Abstract
Titin (also known as connectin) is a giant filamentous protein whose elastic properties greatly contribute to the passive force in muscle. In the sarcomere, the elastic I-band segment of titin may interact with the thin filaments, possibly affecting the molecule's elastic behavior. Indeed, several studies have indicated that interactions between titin and actin occur in vitro and may occur in the sarcomere as well. To explore the properties of titin alone, one must first eliminate the modulating effect of the thin filaments by selectively removing them. In the present work, thin filaments were selectively removed from the cardiac myocyte by using a gelsolin fragment. Partial extraction left behind approximately 100-nm-long thin filaments protruding from the Z-line, whereas the rest of the I-band became devoid of thin filaments, exposing titin. By applying a much more extensive gelsolin treatment, we also removed the remaining short thin filaments near the Z-line. After extraction, the extensibility of titin was studied by using immunoelectron microscopy, and the passive force-sarcomere length relation was determined by using mechanical techniques. Titin's regional extensibility was not detectably affected by partial thin-filament extraction. Passive force, on the other hand, was reduced at sarcomere lengths longer than approximately 2.1 microm, with a 33 +/- 9% reduction at 2.6 microm. After a complete extraction, the slack sarcomere length was reduced to approximately 1.7 microm. The segment of titin near the Z-line, which is otherwise inextensible, collapsed toward the Z-line in sarcomeres shorter than approximately 2.0 microm, but it was extended in sarcomeres longer than approximately 2.3 microm. Passive force became elevated at sarcomere lengths between approximately 1.7 and