Abstract
Rabbit psoas muscle myofibrils, in the presence of the fluorescent nucleotide analog 2'(3')-O-[N-[2-[[Cy3]amido]ethyl]carbamoyl]-adenosine 5' triphosphate (Cy3-EDA-ATP), showed selective fluorescence staining of the A-band with a reduced fluorescence at the M-line. Addition of Cy3-EDA-ATP to a myofibril in the presence of Ca2+ caused auxotonic shortening against a compliant glass microneedle. These results indicate that Cy3-EDA-ATP is a substrate for myosin in the myofibril system. The kinetics of nucleotide release from a single myofibril, held isometrically between two needles, were measured by the displacement of prebound Cy3-EDA-ATP on flash photolysis of caged ATP. The A-band fluorescence of the myofibril decayed exponentially with a rate constant of 0.3 s(-1) at 8 degrees C, an order of magnitude faster than that for isolated thick filaments in the absence of actin. When a myofibril was imposed to shorten with a constant velocity by a piezoelectric actuator, the nucleotide displacement rate constant initially increased to 0.7 s(-1) with increasing shortening velocity and then declined with a further increase in shortening velocity. These results demonstrate that the displacement rates of Cy3-EDA-nucleotides bound to the cross-bridges in the contracting myofibril reflect a process that shows strain dependence.
Full text
PDF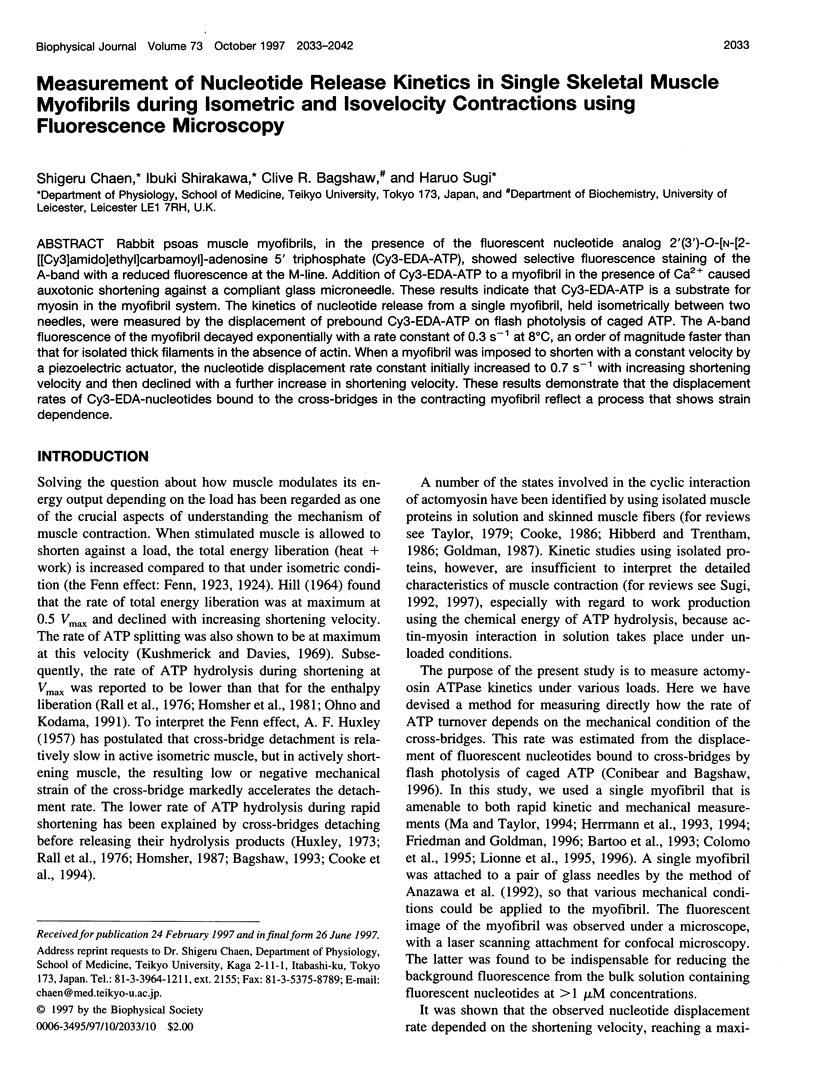
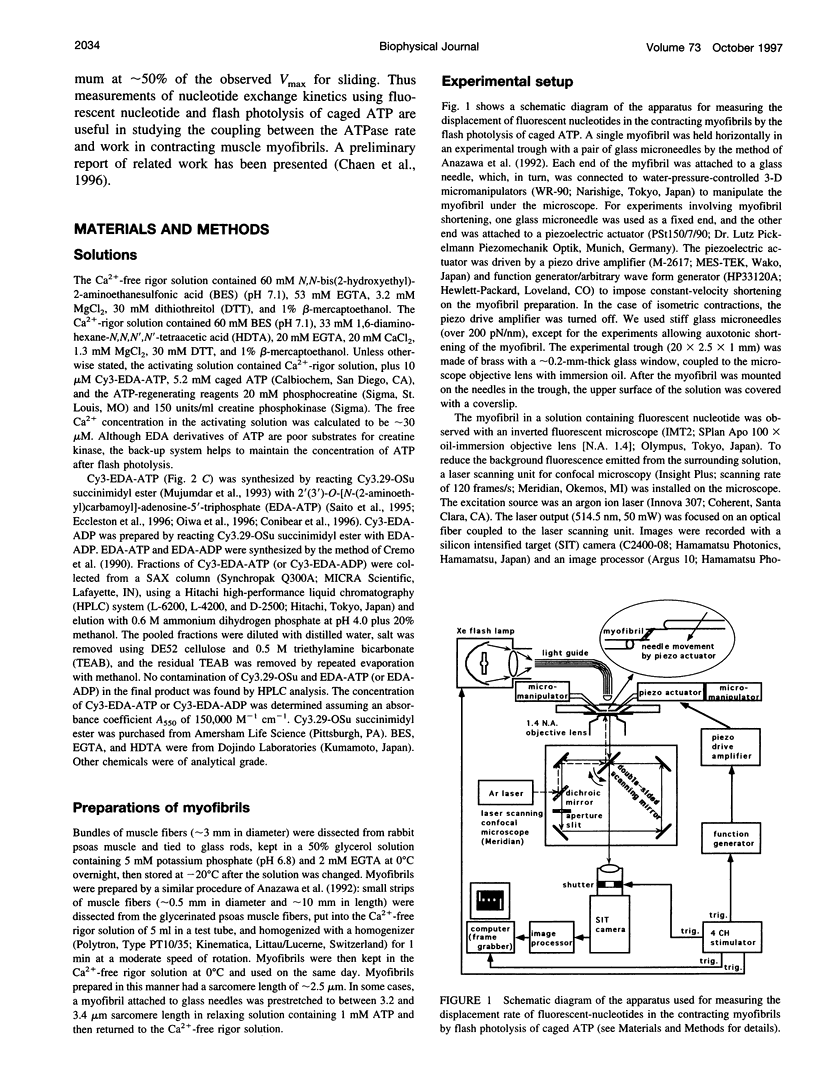
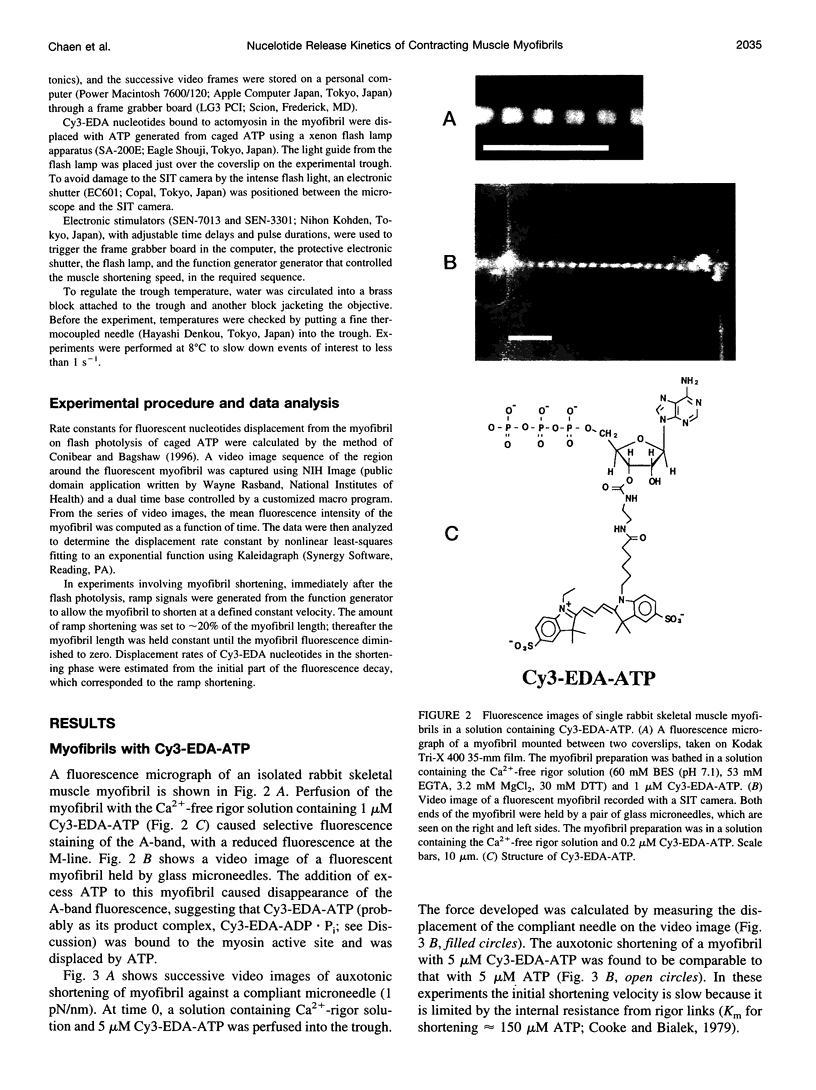
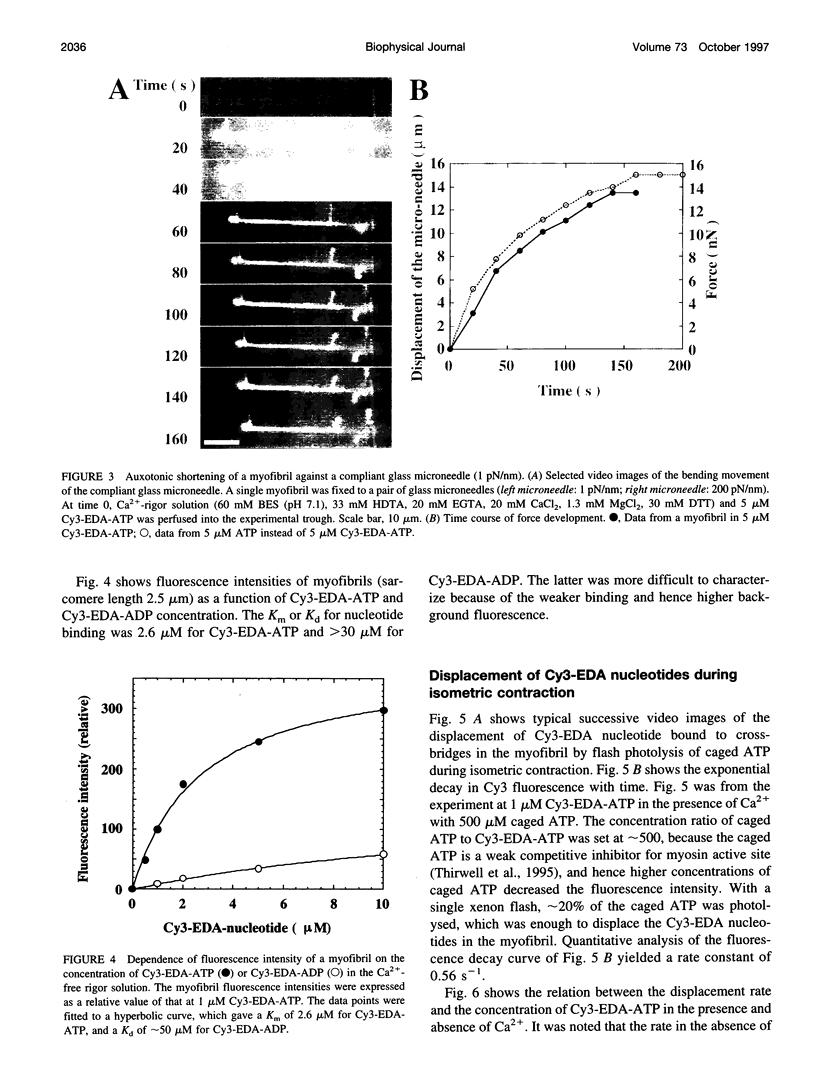
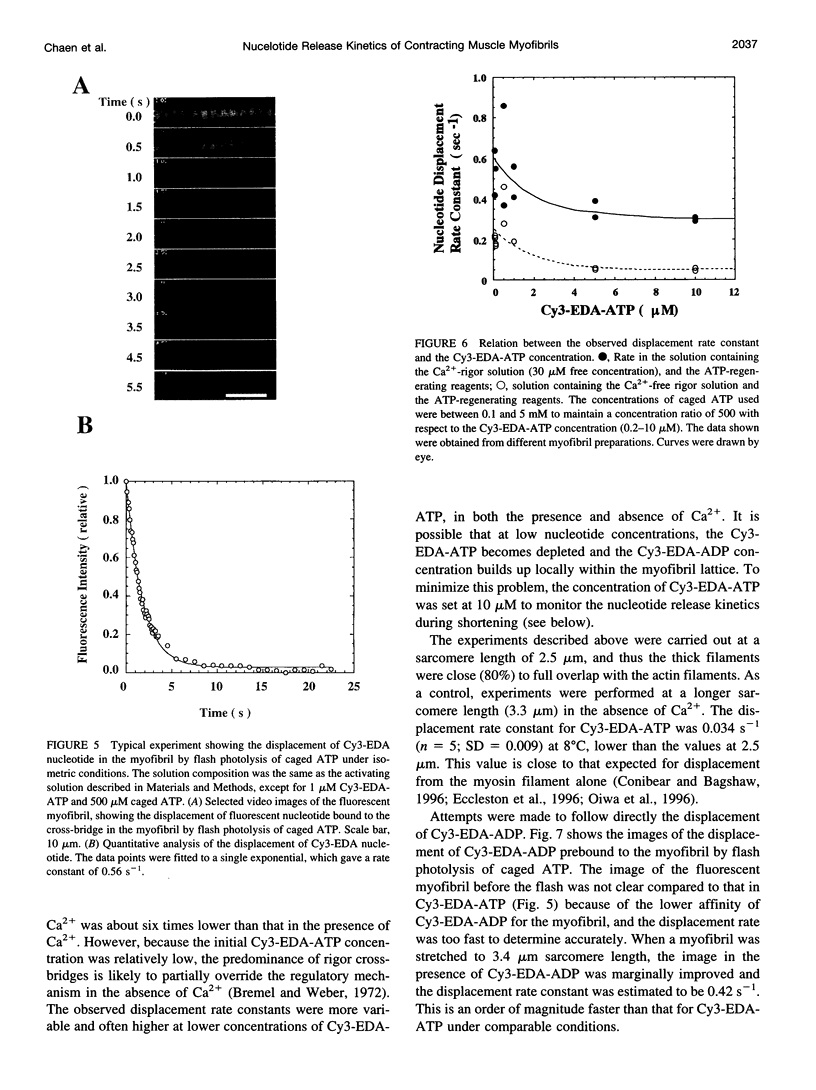
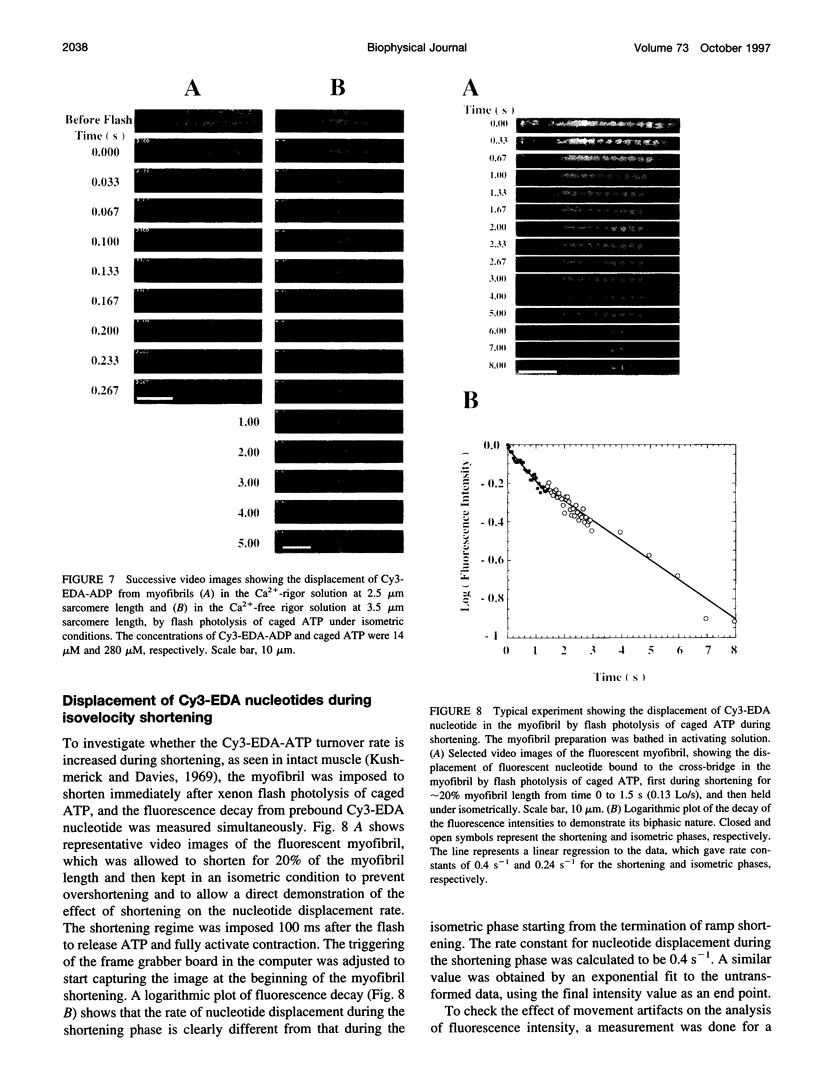
