Abstract
We present a theory for estimation of the dendritic electrotonic length constant and the membrane time constant from the transmembrane potential (TMP) induced by an applied electric field. The theory is adapted to morphologically defined neurons with homogeneous passive electric properties. Frequency characteristics and transients at the onset and offset of the DC field are considered. Two relations are useful for estimating the electrotonic parameters: 1) steady-state polarization versus the dendritic electrotonic length constant; 2) membrane time constant versus length constant. These relations are monotonic and may provide a unique estimate of the electrotonic parameters for 3D-reconstructed neurons. Equivalent tip-to-tip electrotonic length of the dendritic tree was estimated by measuring the equalization time of the field-induced TMP. For 11 turtle spinal motoneurons, the electrotonic length from tip to tip of the dendrites was in the range of 1-2.5 lambda, whereas classical estimation using injection of current pulses gave an average dendrite length of 0.9-1.1 lambda. For seven ventral horn interneurons, the estimates were 0.7-2.6 lambda and 0.6-0.9 lambda, respectively. The measurements of the field-induced polarization promise to be a useful addition to the conventional methods using microelectrode stimulation.
Full text
PDF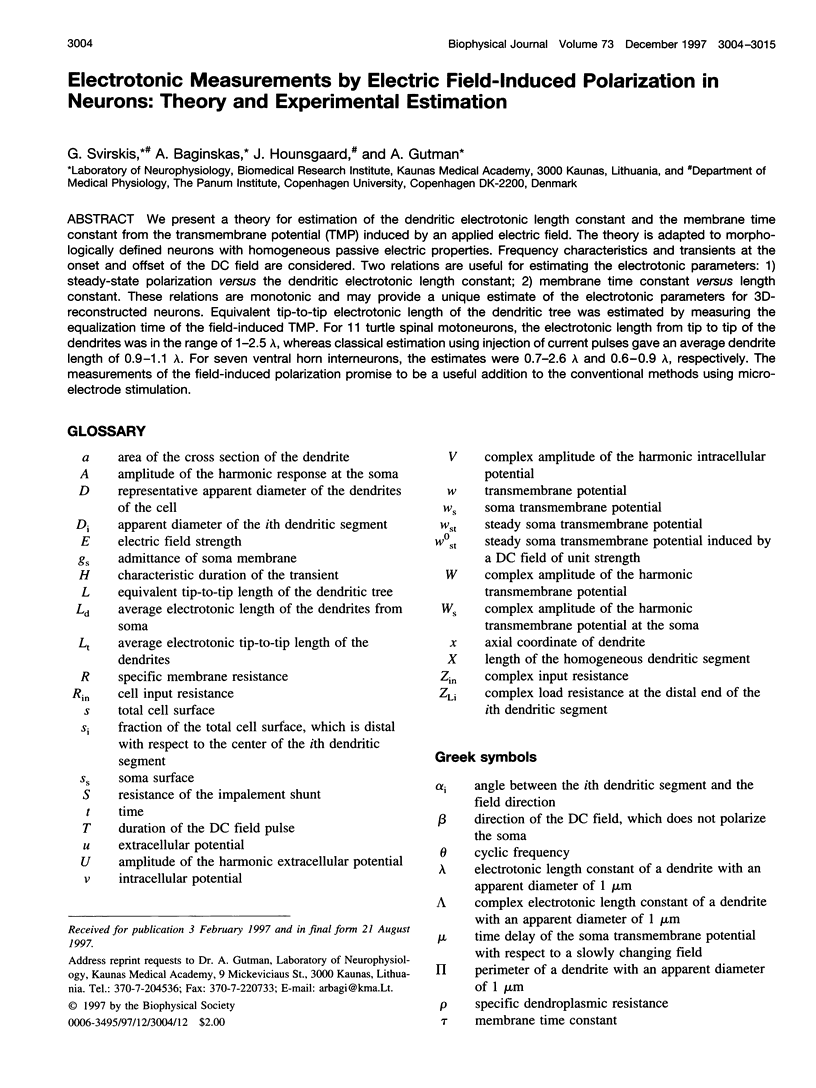
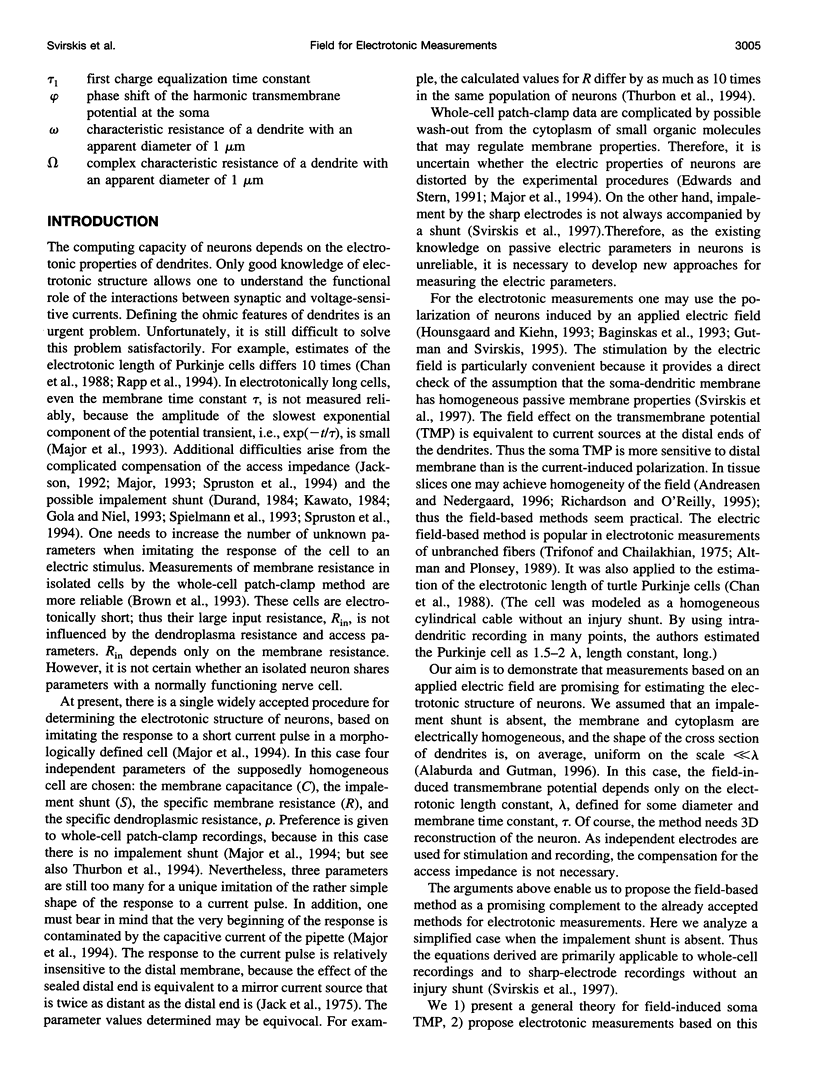
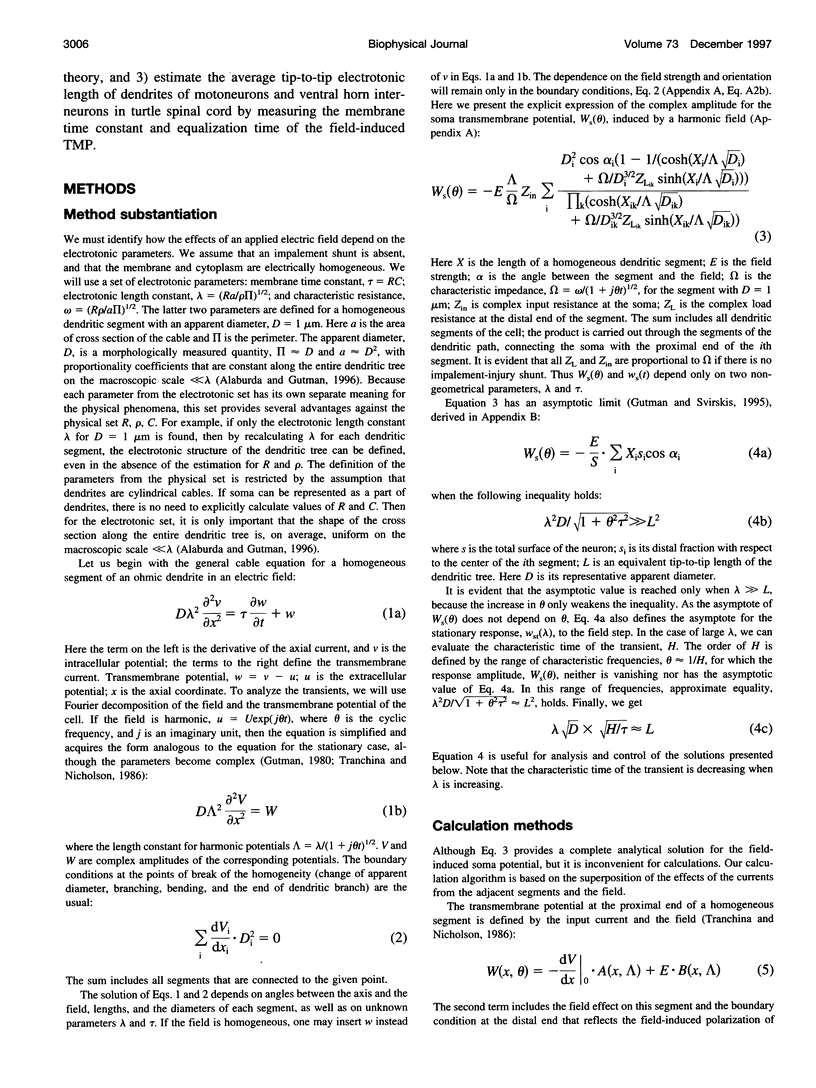
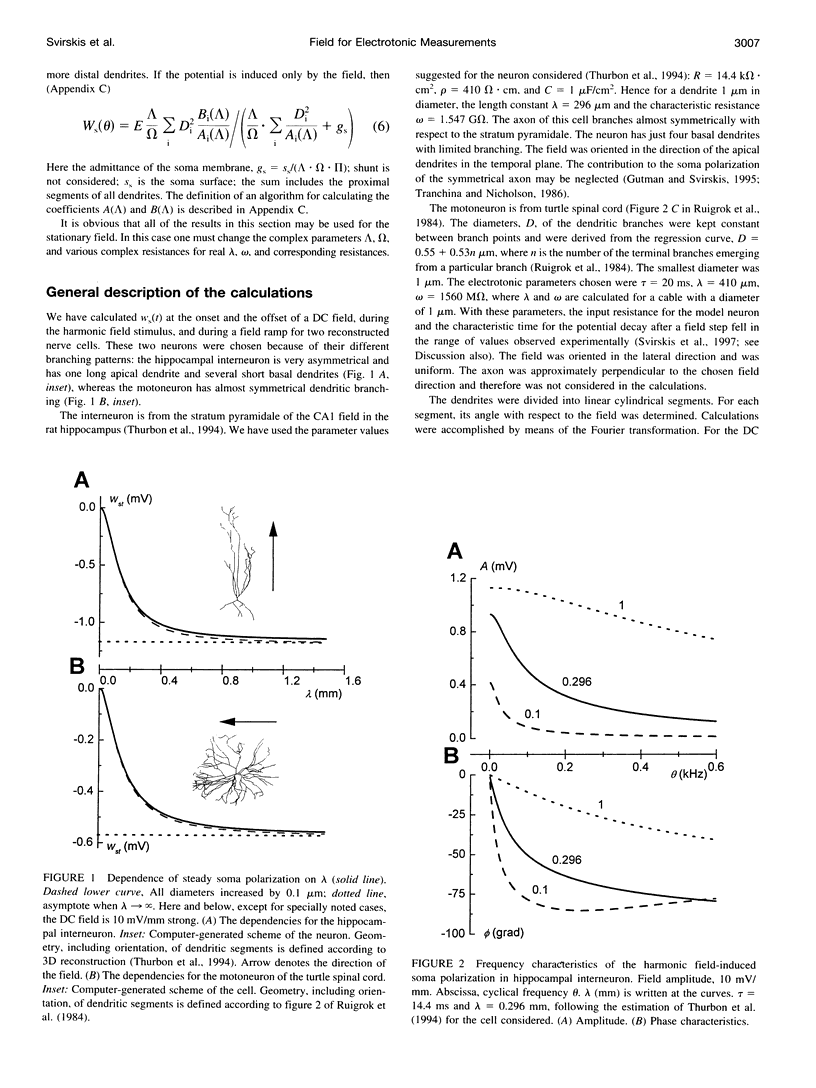
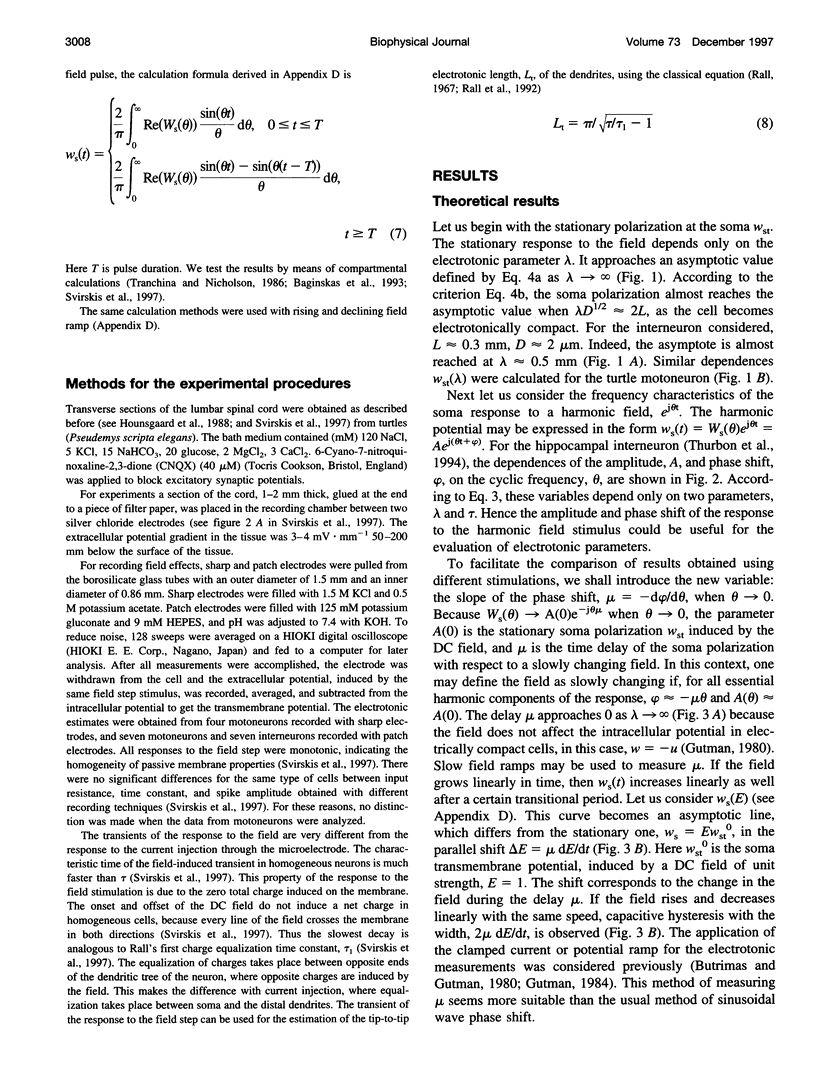
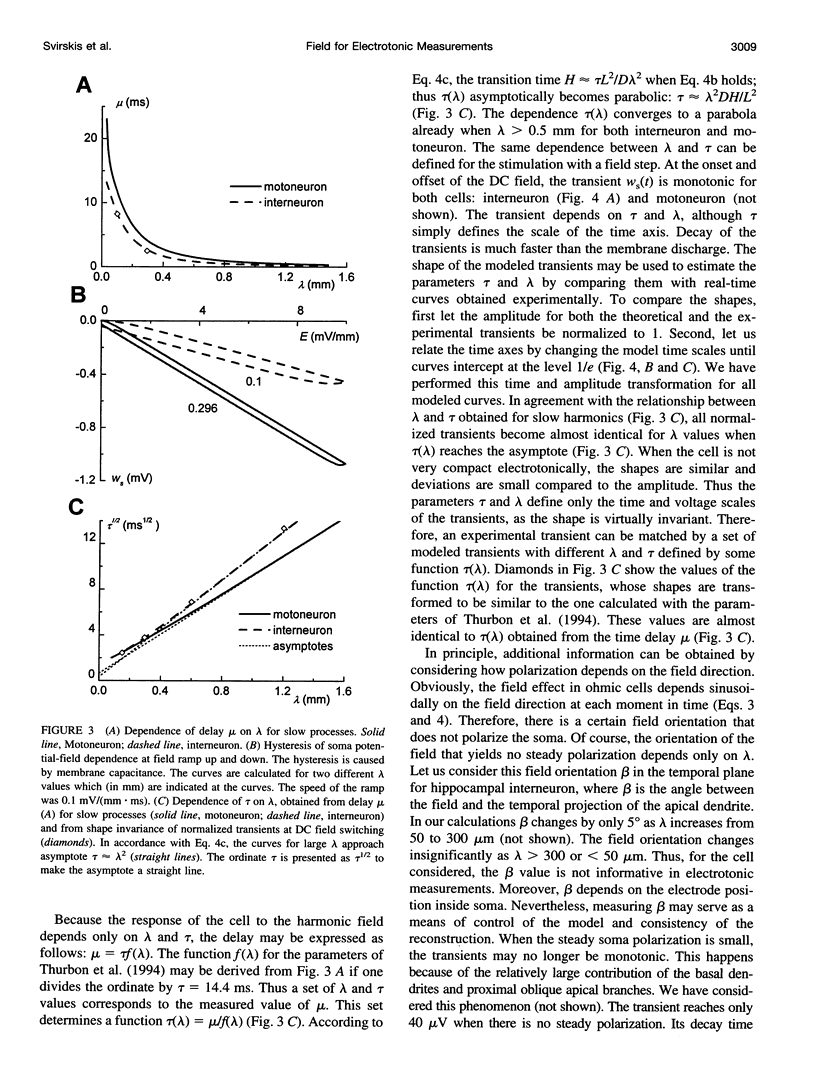
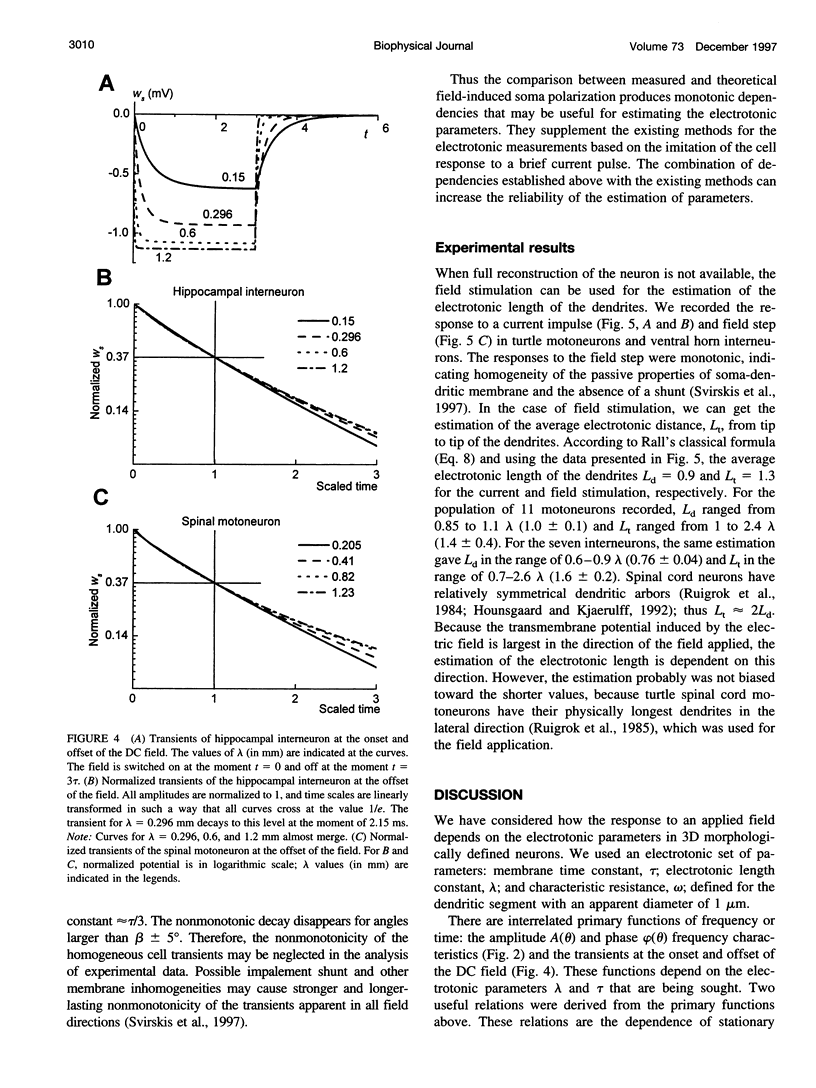
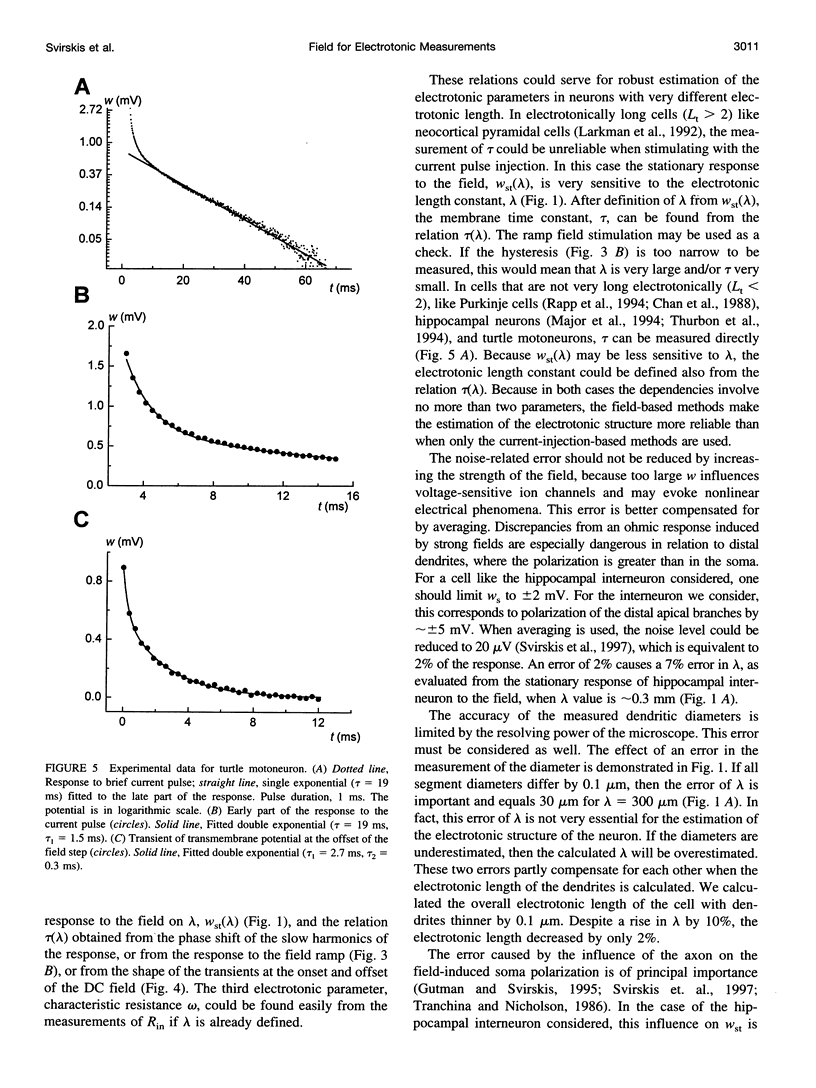
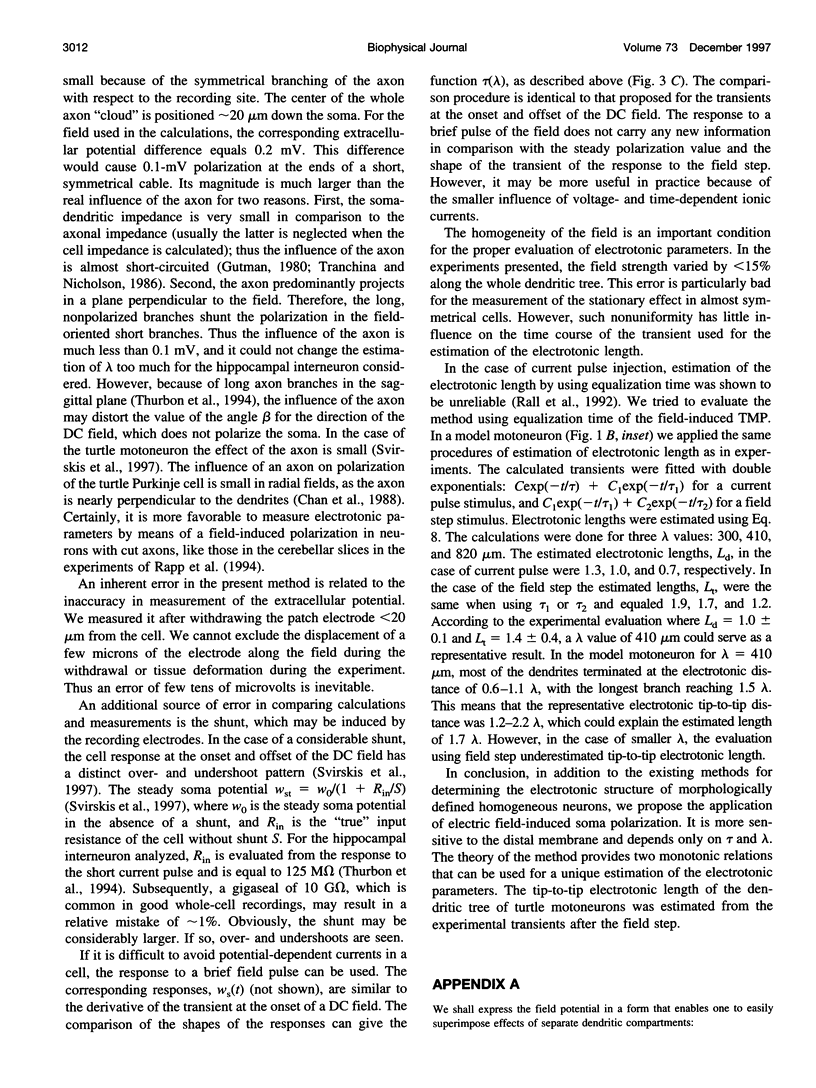
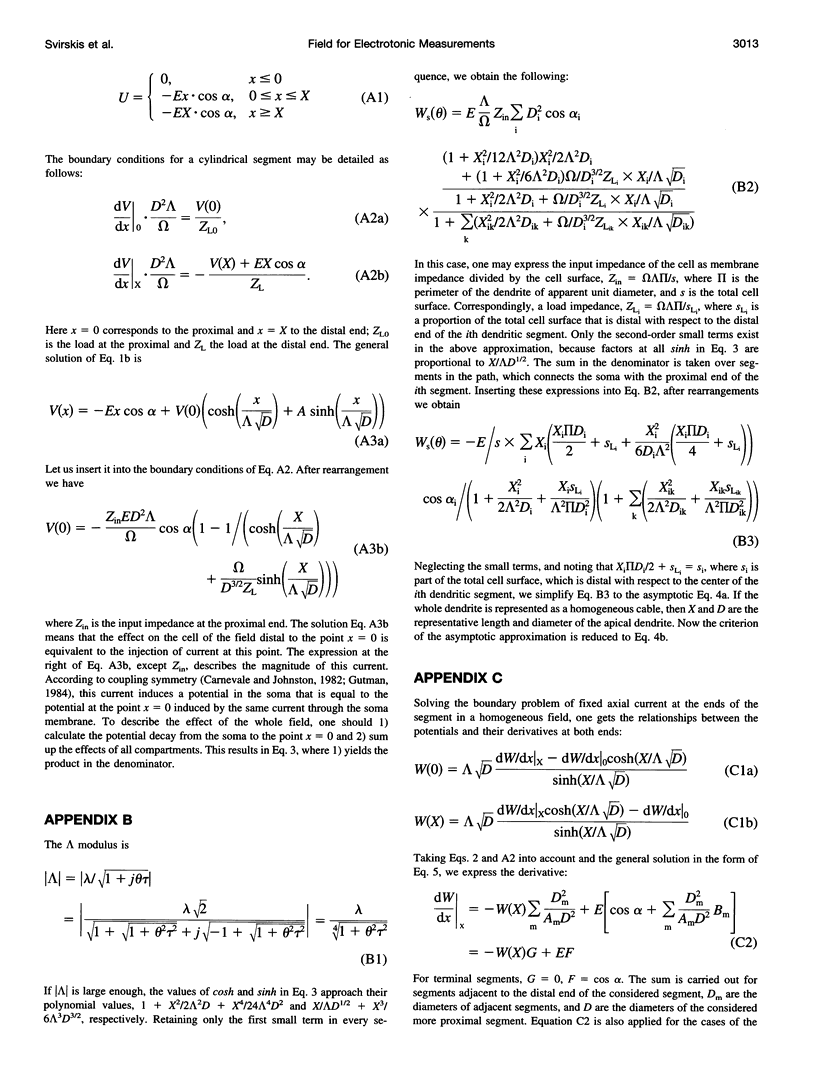
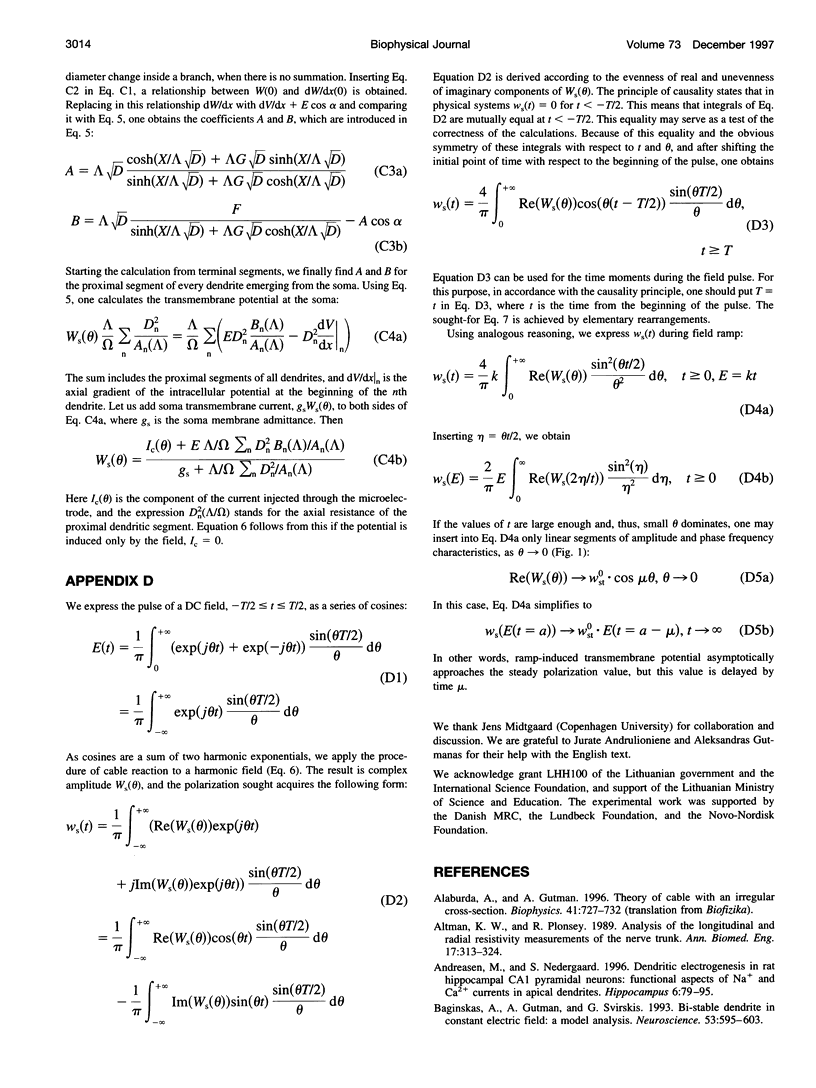
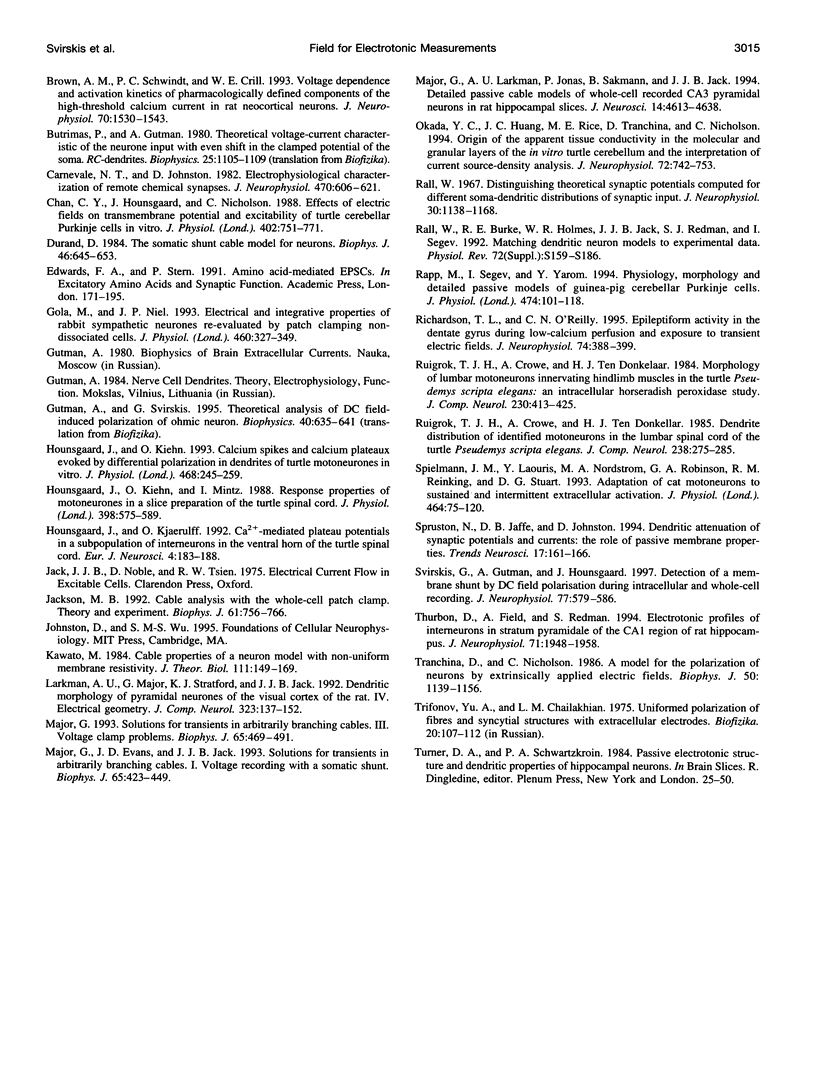
Selected References
These references are in PubMed. This may not be the complete list of references from this article.
- Altman K. W., Plonsey R. Analysis of the longitudinal and radial resistivity measurements of the nerve trunk. Ann Biomed Eng. 1989;17(4):313–324. doi: 10.1007/BF02368054. [DOI] [PubMed] [Google Scholar]
