Abstract
1. Ion channels expressed in human dermal fibroblasts are characterized using the patch-clamp technique. 2. A number of different ion channels were found but their expression occurred at various frequencies. The most commonly found phenotype was the expression of voltage-gated K+ current. This 'typical' K+ current was seen in about 60% of the cells recorded. 3. Subtypes of voltage-gated K+ channels could be discerned by differences in gating kinetics. One has fast inactivation and resembles the 'A' K+ current. Additional subtypes were sometimes discerned based on activation kinetics. 4. The large-conductance Ca(2+)-activated K+ channel (maxi-K+) could be found in nearly every cell but required large depolarizations to activate using the standard Ca(2+)-buffered pipette solution (10(-8) M [Ca2+]i). 5. Inward rectifier K+ channels were seen in a low percentage of cells. The inward rectifier K+ current was sensitive to 'wash-out' if guanosine 5'-O-(3-thiotriphosphate) (GTP gamma S) was included in the pipette solution dialysing the cell. 6. Tetrodotoxin (TTX)-sensitive voltage-gated Na+ channels were seen but in a lower number of cells recorded, about 20%. Evidence for subtypes of Na+ channels were sometimes seen based on differences in gating kinetics. 7. An ATP-dependent osmotically activated Cl- current was also found. This current showed some outward rectification but was otherwise voltage independent. 8. In addition, a cell-to-cell contact-associated K+ current was described. This current was linear over the voltage ranges used and whose gating correlated with the existence of gap junctions. 9. These currents were characterized to determine the baseline behaviour of unstimulated cells and to compare to bradykinin-stimulated cells described in the following paper. As unexcitable cells, human dermal fibroblasts are capable of expressing a surprising diversity of ion channel phenotypes and of ion channel modulations.
Full text
