Abstract
1. Patch-clamp and computer-modelling techniques were used to study the activation of Na(+)-activated K+ channels (IK(Na] in dissociated neurones from the embryonic chick ciliary ganglion and the embryonic chick brain stem. 2. Numerical solutions of diffusion equations suggested that Na+ accumulation as a result of Na+ influx through voltage-sensitive Na+ channels (INa) is insufficient to allow for alteration in the gating of IK(Na) channels. 3. Whole-cell recordings using two independent micropipettes were made from chick ciliary-ganglion neurones. These showed that transient outward currents were present only when there were clear indications of incomplete voltage clamp. 4. Single-electrode whole-cell recordings from ciliary-ganglion neurones showed that transient tetrodotoxin (TTX)-sensitive outward currents were present, but only when partial TTX blockade produced significant alterations in the kinetics of INa. In cells that were properly voltage clamped, there was no effect of TTX on the kinetics of INa or on voltage-evoked outward currents. 5. Examination of the relationship between peak INa and the command potential showed that transient outward currents were only present in neurones that showed sharp deviations from the behaviour expected of a cell that is adequately voltage clamped. Transient outward currents were not present in cells that were adequately voltage clamped. 6. Application of TTX to isolated outside-out patches obtained from ciliary ganglion neurones eliminated voltage-evoked inward currents but had no effect on outward currents. 