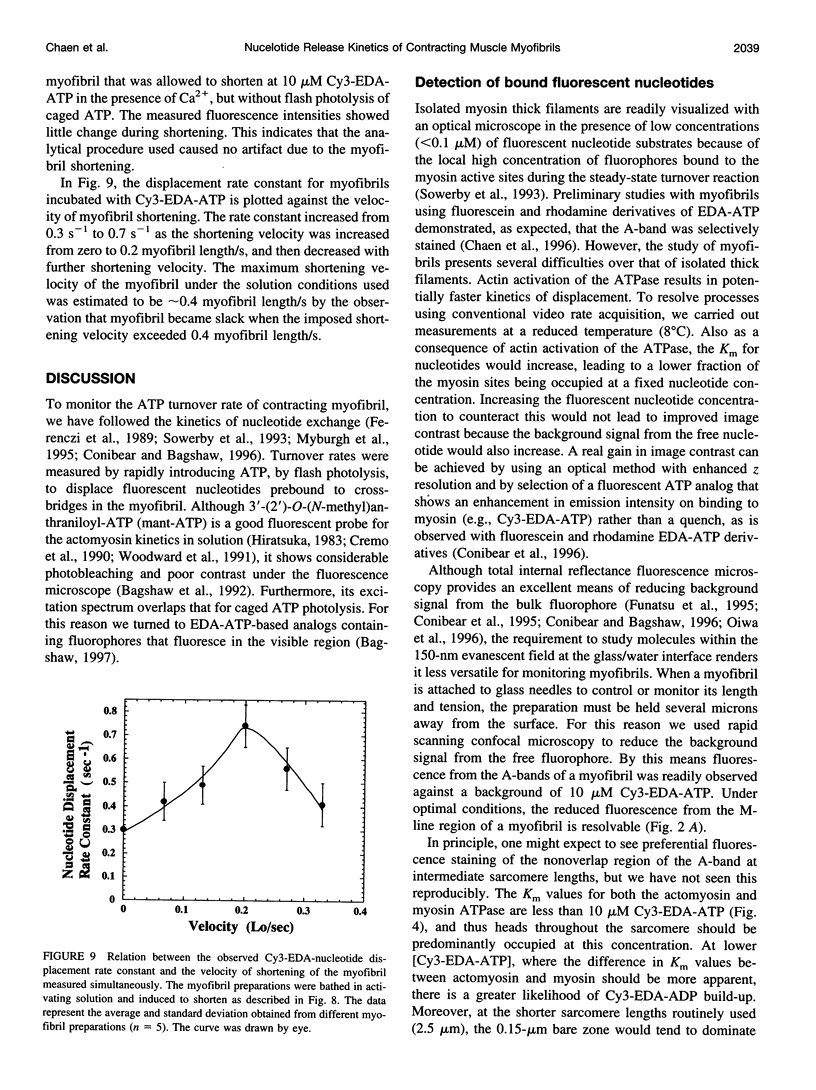
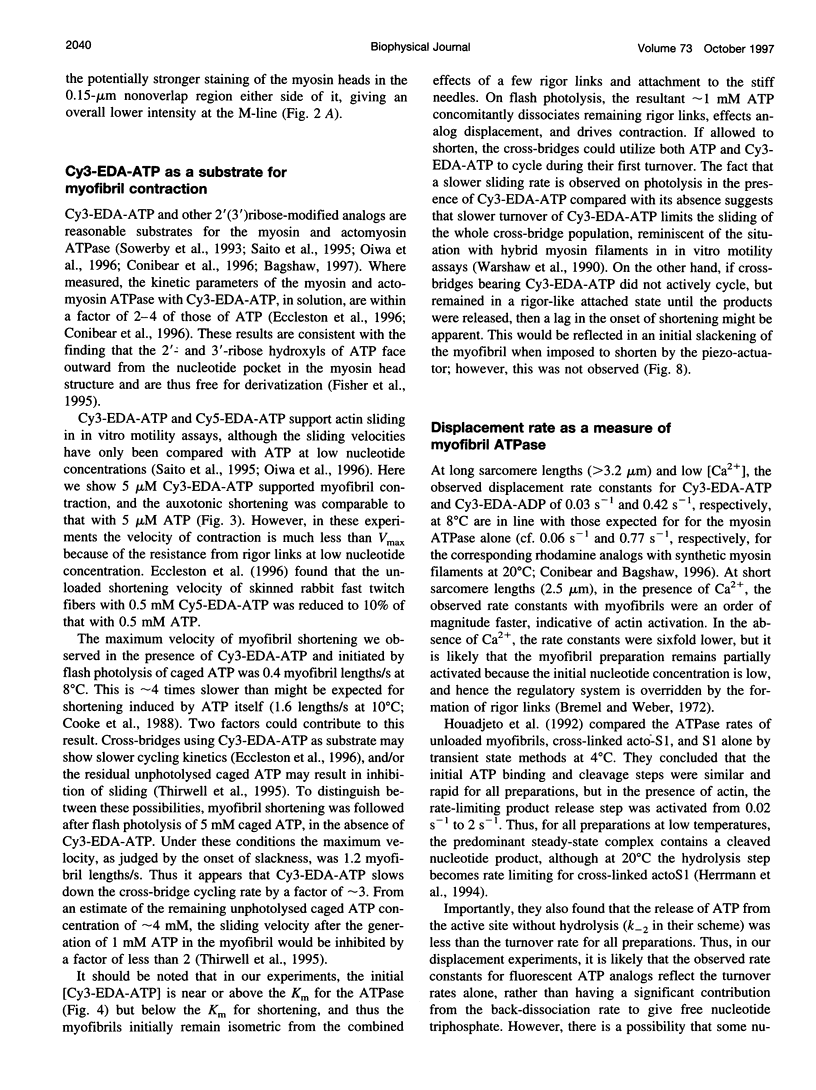
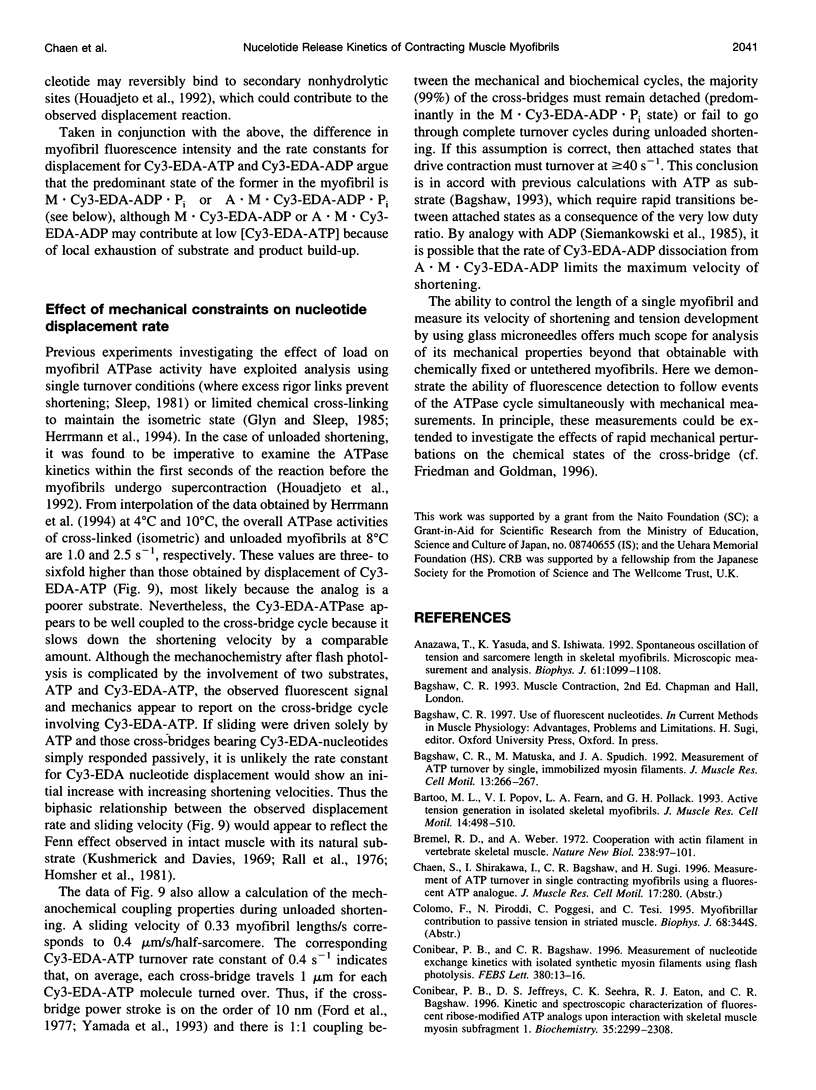
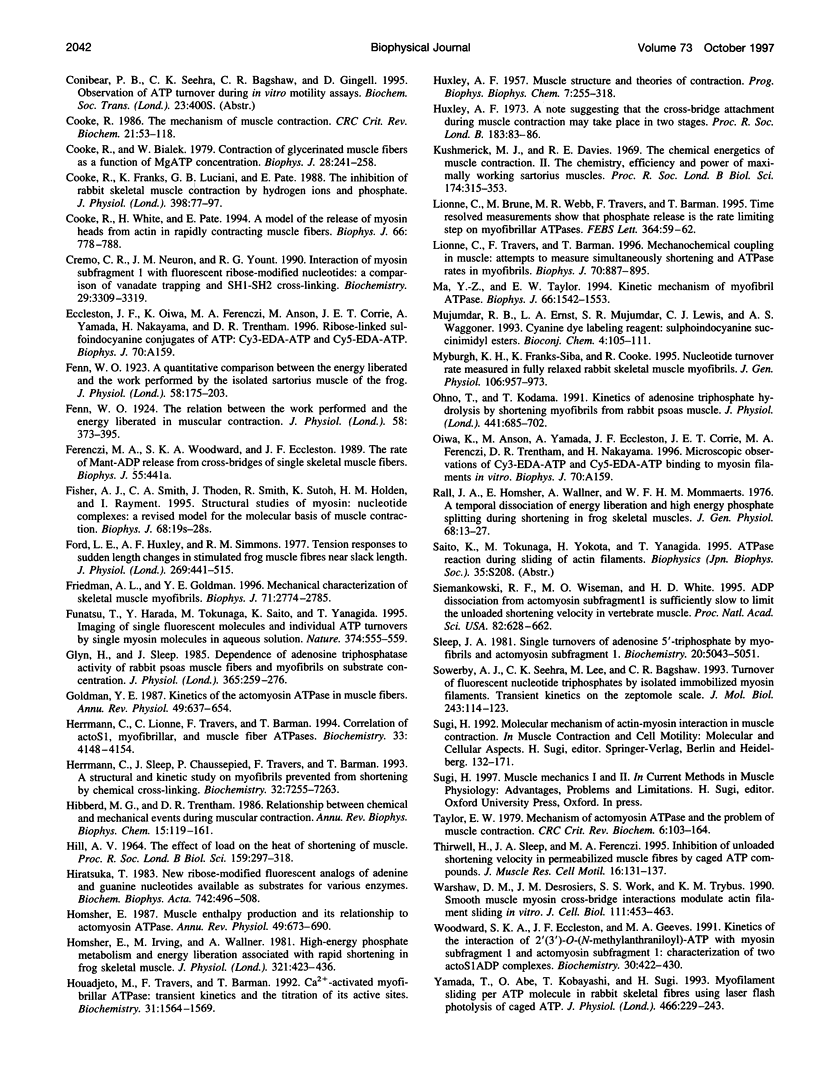
Images in this article
Selected References
These references are in PubMed. This may not be the complete list of references from this article.
- Anazawa T., Yasuda K., Ishiwata S. Spontaneous oscillation of tension and sarcomere length in skeletal myofibrils. Microscopic measurement and analysis. Biophys J. 1992 May;61(5):1099–1108. doi: 10.1016/S0006-3495(92)81919-7. [DOI] [PMC free article] [PubMed] [Google Scholar]
- Bartoo M. L., Popov V. I., Fearn L. A., Pollack G. H. Active tension generation in isolated skeletal myofibrils. J Muscle Res Cell Motil. 1993 Oct;14(5):498–510. doi: 10.1007/BF00297212. [DOI] [PubMed] [Google Scholar]
- Bremel R. D., Weber A. Cooperation within actin filament in vertebrate skeletal muscle. Nat New Biol. 1972 Jul 26;238(82):97–101. doi: 10.1038/newbio238097a0. [DOI] [PubMed] [Google Scholar]
- Conibear P. B., Bagshaw C. R. Measurement of nucleotide exchange kinetics with isolated synthetic myosin filaments using flash photolysis. FEBS Lett. 1996 Feb 12;380(1-2):13–16. doi: 10.1016/0014-5793(95)01538-8. [DOI] [PubMed] [Google Scholar]
- Conibear P. B., Jeffreys D. S., Seehra C. K., Eaton R. J., Bagshaw C. R. Kinetic and spectroscopic characterization of fluorescent ribose-modified ATP analogs upon interaction with skeletal muscle myosin subfragment 1. Biochemistry. 1996 Feb 20;35(7):2299–2308. doi: 10.1021/bi951824+. [DOI] [PubMed] [Google Scholar]
