Abstract
The effect of solubilized hydrophobic peptides on the phase behavior of dioleoylphosphatidylcholine (DOPC)/water system was studied by 2H- and 31P-NMR spectroscopy and by x-ray diffraction, and partial phase diagrams were constructed. The utilized peptides were HCO-AWW(LA)5WWA-NHCH2CH2OH (WALP16), which is an artificial peptide designed to resemble a transmembrane part of a membrane protein; and VEYAGIALFFVAAVLTLWSMLQYLSAAR (Pgs peptide E), a peptide that is identical to one of the putative transmembrane segments of the membrane-associated protein phosphatidylglycerophosphate synthase (Pgs) in Escherichia coli. Circular dichroism spectroscopy suggests that both peptides are mostly alpha-helical in DOPC vesicles. The most striking features in the phase diagram of the WALP16/DOPC/water system are 1) a single lamellar liquid crystalline (L alpha) phase forms only at very low peptide concentrations. 2) At low water content and above a peptide/lipid molar ratio of approximately 1:75 a reversed hexagonal liquid crystalline (H[II]) phase coexists with an L alpha phase, while in excess water this phase forms at a peptide/lipid molar ratio of approximately 1:25. 3) At peptide/lipid ratios > or =1:6 a single H(II) phase is stable. Also, the Pgs peptide E strongly affects the phase behavior, and a single L alpha phase is only found at low peptide concentrations (peptide/lipid molar ratios <1:50), and water concentrations <45% (w/w). Higher peptide content results in coexistence of L alpha and isotropic phases. Generally, the fraction of the isotropic phase increases with increasing temperature and water concentration, and at 80% (w/w) water content only a single isotropic phase is stable at 55 degrees C. Thus, both peptides were found to be able to induce nonlamellar phases, although different in