Abstract
Linear dichroic difference Fourier transform infrared spectra upon formation of the M photointermediate were recorded with oriented purple membranes. The purpose was to determine the angle of the directions of the dipole moments of 1) the water molecule whose O-H stretching vibration appears at 3643 cm-1 for the unphotolyzed state and 3671 cm-1 for the M intermediate, and 2) the C=O bond of protonated Asp85 in the M intermediate. The angle of 36 degrees we find for the C=O of the protonated Asp85 in the M intermediate is not markedly different from 26 degrees for unprotonated Asp85 in the model based on cryoelectron diffraction, indicating the absence of gross orientation changes in Asp85 upon its protonation. The O-H band at 3671 cm-1 of a water molecule in the M intermediate, although its position has not determined, is fixed almost parallel to the membrane plane. For the unphotolyzed state the angle of the water O-H to the membrane normal was determined to be 60 degrees. On the basis of these data and the structural model, we place the water molecule in the unphotolyzed state at a position where it forms hydrogen bonds with the Schiff base, Asp85, Asp212, and Trp86.
Full text
PDF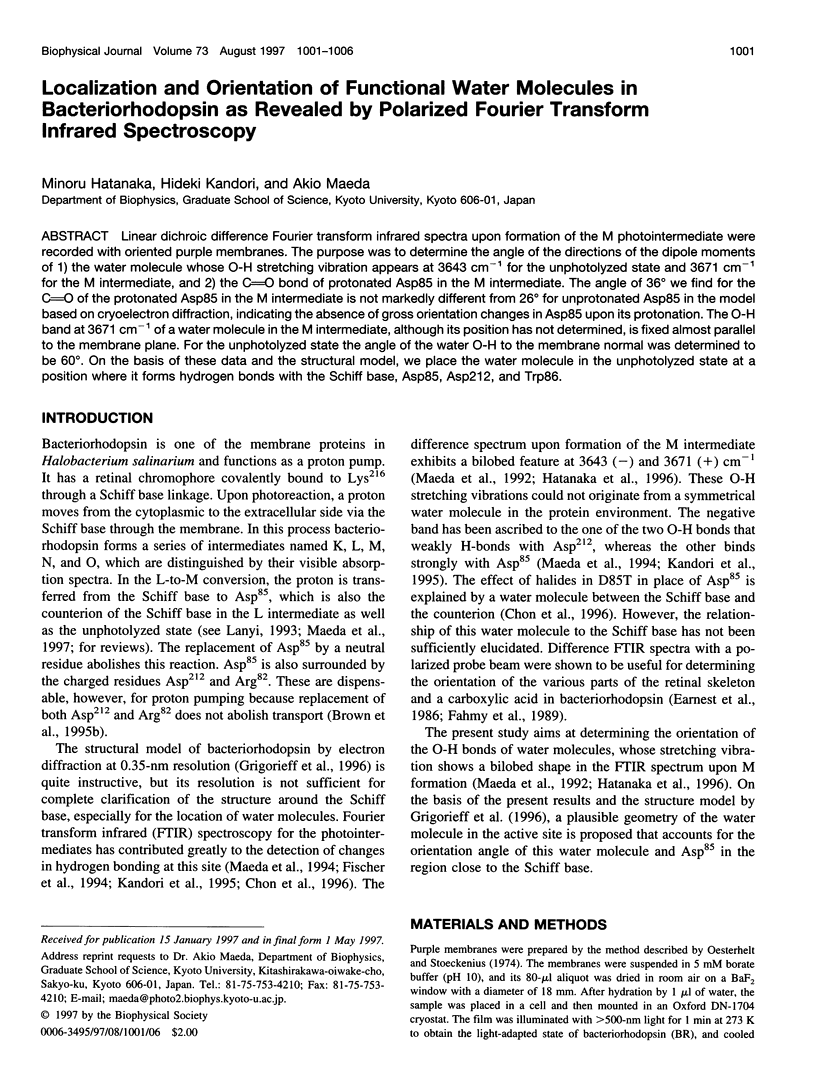
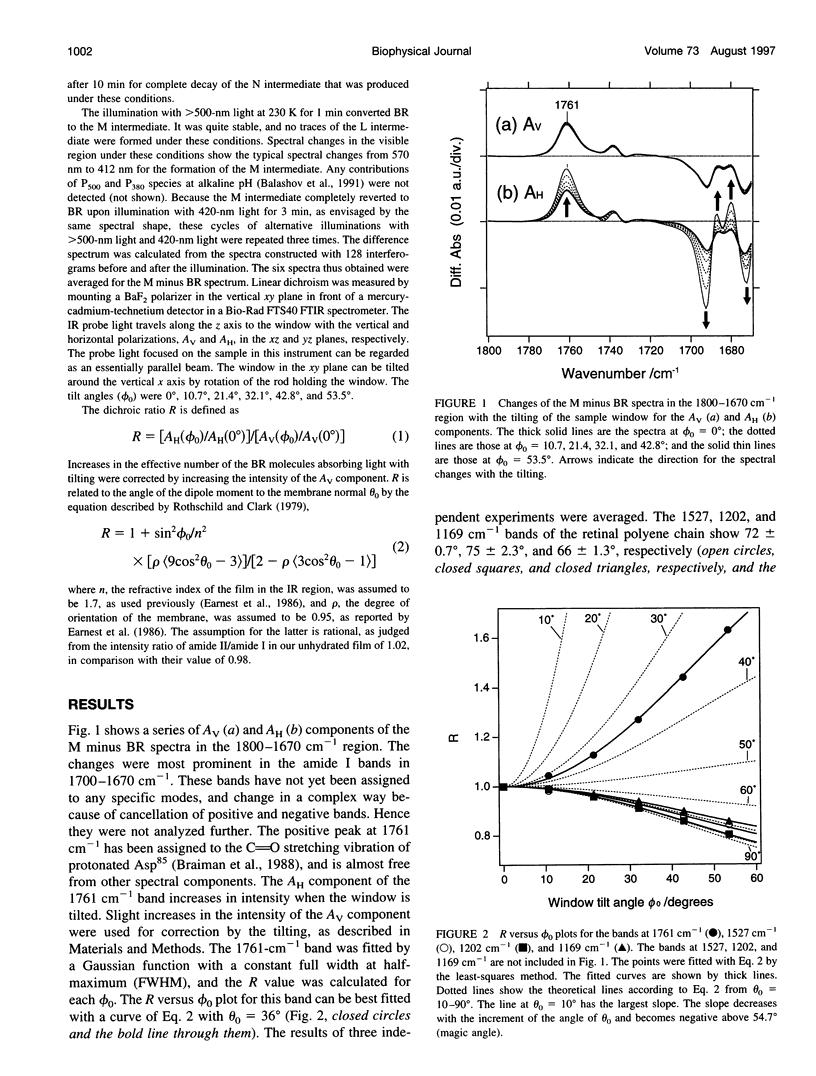
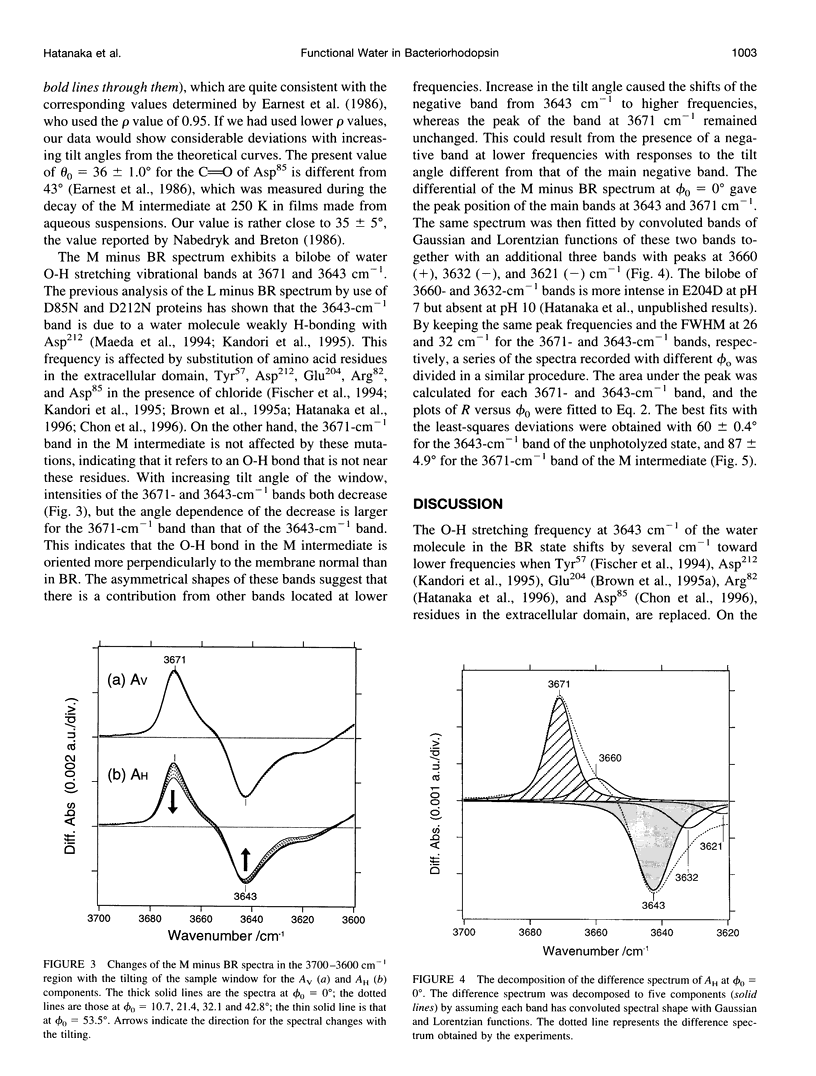