- Conibear P. B., Seehra C. K., Bagshaw C. R., Gingell D. Observation of ATP turnover during in vitro motility assays. Biochem Soc Trans. 1995 Aug;23(3):400S–400S. doi: 10.1042/bst023400s. [DOI] [PubMed] [Google Scholar]
- Cooke R., Bialek W. Contraction of glycerinated muscle fibers as a function of the ATP concentration. Biophys J. 1979 Nov;28(2):241–258. doi: 10.1016/S0006-3495(79)85174-7. [DOI] [PMC free article] [PubMed] [Google Scholar]
- Cooke R., Franks K., Luciani G. B., Pate E. The inhibition of rabbit skeletal muscle contraction by hydrogen ions and phosphate. J Physiol. 1988 Jan;395:77–97. doi: 10.1113/jphysiol.1988.sp016909. [DOI] [PMC free article] [PubMed] [Google Scholar]
- Cooke R. The mechanism of muscle contraction. CRC Crit Rev Biochem. 1986;21(1):53–118. doi: 10.3109/10409238609113609. [DOI] [PubMed] [Google Scholar]
- Cooke R., White H., Pate E. A model of the release of myosin heads from actin in rapidly contracting muscle fibers. Biophys J. 1994 Mar;66(3 Pt 1):778–788. doi: 10.1016/s0006-3495(94)80854-9. [DOI] [PMC free article] [PubMed] [Google Scholar]
- Cremo C. R., Neuron J. M., Yount R. G. Interaction of myosin subfragment 1 with fluorescent ribose-modified nucleotides. A comparison of vanadate trapping and SH1-SH2 cross-linking. Biochemistry. 1990 Apr 3;29(13):3309–3319. doi: 10.1021/bi00465a023. [DOI] [PubMed] [Google Scholar]
- Fenn W. O. A quantitative comparison between the energy liberated and the work performed by the isolated sartorius muscle of the frog. J Physiol. 1923 Dec 28;58(2-3):175–203. doi: 10.1113/jphysiol.1923.sp002115. [DOI] [PMC free article] [PubMed] [Google Scholar]