PDF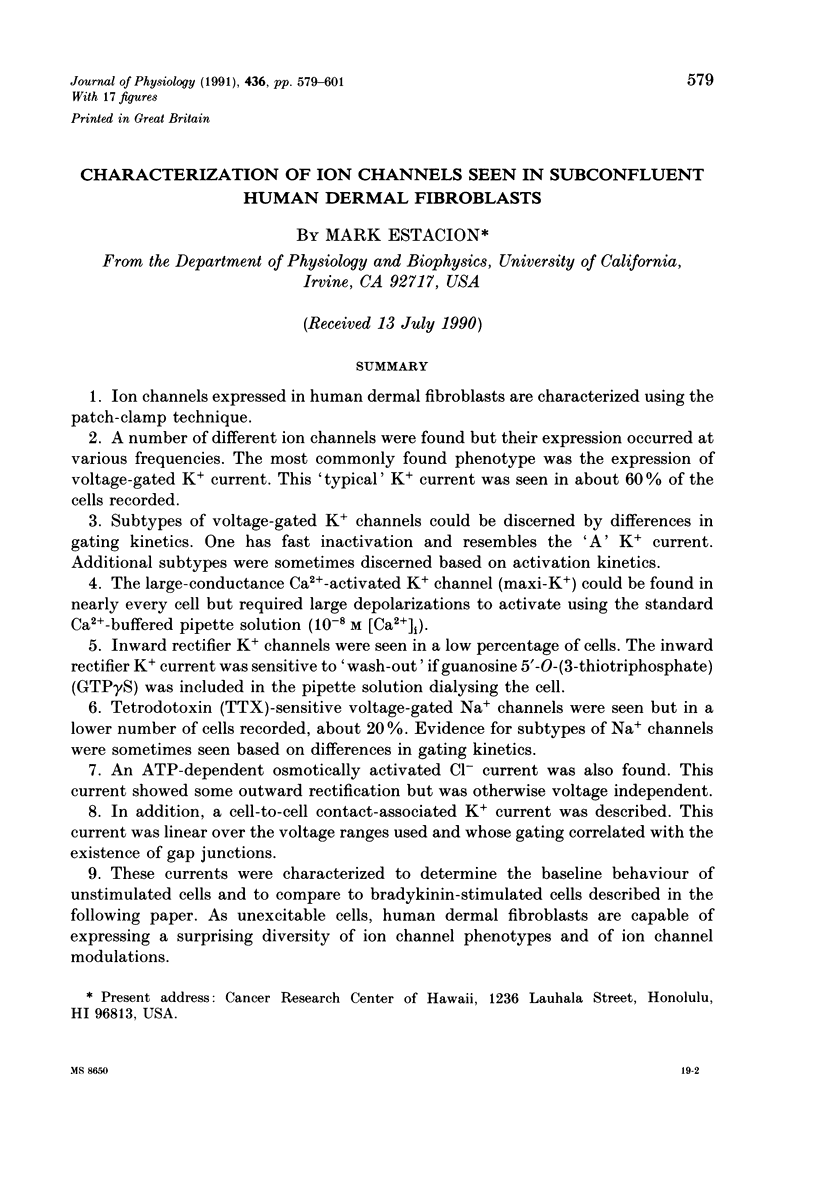
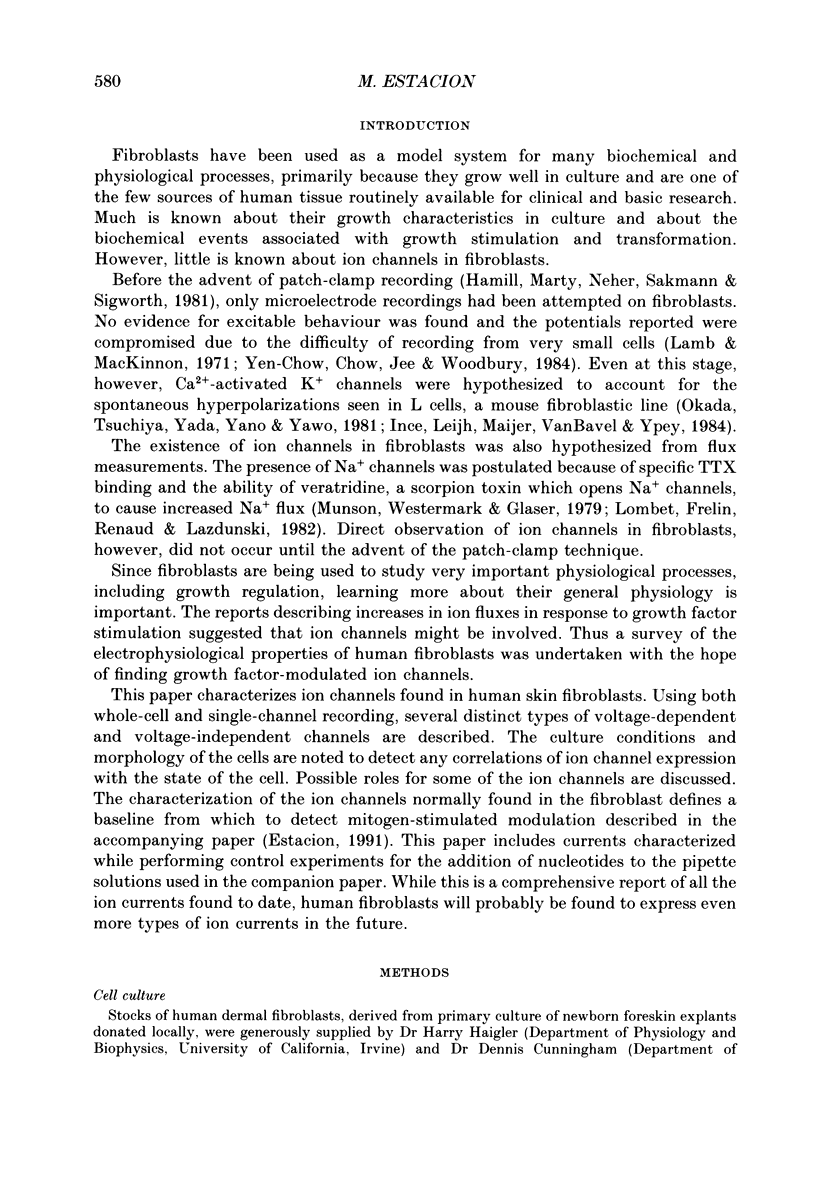
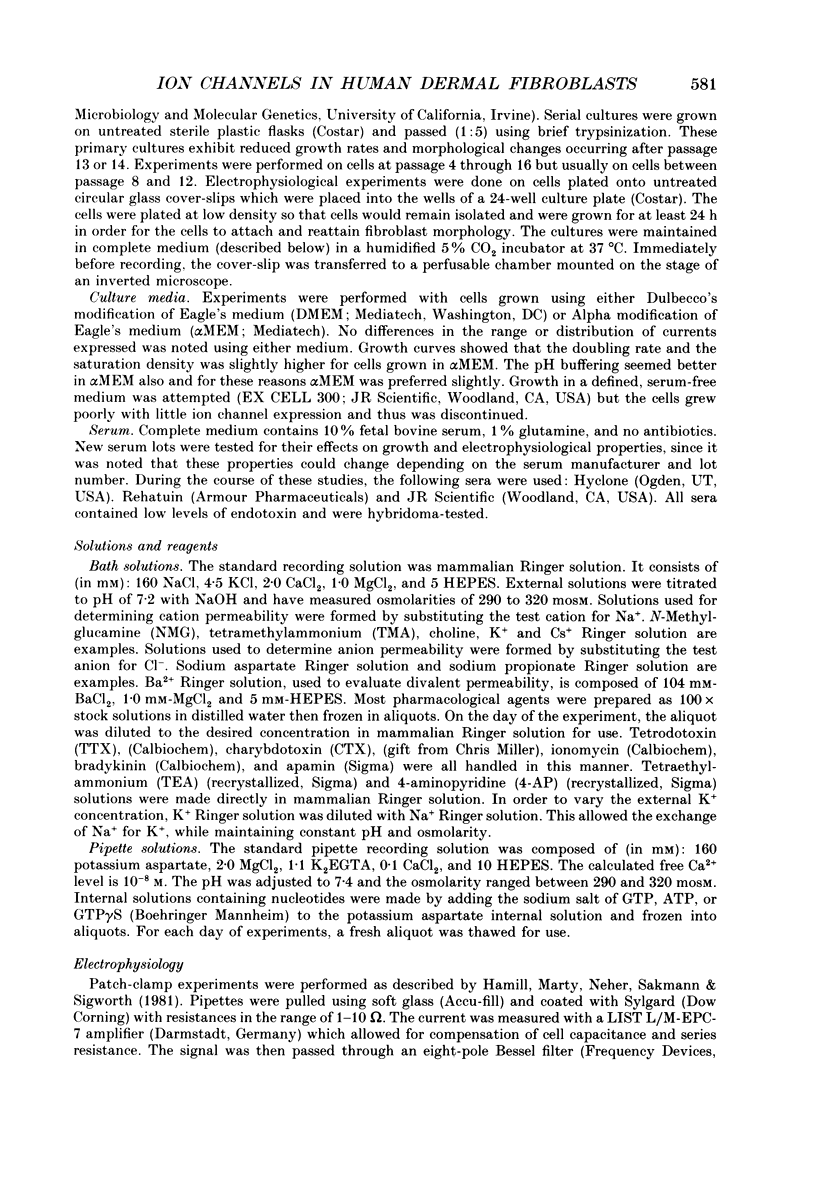
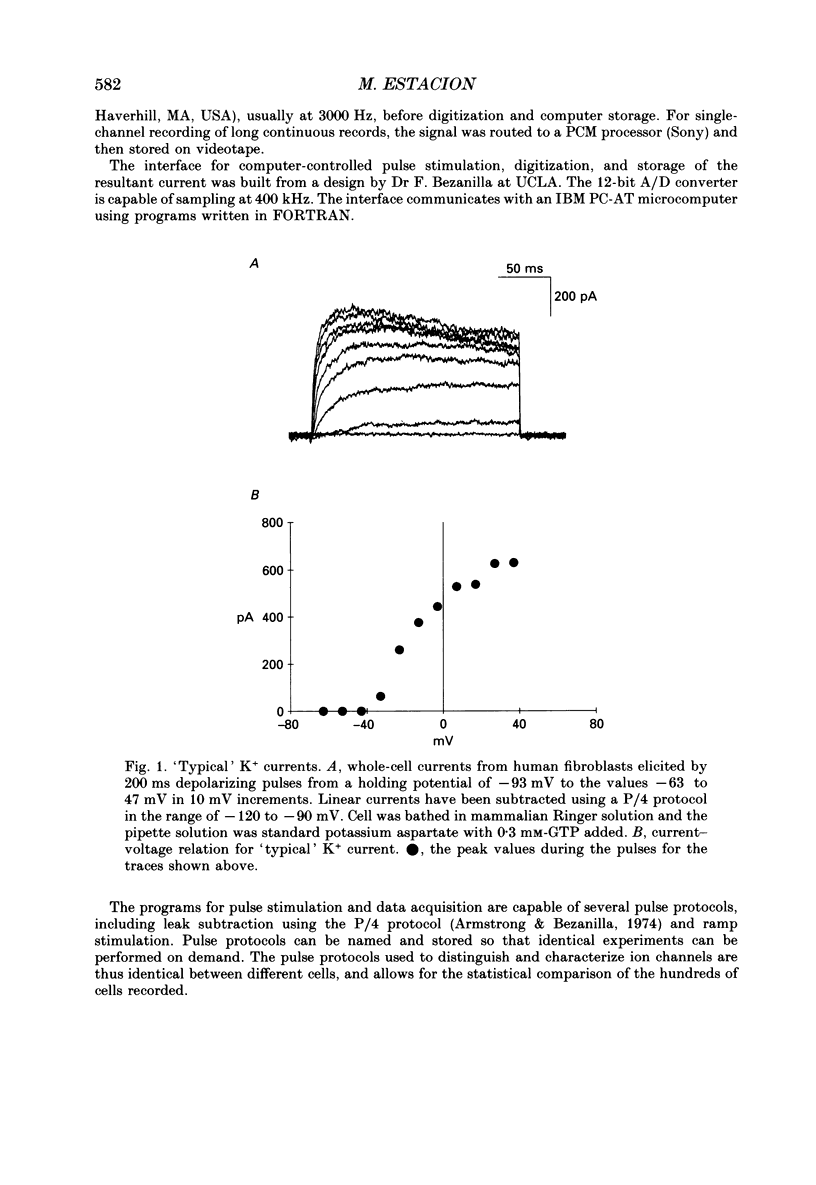
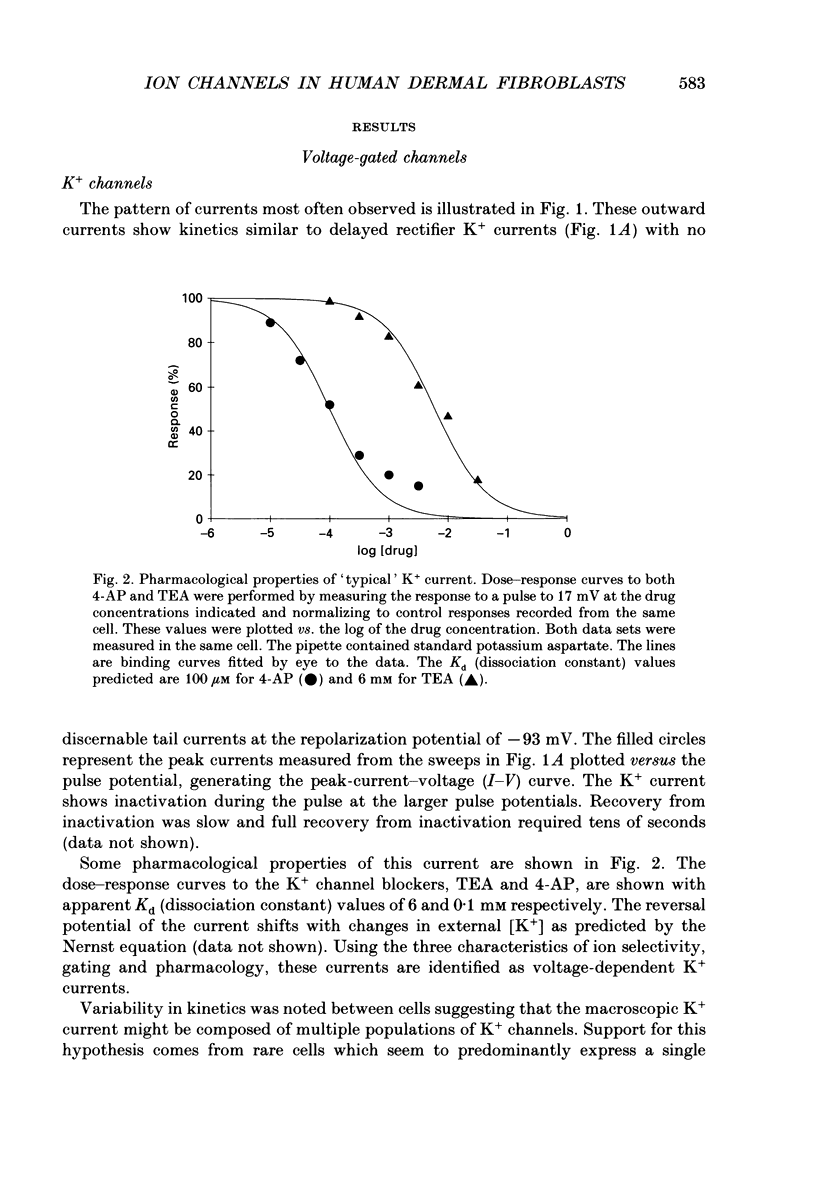
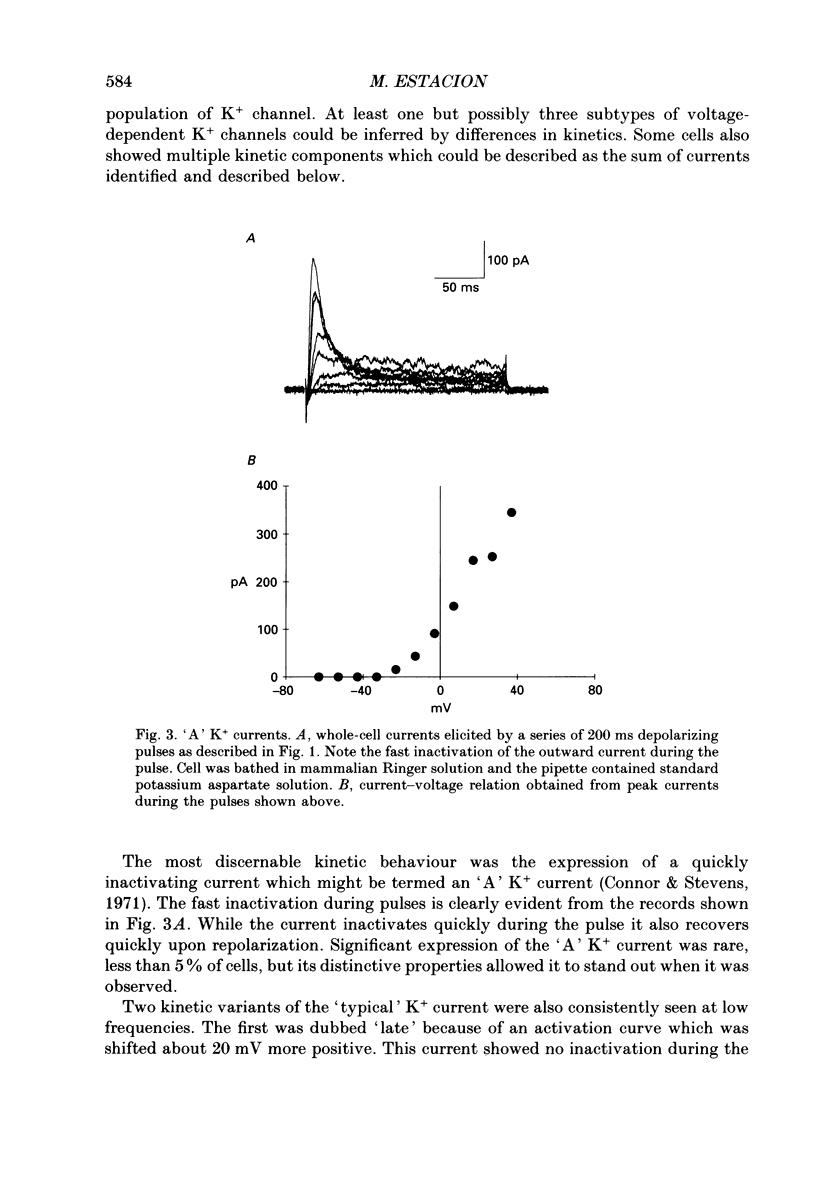
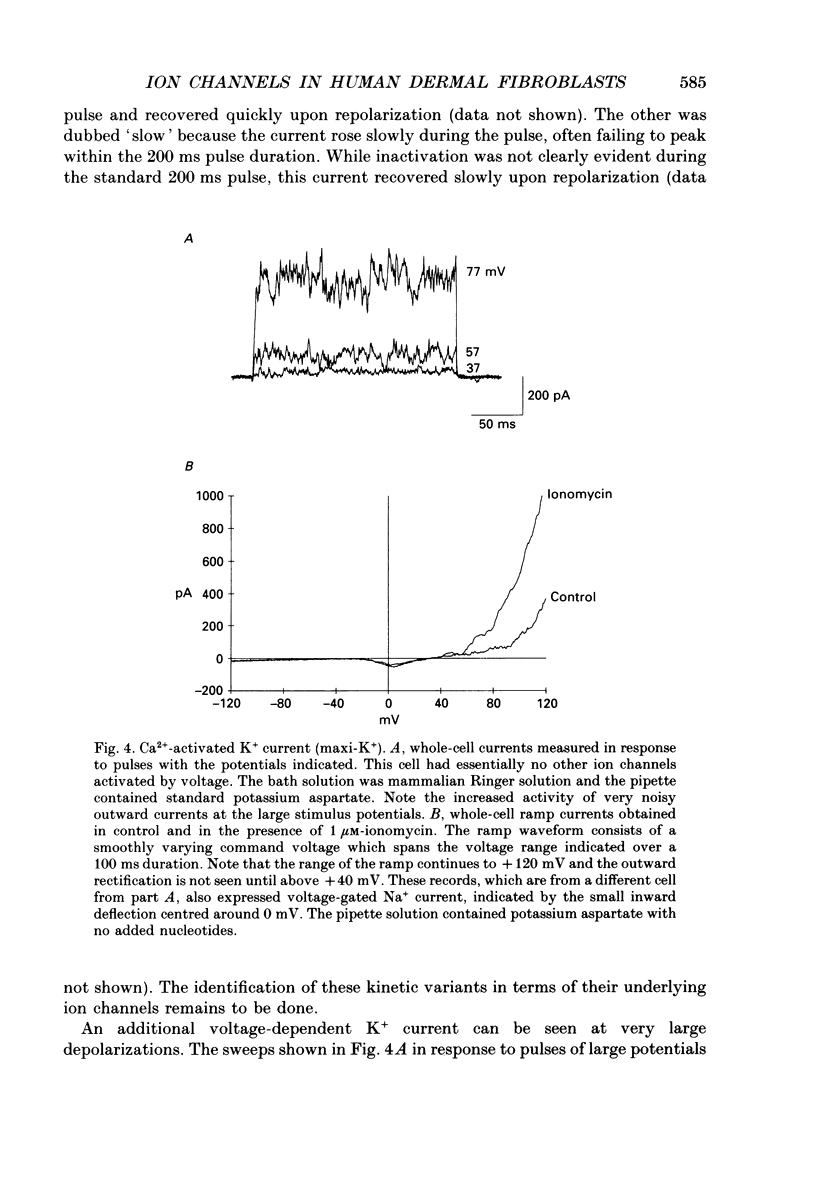
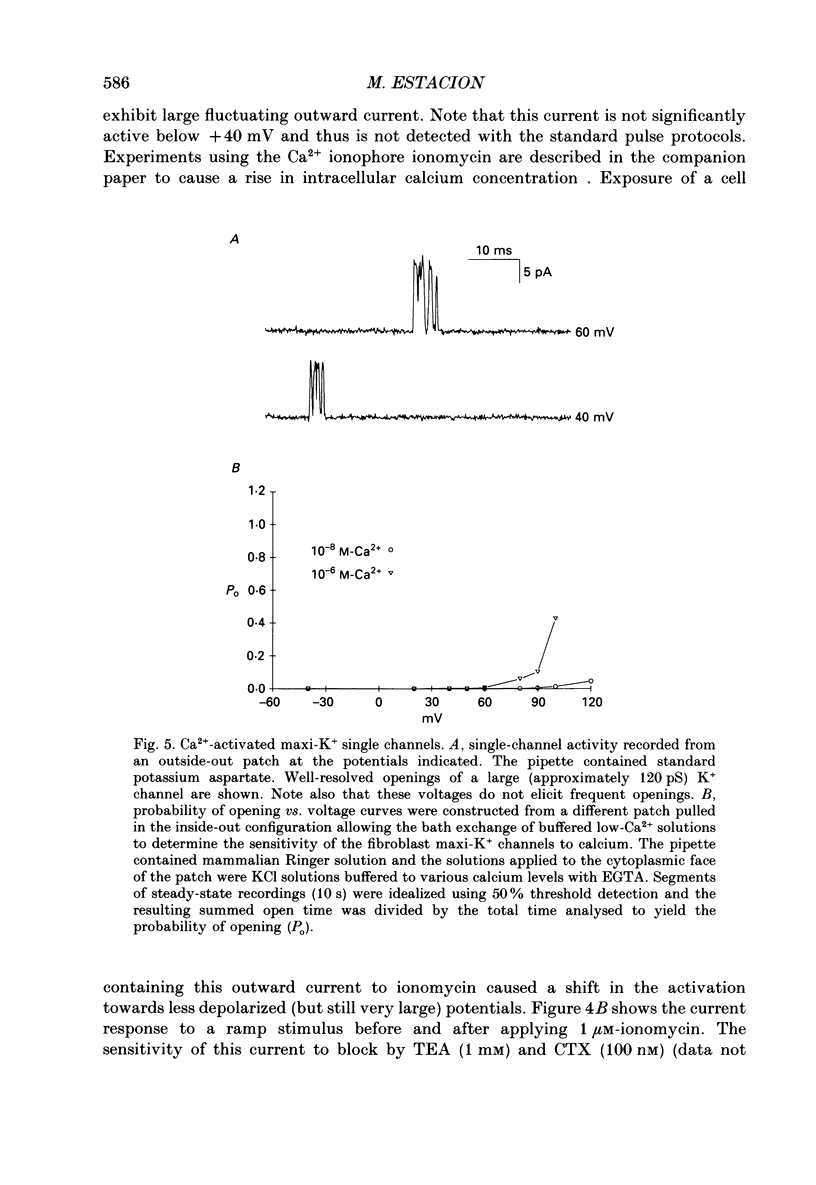
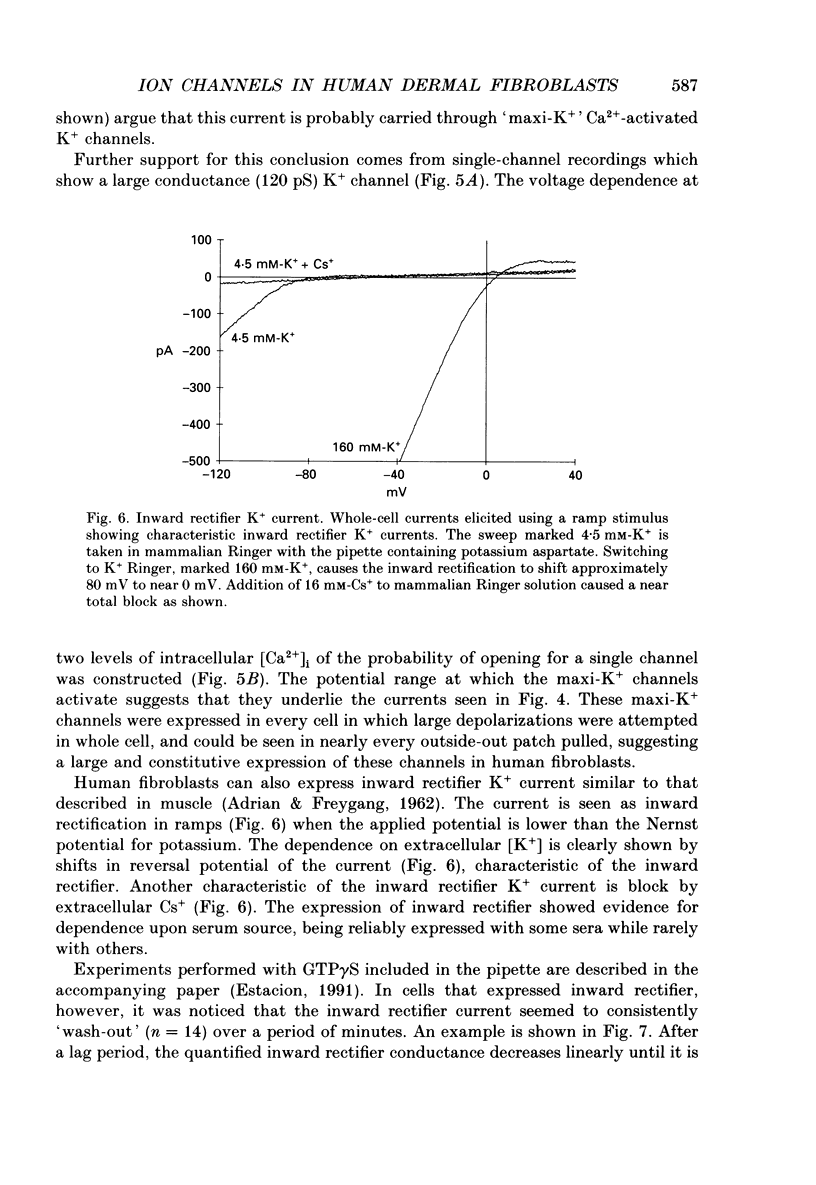
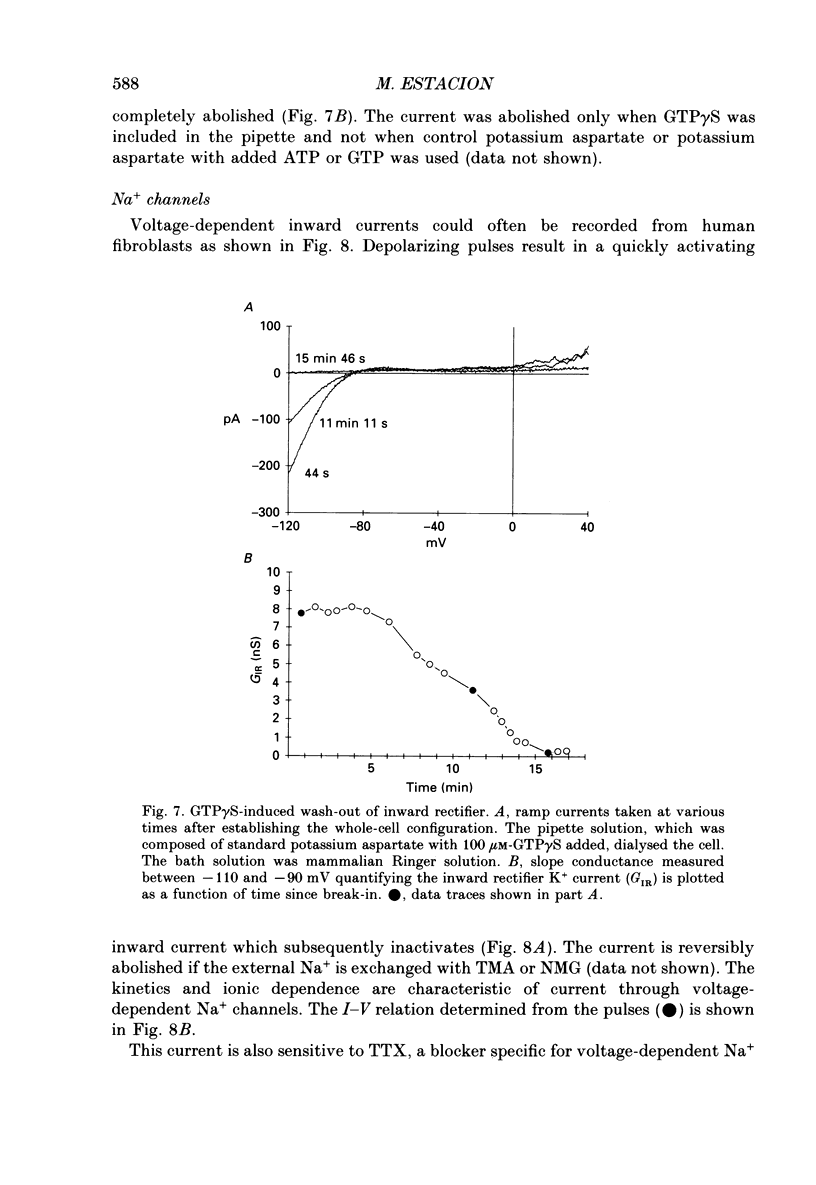
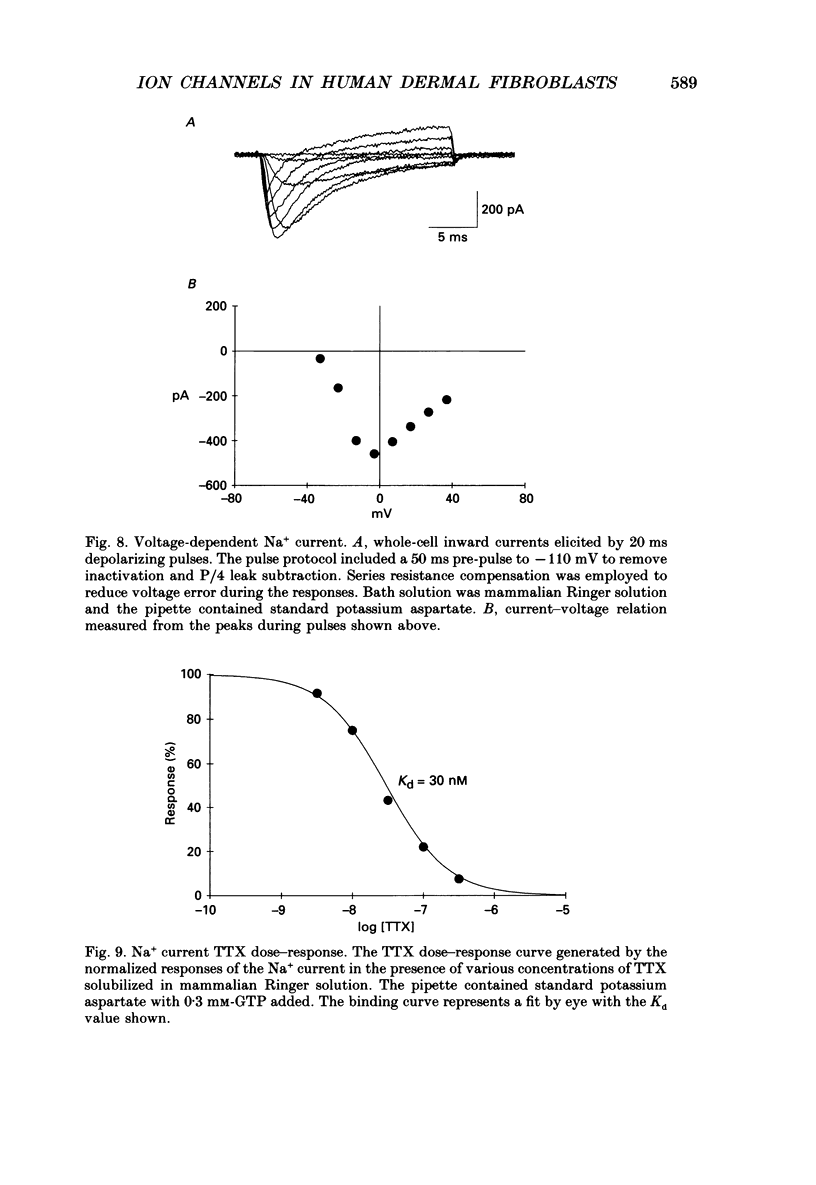
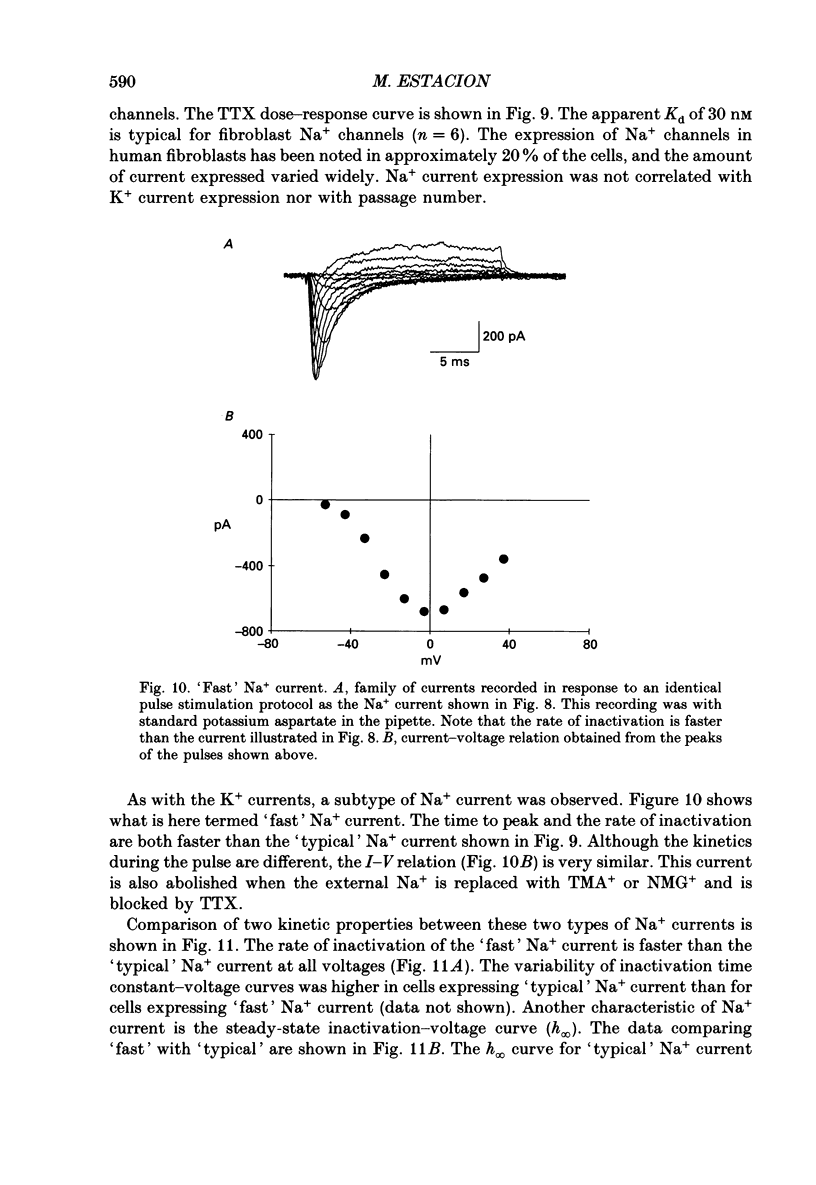
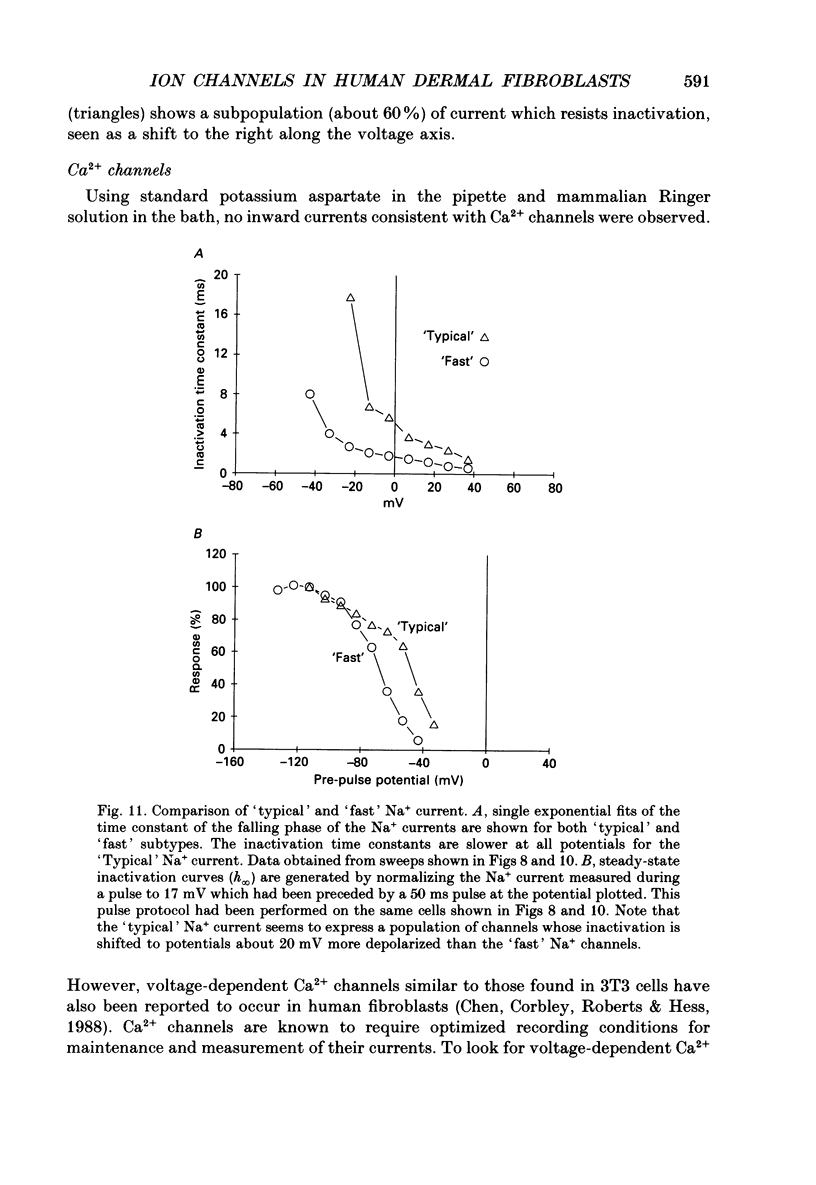
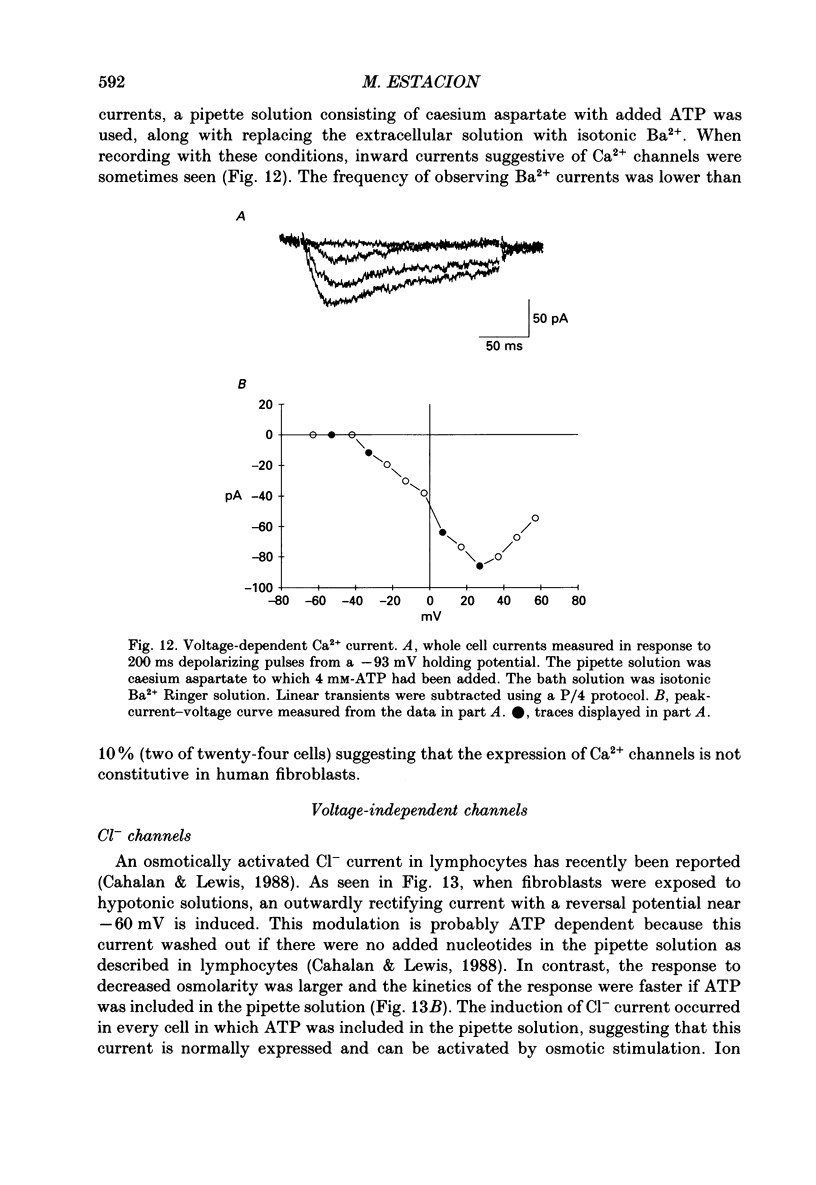
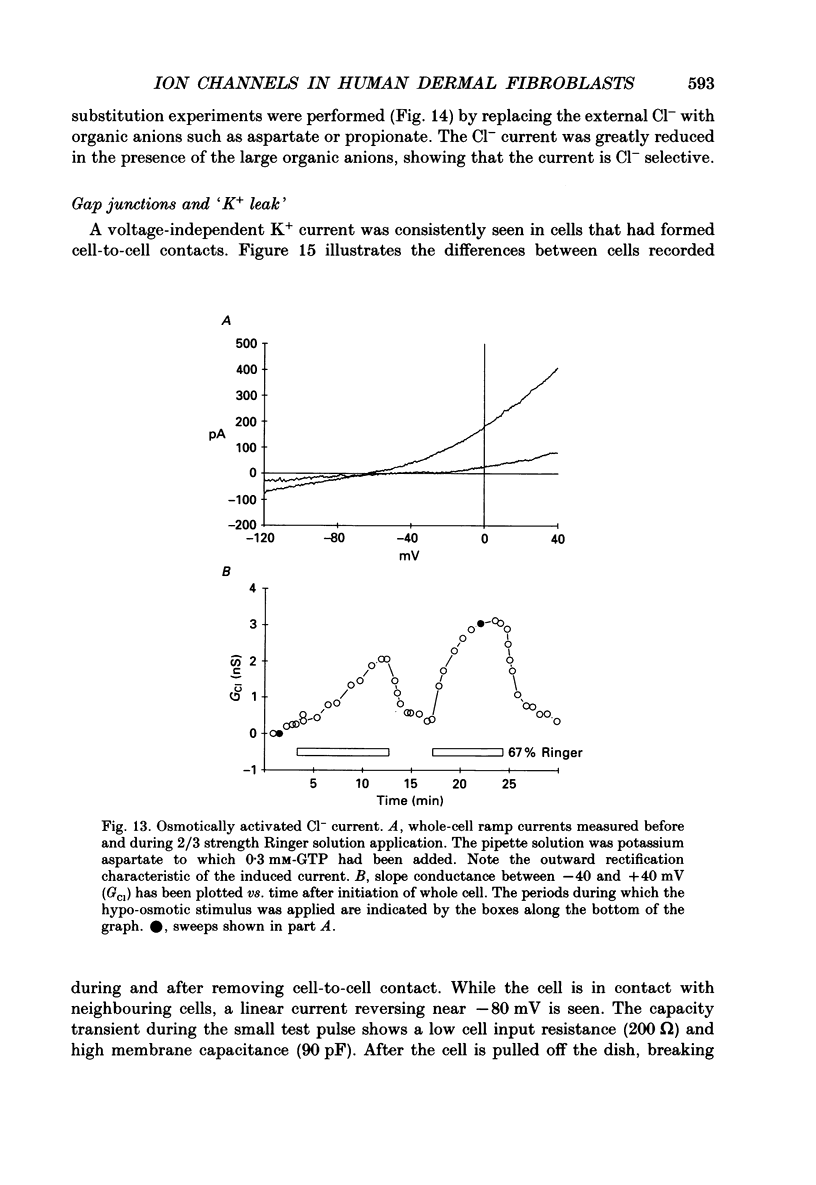
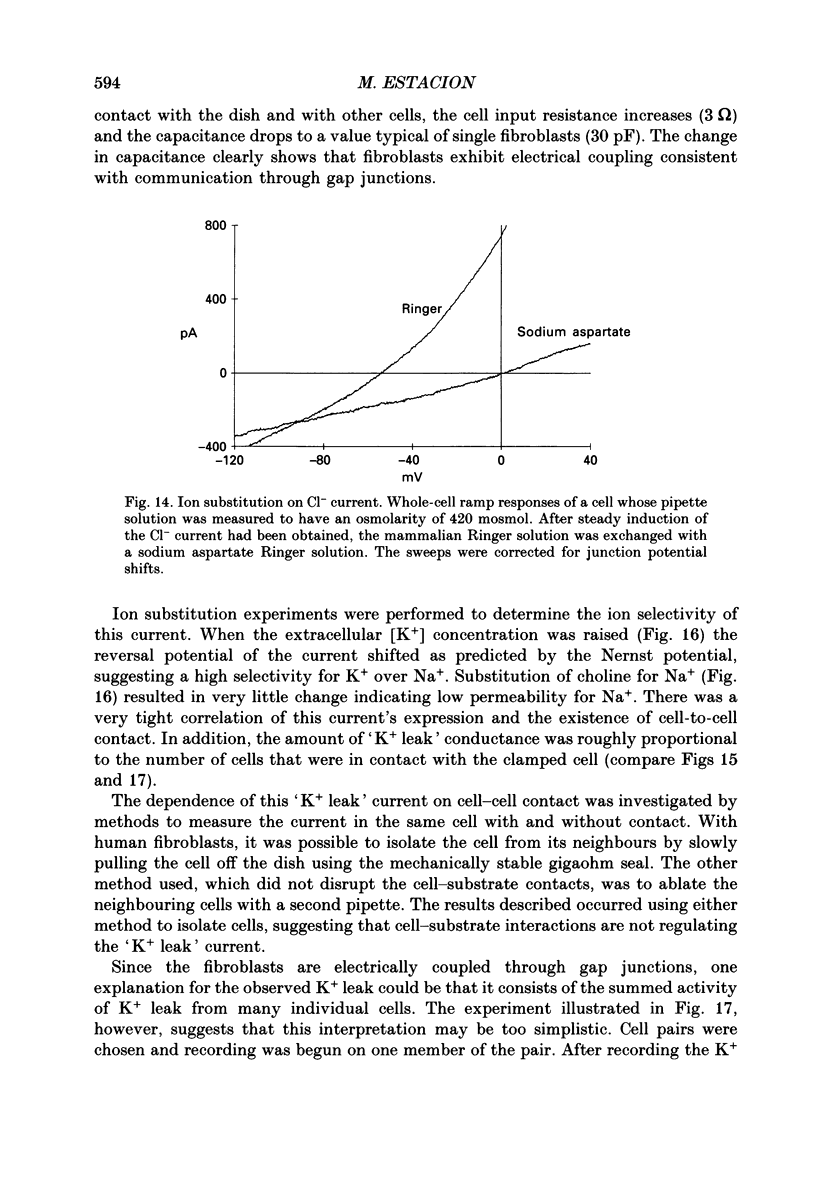
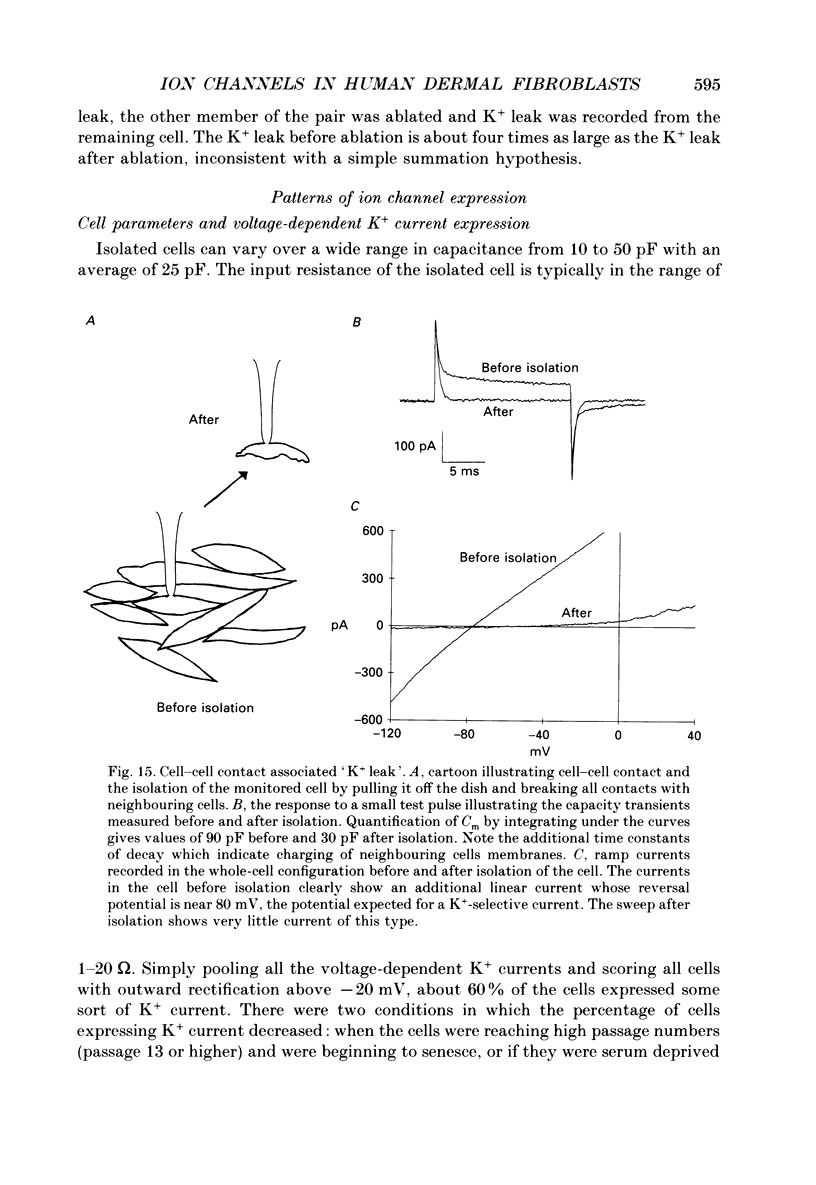
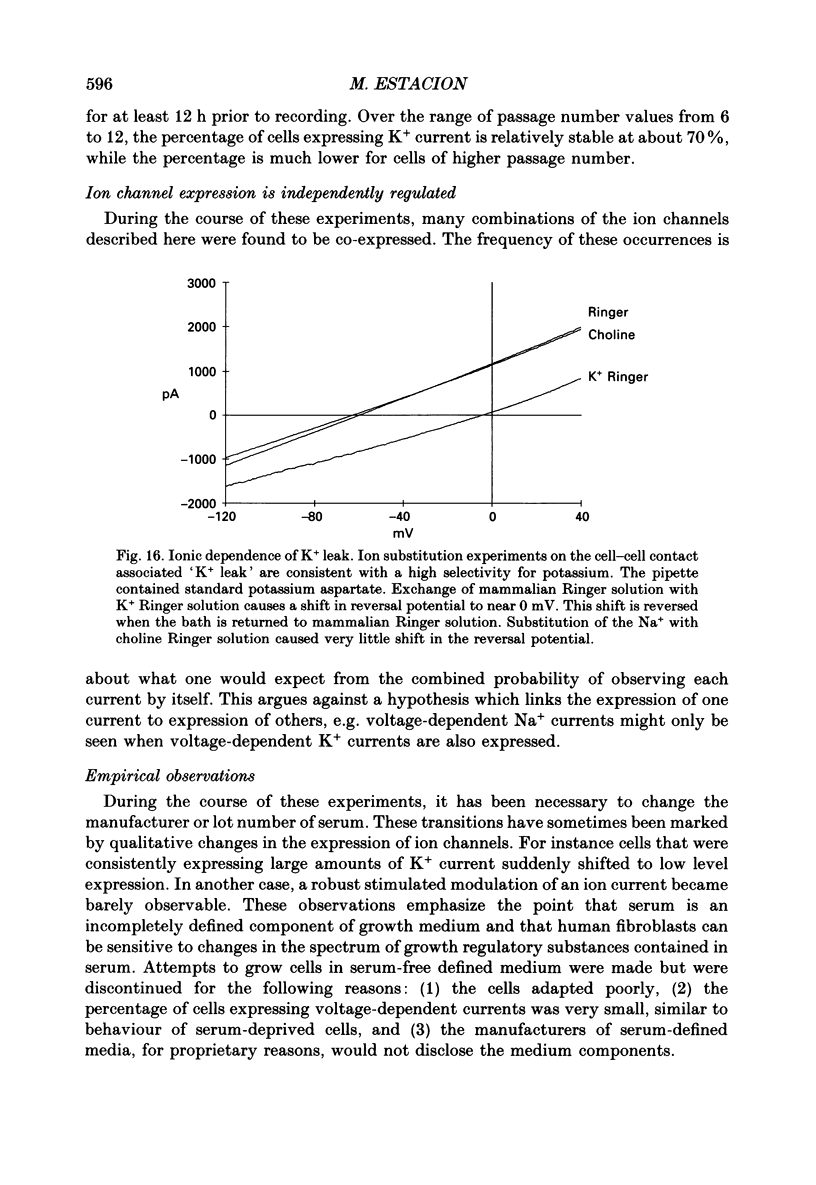
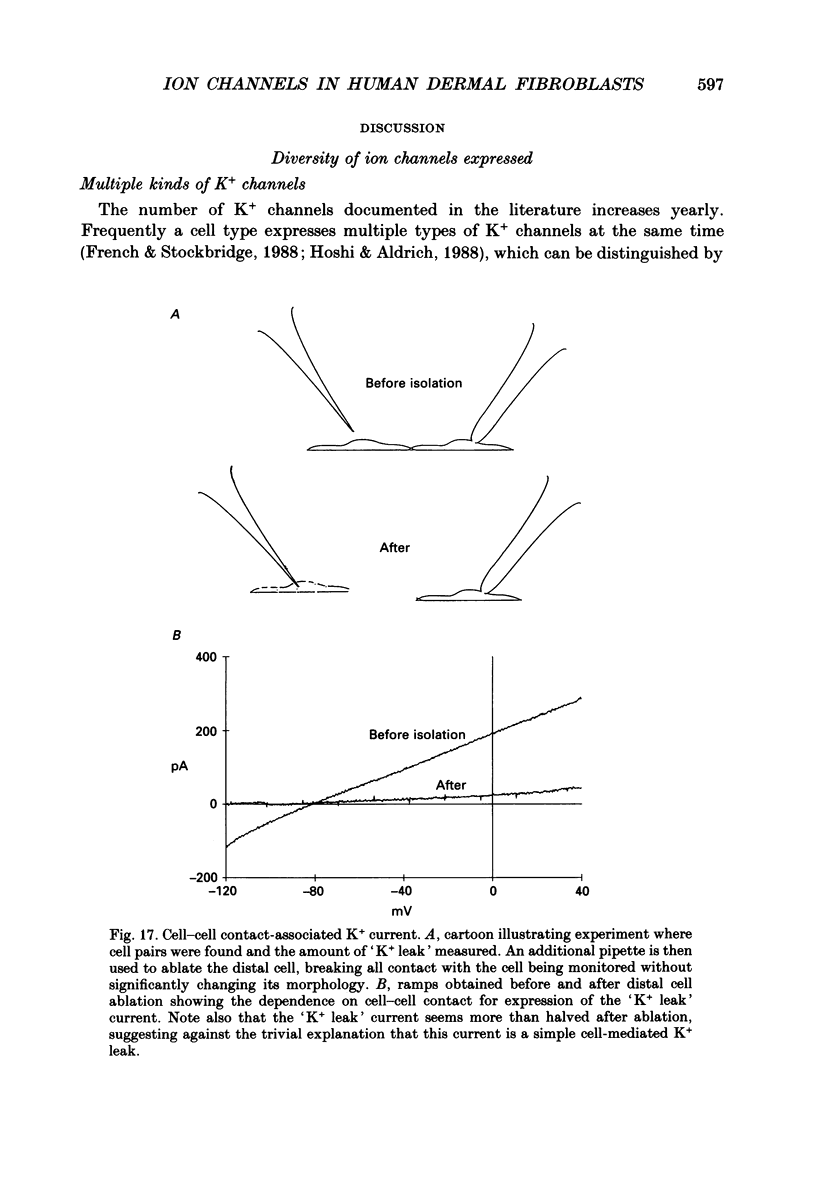
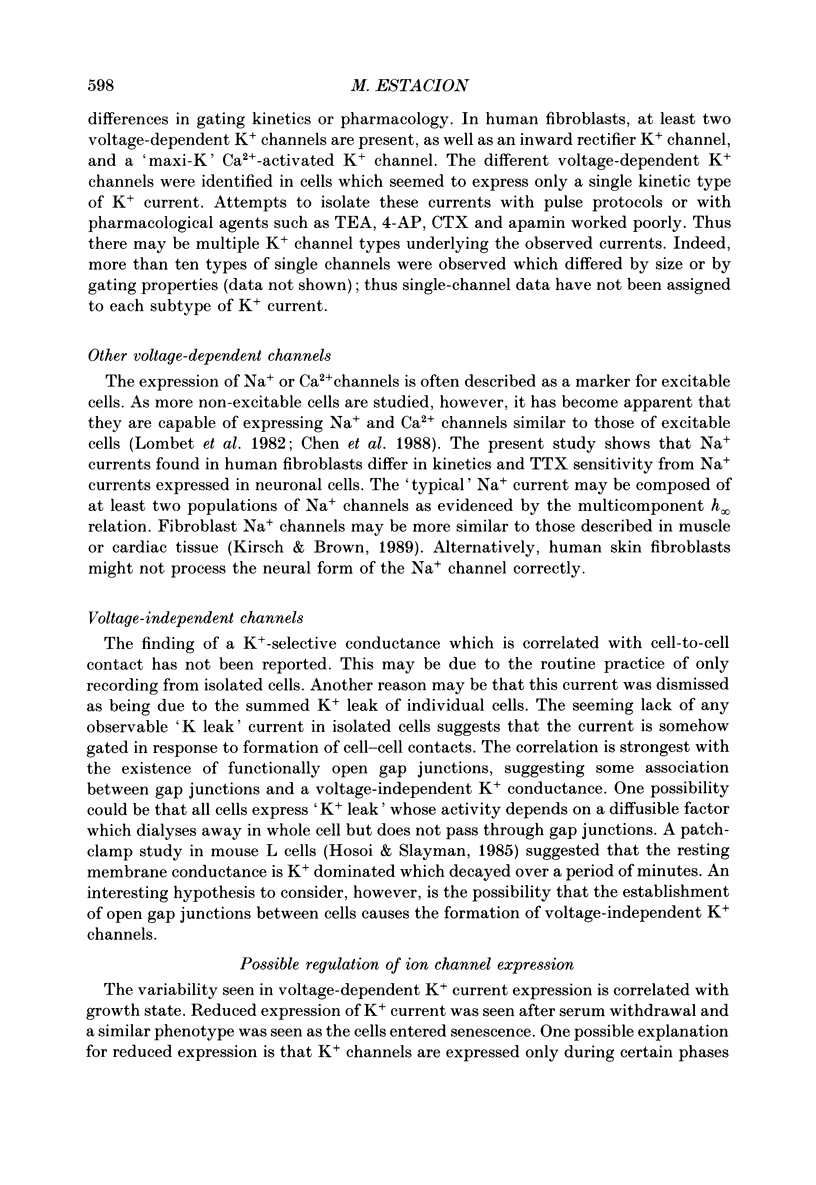
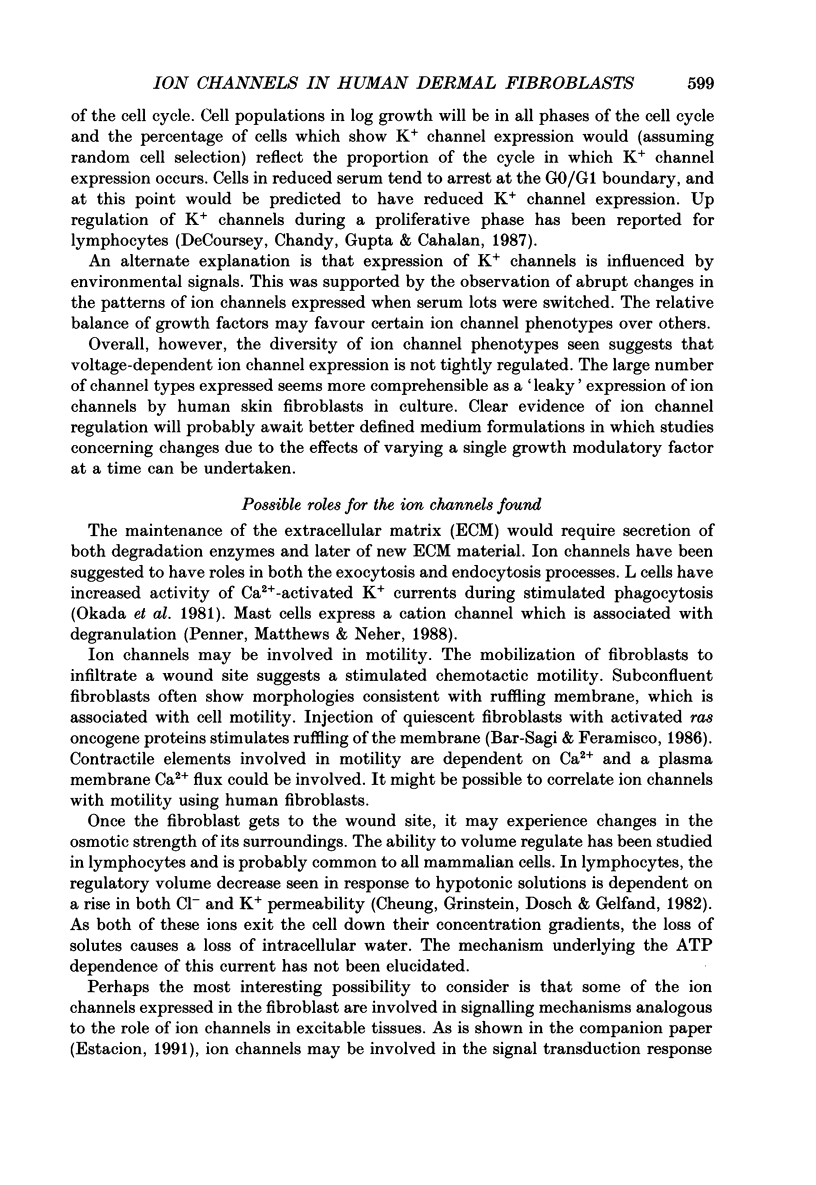
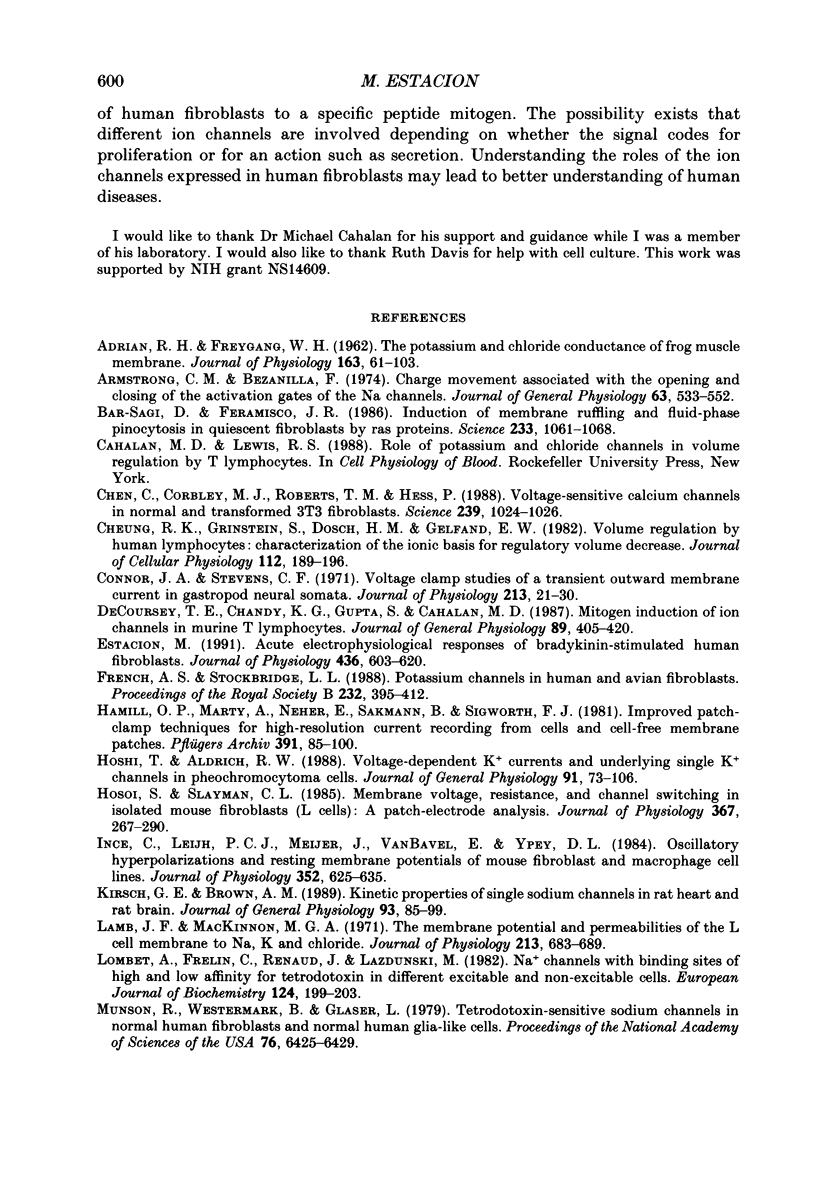
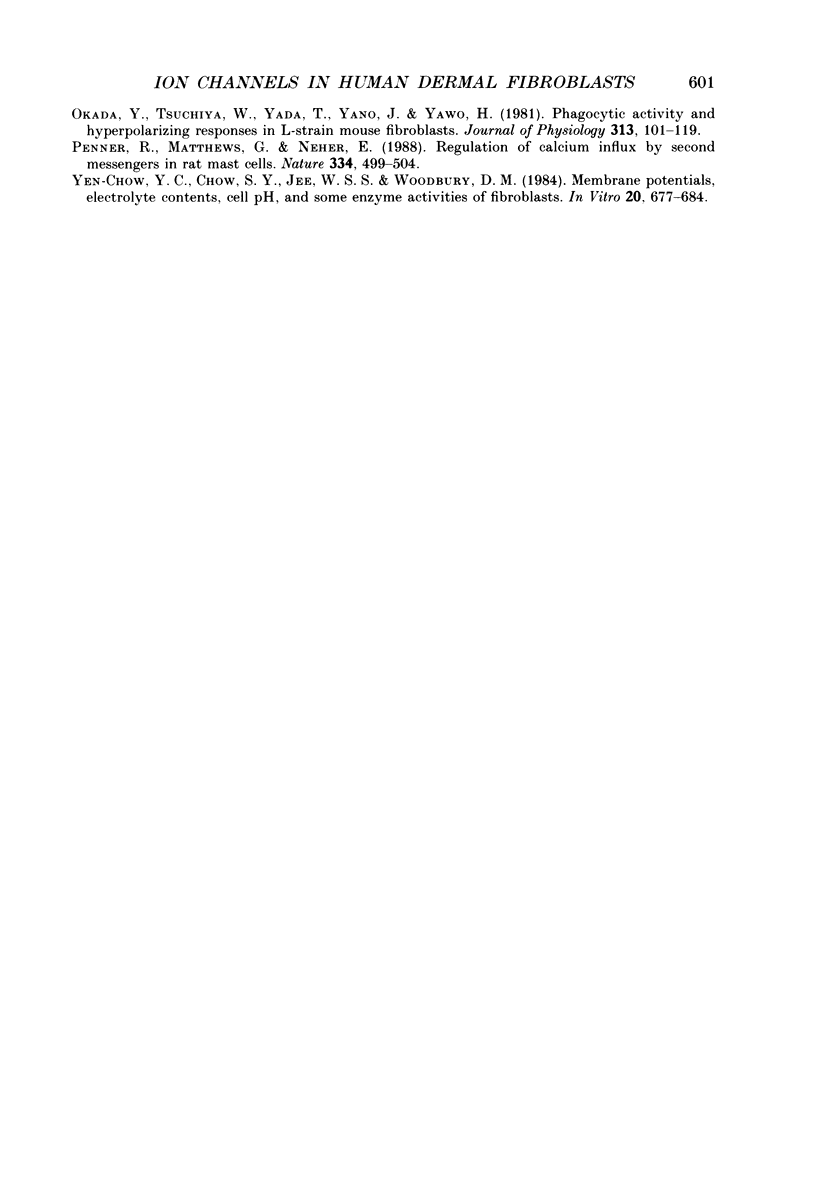
Selected References
