Abstract
1. A method has been developed for calculating the flux of Ca2+ across the sarcoplasmic reticulum (SR) during excitation-contraction coupling in mammalian heart cells. FSR will symbolize the net rate of movement of Ca2+, per litre of accessible cytoplasm, into or out of the sarcoplasmic reticulum. FSR has the units MS-1. 2. A theory of the cytoplasmic [Ca2+]i transient in mammalian heart cells is presented in which the [Ca2+]i transient results from the various cellular processes that tend to increase or decrease cytoplasmic [Ca2+]i. According to the theory, FSR can be calculated if all cellular processes that contribute to the [Ca2+]i transient (other than Ca2+ fluxes across the SR) are either eliminated or are known quantitatively. 3. To obtain the measurements required to apply this theory, [Ca2+]i transients and membrane currents were recorded in guinea-pig single ventricular myocytes subjected to whole-cell voltage clamp and internal perfusion. [Ca2+]i transients were recorded through the use of the Ca2+ indicator, Fura-2 (pentapotassium salt). 4. Ca2+ fluxes through the sodium-calcium exchanger were eliminated in all experiments, by perfusing the cells, internally and externally with Na(+)-free solutions. Ca2+ flux through the sarcolemmal L-type Ca2+ channel was measured as the verapamil-sensitive current. Influx of Ca2+ through all other voltage-dependent pathways was found to be negligible for the calculation of FSR over the time course of a single [Ca2+]i transient. 5. In the combined absence of Ca2+ current, Na(+)-Ca2+ exchange and fluxes across the SR (10 mM-caffeine), the net rate of removal of Ca2+ from the cytoplasm, which includes presumed contributions from sarcolemmal Ca(2+)-ATPase and mitochondrial Ca2+ transport, was found