- Fenn W. O. The relation between the work performed and the energy liberated in muscular contraction. J Physiol. 1924 May 23;58(6):373–395. doi: 10.1113/jphysiol.1924.sp002141. [DOI] [PMC free article] [PubMed] [Google Scholar]
- Fisher A. J., Smith C. A., Thoden J., Smith R., Sutoh K., Holden H. M., Rayment I. Structural studies of myosin:nucleotide complexes: a revised model for the molecular basis of muscle contraction. Biophys J. 1995 Apr;68(4 Suppl):19S–28S. [PMC free article] [PubMed] [Google Scholar]
- Ford L. E., Huxley A. F., Simmons R. M. Tension responses to sudden length change in stimulated frog muscle fibres near slack length. J Physiol. 1977 Jul;269(2):441–515. doi: 10.1113/jphysiol.1977.sp011911. [DOI] [PMC free article] [PubMed] [Google Scholar]
- Friedman A. L., Goldman Y. E. Mechanical characterization of skeletal muscle myofibrils. Biophys J. 1996 Nov;71(5):2774–2785. doi: 10.1016/S0006-3495(96)79470-5. [DOI] [PMC free article] [PubMed] [Google Scholar]
- Funatsu T., Harada Y., Tokunaga M., Saito K., Yanagida T. Imaging of single fluorescent molecules and individual ATP turnovers by single myosin molecules in aqueous solution. Nature. 1995 Apr 6;374(6522):555–559. doi: 10.1038/374555a0. [DOI] [PubMed] [Google Scholar]
- Glyn H., Sleep J. Dependence of adenosine triphosphatase activity of rabbit psoas muscle fibres and myofibrils on substrate concentration. J Physiol. 1985 Aug;365:259–276. doi: 10.1113/jphysiol.1985.sp015770. [DOI] [PMC free article] [PubMed] [Google Scholar]
- Goldman Y. E. Kinetics of the actomyosin ATPase in muscle fibers. Annu Rev Physiol. 1987;49:637–654. doi: 10.1146/annurev.ph.49.030187.003225. [DOI] [PubMed] [Google Scholar]
