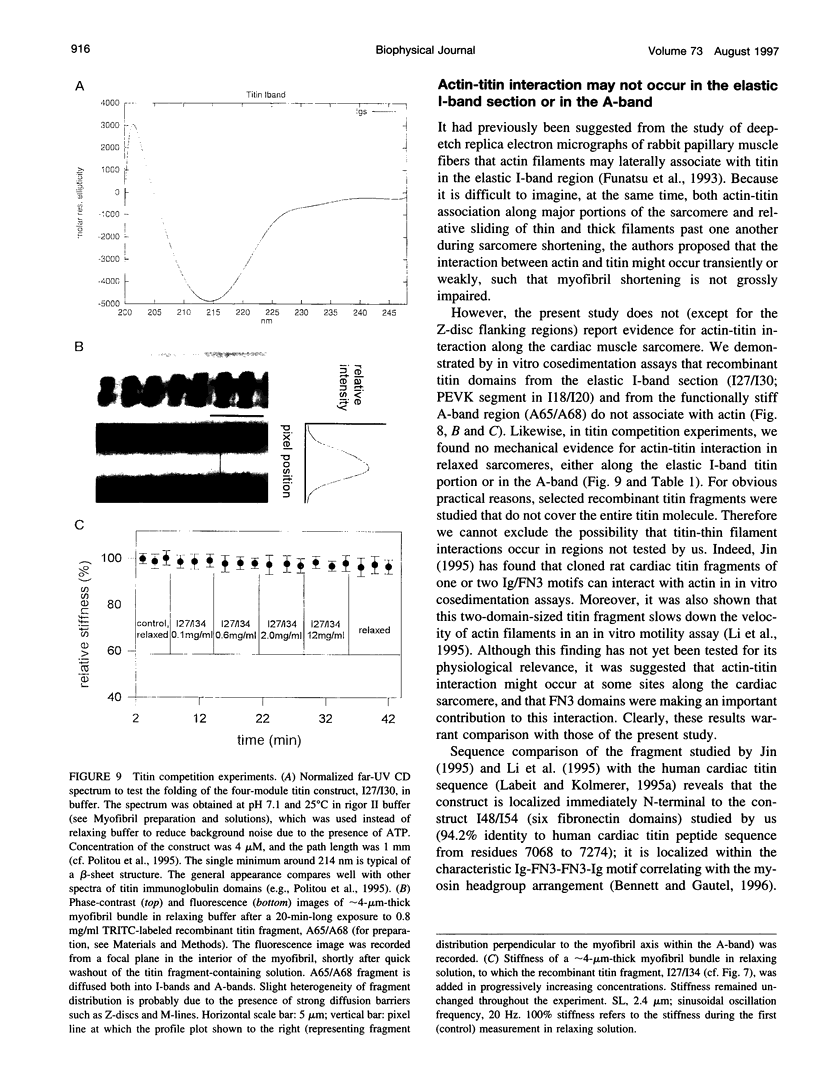
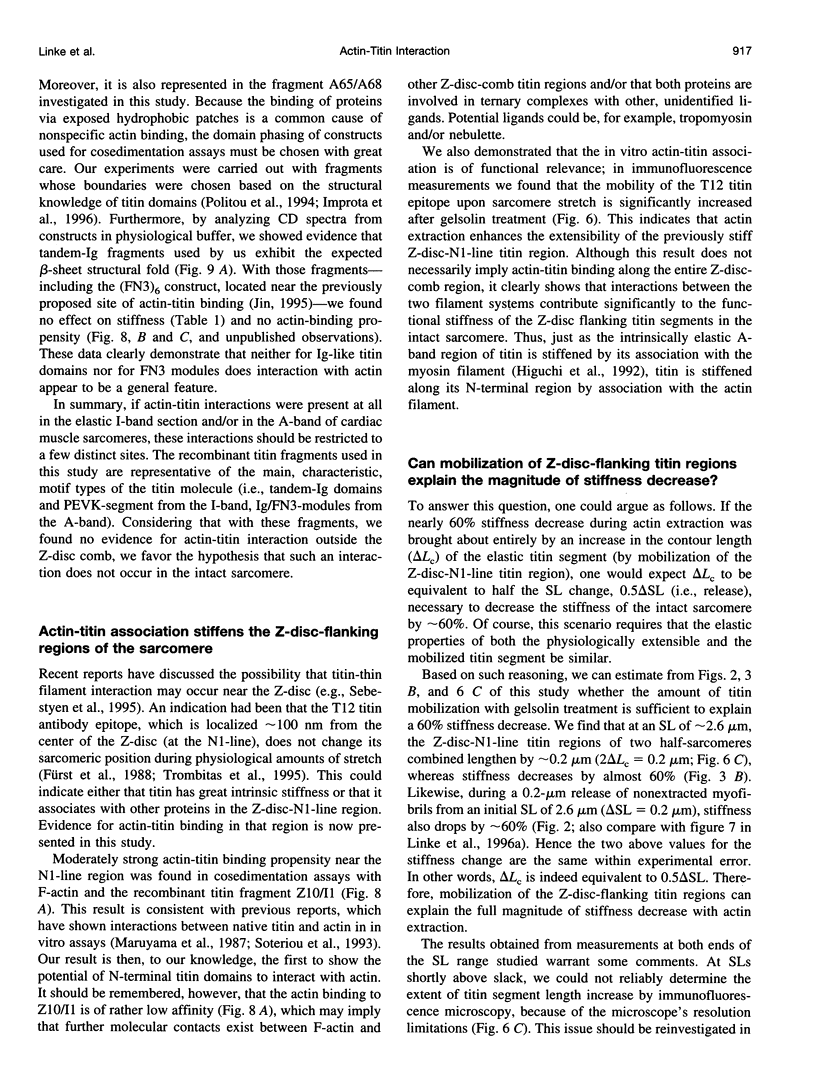
