Abstract
1. In the preceding paper we analysed the force decline in fatigue of isolated mouse muscle fibres. Using the same preparation and stimulation scheme we have now examined another aspect of muscle fatigue, namely slowing of relaxation. 2. Relaxation at the end of a tetanic contraction can usually be divided into two phases, an initial nearly linear force decline, followed by an exponential phase. We have analysed the initial phase in terms of decline rate and duration. In rested fibres the decline rate after a 350 ms tetanus was not affected by a 30% reduction of tetanic tension (obtained by decreasing the stimulation frequency). 3. Relaxation became gradually slower during fatiguing stimulation with a maximum reduction at the time when tetanic tension was reduced to about 75% of the original (end of phase 2, see preceding paper). At this time the decline rate of the linear phase had fallen to 55.2 +/- 2.9% (mean +/- S.E.M., n = 25) and its duration had increased to 151.3 +/- 14.2% of the control (unfatigued 350 ms, 70 Hz tetanus). 4. Short rest periods (duration = 10s), given at various times during fatiguing stimulation, resulted in a clear, but partial, normalization of the relaxation parameters; at the time of maximum slowing the mean decline rate increased from 50.3 to 58.7% and the duration decreased from 167.9 to 144.0% of the control (n = 14). 5. The influence of intracellular acidosis on relaxation was studied by exposing rested fibres to 30% CO2 instead of the normal 5%. This resulted in a decline rate of 43.0 +/- 2.6% and a duration of 221.1 +/- 13.1% of the control (n = 7). 6. In amphibian muscle relaxation is known to become gradually slower during a single, prolonged tetanus. The existence of such an effect also in the present preparation was studied by giving 