7. Isolated inside-out patches obtained from ciliary-ganglion neurones did not contain IK(Na) channels. These patches usually contained Ca(2+)-activated K+ channels (IK(Ca] with a unitary conductance of around 200 pS when [K+]o = 150 mM and [K+]i = 75 mM. 8. Two-electrode whole-cell recordings from cultured brain stem neurones showed that transient outward currents were present only when there were clear indications of incomplete voltage clamp. 9. Application of TTX caused blockade of inward but not outward currents in brain stem neurones voltage clamped with a single whole-cell pipette. TTX had no effect on the kinetics of INa. Application of TTX to outside-out patches isolated from the same cells blocked only the inward currents. 10. Isolated inside-out patches obtained from brain stem neurones contained IK(Na) channels that could be activated by exposure of the cytoplasmic face of the patch membrane to 75 mM-Na+. These channels had a predominant conductance state of around 100 pS when [K+]o = 150 mM and [K+]i = 75 mM. The IK(Na) channels were not activated by 1 mM-Ca2+.(ABSTRACT TRUNCATED AT 400 WORDS)
Full text
PDF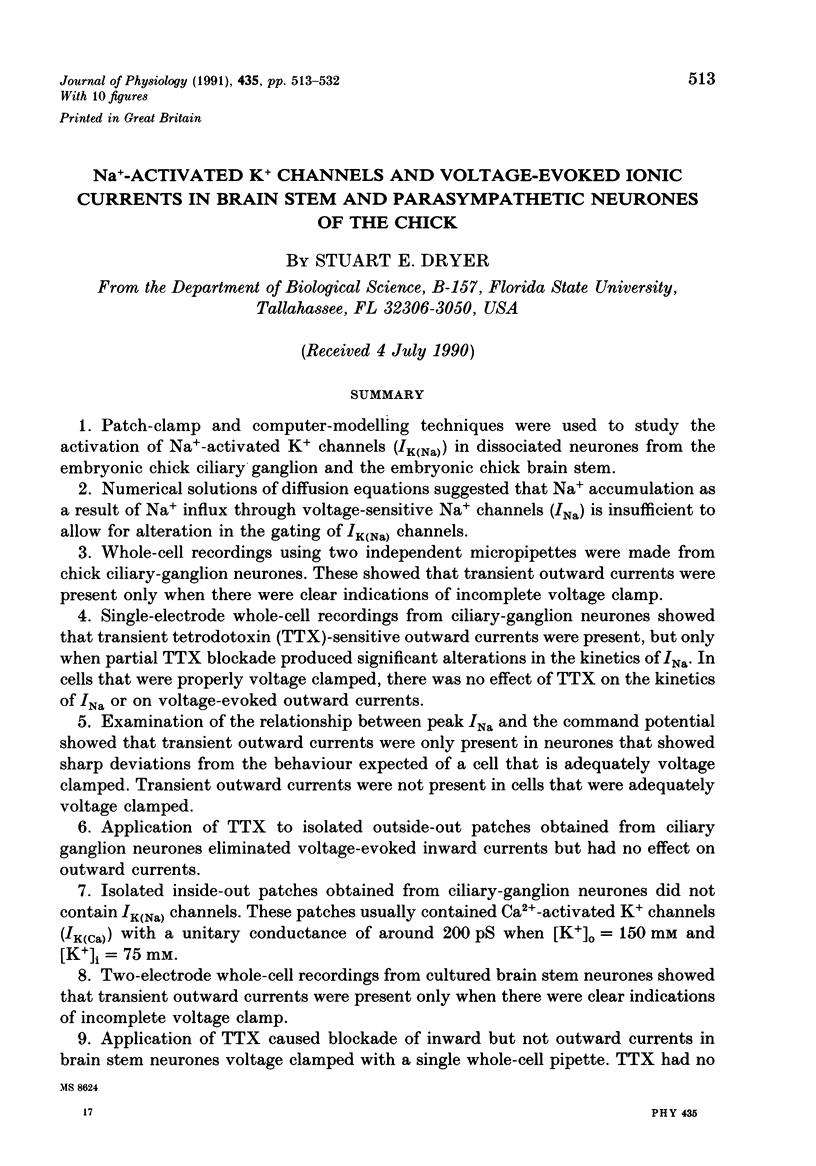
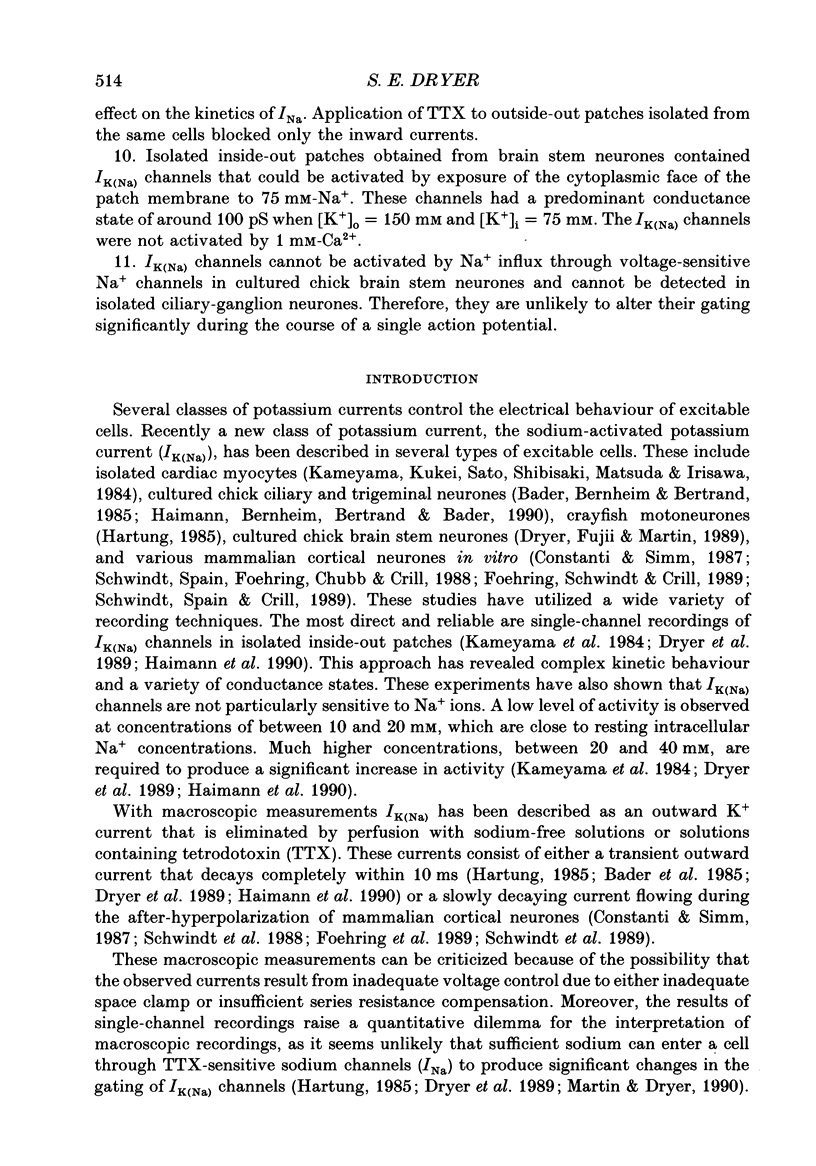
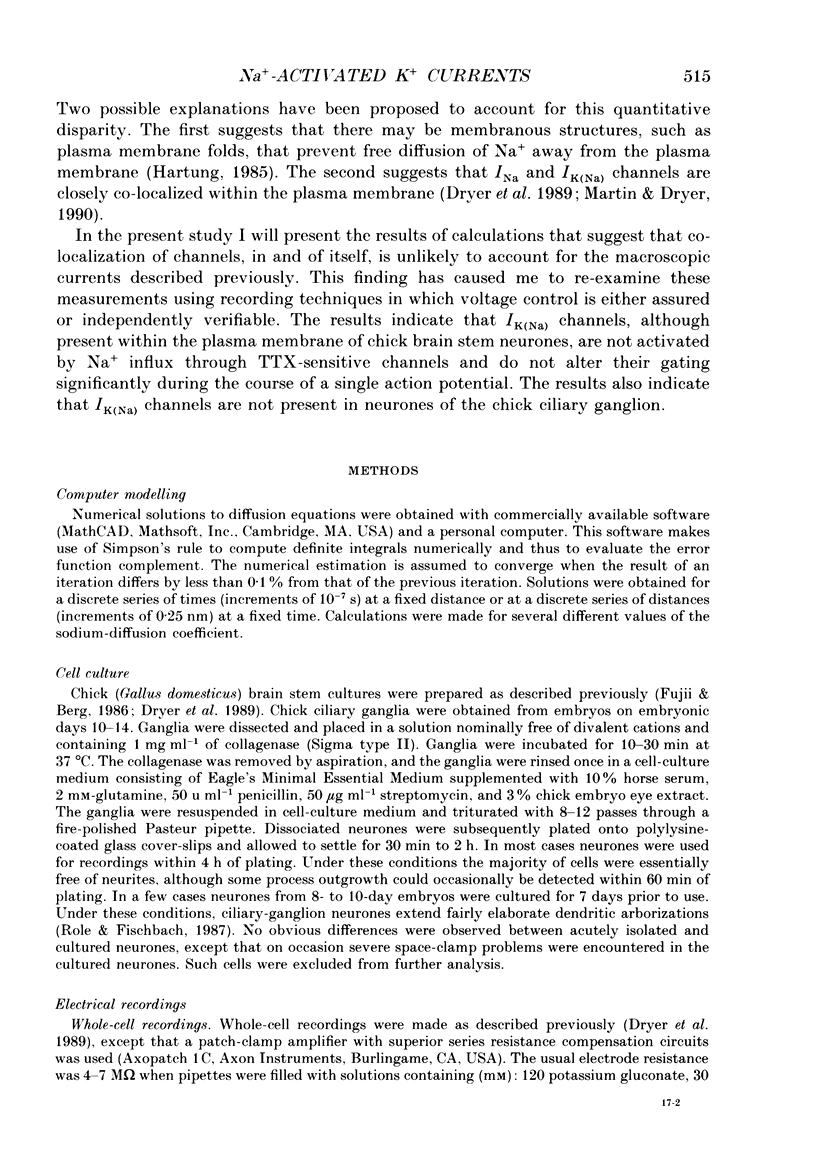
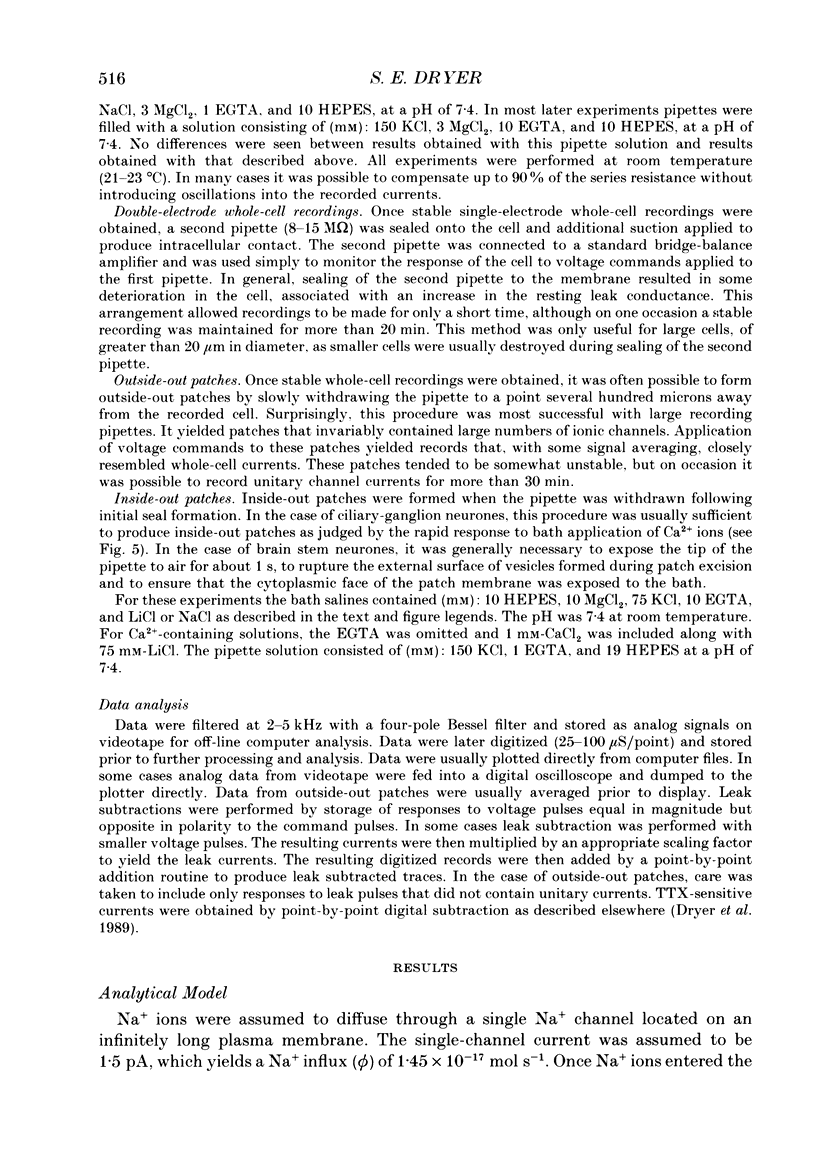
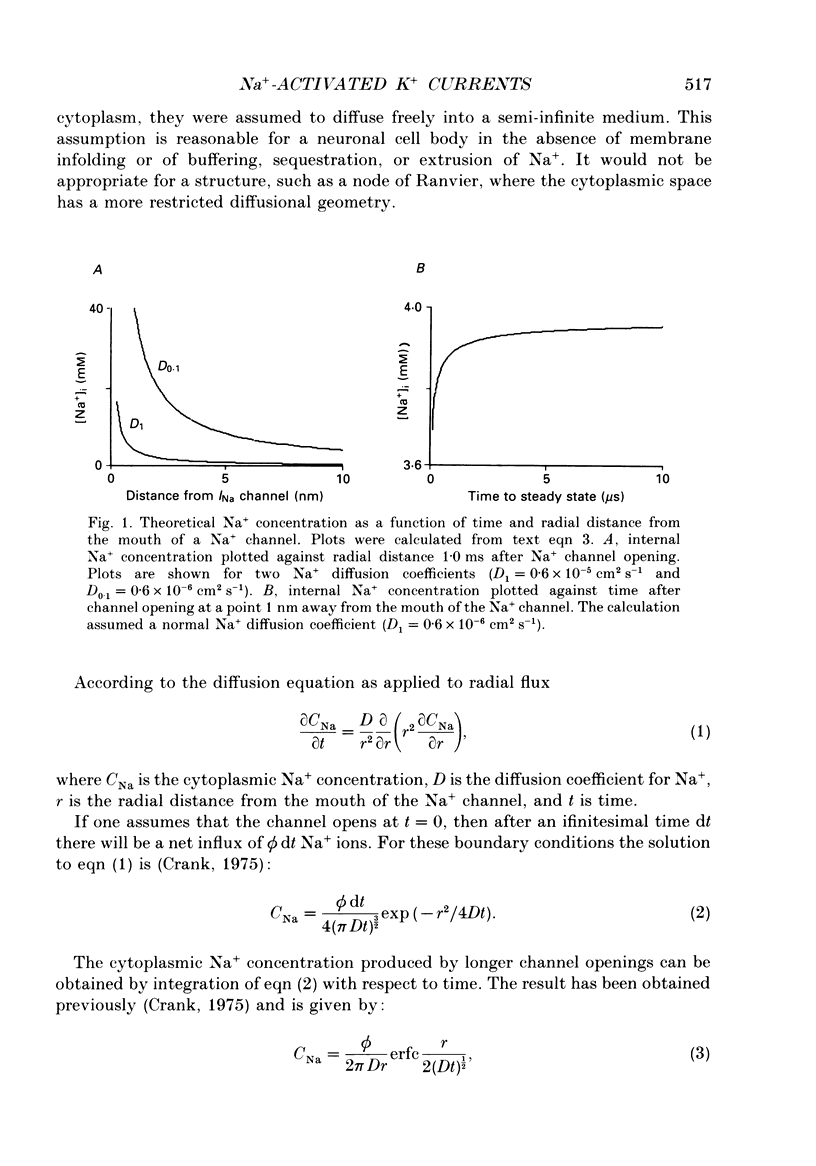
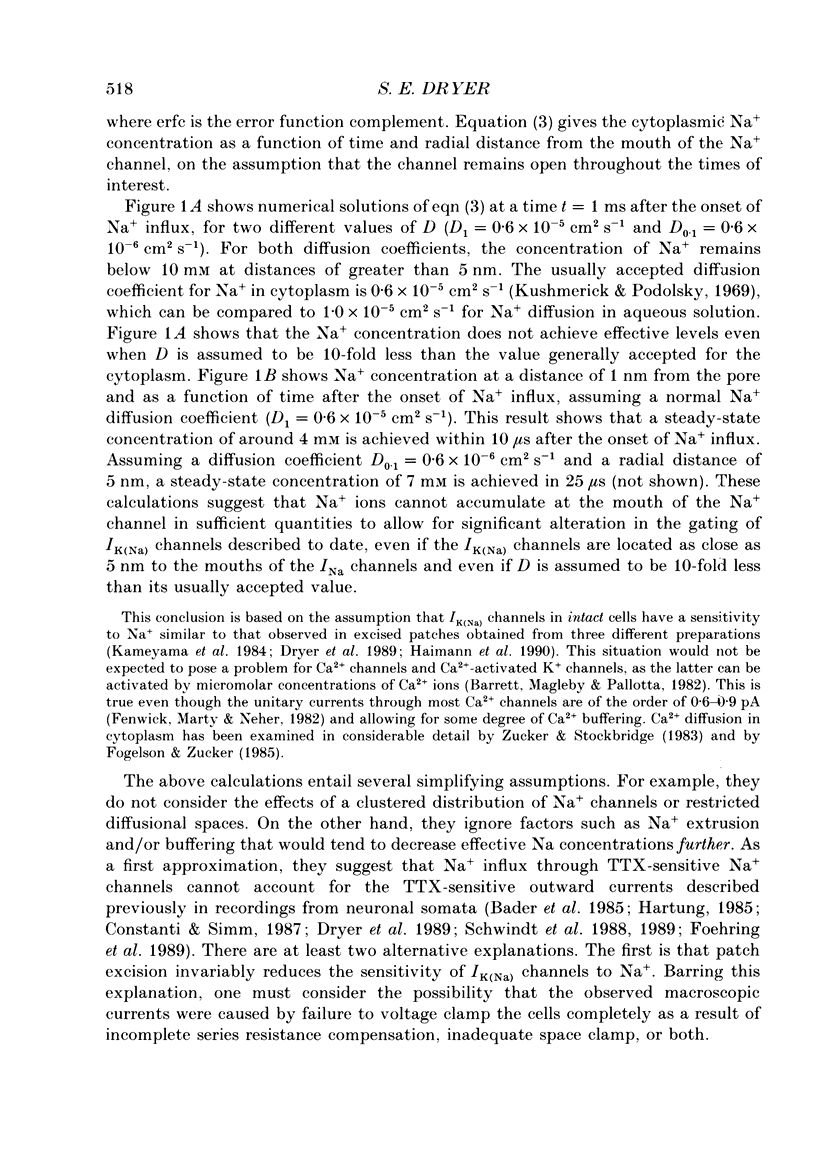
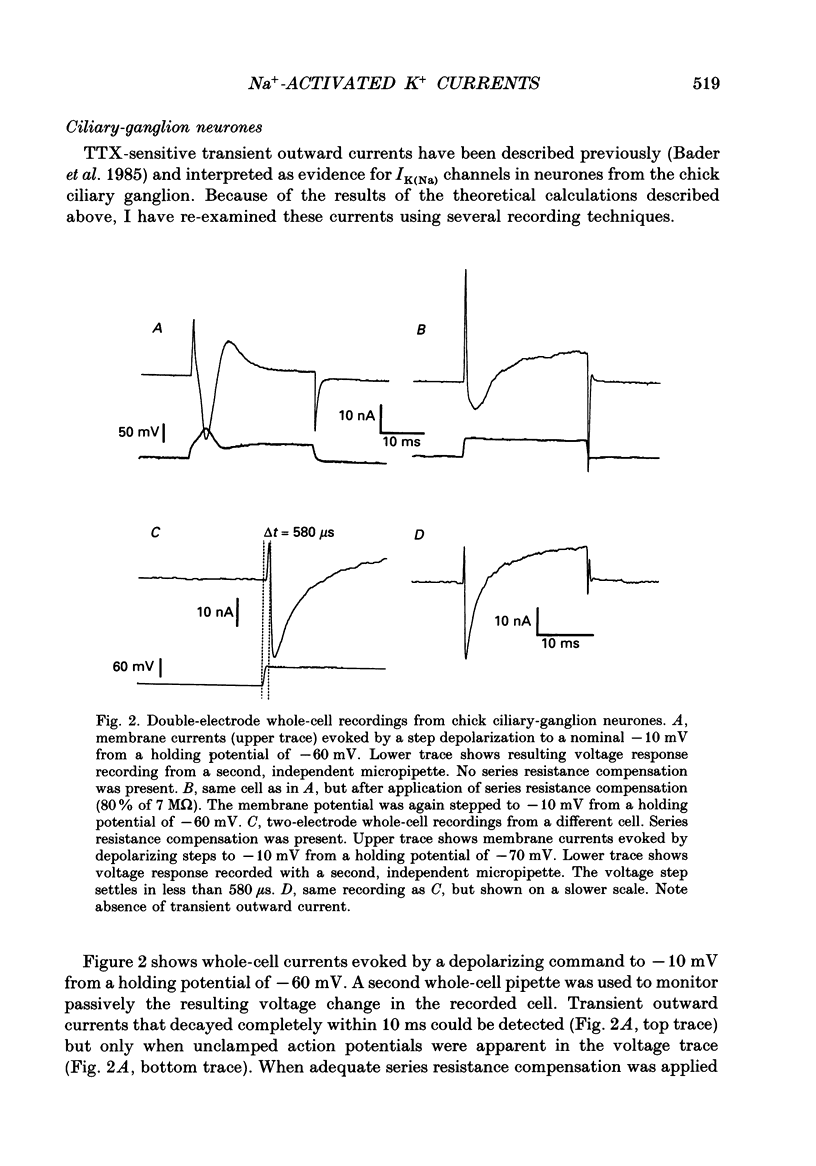
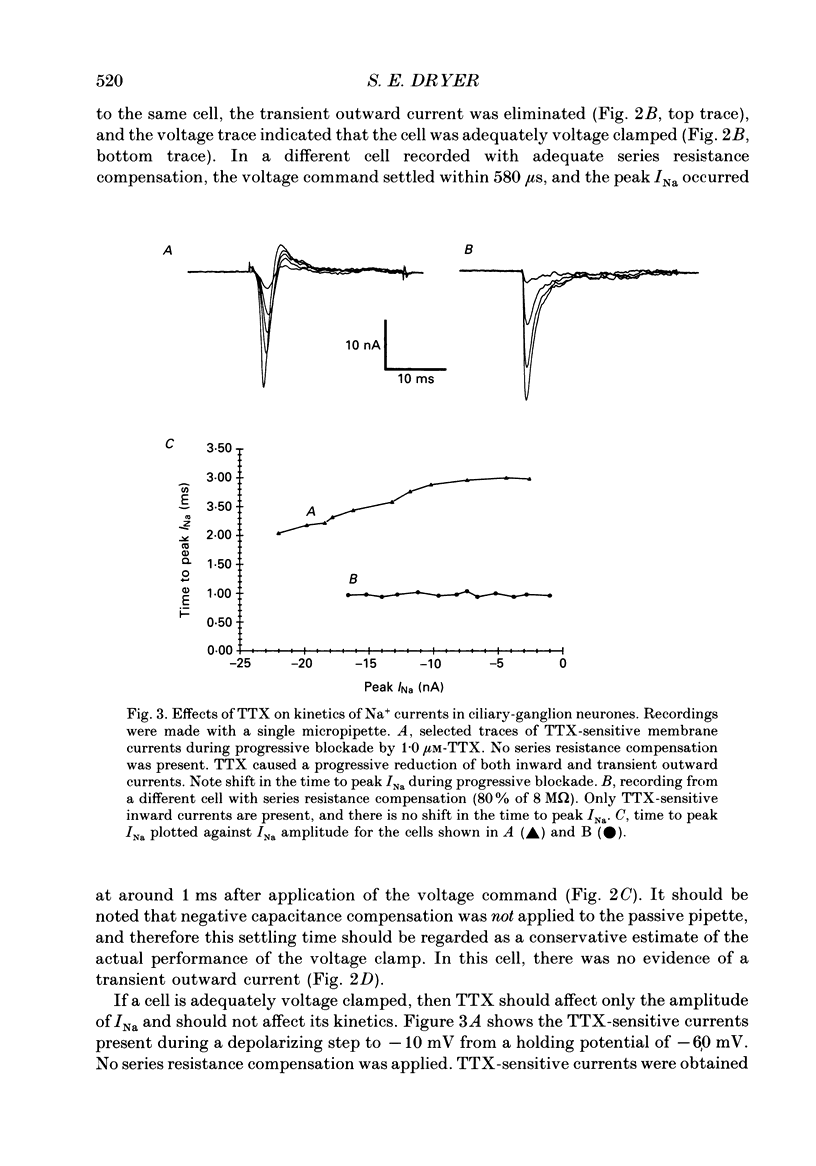
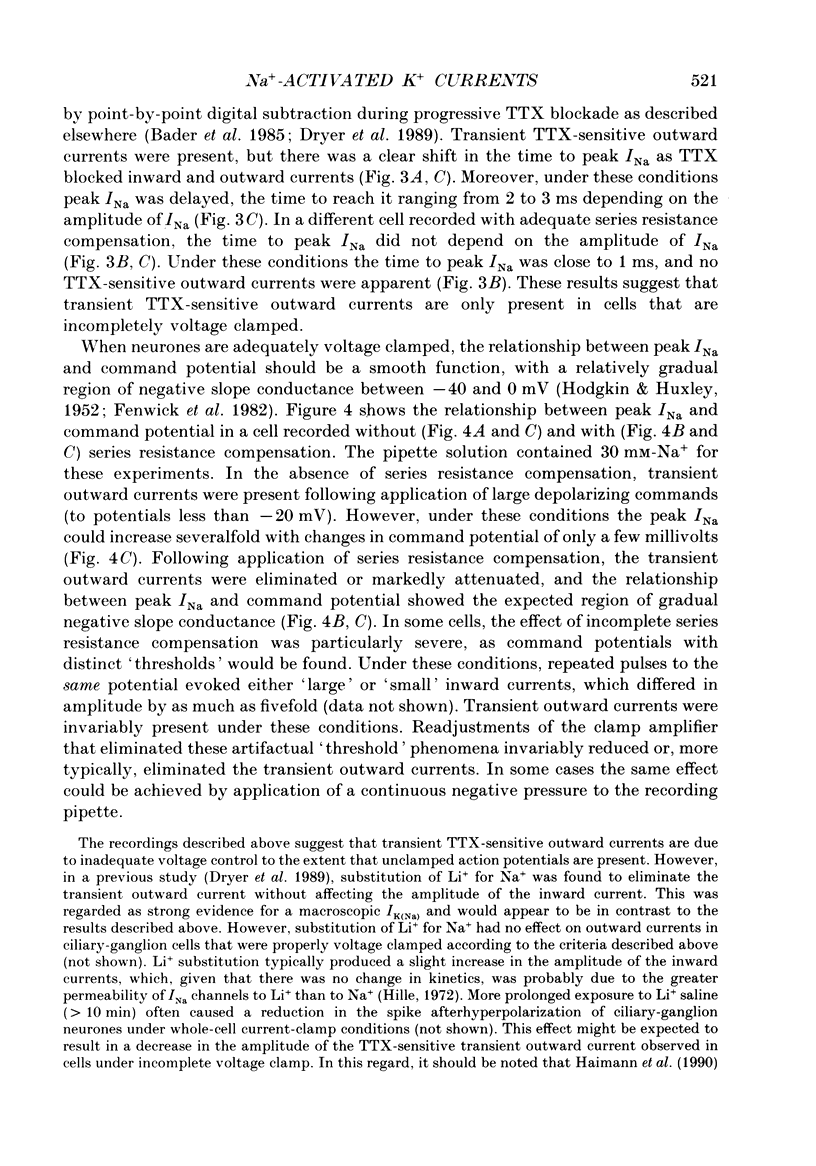
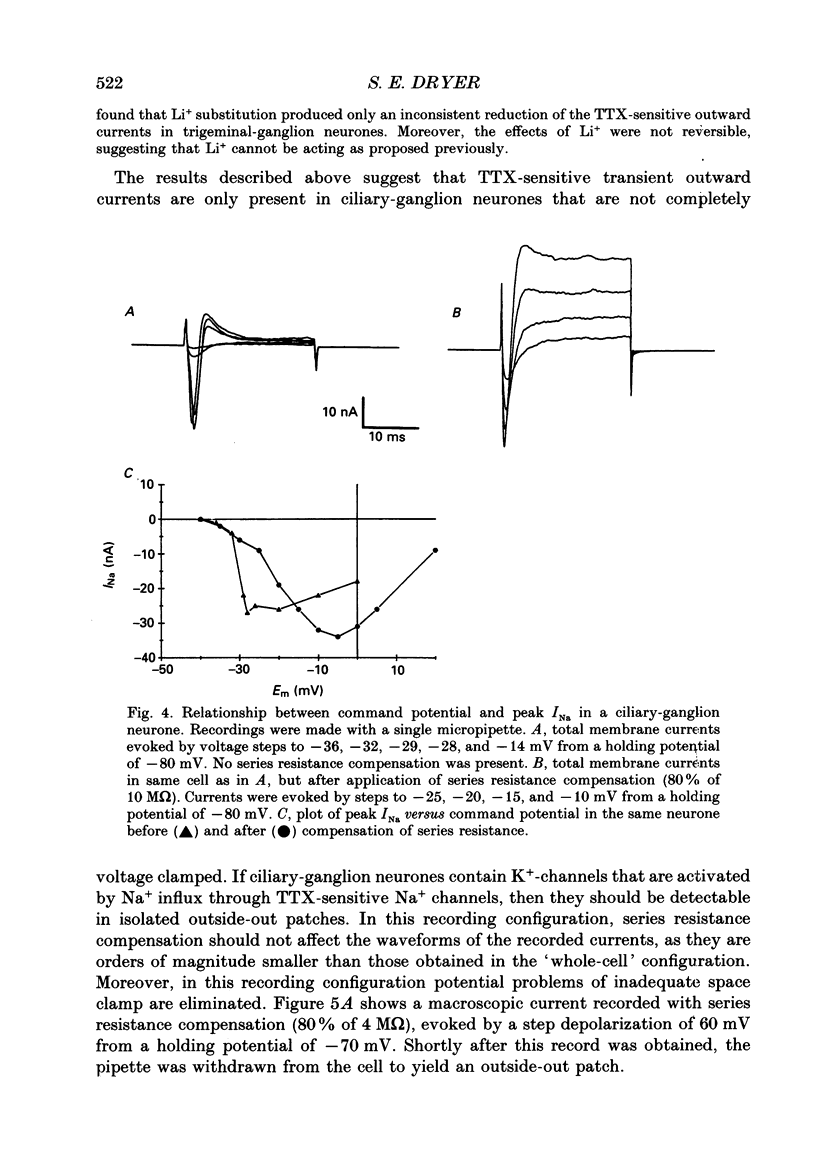