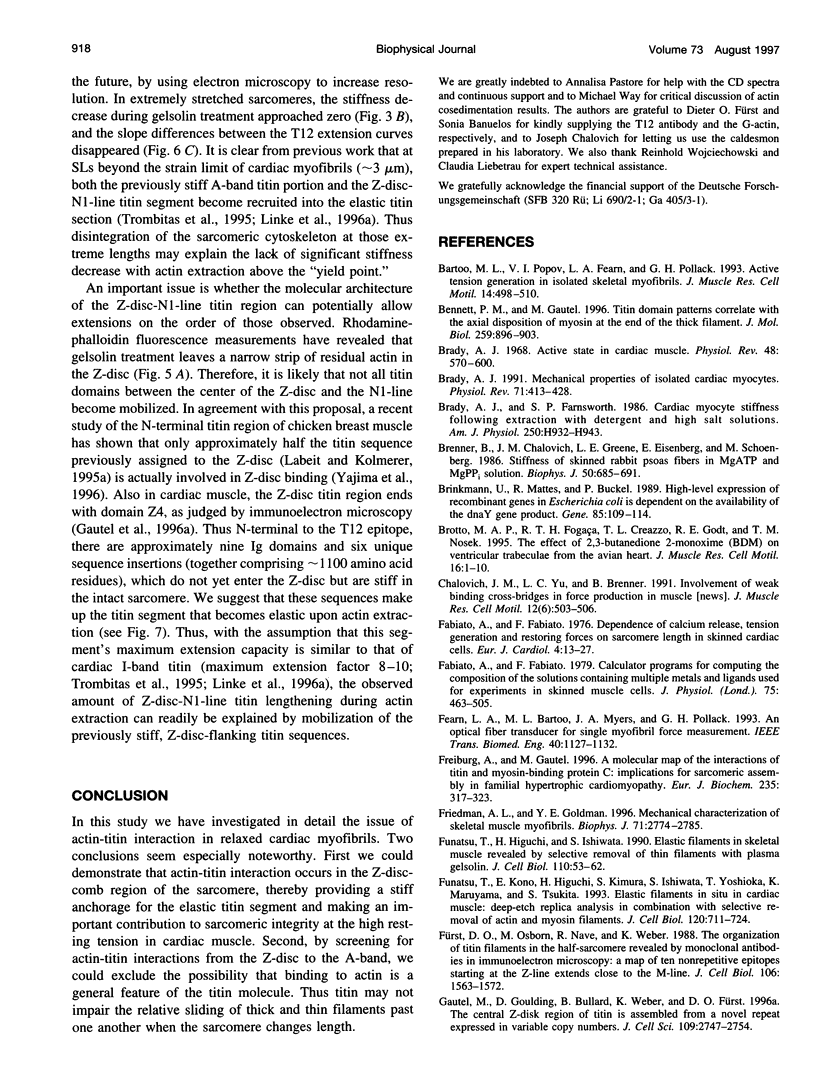
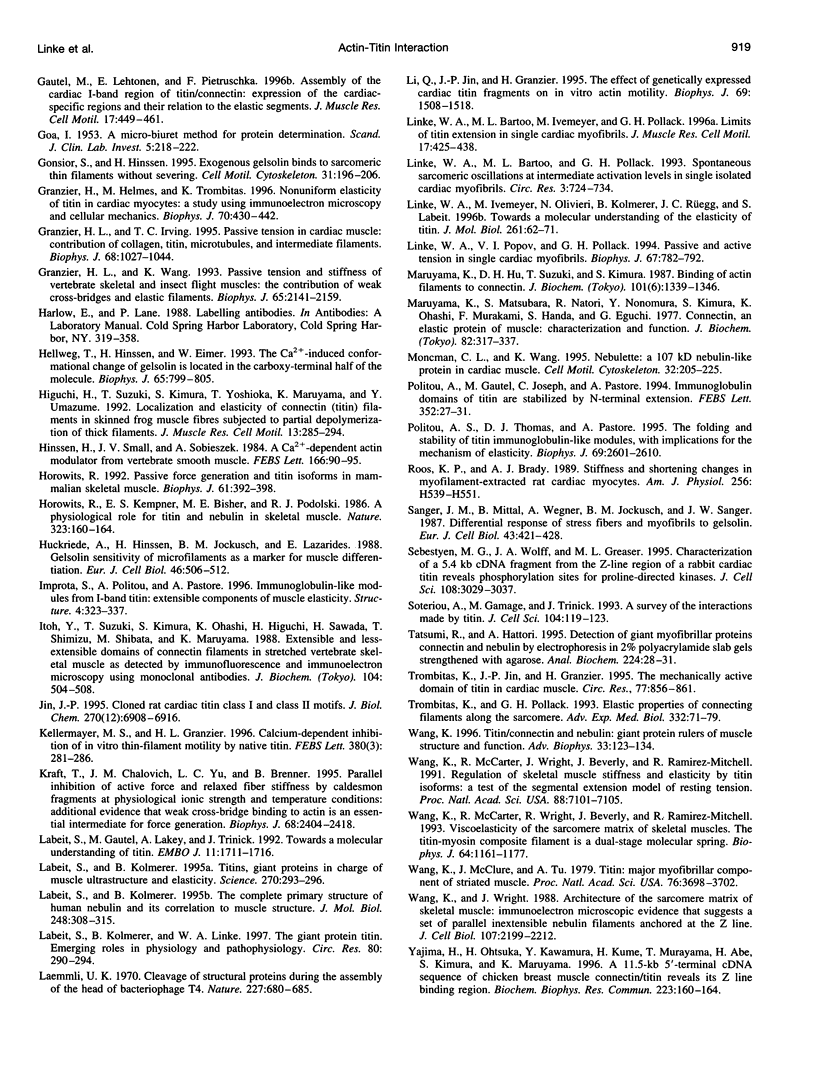
Images in this article
Selected References
These references are in PubMed. This may not be the complete list of references from this article.