structure, in the DOPC/water system. The phase transitions, the extensions of the one-phase regions, and the phase structures observed for the two systems are discussed in terms of the molecular structure of the two peptides and the matching between the hydrophobic lengths of the peptides and the bilayer thickness of DOPC.
Full text
PDF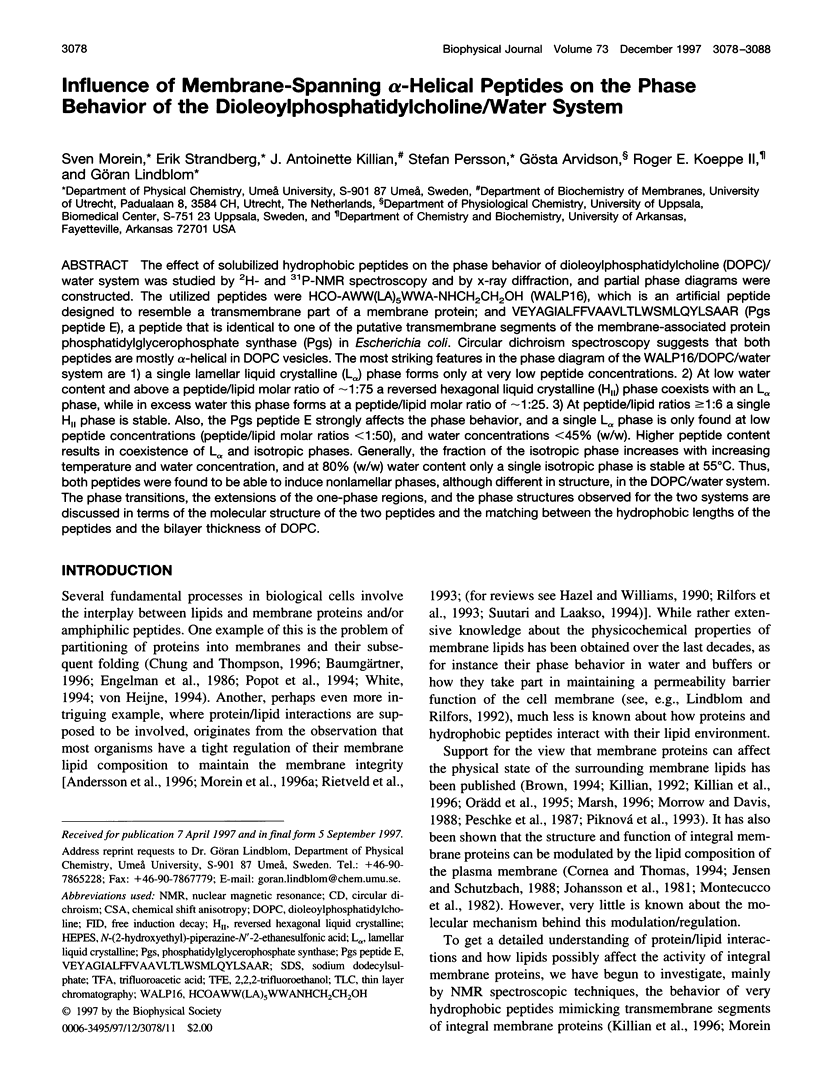
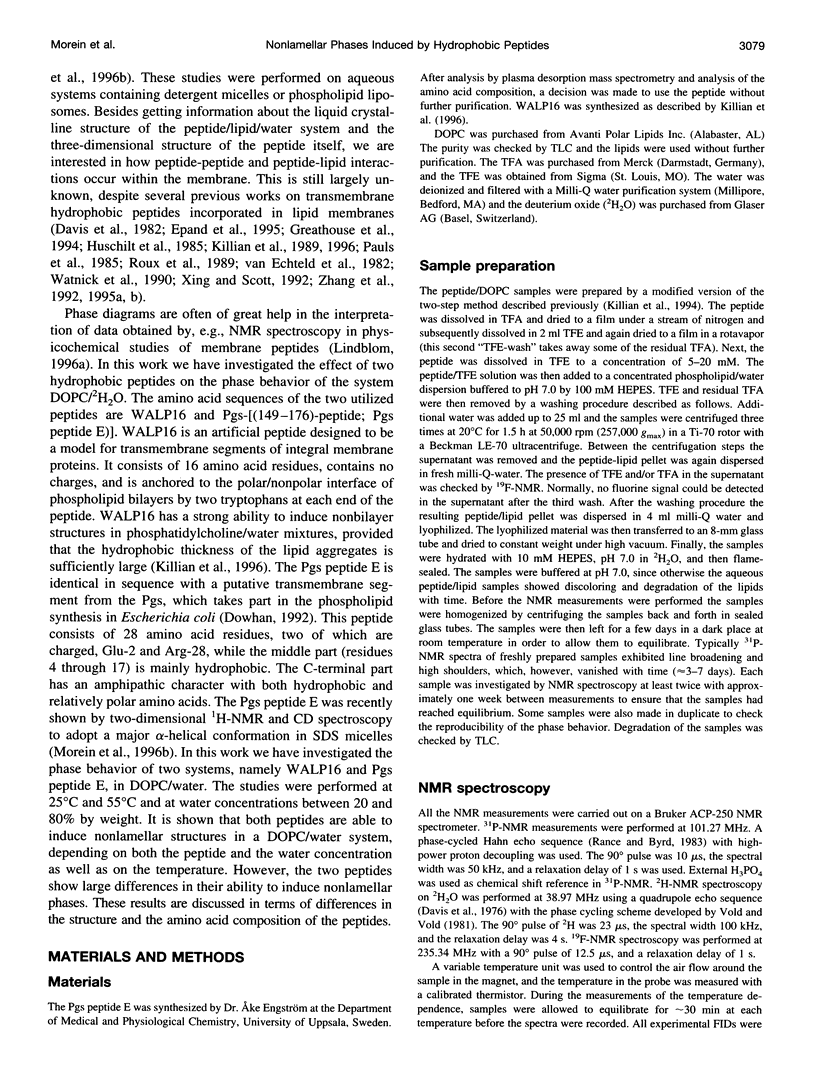
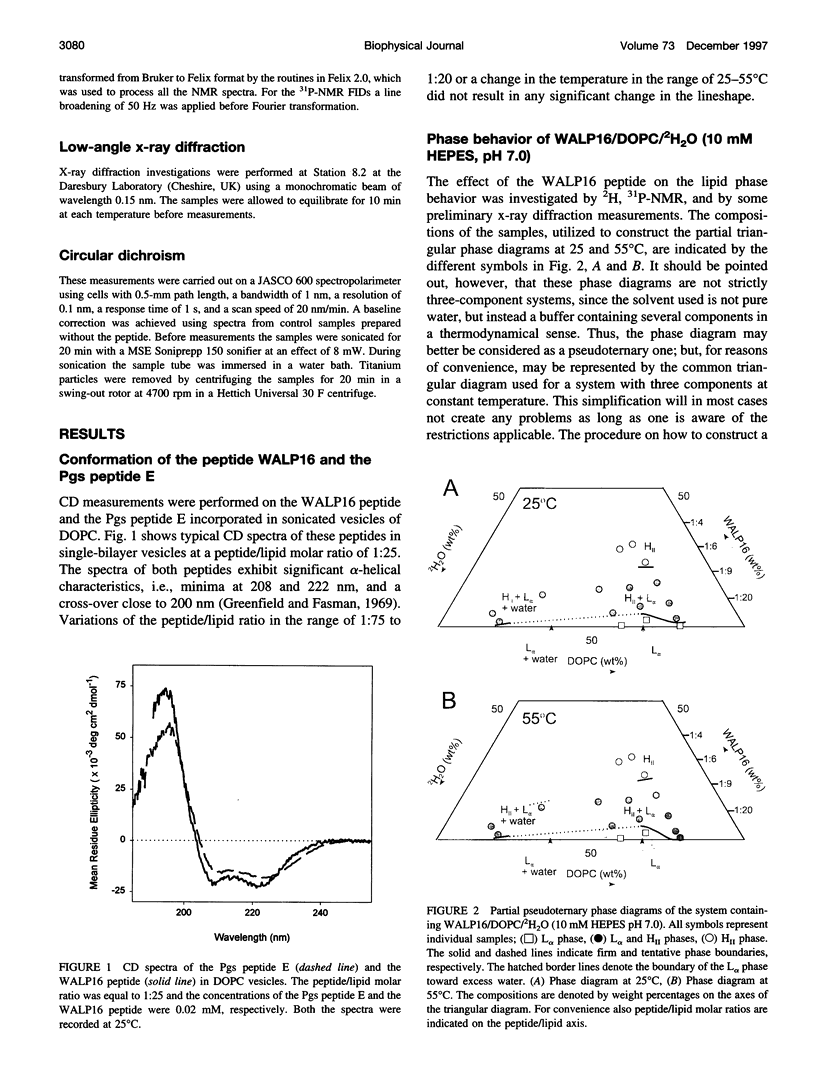
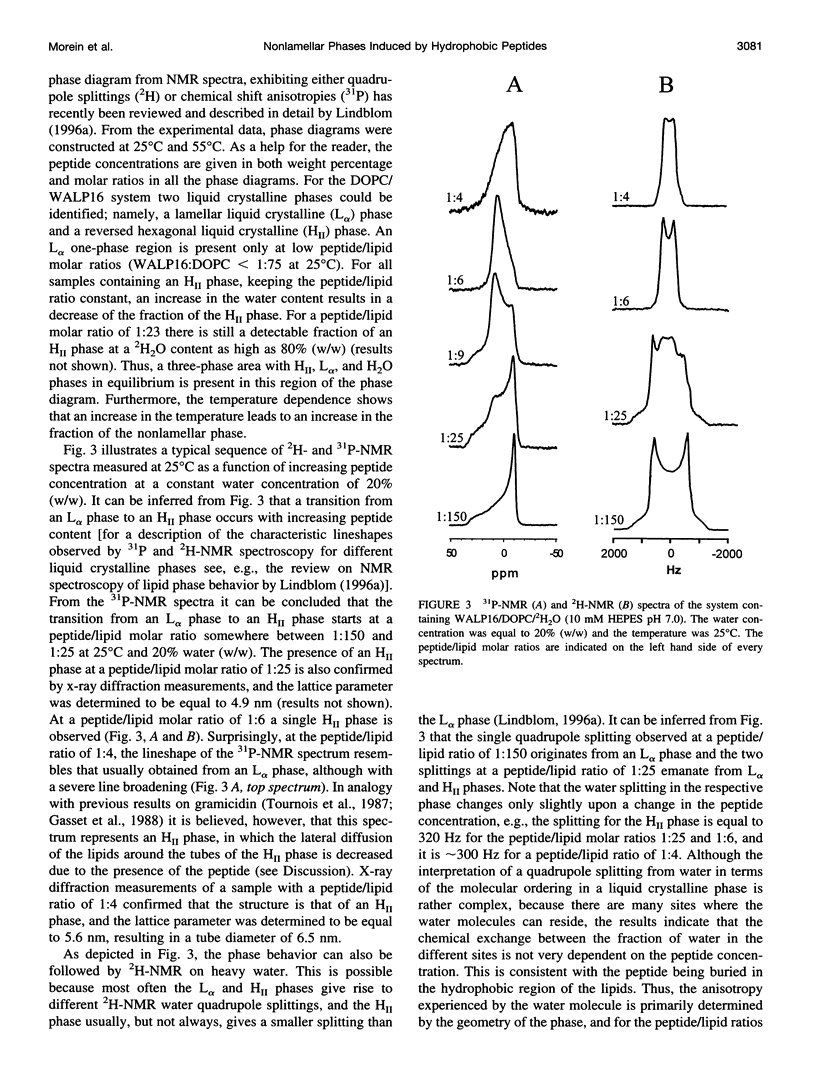
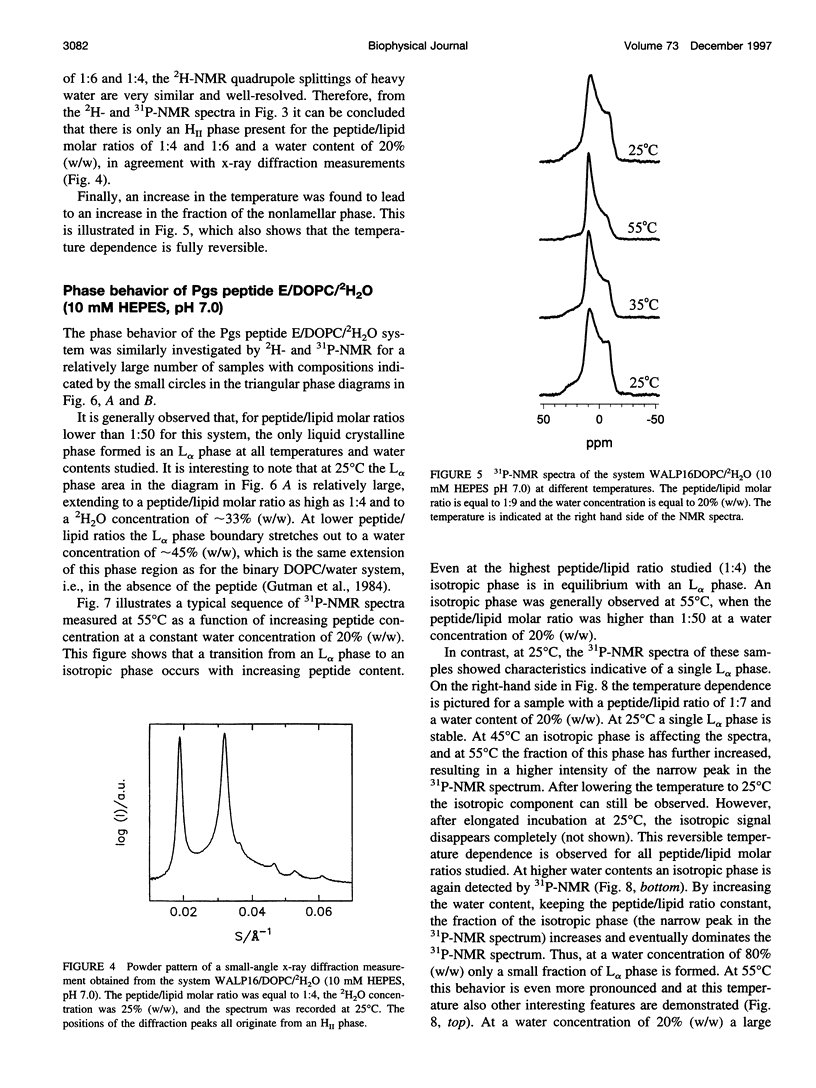
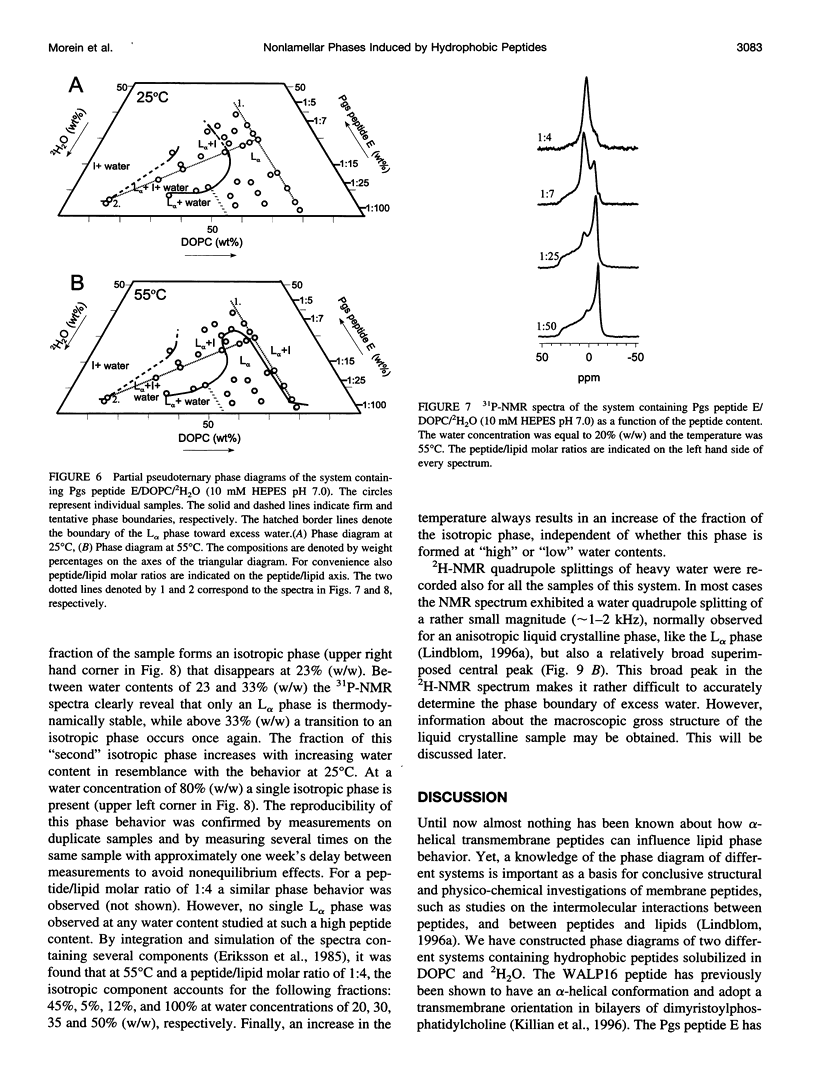
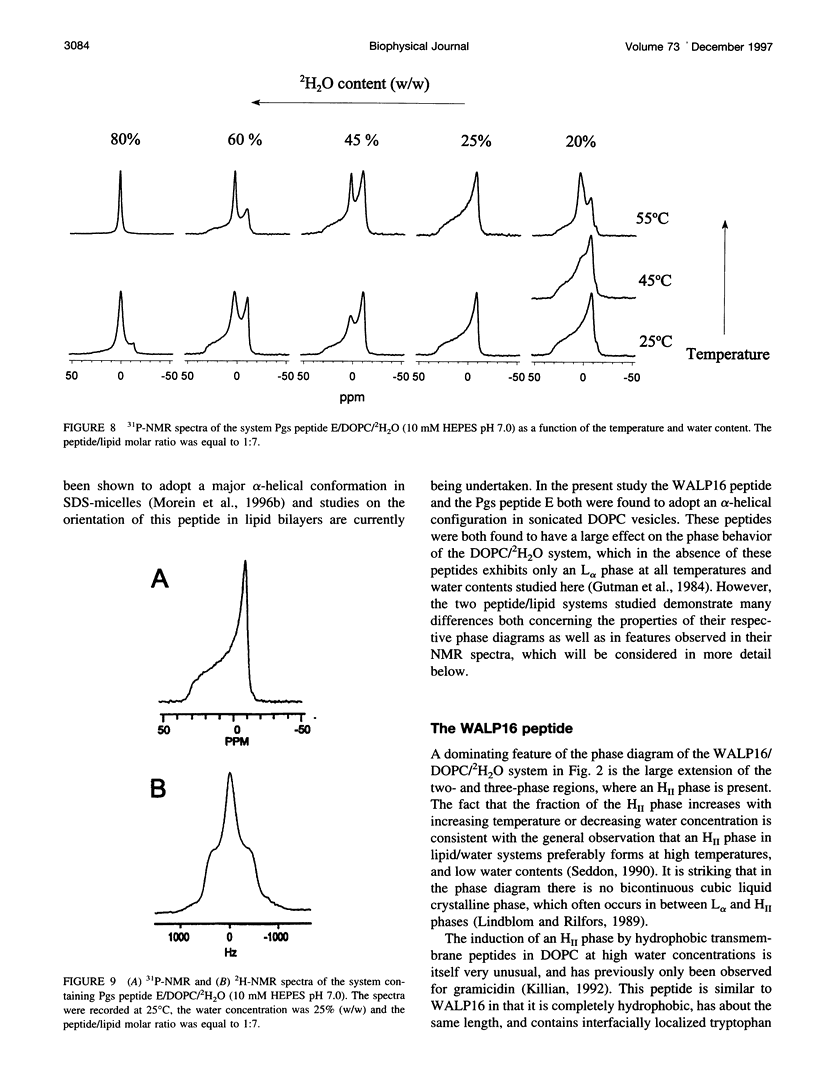
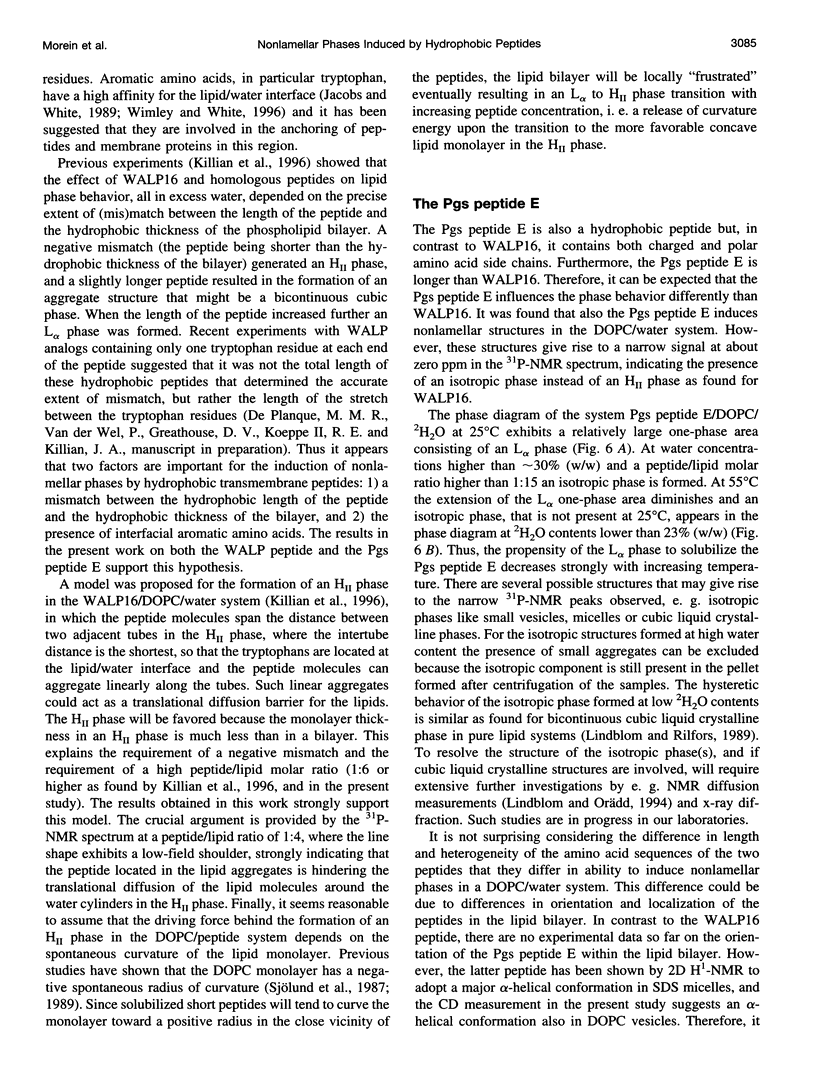
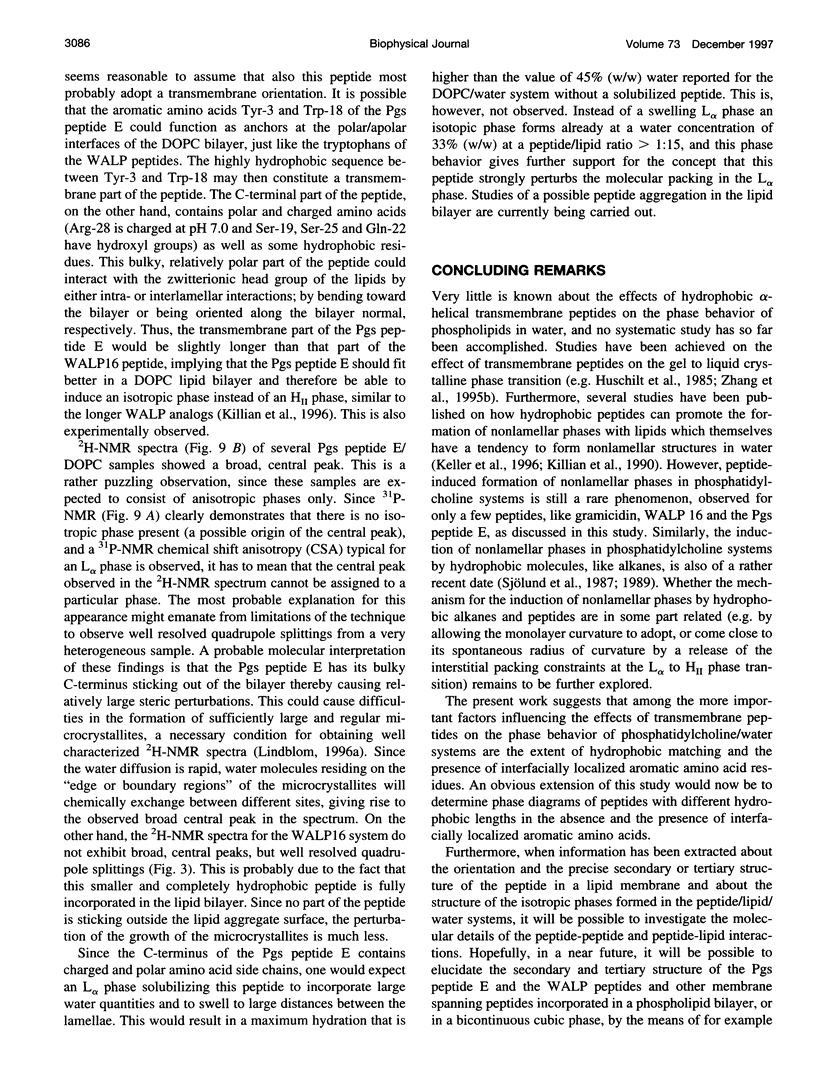
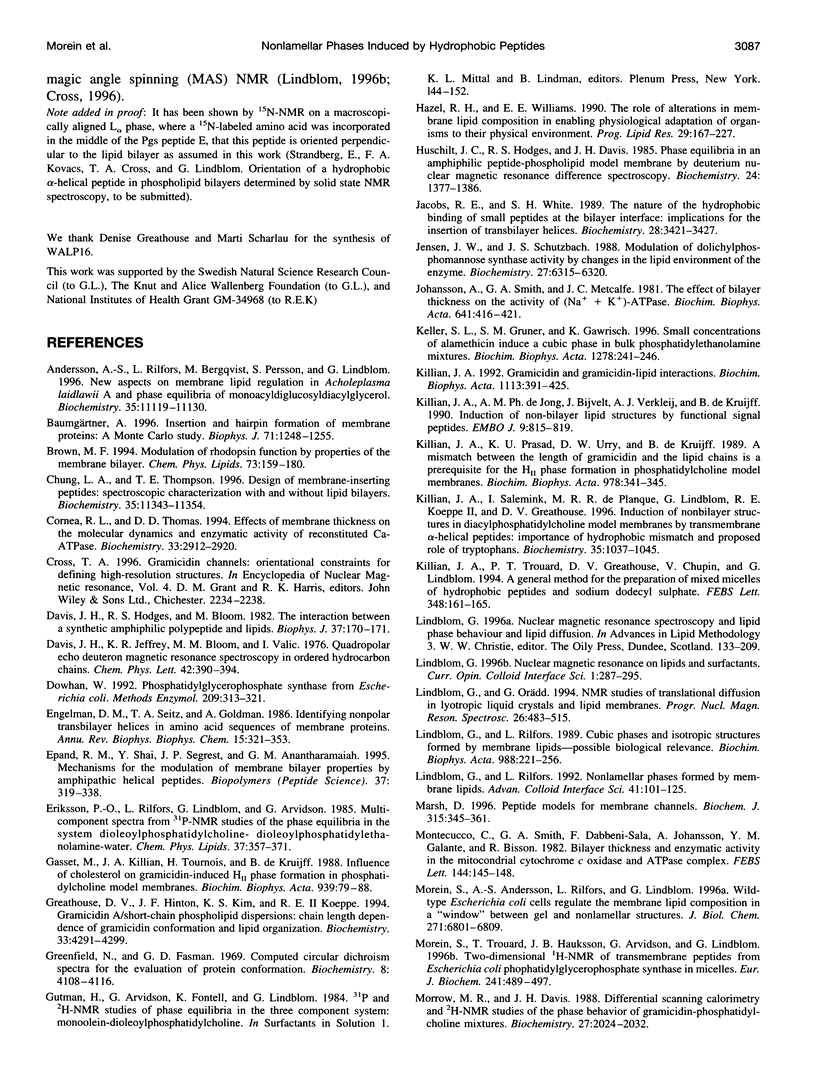
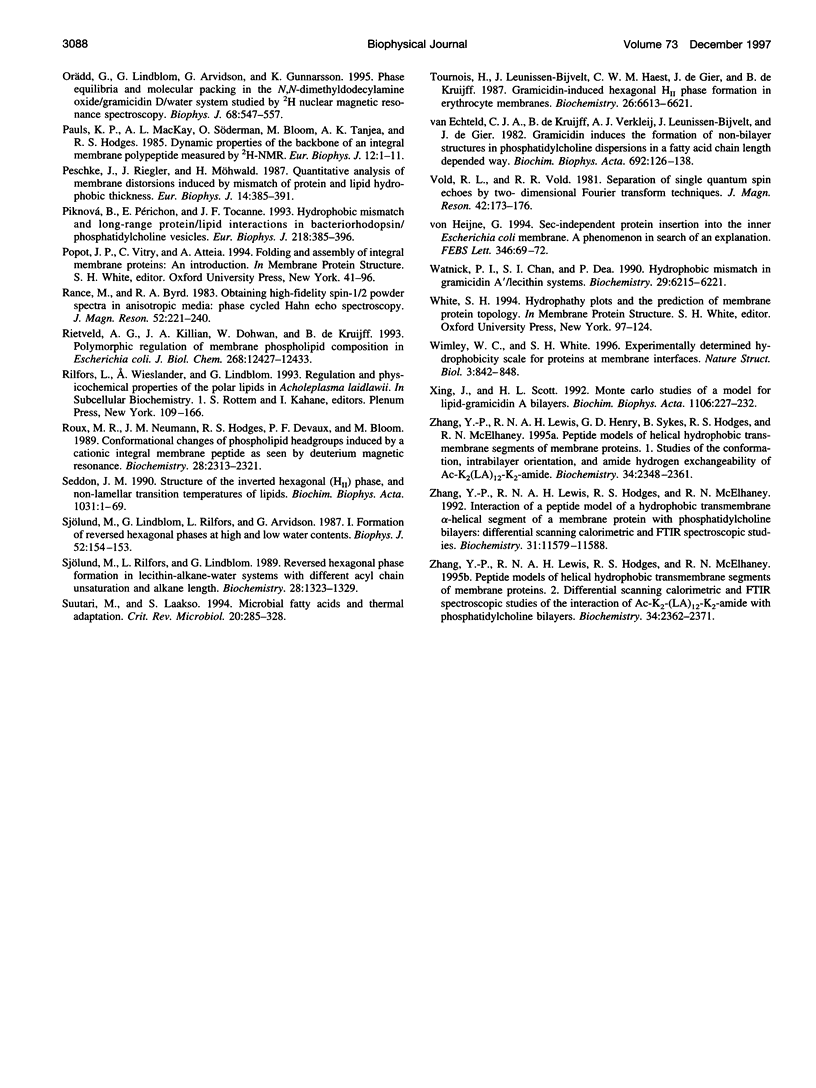
Images in this article
Selected References
These references are in PubMed. This may not be the complete list of references from this article.
- Andersson A. S., Rilfors L., Bergqvist M., Persson S., Lindblom G. New aspects on membrane lipid regulation in Acholeplasma laidlawii A and phase equilibria of monoacyldiglucosyldiacylglycerol. Biochemistry. 1996 Aug 27;35(34):11119–11130. doi: 10.1021/bi960561w. [DOI] [PubMed] [Google Scholar]
- Baumgärtner A. Insertion and hairpin formation of membrane proteins: a Monte Carlo study. Biophys J. 1996 Sep;71(3):1248–1255. doi: 10.1016/S0006-3495(96)79324-4. [DOI] [PMC free article] [PubMed] [Google Scholar]
- Brown M. F. Modulation of rhodopsin function by properties of the membrane bilayer. Chem Phys Lipids. 1994 Sep 6;73(1-2):159–180. doi: 10.1016/0009-3084(94)90180-5. [DOI] [PubMed] [Google Scholar]
- Chung L. A., Thompson T. E. Design of membrane-inserting peptides: spectroscopic characterization with and without lipid bilayers. Biochemistry. 1996 Sep 3;35(35):11343–11354. doi: 10.1021/bi960080c. [DOI] [PubMed] [Google Scholar]