approximately 2.1 microm, but was reduced at sarcomere lengths of >2.3 microm. These changes can be accounted for by modeling titin as two wormlike chains in series, one of which increases its contour length by recruitment of the titin segment near the Z-line into the elastic pool.
Full text
PDF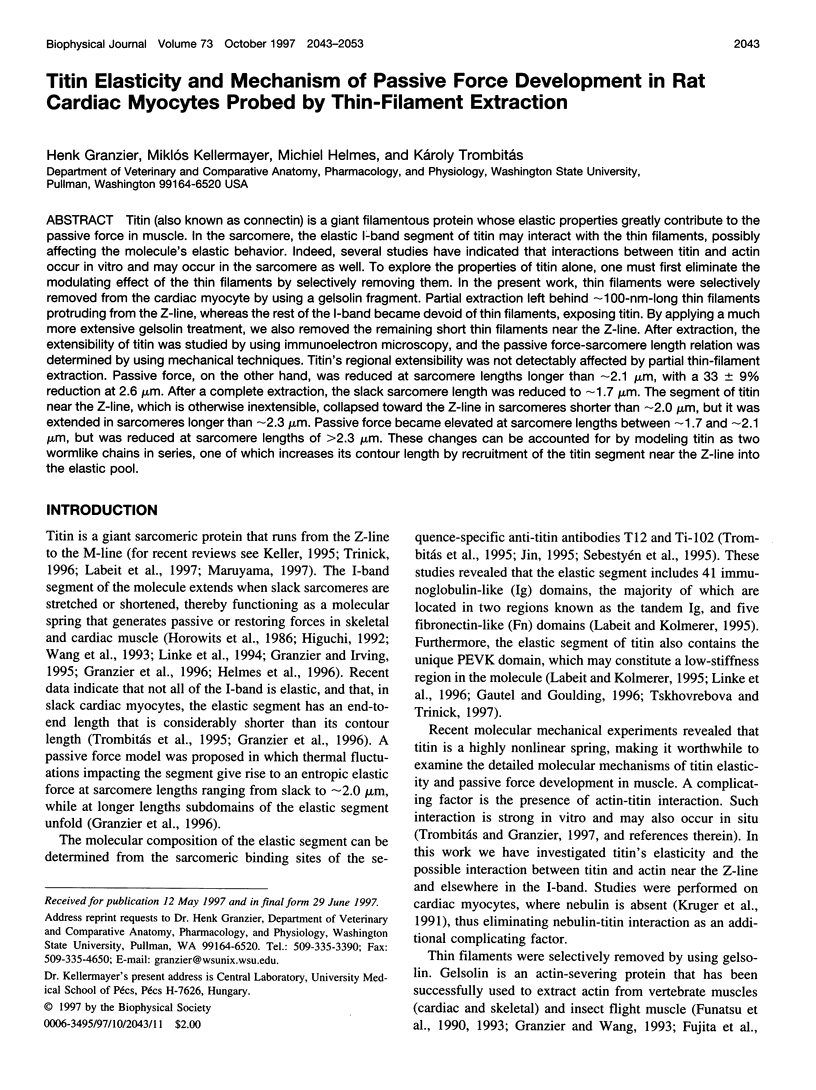
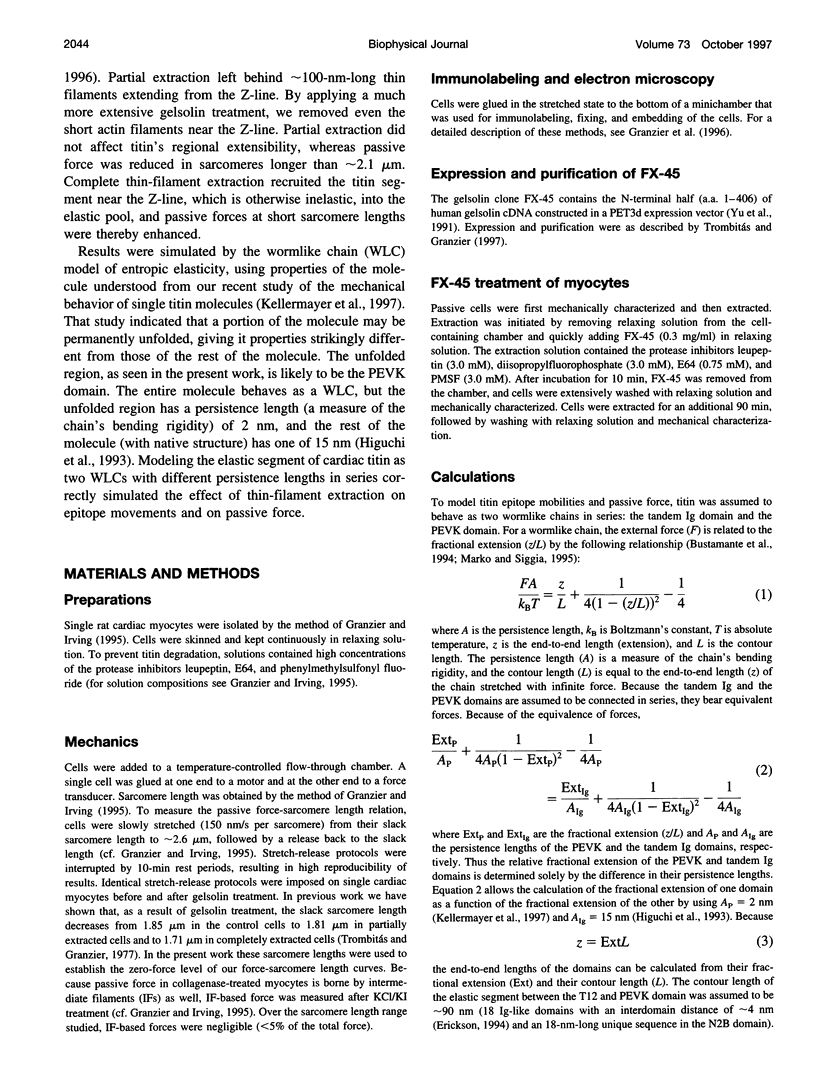
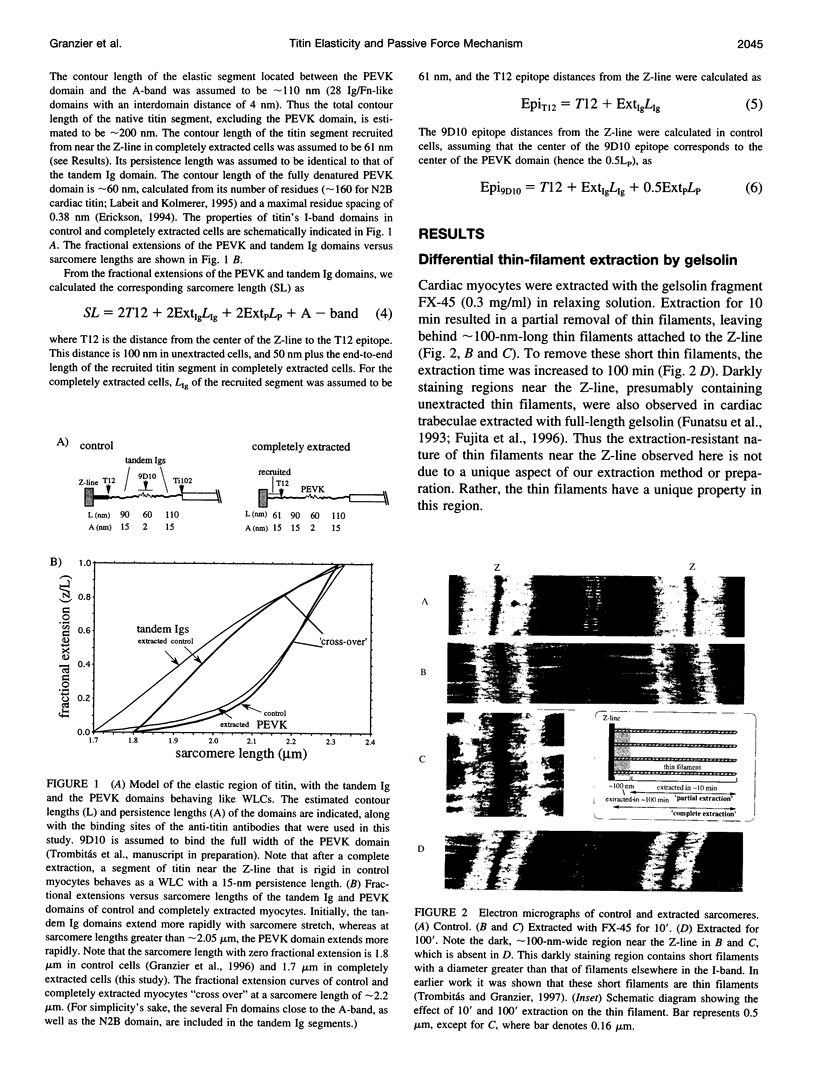
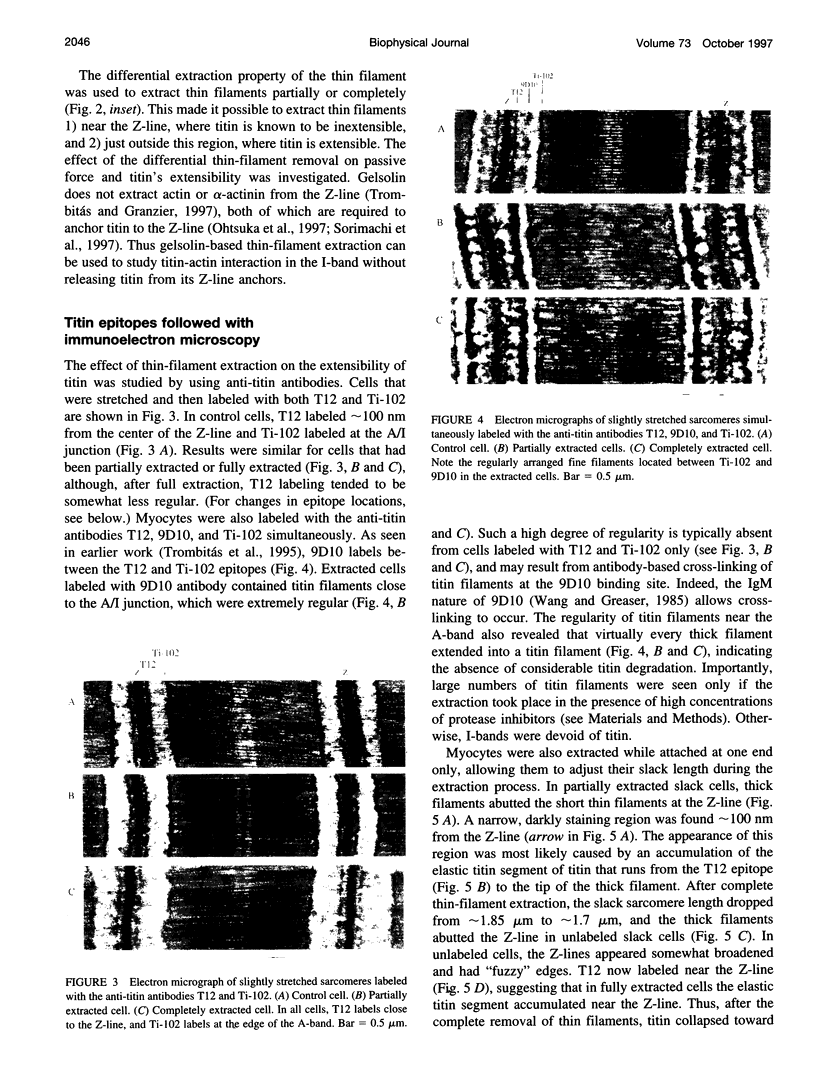
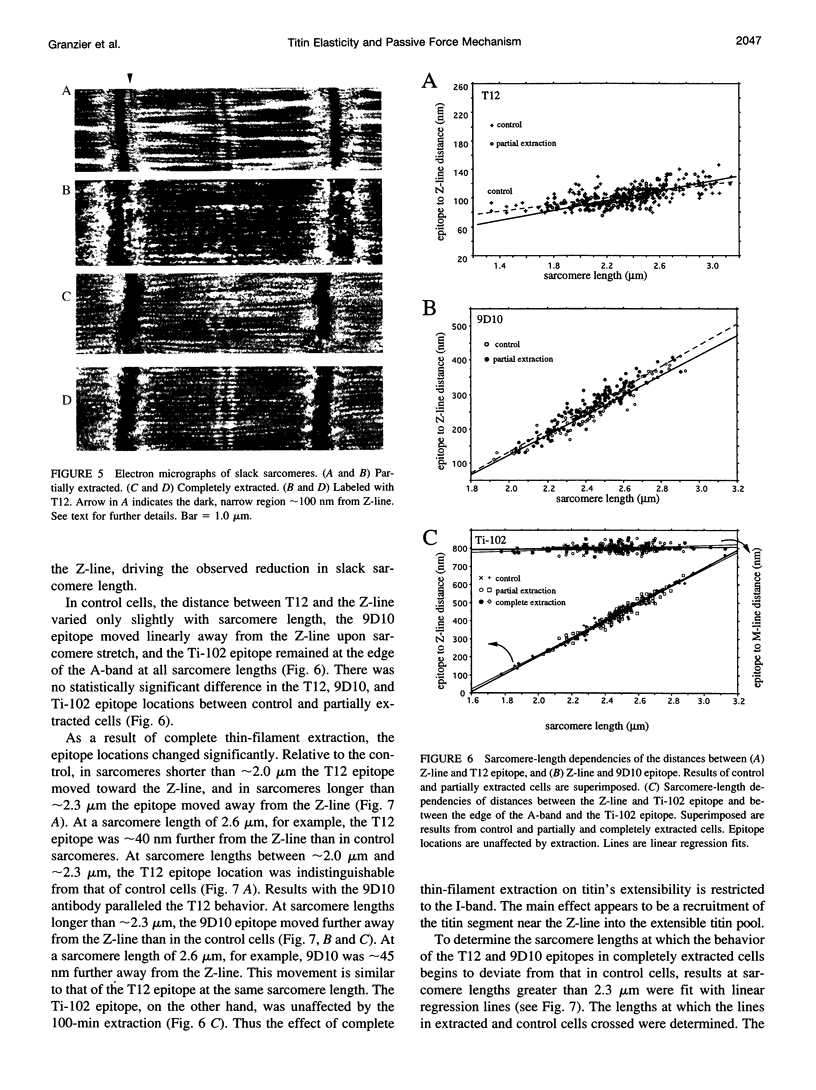
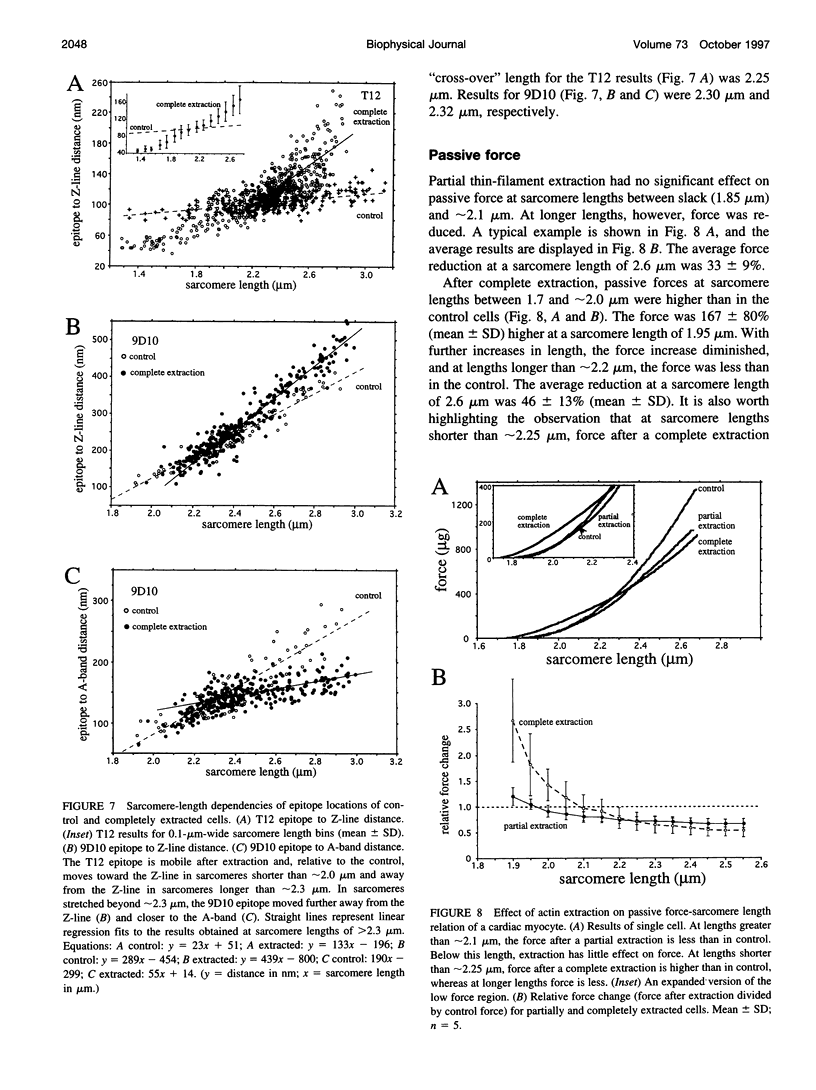
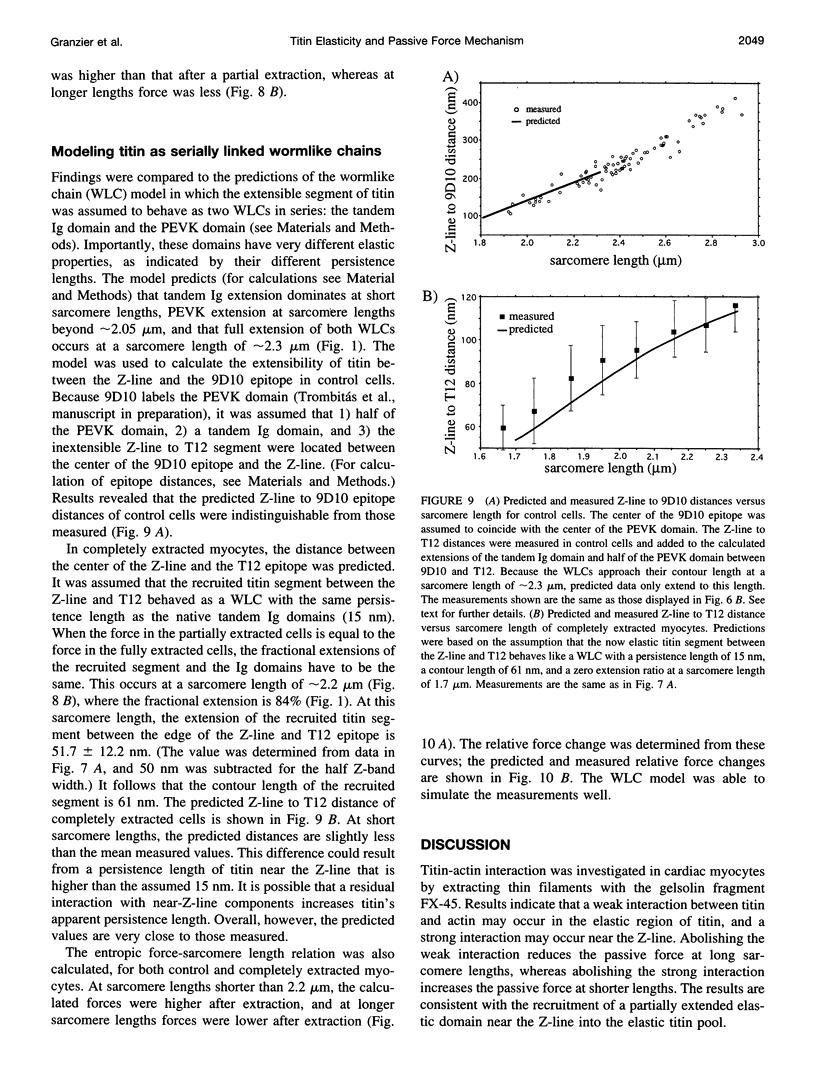
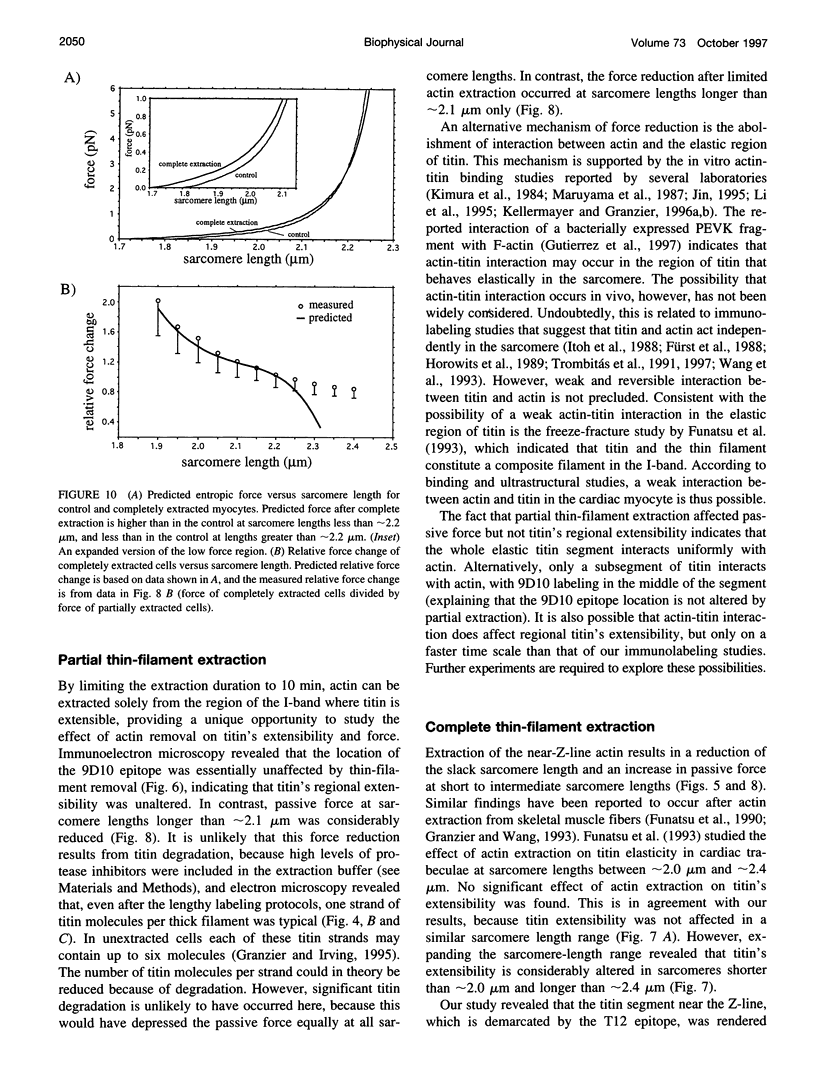
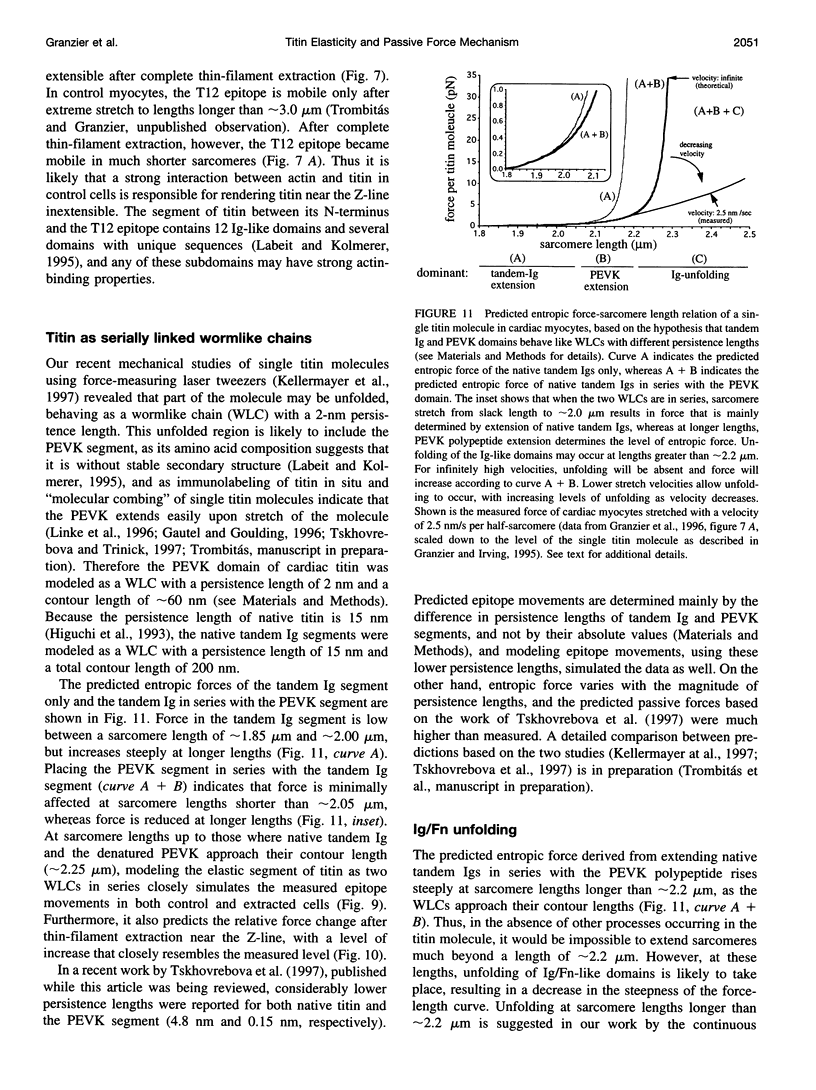
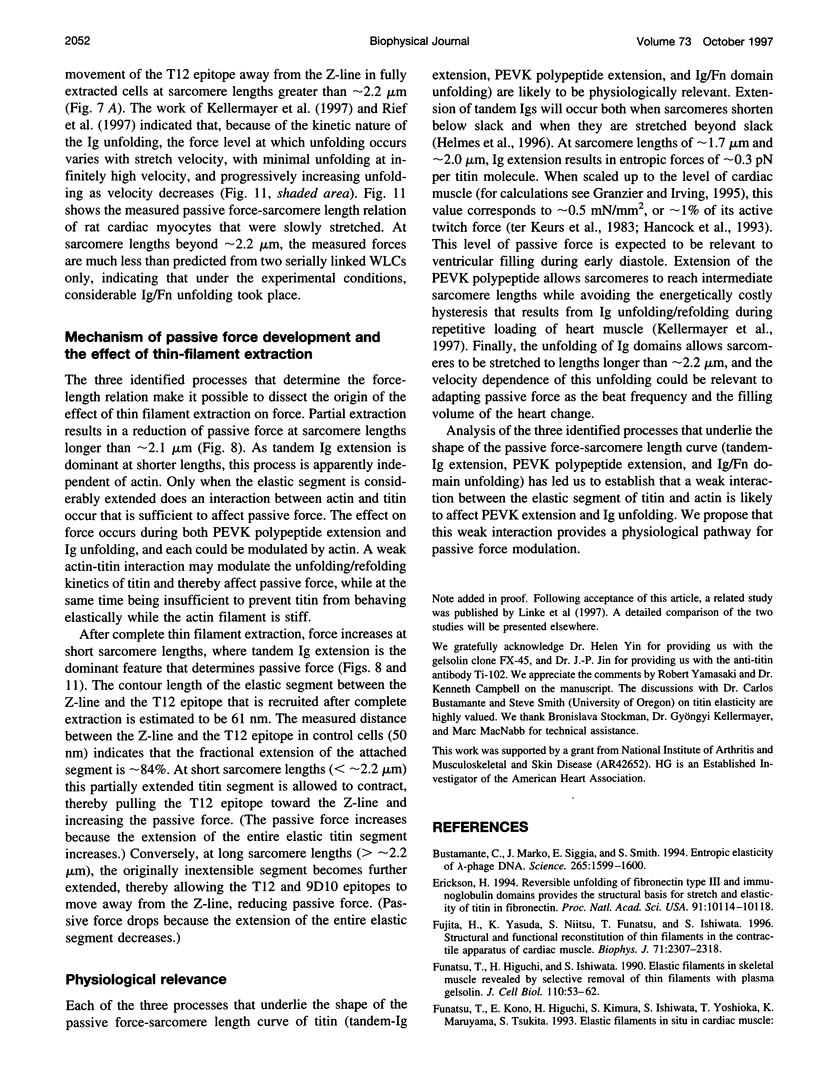
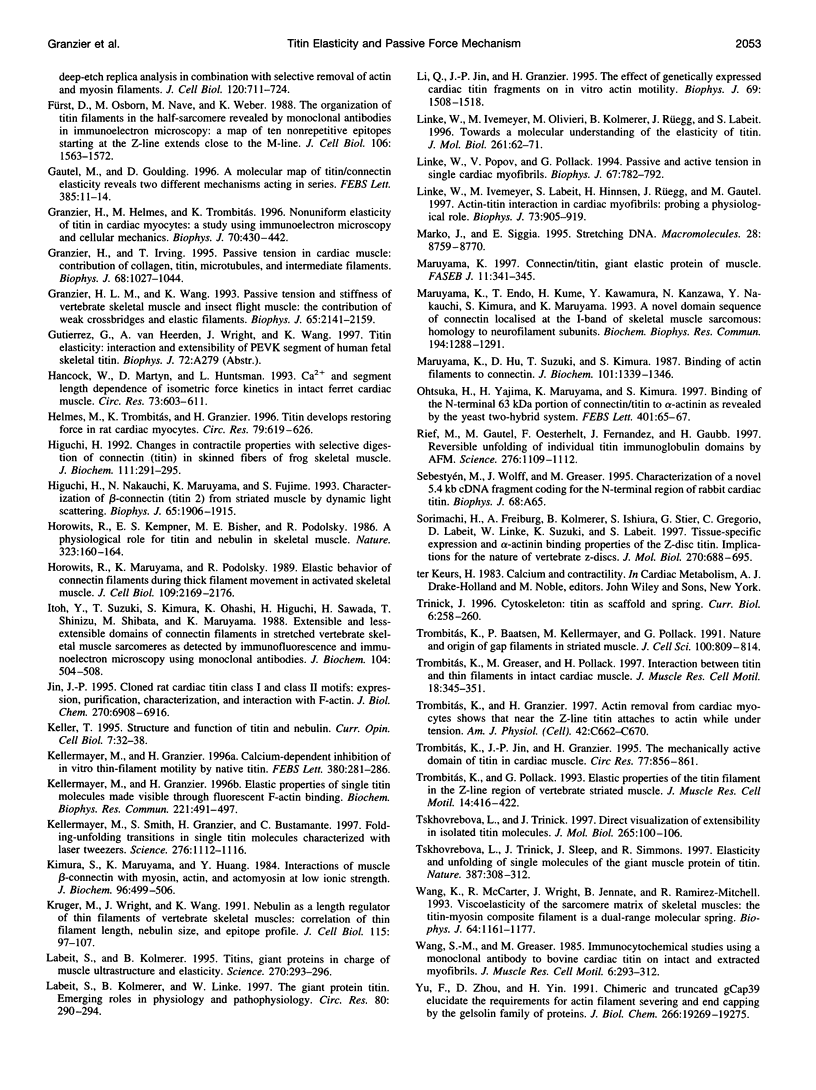
Images in this article
Selected References
These references are in PubMed. This may not be the complete list of references from this article.
- Bustamante C., Marko J. F., Siggia E. D., Smith S. Entropic elasticity of lambda-phage DNA. Science. 1994 Sep 9;265(5178):1599–1600. doi: 10.1126/science.8079175. [DOI] [PubMed] [Google Scholar]
- Erickson H. P. Reversible unfolding of fibronectin type III and immunoglobulin domains provides the structural basis for stretch and elasticity of titin and fibronectin. Proc Natl Acad Sci U S A. 1994 Oct 11;91(21):10114–10118. doi: 10.1073/pnas.91.21.10114. [DOI] [PMC free article] [PubMed] [Google Scholar]
- Fujita H., Yasuda K., Niitsu S., Funatsu T., Ishiwata S. Structural and functional reconstitution of thin filaments in the contractile apparatus of cardiac muscle. Biophys J. 1996 Nov;71(5):2307–2318. doi: 10.1016/S0006-3495(96)79465-1. [DOI] [PMC free article] [PubMed] [Google Scholar]
- Funatsu T., Higuchi H., Ishiwata S. Elastic filaments in skeletal muscle revealed by selective removal of thin filaments with plasma gelsolin. J Cell Biol. 1990 Jan;110(1):53–62. doi: 10.1083/jcb.110.1.53. [DOI] [PMC free article] [PubMed] [Google Scholar]