These references are in PubMed. This may not be the complete list of references from this article.
- Adrian R. H., Freygang W. H. The potassium and chloride conductance of frog muscle membrane. J Physiol. 1962 Aug;163(1):61–103. doi: 10.1113/jphysiol.1962.sp006959. [DOI] [PMC free article] [PubMed] [Google Scholar]
- Armstrong C. M., Bezanilla F. Charge movement associated with the opening and closing of the activation gates of the Na channels. J Gen Physiol. 1974 May;63(5):533–552. doi: 10.1085/jgp.63.5.533. [DOI] [PMC free article] [PubMed] [Google Scholar]
- Bar-Sagi D., Feramisco J. R. Induction of membrane ruffling and fluid-phase pinocytosis in quiescent fibroblasts by ras proteins. Science. 1986 Sep 5;233(4768):1061–1068. doi: 10.1126/science.3090687. [DOI] [PubMed] [Google Scholar]
- Chen C. F., Corbley M. J., Roberts T. M., Hess P. Voltage-sensitive calcium channels in normal and transformed 3T3 fibroblasts. Science. 1988 Feb 26;239(4843):1024–1026. doi: 10.1126/science.2449730. [DOI] [PubMed] [Google Scholar]
- Cheung R. K., Grinstein S., Dosch H. M., Gelfand E. W. Volume regulation by human lymphocytes: characterization of the ionic basis for regulatory volume decrease. J Cell Physiol. 1982 Aug;112(2):189–196. doi: 10.1002/jcp.1041120206. [DOI] [PubMed] [Google Scholar]
- Connor J. A., Stevens C. F. Voltage clamp studies of a transient outward membrane current in gastropod neural somata. J Physiol. 1971 Feb;213(1):21–30. doi: 10.1113/jphysiol.1971.sp009365. [DOI] [PMC free article] [PubMed] [Google Scholar]
- Decoursey T. E., Chandy K. G., Gupta S., Cahalan M. D. Mitogen induction of ion channels in murine T lymphocytes. J Gen Physiol. 1987 Mar;89(3):405–420. doi: 10.1085/jgp.89.3.405. [DOI] [PMC free article] [PubMed] [Google Scholar]
- Estacion M. Acute electrophysiological responses of bradykinin-stimulated human fibroblasts. J Physiol. 1991 May;436:603–620. doi: 10.1113/jphysiol.1991.sp018569. [DOI] [PMC free article] [PubMed] [Google Scholar]