- Cornea R. L., Thomas D. D. Effects of membrane thickness on the molecular dynamics and enzymatic activity of reconstituted Ca-ATPase. Biochemistry. 1994 Mar 15;33(10):2912–2920. doi: 10.1021/bi00176a022. [DOI] [PubMed] [Google Scholar]
- Davis J. H., Hodges R. S., Bloom M. The Interaction between a Synthetic Amphiphilic Polypeptide and Lipids. Biophys J. 1982 Jan;37(1):170–171. doi: 10.1016/S0006-3495(82)84655-9. [DOI] [PMC free article] [PubMed] [Google Scholar]
- Dowhan W. Phosphatidylglycerophosphate synthase from Escherichia coli. Methods Enzymol. 1992;209:313–321. doi: 10.1016/0076-6879(92)09039-6. [DOI] [PubMed] [Google Scholar]
- Engelman D. M., Steitz T. A., Goldman A. Identifying nonpolar transbilayer helices in amino acid sequences of membrane proteins. Annu Rev Biophys Biophys Chem. 1986;15:321–353. doi: 10.1146/annurev.bb.15.060186.001541. [DOI] [PubMed] [Google Scholar]
- Epand R. M., Shai Y., Segrest J. P., Anantharamaiah G. M. Mechanisms for the modulation of membrane bilayer properties by amphipathic helical peptides. Biopolymers. 1995;37(5):319–338. doi: 10.1002/bip.360370504. [DOI] [PubMed] [Google Scholar]
- Gasset M., Killian J. A., Tournois H., de Kruijff B. Influence of cholesterol on gramicidin-induced HII phase formation in phosphatidylcholine model membranes. Biochim Biophys Acta. 1988 Mar 22;939(1):79–88. doi: 10.1016/0005-2736(88)90049-1. [DOI] [PubMed] [Google Scholar]
- Greathouse D. V., Hinton J. F., Kim K. S., Koeppe R. E., 2nd Gramicidin A/short-chain phospholipid dispersions: chain length dependence of gramicidin conformation and lipid organization. Biochemistry. 1994 Apr 12;33(14):4291–4299. doi: 10.1021/bi00180a025. [DOI] [PubMed] [Google Scholar]