'interrupted' tetani with a total duration of about 2 s. In rested fibres the mean rate of relaxation was found to fall from 140.9 to 71.8% (n = 11) of the control (end of 350 ms stimulation) with a time constant of about 0.5 s. Thus, a marked slowing during a long tetanus occurs also in mammalian muscle. 7. A distinct slowing of relaxation during prolonged tetani was observed also in the fatigued and in the acidified state. Under these two conditions the mean rate of relaxation fell from 87.0 to 34.0% (n = 3) and from 74.0 to 23.0% (n = 5) of the control, respectively, with time constants similar to that in the rested state.(ABSTRACT TRUNCATED AT 400 WORDS)
Full text
PDF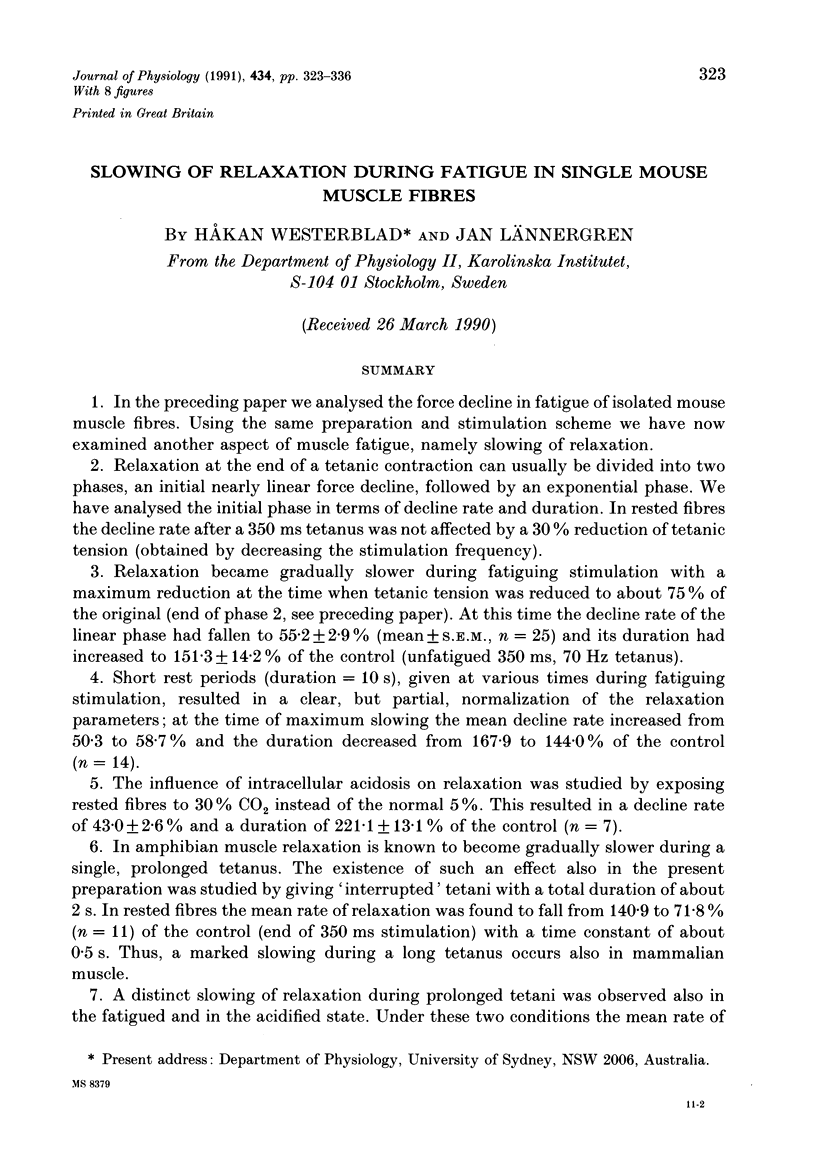
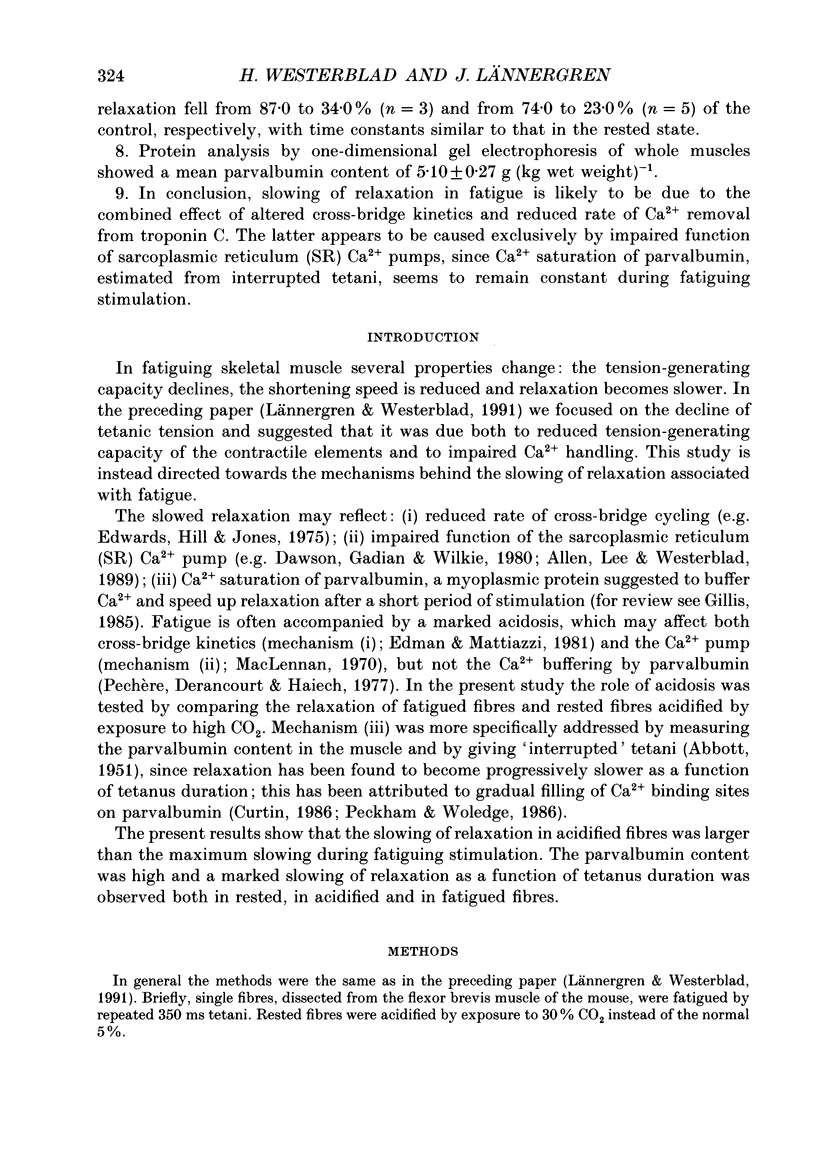
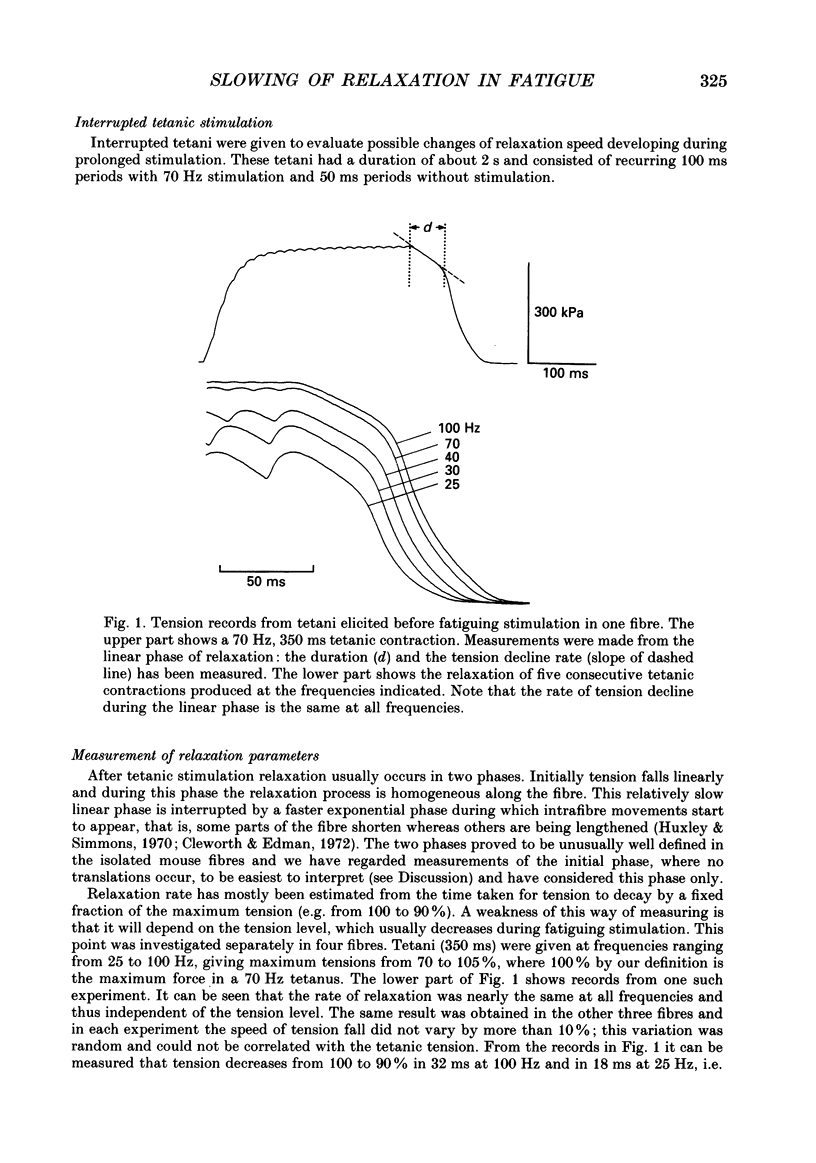
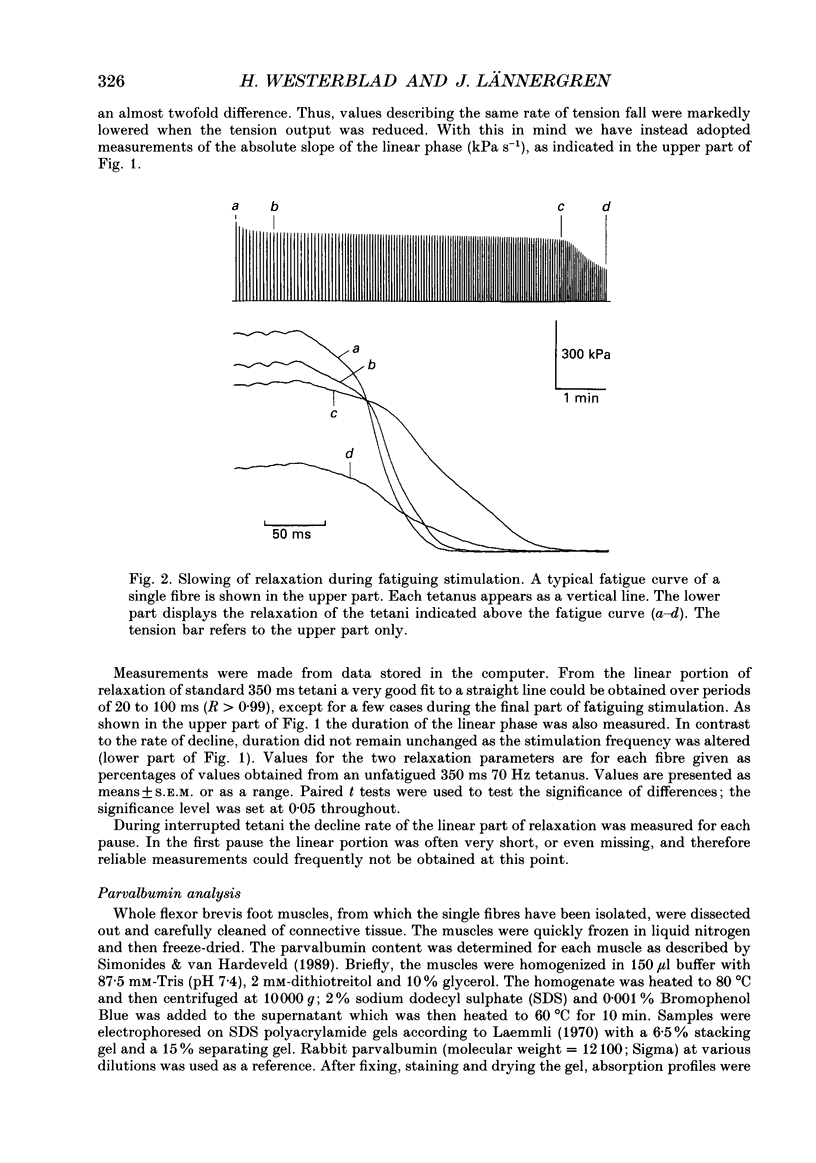
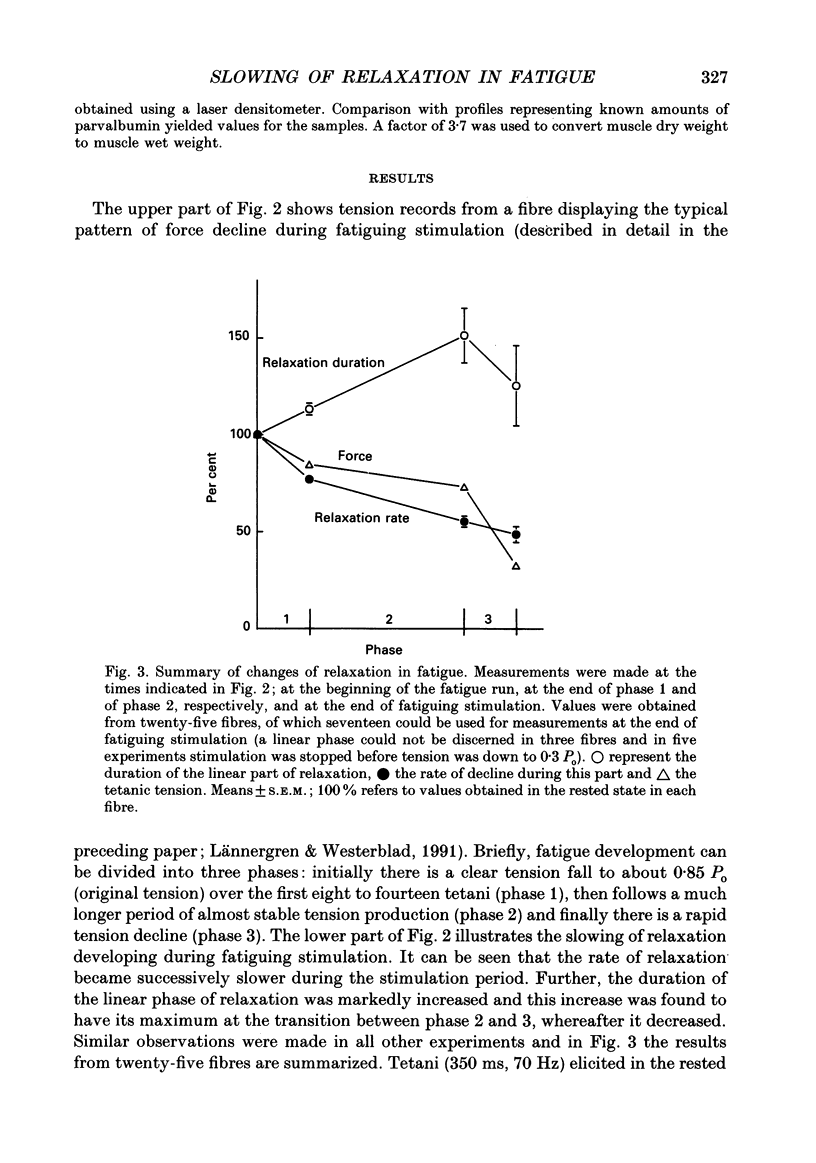
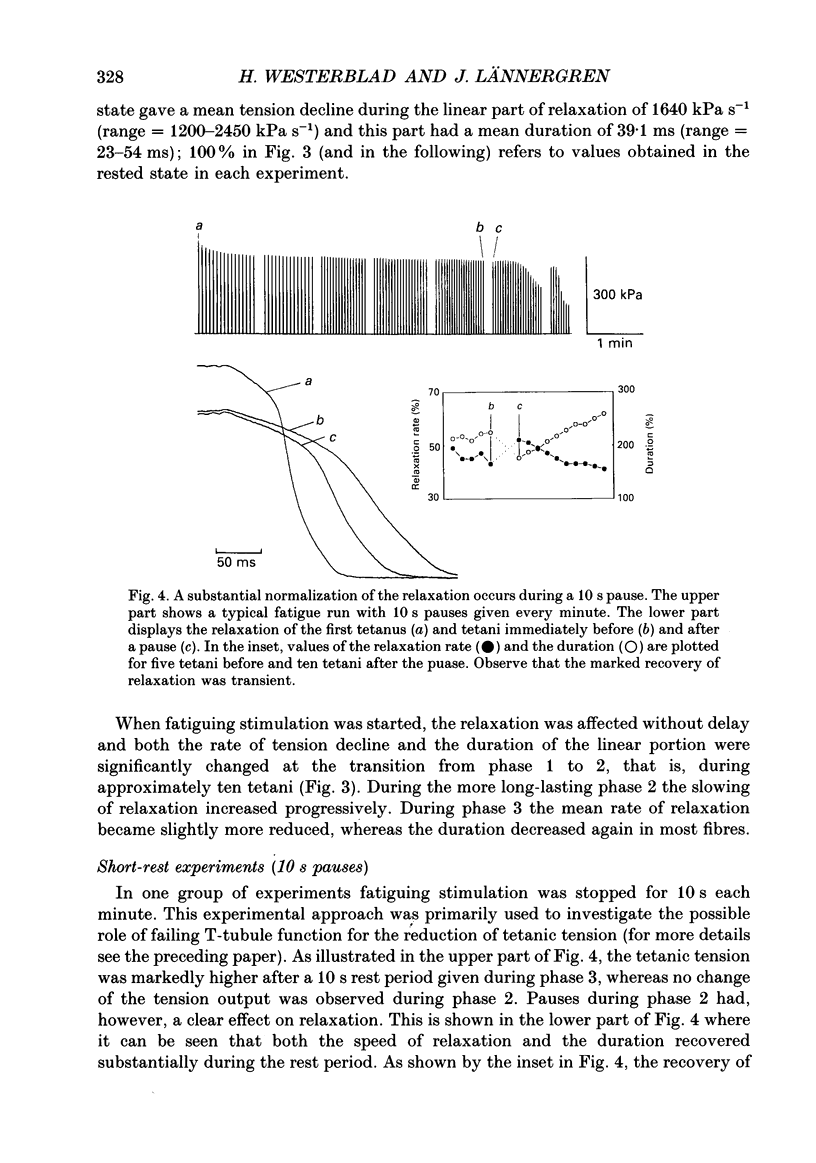