- Bartoo M. L., Popov V. I., Fearn L. A., Pollack G. H. Active tension generation in isolated skeletal myofibrils. J Muscle Res Cell Motil. 1993 Oct;14(5):498–510. doi: 10.1007/BF00297212. [DOI] [PubMed] [Google Scholar]
- Bennett P. M., Gautel M. Titin domain patterns correlate with the axial disposition of myosin at the end of the thick filament. J Mol Biol. 1996 Jun 28;259(5):896–903. doi: 10.1006/jmbi.1996.0367. [DOI] [PubMed] [Google Scholar]
- Brady A. J. Active state in cardiac muscle. Physiol Rev. 1968 Jul;48(3):570–600. doi: 10.1152/physrev.1968.48.3.570. [DOI] [PubMed] [Google Scholar]
- Brady A. J., Farnsworth S. P. Cardiac myocyte stiffness following extraction with detergent and high salt solutions. Am J Physiol. 1986 Jun;250(6 Pt 2):H932–H943. doi: 10.1152/ajpheart.1986.250.6.H932. [DOI] [PubMed] [Google Scholar]
- Brady A. J. Mechanical properties of isolated cardiac myocytes. Physiol Rev. 1991 Apr;71(2):413–428. doi: 10.1152/physrev.1991.71.2.413. [DOI] [PubMed] [Google Scholar]
- Brenner B., Chalovich J. M., Greene L. E., Eisenberg E., Schoenberg M. Stiffness of skinned rabbit psoas fibers in MgATP and MgPPi solution. Biophys J. 1986 Oct;50(4):685–691. doi: 10.1016/S0006-3495(86)83509-3. [DOI] [PMC free article] [PubMed] [Google Scholar]
- Brinkmann U., Mattes R. E., Buckel P. High-level expression of recombinant genes in Escherichia coli is dependent on the availability of the dnaY gene product. Gene. 1989 Dec 21;85(1):109–114. doi: 10.1016/0378-1119(89)90470-8. [DOI] [PubMed] [Google Scholar]
- Brotto M. A., Fogaça R. T., Creazzo T. L., Godt R. E., Nosek T. M. The effect of 2,3-butanedione 2-monoxime (BDM) on ventricular trabeculae from the avian heart. J Muscle Res Cell Motil. 1995 Feb;16(1):1–10. doi: 10.1007/BF00125305. [DOI] [PubMed] [Google Scholar]
- Chalovich J. M., Yu L. C., Brenner B. Involvement of weak binding crossbridges in force production in muscle. J Muscle Res Cell Motil. 1991 Dec;12(6):503–506. doi: 10.1007/BF01738438. [DOI] [PubMed] [Google Scholar]
- Fabiato A., Fabiato F. Calculator programs for computing the composition of the solutions containing multiple metals and ligands used for experiments in skinned muscle cells. J Physiol (Paris) 1979;75(5):463–505. [PubMed] [Google Scholar]
- Fabiato A., Fabiato F. Dependence of calcium release, tension generation and restoring forces on sarcomere length in skinned cardiac cells. Eur J Cardiol. 1976 May;4 (Suppl):13–27. [PubMed] [Google Scholar]
- Fearn L. A., Bartoo M. L., Myers J. A., Pollack G. H. An optical fiber transducer for single myofibril force measurement. IEEE Trans Biomed Eng. 1993 Nov;40(11):1127–1132. doi: 10.1109/10.245630. [DOI] [PubMed] [Google Scholar]
- Freiburg A., Gautel M. A molecular map of the interactions between titin and myosin-binding protein C. Implications for sarcomeric assembly in familial hypertrophic cardiomyopathy. Eur J Biochem. 1996 Jan 15;235(1-2):317–323. doi: 10.1111/j.1432-1033.1996.00317.x. [DOI] [PubMed] [Google Scholar]