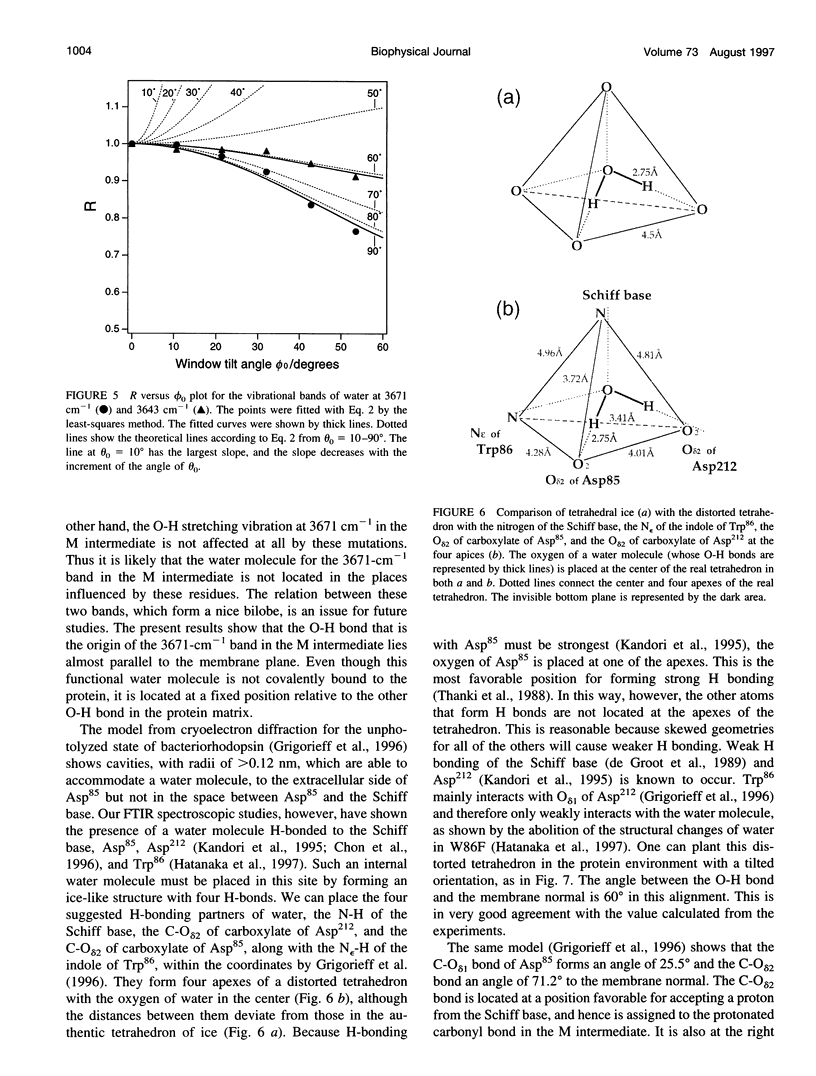
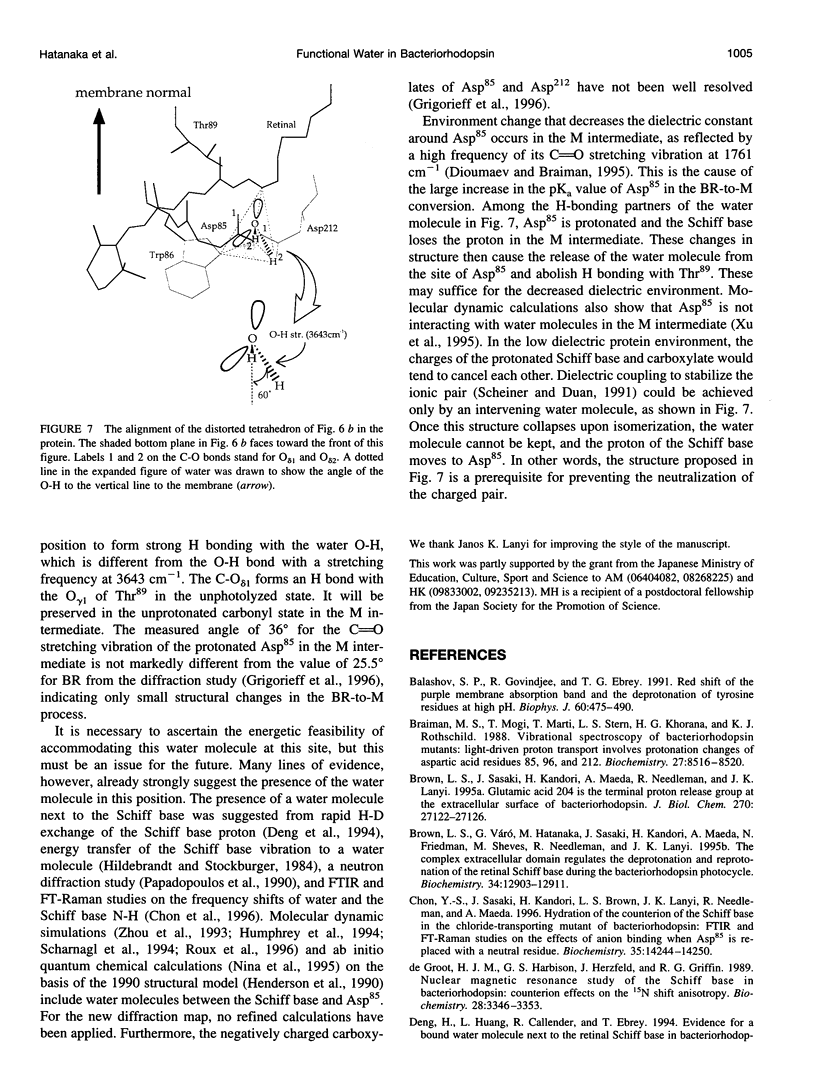
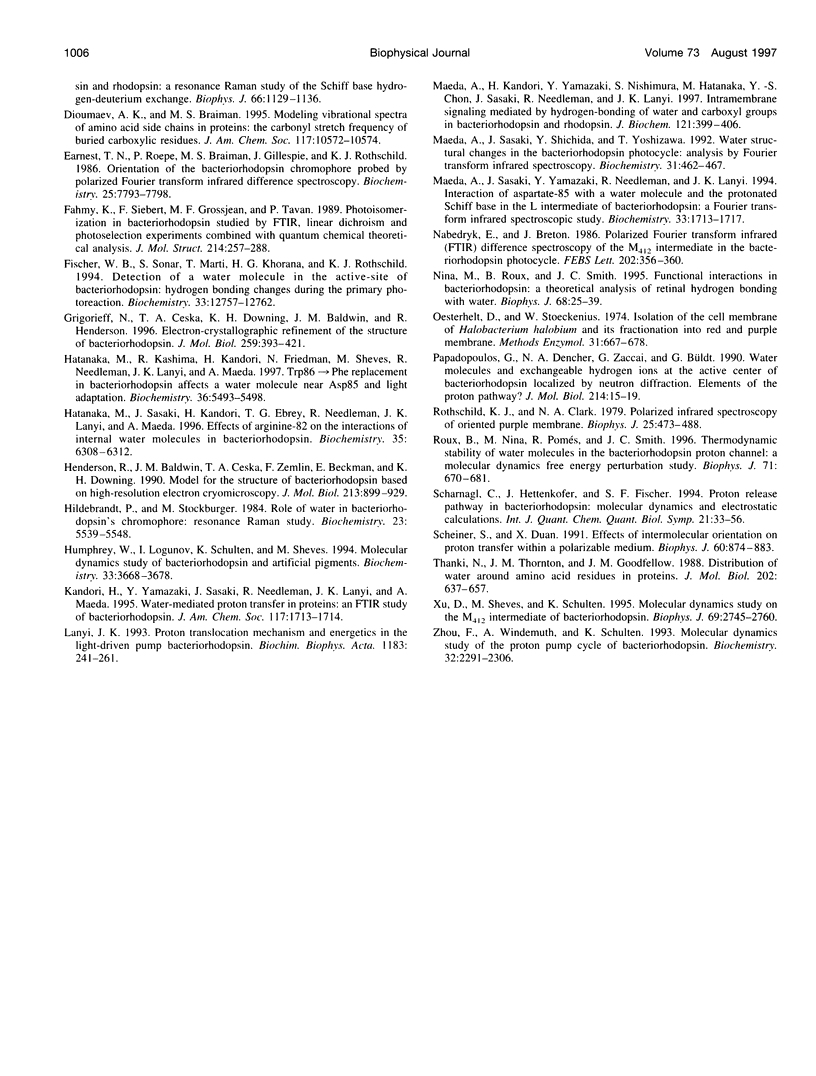
Images in this article
Selected References
These references are in PubMed. This may not be the complete list of references from this article.
- Balashov S. P., Govindjee R., Ebrey T. G. Redshift of the purple membrane absorption band and the deprotonation of tyrosine residues at high pH: Origin of the parallel photocycles of trans-bacteriorhodopsin. Biophys J. 1991 Aug;60(2):475–490. doi: 10.1016/S0006-3495(91)82074-4. [DOI] [PMC free article] [PubMed] [Google Scholar]
- Braiman M. S., Mogi T., Marti T., Stern L. J., Khorana H. G., Rothschild K. J. Vibrational spectroscopy of bacteriorhodopsin mutants: light-driven proton transport involves protonation changes of aspartic acid residues 85, 96, and 212. Biochemistry. 1988 Nov 15;27(23):8516–8520. doi: 10.1021/bi00423a002. [DOI] [PubMed] [Google Scholar]
- Brown L. S., Sasaki J., Kandori H., Maeda A., Needleman R., Lanyi J. K. Glutamic acid 204 is the terminal proton release group at the extracellular surface of bacteriorhodopsin. J Biol Chem. 1995 Nov 10;270(45):27122–27126. doi: 10.1074/jbc.270.45.27122. [DOI] [PubMed] [Google Scholar]