- Greenfield N., Fasman G. D. Computed circular dichroism spectra for the evaluation of protein conformation. Biochemistry. 1969 Oct;8(10):4108–4116. doi: 10.1021/bi00838a031. [DOI] [PubMed] [Google Scholar]
- Hazel J. R., Williams E. E. The role of alterations in membrane lipid composition in enabling physiological adaptation of organisms to their physical environment. Prog Lipid Res. 1990;29(3):167–227. doi: 10.1016/0163-7827(90)90002-3. [DOI] [PubMed] [Google Scholar]
- Jacobs R. E., White S. H. The nature of the hydrophobic binding of small peptides at the bilayer interface: implications for the insertion of transbilayer helices. Biochemistry. 1989 Apr 18;28(8):3421–3437. doi: 10.1021/bi00434a042. [DOI] [PubMed] [Google Scholar]
- Jensen J. W., Schutzbach J. S. Modulation of dolichyl-phosphomannose synthase activity by changes in the lipid environment of the enzyme. Biochemistry. 1988 Aug 23;27(17):6315–6320. doi: 10.1021/bi00417a017. [DOI] [PubMed] [Google Scholar]
- Johannsson A., Smith G. A., Metcalfe J. C. The effect of bilayer thickness on the activity of (Na+ + K+)-ATPase. Biochim Biophys Acta. 1981 Mar 6;641(2):416–421. doi: 10.1016/0005-2736(81)90498-3. [DOI] [PubMed] [Google Scholar]
- Keller S. L., Gruner S. M., Gawrisch K. Small concentrations of alamethicin induce a cubic phase in bulk phosphatidylethanolamine mixtures. Biochim Biophys Acta. 1996 Jan 31;1278(2):241–246. doi: 10.1016/0005-2736(95)00229-4. [DOI] [PubMed] [Google Scholar]
- Killian J. A. Gramicidin and gramicidin-lipid interactions. Biochim Biophys Acta. 1992 Dec 11;1113(3-4):391–425. doi: 10.1016/0304-4157(92)90008-x. [DOI] [PubMed] [Google Scholar]
- Killian J. A., Prasad K. U., Urry D. W., de Kruijff B. A mismatch between the length of gramicidin and the lipid acyl chains is a prerequisite for HII phase formation in phosphatidylcholine model membranes. Biochim Biophys Acta. 1989 Jan 30;978(2):341–345. doi: 10.1016/0005-2736(89)90135-1. [DOI] [PubMed] [Google Scholar]