- Friedman A. L., Goldman Y. E. Mechanical characterization of skeletal muscle myofibrils. Biophys J. 1996 Nov;71(5):2774–2785. doi: 10.1016/S0006-3495(96)79470-5. [DOI] [PMC free article] [PubMed] [Google Scholar]
- Funatsu T., Higuchi H., Ishiwata S. Elastic filaments in skeletal muscle revealed by selective removal of thin filaments with plasma gelsolin. J Cell Biol. 1990 Jan;110(1):53–62. doi: 10.1083/jcb.110.1.53. [DOI] [PMC free article] [PubMed] [Google Scholar]
- Funatsu T., Kono E., Higuchi H., Kimura S., Ishiwata S., Yoshioka T., Maruyama K., Tsukita S. Elastic filaments in situ in cardiac muscle: deep-etch replica analysis in combination with selective removal of actin and myosin filaments. J Cell Biol. 1993 Feb;120(3):711–724. doi: 10.1083/jcb.120.3.711. [DOI] [PMC free article] [PubMed] [Google Scholar]
- Fürst D. O., Osborn M., Nave R., Weber K. The organization of titin filaments in the half-sarcomere revealed by monoclonal antibodies in immunoelectron microscopy: a map of ten nonrepetitive epitopes starting at the Z line extends close to the M line. J Cell Biol. 1988 May;106(5):1563–1572. doi: 10.1083/jcb.106.5.1563. [DOI] [PMC free article] [PubMed] [Google Scholar]
- GOA J. A micro biuret method for protein determination; determination of total protein in cerebrospinal fluid. Scand J Clin Lab Invest. 1953;5(3):218–222. doi: 10.3109/00365515309094189. [DOI] [PubMed] [Google Scholar]
- Gautel M., Goulding D., Bullard B., Weber K., Fürst D. O. The central Z-disk region of titin is assembled from a novel repeat in variable copy numbers. J Cell Sci. 1996 Nov;109(Pt 11):2747–2754. doi: 10.1242/jcs.109.11.2747. [DOI] [PubMed] [Google Scholar]
- Gautel M., Lehtonen E., Pietruschka F. Assembly of the cardiac I-band region of titin/connectin: expression of the cardiac-specific regions and their structural relation to the elastic segments. J Muscle Res Cell Motil. 1996 Aug;17(4):449–461. doi: 10.1007/BF00123361. [DOI] [PubMed] [Google Scholar]
- Gonsior S., Hinssen H. Exogenous gelsolin binds to sarcomeric thin filaments without severing. Cell Motil Cytoskeleton. 1995;31(3):196–206. doi: 10.1002/cm.970310303. [DOI] [PubMed] [Google Scholar]
- Granzier H. L., Irving T. C. Passive tension in cardiac muscle: contribution of collagen, titin, microtubules, and intermediate filaments. Biophys J. 1995 Mar;68(3):1027–1044. doi: 10.1016/S0006-3495(95)80278-X. [DOI] [PMC free article] [PubMed] [Google Scholar]
- Granzier H. L., Wang K. Passive tension and stiffness of vertebrate skeletal and insect flight muscles: the contribution of weak cross-bridges and elastic filaments. Biophys J. 1993 Nov;65(5):2141–2159. doi: 10.1016/S0006-3495(93)81262-1. [DOI] [PMC free article] [PubMed] [Google Scholar]
- Granzier H., Helmes M., Trombitás K. Nonuniform elasticity of titin in cardiac myocytes: a study using immunoelectron microscopy and cellular mechanics. Biophys J. 1996 Jan;70(1):430–442. doi: 10.1016/S0006-3495(96)79586-3. [DOI] [PMC free article] [PubMed] [Google Scholar]
- Hellweg T., Hinssen H., Eimer W. The Ca(2+)-induced conformational change of gelsolin is located in the carboxyl-terminal half of the molecule. Biophys J. 1993 Aug;65(2):799–805. doi: 10.1016/S0006-3495(93)81121-4. [DOI] [PMC free article] [PubMed] [Google Scholar]