- Andreasen M., Nedergaard S. Dendritic electrogenesis in rat hippocampal CA1 pyramidal neurons: functional aspects of Na+ and Ca2+ currents in apical dendrites. Hippocampus. 1996;6(1):79–95. doi: 10.1002/(SICI)1098-1063(1996)6:1<79::AID-HIPO13>3.0.CO;2-H. [DOI] [PubMed] [Google Scholar]
- Baginskas A., Gutman A., Svirskis G. Bi-stable dendrite in constant electric field: a model analysis. Neuroscience. 1993 Mar;53(2):595–603. doi: 10.1016/0306-4522(93)90224-4. [DOI] [PubMed] [Google Scholar]
- Brown A. M., Schwindt P. C., Crill W. E. Voltage dependence and activation kinetics of pharmacologically defined components of the high-threshold calcium current in rat neocortical neurons. J Neurophysiol. 1993 Oct;70(4):1530–1543. doi: 10.1152/jn.1993.70.4.1530. [DOI] [PubMed] [Google Scholar]
- Carnevale N. T., Johnston D. Electrophysiological characterization of remote chemical synapses. J Neurophysiol. 1982 Apr;47(4):606–621. doi: 10.1152/jn.1982.47.4.606. [DOI] [PubMed] [Google Scholar]
- Chan C. Y., Hounsgaard J., Nicholson C. Effects of electric fields on transmembrane potential and excitability of turtle cerebellar Purkinje cells in vitro. J Physiol. 1988 Aug;402:751–771. doi: 10.1113/jphysiol.1988.sp017232. [DOI] [PMC free article] [PubMed] [Google Scholar]