- Killian J. A., Salemink I., de Planque M. R., Lindblom G., Koeppe R. E., 2nd, Greathouse D. V. Induction of nonbilayer structures in diacylphosphatidylcholine model membranes by transmembrane alpha-helical peptides: importance of hydrophobic mismatch and proposed role of tryptophans. Biochemistry. 1996 Jan 23;35(3):1037–1045. doi: 10.1021/bi9519258. [DOI] [PubMed] [Google Scholar]
- Killian J. A., Trouard T. P., Greathouse D. V., Chupin V., Lindblom G. A general method for the preparation of mixed micelles of hydrophobic peptides and sodium dodecyl sulphate. FEBS Lett. 1994 Jul 11;348(2):161–165. doi: 10.1016/0014-5793(94)00594-x. [DOI] [PubMed] [Google Scholar]
- Killian J. A., de Jong A. M., Bijvelt J., Verkleij A. J., de Kruijff B. Induction of non-bilayer lipid structures by functional signal peptides. EMBO J. 1990 Mar;9(3):815–819. doi: 10.1002/j.1460-2075.1990.tb08178.x. [DOI] [PMC free article] [PubMed] [Google Scholar]
- Lindblom G., Rilfors L. Nonlamellar phases formed by membrane lipids. Adv Colloid Interface Sci. 1992 Sep 28;41:101–125. doi: 10.1016/0001-8686(92)80009-m. [DOI] [PubMed] [Google Scholar]
- Marsh D. Peptide models for membrane channels. Biochem J. 1996 Apr 15;315(Pt 2):345–361. doi: 10.1042/bj3150345. [DOI] [PMC free article] [PubMed] [Google Scholar]
- Montecucco C., Smith G. A., Dabbeni-sala F., Johannsson A., Galante Y. M., Bisson R. Bilayer thickness and enzymatic activity in the mitochondrial cytochrome c oxidase and ATPase complex. FEBS Lett. 1982 Jul 19;144(1):145–148. doi: 10.1016/0014-5793(82)80588-7. [DOI] [PubMed] [Google Scholar]
- Morein S., Andersson A., Rilfors L., Lindblom G. Wild-type Escherichia coli cells regulate the membrane lipid composition in a "window" between gel and non-lamellar structures. J Biol Chem. 1996 Mar 22;271(12):6801–6809. doi: 10.1074/jbc.271.12.6801. [DOI] [PubMed] [Google Scholar]