- Funatsu T., Kono E., Higuchi H., Kimura S., Ishiwata S., Yoshioka T., Maruyama K., Tsukita S. Elastic filaments in situ in cardiac muscle: deep-etch replica analysis in combination with selective removal of actin and myosin filaments. J Cell Biol. 1993 Feb;120(3):711–724. doi: 10.1083/jcb.120.3.711. [DOI] [PMC free article] [PubMed] [Google Scholar]
- Fürst D. O., Osborn M., Nave R., Weber K. The organization of titin filaments in the half-sarcomere revealed by monoclonal antibodies in immunoelectron microscopy: a map of ten nonrepetitive epitopes starting at the Z line extends close to the M line. J Cell Biol. 1988 May;106(5):1563–1572. doi: 10.1083/jcb.106.5.1563. [DOI] [PMC free article] [PubMed] [Google Scholar]
- Gautel M., Goulding D. A molecular map of titin/connectin elasticity reveals two different mechanisms acting in series. FEBS Lett. 1996 Apr 29;385(1-2):11–14. doi: 10.1016/0014-5793(96)00338-9. [DOI] [PubMed] [Google Scholar]
- Granzier H. L., Irving T. C. Passive tension in cardiac muscle: contribution of collagen, titin, microtubules, and intermediate filaments. Biophys J. 1995 Mar;68(3):1027–1044. doi: 10.1016/S0006-3495(95)80278-X. [DOI] [PMC free article] [PubMed] [Google Scholar]
- Granzier H. L., Wang K. Passive tension and stiffness of vertebrate skeletal and insect flight muscles: the contribution of weak cross-bridges and elastic filaments. Biophys J. 1993 Nov;65(5):2141–2159. doi: 10.1016/S0006-3495(93)81262-1. [DOI] [PMC free article] [PubMed] [Google Scholar]
- Granzier H., Helmes M., Trombitás K. Nonuniform elasticity of titin in cardiac myocytes: a study using immunoelectron microscopy and cellular mechanics. Biophys J. 1996 Jan;70(1):430–442. doi: 10.1016/S0006-3495(96)79586-3. [DOI] [PMC free article] [PubMed] [Google Scholar]