- Durand D. The somatic shunt cable model for neurons. Biophys J. 1984 Nov;46(5):645–653. doi: 10.1016/S0006-3495(84)84063-1. [DOI] [PMC free article] [PubMed] [Google Scholar]
- Gola M., Niel J. P. Electrical and integrative properties of rabbit sympathetic neurones re-evaluated by patch clamping non-dissociated cells. J Physiol. 1993 Jan;460:327–349. doi: 10.1113/jphysiol.1993.sp019474. [DOI] [PMC free article] [PubMed] [Google Scholar]
- Hounsgaard J., Kiehn O. Calcium spikes and calcium plateaux evoked by differential polarization in dendrites of turtle motoneurones in vitro. J Physiol. 1993 Aug;468:245–259. doi: 10.1113/jphysiol.1993.sp019769. [DOI] [PMC free article] [PubMed] [Google Scholar]
- Hounsgaard J., Kiehn O., Mintz I. Response properties of motoneurones in a slice preparation of the turtle spinal cord. J Physiol. 1988 Apr;398:575–589. doi: 10.1113/jphysiol.1988.sp017058. [DOI] [PMC free article] [PubMed] [Google Scholar]
- Hounsgaard J., Kjaerulff O. Ca2+-Mediated Plateau Potentials in a Subpopulation of Interneurons in the Ventral Horn of the Turtle Spinal Cord. Eur J Neurosci. 1992;4(2):183–188. doi: 10.1111/j.1460-9568.1992.tb00865.x. [DOI] [PubMed] [Google Scholar]
