Abstract
1. The patch clamp technique was used to study the effects of internal and external pH on the Ca(2+)- and voltage-activated maxi K+ channel present in the apical membrane of Necturus gall-bladder epithelial cells. 2. When the pH of the solution bathing the cytosolic side of inside-out patches (pHi) was lowered from 7.9 to 6.9, with internal free Ca2+ concentration ([Ca2+]i) buffered below saturation levels for the channel gating sites, channel open probability (Po) decreased. At saturating Ca2+ concentrations, Po was near 1.0, and unaffected by pHi. The results are consistent with a competitive interaction between Ca2+ and H+ at regulatory binding sites. Kinetic analysis assuming competitive binding yields a Hill coefficient for H+ of 1.3. 3. At sub-maximal [Ca2+]i, changing the pH of the solution bathing the extracellular surface of the patch (pHo) between 8 and 7, had no effect on maxi K+ channel Po, but lowering pHo to 6 or 5 significantly reduced Po. At saturating [Ca2+]i, Po was independent of pHo. 4. There were no effects of either pHi or pHo on single-channel conductance. 5. Inasmuch as reductions in either pHo or pHi decrease maxi K+ channel Po, changes in maxi K+ channel activity account in part for the reduction of apical membrane K+ conductance elicited by acidification of the bathing medium. The dominant effect of pH on maxi K+ channels is on the cytosolic surface of the membrane. 6. The change in Po elicited by small changes in [H+]i (delta Po/delta [H+]i) is -7.6 microM-1, compared to delta Po/delta [Ca2+]i = 2.6 microM-1, both at Vm = -30 mV and at physiological intracellular [H+] and [Ca2+]. This implies that [H+]i and [Ca2+]i have opposite effects on channel Po at physiological levels and underlines the importance of pHi in channel gating.
Full text
PDF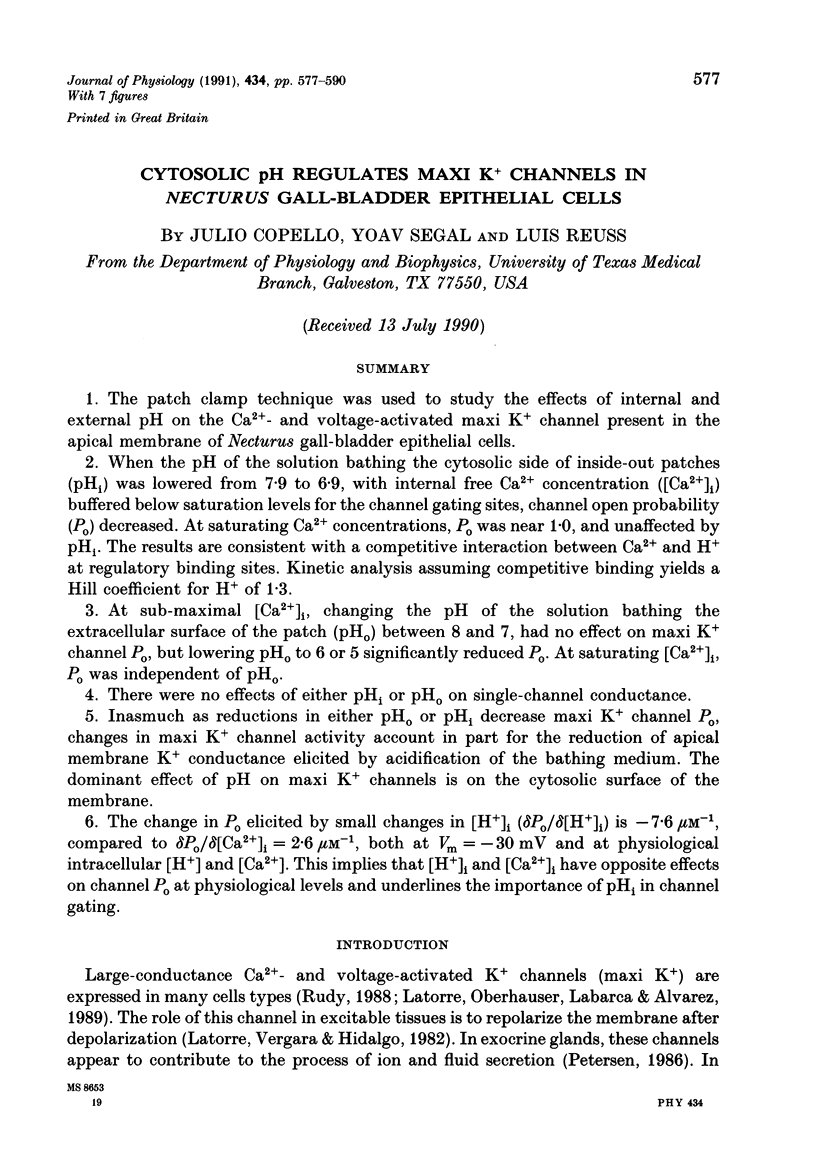
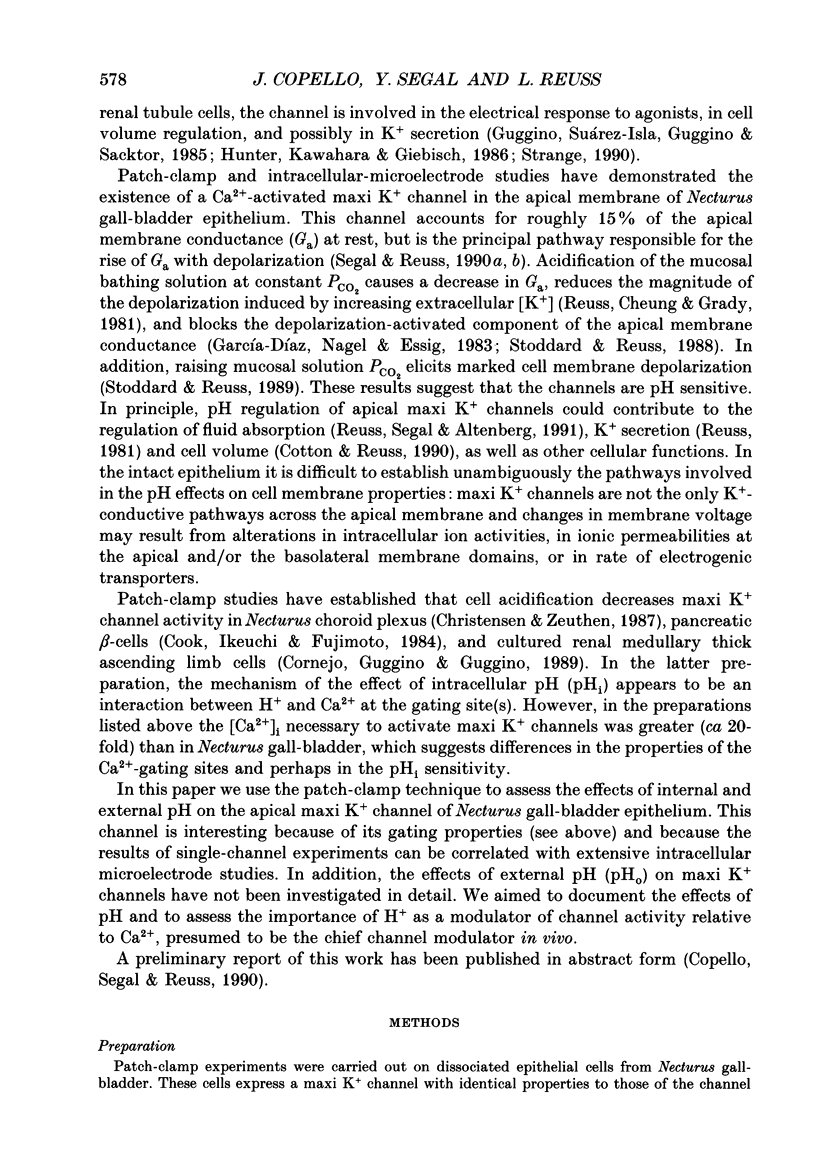
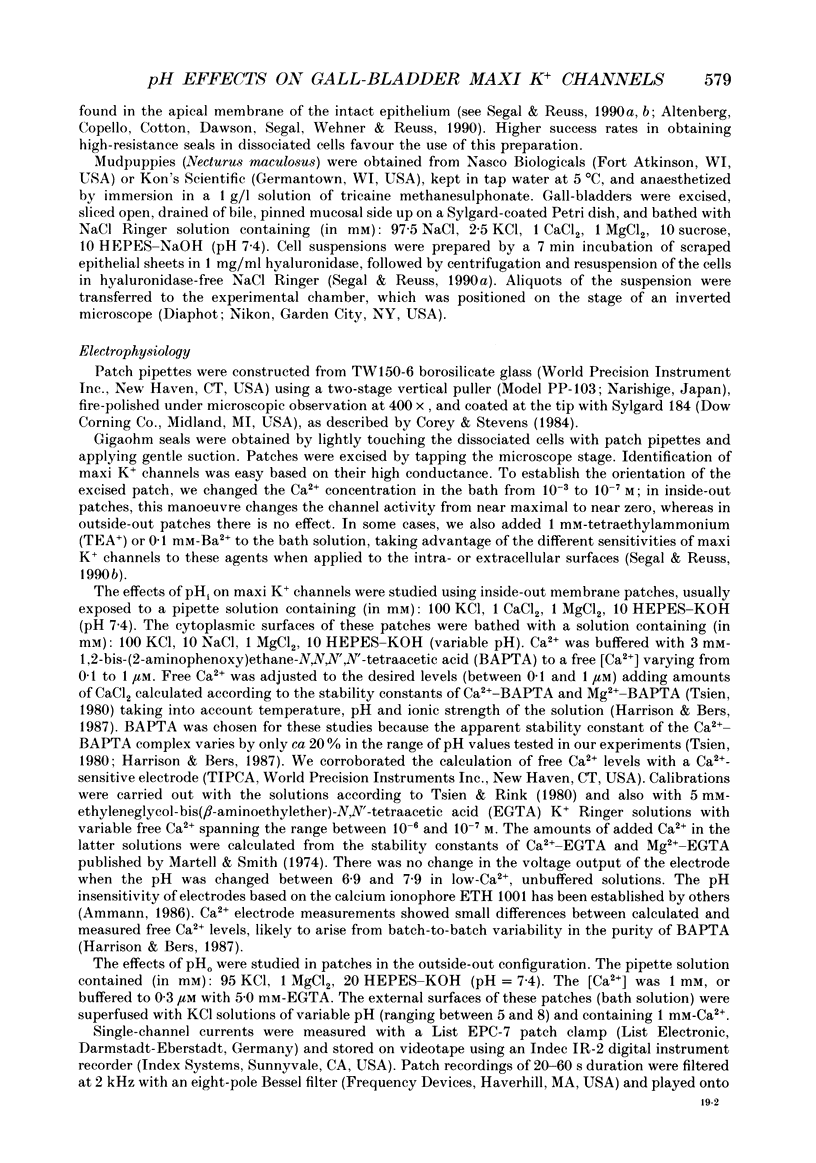
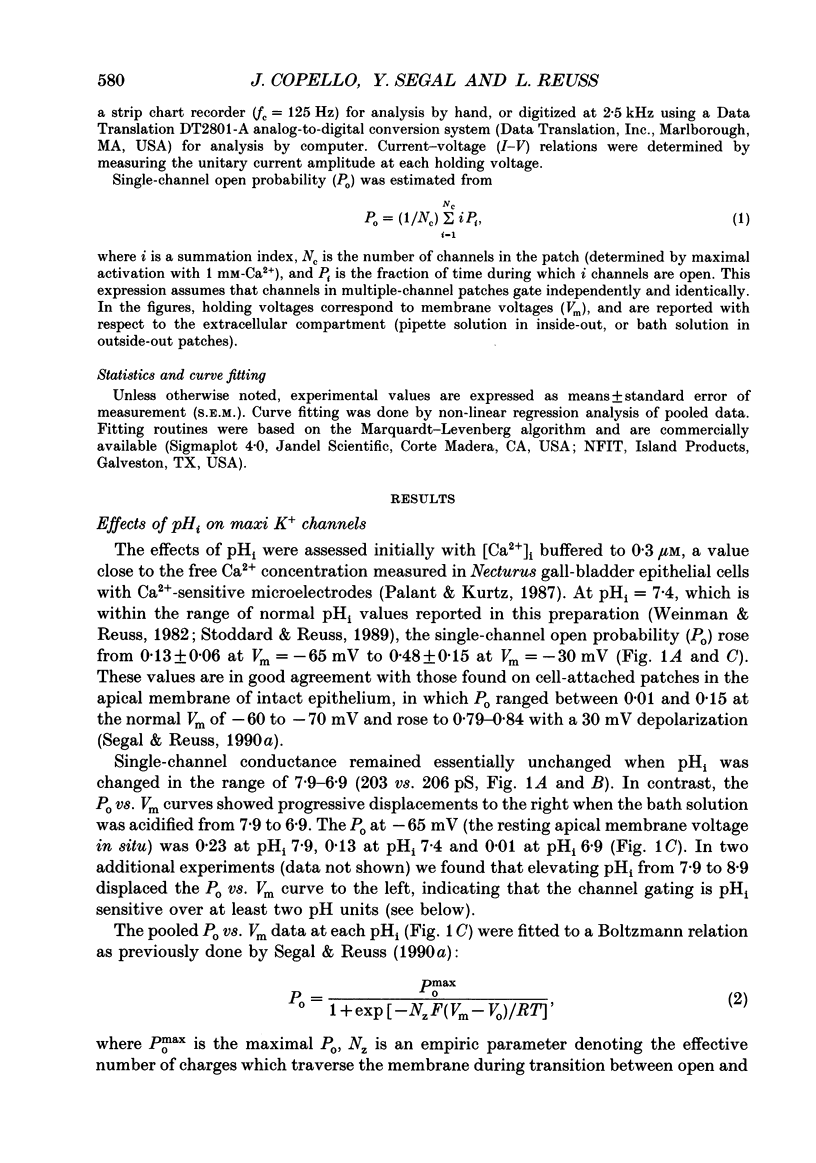
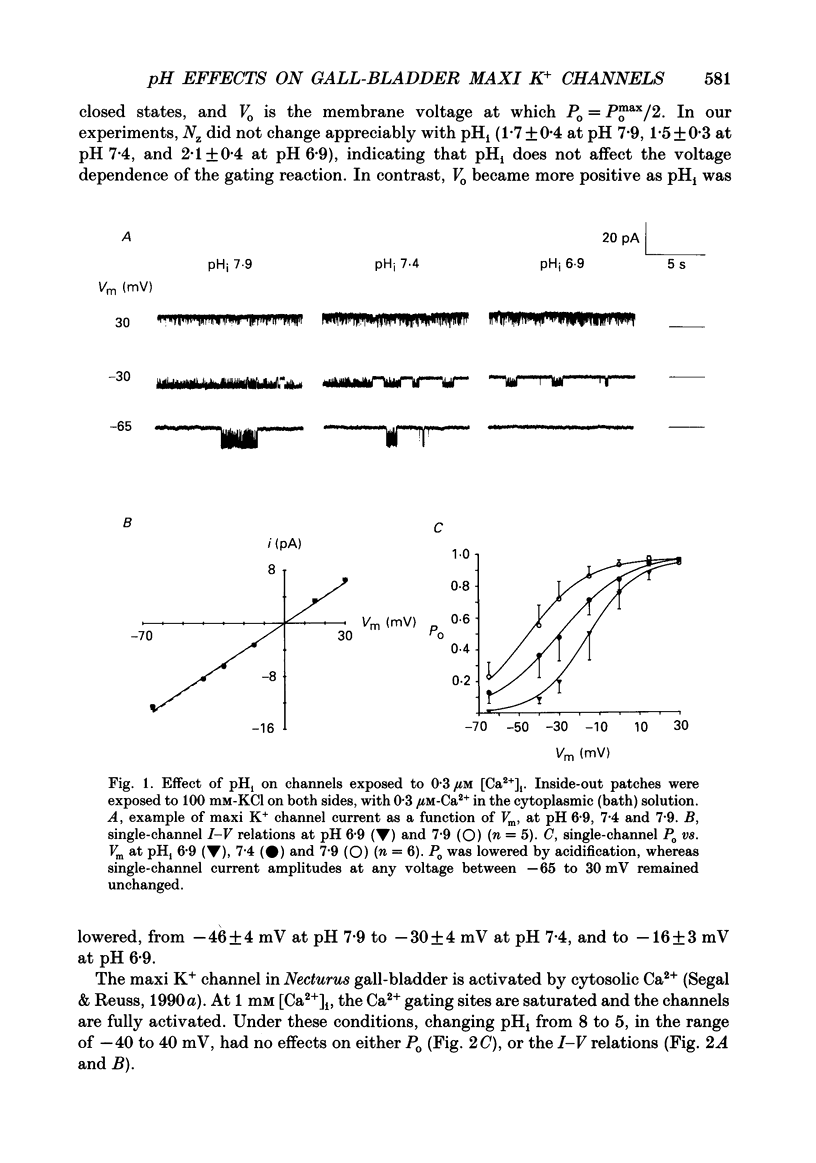
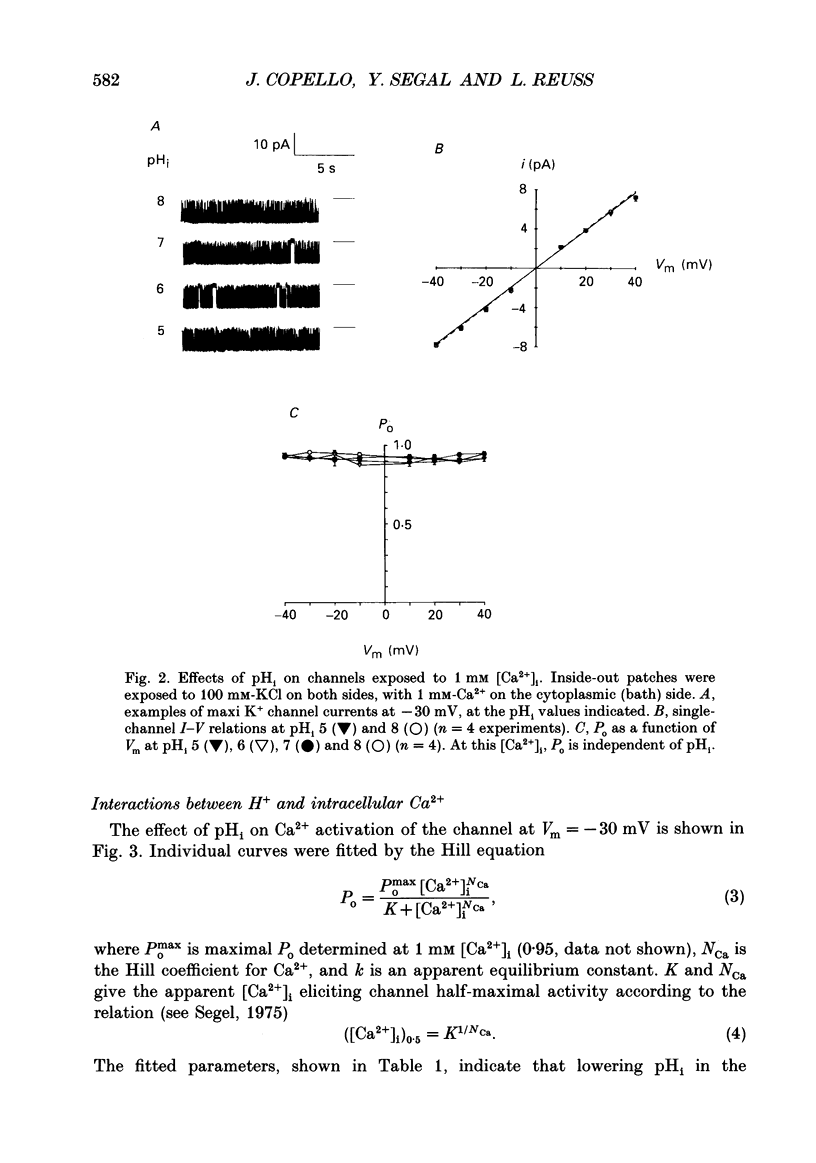
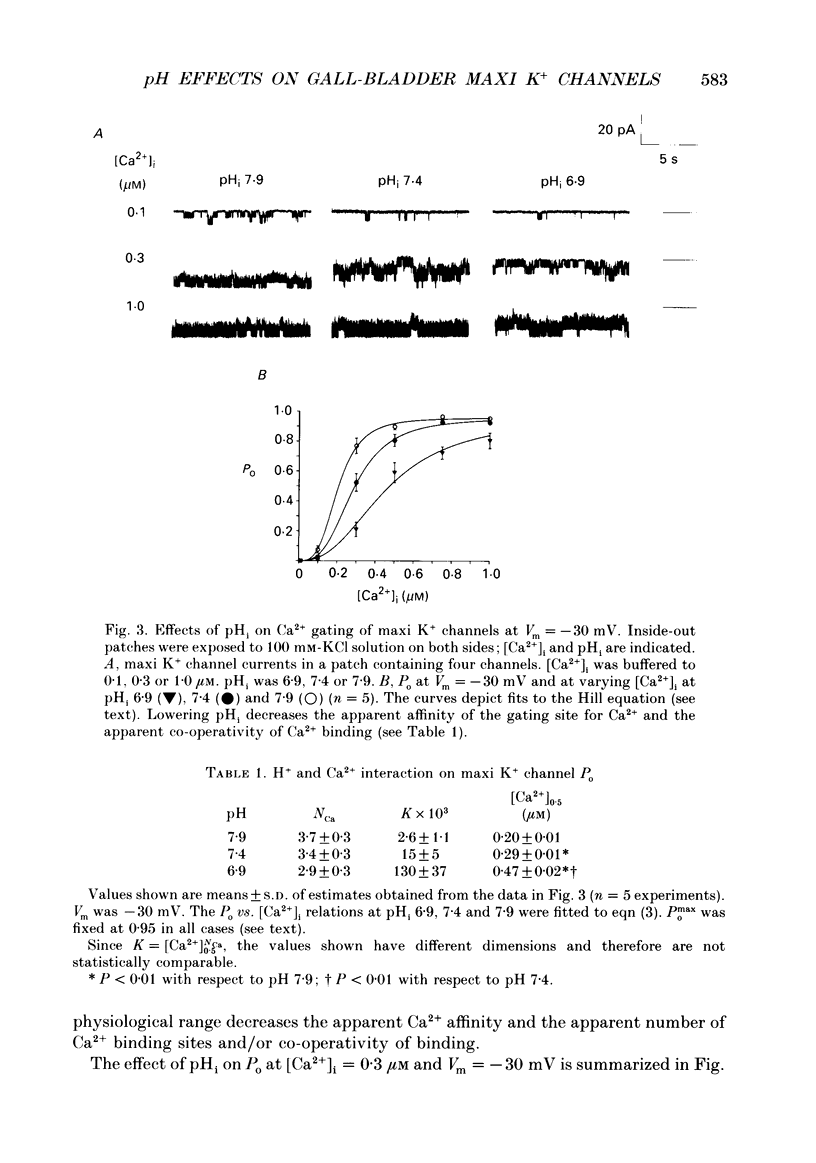
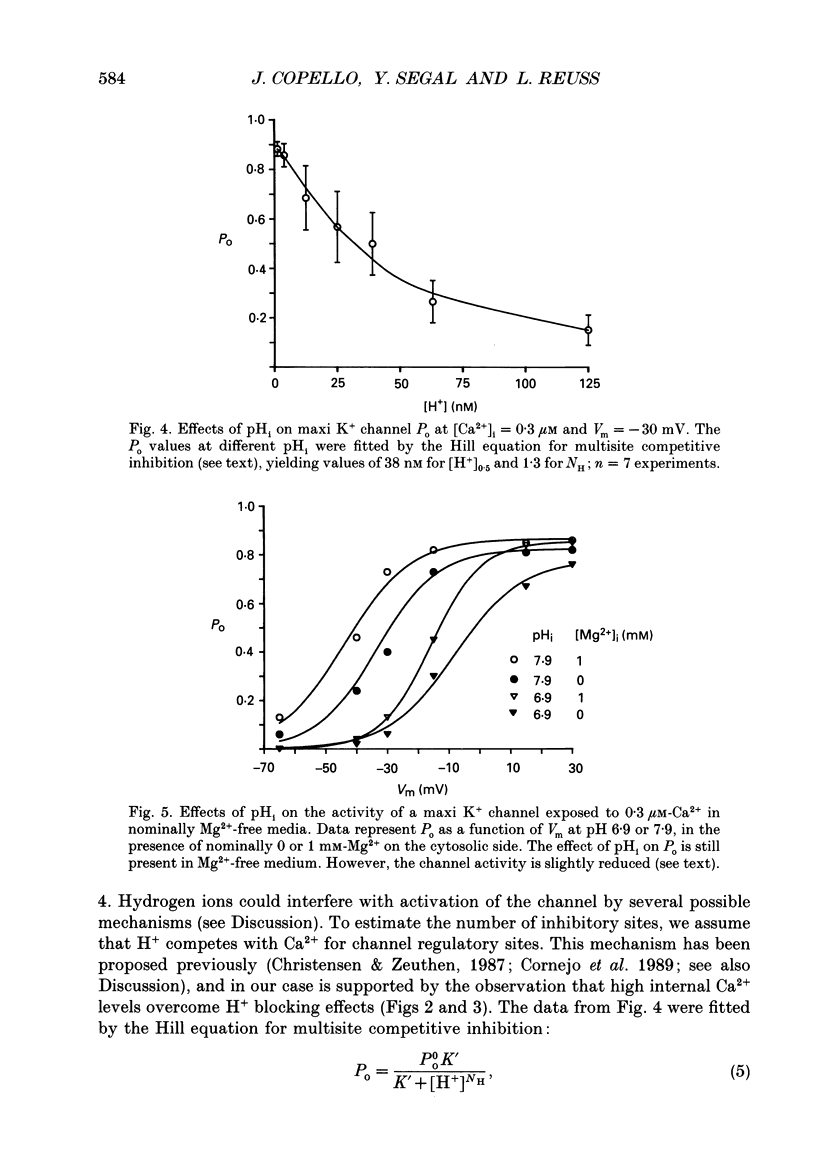
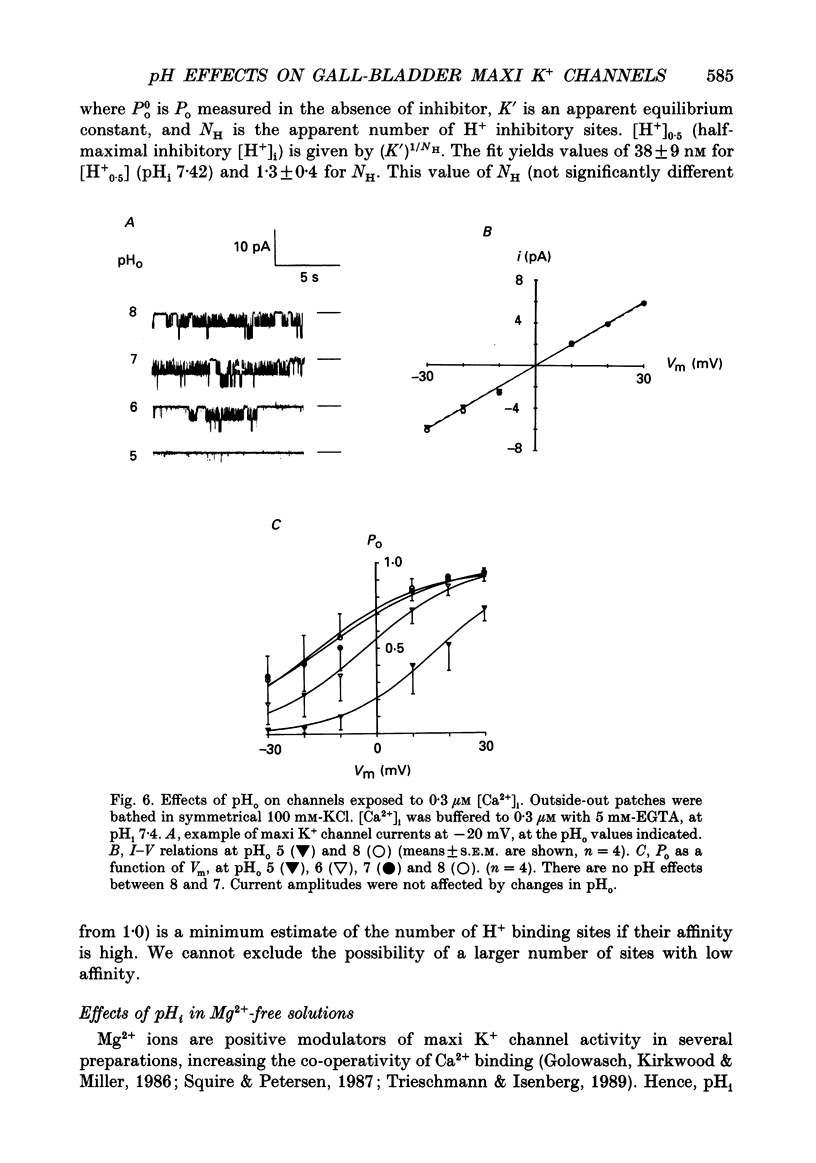
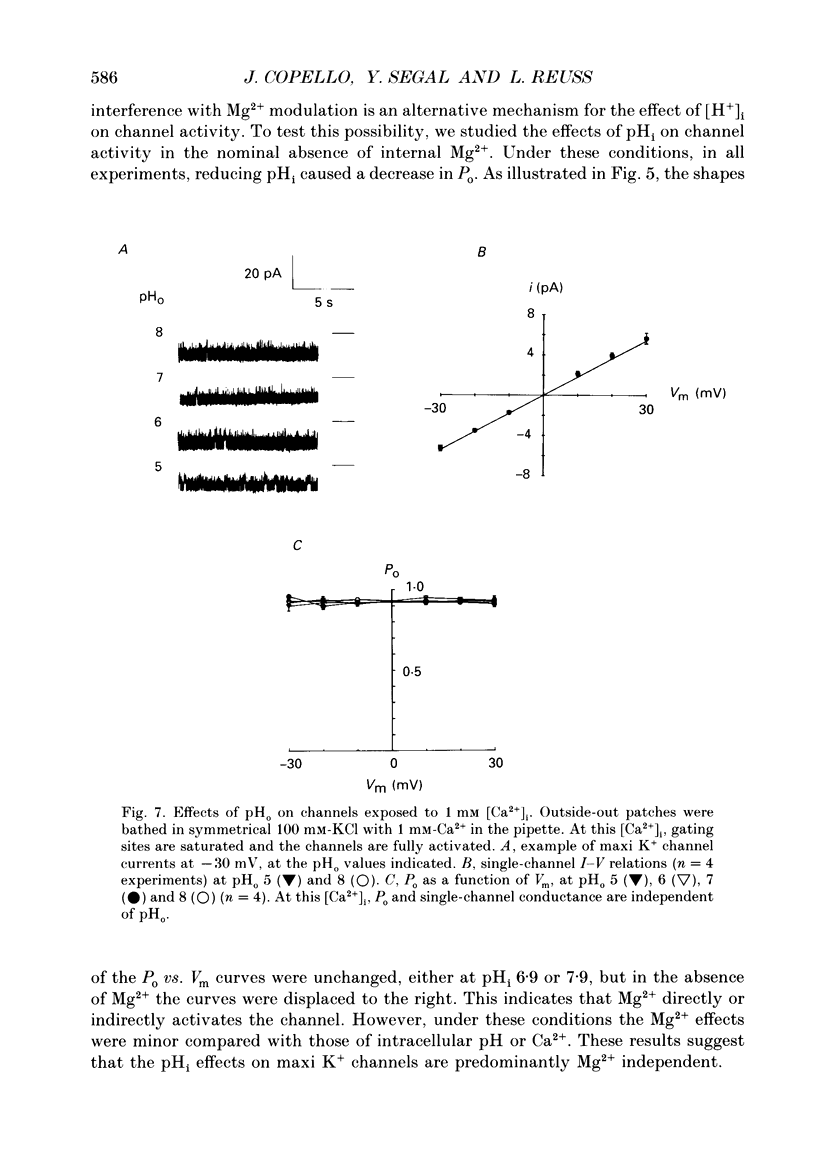
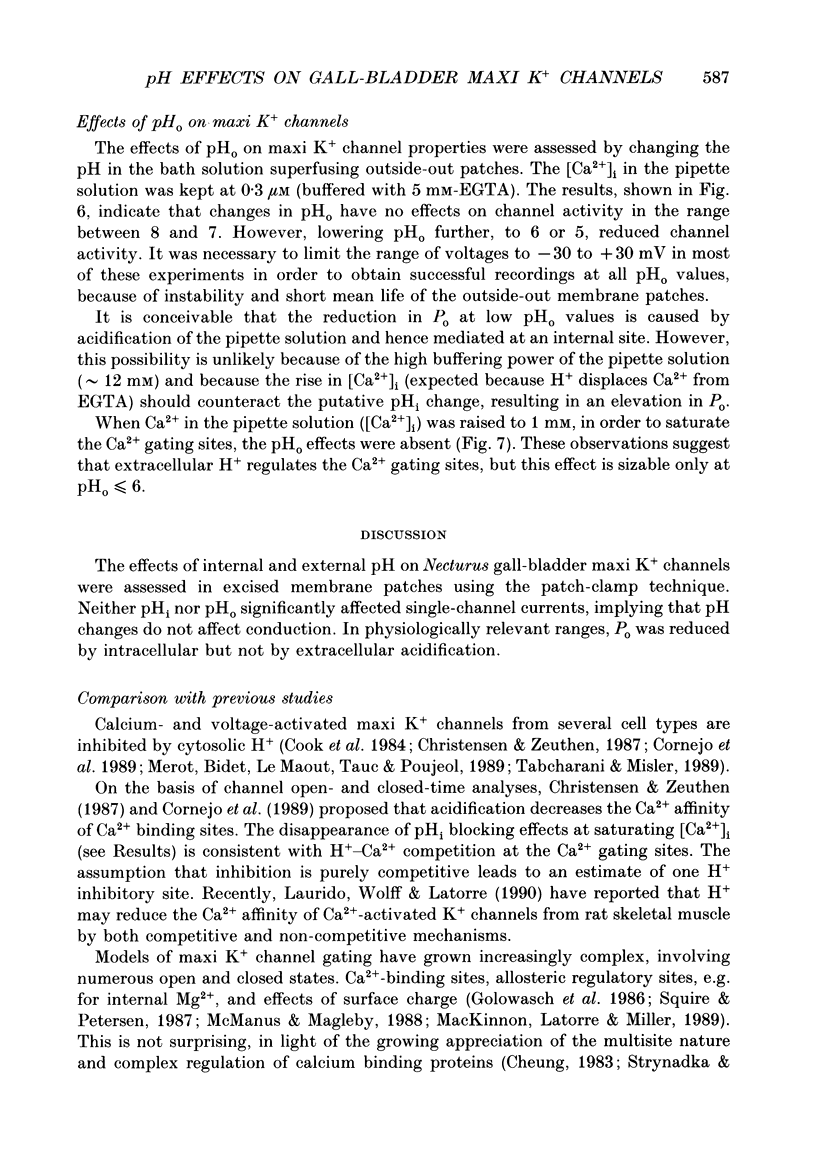
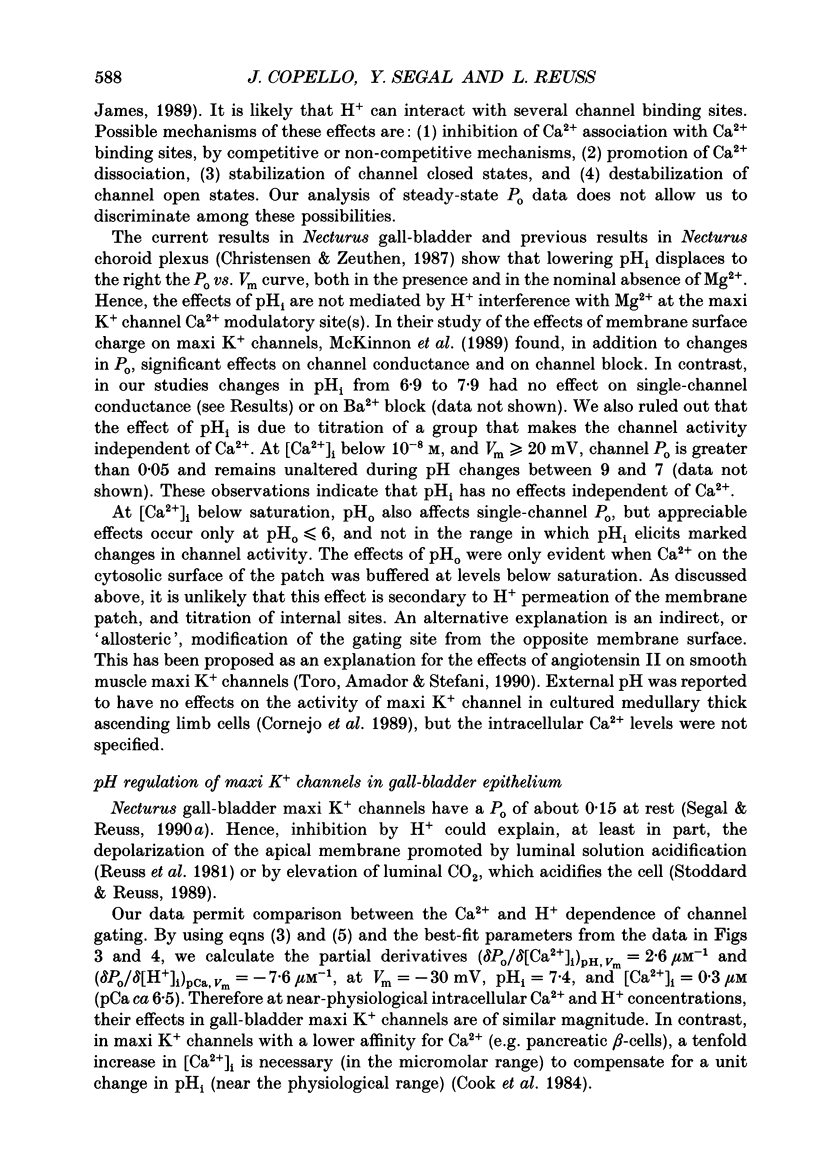
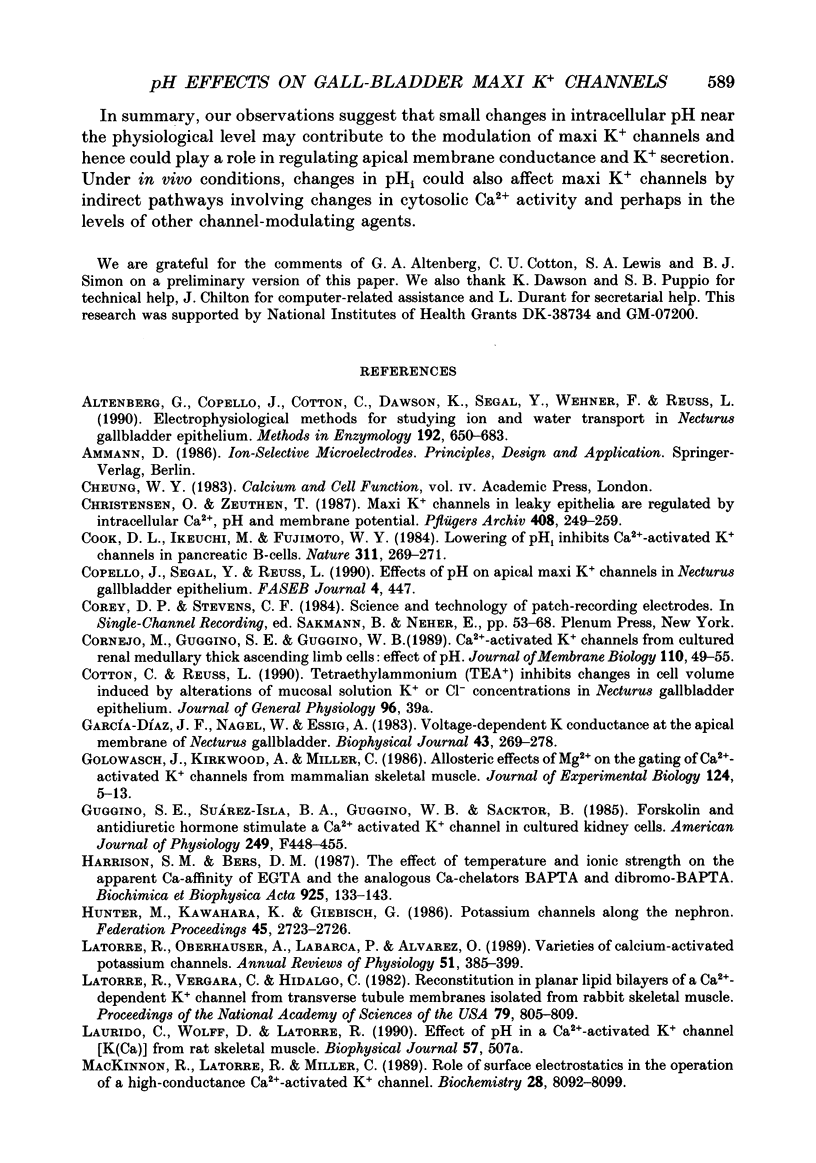
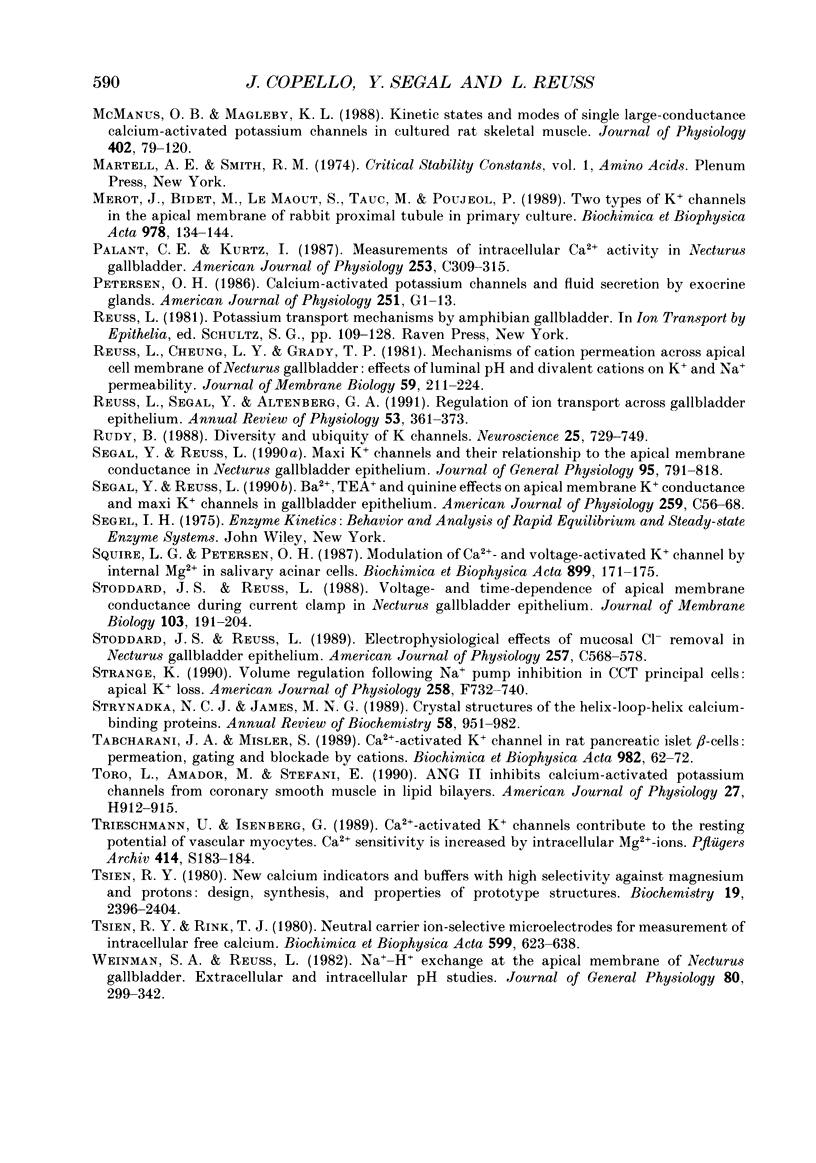
Selected References
These references are in PubMed. This may not be the complete list of references from this article.