- Hancock W. O., Martyn D. A., Huntsman L. L. Ca2+ and segment length dependence of isometric force kinetics in intact ferret cardiac muscle. Circ Res. 1993 Oct;73(4):603–611. doi: 10.1161/01.res.73.4.603. [DOI] [PubMed] [Google Scholar]
- Helmes M., Trombitás K., Granzier H. Titin develops restoring force in rat cardiac myocytes. Circ Res. 1996 Sep;79(3):619–626. doi: 10.1161/01.res.79.3.619. [DOI] [PubMed] [Google Scholar]
- Higuchi H. Changes in contractile properties with selective digestion of connectin (titin) in skinned fibers of frog skeletal muscle. J Biochem. 1992 Mar;111(3):291–295. doi: 10.1093/oxfordjournals.jbchem.a123752. [DOI] [PubMed] [Google Scholar]
- Higuchi H., Nakauchi Y., Maruyama K., Fujime S. Characterization of beta-connectin (titin 2) from striated muscle by dynamic light scattering. Biophys J. 1993 Nov;65(5):1906–1915. doi: 10.1016/S0006-3495(93)81261-X. [DOI] [PMC free article] [PubMed] [Google Scholar]
- Horowits R., Kempner E. S., Bisher M. E., Podolsky R. J. A physiological role for titin and nebulin in skeletal muscle. Nature. 1986 Sep 11;323(6084):160–164. doi: 10.1038/323160a0. [DOI] [PubMed] [Google Scholar]
- Horowits R., Maruyama K., Podolsky R. J. Elastic behavior of connectin filaments during thick filament movement in activated skeletal muscle. J Cell Biol. 1989 Nov;109(5):2169–2176. doi: 10.1083/jcb.109.5.2169. [DOI] [PMC free article] [PubMed] [Google Scholar]
- Itoh Y., Suzuki T., Kimura S., Ohashi K., Higuchi H., Sawada H., Shimizu T., Shibata M., Maruyama K. Extensible and less-extensible domains of connectin filaments in stretched vertebrate skeletal muscle sarcomeres as detected by immunofluorescence and immunoelectron microscopy using monoclonal antibodies. J Biochem. 1988 Oct;104(4):504–508. doi: 10.1093/oxfordjournals.jbchem.a122499. [DOI] [PubMed] [Google Scholar]