- Jackson M. B. Cable analysis with the whole-cell patch clamp. Theory and experiment. Biophys J. 1992 Mar;61(3):756–766. doi: 10.1016/S0006-3495(92)81880-5. [DOI] [PMC free article] [PubMed] [Google Scholar]
- Kawato M. Cable properties of a neuron model with non-uniform membrane resistivity. J Theor Biol. 1984 Nov 7;111(1):149–169. doi: 10.1016/s0022-5193(84)80202-7. [DOI] [PubMed] [Google Scholar]
- Larkman A. U., Major G., Stratford K. J., Jack J. J. Dendritic morphology of pyramidal neurones of the visual cortex of the rat. IV: Electrical geometry. J Comp Neurol. 1992 Sep 8;323(2):137–152. doi: 10.1002/cne.903230202. [DOI] [PubMed] [Google Scholar]
- Major G., Evans J. D., Jack J. J. Solutions for transients in arbitrarily branching cables: I. Voltage recording with a somatic shunt. Biophys J. 1993 Jul;65(1):423–449. doi: 10.1016/S0006-3495(93)81037-3. [DOI] [PMC free article] [PubMed] [Google Scholar]
- Major G., Larkman A. U., Jonas P., Sakmann B., Jack J. J. Detailed passive cable models of whole-cell recorded CA3 pyramidal neurons in rat hippocampal slices. J Neurosci. 1994 Aug;14(8):4613–4638. doi: 10.1523/JNEUROSCI.14-08-04613.1994. [DOI] [PMC free article] [PubMed] [Google Scholar]