- Higuchi H., Suzuki T., Kimura S., Yoshioka T., Maruyama K., Umazume Y. Localization and elasticity of connectin (titin) filaments in skinned frog muscle fibres subjected to partial depolymerization of thick filaments. J Muscle Res Cell Motil. 1992 Jun;13(3):285–294. doi: 10.1007/BF01766456. [DOI] [PubMed] [Google Scholar]
- Hinssen H., Small J. V., Sobieszek A. A Ca2+-dependent actin modulator from vertebrate smooth muscle. FEBS Lett. 1984 Jan 23;166(1):90–95. doi: 10.1016/0014-5793(84)80051-4. [DOI] [PubMed] [Google Scholar]
- Horowits R., Kempner E. S., Bisher M. E., Podolsky R. J. A physiological role for titin and nebulin in skeletal muscle. Nature. 1986 Sep 11;323(6084):160–164. doi: 10.1038/323160a0. [DOI] [PubMed] [Google Scholar]
- Horowits R. Passive force generation and titin isoforms in mammalian skeletal muscle. Biophys J. 1992 Feb;61(2):392–398. doi: 10.1016/S0006-3495(92)81845-3. [DOI] [PMC free article] [PubMed] [Google Scholar]
- Huckriede A., Hinssen H., Jockusch B. M., Lazarides E. Gelsolin sensitivity of microfilaments as a marker for muscle differentiation. Eur J Cell Biol. 1988 Aug;46(3):506–512. [PubMed] [Google Scholar]
- Improta S., Politou A. S., Pastore A. Immunoglobulin-like modules from titin I-band: extensible components of muscle elasticity. Structure. 1996 Mar 15;4(3):323–337. doi: 10.1016/s0969-2126(96)00036-6. [DOI] [PubMed] [Google Scholar]
- Itoh Y., Suzuki T., Kimura S., Ohashi K., Higuchi H., Sawada H., Shimizu T., Shibata M., Maruyama K. Extensible and less-extensible domains of connectin filaments in stretched vertebrate skeletal muscle sarcomeres as detected by immunofluorescence and immunoelectron microscopy using monoclonal antibodies. J Biochem. 1988 Oct;104(4):504–508. doi: 10.1093/oxfordjournals.jbchem.a122499. [DOI] [PubMed] [Google Scholar]
- Jin J. P. Cloned rat cardiac titin class I and class II motifs. Expression, purification, characterization, and interaction with F-actin. J Biol Chem. 1995 Mar 24;270(12):6908–6916. [PubMed] [Google Scholar]
- Kellermayer M. S., Granzier H. L. Calcium-dependent inhibition of in vitro thin-filament motility by native titin. FEBS Lett. 1996 Feb 19;380(3):281–286. doi: 10.1016/0014-5793(96)00055-5. [DOI] [PubMed] [Google Scholar]
- Kraft T., Chalovich J. M., Yu L. C., Brenner B. Parallel inhibition of active force and relaxed fiber stiffness by caldesmon fragments at physiological ionic strength and temperature conditions: additional evidence that weak cross-bridge binding to actin is an essential intermediate for force generation. Biophys J. 1995 Jun;68(6):2404–2418. doi: 10.1016/S0006-3495(95)80423-6. [DOI] [PMC free article] [PubMed] [Google Scholar]
- Labeit S., Gautel M., Lakey A., Trinick J. Towards a molecular understanding of titin. EMBO J. 1992 May;11(5):1711–1716. doi: 10.1002/j.1460-2075.1992.tb05222.x. [DOI] [PMC free article] [PubMed] [Google Scholar]
- Labeit S., Kolmerer B., Linke W. A. The giant protein titin. Emerging roles in physiology and pathophysiology. Circ Res. 1997 Feb;80(2):290–294. doi: 10.1161/01.res.80.2.290. [DOI] [PubMed] [Google Scholar]