- Jin J. P. Cloned rat cardiac titin class I and class II motifs. Expression, purification, characterization, and interaction with F-actin. J Biol Chem. 1995 Mar 24;270(12):6908–6916. [PubMed] [Google Scholar]
- Keller T. C., 3rd Structure and function of titin and nebulin. Curr Opin Cell Biol. 1995 Feb;7(1):32–38. doi: 10.1016/0955-0674(95)80042-5. [DOI] [PubMed] [Google Scholar]
- Kellermayer M. S., Granzier H. L. Calcium-dependent inhibition of in vitro thin-filament motility by native titin. FEBS Lett. 1996 Feb 19;380(3):281–286. doi: 10.1016/0014-5793(96)00055-5. [DOI] [PubMed] [Google Scholar]
- Kellermayer M. S., Granzier H. L. Elastic properties of single titin molecules made visible through fluorescent F-actin binding. Biochem Biophys Res Commun. 1996 Apr 25;221(3):491–497. doi: 10.1006/bbrc.1996.0624. [DOI] [PubMed] [Google Scholar]
- Kellermayer M. S., Smith S. B., Granzier H. L., Bustamante C. Folding-unfolding transitions in single titin molecules characterized with laser tweezers. Science. 1997 May 16;276(5315):1112–1116. doi: 10.1126/science.276.5315.1112. [DOI] [PubMed] [Google Scholar]
- Kimura S., Maruyama K., Huang Y. P. Interactions of muscle beta-connectin with myosin, actin, and actomyosin at low ionic strengths. J Biochem. 1984 Aug;96(2):499–506. doi: 10.1093/oxfordjournals.jbchem.a134862. [DOI] [PubMed] [Google Scholar]
- Kruger M., Wright J., Wang K. Nebulin as a length regulator of thin filaments of vertebrate skeletal muscles: correlation of thin filament length, nebulin size, and epitope profile. J Cell Biol. 1991 Oct;115(1):97–107. doi: 10.1083/jcb.115.1.97. [DOI] [PMC free article] [PubMed] [Google Scholar]