- HILL A. V. THE EFFECT OF LOAD ON THE HEAT OF SHORTENING OF MUSCLE. Proc R Soc Lond B Biol Sci. 1964 Jan 14;159:297–318. doi: 10.1098/rspb.1964.0004. [DOI] [PubMed] [Google Scholar]
- HUXLEY A. F. Muscle structure and theories of contraction. Prog Biophys Biophys Chem. 1957;7:255–318. [PubMed] [Google Scholar]
- Herrmann C., Lionne C., Travers F., Barman T. Correlation of ActoS1, myofibrillar, and muscle fiber ATPases. Biochemistry. 1994 Apr 12;33(14):4148–4154. doi: 10.1021/bi00180a007. [DOI] [PubMed] [Google Scholar]
- Herrmann C., Sleep J., Chaussepied P., Travers F., Barman T. A structural and kinetic study on myofibrils prevented from shortening by chemical cross-linking. Biochemistry. 1993 Jul 20;32(28):7255–7263. doi: 10.1021/bi00079a023. [DOI] [PubMed] [Google Scholar]
- Hibberd M. G., Trentham D. R. Relationships between chemical and mechanical events during muscular contraction. Annu Rev Biophys Biophys Chem. 1986;15:119–161. doi: 10.1146/annurev.bb.15.060186.001003. [DOI] [PubMed] [Google Scholar]
- Hiratsuka T. New ribose-modified fluorescent analogs of adenine and guanine nucleotides available as substrates for various enzymes. Biochim Biophys Acta. 1983 Feb 15;742(3):496–508. doi: 10.1016/0167-4838(83)90267-4. [DOI] [PubMed] [Google Scholar]
- Homsher E., Irving M., Wallner A. High-energy phosphate metabolism and energy liberation associated with rapid shortening in frog skeletal muscle. J Physiol. 1981 Dec;321:423–436. doi: 10.1113/jphysiol.1981.sp013994. [DOI] [PMC free article] [PubMed] [Google Scholar]