- Labeit S., Kolmerer B. The complete primary structure of human nebulin and its correlation to muscle structure. J Mol Biol. 1995 Apr 28;248(2):308–315. doi: 10.1016/s0022-2836(95)80052-2. [DOI] [PubMed] [Google Scholar]
- Labeit S., Kolmerer B. Titins: giant proteins in charge of muscle ultrastructure and elasticity. Science. 1995 Oct 13;270(5234):293–296. doi: 10.1126/science.270.5234.293. [DOI] [PubMed] [Google Scholar]
- Laemmli U. K. Cleavage of structural proteins during the assembly of the head of bacteriophage T4. Nature. 1970 Aug 15;227(5259):680–685. doi: 10.1038/227680a0. [DOI] [PubMed] [Google Scholar]
- Li Q., Jin J. P., Granzier H. L. The effect of genetically expressed cardiac titin fragments on in vitro actin motility. Biophys J. 1995 Oct;69(4):1508–1518. doi: 10.1016/S0006-3495(95)80021-4. [DOI] [PMC free article] [PubMed] [Google Scholar]
- Linke W. A., Bartoo M. L., Ivemeyer M., Pollack G. H. Limits of titin extension in single cardiac myofibrils. J Muscle Res Cell Motil. 1996 Aug;17(4):425–438. doi: 10.1007/BF00123359. [DOI] [PubMed] [Google Scholar]
- Linke W. A., Bartoo M. L., Pollack G. H. Spontaneous sarcomeric oscillations at intermediate activation levels in single isolated cardiac myofibrils. Circ Res. 1993 Oct;73(4):724–734. doi: 10.1161/01.res.73.4.724. [DOI] [PubMed] [Google Scholar]
- Linke W. A., Ivemeyer M., Olivieri N., Kolmerer B., Rüegg J. C., Labeit S. Towards a molecular understanding of the elasticity of titin. J Mol Biol. 1996 Aug 9;261(1):62–71. doi: 10.1006/jmbi.1996.0441. [DOI] [PubMed] [Google Scholar]
- Linke W. A., Popov V. I., Pollack G. H. Passive and active tension in single cardiac myofibrils. Biophys J. 1994 Aug;67(2):782–792. doi: 10.1016/S0006-3495(94)80538-7. [DOI] [PMC free article] [PubMed] [Google Scholar]
- Maruyama K., Hu D. H., Suzuki T., Kimura S. Binding of actin filaments to connectin. J Biochem. 1987 Jun;101(6):1339–1346. doi: 10.1093/oxfordjournals.jbchem.a122001. [DOI] [PubMed] [Google Scholar]
- Maruyama K., Matsubara S., Natori R., Nonomura Y., Kimura S. Connectin, an elastic protein of muscle. Characterization and Function. J Biochem. 1977 Aug;82(2):317–337. [PubMed] [Google Scholar]
- Moncman C. L., Wang K. Nebulette: a 107 kD nebulin-like protein in cardiac muscle. Cell Motil Cytoskeleton. 1995;32(3):205–225. doi: 10.1002/cm.970320305. [DOI] [PubMed] [Google Scholar]
- Politou A. S., Gautel M., Joseph C., Pastore A. Immunoglobulin-type domains of titin are stabilized by amino-terminal extension. FEBS Lett. 1994 Sep 19;352(1):27–31. doi: 10.1016/0014-5793(94)00911-2. [DOI] [PubMed] [Google Scholar]
- Politou A. S., Thomas D. J., Pastore A. The folding and stability of titin immunoglobulin-like modules, with implications for the mechanism of elasticity. Biophys J. 1995 Dec;69(6):2601–2610. doi: 10.1016/S0006-3495(95)80131-1. [DOI] [PMC free article] [PubMed] [Google Scholar]
- Roos K. P., Brady A. J. Stiffness and shortening changes in myofilament-extracted rat cardiac myocytes. Am J Physiol. 1989 Feb;256(2 Pt 2):H539–H551. doi: 10.1152/ajpheart.1989.256.2.H539. [DOI] [PubMed] [Google Scholar]