- Labeit S., Kolmerer B., Linke W. A. The giant protein titin. Emerging roles in physiology and pathophysiology. Circ Res. 1997 Feb;80(2):290–294. doi: 10.1161/01.res.80.2.290. [DOI] [PubMed] [Google Scholar]
- Labeit S., Kolmerer B. Titins: giant proteins in charge of muscle ultrastructure and elasticity. Science. 1995 Oct 13;270(5234):293–296. doi: 10.1126/science.270.5234.293. [DOI] [PubMed] [Google Scholar]
- Li Q., Jin J. P., Granzier H. L. The effect of genetically expressed cardiac titin fragments on in vitro actin motility. Biophys J. 1995 Oct;69(4):1508–1518. doi: 10.1016/S0006-3495(95)80021-4. [DOI] [PMC free article] [PubMed] [Google Scholar]
- Linke W. A., Ivemeyer M., Labeit S., Hinssen H., Rüegg J. C., Gautel M. Actin-titin interaction in cardiac myofibrils: probing a physiological role. Biophys J. 1997 Aug;73(2):905–919. doi: 10.1016/S0006-3495(97)78123-2. [DOI] [PMC free article] [PubMed] [Google Scholar]
- Linke W. A., Ivemeyer M., Olivieri N., Kolmerer B., Rüegg J. C., Labeit S. Towards a molecular understanding of the elasticity of titin. J Mol Biol. 1996 Aug 9;261(1):62–71. doi: 10.1006/jmbi.1996.0441. [DOI] [PubMed] [Google Scholar]
- Linke W. A., Popov V. I., Pollack G. H. Passive and active tension in single cardiac myofibrils. Biophys J. 1994 Aug;67(2):782–792. doi: 10.1016/S0006-3495(94)80538-7. [DOI] [PMC free article] [PubMed] [Google Scholar]
- Maruyama K. Connectin/titin, giant elastic protein of muscle. FASEB J. 1997 Apr;11(5):341–345. doi: 10.1096/fasebj.11.5.9141500. [DOI] [PubMed] [Google Scholar]
- Maruyama K., Endo T., Kume H., Kawamura Y., Kanzawa N., Nakauchi Y., Kimura S., Kawashima S., Maruyama K. A novel domain sequence of connectin localized at the I band of skeletal muscle sarcomeres: homology to neurofilament subunits. Biochem Biophys Res Commun. 1993 Aug 16;194(3):1288–1291. doi: 10.1006/bbrc.1993.1963. [DOI] [PubMed] [Google Scholar]