- Major G. Solutions for transients in arbitrarily branching cables: III. Voltage clamp problems. Biophys J. 1993 Jul;65(1):469–491. doi: 10.1016/S0006-3495(93)81039-7. [DOI] [PMC free article] [PubMed] [Google Scholar]
- Okada Y. C., Huang J. C., Rice M. E., Tranchina D., Nicholson C. Origin of the apparent tissue conductivity in the molecular and granular layers of the in vitro turtle cerebellum and the interpretation of current source-density analysis. J Neurophysiol. 1994 Aug;72(2):742–753. doi: 10.1152/jn.1994.72.2.742. [DOI] [PubMed] [Google Scholar]
- Rall W., Burke R. E., Holmes W. R., Jack J. J., Redman S. J., Segev I. Matching dendritic neuron models to experimental data. Physiol Rev. 1992 Oct;72(4 Suppl):S159–S186. doi: 10.1152/physrev.1992.72.suppl_4.S159. [DOI] [PubMed] [Google Scholar]
- Rall W. Distinguishing theoretical synaptic potentials computed for different soma-dendritic distributions of synaptic input. J Neurophysiol. 1967 Sep;30(5):1138–1168. doi: 10.1152/jn.1967.30.5.1138. [DOI] [PubMed] [Google Scholar]