- Brown L. S., Váró G., Hatanaka M., Sasaki J., Kandori H., Maeda A., Friedman N., Sheves M., Nedleman R., Lanyi J. K. The complex extracellular domain regulates the deprotonation and reprotonation of the retinal Schiff base during the bacteriorhodopsin photocycle. Biochemistry. 1995 Oct 3;34(39):12903–12911. doi: 10.1021/bi00039a053. [DOI] [PubMed] [Google Scholar]
- Chon Y. S., Sasaki J., Kandori H., Brown L. S., Lanyi J. K., Needleman R., Maeda A. Hydration of the counterion of the Schiff base in the chloride-transporting mutant of bacteriorhodopsin: FTIR and FT-raman studies of the effects of anion binding when Asp85 is replaced with a neutral residue. Biochemistry. 1996 Nov 12;35(45):14244–14250. doi: 10.1021/bi9606197. [DOI] [PubMed] [Google Scholar]
- Deng H., Huang L., Callender R., Ebrey T. Evidence for a bound water molecule next to the retinal Schiff base in bacteriorhodopsin and rhodopsin: a resonance Raman study of the Schiff base hydrogen/deuterium exchange. Biophys J. 1994 Apr;66(4):1129–1136. doi: 10.1016/S0006-3495(94)80893-8. [DOI] [PMC free article] [PubMed] [Google Scholar]
- Earnest T. N., Roepe P., Braiman M. S., Gillespie J., Rothschild K. J. Orientation of the bacteriorhodopsin chromophore probed by polarized Fourier transform infrared difference spectroscopy. Biochemistry. 1986 Dec 2;25(24):7793–7798. doi: 10.1021/bi00372a002. [DOI] [PubMed] [Google Scholar]