- Altenberg G., Copello J., Cotton C., Dawson K., Segal Y., Wehner F., Reuss L. Electrophysiological methods for studying ion and water transport in Necturus gall bladder epithelium. Methods Enzymol. 1990;192:650–683. doi: 10.1016/0076-6879(90)92101-i. [DOI] [PubMed] [Google Scholar]
- Christensen O., Zeuthen T. Maxi K+ channels in leaky epithelia are regulated by intracellular Ca2+, pH and membrane potential. Pflugers Arch. 1987 Mar;408(3):249–259. doi: 10.1007/BF02181467. [DOI] [PubMed] [Google Scholar]
- Cook D. L., Ikeuchi M., Fujimoto W. Y. Lowering of pHi inhibits Ca2+-activated K+ channels in pancreatic B-cells. Nature. 1984 Sep 20;311(5983):269–271. doi: 10.1038/311269a0. [DOI] [PubMed] [Google Scholar]
- Cornejo M., Guggino S. E., Guggino W. B. Ca2+-activated K+ channels from cultured renal medullary thick ascending limb cells: effects of pH. J Membr Biol. 1989 Aug;110(1):49–55. doi: 10.1007/BF01870992. [DOI] [PubMed] [Google Scholar]
- García-Díaz J. F., Nagel W., Essig A. Voltage-dependent K conductance at the apical membrane of Necturus gallbladder. Biophys J. 1983 Sep;43(3):269–278. doi: 10.1016/S0006-3495(83)84350-1. [DOI] [PMC free article] [PubMed] [Google Scholar]