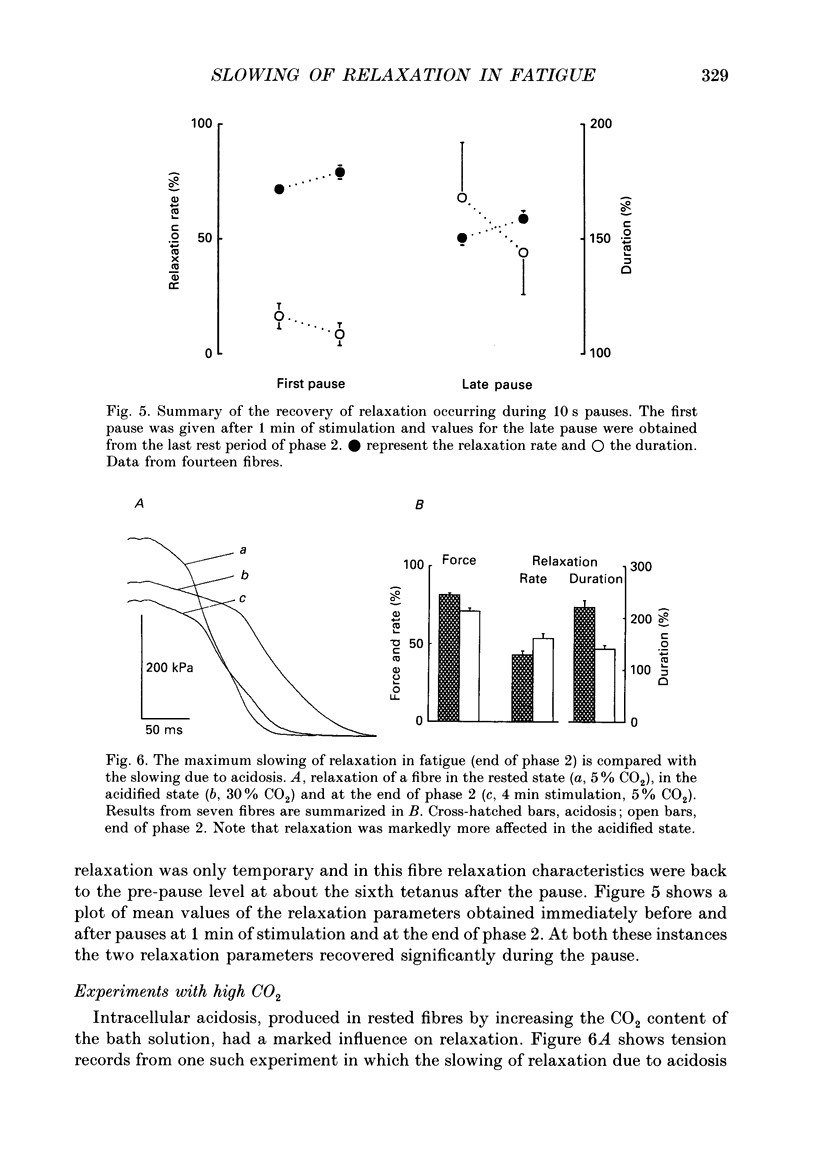
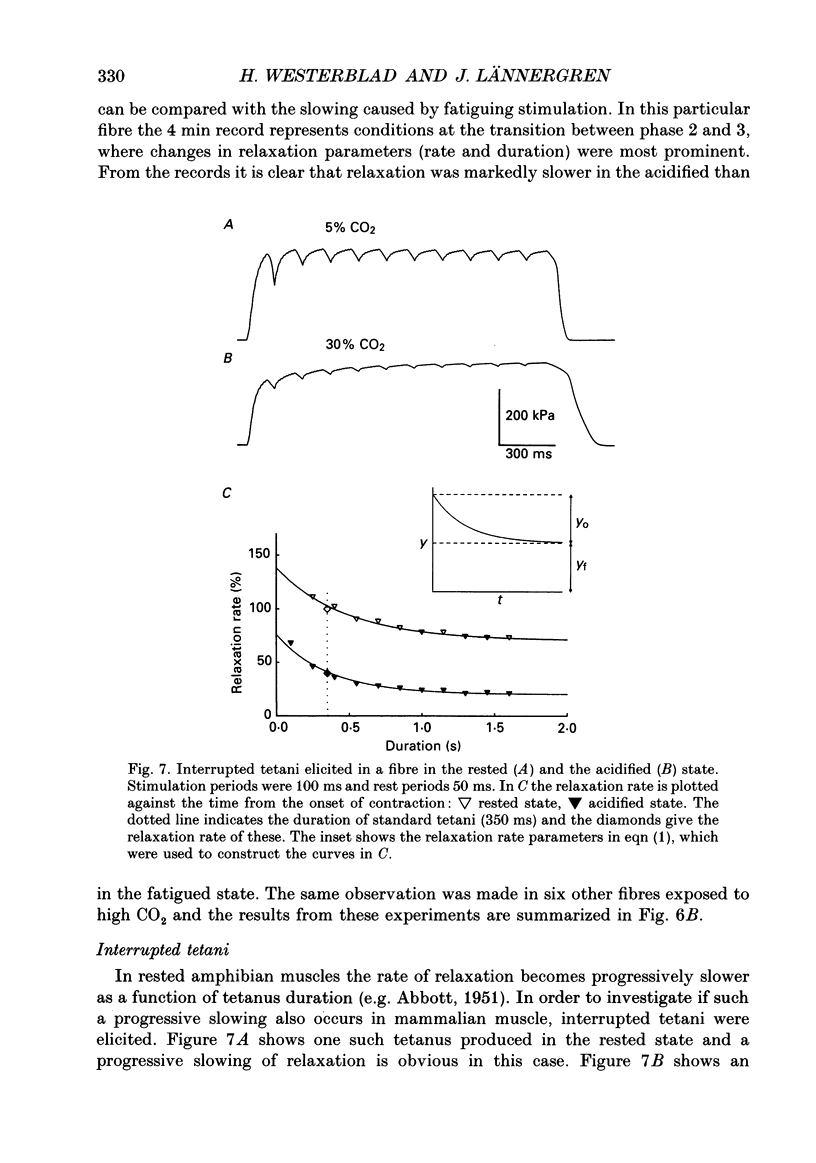
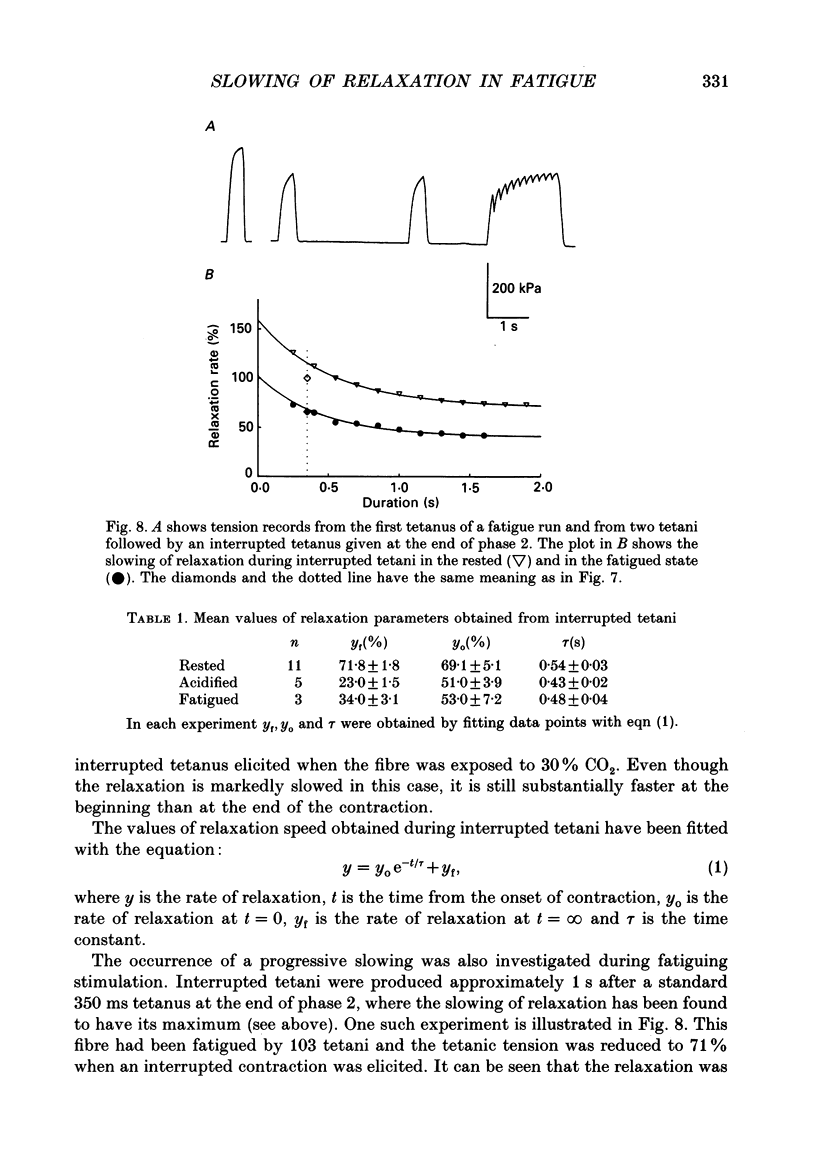
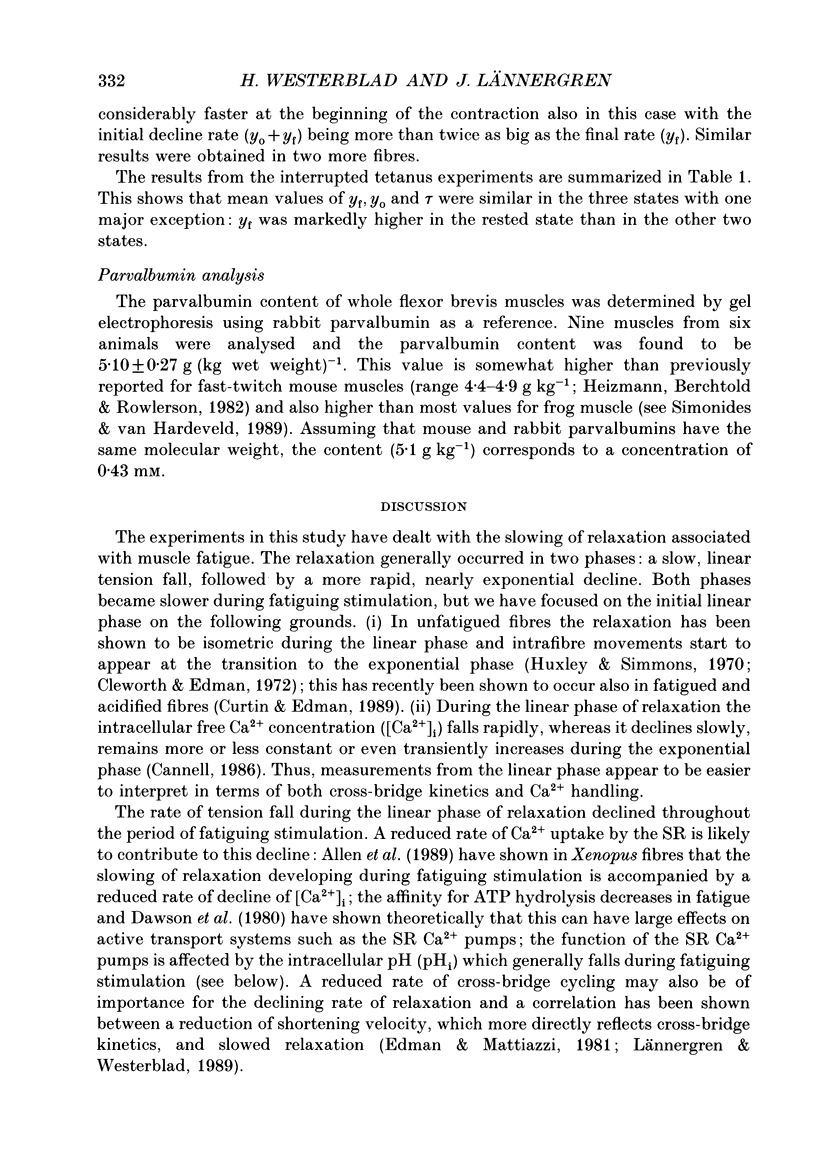
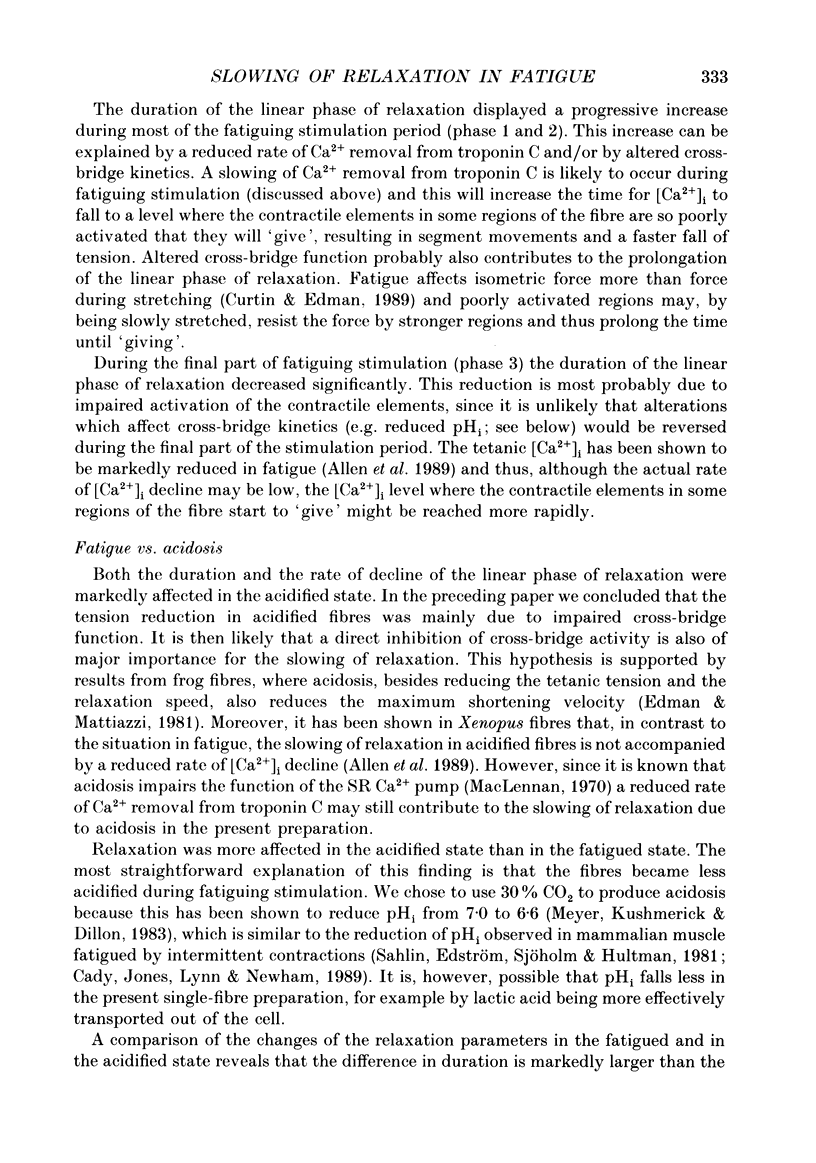
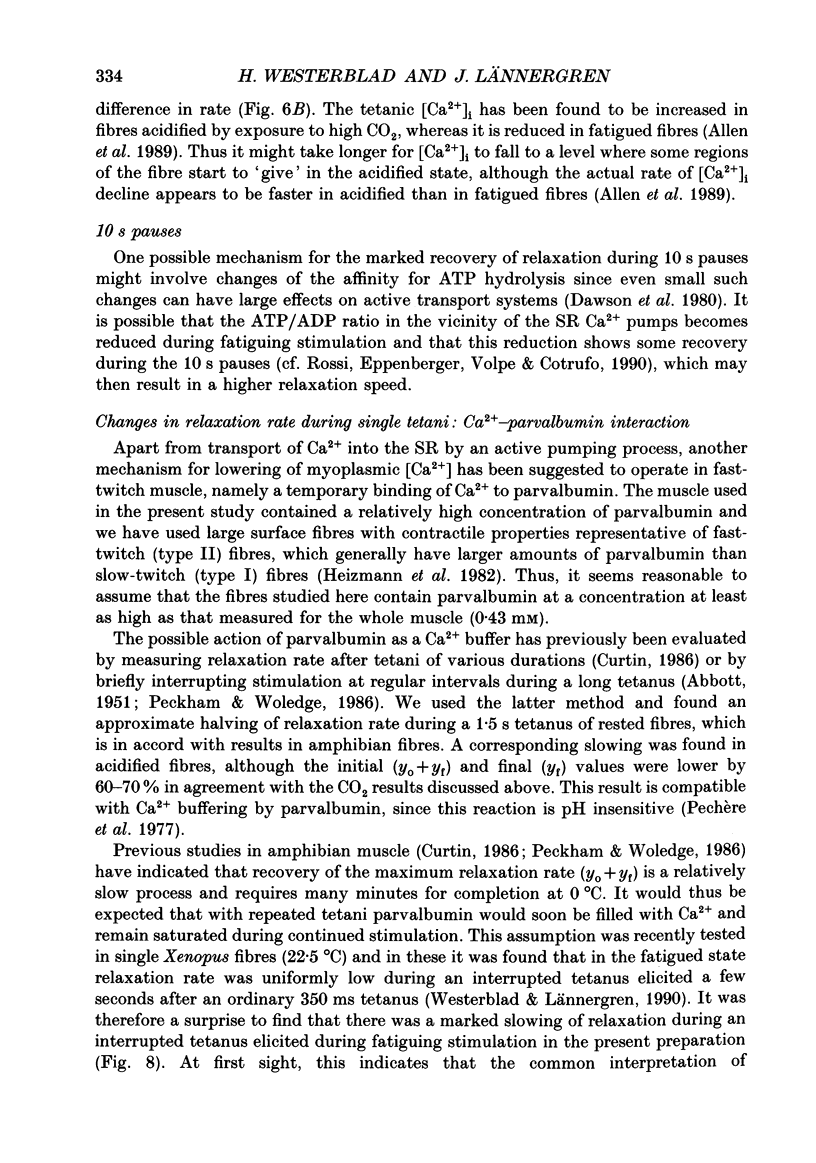
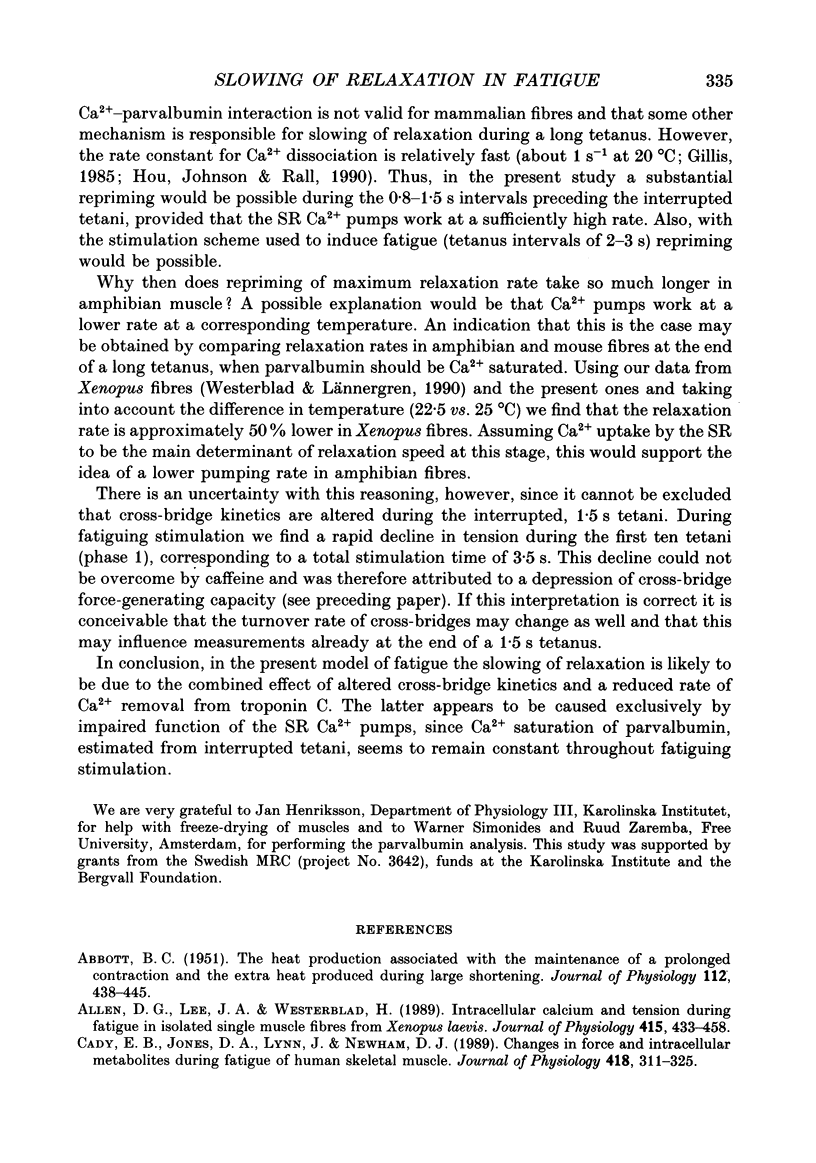
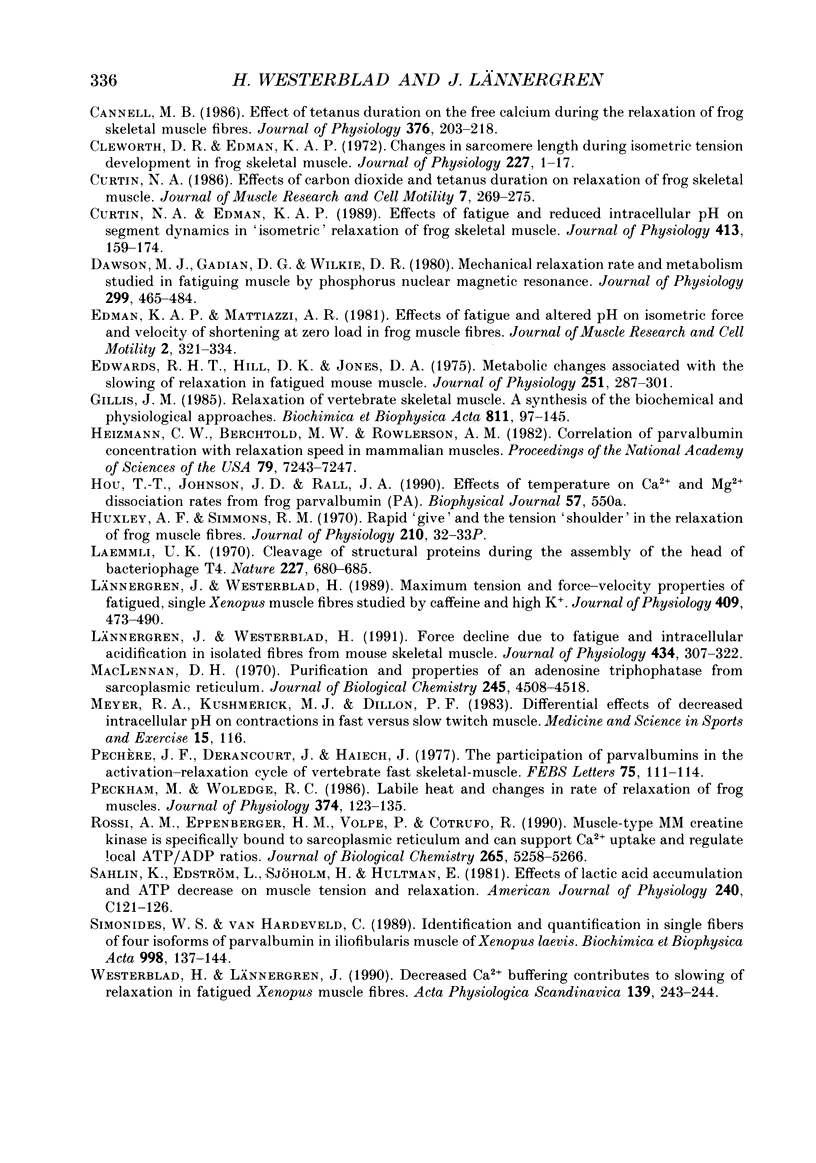
Selected References
These references are in PubMed. This may not be the complete list of references from this article.