- Morein S., Trouard T. P., Hauksson J. B., Rilfors L., Arvidson G., Lindblom G. Two-dimensional 1H-NMR of transmembrane peptides from Escherichia coli phosphatidylglycerophosphate synthase in micelles. Eur J Biochem. 1996 Oct 15;241(2):489–497. doi: 10.1111/j.1432-1033.1996.00489.x. [DOI] [PubMed] [Google Scholar]
- Morrow M. R., Davis J. H. Differential scanning calorimetry and 2H NMR studies of the phase behavior of gramicidin-phosphatidylcholine mixtures. Biochemistry. 1988 Mar 22;27(6):2024–2032. doi: 10.1021/bi00406a032. [DOI] [PubMed] [Google Scholar]
- Orädd G., Lindblom G., Arvidson G., Gunnarsson K. Phase equilibria and molecular packing in the N,N-dimethyldodecylamine oxide/gramicidin D/water system studied by 2H nuclear magnetic resonance spectroscopy. Biophys J. 1995 Feb;68(2):547–557. doi: 10.1016/S0006-3495(95)80216-X. [DOI] [PMC free article] [PubMed] [Google Scholar]
- Pauls K. P., MacKay A. L., Söderman O., Bloom M., Tanjea A. K., Hodges R. S. Dynamic properties of the backbone of an integral membrane polypeptide measured by 2H-NMR. Eur Biophys J. 1985;12(1):1–11. doi: 10.1007/BF00254089. [DOI] [PubMed] [Google Scholar]
- Piknová B., Pérochon E., Tocanne J. F. Hydrophobic mismatch and long-range protein/lipid interactions in bacteriorhodopsin/phosphatidylcholine vesicles. Eur J Biochem. 1993 Dec 1;218(2):385–396. doi: 10.1111/j.1432-1033.1993.tb18388.x. [DOI] [PubMed] [Google Scholar]
- Rietveld A. G., Killian J. A., Dowhan W., de Kruijff B. Polymorphic regulation of membrane phospholipid composition in Escherichia coli. J Biol Chem. 1993 Jun 15;268(17):12427–12433. [PubMed] [Google Scholar]