- Fischer W. B., Sonar S., Marti T., Khorana H. G., Rothschild K. J. Detection of a water molecule in the active-site of bacteriorhodopsin: hydrogen bonding changes during the primary photoreaction. Biochemistry. 1994 Nov 1;33(43):12757–12762. doi: 10.1021/bi00209a005. [DOI] [PubMed] [Google Scholar]
- Grigorieff N., Ceska T. A., Downing K. H., Baldwin J. M., Henderson R. Electron-crystallographic refinement of the structure of bacteriorhodopsin. J Mol Biol. 1996 Jun 14;259(3):393–421. doi: 10.1006/jmbi.1996.0328. [DOI] [PubMed] [Google Scholar]
- Hatanaka M., Kashima R., Kandori H., Friedman N., Sheves M., Needleman R., Lanyi J. K., Maeda A. Trp86 --> Phe replacement in bacteriorhodopsin affects a water molecule near Asp85 and light adaptation. Biochemistry. 1997 May 6;36(18):5493–5498. doi: 10.1021/bi970081k. [DOI] [PubMed] [Google Scholar]
- Hatanaka M., Sasaki J., Kandori H., Ebrey T. G., Needleman R., Lanyi J. K., Maeda A. Effects of arginine-82 on the interactions of internal water molecules in bacteriorhodopsin. Biochemistry. 1996 May 21;35(20):6308–6312. doi: 10.1021/bi952973v. [DOI] [PubMed] [Google Scholar]
- Henderson R., Baldwin J. M., Ceska T. A., Zemlin F., Beckmann E., Downing K. H. Model for the structure of bacteriorhodopsin based on high-resolution electron cryo-microscopy. J Mol Biol. 1990 Jun 20;213(4):899–929. doi: 10.1016/S0022-2836(05)80271-2. [DOI] [PubMed] [Google Scholar]