- Golowasch J., Kirkwood A., Miller C. Allosteric effects of Mg2+ on the gating of Ca2+-activated K+ channels from mammalian skeletal muscle. J Exp Biol. 1986 Sep;124:5–13. doi: 10.1242/jeb.124.1.5. [DOI] [PubMed] [Google Scholar]
- Guggino S. E., Suarez-Isla B. A., Guggino W. B., Sacktor B. Forskolin and antidiuretic hormone stimulate a Ca2+-activated K+ channel in cultured kidney cells. Am J Physiol. 1985 Sep;249(3 Pt 2):F448–F455. doi: 10.1152/ajprenal.1985.249.3.F448. [DOI] [PubMed] [Google Scholar]
- Harrison S. M., Bers D. M. The effect of temperature and ionic strength on the apparent Ca-affinity of EGTA and the analogous Ca-chelators BAPTA and dibromo-BAPTA. Biochim Biophys Acta. 1987 Aug 13;925(2):133–143. doi: 10.1016/0304-4165(87)90102-4. [DOI] [PubMed] [Google Scholar]
- Hunter M., Kawahara K., Giebisch G. Potassium channels along the nephron. Fed Proc. 1986 Nov;45(12):2723–2726. [PubMed] [Google Scholar]
- Latorre R., Oberhauser A., Labarca P., Alvarez O. Varieties of calcium-activated potassium channels. Annu Rev Physiol. 1989;51:385–399. doi: 10.1146/annurev.ph.51.030189.002125. [DOI] [PubMed] [Google Scholar]