- Homsher E. Muscle enthalpy production and its relationship to actomyosin ATPase. Annu Rev Physiol. 1987;49:673–690. doi: 10.1146/annurev.ph.49.030187.003325. [DOI] [PubMed] [Google Scholar]
- Houadjeto M., Travers F., Barman T. Ca(2+)-activated myofibrillar ATPase: transient kinetics and the titration of its active sites. Biochemistry. 1992 Feb 11;31(5):1564–1569. doi: 10.1021/bi00120a038. [DOI] [PubMed] [Google Scholar]
- Huxley A. F. A note suggesting that the cross-bridge attachment during muscle contraction may take place in two stages. Proc R Soc Lond B Biol Sci. 1973 Feb 27;183(1070):83–86. doi: 10.1098/rspb.1973.0006. [DOI] [PubMed] [Google Scholar]
- Kushmerick M. J., Davies R. E. The chemical energetics of muscle contraction. II. The chemistry, efficiency and power of maximally working sartorius muscles. Appendix. Free energy and enthalpy of atp hydrolysis in the sarcoplasm. Proc R Soc Lond B Biol Sci. 1969 Dec 23;174(1036):315–353. doi: 10.1098/rspb.1969.0096. [DOI] [PubMed] [Google Scholar]
- Lionne C., Brune M., Webb M. R., Travers F., Barman T. Time resolved measurements show that phosphate release is the rate limiting step on myofibrillar ATPases. FEBS Lett. 1995 May 1;364(1):59–62. doi: 10.1016/0014-5793(95)00356-e. [DOI] [PubMed] [Google Scholar]
- Lionne C., Travers F., Barman T. Mechanochemical coupling in muscle: attempts to measure simultaneously shortening and ATPase rates in myofibrils. Biophys J. 1996 Feb;70(2):887–895. doi: 10.1016/S0006-3495(96)79632-7. [DOI] [PMC free article] [PubMed] [Google Scholar]