- Humphrey W., Logunov I., Schulten K., Sheves M. Molecular dynamics study of bacteriorhodopsin and artificial pigments. Biochemistry. 1994 Mar 29;33(12):3668–3678. doi: 10.1021/bi00178a025. [DOI] [PubMed] [Google Scholar]
- Lanyi J. K. Proton translocation mechanism and energetics in the light-driven pump bacteriorhodopsin. Biochim Biophys Acta. 1993 Dec 7;1183(2):241–261. doi: 10.1016/0005-2728(93)90226-6. [DOI] [PubMed] [Google Scholar]
- Maeda A., Kandori H., Yamazaki Y., Nishimura S., Hatanaka M., Chon Y. S., Sasaki J., Needleman R., Lanyi J. K. Intramembrane signaling mediated by hydrogen-bonding of water and carboxyl groups in bacteriorhodopsin and rhodopsin. J Biochem. 1997 Mar;121(3):399–406. doi: 10.1093/oxfordjournals.jbchem.a021602. [DOI] [PubMed] [Google Scholar]
- Maeda A., Sasaki J., Shichida Y., Yoshizawa T. Water structural changes in the bacteriorhodopsin photocycle: analysis by Fourier transform infrared spectroscopy. Biochemistry. 1992 Jan 21;31(2):462–467. doi: 10.1021/bi00117a023. [DOI] [PubMed] [Google Scholar]
- Maeda A., Sasaki J., Yamazaki Y., Needleman R., Lanyi J. K. Interaction of aspartate-85 with a water molecule and the protonated Schiff base in the L intermediate of bacteriorhodopsin: a Fourier-transform infrared spectroscopic study. Biochemistry. 1994 Feb 22;33(7):1713–1717. doi: 10.1021/bi00173a013. [DOI] [PubMed] [Google Scholar]