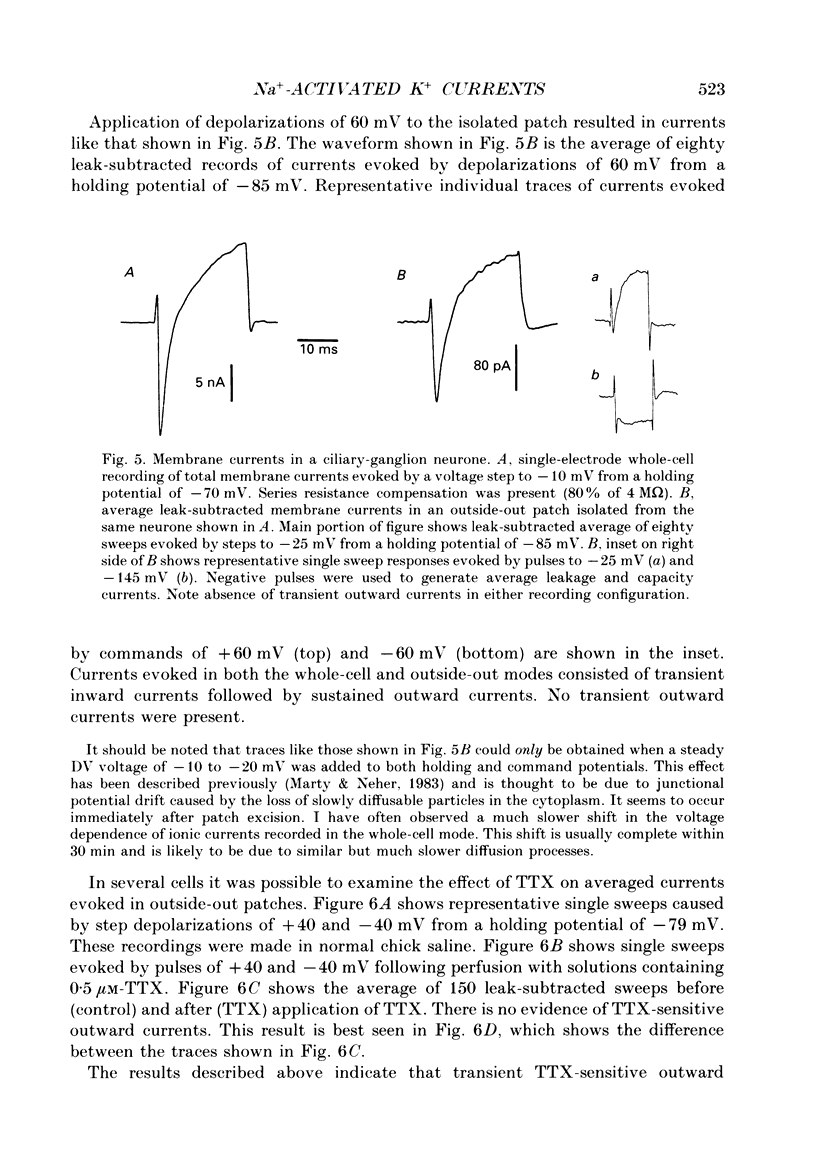
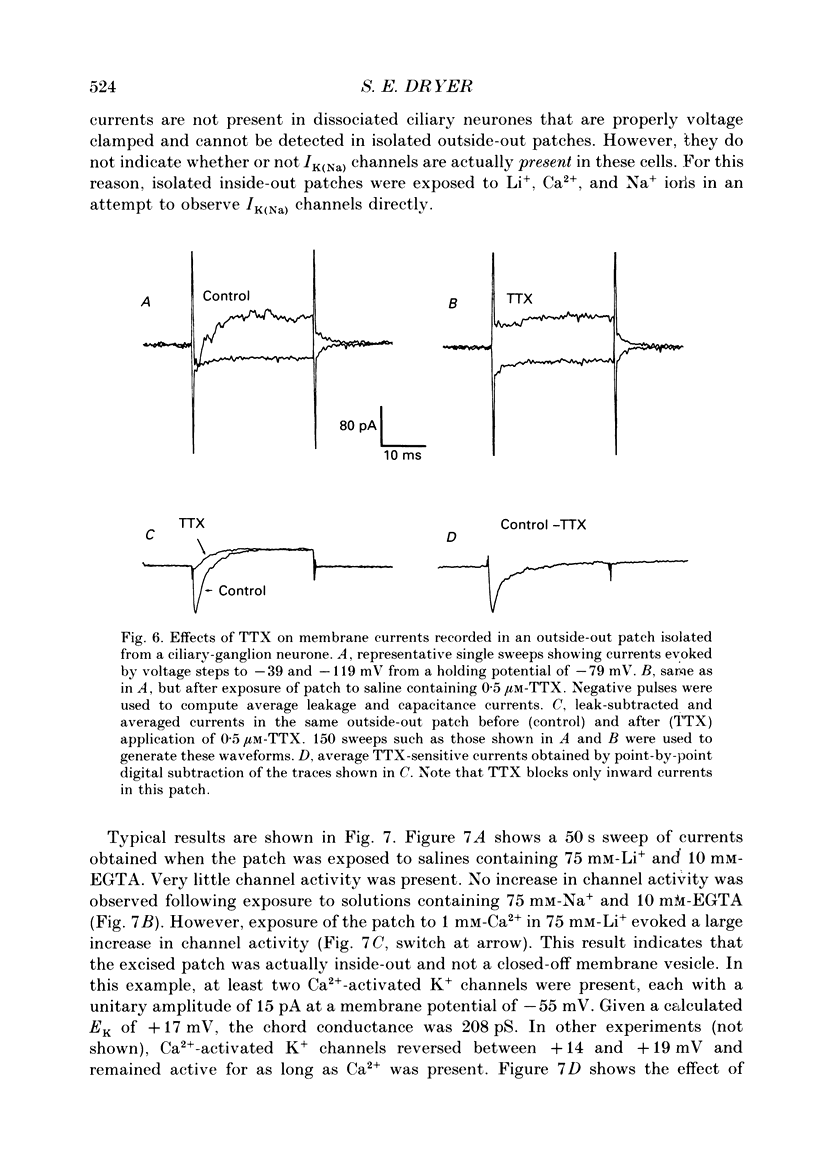
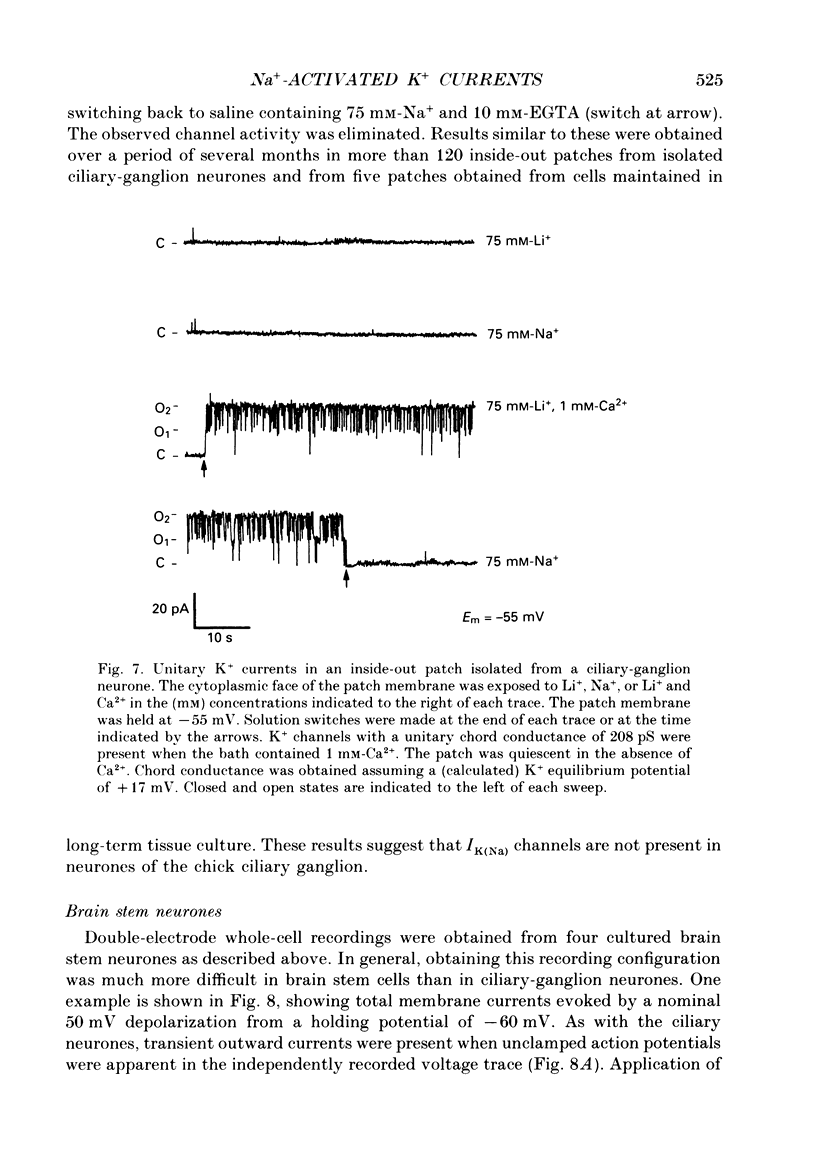
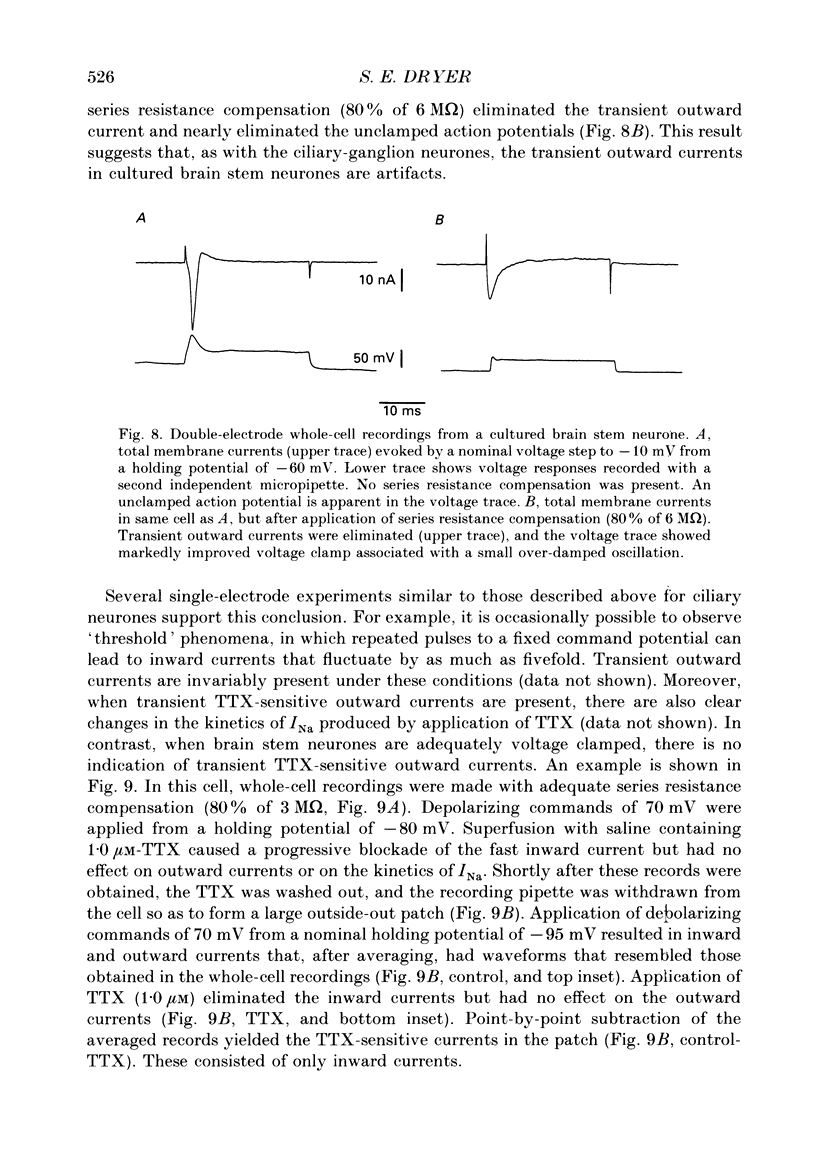
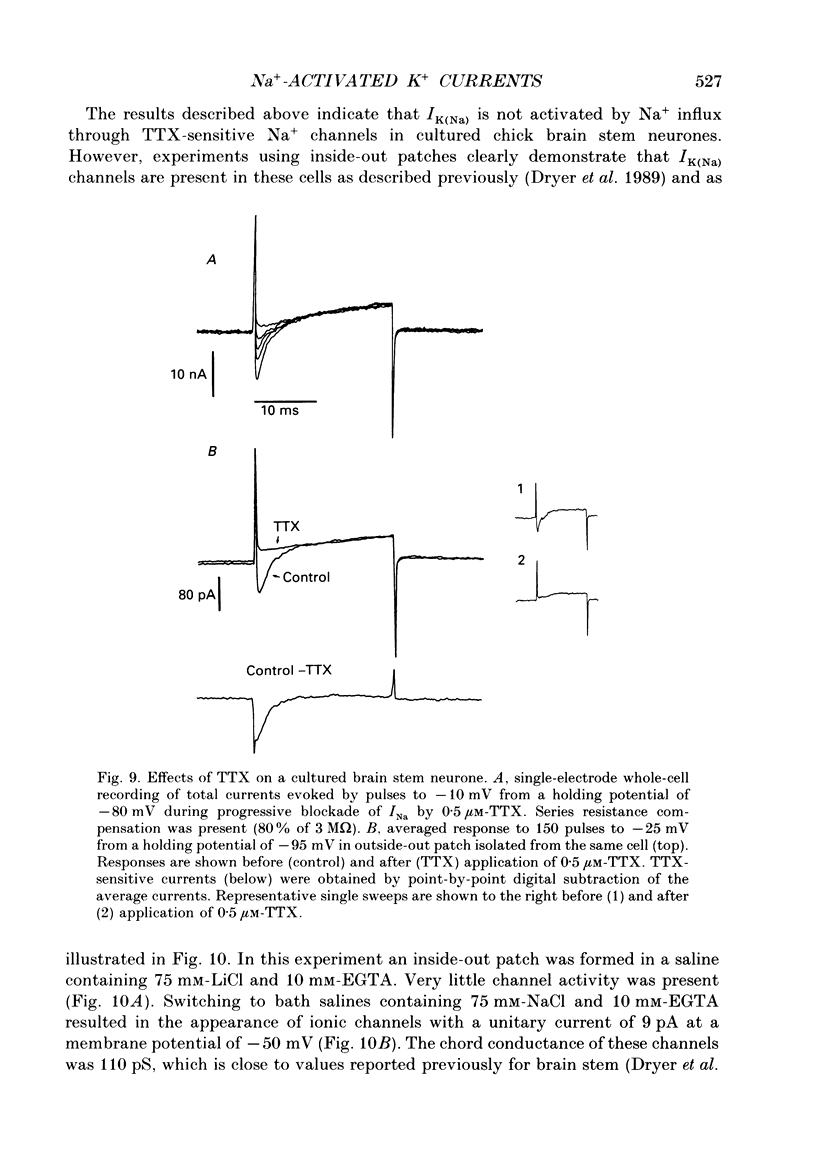
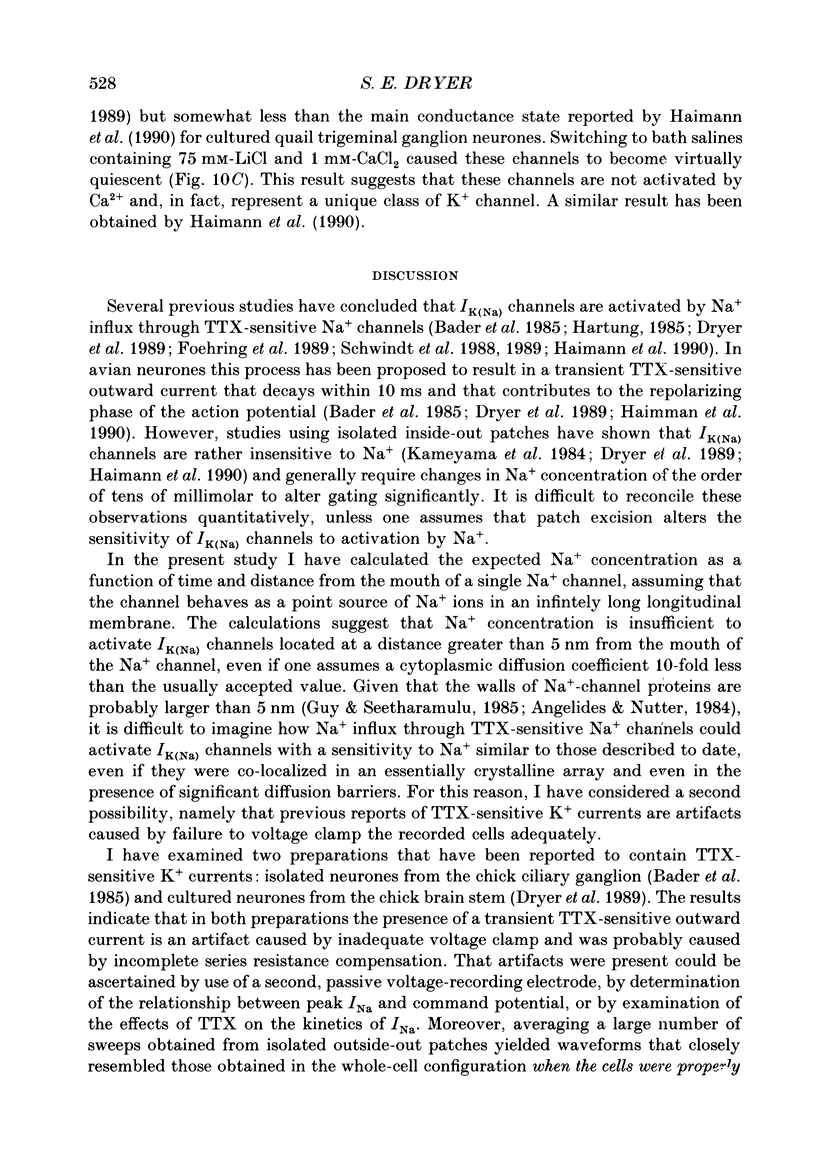
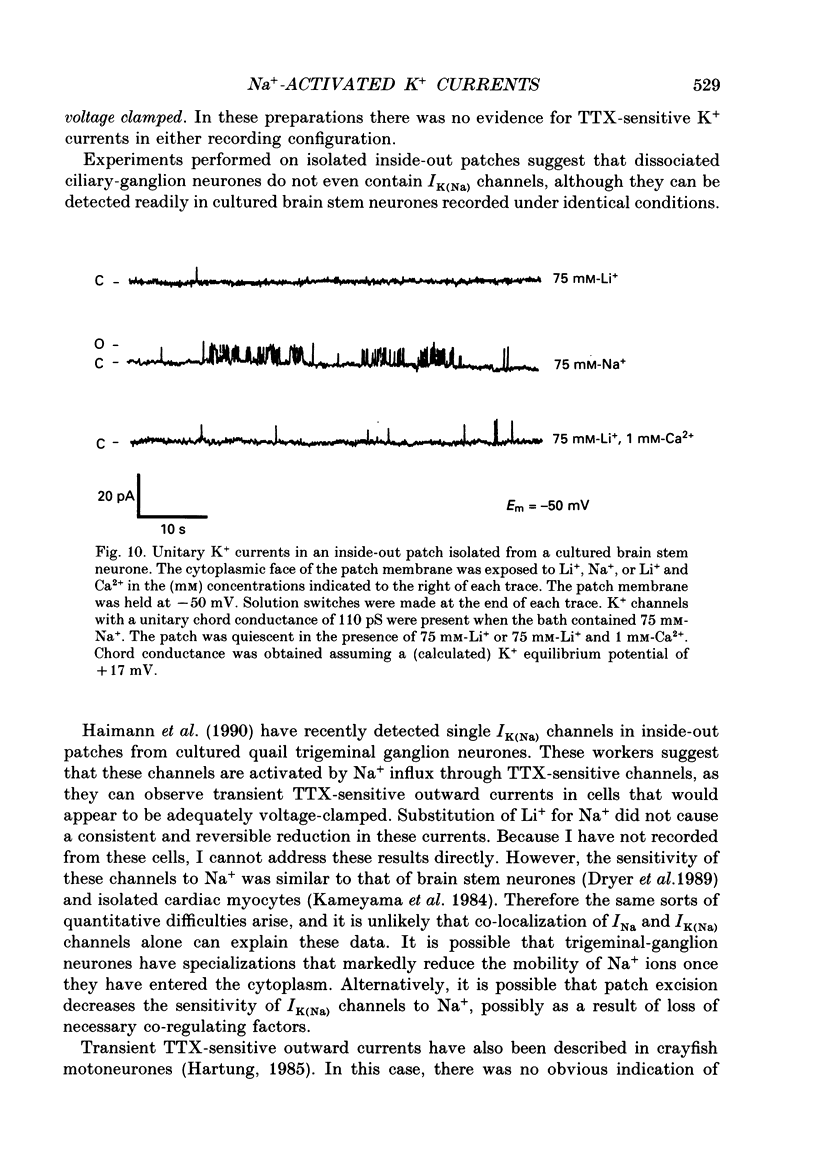
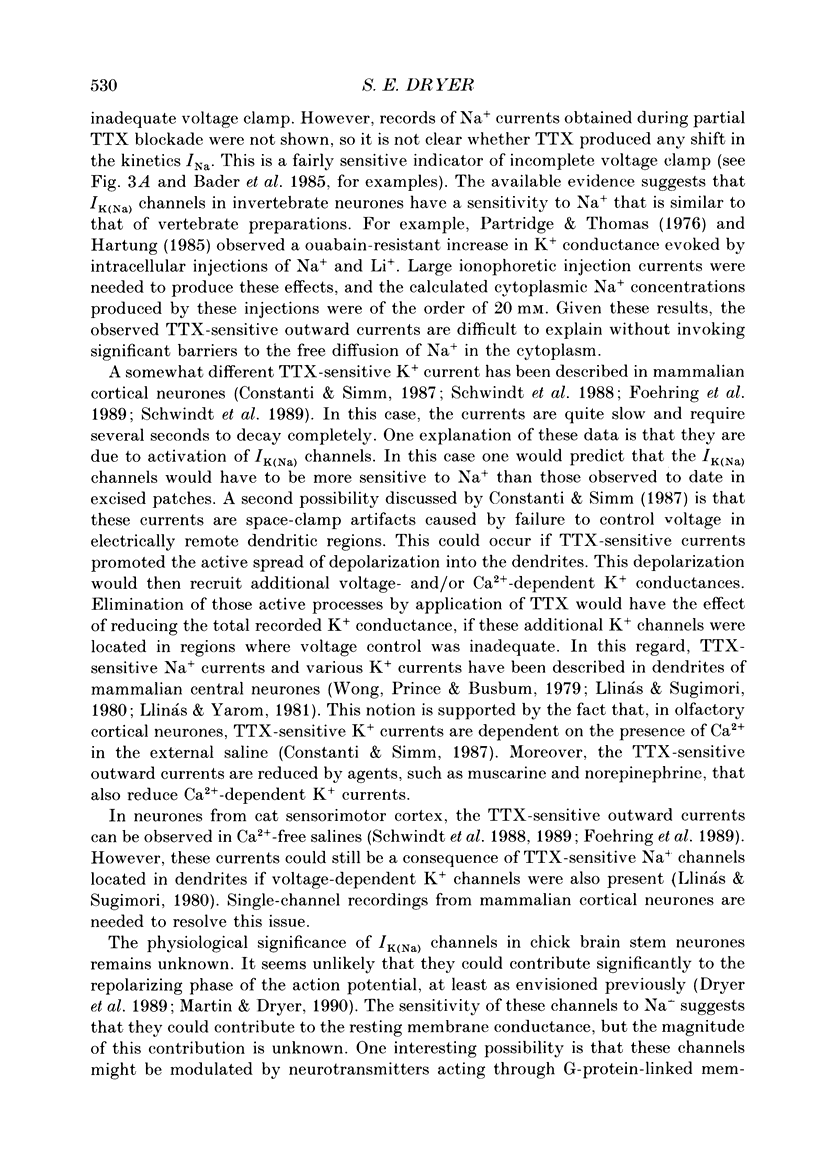
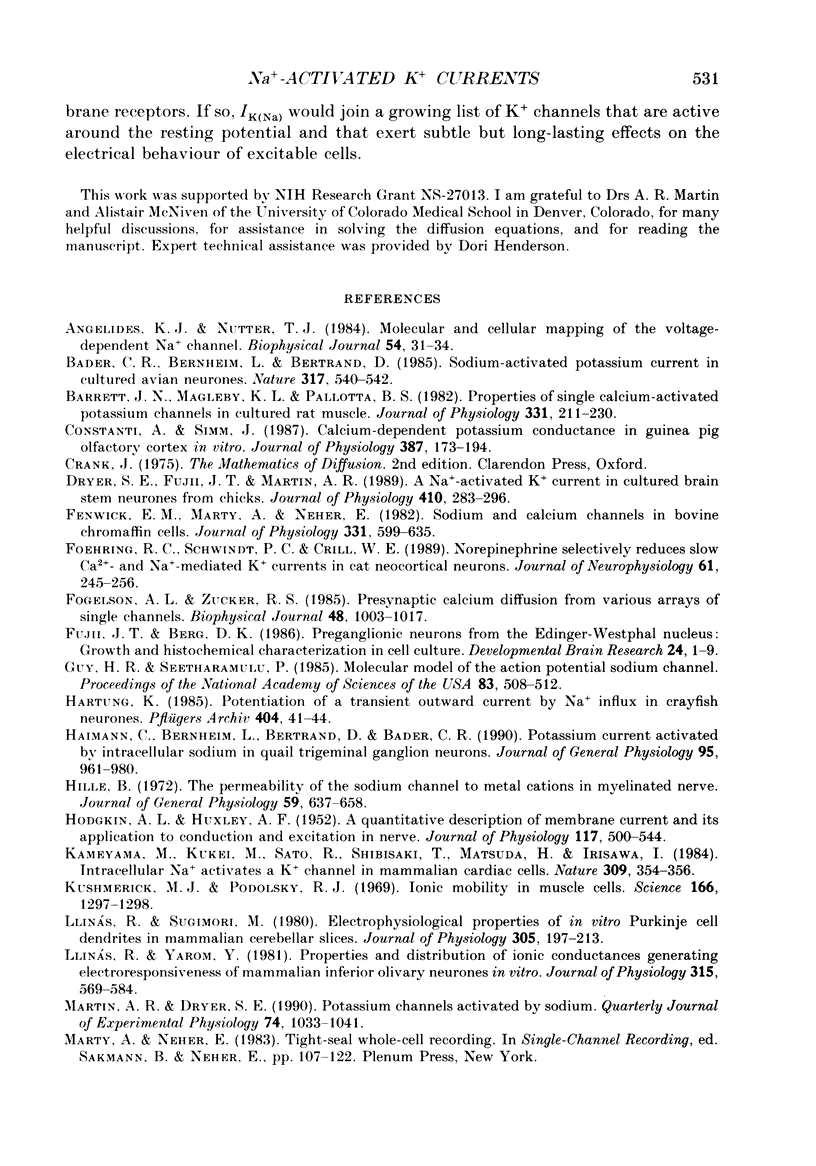
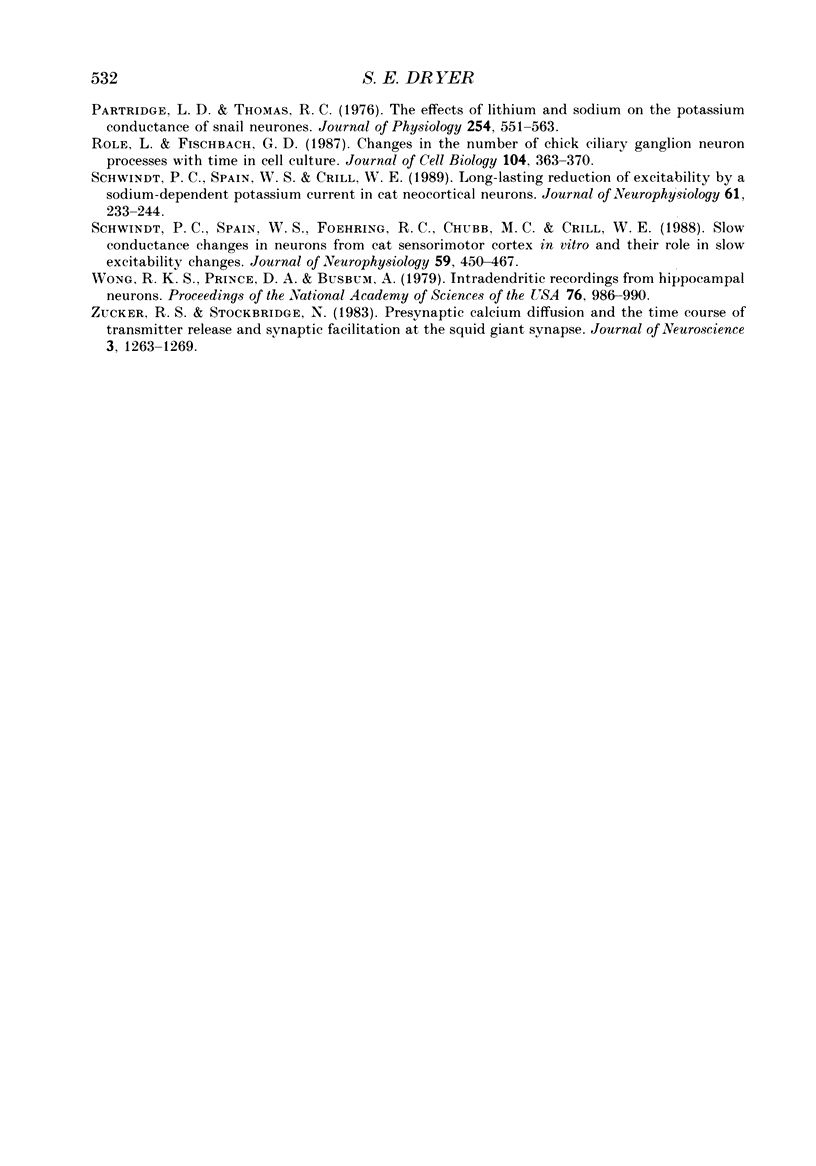
Selected References
These references are in PubMed. This may not be the complete list of references from this article.