- Latorre R., Vergara C., Hidalgo C. Reconstitution in planar lipid bilayers of a Ca2+-dependent K+ channel from transverse tubule membranes isolated from rabbit skeletal muscle. Proc Natl Acad Sci U S A. 1982 Feb;79(3):805–809. doi: 10.1073/pnas.79.3.805. [DOI] [PMC free article] [PubMed] [Google Scholar]
- MacKinnon R., Latorre R., Miller C. Role of surface electrostatics in the operation of a high-conductance Ca2+-activated K+ channel. Biochemistry. 1989 Oct 3;28(20):8092–8099. doi: 10.1021/bi00446a020. [DOI] [PubMed] [Google Scholar]
- McManus O. B., Magleby K. L. Kinetic states and modes of single large-conductance calcium-activated potassium channels in cultured rat skeletal muscle. J Physiol. 1988 Aug;402:79–120. doi: 10.1113/jphysiol.1988.sp017195. [DOI] [PMC free article] [PubMed] [Google Scholar]
- Merot J., Bidet M., Le Maout S., Tauc M., Poujeol P. Two types of K+ channels in the apical membrane of rabbit proximal tubule in primary culture. Biochim Biophys Acta. 1989 Jan 16;978(1):134–144. doi: 10.1016/0005-2736(89)90508-7. [DOI] [PubMed] [Google Scholar]
- Palant C. E., Kurtz I. Measurement of intracellular Ca2+ activity in Necturus gallbladder. Am J Physiol. 1987 Aug;253(2 Pt 1):C309–C315. doi: 10.1152/ajpcell.1987.253.2.C309. [DOI] [PubMed] [Google Scholar]