- ABBOTT B. C. The heat production associated with the maintenance of a prolonged contraction and the extra heat produced during large shortening. J Physiol. 1951 Feb;112(3-4):438–445. doi: 10.1113/jphysiol.1951.sp004541. [DOI] [PMC free article] [PubMed] [Google Scholar]
- Allen D. G., Lee J. A., Westerblad H. Intracellular calcium and tension during fatigue in isolated single muscle fibres from Xenopus laevis. J Physiol. 1989 Aug;415:433–458. doi: 10.1113/jphysiol.1989.sp017730. [DOI] [PMC free article] [PubMed] [Google Scholar]
- Cady E. B., Jones D. A., Lynn J., Newham D. J. Changes in force and intracellular metabolites during fatigue of human skeletal muscle. J Physiol. 1989 Nov;418:311–325. doi: 10.1113/jphysiol.1989.sp017842. [DOI] [PMC free article] [PubMed] [Google Scholar]
- Cannell M. B. Effect of tetanus duration on the free calcium during the relaxation of frog skeletal muscle fibres. J Physiol. 1986 Jul;376:203–218. doi: 10.1113/jphysiol.1986.sp016149. [DOI] [PMC free article] [PubMed] [Google Scholar]
- Cleworth D. R., Edman K. A. Changes in sarcomere length during isometric tension development in frog skeletal muscle. J Physiol. 1972 Dec;227(1):1–17. doi: 10.1113/jphysiol.1972.sp010016. [DOI] [PMC free article] [PubMed] [Google Scholar]
- Curtin N. A., Edman K. A. Effects of fatigue and reduced intracellular pH on segment dynamics in 'isometric' relaxation of frog muscle fibres. J Physiol. 1989 Jun;413:159–174. doi: 10.1113/jphysiol.1989.sp017647. [DOI] [PMC free article] [PubMed] [Google Scholar]
- Curtin N. A. Effects of carbon dioxide and tetanus duration on relaxation of frog skeletal muscle. J Muscle Res Cell Motil. 1986 Jun;7(3):269–275. doi: 10.1007/BF01753560. [DOI] [PubMed] [Google Scholar]
- Dawson M. J., Gadian D. G., Wilkie D. R. Mechanical relaxation rate and metabolism studied in fatiguing muscle by phosphorus nuclear magnetic resonance. J Physiol. 1980 Feb;299:465–484. doi: 10.1113/jphysiol.1980.sp013137. [DOI] [PMC free article] [PubMed] [Google Scholar]
- Edman K. A., Mattiazzi A. R. Effects of fatigue and altered pH on isometric force and velocity of shortening at zero load in frog muscle fibres. J Muscle Res Cell Motil. 1981 Sep;2(3):321–334. doi: 10.1007/BF00713270. [DOI] [PubMed] [Google Scholar]
- Edwards R. H., Hill D. K., Jones D. A. Metabolic changes associated with the slowing of relaxation in fatigued mouse muscle. J Physiol. 1975 Oct;251(2):287–301. doi: 10.1113/jphysiol.1975.sp011093. [DOI] [PMC free article] [PubMed] [Google Scholar]
- Gillis J. M. Relaxation of vertebrate skeletal muscle. A synthesis of the biochemical and physiological approaches. Biochim Biophys Acta. 1985 Jun 3;811(2):97–145. doi: 10.1016/0304-4173(85)90016-3. [DOI] [PubMed] [Google Scholar]
- Heizmann C. W., Berchtold M. W., Rowlerson A. M. Correlation of parvalbumin concentration with relaxation speed in mammalian muscles. Proc Natl Acad Sci U S A. 1982 Dec;79(23):7243–7247. doi: 10.1073/pnas.79.23.7243. [DOI] [PMC free article] [PubMed] [Google Scholar]
- Huxley A. F., Simmons R. M. Rapid 'give' and the tension 'shoulder' in the relaxation of frog muscle fibres. J Physiol. 1970 Sep;210(1):32P–33P. [PubMed] [Google Scholar]
- Laemmli U. K. Cleavage of structural proteins during the assembly of the head of bacteriophage T4. Nature. 1970 Aug 15;227(5259):680–685. doi: 10.1038/227680a0. [DOI] [PubMed] [Google Scholar]
- Lännergren J., Westerblad H. Force decline due to fatigue and intracellular acidification in isolated fibres from mouse skeletal muscle. J Physiol. 1991 Mar;434:307–322. doi: 10.1113/jphysiol.1991.sp018471. [DOI] [PMC free article] [PubMed] [Google Scholar]
- Lännergren J., Westerblad H. Maximum tension and force-velocity properties of fatigued, single Xenopus muscle fibres studied by caffeine and high K+. J Physiol. 1989 Feb;409:473–490. doi: 10.1113/jphysiol.1989.sp017508. [DOI] [PMC free article] [PubMed] [Google Scholar]
- MacLennan D. H. Purification and properties of an adenosine triphosphatase from sarcoplasmic reticulum. J Biol Chem. 1970 Sep 10;245(17):4508–4518. [PubMed] [Google Scholar]
- Pechère J. F., Derancourt J., Haiech J. The participation of parvalbumins in the activation-relaxation cycle of vertebrate fast skeletal-muscle. FEBS Lett. 1977 Mar 15;75(1):111–114. doi: 10.1016/0014-5793(77)80064-1. [DOI] [PubMed] [Google Scholar]
- Peckham M., Woledge R. C. Labile heat and changes in rate of relaxation of frog muscles. J Physiol. 1986 May;374:123–135. doi: 10.1113/jphysiol.1986.sp016070. [DOI] [PMC free article] [PubMed] [Google Scholar]
- Rossi A. M., Eppenberger H. M., Volpe P., Cotrufo R., Wallimann T. Muscle-type MM creatine kinase is specifically bound to sarcoplasmic reticulum and can support Ca2+ uptake and regulate local ATP/ADP ratios. J Biol Chem. 1990 Mar 25;265(9):5258–5266. [PubMed] [Google Scholar]
- Sahlin K., Edström L., Sjöholm H., Hultman E. Effects of lactic acid accumulation and ATP decrease on muscle tension and relaxation. Am J Physiol. 1981 Mar;240(3):C121–C126. doi: 10.1152/ajpcell.1981.240.3.C121. [DOI] [PubMed] [Google Scholar]
- Simonides W. S., van Hardeveld C. Identification and quantification in single muscle fibers of four isoforms of parvalbumin in the iliofibularis muscle of Xenopus laevis. Biochim Biophys Acta. 1989 Oct 5;998(2):137–144. doi: 10.1016/0167-4838(89)90265-3. [DOI] [PubMed] [Google Scholar]
- Westerblad H., Lännergren J. Decreased Ca2-buffering contributes to slowing of relaxation in fatigued Xenopus muscle fibres. Acta Physiol Scand. 1990 May;139(1):243–244. doi: 10.1111/j.1748-1716.1990.tb08918.x. [DOI] [PubMed] [Google Scholar]