- Nina M., Roux B., Smith J. C. Functional interactions in bacteriorhodopsin: a theoretical analysis of retinal hydrogen bonding with water. Biophys J. 1995 Jan;68(1):25–39. doi: 10.1016/S0006-3495(95)80184-0. [DOI] [PMC free article] [PubMed] [Google Scholar]
- Oesterhelt D., Stoeckenius W. Isolation of the cell membrane of Halobacterium halobium and its fractionation into red and purple membrane. Methods Enzymol. 1974;31:667–678. doi: 10.1016/0076-6879(74)31072-5. [DOI] [PubMed] [Google Scholar]
- Papadopoulos G., Dencher N. A., Zaccai G., Büldt G. Water molecules and exchangeable hydrogen ions at the active centre of bacteriorhodopsin localized by neutron diffraction. Elements of the proton pathway? J Mol Biol. 1990 Jul 5;214(1):15–19. doi: 10.1016/0022-2836(90)90140-h. [DOI] [PubMed] [Google Scholar]
- Rothschild K. J., Clark N. A. Polarized infrared spectroscopy of oriented purple membrane. Biophys J. 1979 Mar;25(3):473–487. doi: 10.1016/S0006-3495(79)85317-5. [DOI] [PMC free article] [PubMed] [Google Scholar]