to be a negligible quantity in the calculation of FSR, over the time course of a single [Ca2+]i transient. 6. Calculation of FSR requires that the Ca(2+)-binding capacity of cytoplasm be known. [Ca2+]i transients recorded during measurable total Ca2+ influx into the cytoplasm (verapamil-sensitive current in the absence of fluxes across the SR) were compared with theoretical Ca2+ transients computed on the assumption that the entering Ca2+ could bind only to intracellular ligands (values for ligands taken from literature) and to Fura-2 (30 microM). The slope of the regression line relating calculated total change in [Ca2+]i to the measured total Ca2+ influx was 0.99, not different from the perfect theoretical slope of 1.0 (correlation coefficient, 0.81; standard deviation of slope, 0.14; n = 7).4+ the SR and FSR had a similar time course to that on depolarization. 10. The unidirectional efflux of Ca2+ from the SR, symbolized FSR, rel was calculated utilizing assumed characteristics of the Ca2+ pump of the SR. The value of FSR, rel was not affected by repolarization from voltage-clamp pulses greater than 150 ms in duration.(ABSTRACT TRUNCATED AT 400 WORDS)
Full text
PDF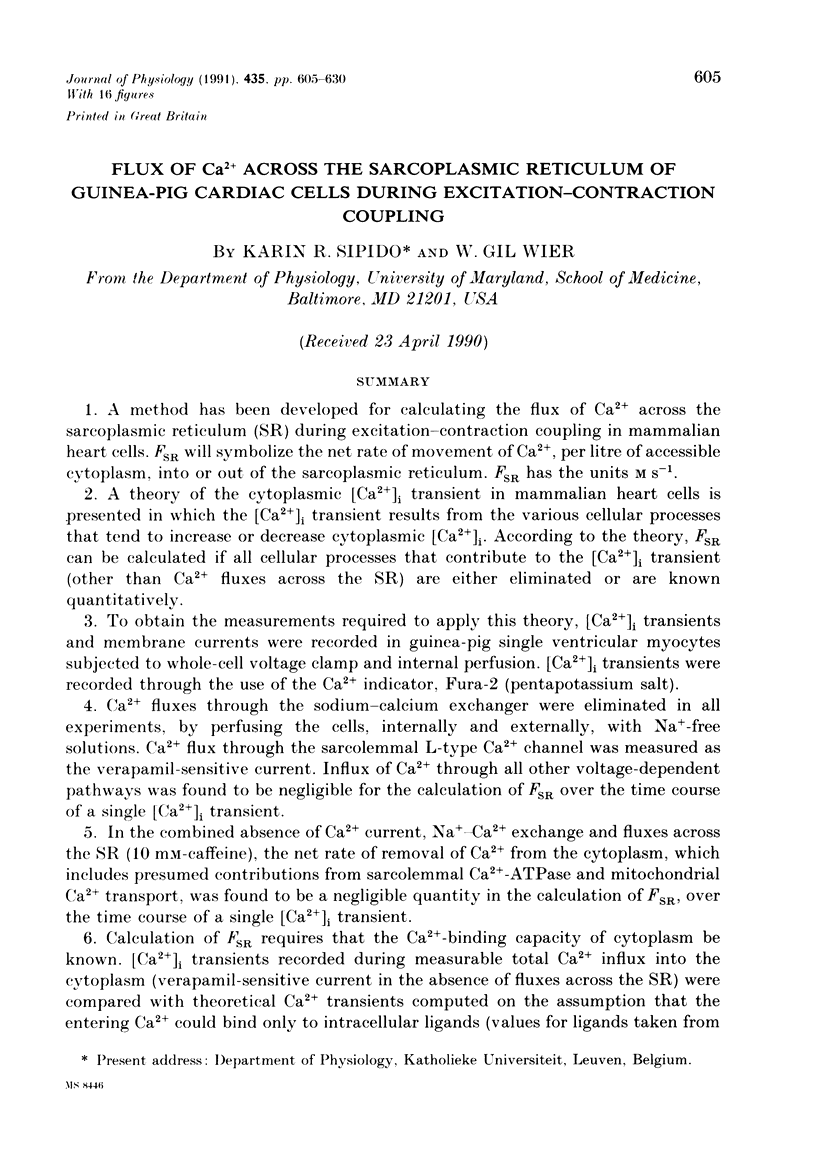
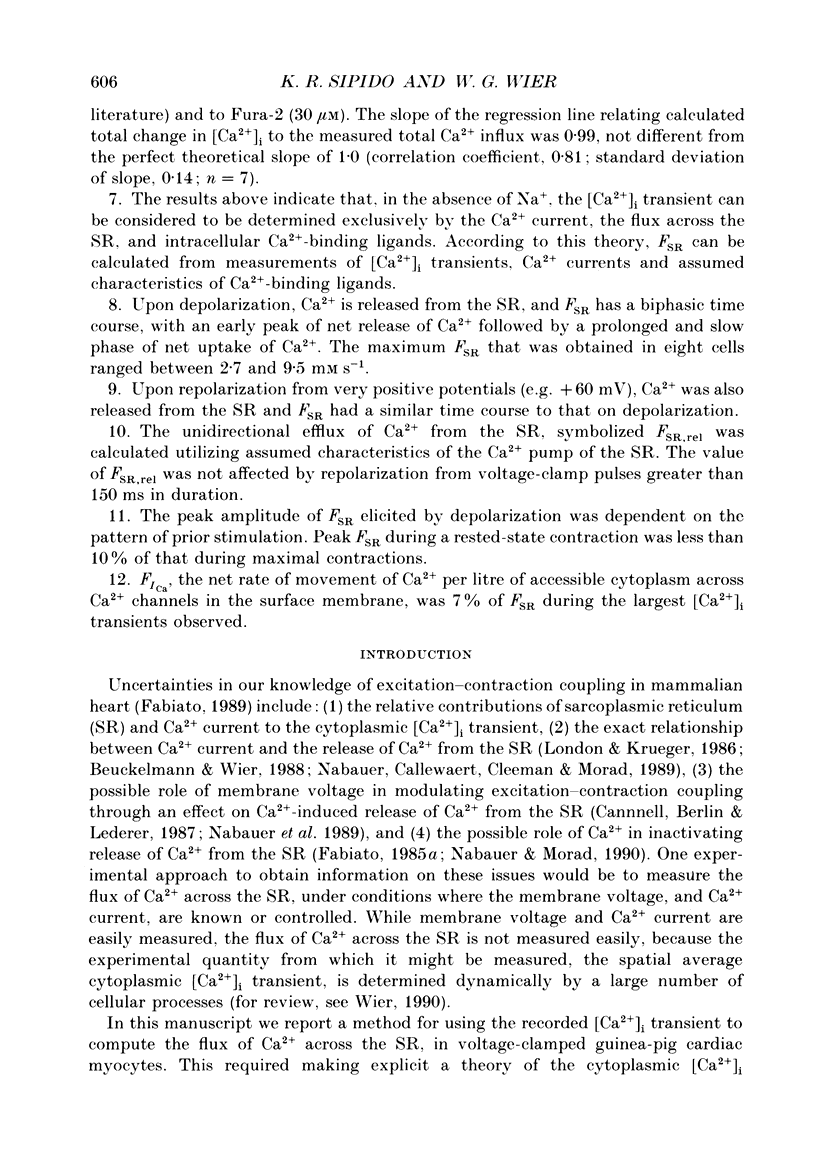
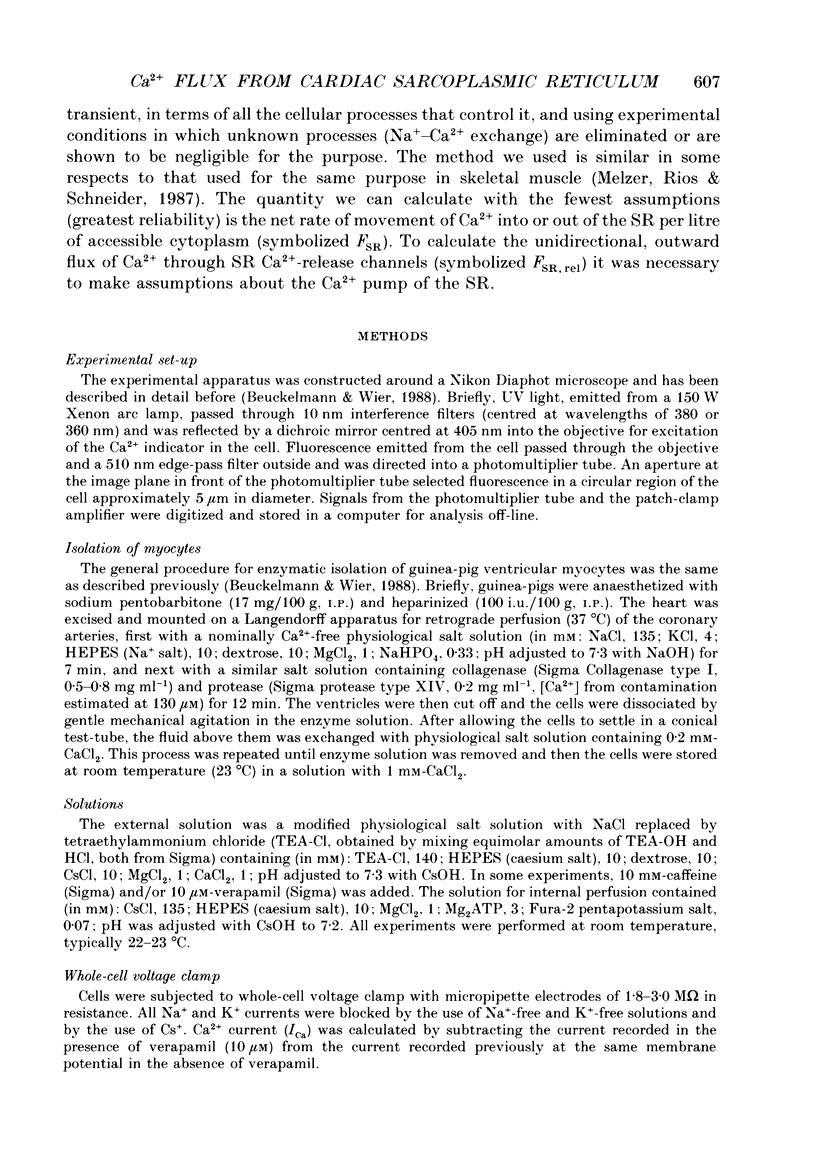
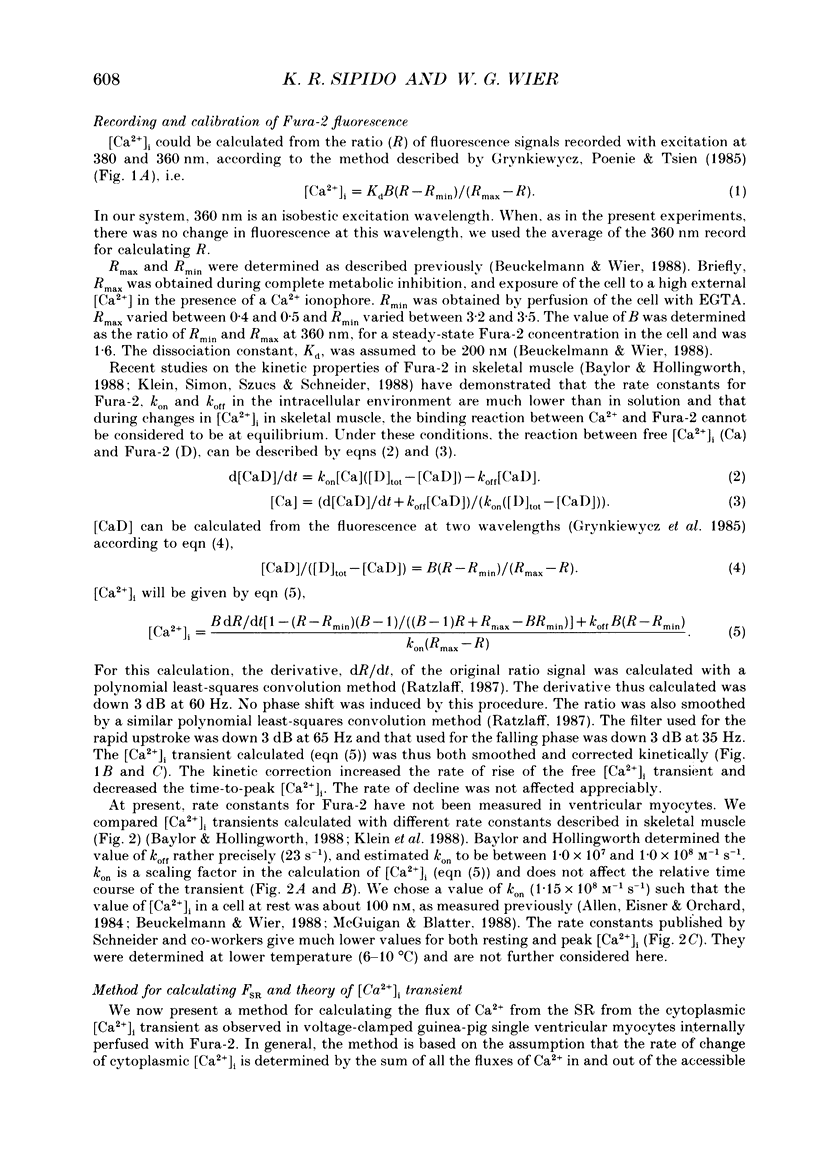
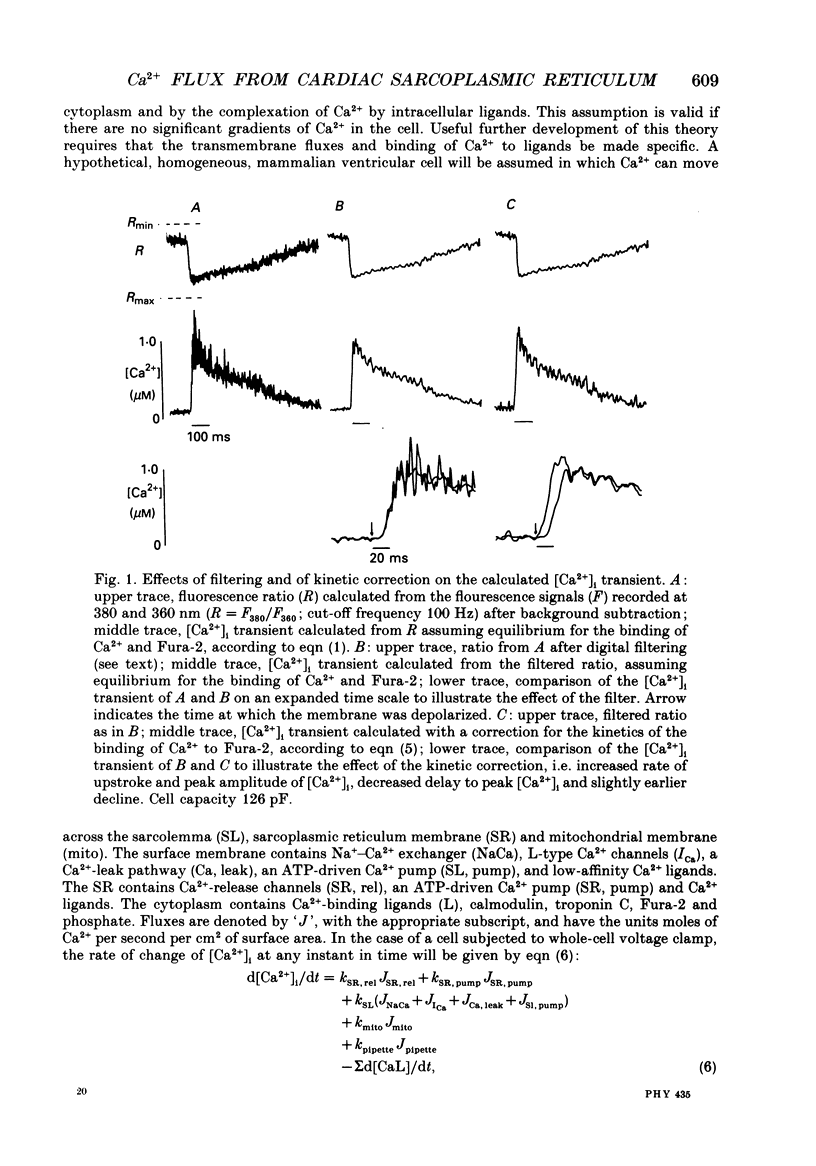
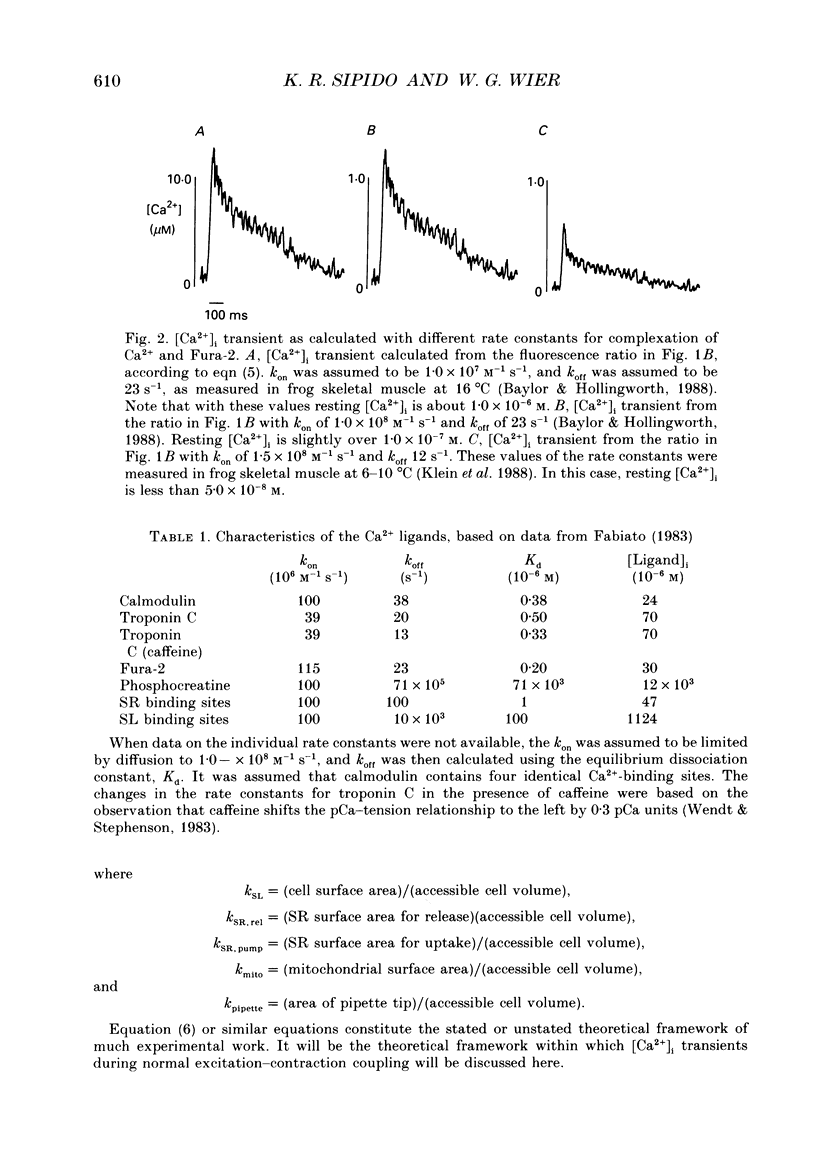
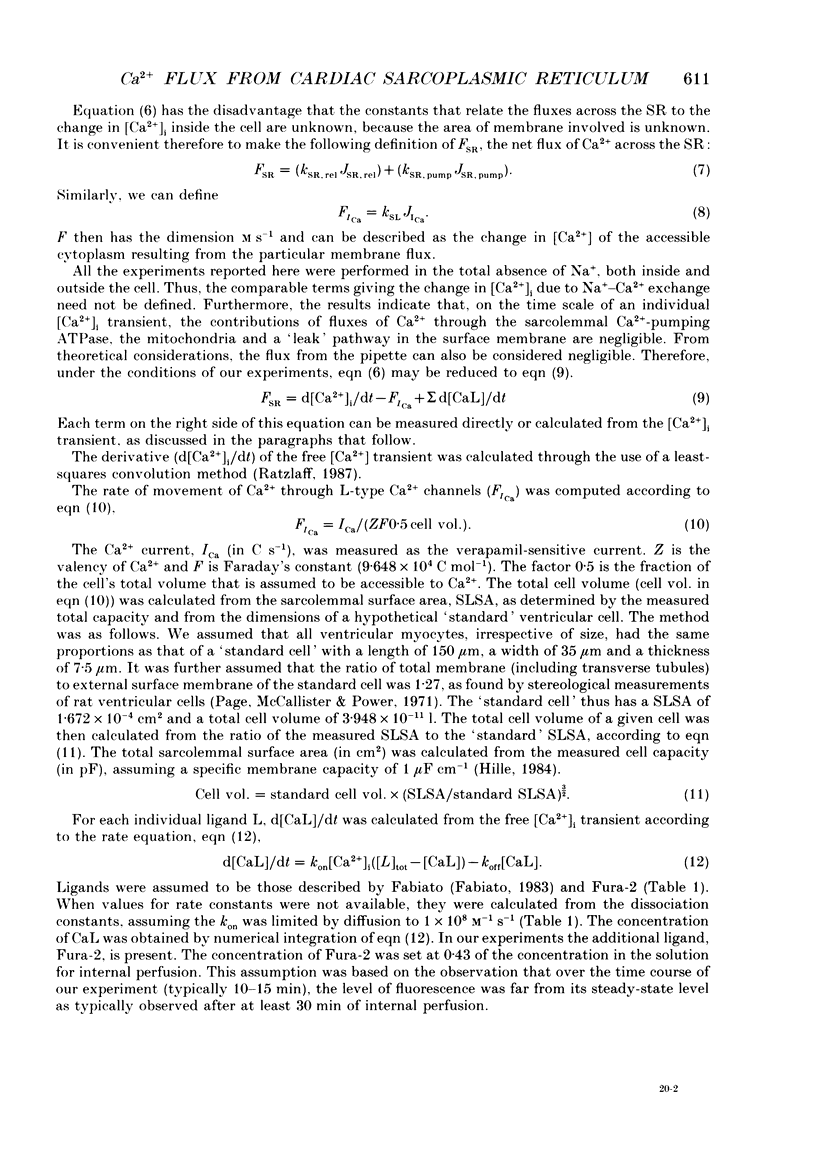
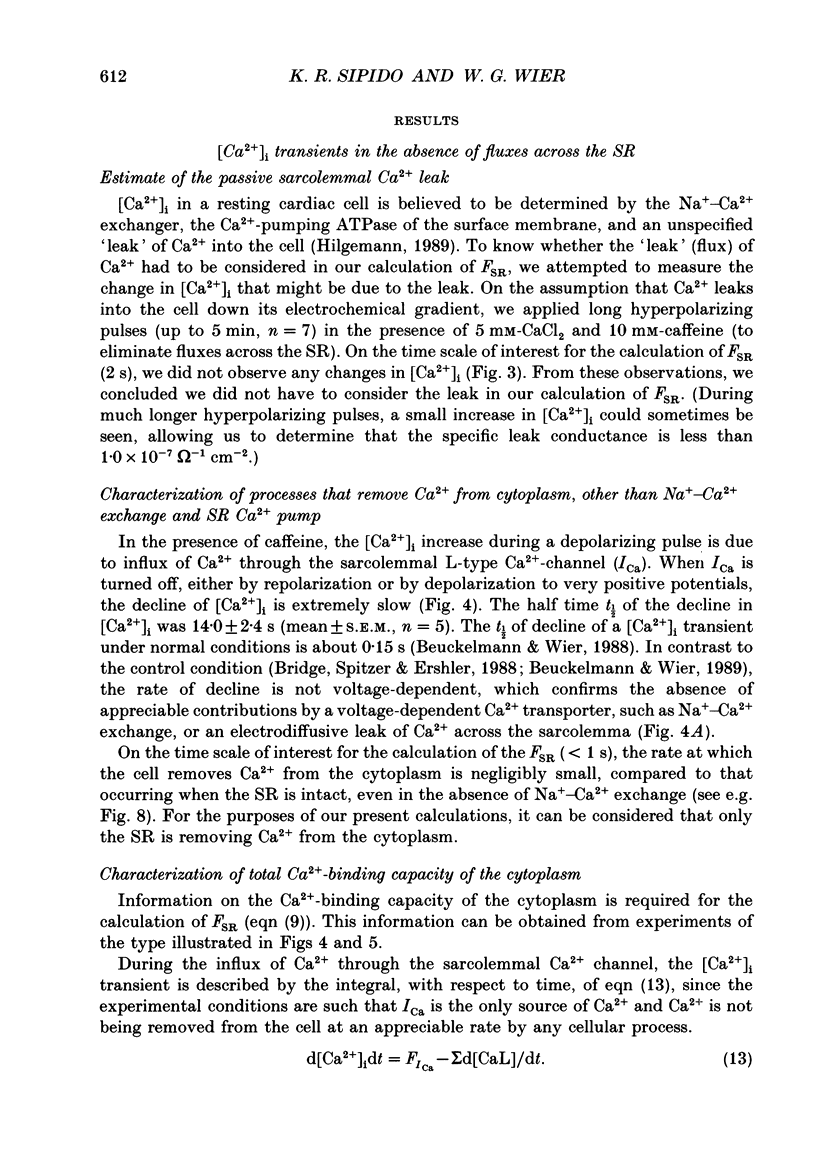
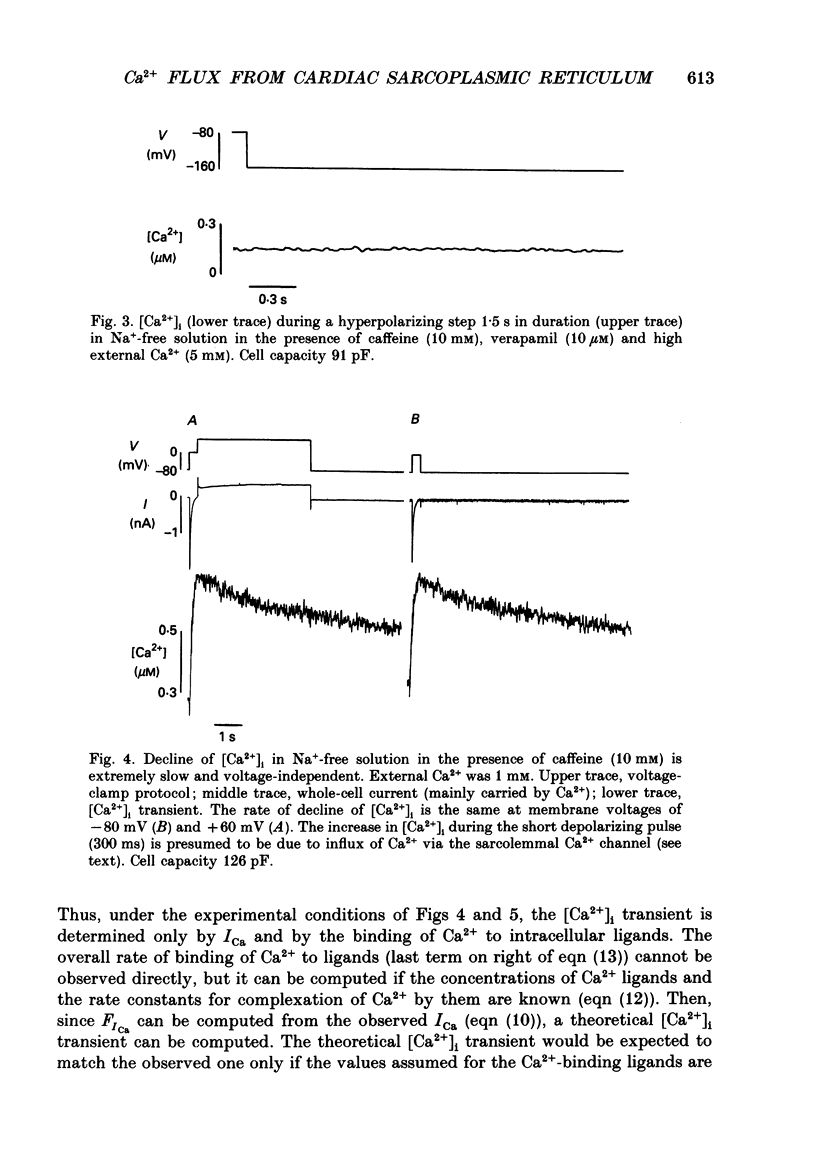
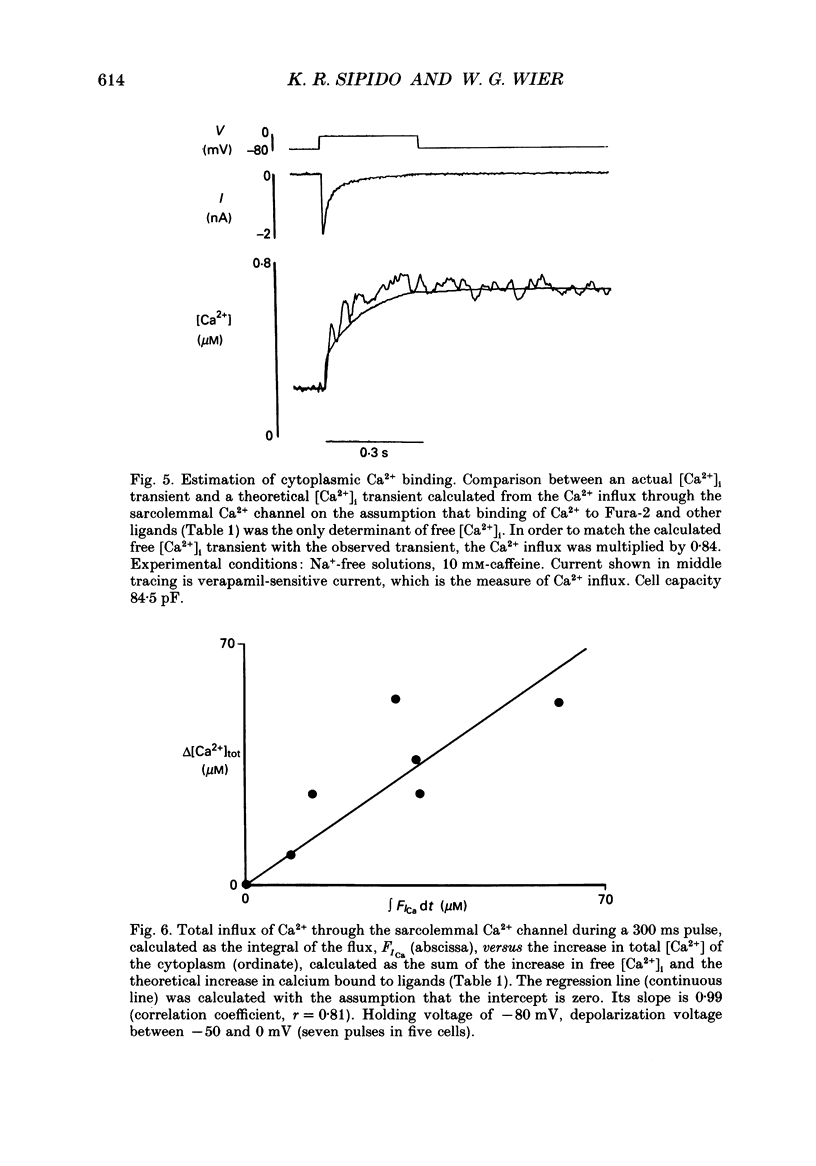
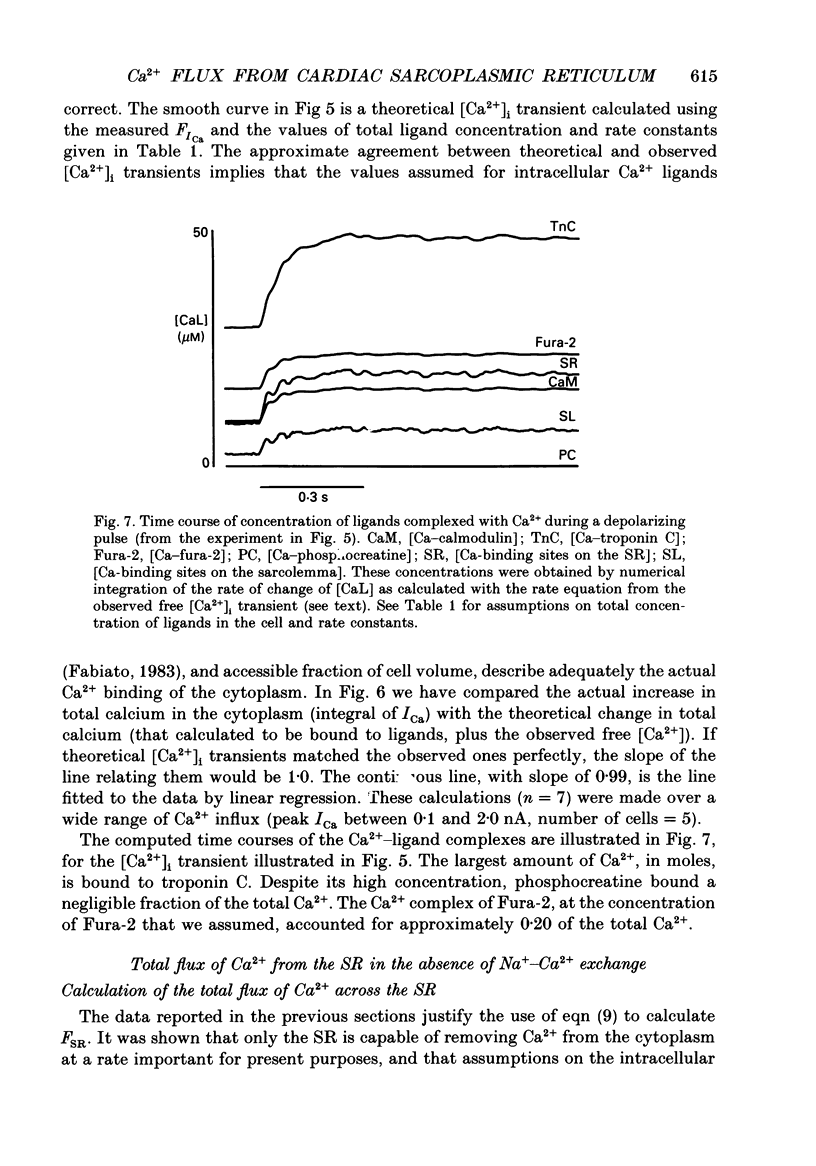
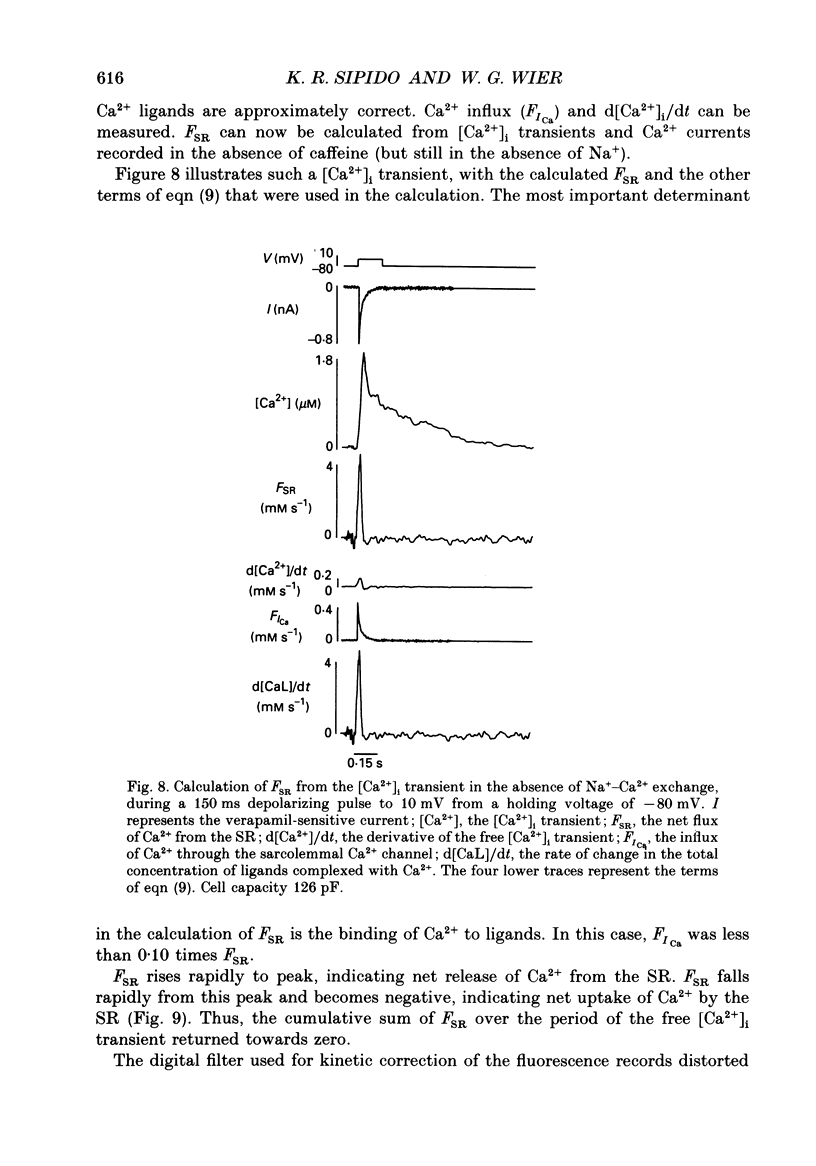
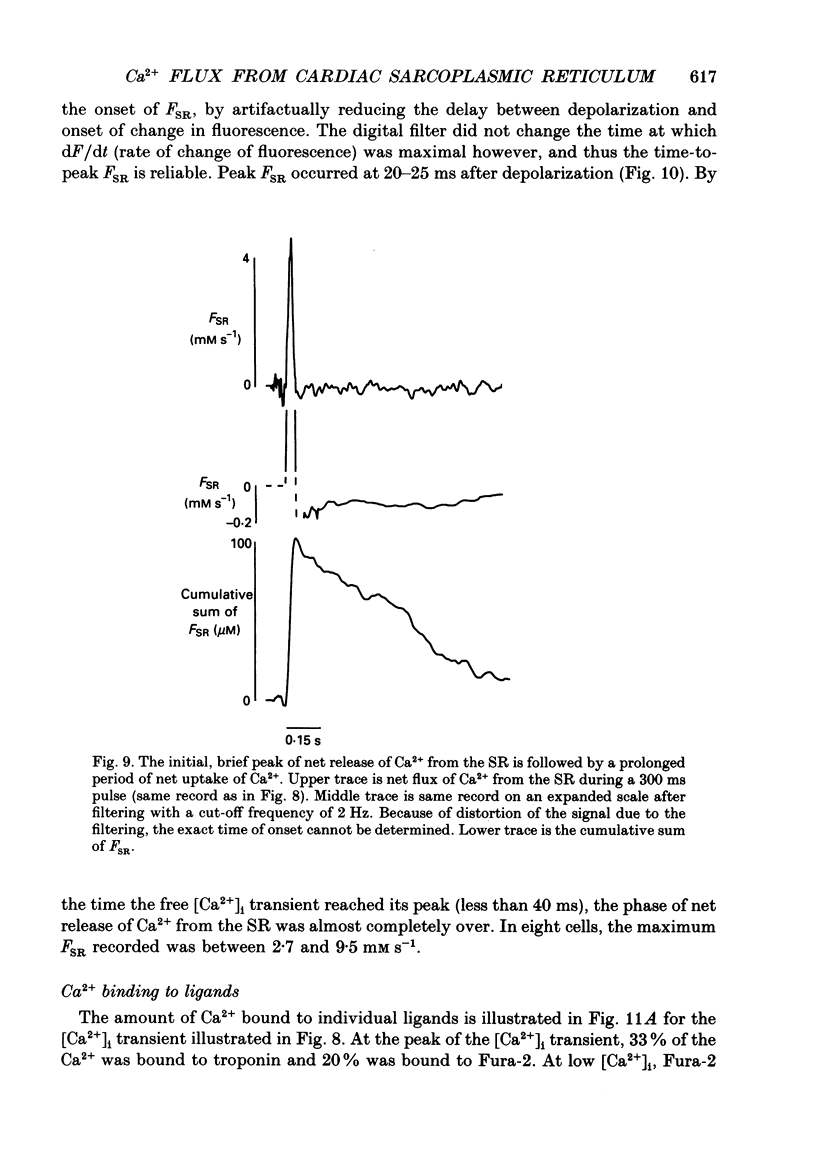
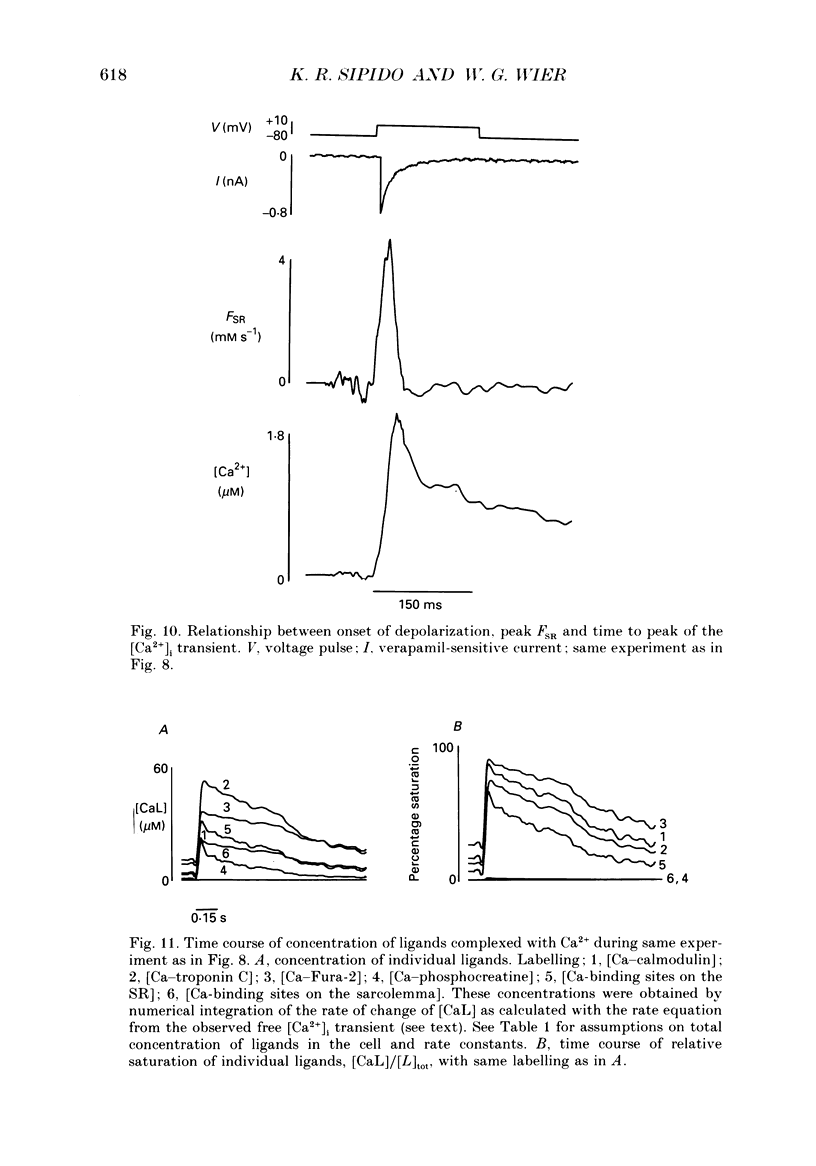