- Petersen O. H. Calcium-activated potassium channels and fluid secretion by exocrine glands. Am J Physiol. 1986 Jul;251(1 Pt 1):G1–13. doi: 10.1152/ajpgi.1986.251.1.G1. [DOI] [PubMed] [Google Scholar]
- Reuss L., Cheung L. Y., Grady T. P. Mechanisms of cation permeation across apical cell membrane of Necturus gallbladder: effects of luminal pH and divalent cations on K+ and Na+ permeability. J Membr Biol. 1981 Apr 30;59(3):211–224. doi: 10.1007/BF01875426. [DOI] [PubMed] [Google Scholar]
- Reuss L., Segal Y., Altenberg G. Regulation of ion transport across gallbladder epithelium. Annu Rev Physiol. 1991;53:361–373. doi: 10.1146/annurev.ph.53.030191.002045. [DOI] [PubMed] [Google Scholar]
- Rudy B. Diversity and ubiquity of K channels. Neuroscience. 1988 Jun;25(3):729–749. doi: 10.1016/0306-4522(88)90033-4. [DOI] [PubMed] [Google Scholar]
- Segal Y., Reuss L. Maxi K+ channels and their relationship to the apical membrane conductance in Necturus gallbladder epithelium. J Gen Physiol. 1990 May;95(5):791–818. doi: 10.1085/jgp.95.5.791. [DOI] [PMC free article] [PubMed] [Google Scholar]
- Squire L. G., Petersen O. H. Modulation of Ca2+- and voltage-activated K+ channels by internal Mg2+ in salivary acinar cells. Biochim Biophys Acta. 1987 May 29;899(2):171–175. doi: 10.1016/0005-2736(87)90397-x. [DOI] [PubMed] [Google Scholar]