- Rilfors L., Wieslander A., Lindblom G. Regulation and physicochemical properties of the polar lipids in Acholeplasma laidlawii. Subcell Biochem. 1993;20:109–166. doi: 10.1007/978-1-4615-2924-8_4. [DOI] [PubMed] [Google Scholar]
- Roux M., Neumann J. M., Hodges R. S., Devaux P. F., Bloom M. Conformational changes of phospholipid headgroups induced by a cationic integral membrane peptide as seen by deuterium magnetic resonance. Biochemistry. 1989 Mar 7;28(5):2313–2321. doi: 10.1021/bi00431a050. [DOI] [PubMed] [Google Scholar]
- Seddon J. M. Structure of the inverted hexagonal (HII) phase, and non-lamellar phase transitions of lipids. Biochim Biophys Acta. 1990 Feb 28;1031(1):1–69. doi: 10.1016/0304-4157(90)90002-t. [DOI] [PubMed] [Google Scholar]
- Sjölund M., Lindblom G., Rilfors L., Arvidson G. Hydrophobic molecules in lecithin-water systems. I. Formation of reversed hexagonal phases at high and low water contents. Biophys J. 1987 Aug;52(2):145–153. doi: 10.1016/S0006-3495(87)83202-2. [DOI] [PMC free article] [PubMed] [Google Scholar]
- Sjölund M., Rilfors L., Lindblom G. Reversed hexagonal phase formation in lecithin-alkane-water systems with different acyl chain unsaturation and alkane length. Biochemistry. 1989 Feb 7;28(3):1323–1329. doi: 10.1021/bi00429a057. [DOI] [PubMed] [Google Scholar]
- Suutari M., Laakso S. Microbial fatty acids and thermal adaptation. Crit Rev Microbiol. 1994;20(4):285–328. doi: 10.3109/10408419409113560. [DOI] [PubMed] [Google Scholar]
- Tournois H., Leunissen-Bijvelt J., Haest C. W., de Gier J., de Kruijff B. Gramicidin-induced hexagonal HII phase formation in erythrocyte membranes. Biochemistry. 1987 Oct 20;26(21):6613–6621. doi: 10.1021/bi00395a008. [DOI] [PubMed] [Google Scholar]
- Watnick P. I., Chan S. I., Dea P. Hydrophobic mismatch in gramicidin A'/lecithin systems. Biochemistry. 1990 Jul 3;29(26):6215–6221. doi: 10.1021/bi00478a015. [DOI] [PubMed] [Google Scholar]