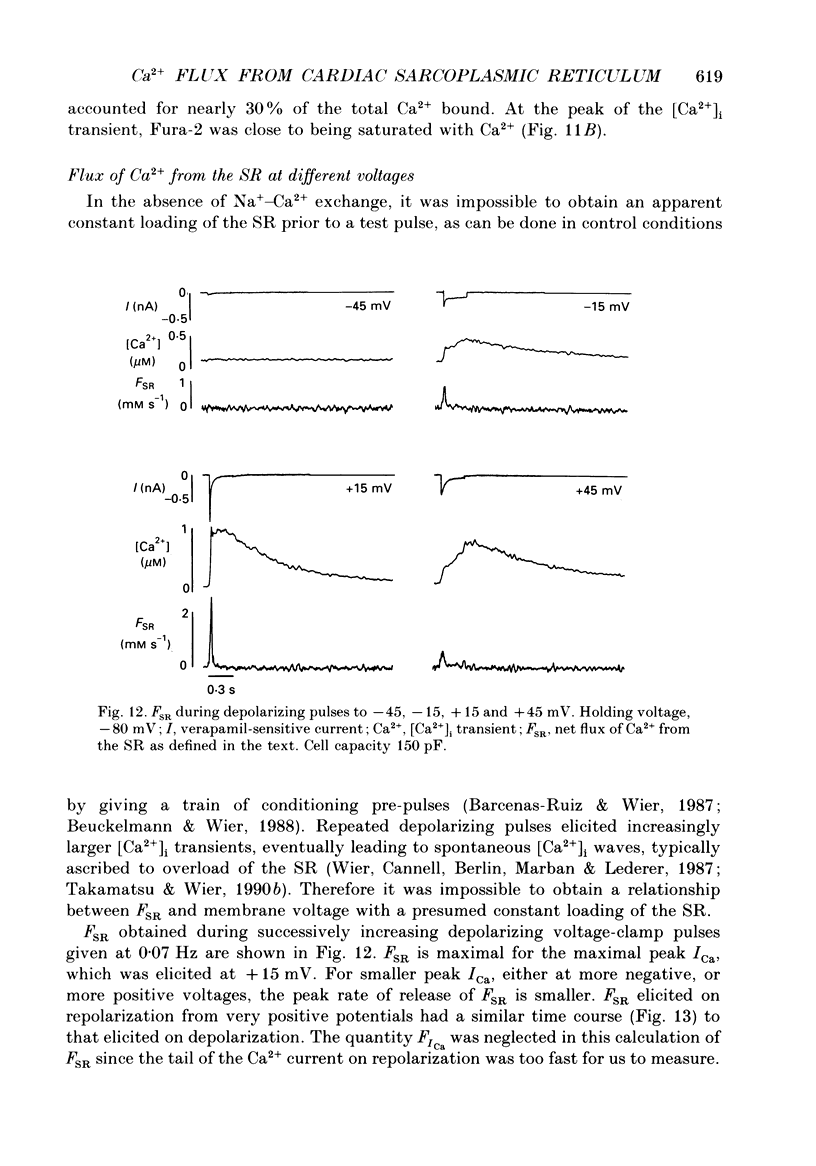
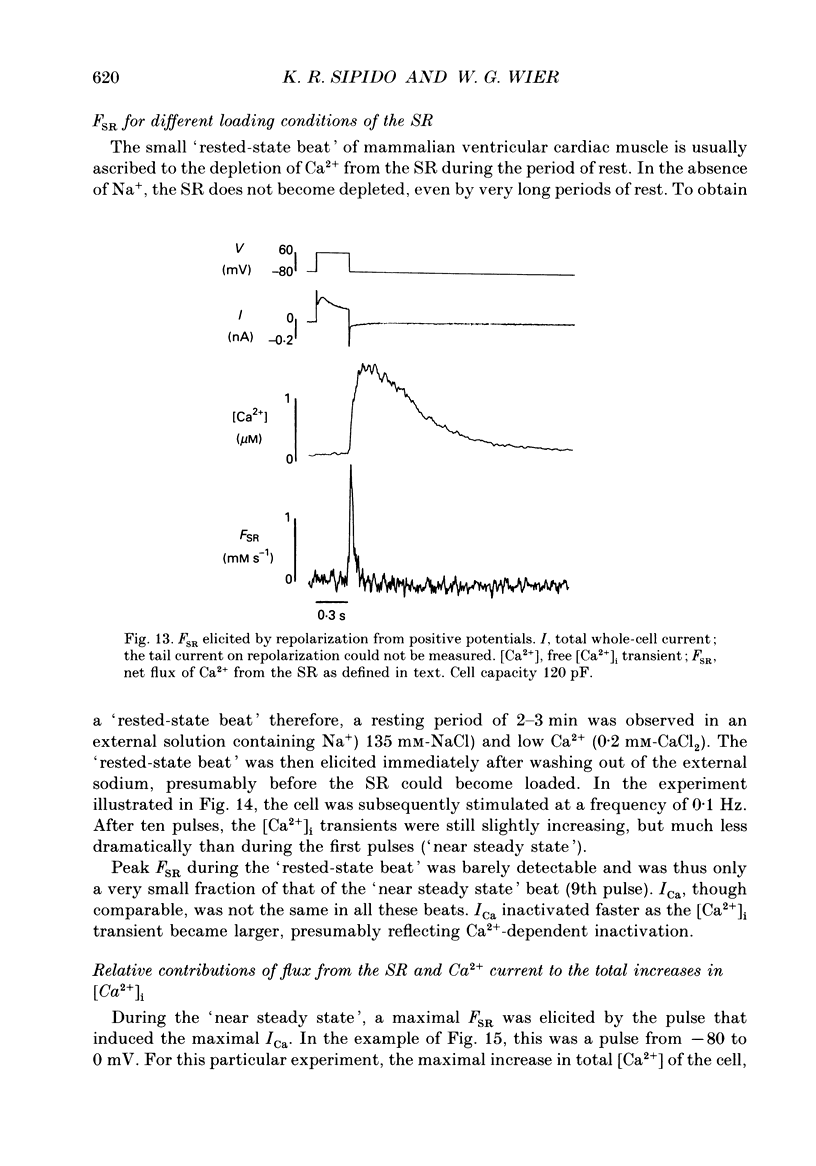
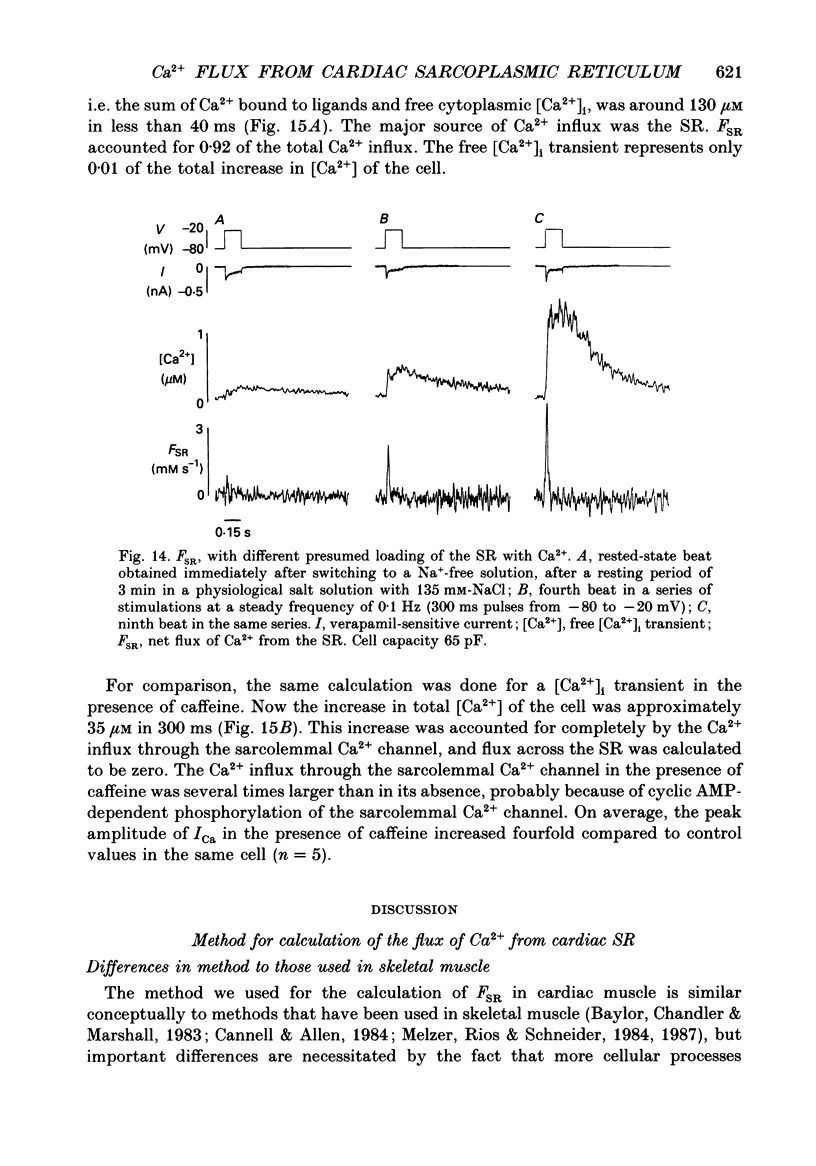
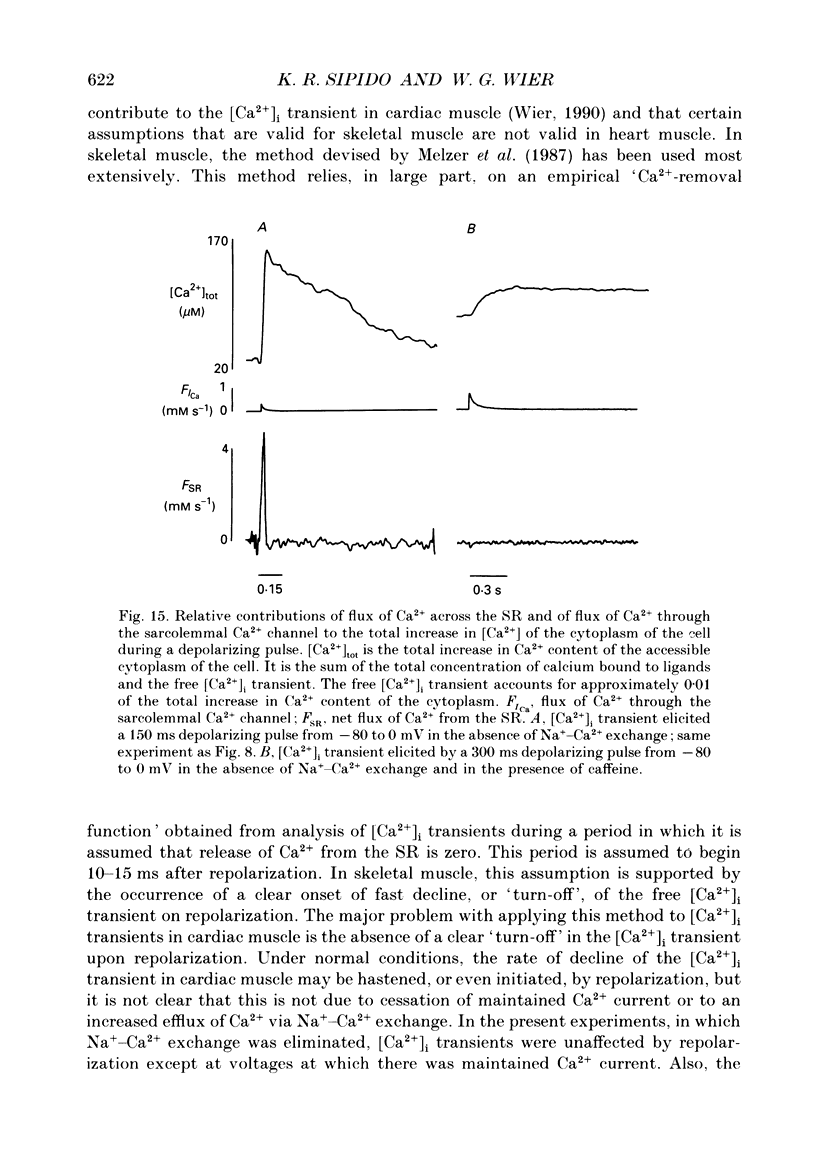
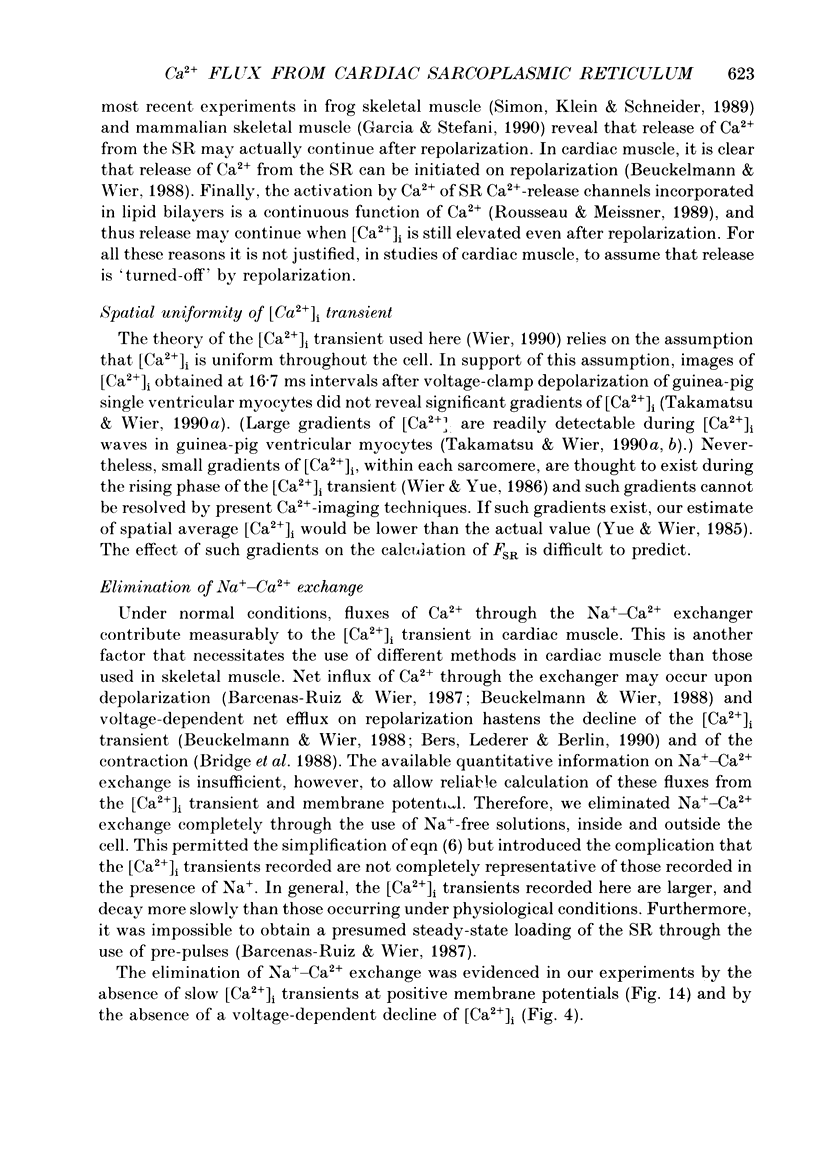
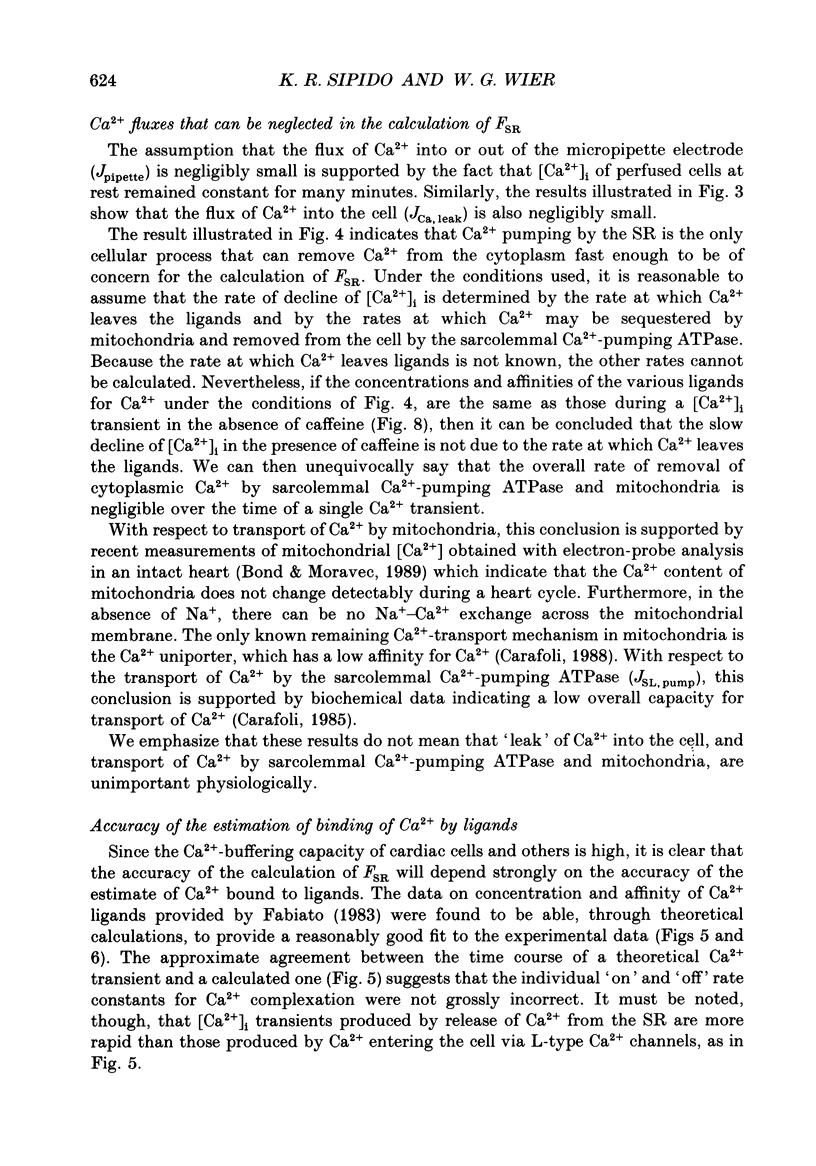
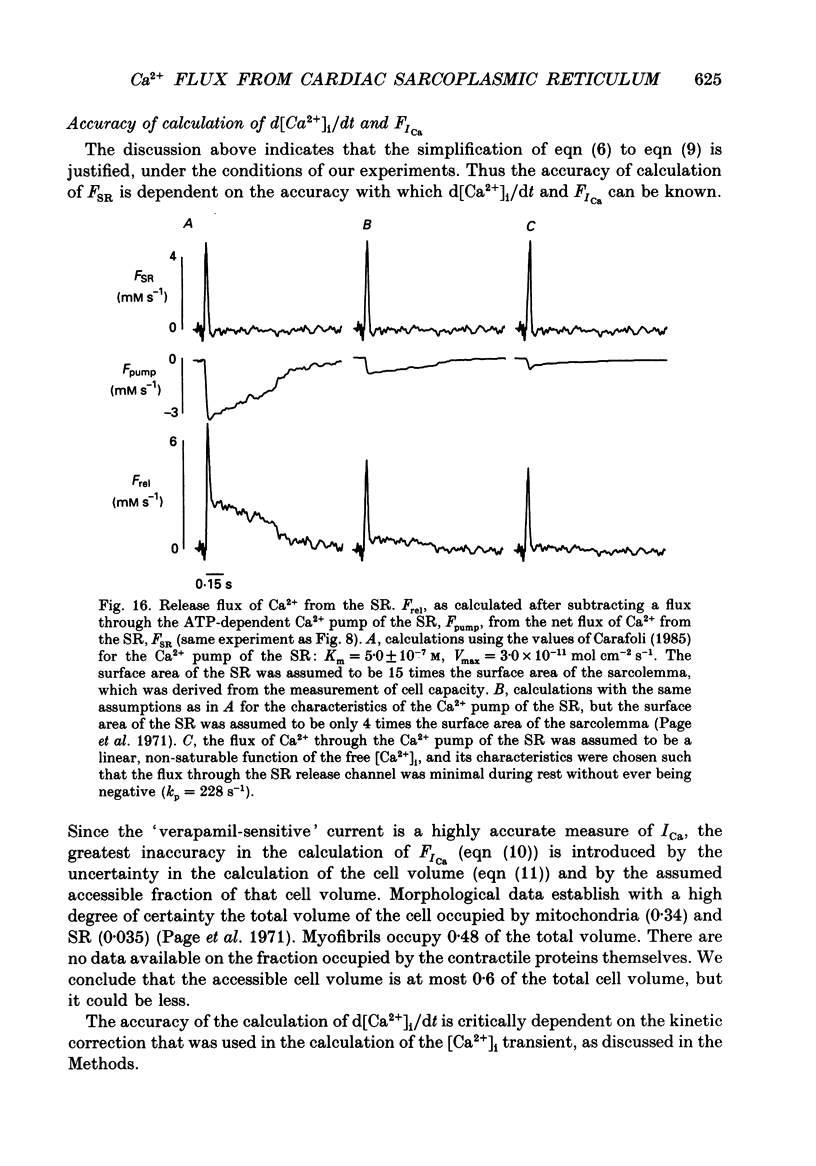
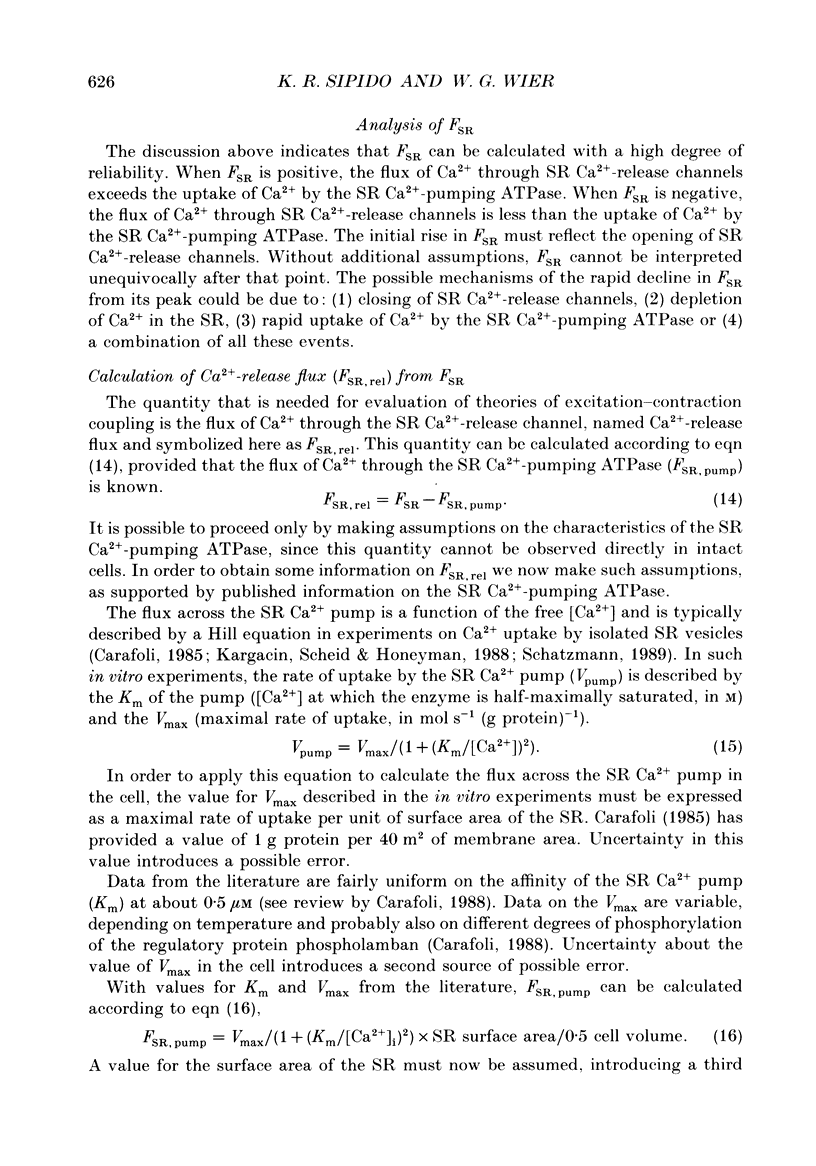
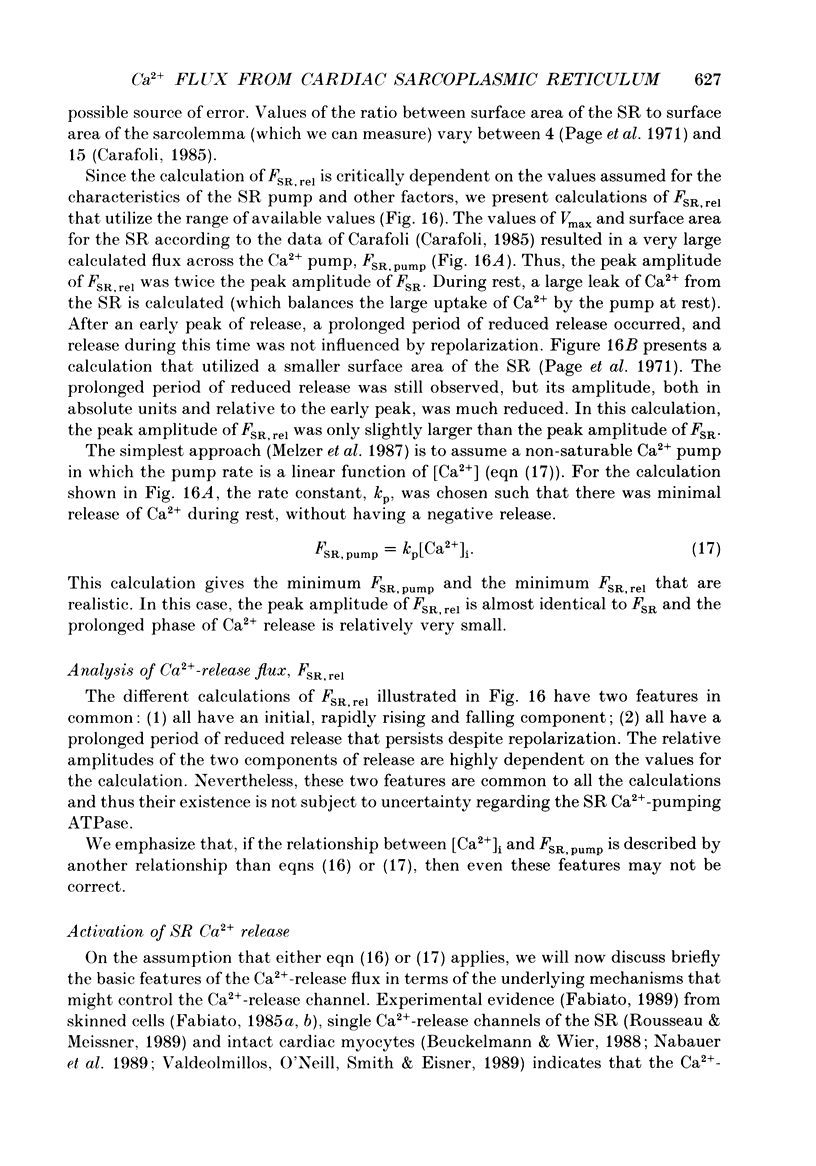
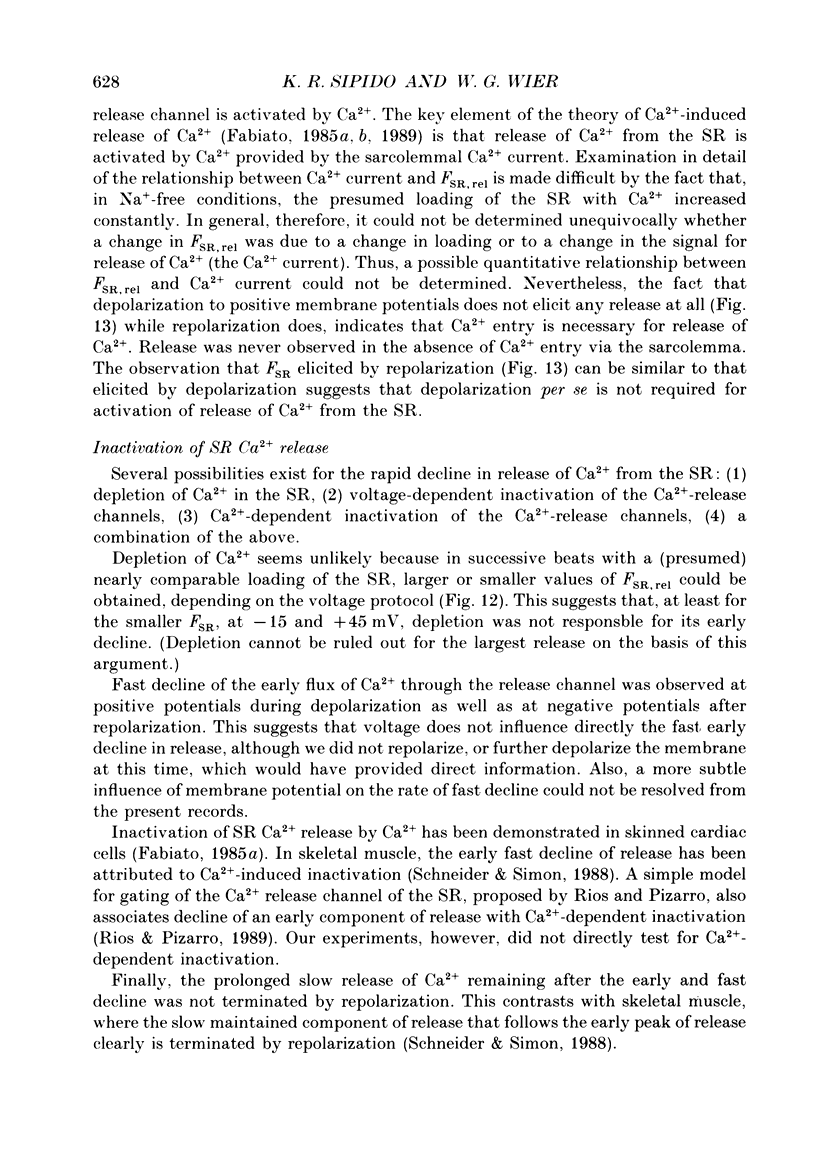
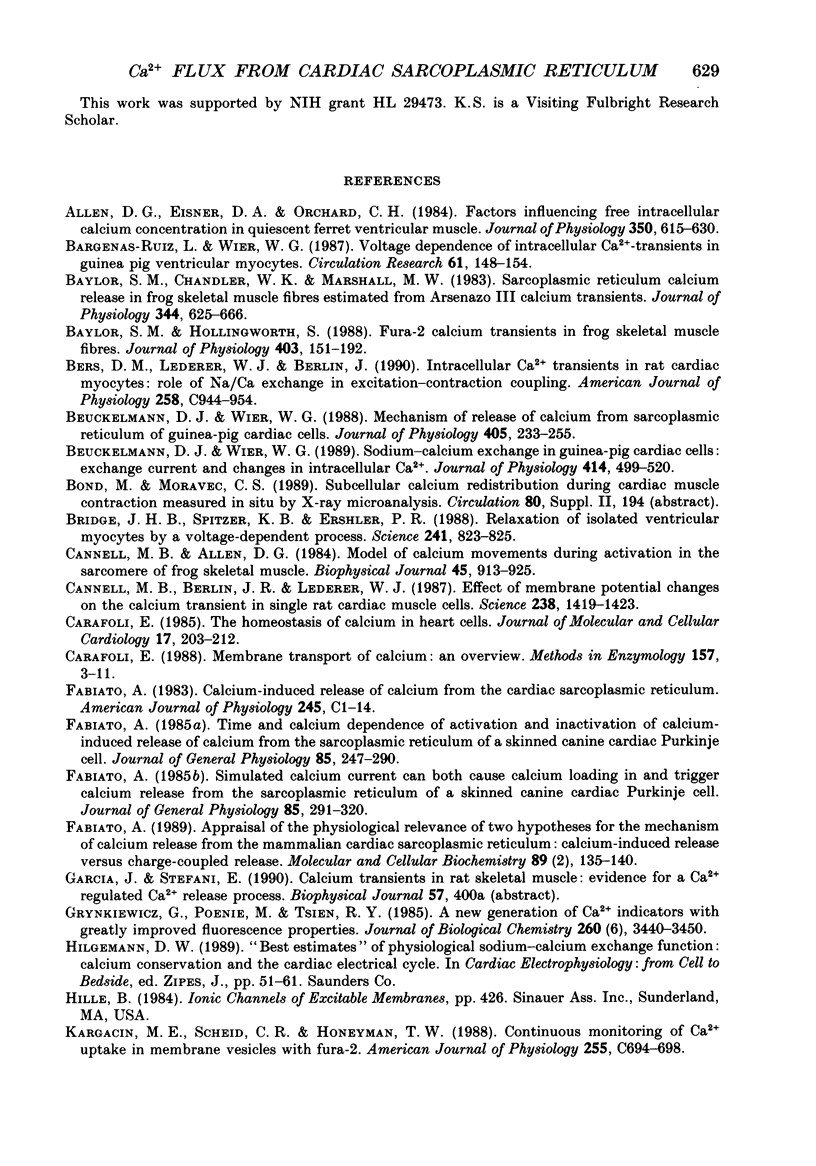
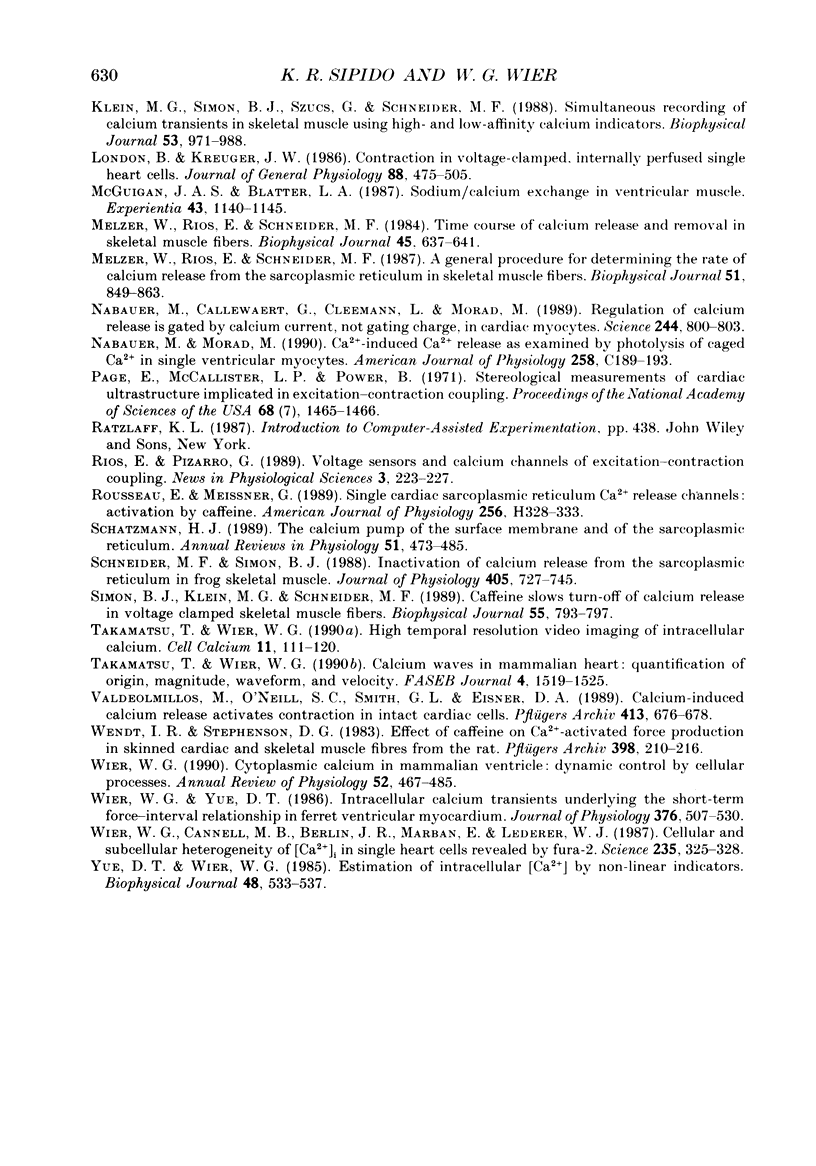
Selected References
These references are in PubMed. This may not be the complete list of references from this article.