- Rapp M., Segev I., Yarom Y. Physiology, morphology and detailed passive models of guinea-pig cerebellar Purkinje cells. J Physiol. 1994 Jan 1;474(1):101–118. doi: 10.1113/jphysiol.1994.sp020006. [DOI] [PMC free article] [PubMed] [Google Scholar]
- Richardson T. L., O'Reilly C. N. Epileptiform activity in the dentate gyrus during low-calcium perfusion and exposure to transient electric fields. J Neurophysiol. 1995 Jul;74(1):388–399. doi: 10.1152/jn.1995.74.1.388. [DOI] [PubMed] [Google Scholar]
- Ruigrok T. J., Crowe A., ten Donkelaar H. J. Dendrite distribution of identified motoneurons in the lumbar spinal cord of the turtle Pseudemys scripta elegans. J Comp Neurol. 1985 Aug 15;238(3):275–285. doi: 10.1002/cne.902380304. [DOI] [PubMed] [Google Scholar]
- Ruigrok T. J., Crowe A., ten Donkelaar H. J. Morphology of lumbar motoneurons innervating hindlimb muscles in the turtle Pseudemys scripta elegans: an intracellular horseradish peroxidase study. J Comp Neurol. 1984 Dec 10;230(3):413–425. doi: 10.1002/cne.902300309. [DOI] [PubMed] [Google Scholar]