- Stoddard J. S., Reuss L. Electrophysiological effects of mucosal Cl- removal in Necturus gallbladder epithelium. Am J Physiol. 1989 Sep;257(3 Pt 1):C568–C578. doi: 10.1152/ajpcell.1989.257.3.C568. [DOI] [PubMed] [Google Scholar]
- Stoddard J. S., Reuss L. Voltage- and time dependence of apical membrane conductance during current clamp in Necturus gallbladder epithelium. J Membr Biol. 1988 Jul;103(2):191–204. doi: 10.1007/BF01870949. [DOI] [PubMed] [Google Scholar]
- Strange K. Volume regulation following Na+ pump inhibition in CCT principal cells: apical K+ loss. Am J Physiol. 1990 Mar;258(3 Pt 2):F732–F740. doi: 10.1152/ajprenal.1990.258.3.F732. [DOI] [PubMed] [Google Scholar]
- Strynadka N. C., James M. N. Crystal structures of the helix-loop-helix calcium-binding proteins. Annu Rev Biochem. 1989;58:951–998. doi: 10.1146/annurev.bi.58.070189.004511. [DOI] [PubMed] [Google Scholar]
- Tabcharani J. A., Misler S. Ca2+-activated K+ channel in rat pancreatic islet B cells: permeation, gating and blockade by cations. Biochim Biophys Acta. 1989 Jun 26;982(1):62–72. doi: 10.1016/0005-2736(89)90174-0. [DOI] [PubMed] [Google Scholar]