- Ma Y. Z., Taylor E. W. Kinetic mechanism of myofibril ATPase. Biophys J. 1994 May;66(5):1542–1553. doi: 10.1016/S0006-3495(94)80945-2. [DOI] [PMC free article] [PubMed] [Google Scholar]
- Mujumdar R. B., Ernst L. A., Mujumdar S. R., Lewis C. J., Waggoner A. S. Cyanine dye labeling reagents: sulfoindocyanine succinimidyl esters. Bioconjug Chem. 1993 Mar-Apr;4(2):105–111. doi: 10.1021/bc00020a001. [DOI] [PubMed] [Google Scholar]
- Myburgh K. H., Franks-Skiba K., Cooke R. Nucleotide turnover rate measured in fully relaxed rabbit skeletal muscle myofibrils. J Gen Physiol. 1995 Nov;106(5):957–973. doi: 10.1085/jgp.106.5.957. [DOI] [PMC free article] [PubMed] [Google Scholar]
- Ohno T., Kodama T. Kinetics of adenosine triphosphate hydrolysis by shortening myofibrils from rabbit psoas muscle. J Physiol. 1991 Sep;441:685–702. doi: 10.1113/jphysiol.1991.sp018773. [DOI] [PMC free article] [PubMed] [Google Scholar]
- Rall J. A., Homsher E., Wallner A., Mommaerts W. F. A temporal dissociation of energy liberation and high energy phosphate splitting during shortening in frog skeletal muscles. J Gen Physiol. 1976 Jul;68(1):13–27. doi: 10.1085/jgp.68.1.13. [DOI] [PMC free article] [PubMed] [Google Scholar]
- Siemankowski R. F., Wiseman M. O., White H. D. ADP dissociation from actomyosin subfragment 1 is sufficiently slow to limit the unloaded shortening velocity in vertebrate muscle. Proc Natl Acad Sci U S A. 1985 Feb;82(3):658–662. doi: 10.1073/pnas.82.3.658. [DOI] [PMC free article] [PubMed] [Google Scholar]
- Sleep J. A. Single turnovers of adenosine 5'-triphosphate by myofibrils and actomyosin subfragment 1. Biochemistry. 1981 Aug 18;20(17):5043–5051. doi: 10.1021/bi00520a034. [DOI] [PubMed] [Google Scholar]