- Wimley W. C., White S. H. Experimentally determined hydrophobicity scale for proteins at membrane interfaces. Nat Struct Biol. 1996 Oct;3(10):842–848. doi: 10.1038/nsb1096-842. [DOI] [PubMed] [Google Scholar]
- Xing J., Scott H. L. Monte Carlo studies of a model for lipid-gramicidin A bilayers. Biochim Biophys Acta. 1992 Apr 29;1106(1):227–232. doi: 10.1016/0005-2736(92)90243-f. [DOI] [PubMed] [Google Scholar]
- Zhang Y. P., Lewis R. N., Henry G. D., Sykes B. D., Hodges R. S., McElhaney R. N. Peptide models of helical hydrophobic transmembrane segments of membrane proteins. 1. Studies of the conformation, intrabilayer orientation, and amide hydrogen exchangeability of Ac-K2-(LA)12-K2-amide. Biochemistry. 1995 Feb 21;34(7):2348–2361. doi: 10.1021/bi00007a031. [DOI] [PubMed] [Google Scholar]
- Zhang Y. P., Lewis R. N., Hodges R. S., McElhaney R. N. Interaction of a peptide model of a hydrophobic transmembrane alpha-helical segment of a membrane protein with phosphatidylcholine bilayers: differential scanning calorimetric and FTIR spectroscopic studies. Biochemistry. 1992 Nov 24;31(46):11579–11588. doi: 10.1021/bi00161a042. [DOI] [PubMed] [Google Scholar]
- Zhang Y. P., Lewis R. N., Hodges R. S., McElhaney R. N. Peptide models of helical hydrophobic transmembrane segments of membrane proteins. 2. Differential scanning calorimetric and FTIR spectroscopic studies of the interaction of Ac-K2-(LA)12-K2-amide with phosphatidylcholine bilayers. Biochemistry. 1995 Feb 21;34(7):2362–2371. doi: 10.1021/bi00007a032. [DOI] [PubMed] [Google Scholar]
- von Heijne G. Sec-independent protein insertion into the inner E. coli membrane. A phenomenon in search of an explanation. FEBS Lett. 1994 Jun 6;346(1):69–72. doi: 10.1016/0014-5793(94)00296-7. [DOI] [PubMed] [Google Scholar]