- Angelides K. J., Nutter T. J. Molecular and cellular mapping of the voltage-dependent na channel. Biophys J. 1984 Jan;45(1):31–34. doi: 10.1016/S0006-3495(84)84096-5. [DOI] [PMC free article] [PubMed] [Google Scholar]
- Bader C. R., Bernheim L., Bertrand D. Sodium-activated potassium current in cultured avian neurones. Nature. 1985 Oct 10;317(6037):540–542. doi: 10.1038/317540a0. [DOI] [PubMed] [Google Scholar]
- Barrett J. N., Magleby K. L., Pallotta B. S. Properties of single calcium-activated potassium channels in cultured rat muscle. J Physiol. 1982 Oct;331:211–230. doi: 10.1113/jphysiol.1982.sp014370. [DOI] [PMC free article] [PubMed] [Google Scholar]
- Constanti A., Sim J. A. Calcium-dependent potassium conductance in guinea-pig olfactory cortex neurones in vitro. J Physiol. 1987 Jun;387:173–194. doi: 10.1113/jphysiol.1987.sp016569. [DOI] [PMC free article] [PubMed] [Google Scholar]
- Dryer S. E., Fujii J. T., Martin A. R. A Na+-activated K+ current in cultured brain stem neurones from chicks. J Physiol. 1989 Mar;410:283–296. doi: 10.1113/jphysiol.1989.sp017533. [DOI] [PMC free article] [PubMed] [Google Scholar]
- Fenwick E. M., Marty A., Neher E. Sodium and calcium channels in bovine chromaffin cells. J Physiol. 1982 Oct;331:599–635. doi: 10.1113/jphysiol.1982.sp014394. [DOI] [PMC free article] [PubMed] [Google Scholar]
- Foehring R. C., Schwindt P. C., Crill W. E. Norepinephrine selectively reduces slow Ca2+- and Na+-mediated K+ currents in cat neocortical neurons. J Neurophysiol. 1989 Feb;61(2):245–256. doi: 10.1152/jn.1989.61.2.245. [DOI] [PubMed] [Google Scholar]
- Fogelson A. L., Zucker R. S. Presynaptic calcium diffusion from various arrays of single channels. Implications for transmitter release and synaptic facilitation. Biophys J. 1985 Dec;48(6):1003–1017. doi: 10.1016/S0006-3495(85)83863-7. [DOI] [PMC free article] [PubMed] [Google Scholar]
- Guy H. R., Seetharamulu P. Molecular model of the action potential sodium channel. Proc Natl Acad Sci U S A. 1986 Jan;83(2):508–512. doi: 10.1073/pnas.83.2.508. [DOI] [PMC free article] [PubMed] [Google Scholar]
- HODGKIN A. L., HUXLEY A. F. A quantitative description of membrane current and its application to conduction and excitation in nerve. J Physiol. 1952 Aug;117(4):500–544. doi: 10.1113/jphysiol.1952.sp004764. [DOI] [PMC free article] [PubMed] [Google Scholar]
- Haimann C., Bernheim L., Bertrand D., Bader C. R. Potassium current activated by intracellular sodium in quail trigeminal ganglion neurons. J Gen Physiol. 1990 May;95(5):961–979. doi: 10.1085/jgp.95.5.961. [DOI] [PMC free article] [PubMed] [Google Scholar]
- Hartung K. Potentiation of a transient outward current by Na+ influx in crayfish neurones. Pflugers Arch. 1985 May;404(1):41–44. doi: 10.1007/BF00581488. [DOI] [PubMed] [Google Scholar]
- Hille B. The permeability of the sodium channel to metal cations in myelinated nerve. J Gen Physiol. 1972 Jun;59(6):637–658. doi: 10.1085/jgp.59.6.637. [DOI] [PMC free article] [PubMed] [Google Scholar]
- Kameyama M., Kakei M., Sato R., Shibasaki T., Matsuda H., Irisawa H. Intracellular Na+ activates a K+ channel in mammalian cardiac cells. Nature. 1984 May 24;309(5966):354–356. doi: 10.1038/309354a0. [DOI] [PubMed] [Google Scholar]
- Kushmerick M. J., Podolsky R. J. Ionic mobility in muscle cells. Science. 1969 Dec 5;166(3910):1297–1298. doi: 10.1126/science.166.3910.1297. [DOI] [PubMed] [Google Scholar]
- Llinás R., Sugimori M. Electrophysiological properties of in vitro Purkinje cell dendrites in mammalian cerebellar slices. J Physiol. 1980 Aug;305:197–213. doi: 10.1113/jphysiol.1980.sp013358. [DOI] [PMC free article] [PubMed] [Google Scholar]
- Llinás R., Yarom Y. Properties and distribution of ionic conductances generating electroresponsiveness of mammalian inferior olivary neurones in vitro. J Physiol. 1981 Jun;315:569–584. doi: 10.1113/jphysiol.1981.sp013764. [DOI] [PMC free article] [PubMed] [Google Scholar]
- Martin A. R., Dryer S. E. Potassium channels activated by sodium. Q J Exp Physiol. 1989 Dec;74(7):1033–1041. doi: 10.1113/expphysiol.1989.sp003331. [DOI] [PubMed] [Google Scholar]
- Partridge L. D., Thomas R. C. The effects of lithium and sodium on the potassium conductance of snail neurones. J Physiol. 1976 Jan;254(3):551–563. doi: 10.1113/jphysiol.1976.sp011246. [DOI] [PMC free article] [PubMed] [Google Scholar]
- Role L. W., Fischbach G. D. Changes in the number of chick ciliary ganglion neuron processes with time in cell culture. J Cell Biol. 1987 Feb;104(2):363–370. doi: 10.1083/jcb.104.2.363. [DOI] [PMC free article] [PubMed] [Google Scholar]
- Schwindt P. C., Spain W. J., Crill W. E. Long-lasting reduction of excitability by a sodium-dependent potassium current in cat neocortical neurons. J Neurophysiol. 1989 Feb;61(2):233–244. doi: 10.1152/jn.1989.61.2.233. [DOI] [PubMed] [Google Scholar]
- Schwindt P. C., Spain W. J., Foehring R. C., Chubb M. C., Crill W. E. Slow conductances in neurons from cat sensorimotor cortex in vitro and their role in slow excitability changes. J Neurophysiol. 1988 Feb;59(2):450–467. doi: 10.1152/jn.1988.59.2.450. [DOI] [PubMed] [Google Scholar]
- Wong R. K., Prince D. A., Basbaum A. I. Intradendritic recordings from hippocampal neurons. Proc Natl Acad Sci U S A. 1979 Feb;76(2):986–990. doi: 10.1073/pnas.76.2.986. [DOI] [PMC free article] [PubMed] [Google Scholar]
- Zucker R. S., Stockbridge N. Presynaptic calcium diffusion and the time courses of transmitter release and synaptic facilitation at the squid giant synapse. J Neurosci. 1983 Jun;3(6):1263–1269. doi: 10.1523/JNEUROSCI.03-06-01263.1983. [DOI] [PMC free article] [PubMed] [Google Scholar]