- Toro L., Amador M., Stefani E. ANG II inhibits calcium-activated potassium channels from coronary smooth muscle in lipid bilayers. Am J Physiol. 1990 Mar;258(3 Pt 2):H912–H915. doi: 10.1152/ajpheart.1990.258.3.H912. [DOI] [PubMed] [Google Scholar]
- Trieschmann U., Isenberg G. Ca2+-activated K+ channels contribute to the resting potential of vascular myocytes. Ca2+-sensitivity is increased by intracellular Mg2+-ions. Pflugers Arch. 1989;414 (Suppl 1):S183–S184. doi: 10.1007/BF00582296. [DOI] [PubMed] [Google Scholar]
- Tsien R. Y. New calcium indicators and buffers with high selectivity against magnesium and protons: design, synthesis, and properties of prototype structures. Biochemistry. 1980 May 27;19(11):2396–2404. doi: 10.1021/bi00552a018. [DOI] [PubMed] [Google Scholar]
- Tsien R. Y., Rink T. J. Neutral carrier ion-selective microelectrodes for measurement of intracellular free calcium. Biochim Biophys Acta. 1980 Jul;599(2):623–638. doi: 10.1016/0005-2736(80)90205-9. [DOI] [PubMed] [Google Scholar]
- Weinman S. A., Reuss L. Na+-H+ exchange at the apical membrane of Necturus gallbladder. Extracellular and intracellular pH studies. J Gen Physiol. 1982 Aug;80(2):299–321. doi: 10.1085/jgp.80.2.299. [DOI] [PMC free article] [PubMed] [Google Scholar]