- Allen D. G., Eisner D. A., Orchard C. H. Factors influencing free intracellular calcium concentration in quiescent ferret ventricular muscle. J Physiol. 1984 May;350:615–630. doi: 10.1113/jphysiol.1984.sp015221. [DOI] [PMC free article] [PubMed] [Google Scholar]
- Barcenas-Ruiz L., Wier W. G. Voltage dependence of intracellular [Ca2+]i transients in guinea pig ventricular myocytes. Circ Res. 1987 Jul;61(1):148–154. doi: 10.1161/01.res.61.1.148. [DOI] [PubMed] [Google Scholar]
- Baylor S. M., Chandler W. K., Marshall M. W. Sarcoplasmic reticulum calcium release in frog skeletal muscle fibres estimated from Arsenazo III calcium transients. J Physiol. 1983 Nov;344:625–666. doi: 10.1113/jphysiol.1983.sp014959. [DOI] [PMC free article] [PubMed] [Google Scholar]
- Baylor S. M., Hollingworth S. Fura-2 calcium transients in frog skeletal muscle fibres. J Physiol. 1988 Sep;403:151–192. doi: 10.1113/jphysiol.1988.sp017244. [DOI] [PMC free article] [PubMed] [Google Scholar]
- Bers D. M., Lederer W. J., Berlin J. R. Intracellular Ca transients in rat cardiac myocytes: role of Na-Ca exchange in excitation-contraction coupling. Am J Physiol. 1990 May;258(5 Pt 1):C944–C954. doi: 10.1152/ajpcell.1990.258.5.C944. [DOI] [PubMed] [Google Scholar]
- Beuckelmann D. J., Wier W. G. Mechanism of release of calcium from sarcoplasmic reticulum of guinea-pig cardiac cells. J Physiol. 1988 Nov;405:233–255. doi: 10.1113/jphysiol.1988.sp017331. [DOI] [PMC free article] [PubMed] [Google Scholar]
- Beuckelmann D. J., Wier W. G. Sodium-calcium exchange in guinea-pig cardiac cells: exchange current and changes in intracellular Ca2+. J Physiol. 1989 Jul;414:499–520. doi: 10.1113/jphysiol.1989.sp017700. [DOI] [PMC free article] [PubMed] [Google Scholar]
- Bridge J. H., Spitzer K. W., Ershler P. R. Relaxation of isolated ventricular cardiomyocytes by a voltage-dependent process. Science. 1988 Aug 12;241(4867):823–825. doi: 10.1126/science.3406740. [DOI] [PubMed] [Google Scholar]
- Cannell M. B., Allen D. G. Model of calcium movements during activation in the sarcomere of frog skeletal muscle. Biophys J. 1984 May;45(5):913–925. doi: 10.1016/S0006-3495(84)84238-1. [DOI] [PMC free article] [PubMed] [Google Scholar]
- Cannell M. B., Berlin J. R., Lederer W. J. Effect of membrane potential changes on the calcium transient in single rat cardiac muscle cells. Science. 1987 Dec 4;238(4832):1419–1423. doi: 10.1126/science.2446391. [DOI] [PubMed] [Google Scholar]
- Carafoli E. Membrane transport of calcium: an overview. Methods Enzymol. 1988;157:3–11. doi: 10.1016/0076-6879(88)57063-5. [DOI] [PubMed] [Google Scholar]
- Carafoli E. The homeostasis of calcium in heart cells. J Mol Cell Cardiol. 1985 Mar;17(3):203–212. doi: 10.1016/s0022-2828(85)80003-1. [DOI] [PubMed] [Google Scholar]
- Fabiato A. Appraisal of the physiological relevance of two hypothesis for the mechanism of calcium release from the mammalian cardiac sarcoplasmic reticulum: calcium-induced release versus charge-coupled release. Mol Cell Biochem. 1989 Sep 7;89(2):135–140. doi: 10.1007/BF00220765. [DOI] [PubMed] [Google Scholar]
- Fabiato A. Calcium-induced release of calcium from the cardiac sarcoplasmic reticulum. Am J Physiol. 1983 Jul;245(1):C1–14. doi: 10.1152/ajpcell.1983.245.1.C1. [DOI] [PubMed] [Google Scholar]
- Fabiato A. Simulated calcium current can both cause calcium loading in and trigger calcium release from the sarcoplasmic reticulum of a skinned canine cardiac Purkinje cell. J Gen Physiol. 1985 Feb;85(2):291–320. doi: 10.1085/jgp.85.2.291. [DOI] [PMC free article] [PubMed] [Google Scholar]
- Fabiato A. Time and calcium dependence of activation and inactivation of calcium-induced release of calcium from the sarcoplasmic reticulum of a skinned canine cardiac Purkinje cell. J Gen Physiol. 1985 Feb;85(2):247–289. doi: 10.1085/jgp.85.2.247. [DOI] [PMC free article] [PubMed] [Google Scholar]
- Grynkiewicz G., Poenie M., Tsien R. Y. A new generation of Ca2+ indicators with greatly improved fluorescence properties. J Biol Chem. 1985 Mar 25;260(6):3440–3450. [PubMed] [Google Scholar]
- Kargacin M. E., Scheid C. R., Honeyman T. W. Continuous monitoring of Ca2+ uptake in membrane vesicles with fura-2. Am J Physiol. 1988 Nov;255(5 Pt 1):C694–C698. doi: 10.1152/ajpcell.1988.255.5.C694. [DOI] [PubMed] [Google Scholar]
- Klein M. G., Simon B. J., Szucs G., Schneider M. F. Simultaneous recording of calcium transients in skeletal muscle using high- and low-affinity calcium indicators. Biophys J. 1988 Jun;53(6):971–988. doi: 10.1016/S0006-3495(88)83178-3. [DOI] [PMC free article] [PubMed] [Google Scholar]
- London B., Krueger J. W. Contraction in voltage-clamped, internally perfused single heart cells. J Gen Physiol. 1986 Oct;88(4):475–505. doi: 10.1085/jgp.88.4.475. [DOI] [PMC free article] [PubMed] [Google Scholar]
- McGuigan J. A., Blatter L. A. Sodium/calcium exchange in ventricular muscle. Experientia. 1987 Dec 1;43(11-12):1140–1145. doi: 10.1007/BF01945512. [DOI] [PubMed] [Google Scholar]
- Melzer W., Rios E., Schneider M. F. A general procedure for determining the rate of calcium release from the sarcoplasmic reticulum in skeletal muscle fibers. Biophys J. 1987 Jun;51(6):849–863. doi: 10.1016/S0006-3495(87)83413-6. [DOI] [PMC free article] [PubMed] [Google Scholar]
- Melzer W., Rios E., Schneider M. F. Time course of calcium release and removal in skeletal muscle fibers. Biophys J. 1984 Mar;45(3):637–641. doi: 10.1016/S0006-3495(84)84203-4. [DOI] [PMC free article] [PubMed] [Google Scholar]
- Näbauer M., Callewaert G., Cleemann L., Morad M. Regulation of calcium release is gated by calcium current, not gating charge, in cardiac myocytes. Science. 1989 May 19;244(4906):800–803. doi: 10.1126/science.2543067. [DOI] [PubMed] [Google Scholar]
- Näbauer M., Morad M. Ca2(+)-induced Ca2+ release as examined by photolysis of caged Ca2+ in single ventricular myocytes. Am J Physiol. 1990 Jan;258(1 Pt 1):C189–C193. doi: 10.1152/ajpcell.1990.258.1.C189. [DOI] [PubMed] [Google Scholar]
- Page E., McCallister L. P., Power B. Sterological measurements of cardiac ultrastructures implicated in excitation-contraction coupling. Proc Natl Acad Sci U S A. 1971 Jul;68(7):1465–1466. doi: 10.1073/pnas.68.7.1465. [DOI] [PMC free article] [PubMed] [Google Scholar]
- Rousseau E., Meissner G. Single cardiac sarcoplasmic reticulum Ca2+-release channel: activation by caffeine. Am J Physiol. 1989 Feb;256(2 Pt 2):H328–H333. doi: 10.1152/ajpheart.1989.256.2.H328. [DOI] [PubMed] [Google Scholar]
- Schatzmann H. J. The calcium pump of the surface membrane and of the sarcoplasmic reticulum. Annu Rev Physiol. 1989;51:473–485. doi: 10.1146/annurev.ph.51.030189.002353. [DOI] [PubMed] [Google Scholar]
- Schneider M. F., Simon B. J. Inactivation of calcium release from the sarcoplasmic reticulum in frog skeletal muscle. J Physiol. 1988 Nov;405:727–745. doi: 10.1113/jphysiol.1988.sp017358. [DOI] [PMC free article] [PubMed] [Google Scholar]
- Simon B. J., Klein M. G., Schneider M. F. Caffeine slows turn-off of calcium release in voltage clamped skeletal muscle fibers. Biophys J. 1989 Apr;55(4):793–797. doi: 10.1016/S0006-3495(89)82878-4. [DOI] [PMC free article] [PubMed] [Google Scholar]
- Takamatsu T., Wier W. G. Calcium waves in mammalian heart: quantification of origin, magnitude, waveform, and velocity. FASEB J. 1990 Mar;4(5):1519–1525. doi: 10.1096/fasebj.4.5.2307330. [DOI] [PubMed] [Google Scholar]
- Takamatsu T., Wier W. G. High temporal resolution video imaging of intracellular calcium. Cell Calcium. 1990 Feb-Mar;11(2-3):111–120. doi: 10.1016/0143-4160(90)90064-2. [DOI] [PubMed] [Google Scholar]
- Valdeolmillos M., O'Neill S. C., Smith G. L., Eisner D. A. Calcium-induced calcium release activates contraction in intact cardiac cells. Pflugers Arch. 1989 Apr;413(6):676–678. doi: 10.1007/BF00581820. [DOI] [PubMed] [Google Scholar]
- Wendt I. R., Stephenson D. G. Effects of caffeine on Ca-activated force production in skinned cardiac and skeletal muscle fibres of the rat. Pflugers Arch. 1983 Aug;398(3):210–216. doi: 10.1007/BF00657153. [DOI] [PubMed] [Google Scholar]
- Wier W. G., Cannell M. B., Berlin J. R., Marban E., Lederer W. J. Cellular and subcellular heterogeneity of [Ca2+]i in single heart cells revealed by fura-2. Science. 1987 Jan 16;235(4786):325–328. doi: 10.1126/science.3798114. [DOI] [PubMed] [Google Scholar]
- Wier W. G. Cytoplasmic [Ca2+] in mammalian ventricle: dynamic control by cellular processes. Annu Rev Physiol. 1990;52:467–485. doi: 10.1146/annurev.ph.52.030190.002343. [DOI] [PubMed] [Google Scholar]
- Wier W. G., Yue D. T. Intracellular calcium transients underlying the short-term force-interval relationship in ferret ventricular myocardium. J Physiol. 1986 Jul;376:507–530. doi: 10.1113/jphysiol.1986.sp016167. [DOI] [PMC free article] [PubMed] [Google Scholar]
- Yue D. T., Wier W. G. Estimation of intracellular [Ca2+] by nonlinear indicators. A quantitative analysis. Biophys J. 1985 Sep;48(3):533–537. doi: 10.1016/S0006-3495(85)83810-8. [DOI] [PMC free article] [PubMed] [Google Scholar]