- Spielmann J. M., Laouris Y., Nordstrom M. A., Robinson G. A., Reinking R. M., Stuart D. G. Adaptation of cat motoneurons to sustained and intermittent extracellular activation. J Physiol. 1993 May;464:75–120. doi: 10.1113/jphysiol.1993.sp019625. [DOI] [PMC free article] [PubMed] [Google Scholar]
- Spruston N., Jaffe D. B., Johnston D. Dendritic attenuation of synaptic potentials and currents: the role of passive membrane properties. Trends Neurosci. 1994 Apr;17(4):161–166. doi: 10.1016/0166-2236(94)90094-9. [DOI] [PubMed] [Google Scholar]
- Svirskis G., Gutman A., Hounsgaard J. Detection of a membrane shunt by DC field polarization during intracellular and whole cell recording. J Neurophysiol. 1997 Feb;77(2):579–586. doi: 10.1152/jn.1997.77.2.579. [DOI] [PubMed] [Google Scholar]
- Thurbon D., Field A., Redman S. Electrotonic profiles of interneurons in stratum pyramidale of the CA1 region of rat hippocampus. J Neurophysiol. 1994 May;71(5):1948–1958. doi: 10.1152/jn.1994.71.5.1948. [DOI] [PubMed] [Google Scholar]
- Tranchina D., Nicholson C. A model for the polarization of neurons by extrinsically applied electric fields. Biophys J. 1986 Dec;50(6):1139–1156. doi: 10.1016/S0006-3495(86)83558-5. [DOI] [PMC free article] [PubMed] [Google Scholar]