- Maruyama K., Hu D. H., Suzuki T., Kimura S. Binding of actin filaments to connectin. J Biochem. 1987 Jun;101(6):1339–1346. doi: 10.1093/oxfordjournals.jbchem.a122001. [DOI] [PubMed] [Google Scholar]
- Ohtsuka H., Yajima H., Maruyama K., Kimura S. Binding of the N-terminal 63 kDa portion of connectin/titin to alpha-actinin as revealed by the yeast two-hybrid system. FEBS Lett. 1997 Jan 13;401(1):65–67. doi: 10.1016/s0014-5793(96)01432-9. [DOI] [PubMed] [Google Scholar]
- Rief M., Gautel M., Oesterhelt F., Fernandez J. M., Gaub H. E. Reversible unfolding of individual titin immunoglobulin domains by AFM. Science. 1997 May 16;276(5315):1109–1112. doi: 10.1126/science.276.5315.1109. [DOI] [PubMed] [Google Scholar]
- Sorimachi H., Freiburg A., Kolmerer B., Ishiura S., Stier G., Gregorio C. C., Labeit D., Linke W. A., Suzuki K., Labeit S. Tissue-specific expression and alpha-actinin binding properties of the Z-disc titin: implications for the nature of vertebrate Z-discs. J Mol Biol. 1997 Aug 1;270(5):688–695. doi: 10.1006/jmbi.1997.1145. [DOI] [PubMed] [Google Scholar]
- Trinick J. Titin as a scaffold and spring. Cytoskeleton. Curr Biol. 1996 Mar 1;6(3):258–260. doi: 10.1016/s0960-9822(02)00472-4. [DOI] [PubMed] [Google Scholar]
- Trombitás K., Baatsen P. H., Kellermayer M. S., Pollack G. H. Nature and origin of gap filaments in striated muscle. J Cell Sci. 1991 Dec;100(Pt 4):809–814. doi: 10.1242/jcs.100.4.809. [DOI] [PubMed] [Google Scholar]
- Trombitás K., Granzier H. Actin removal from cardiac myocytes shows that near Z line titin attaches to actin while under tension. Am J Physiol. 1997 Aug;273(2 Pt 1):C662–C670. doi: 10.1152/ajpcell.1997.273.2.C662. [DOI] [PubMed] [Google Scholar]