- Sowerby A. J., Seehra C. K., Lee M., Bagshaw C. R. Turnover of fluorescent nucleoside triphosphates by isolated immobilized myosin filaments. Transient kinetics on the zeptomole scale. J Mol Biol. 1993 Nov 5;234(1):114–123. doi: 10.1006/jmbi.1993.1567. [DOI] [PubMed] [Google Scholar]
- Taylor E. W. Mechanism of actomyosin ATPase and the problem of muscle contraction. CRC Crit Rev Biochem. 1979;6(2):103–164. doi: 10.3109/10409237909102562. [DOI] [PubMed] [Google Scholar]
- Thirlwell H., Sleep J. A., Ferenczi M. A. Inhibition of unloaded shortening velocity in permeabilized muscle fibres by caged ATP compounds. J Muscle Res Cell Motil. 1995 Apr;16(2):131–137. doi: 10.1007/BF00122531. [DOI] [PubMed] [Google Scholar]
- Warshaw D. M., Desrosiers J. M., Work S. S., Trybus K. M. Smooth muscle myosin cross-bridge interactions modulate actin filament sliding velocity in vitro. J Cell Biol. 1990 Aug;111(2):453–463. doi: 10.1083/jcb.111.2.453. [DOI] [PMC free article] [PubMed] [Google Scholar]
- Woodward S. K., Eccleston J. F., Geeves M. A. Kinetics of the interaction of 2'(3')-O-(N-methylanthraniloyl)-ATP with myosin subfragment 1 and actomyosin subfragment 1: characterization of two acto-S1-ADP complexes. Biochemistry. 1991 Jan 15;30(2):422–430. doi: 10.1021/bi00216a017. [DOI] [PubMed] [Google Scholar]
- Yamada T., Abe O., Kobayashi T., Sugi H. Myofilament sliding per ATP molecule in rabbit muscle fibres studied using laser flash photolysis of caged ATP. J Physiol. 1993 Jul;466:229–243. [PMC free article] [PubMed] [Google Scholar]