- French A. S., Stockbridge L. L. Potassium channels in human and avian fibroblasts. Proc R Soc Lond B Biol Sci. 1988 Jan 22;232(1269):395–412. doi: 10.1098/rspb.1988.0003. [DOI] [PubMed] [Google Scholar]
- Hamill O. P., Marty A., Neher E., Sakmann B., Sigworth F. J. Improved patch-clamp techniques for high-resolution current recording from cells and cell-free membrane patches. Pflugers Arch. 1981 Aug;391(2):85–100. doi: 10.1007/BF00656997. [DOI] [PubMed] [Google Scholar]
- Hoshi T., Aldrich R. W. Voltage-dependent K+ currents and underlying single K+ channels in pheochromocytoma cells. J Gen Physiol. 1988 Jan;91(1):73–106. doi: 10.1085/jgp.91.1.73. [DOI] [PMC free article] [PubMed] [Google Scholar]
- Hosoi S., Slayman C. L. Membrane voltage, resistance, and channel switching in isolated mouse fibroblasts (L cells): a patch-electrode analysis. J Physiol. 1985 Oct;367:267–290. doi: 10.1113/jphysiol.1985.sp015824. [DOI] [PMC free article] [PubMed] [Google Scholar]
- Ince C., Leijh P. C., Meijer J., Van Bavel E., Ypey D. L. Oscillatory hyperpolarizations and resting membrane potentials of mouse fibroblast and macrophage cell lines. J Physiol. 1984 Jul;352:625–635. doi: 10.1113/jphysiol.1984.sp015313. [DOI] [PMC free article] [PubMed] [Google Scholar]
- Kirsch G. E., Brown A. M. Kinetic properties of single sodium channels in rat heart and rat brain. J Gen Physiol. 1989 Jan;93(1):85–99. doi: 10.1085/jgp.93.1.85. [DOI] [PMC free article] [PubMed] [Google Scholar]
- Lamb J. F., MacKinnon M. G. The membrane potential and permeabilities of the L cell membrane to Na, K and chloride. J Physiol. 1971 Mar;213(3):683–689. doi: 10.1113/jphysiol.1971.sp009408. [DOI] [PMC free article] [PubMed] [Google Scholar]
- Lombet A., Frelin C., Renaud J. F., Lazdunski M. Na+ channels with binding sites of high and low affinity for tetrodotoxin in different excitable and non-excitable cells. Eur J Biochem. 1982 May;124(1):199–203. doi: 10.1111/j.1432-1033.1982.tb05925.x. [DOI] [PubMed] [Google Scholar]
- Munson R., Jr, Westermark B., Glaser L. Tetrodotoxin-sensitive sodium channels in normal human fibroblasts and normal human glia-like cells. Proc Natl Acad Sci U S A. 1979 Dec;76(12):6425–6429. doi: 10.1073/pnas.76.12.6425. [DOI] [PMC free article] [PubMed] [Google Scholar]
- Okada Y., Tsuchiya W., Yada T., Yano J., Yawo H. Phagocytic activity and hyperpolarizing responses in L-strain mouse fibroblasts. J Physiol. 1981;313:101–119. doi: 10.1113/jphysiol.1981.sp013653. [DOI] [PMC free article] [PubMed] [Google Scholar]
- Penner R., Matthews G., Neher E. Regulation of calcium influx by second messengers in rat mast cells. Nature. 1988 Aug 11;334(6182):499–504. doi: 10.1038/334499a0. [DOI] [PubMed] [Google Scholar]
- Yen-Chow Y. C., Chow S. Y., Jee W. S., Woodbury D. M. Membrane potentials, electrolyte contents, cell pH, and some enzyme activities of fibroblasts. In Vitro. 1984 Sep;20(9):677–684. doi: 10.1007/BF02618872. [DOI] [PubMed] [Google Scholar]