- Roux B., Nina M., Pomès R., Smith J. C. Thermodynamic stability of water molecules in the bacteriorhodopsin proton channel: a molecular dynamics free energy perturbation study. Biophys J. 1996 Aug;71(2):670–681. doi: 10.1016/S0006-3495(96)79267-6. [DOI] [PMC free article] [PubMed] [Google Scholar]
- Scheiner S., Duan X. Effect of intermolecular orientation upon proton transfer within a polarizable medium. Biophys J. 1991 Oct;60(4):874–883. doi: 10.1016/S0006-3495(91)82121-X. [DOI] [PMC free article] [PubMed] [Google Scholar]
- Thanki N., Thornton J. M., Goodfellow J. M. Distributions of water around amino acid residues in proteins. J Mol Biol. 1988 Aug 5;202(3):637–657. doi: 10.1016/0022-2836(88)90292-6. [DOI] [PubMed] [Google Scholar]
- Xu D., Sheves M., Schulten K. Molecular dynamics study of the M412 intermediate of bacteriorhodopsin. Biophys J. 1995 Dec;69(6):2745–2760. doi: 10.1016/S0006-3495(95)80146-3. [DOI] [PMC free article] [PubMed] [Google Scholar]
- Zhou F., Windemuth A., Schulten K. Molecular dynamics study of the proton pump cycle of bacteriorhodopsin. Biochemistry. 1993 Mar 9;32(9):2291–2306. doi: 10.1021/bi00060a022. [DOI] [PubMed] [Google Scholar]
- de Groot H. J., Harbison G. S., Herzfeld J., Griffin R. G. Nuclear magnetic resonance study of the Schiff base in bacteriorhodopsin: counterion effects on the 15N shift anisotropy. Biochemistry. 1989 Apr 18;28(8):3346–3353. doi: 10.1021/bi00434a033. [DOI] [PubMed] [Google Scholar]