- Trombitás K., Greaser M. L., Pollack G. H. Interaction between titin and thin filaments in intact cardiac muscle. J Muscle Res Cell Motil. 1997 Jun;18(3):345–351. doi: 10.1023/a:1018626210300. [DOI] [PubMed] [Google Scholar]
- Trombitás K., Jin J. P., Granzier H. The mechanically active domain of titin in cardiac muscle. Circ Res. 1995 Oct;77(4):856–861. doi: 10.1161/01.res.77.4.856. [DOI] [PubMed] [Google Scholar]
- Trombitás K., Pollack G. H. Elastic properties of the titin filament in the Z-line region of vertebrate striated muscle. J Muscle Res Cell Motil. 1993 Aug;14(4):416–422. doi: 10.1007/BF00121293. [DOI] [PubMed] [Google Scholar]
- Tskhovrebova L., Trinick J. Direct visualization of extensibility in isolated titin molecules. J Mol Biol. 1997 Jan 17;265(2):100–106. doi: 10.1006/jmbi.1996.0717. [DOI] [PubMed] [Google Scholar]
- Tskhovrebova L., Trinick J., Sleep J. A., Simmons R. M. Elasticity and unfolding of single molecules of the giant muscle protein titin. Nature. 1997 May 15;387(6630):308–312. doi: 10.1038/387308a0. [DOI] [PubMed] [Google Scholar]
- Wang K., McCarter R., Wright J., Beverly J., Ramirez-Mitchell R. Viscoelasticity of the sarcomere matrix of skeletal muscles. The titin-myosin composite filament is a dual-stage molecular spring. Biophys J. 1993 Apr;64(4):1161–1177. doi: 10.1016/S0006-3495(93)81482-6. [DOI] [PMC free article] [PubMed] [Google Scholar]
- Wang S. M., Greaser M. L. Immunocytochemical studies using a monoclonal antibody to bovine cardiac titin on intact and extracted myofibrils. J Muscle Res Cell Motil. 1985 Jun;6(3):293–312. doi: 10.1007/BF00713171. [DOI] [PubMed] [Google Scholar]
- Yu F. X., Zhou D. M., Yin H. L. Chimeric and truncated gCap39 elucidate the requirements for actin filament severing and end capping by the gelsolin family of proteins. J Biol Chem. 1991 Oct 15;266(29):19269–19275. [PubMed] [Google Scholar]