- Sanger J. M., Mittal B., Wegner A., Jockusch B. M., Sanger J. W. Differential response of stress fibers and myofibrils to gelsolin. Eur J Cell Biol. 1987 Jun;43(3):421–428. [PubMed] [Google Scholar]
- Sebestyén M. G., Wolff J. A., Greaser M. L. Characterization of a 5.4 kb cDNA fragment from the Z-line region of rabbit cardiac titin reveals phosphorylation sites for proline-directed kinases. J Cell Sci. 1995 Sep;108(Pt 9):3029–3037. doi: 10.1242/jcs.108.9.3029. [DOI] [PubMed] [Google Scholar]
- Soteriou A., Gamage M., Trinick J. A survey of interactions made by the giant protein titin. J Cell Sci. 1993 Jan;104(Pt 1):119–123. doi: 10.1242/jcs.104.1.119. [DOI] [PubMed] [Google Scholar]
- Tatsumi R., Hattori A. Detection of giant myofibrillar proteins connectin and nebulin by electrophoresis in 2% polyacrylamide slab gels strengthened with agarose. Anal Biochem. 1995 Jan 1;224(1):28–31. doi: 10.1006/abio.1995.1004. [DOI] [PubMed] [Google Scholar]
- Trombitás K., Jin J. P., Granzier H. The mechanically active domain of titin in cardiac muscle. Circ Res. 1995 Oct;77(4):856–861. doi: 10.1161/01.res.77.4.856. [DOI] [PubMed] [Google Scholar]
- Trombitás K., Pollack G. H. Elastic properties of connecting filaments along the sarcomere. Adv Exp Med Biol. 1993;332:71–79. doi: 10.1007/978-1-4615-2872-2_7. [DOI] [PubMed] [Google Scholar]
- Wang K., McCarter R., Wright J., Beverly J., Ramirez-Mitchell R. Regulation of skeletal muscle stiffness and elasticity by titin isoforms: a test of the segmental extension model of resting tension. Proc Natl Acad Sci U S A. 1991 Aug 15;88(16):7101–7105. doi: 10.1073/pnas.88.16.7101. [DOI] [PMC free article] [PubMed] [Google Scholar]
- Wang K., McCarter R., Wright J., Beverly J., Ramirez-Mitchell R. Viscoelasticity of the sarcomere matrix of skeletal muscles. The titin-myosin composite filament is a dual-stage molecular spring. Biophys J. 1993 Apr;64(4):1161–1177. doi: 10.1016/S0006-3495(93)81482-6. [DOI] [PMC free article] [PubMed] [Google Scholar]
- Wang K., McClure J., Tu A. Titin: major myofibrillar components of striated muscle. Proc Natl Acad Sci U S A. 1979 Aug;76(8):3698–3702. doi: 10.1073/pnas.76.8.3698. [DOI] [PMC free article] [PubMed] [Google Scholar]
- Wang K. Titin/connectin and nebulin: giant protein rulers of muscle structure and function. Adv Biophys. 1996;33:123–134. [PubMed] [Google Scholar]
- Wang K., Wright J. Architecture of the sarcomere matrix of skeletal muscle: immunoelectron microscopic evidence that suggests a set of parallel inextensible nebulin filaments anchored at the Z line. J Cell Biol. 1988 Dec;107(6 Pt 1):2199–2212. doi: 10.1083/jcb.107.6.2199. [DOI] [PMC free article] [PubMed] [Google Scholar]
- Yajima H., Ohtsuka H., Kawamura Y., Kume H., Murayama T., Abe H., Kimura S., Maruyama K. A 11.5-kb 5'-terminal cDNA sequence of chicken breast muscle connectin/titin reveals its Z line binding region. Biochem Biophys Res Commun. 1996 Jun 5;223(1):160–164. doi: 10.1006/bbrc.1996.0862. [DOI] [PubMed] [Google Scholar]